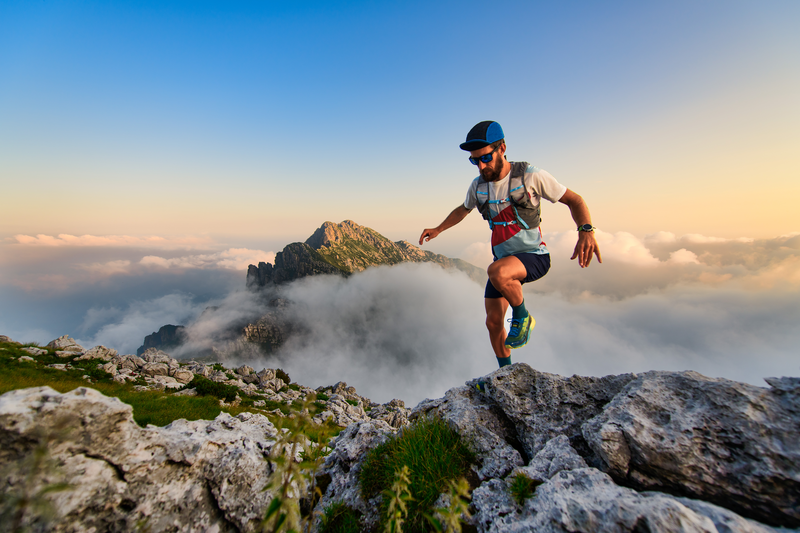
95% of researchers rate our articles as excellent or good
Learn more about the work of our research integrity team to safeguard the quality of each article we publish.
Find out more
REVIEW article
Front. Immunol. , 22 August 2023
Sec. Molecular Innate Immunity
Volume 14 - 2023 | https://doi.org/10.3389/fimmu.2023.1209970
This article is part of the Research Topic O-GlcNAcylation and the Immune System View all 14 articles
O-GlcNAcylation is a post-translational modification of proteins that involves the addition of O-GlcNAc to serine or threonine residues of nuclear or cytoplasmic proteins, catalyzed by O-GlcNAc transferase (OGT). This modification is highly dynamic and can be reversed by O-GlcNAcase (OGA). O-GlcNAcylation is widespread in the immune system, which engages in multiple physiologic and pathophysiologic processes. There is substantial evidence indicating that both the hexosamine biosynthesis pathway (HBP) and O-GlcNAcylation are critically involved in regulating immune cell function. However, the precise role of O-GlcNAcylation in the immune system needs to be adequately elucidated. This review offers a thorough synopsis of the present research on protein O-GlcNAcylation, accentuating the molecular mechanisms that control immune cells’ growth, maturation, and performance via this PTM.
Hart and Torres reported the initial identification of protein O-GlcNAcylation when they studied the changes on cell surface proteins of lymphocytes, and they found that most of these proteins are located intracellularly (1–3). O-GlcNAcylation is among the most commonly occurring post-translational modifications (PTM) and is a dynamic PTM analogous to phosphorylation (4). O-GlcNAcylation affects a protein’s structural composition and stability and is crucial for signal transduction, metabolic pathways, immune regulation, oncogenesis and metastasis (5–9). In this process, O-GlcNAc transferase (OGT) catalyzes the addition of monosaccharides, while O-GlcNAcase (OGA) facilitates their removal (10, 11). O-GlcNAcylation of proteins is mediated by the hexosamine biosynthetic pathway, which accounts for approximately 2-5% of glucose utilization (Figure 1). Despite the intricate network of cells and proteins within the immune system, the current study has confirmed that O-GlcNAcylation has been shown to promote the growth and activation of T and B cells (12, 13). O-GlcNAcylation has also been shown to exert regulatory effects on inflammation and the innate antiviral immune response mediated by macrophages (14, 15). Additionally, it has been found to enhance the functionality of activated neutrophils while suppressing the activity of natural killer cells (2). Figure 2 outlines the biological functions of O-GlcNAcylation in the different immune cells mentioned in this review.
Figure 1 Hexosamine biosynthesis pathway (HBP) is an essential part in the formation of O-GlcNAc. HBP is a branch of glucose metabolism where the vast majority of glucose is used for energy production, glycogen synthesis, and gluconogenesis into fats and proteins, while a small percentage of glucose is used by HBP to produce UDP-GlcNAc. UDP-GlcNAc is catalyzed by OGT to transfer O-GlcNAc to the serine and threonine sites of proteins to complete the O-GlcNAcylation of proteins.
Figure 2 O-GlcNAcylation in different immune cells. (A) In monocytes, O-GlcNAcylation helps translocation of the transcription factor Elf-1 into the nucleus and inhibits Tolip transcription. OGT can mediate the transcription of MIP-1 cytokine induced by glucose. (B) OGT inhibit mTORC1/S6K1 signaling pathway and the proinflammatory differentiation of macrophages. OGT-mediated O-GlcNAcylation of STAT3 in macrophages inhibit the phosphorylation of STAT3 and the expression of IL-10. (C) OGT inhibitor OSMI-1 disrupts the MEK/ERK signaling pathway and mTOR/AKT signaling pathway through the inhibition of O-GlcNAcylation in DC cells, and affects the activation and release of inflammatory factors in DC cells. (D) N-formylmethionine-leucyl-phenylalanine, GlcNH, PUGNAc promote the O-GlcNAcylation of neutrophils and activate the MAPK signaling pathway, ultimately promoting the migration and movement of neutrophils. (E) IL-2 and IL-15 can cause the O-GlcNAcylation of NK cells to increase, reducing the activity of EZH2 to inhibit the maturation of NK cells. (F) In B cells, OGT promote the phosphorylation process of Lsp1 by mediating the O-GlcNAcylation of Lsp1, thus inducing apoptosis. O-GlcNAcylation of Lyn plays a key role in BCR-mediated B-cell activation. Decreased O-GlcNAcylation in B cells down-regulates c-Myc expression, leading to cell cycle arrest. (G) T cell activation is dependent on the O-GlcNAcylation of NFAT and NF-κB. miR-15b inhibit the expression of OGT and induce the decline of O-GlcNAcylation, which ultimately leads to the enhancement of Th17 cell differentiation.
Innate immunity is also known as nonspecific immunity. The primary function of innate immunity is anti-infection, which is the primary defense system against the invasion of pathogens (16, 17). Upon encountering infection or injury, innate immune cells recognize pathogen-associated molecular patterns (PAMPs), which trigger inflammation and initiate a sequence of inflammatory responses (18, 19). Innate immune cells, such as circulating leukocytes including monocytes and neutrophils, as well as macrophages, natural killer cells (NK cells), and dendritic cells (DCs), are vital in this process (17). Innate immune cells exert their regulatory function through O-GlcNAcylation on their surface and its corresponding receptors. The modification of proteins involved in the NF-κB pathway and other inflammation-associated signaling pathways by O-GlcNAcylation is vital for regulating the functionality of innate immune cells (20). In this section, we illustrate O-GlcNAcylation alteration in innate immune cells (monocytes, macrophages, dendritic cells, neutrophils and natural killer cells) and their subsequent reflection and progress.
Human monocytes make up approximately 10% of peripheral leukocytes. In comparison, mice monocytes account for 4% (21). Monocytes originate from the common myeloid progenitor (CMP) differentiated from hematopoietic stem cells (HSCS). They are considered to be the precursor of macrophages and dendritic cells (21, 22). The latest research suggests that there are two distinct differentiation monocyte pathways: (1) CMP → Granulocyte-monocyte progenitor (GMP) → monocytes, (2) CMP → Monocyte-Dendritic cell progenitor (MDP) → monocytes (23). Monocytes can also be considered immature macrophages. Distinct developmental pathways from blood monocytes give rise to diverse subsets of human macrophages (24). One study demonstrated that O-GlcNAc modification is essential for the nuclear translocation of the transcription factor Elf-1, which suppresses Tollip gene transcription in monocytes (25). Zhou W et al. indicated that glucosamine could recruit Ly6Clow monocytes and promote recovery from cardiac ischemia in a STAT1 and O-GlcNAcylation dependent manner (26). Currently, it is known that prolonged exposure of monocytes to high levels of glucose can induce a pro-inflammatory phenotype, but the precise role of O-GlcNAcylation in regulating this process in monocytes remains unclear (27). Kato S et al. showed that OGT is essential for MIP-1 genes expression induced by glucose in monocytes, with O-GlcNAcylation and OGT recruitment observed at the MIP-1α promoter. However, HCF-1 was not detected at the MIP-1α promoter in the absence of its activation (28). At present, more evidence needed to reveal the direct regulation of glucose metabolism and pro-inflammatory phenotype by O-GlcNAcylation.
Macrophages have diverse origins and are widely distributed throughout various tissues (29). One subset of macrophages is derived from the embryonic yolk sac and fetal liver (30), and another subset is derived from monocytic differentiation (31, 32). As a crucial cell type in innate immunity, macrophages participate in the elimination of damaged and aging cells, and the stimulation of adaptive immunity through antigen presentation. In addition to phagocytosis and immune prevention, they are also involved in tissue metabolism and the maintenance of tissue homeostasis (33, 34). O-GlcNAcylation of p65 near the S536 site can enhance the binding of p65 to IκB, promoting macrophage conversion to the M2 phenotype and alleviating the inflammatory response (35). Yang X et al. suggested that overnutrition triggers the O-GlcNAc signaling pathway in macrophages, leading to the suppression of proinflammatory activation and protecting against obesity and metabolic dysfunction caused by dietary factors (10). Moreover, a study showed that OGT could hinder macrophage proinflammatory activation by repressing the mTORC1/S6K1 signaling pathway (36). The modulation of iNOS in macrophages is pivotal for maintaining the immune system and blocking the O-GlcNAc cycling mechanism leading to an elevation in pro-inflammatory cytokines including IL-1β, IL-6, and IL-12 (37). In human carotid atherosclerotic plaques, a significant number of M1 macrophages (iNOS+) could be modified by O-GlcNAc glycosylation. In contrast, M2 macrophages (Arg-1+) only occasionally demonstrate O-GlcNAc positivity. A study indicated that elevated blood sugar levels might induce a shift in macrophage polarization towards an alternative phenotype through increased O-GlcNAcylation, which may promote immune evasion. They showed that increased glucose flux promotes tumor growth and immune escape by upregulating O-GlcNAcylation in tumor-associated macrophages (TAMs), leading to a shift in macrophage polarization towards an M2-like phenotype (38). Wen H et al. demonstrated that O-GlcNAcylation of STAT3 on threonine 717 (T717) exerts a negative regulation on its phosphorylation and modulates gene expression in macrophages (39). Shevde LA et al. suggested that tumorigenic Hedgehog (Hh) signaling is vital for programming the metabolic circuitry that regulates macrophage polarization. The study showed that suppression of the Hh pathway in M2 macrophages decreased UDP-GlcNAc biosynthesis flux pathway. This led to a reduction in O-GlcNAc modification of STAT6, which inhibited the immune-suppressive program of M2 macrophages. Furthermore, the study demonstrated that M2 macrophages, which require high metabolic demands, reprogram their metabolic and bio-energetic pathways from fatty acid oxidation to glycolysis (40). The above studies hint that immune-metabolic regulation of O-GlcNAcylation in macrophages may have the prospect of clinical application.
Dendritic cells (DC) recognize pathogens in innate immunity and activate immune cells in adaptive immunity (41). DC maintain peripheral T cells in a static state in the absence of inflammatory stimuli and identify signals associated with pathogens or inflammation continually (42, 43). If pattern recognition receptors (PRRs) on DC recognize pathogen-associated molecular patterns (PAMPs) or damage-associated molecular patterns (DAMPs), DC become activated or mature (44, 45). DC can express a broad range of PRRs, including surface and intra-nuclear toll-like receptors (TLRs), C-type lectins, and cytosolic sensors. After PRR activation, DC will change gene transcription, cell morphology, and cell metabolism and eventually mature and effectively activate T lymphocytes (44). Gibec D et al. suggested the impact of O-GlcNAcylation in human monocyte-derived dendritic cells (moDCs). They found that the reduction of OGT affected the AKT and MEK/ERK signaling pathways in moDCs and resulted in alterations to the maturation process of immature moDCs. Simultaneously, they observed that HLA-DR, CD14, and CD86 expression increased. In contrast, the expression of CD80 and DC-SIGN was reduced (46). A recent study showed that inhibiting OGA promotes the differentiation of CD34+ hematopoietic stem and progenitor cells (HSPCs) and acute myeloid leukemia (AML) cells into DC via STAT3/5 signaling (47). Further, cullin family E3 ubiquitin ligase (CUL3) reduces expression of OGT, leading to inhibition of STAT3 O-GlcNAcylation. However, the current studies about O-GlcNAcylation in dendritic cells remain insufficient. At present, studies on O-GlcNAcylation in dendritic cells are still lacking, and more studies are needed to clarify the function of O-GlcNAcylation in dendritic cells in the future.
Neutrophils are polymorphonuclear granulocytes (PMNs) derived from bone marrow stem cells (48, 49). Neutrophils phagocytose and kill microorganisms by participating in the inflammatory response and cell apoptosis (50, 51). Some hold the opinion that neutrophils are at the crossroads of innate and adaptive immunity (52). Previous studies indicated that the level of proteins in neutrophils that undergo O-GlcNAcylation increased rapidly within 2 minutes after exposure to N-formylmethionine-leucyl-phenylalanine (53). Marchase RB and Kneass ZT reported that protein O-GlcNAcylation in PMNs could be significantly increased by both glucosamine (GlcN) and agonist, with the two treatments displaying similar and additive effects (54). Some studies showed that substrates or inhibitors increase the overall O-GlcNAcylation level and promote the activation of p38 and ERK in neutrophils. Elevated O-GlcNAcylation levels enhanced neutrophil migration and motility (55). Meanwhile, the administration of PUGNAc (OGA inhibitor) or GlcN leads to the upregulation of Rac activity. GlcN and PUGNAc increase both the basal and fMLP-induced activity of a central mediator of cellular motility, the small GTPase Rac (56). Rac has been shown to be crucial in regulating the mobilization of neutrophils and activating downstream signaling pathways, such as p38 and p44/42 MAPK signaling (55–57). To sum up, O-GlcNAcylation facilitates neutrophil mobilization by increasing Rac activation and is a process of dynamic change in neutrophils. Moreover, this dynamic change may reflect some pathophysiologic status in immune related disease.
As part of the innate immune system’s effector lymphocytes, Natural killer (NK) cells serve to control the growth of tumors and microbial infections by restricting their spread and minimizing accompanying tissue damage (58). NK cells, accounting for around 15% of circulating lymphocytes, are considered the third primary type of lymphocyte, alongside T cells and B cells (59). NK cells were a crucial component of the innate immune system, mainly distributed in the peripheral blood, liver and spleen (60). In vivo, NK cells were mostly identified by the absence of CD4 and the presence of CD56 (61). GST-sHLA-G1α chain could inhibit the alteration of O-GlcNAc levels in NK cells. One study indicated that GST-sHLA-G1a chain could bind to its receptor ILT-2 on NK92 cells and recruited SHP-1, which consequently dephosphorylated some important protein tyrosine kinases (PTK) and blocked the activation of MEK and ERK (62). Reshmi et al suggested that exposing NK cells to the cytokines IL-2 and IL-15 resulted in heightened O-GlcNAcylation of various cellular proteins (63). When the O-GlcNAcylation of NK cells was inhibited by the chemical molecule OSMI-1, the expression of NKG2D, NKG2A, NKp44, TNF-α, IFN-γ, granulysin, soluble Fas ligand, perforin, and granzyme B were decreased. This resulted in a reduction of NK cell cytotoxicity against cancer cells. However, when OGA was inhibited, and O-GlcNAcylation was increased, there was no effect on NK cell cytotoxicity. Experiments conducted in vivo revealed that pretreatment with OSMI-1, an inhibitor of O-GlcNAcylation, resulted in a reduction in the cytotoxic activity of NK cells against tumor cells (63). Upon NK cell stimulation, NFAT has been proved to relocate from the cytosol to the nucleus (64). The impact of O-GlcNAcylation of NFAT on NK cell function remains uncertain. Therefore, further investigation is needed to explore the plausible role of O-GlcNAcylated variants of NF-κB and NFAT transcription factors in regulating the cytotoxic capabilities of NK cells. By inhibiting the activity of enhancer of zest homolog 2 (EZH2)’s histone methyl transferase; progenitor cells can be differentiated into mature NK cells. In addition, O-GlcNAcylation of EZH2 at serine 75 regulates NK cell development and EZH2 protein stability and function (65, 66). O-GlcNAcylation is crucial in preventing c-Myc clearance and decreasing UDP-GlcNAc levels due to glutamine depletion (67). NK cells are lymphocytes with anti-tumor functions and the cytotoxicity depends on c-Myc. Therefore, the cytotoxic activity of NK cells against tumor cells may contribute to the immunotherapy of cancer.
Adaptive immunity, also known as specific immunity or acquired immunity, is the immune response that is developed by an organism after exposure to specific antigens (68). Adaptive immunity has developed a sophisticated system of recognition for both self-antigens and nonself-antigens, allowing for a broader and more precise immune response (69). It is generally formed after the stimulation of antigenic substances such as microorganisms (immunoglobulins, immune lymphocytes), and can react specifically with the antigen. T and B cells are the major components of acquired immunity (70). Guerini D et al demonstrated the crucial involvement of OGT in the activation of T and B lymphocytes (71). Proper proliferation and development of most immune cells rely on sufficient O-GlcNAcylation. Decreased O-GlcNAcylation of NF-κB, NFAT, and c-Myc in T and B cells, as well as NF-κB in macrophages, results in loss of their function (72, 73).
T lymphocytes (T cells), also known as thymus-dependent lymphocytes, are the main components of lymphocytes. T cells orchestrate various facets of adaptive immunity, encompassing reactions against pathogens, allergens, and cancer cells (74). After experiencing hematopoietic cell stress caused by chemotherapy, radiotherapy, infection, or transplantation, the extent of immune reconstitution, particularly of T cells, is closely related to patient outcomes (75). The process of T cell activation is reliant on O-GlcNAcylation and this effect is partially attributable to the O-GlcNAcylation of NF-κB and NFAT, which promotes their translocation from the cytoplasm to the nucleus and drives the proliferative response (71). The differentiation of T helper cells, including Th1, Th2, and Th17 cells, depend crucially on O-GlcNAcylation of NFAT (76). During the initial stages of T-cell activation, there is a notable surge in O-GlcNAcylation levels of nuclear proteins, while O-GlcNAcylation on specific cytosolic proteins swiftly declines (77). Elevated levels of O-GlcNAcylation promoted pro-inflammatory CD4+ differentiation and pro-inflammatory Th1 and Th17 effector cells are recognized to be augmented in situations of altered metabolism, such as type 1 and 2 diabetes and inflammatory bowel disease (73). O-GlcNAcylation is activated in primary human T cells. OGT but not OGA function is linked to OGT localization and downstream of TCR signaling. Furthermore, LCK and ZAP-70 were identified as new O-GlcNAcylated proteins among these proximal kinases (78). Several studies hinted at the function of O-GlcNAc in CD4+ T cell differentiation in autoimmune diseases. In systemic lupus erythematosus, T cells in female patients display elevated levels of OGT expression and OGT demethylation (79). Cantrell DA et al. suggested that the presence of c-Myc is essential for the maintenance of protein O-GlcNAcylation in T cells. Loss of OGT impeded the renewal of T cell progenitors, malignant transformation, and clonal expansion of peripheral T cells (80). Additionally, in T cells of patients diagnosed with multiple sclerosis, reduced expression of miR-15-b upstream of OGT leads to increased Th17 cell differentiation (81). Peng Wu et al. showed that the O-GlcNAc level was elevated in vitro differentiated effector CD8+ T cells and in vivo activated effector CD8+ T cells, as well as in vitro differentiated memory-like CD8+ T cells (82). Many O-GlcNAcylation proteins are newly identified in T cell subsets, so further investigation is necessary to fully understand the exact mechanisms through which O-GlcNAc impacts T-cell biology.
B cells are one type of lymphocyte mainly derived from bone marrow (83). B cells are the main cells of humoral immunity, which mainly produce immune effects by secreting antibodies (84, 85). Hyper-O-GlcNAcylation activates NF-κB signaling through crosstalk with phosphorylation and acetylation. O-GlcNAcylation of p65 at Thr-305 and Ser-319 can increase CREB-binding protein (CBP)/p300-dependent by activating acetylation of p65 at Lys-310, and contribute to NF-κB transcriptional activation (86). Upon B-cell receptor (BCR) cross-linking, O-GlcNAcylation is increased. O-GlcNAcylation of lymphocyte-specific protein-1 (Lsp1) in activated B cells is a crucial determinant that initiates apoptosis. The O-GlcNAcylation of Lsp1 at S209 is necessary for the recruitment of PKC-β1, which subsequently triggers S243 phosphorylation, resulting in ERK activation and the reduction of BCL-xL and BCL-2 (87). Lin KI et al. suggested that lack of Ogt could enhance mature B cell apoptosis, thus disrupting the homeostasis of B cells. Meanwhile, Lyn O-GlcNAcylation at serine 19 is essential for BCR-mediated B cell activation (88). Zhang B et al. indicated that the O-GlcNAcylation in pre-B acute lymphocytic leukemia exacerbates the progression of the disease through PI3K/Akt/c-Myc pathway (89). Park SK et al. showed that enhanced O-GlcNAcylation of c-myc facilitates the proliferation of pre-B cells by upregulating the expression of A-type and E-type cyclins. Through overexpression of c-Myc in insect cells, they provided evidence that O-GlcNAc occurs at c-Myc threonine 58, a frequently mutated hot spot in human lymphomas (90, 91). These results indicated that O-GlcNAcylation-dependent expression of c-Myc might be a potential therapeutic target for pre-B cell-derived leukemia. Overall, O-GlcNAcylation is crucial for maintaining various types of B cells and plasma cells.
Cardiovascular disease is a group of circulatory system diseases mainly characterized by heart and cardiovascular abnormalities, among which the common ones are hypertension, myocardial infarction, myocardial hypertrophy, diabetic heart disease, etc. Increased O-GlcNAcylation of cardiomyocytes has been reported in various cardiovascular diseases. Umapathi P et al. showed that lowering O-GlcNAcylation prevented myocardial hypertrophy in mice after TAC surgery, and increasing O-GlcNAcylation induced progressive cardiomyopathy in mice (92). Kronlage M et al. suggested that histone deacetylase 4 (HDAC4) plays a protective role in diabetic hearts by knocking it down in cardiomyocytes of diabetic mice. They further found that the N-terminal protein fragment of HDAC4 is a key factor in the cardioprotective effect and that the O-GlcNAcylization at serine-632 is an important step in the production of the N-terminal protein of HDAC4 (93). Ischemia-reperfusion (I/R) injury is a complex problem in treating myocardial infarction. Ou W et al. reported that hypoxic acclimation enhanced antioxidant effects by activating G6P dehydrogenase through O-glcnacylation in the heart; thereby protecting cardiomyocyte mitochondria and reducing I/R damage (94).
In recent years, the regulatory role of O-GlcNAcylation in cancer has received extensive attention. The redistribution of glucose utilization is one of the essential links in cancer. Shi Q et al. showed that M2 tumor-associated macrophages have the highest glucose uptake capacity in tumors and promote O-GlcNAcylation in the hexosamine biosynthesis pathway, eventually promoting tumor metastasis and drug resistance (95). Wang X et al. reported that glucose-induced O-GlcNAcylation promotes ferroptosis in mesenchymal pancreatic cancer cells. Further, they demonstrated that ZEB1, mesenchymal property transcription factor, O-GlcNAcylation at Ser555 promotes glucose-driven iron death in mesenchymal pancreatic cancer cells (96). Lee DE et al. indicated that inhibition of O-GlcNAcylation can promote autophagy of HCT116 cells of colon cancer, while metformin can induce endoplasmic reticulum stress of HCT116 cells to enhance autophagy. Their follow-up study found that treatment of HCT116 cells with a combination of metformin and OSMI-1 led to sustained induction of autophagy, which in turn enhanced apoptosis through a synergistic effect (97).
Protein O-GlcNAcylation regulates several immune signaling pathways, such as NF-κB, mTOR/S6K1, ERK, FOXO1, CPT2, PGC-1α, JNK, and p38, MAPK. Protein O-GlcNAcylation is closely related to the activation of immune signaling and inflammatory responses. An expanding body of research has revealed O-GlcNAcylation as a vital homeostatic regulator at the intersection of inflammation and metabolism which extensively engaged in cardiovascular diseases and cancer. More and more evidence proved a direct correlation between energy metabolism and O-GlcNAcylation and O-GlcNAcylation plays a dual role of protection and damage in diseases. These suggest that targeting O-GlcNAcylation may have therapeutic potential for treating cancer, cardiovascular diseases, and other immune-related disorders. The implications of O-GlcNAc signaling in immune regulation, particularly in the context of metabolic perturbations, may be far-reaching.
HC and WX wrote the initial draft of the manuscript. HZ prepared the figure and revised the manuscript. QW conducted the literature search. ZL and SL revised and contributed to the final draft. All authors have contribution to the article and approved it for publication.
This work was supported by the National Natural Science Foundation of China (No.82070425, ZL), the Natural Science Foundation of Hubei Province of China (No.2021CFA011, ZL) and the Program of Excellent Doctoral (Postdoctoral) of Zhongnan Hospital, Wuhan University (No. ZNYB2020027, HC).
The authors declare that the research was conducted in the absence of any commercial or financial relationships that could be construed as a potential conflict of interest.
All claims expressed in this article are solely those of the authors and do not necessarily represent those of their affiliated organizations, or those of the publisher, the editors and the reviewers. Any product that may be evaluated in this article, or claim that may be made by its manufacturer, is not guaranteed or endorsed by the publisher.
1. Torres CR, Hart GW. Topography and polypeptide distribution of terminal N-acetylglucosamine residues on the surfaces of intact lymphocytes. Evidence for O-linked GlcNAc. J Biol Chem (1984) 259:3308–17. doi: 10.1016/S0021-9258(17)43295-9
2. Nie H, Yi W. O-GlcNAcylation, a sweet link to the pathology of diseases. J Zhejiang University B Sci (2019) 20:437–48. doi: 10.1631/jzus.B1900150
3. Ma J, Wu C, Hart GW. Analytical and biochemical perspectives of protein O-glcNAcylation. Chem Rev (2021) 121:1513–81. doi: 10.1021/acs.chemrev.0c00884
4. Kristic J, Lauc G. Ubiquitous importance of protein glycosylation. Methods Mol Biol (2017) 1503:1–12. doi: 10.1007/978-1-4939-6493-2_1
5. Rodrigues JG, Balmana M, Macedo JA, Pocas J, Fernandes A, De-Freitas-Junior J, et al. Glycosylation in cancer: Selected roles in tumour progression, immune modulation and metastasis. Cell Immunol (2018) 333:46–57. doi: 10.1016/j.cellimm.2018.03.007
6. Zhou JY, Oswald DM, Oliva KD, Kreisman L, Cobb BA. The glycoscience of immunity. Trends Immunol (2018) 39:523–35. doi: 10.1016/j.it.2018.04.004
7. Yi W, Clark PM, Mason DE, Keenan MC, Hill C, Goddard WR, et al. Phosphofructokinase 1 glycosylation regulates cell growth and metabolism. SCIENCE (2012) 337:975–80. doi: 10.1126/science.1222278
8. Nie H, Ju H, Fan J, Shi X, Cheng Y, Cang X, et al. O-GlcNAcylation of PGK1 coordinates glycolysis and TCA cycle to promote tumor growth. Nat Commun (2020) 11:36. doi: 10.1038/s41467-019-13601-8
9. Ng YH, Okolo CA, Erickson JR, Baldi JC, Jones PP. Protein O-glcNAcylation in the heart. Acta Physiol (2021) 233(1):e13696. doi: 10.1111/apha.13696
10. Yang X, Qian K. Protein O-GlcNAcylation: emerging mechanisms and functions. Nat Rev Mol Cell Biol (2017) 18:452–65. doi: 10.1038/nrm.2017.22
11. Chatham JC, Zhang J, Wende AR. Role of O-linked N-acetylglucosamine protein modification in cellular (Patho)Physiology. Physiol Rev (2021) 101:427–93. doi: 10.1152/physrev.00043.2019
12. Chang Y, Weng C, Lin K. O-GlcNAcylation and its role in the immune system. J BioMed Sci (2020) 27(1):57. doi: 10.1186/s12929-020-00648-9
13. Abramowitz LK, Hanover JA. T cell development and the physiological role of O-GlcNAc. FEBS Lett (2018) 592:3943–9. doi: 10.1002/1873-3468.13159
14. Li T, Li X, Attri KS, Liu C, Li L, Herring LE, et al. O-glcNAc transferase links glucose metabolism to MAVS-mediated antiviral innate immunity. Cell Host Microbe (2018) 24:791–803. doi: 10.1016/j.chom.2018.11.001
15. Li X, Gong W, Wang H, Li T, Attri KS, Lewis RE, et al. O-glcNAc transferase suppresses inflammation and necroptosis by targeting receptor-interacting serine/threonine-protein kinase 3. IMMUNITY (2019) 50:576–90. doi: 10.1016/j.immuni.2019.01.007
16. Duan T, Du Y, Xing C, Wang HY, Wang RF. Toll-like receptor signaling and its role in cell-mediated immunity. Front Immunol (2022) 13:812774. doi: 10.3389/fimmu.2022.812774
17. Akira S, Uematsu S, Takeuchi O. Pathogen recognition and innate immunity. CELL (2006) 124:783–801. doi: 10.1016/j.cell.2006.02.015
18. Zindel J, Kubes P. DAMPs, PAMPs, and LAMPs in immunity and sterile inflammation. Annu Rev Pathol (2020) 15:493–518. doi: 10.1146/annurev-pathmechdis-012419-032847
19. Gong T, Liu L, Jiang W, Zhou R. DAMP-sensing receptors in sterile inflammation and inflammatory diseases. Nat Rev Immunol (2020) 20:95–112. doi: 10.1038/s41577-019-0215-7
20. Dong H, Liu Z, Wen H. Protein O-glcNAcylation regulates innate immune cell function. Front Immunol (2022) 13:805018. doi: 10.3389/fimmu.2022.805018
21. Guilliams M, Mildner A, Yona S. Developmental and functional heterogeneity of monocytes. IMMUNITY (2018) 49:595–613. doi: 10.1016/j.immuni.2018.10.005
22. Hettinger J, Richards DM, Hansson J, Barra MM, Joschko AC, Krijgsveld J, et al. Origin of monocytes and macrophages in a committed progenitor. Nat Immunol (2013) 14:821–30. doi: 10.1038/ni.2638
23. Ginhoux F, Jung S. Monocytes and macrophages: developmental pathways and tissue homeostasis. Nat Rev Immunol (2014) 14:392–404. doi: 10.1038/nri3671
24. Evren E, Ringqvist E, Tripathi KP, Sleiers N, Rives IC, Alisjahbana A, et al. Distinct developmental pathways from blood monocytes generate human lung macrophage diversity. IMMUNITY (2021) 54:259–75. doi: 10.1016/j.immuni.2020.12.003
25. Sugi Y, Takahashi K, Nakano K, Hosono A, Kaminogawa S. Transcription of the Tollip gene is elevated in intestinal epithelial cells through impaired O-GlcNAcylation-dependent nuclear translocation of the negative regulator Elf-1. Biochem Biophys Res Commun (2011) 412:704–9. doi: 10.1016/j.bbrc.2011.08.035
26. Zhou W, Jiang X, Tang Q, Ding L, Xiao W, Li J, et al. Glucosamine facilitates cardiac ischemic recovery via recruiting Ly6C(low) monocytes in a STAT1 and O-GlcNAcylation-dependent fashion. Clin Transl Med (2022) 12:e762. doi: 10.1002/ctm2.762
27. Baudoin L, Issad T. O-glcNAcylation and inflammation: A vast territory to explore. Front Endocrinol (Lausanne) (2014) 5:235. doi: 10.3389/fendo.2014.00235
28. Chikanishi T, Fujiki R, Hashiba W, Sekine H, Yokoyama A, Kato S. Glucose-induced expression of MIP-1 genes requires O-GlcNAc transferase in monocytes. Biochem Biophys Res Commun (2010) 394:865–70. doi: 10.1016/j.bbrc.2010.02.167
29. Epelman S, Lavine KJ, Randolph GJ. Origin and functions of tissue macrophages. IMMUNITY (2014) 41:21–35. doi: 10.1016/j.immuni.2014.06.013
30. Gomez PE, Klapproth K, Schulz C, Busch K, Azzoni E, Crozet L, et al. Tissue-resident macrophages originate from yolk-sac-derived erythro-myeloid progenitors. NATURE (2015) 518:547–51. doi: 10.1038/nature13989
31. Jakubzick C, Gautier EL, Gibbings SL, Sojka DK, Schlitzer A, Johnson TE, et al. Minimal differentiation of classical monocytes as they survey steady-state tissues and transport antigen to lymph nodes. IMMUNITY (2013) 39:599–610. doi: 10.1016/j.immuni.2013.08.007
32. Hashimoto D, Chow A, Noizat C, Teo P, Beasley MB, Leboeuf M, et al. Tissue-resident macrophages self-maintain locally throughout adult life with minimal contribution from circulating monocytes. IMMUNITY (2013) 38:792–804. doi: 10.1016/j.immuni.2013.04.004
33. Mosser DM, Hamidzadeh K, Goncalves R. Macrophages and the maintenance of homeostasis. Cell Mol Immunol (2021) 18:579–87. doi: 10.1038/s41423-020-00541-3
34. Wculek SK, Dunphy G, Heras-Murillo I, Mastrangelo A, Sancho D. Metabolism of tissue macrophages in homeostasis and pathology. Cell Mol Immunol (2022) 19:384–408. doi: 10.1038/s41423-021-00791-9
35. He Y, Ma X, Li D, Hao J. Thiamet G mediates neuroprotection in experimental stroke by modulating microglia/macrophage polarization and inhibiting NF-kappaB p65 signaling. J Cereb Blood Flow Metab (2017) 37:2938–51. doi: 10.1177/0271678X16679671
36. Yang Y, Li X, Luan HH, Zhang B, Zhang K, Nam JH, et al. OGT suppresses S6K1-mediated macrophage inflammation and metabolic disturbance. Proc Natl Acad Sci U.S.A. (2020) 117:16616–25. doi: 10.1073/pnas.1916121117
37. Abramowitz LK, Hanover JA. Chronically elevated O-glcNAcylation limits nitric oxide production and deregulates specific pro-inflammatory cytokines. Front Immunol (2022) 13:802336. doi: 10.3389/fimmu.2022.802336
38. Rodrigues Mantuano N, Stanczak MA, Oliveira IDA, Kirchhammer N, Filardy AA, Monaco G, et al. Hyperglycemia enhances cancer immune evasion by inducing alternative macrophage polarization through increased O-glcNAcylation. Cancer Immunol Res (2020) 8:1262–72. doi: 10.1158/2326-6066.CIR-19-0904
39. Li X, Zhang Z, Li L, Gong W, Lazenby AJ, Swanson BJ, et al. Myeloid-derived cullin 3 promotes STAT3 phosphorylation by inhibiting OGT expression and protects against intestinal inflammation. J Exp Med (2017) 214:1093–109. doi: 10.1084/jem.20161105
40. Hinshaw DC, Hanna A, Lama-Sherpa T, Metge B, Kammerud SC, Benavides GA, et al. Hedgehog signaling regulates metabolism and polarization of mammary tumor-associated macrophages. Cancer Res (2021) 81:5425–37. doi: 10.1158/0008-5472.CAN-20-1723
41. Randolph GJ, Angeli V, Swartz MA. Dendritic-cell trafficking to lymph nodes through lymphatic vessels. Nat Rev Immunol (2005) 5:617–28. doi: 10.1038/nri1670
42. Alvarez D, Vollmann EH, von Andrian UH. Mechanisms and consequences of dendritic cell migration. IMMUNITY (2008) 29:325–42. doi: 10.1016/j.immuni.2008.08.006
43. Liu J, Zhang X, Cheng Y, Cao X. Dendritic cell migration in inflammation and immunity. Cell Mol Immunol (2021) 18:2461–71. doi: 10.1038/s41423-021-00726-4
44. Kapsenberg ML. Dendritic-cell control of pathogen-driven T-cell polarization. Nat Rev Immunol (2003) 3:984–93. doi: 10.1038/nri1246
45. Takeuchi O, Akira S. Pattern recognition receptors and inflammation. CELL (2010) 140:805–20. doi: 10.1016/j.cell.2010.01.022
46. Weiss M, Anderluh M, Gobec M. Inhibition of O-glcNAc transferase alters the differentiation and maturation process of human monocyte derived dendritic cells. CELLS-BASEL (2021) 10(12):3312. doi: 10.3390/cells10123312
47. Luanpitpong S, Rodboon N, Samart P, Janan M, Klaihmon P, Lorthongpanich C, et al. Inhibition of O-glcNAcase inhibits hematopoietic and leukemic stem cell self-renewal and drives dendritic cell differentiation via STAT3/5 signaling. Stem Cells (2022) 40:1078–93. doi: 10.1093/stmcls/sxac068
48. Dinh HQ, Eggert T, Meyer MA, Zhu YP, Olingy CE, Llewellyn R, et al. Coexpression of CD71 and CD117 identifies an early unipotent neutrophil progenitor population in human bone marrow. IMMUNITY (2020) 53:319–34. doi: 10.1016/j.immuni.2020.07.017
49. Schim VDLI, Sprenkeler E, Tool A, Abinun M, Grainger A, Engelhardt KR, et al. Defective neutrophil development and specific granule deficiency caused by a homozygous splice-site mutation in SMARCD2. J Allergy Clin Immunol (2021) 147:2381–5. doi: 10.1016/j.jaci.2020.11.025
50. Burn GL, Foti A, Marsman G, Patel DF, Zychlinsky A. The neutrophil. IMMUNITY (2021) 54:1377–91. doi: 10.1016/j.immuni.2021.06.006
51. Soehnlein O, Steffens S, Hidalgo A, Weber C. Neutrophils as protagonists and targets in chronic inflammation. Nat Rev Immunol (2017) 17:248–61. doi: 10.1038/nri.2017.10
52. Rosales C. Neutrophils at the crossroads of innate and adaptive immunity. J Leukoc Biol (2020) 108:377–96. doi: 10.1002/JLB.4MIR0220-574RR
53. Madsen-Bouterse SA, Xu Y, Petty HR, Romero R. Quantification of O-GlcNAc protein modification in neutrophils by flow cytometry. Cytometry A (2008) 73:667–72. doi: 10.1002/cyto.a.20569
54. Kneass ZT, Marchase RB. Neutrophils exhibit rapid agonist-induced increases in protein-associated O-GlcNAc. J Biol Chem (2004) 279:45759–65. doi: 10.1074/jbc.M407911200
55. Kneass ZT, Marchase RB. Protein O-GlcNAc modulates motility-associated signaling intermediates in neutrophils. J Biol Chem (2005) 280:14579–85. doi: 10.1074/jbc.M414066200
56. Haltiwanger RS, Grove K, Philipsberg GA. Modulation of O-linked N-acetylglucosamine levels on nuclear and cytoplasmic proteins in vivo using the peptide O-GlcNAc-beta-N-acetylglucosaminidase inhibitor O-(2-acetamido-2-deoxy-D-glucopyranosylidene)amino-N-phenylcarbamate. J Biol Chem (1998) 273:3611–7. doi: 10.1074/jbc.273.6.3611
57. Cicchetti G, Allen PG, Glogauer M. Chemotactic signaling pathways in neutrophils: from receptor to actin assembly. Crit Rev Oral Biol Med (2002) 13:220–8. doi: 10.1177/154411130201300302
58. Vivier E, Tomasello E, Baratin M, Walzer T, Ugolini S. Functions of natural killer cells. Nat Immunol (2008) 9:503–10. doi: 10.1038/ni1582
59. Cooper MA, FehNiger TA, Caligiuri MA. The biology of human natural killer-cell subsets. Trends Immunol (2001) 22:633–40. doi: 10.1016/S1471-4906(01)02060-9
60. Dogra P, Rancan C, Ma W, Toth M, Senda T, Carpenter DJ, et al. Tissue determinants of human NK cell development, function, and residence. CELL (2020) 180:749–63. doi: 10.1016/j.cell.2020.01.022
61. Yuen MF, Norris S. Expression of inhibitory receptors in natural killer (CD3(-)CD56(+)) cells and CD3(+)CD56(+) cells in the peripheral blood lymphocytes and tumor infiltrating lymphocytes in patients with primary hepatocellular carcinoma. Clin Immunol (2001) 101:264–9. doi: 10.1006/clim.2001.5110
62. Yao AY, Tang HY, Wang Y, Feng MF, Zhou RL. Inhibition of the activating signals in NK92 cells by recombinant GST-sHLA-G1a chain. Cell Res (2004) 14:155–60. doi: 10.1038/sj.cr.7290215
63. Feinberg D, Ramakrishnan P, Wong DP, Asthana A, Parameswaran R. Inhibition of O-glcNAcylation decreases the cytotoxic function of natural killer cells. Front Immunol (2022) 13:841299. doi: 10.3389/fimmu.2022.841299
64. Aramburu J, Azzoni L, Rao A, PeRussia B. Activation and expression of the nuclear factors of activated T cells, NFATp and NFATc, in human natural killer cells: regulation upon CD16 ligand binding. J Exp Med (1995) 182:801–10. doi: 10.1084/jem.182.3.801
65. Yin J, Leavenworth JW, Li Y, Luo Q, Xie H, Liu X, et al. Ezh2 regulates differentiation and function of natural killer cells through histone methyltransferase activity. Proc Natl Acad Sci U.S.A. (2015) 112:15988–93. doi: 10.1073/pnas.1521740112
66. Chu CS, Lo PW, Yeh YH, Hsu PH, Peng SH, Teng YC, et al. O-GlcNAcylation regulates EZH2 protein stability and function. Proc Natl Acad Sci U.S.A. (2014) 111:1355–60. doi: 10.1073/pnas.1323226111
67. Loftus RM, Assmann N, Kedia-Mehta N, O'Brien KL, Garcia A, Gillespie C, et al. Amino acid-dependent cMyc expression is essential for NK cell metabolic and functional responses in mice. Nat Commun (2018) 9:2341. doi: 10.1038/s41467-018-04719-2
68. McComb S, Thiriot A, Akache B, Krishnan L, Stark F. Introduction to the immune system. Methods Mol Biol (2019) 2024:1–24. doi: 10.1007/978-1-4939-9597-4_1
69. Bonilla FA, Oettgen HC. Adaptive immunity. J Allergy Clin Immunol (2010) 125:S33–40. doi: 10.1016/j.jaci.2009.09.017
70. O'Donnell N, Zachara NE, Hart GW, Marth JD. Ogt-dependent X-chromosome-linked protein glycosylation is a requisite modification in somatic cell function and embryo viability. Mol Cell Biol (2004) 24:1680–90. doi: 10.1128/MCB.24.4.1680-1690.2004
71. Golks A, Tran TT, Goetschy JF, Guerini D. Requirement for O-linked N-acetylglucosaminyltransferase in lymphocytes activation. EMBO J (2007) 26:4368–79. doi: 10.1038/sj.emboj.7601845
72. de Jesus T, Shukla S, Ramakrishnan P. Too sweet to resist: Control of immune cell function by O-GlcNAcylation. Cell Immunol (2018) 333:85–92. doi: 10.1016/j.cellimm.2018.05.010
73. Machacek M, Slawson C, Fields PE. O-GlcNAc: a novel regulator of immunometabolism. J Bioenerg Biomembr (2018) 50:223–9. doi: 10.1007/s10863-018-9744-1
74. Kumar BV, Connors TJ, Farber DL. Human T cell development, localization, and function throughout life. Immunity (2018) 48:202–13. doi: 10.1016/j.immuni.2018.01.007
75. Velardi E, Tsai JJ, van den Brink M. T cell regeneration after immunological injury. Nat Rev Immunol (2021) 21:277–91. doi: 10.1038/s41577-020-00457-z
76. Machacek M, Saunders H, Zhang Z, Tan EP, Li J, Li T, et al. Elevated O-GlcNAcylation enhances pro-inflammatory Th17 function by altering the intracellular lipid microenvironment. J Biol Chem (2019) 294:8973–90. doi: 10.1074/jbc.RA119.008373
77. Kearse KP, Hart GW. Lymphocyte activation induces rapid changes in nuclear and cytoplasmic glycoproteins. Proc Natl Acad Sci USA (1991) 88:1701–5. doi: 10.1073/pnas.88.5.1701
78. Lund PJ, Elias JE, Davis MM. Global analysis of O-glcNAc glycoproteins in activated human T cells. J Immunol (2016) 197:3086–98. doi: 10.4049/jimmunol.1502031
79. Hewagama A, Gorelik G, Patel D, Liyanarachchi P, McCune WJ, Somers E, et al. Overexpression of X-linked genes in T cells from women with lupus. J Autoimmun (2013) 41:60–71. doi: 10.1016/j.jaut.2012.12.006
80. Swamy M, Pathak S, Grzes KM, Damerow S, Sinclair LV, van Aalten DM, et al. Glucose and glutamine fuel protein O-GlcNAcylation to control T cell self-renewal and Malignancy. Nat Immunol (2016) 17:712–20. doi: 10.1038/ni.3439
81. Liu R, Ma X, Chen L, Yang Y, Zeng Y, Gao J, et al. MicroRNA-15b suppresses th17 differentiation and is associated with pathogenesis of multiple sclerosis by targeting O-glcNAc transferase. J Immunol (2017) 198:2626–39. doi: 10.4049/jimmunol.1601727
82. Lopez AA, Gao Y, Hou X, Lauvau G, Yates JR, Wu P. Profiling of protein O-glcNAcylation in murine CD8(+) effector- and memory-like T cells. ACS Chem Biol (2017) 12:3031–8. doi: 10.1021/acschembio.7b00869
83. Marchalonis JJ. Lymphocyte surface immunoglobulins. Science (1975) 190:20–9. doi: 10.1126/science.1101378
84. Ollila J, Vihinen M. B cells. Int J Biochem Cell Biol (2005) 37:518–23. doi: 10.1016/j.biocel.2004.09.007
85. Ford ES, Sholukh AM, Boytz R, Carmack SS, Klock A, Phasouk K, et al. B cells, antibody-secreting cells, and virus-specific antibodies respond to herpes simplex virus 2 reactivation in skin. J Clin Invest (2021) 131(9):e142088. doi: 10.1172/JCI142088
86. Ma Z, Chalkley RJ, Vosseller K. Hyper-O-GlcNAcylation activates nuclear factor kappa-light-chain-enhancer of activated B cells (NF-kappaB) signaling through interplay with phosphorylation and acetylation. J Biol Chem (2017) 292:9150–63. doi: 10.1074/jbc.M116.766568
87. Wu J, Wu H, Tsai D, Chiang M, Chen Y, Gao S, et al. Temporal regulation of Lsp1 O-GlcNAcylation and phosphorylation during apoptosis of activated B cells. Nat Commun (2016) 7:12526. doi: 10.1038/ncomms12526
88. Wu J, Chiang M, Hsu P, Tsai D, Hung K, Wang Y, et al. O-GlcNAcylation is required for B cell homeostasis and antibody responses. Nat Commun (2017) 8(1):1854. doi: 10.1038/s41467-017-01677-z
89. Zhang B, Zhou P, Li X, Shi Q, Li D, Ju X. Bitterness in sugar. Am J Cancer Res (2017) 7:1337–49.
90. Lee DH, Kwon NE, Lee WJ, Lee MS, Kim DJ, Kim JH, et al. Increased O-glcNAcylation of c-myc promotes pre-B cell proliferation. Cells-Basel (2020) 9(1):158. doi: 10.3390/cells9010158
91. Chou TY, Hart GW, Dang CV. c-Myc is glycosylated at threonine 58, a known phosphorylation site and a mutational hot spot in lymphomas. J Biol Chem (1995) 270:18961–5. doi: 10.1074/jbc.270.32.18961
92. Umapathi P, Mesubi OO, Banerjee PS, Abrol N, Wang Q, Luczak ED, et al. Excessive O-glcNAcylation causes heart failure and sudden death. CIRCULATION (2021) 143:1687–703. doi: 10.1161/CIRCULATIONAHA.120.051911
93. Kronlage M, Dewenter M, Grosso J, Fleming T, Oehl U, Lehmann LH, et al. O-glcNAcylation of histone deacetylase 4 protects the diabetic heart from failure. Circulation (2019) 140:580–94. doi: 10.1161/CIRCULATIONAHA.117.031942
94. Ou W, Liang Y, Qin Y, Wu W, Xie M, Zhang Y, et al. Hypoxic acclimation improves cardiac redox homeostasis and protects heart against ischemia-reperfusion injury through upregulation of O-GlcNAcylation. Redox Biol (2021) 43:101994. doi: 10.1016/j.redox.2021.101994
95. Shi Q, Shen Q, Liu Y, Shi Y, Huang W, Wang X, et al. Increased glucose metabolism in TAMs fuels O-GlcNAcylation of lysosomal Cathepsin B to promote cancer metastasis and chemoresistance. Cancer Cell (2022) 40:1207–22. doi: 10.1016/j.ccell.2022.08.012
96. Wang X, Liu M, Chu Y, Liu Y, Cao X, Zhang H, et al. O-GlcNAcylation of ZEB1 facilitated mesenchymal pancreatic cancer cell ferroptosis. Int J Biol Sci (2022) 18:4135–50. doi: 10.7150/ijbs.71520
Keywords: OGT, OGA, O-GlcNAcylation, innate immunity, immune cells
Citation: Cai H, Xiong W, Zhu H, Wang Q, Liu S and Lu Z (2023) Protein O-GlcNAcylation in multiple immune cells and its therapeutic potential. Front. Immunol. 14:1209970. doi: 10.3389/fimmu.2023.1209970
Received: 21 April 2023; Accepted: 07 August 2023;
Published: 22 August 2023.
Edited by:
Parameswaran Ramakrishnan, Case Western Reserve University, United StatesReviewed by:
Lara Abramowitz, National Institute of Diabetes and Digestive and Kidney Diseases (NIH), United StatesCopyright © 2023 Cai, Xiong, Zhu, Wang, Liu and Lu. This is an open-access article distributed under the terms of the Creative Commons Attribution License (CC BY). The use, distribution or reproduction in other forums is permitted, provided the original author(s) and the copyright owner(s) are credited and that the original publication in this journal is cited, in accordance with accepted academic practice. No use, distribution or reproduction is permitted which does not comply with these terms.
*Correspondence: Shi Liu, bGl1c2hpX2xpdXNoaUB3aHUuZWR1LmNu; Zhibing Lu, bHV6aGliaW5nMjIyQDE2My5jb20=
†These authors have contributed equally to this work and share first authorship
‡These authors have contributed equally to this work
Disclaimer: All claims expressed in this article are solely those of the authors and do not necessarily represent those of their affiliated organizations, or those of the publisher, the editors and the reviewers. Any product that may be evaluated in this article or claim that may be made by its manufacturer is not guaranteed or endorsed by the publisher.
Research integrity at Frontiers
Learn more about the work of our research integrity team to safeguard the quality of each article we publish.