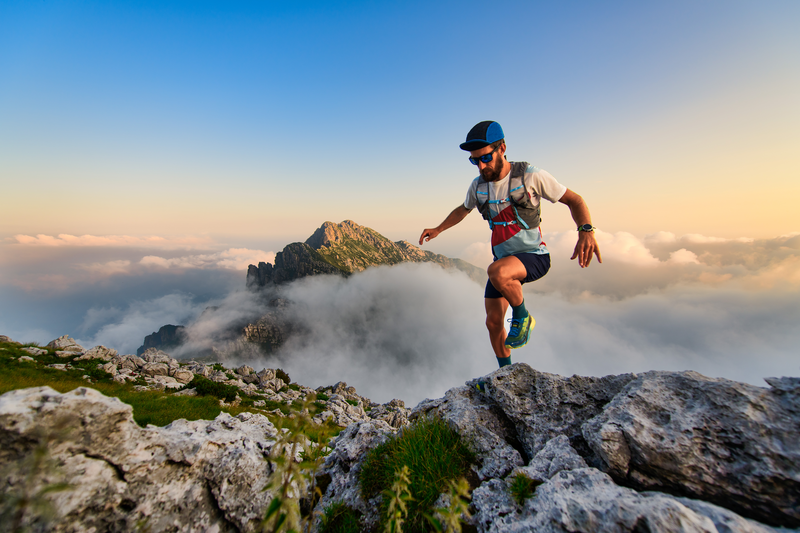
94% of researchers rate our articles as excellent or good
Learn more about the work of our research integrity team to safeguard the quality of each article we publish.
Find out more
ORIGINAL RESEARCH article
Front. Immunol. , 24 August 2023
Sec. Parasite Immunology
Volume 14 - 2023 | https://doi.org/10.3389/fimmu.2023.1208822
Background: Plasmodium falciparum malaria is a leading cause of child mortality in Nigeria. Neonates are born with maternal antibodies from placental transfer which may protect against malaria infection in the first months of life. The IgG dynamics of the transition from passively transferred antimalarial antibodies to actively acquired IgG from natural exposure have not been well elucidated.
Methods: Blood samples collected during a 2018 Nigeria nationwide HIV/AIDS household survey were available for 9,443 children under 5 years of age, with a subset of infants under 2 months of age having maternal samples available (n=41). Samples were assayed for the P. falciparum HRP2 antigen and anti-malarial IgG antibodies. LOESS regression examined the dynamics in IgG response in the first 5 years of life. Correlation with maternal IgG levels was assessed for mother/child pairs.
Results: Consistent decreases were observed in median IgG levels against all Plasmodium spp. antigen targets for the first months of life. At a population level, P. falciparum apical membrane antigen-1 (AMA1) and merozoite surface protein-1 19kD (PfMSP1) IgG decreased during the first 12 months of life before reaching a nadir, whereas IgGs to other targets only declined for the first 4 months of life. Seropositivity showed a similar decline with the lowest seropositivity against AMA1 and PfMSP1 at 10-12 months, though remaining above 50% during the first 2 years of life in higher transmission areas. No protective association was observed between IgG positivity and P. falciparum infection in infants. Maternal antibody levels showed a strong positive correlation with infant antibody levels for all P. falciparum antigens from birth to 2 months of age, but this correlation was lost by 6 months of age.
Discussion: Maternally transferred anti-malarial IgG antibodies rapidly decline during the first 6 months of life, with variations among specific antigens and malaria transmission intensity. From 3-23 months of age, there was a wide range in IgG levels for the blood-stage antigens indicating high individual variation in antibody production as children are infected with malaria. Non-falciparum species-specific antigens showed similar patterns in waning immunity and correlation with paired mother’s IgG levels compared to P. falciparum antigens.
Malaria is a leading cause of morbidity and mortality in sub-Saharan Africa, and in Nigeria, it is estimated that 14% of all under-five deaths are due to malaria (1). In countries with high levels of malaria transmission, infants are typically born with immunoglobulin G (IgG) antibodies against the endemic Plasmodium spp., which are transferred through the placenta while in utero (2). Additionally, there is evidence that anti-Plasmodium IgG can also be passively transferred through breast milk (3). Infants are partially protected against malaria infection and severe malaria for the first 3-6 months of life (4), and it has been well-documented that malaria infections among young infants (< 6 months old) are typically of low parasite density, asymptomatic, and clear spontaneously (5–7). Maternally-derived anti-Plasmodium IgG antibodies are thought to provide protection against this bloodborne pathogen during the first few months of life, though the mechanistic role(s) of these antibodies are not well elucidated for preventing malaria infection or febrile disease (8, 9). Besides passively-transferred IgG, other factors that may prevent malaria infection in infants include high levels of infant lactoferrin (which binds to iron) and secretory IgA (at high concentrations in breast milk) which have been found to inhibit in vitro growth of P. falciparum (10). Fetal hemoglobin (HbF) inhibits parasite development and provides another layer of protection for the first few months of life (6, 11). A study by Amaratunga, et al. suggests that HbF and IgG against P. falciparum PfEMP-1 cooperate to impair the cytoadherence of parasitized red blood cells in infants and thus substantially reduce the severity of Plasmodium infection (11). As HbF and maternal IgG levels decline, infants experience increased susceptibility to malaria infection and adverse outcomes. Depending on the level of malaria transmission in a setting, older infants (or children) have a higher cumulative risk of exposure to Plasmodium infection and would only be able to develop their own immune responses following such malaria parasite exposure; therefore, there may be a gap between the protection provided by neonatal factors together with maternal passive antibody transfer and the acquired response of the young person’s adaptive immune system.
Protection against malaria for the first year of life is dependent on the transmission intensity in the area and the month of infant birth (due to seasonal malaria exposure). Infants born in areas of higher transmission have been found to be generally protected for approximately 3 months (12), while in areas of low transmission, the risk of clinical malaria remains low for the first 6 months of life (13). Consistent with this association between transmission intensity and infants’ protection against malaria, it has been hypothesized that the apparent protection seen in young infants may merely result from limited exposure time to malaria due to their young age (14). Additionally, adults and older children are more often bitten by Anopheles mosquitoes compared to younger children and infants (15, 16). However, some studies have found that higher maternal antibody levels provide more extended protection against P. falciparum infection compared to infants with low maternal antibody levels, irrespective of transmission intensity (17).
This study reports on findings from IgG antibody and antigen assays from blood samples obtained from children and their mothers who were enrolled at their place of residence during the 2018 Nigeria HIV and AIDS Indicator Survey (NAIIS). In order to describe antimalarial IgG dynamics in early life in this Nigerian population, trends in antibody levels over the first 2 years of life and correlation between paired maternal and very young infant antibody levels are assessed. The antibody levels of very young infants (< 2 months old) were also compared between lower and higher P. falciparum transmission areas of Nigeria.
All participants provided informed consent before enrollment into the NAIIS survey. For children <10 years of age, the parent or guardian required written consent for biomarker testing and consent to future testing. Assent was also received from the children that were <10 years of age. Secondary laboratory testing for malaria biomarkers and laboratory data collection were approved by the National Health Research Ethics Committee of Nigeria (NHREC/01/01/2007) and were determined not to be involved in human research by the U.S. Centers for Disease Control and Prevention (CDC) Human Subjects office (project 0900f3eb819d4c63).
Ethical approval for secondary laboratory data collection of malaria biomarkers was approved for the Nigeria Multi-disease Serologic Surveillance using Stored Specimens (NMS4) project by the NHREC and U.S. CDC. All multiplex antigen detection and IgG detection assays were performed at the National Reference Laboratory (NRL) of the Nigeria Centre for Disease Control (NCDC) in Abuja. Multiplex antigen and IgG detection were attempted for all dried blood spot (DBS) specimens for children <15 years of age, and a subset of women of reproductive age (15-44 years). The data analysis shown in this article will focus on children under 5 years of age and paired mothers included in the women of reproductive age cohort.
The NAIIS survey was a cross-sectional national household HIV survey conducted in Nigeria from July through December 2018. The Nigerian Federal Ministry of Health and the National Agency for the Control of AIDS led the national population-based NAIIS survey. Further details on the sampling design are available in the NAIIS report (18).
Among sampled households (N= 101,580), every fourth household was randomly selected for data and blood biomarker collection of children under 15 years of age. Laboratory staff collected 6 mL venous blood from children aged 2-14 years and 1 mL capillary blood (finger or heel stick) from children <2 years old. Whole blood samples were stored in cooler boxes and transported to satellite laboratories for processing into plasma and DBS within 24 hours of collection. Specimens were stored in -20°C freezers and transported to the central laboratory within a week where they were stored in -80°C freezers.
To rehydrate blood samples from DBS, a 6-mm punch (approximately 10 µL whole blood) was taken from each DBS sample and blood was eluted to a 1:40 concentration in blocking buffer (Buffer B: Phosphate Buffered Saline (PBS) pH 7.2, 0.5% Bovine Serum Albumin (BSA), 0.05% Tween 20, 0.02% sodium azide, 0.5% polyvinyl alcohol, 0.8% polyvinylpyrrolidone, and 3 µg/mL Escherichia coli extract) (19). Elutions were stored at 4°C until testing. As described previously (20), samples were assayed at the 1:40 whole blood dilution for P. falciparum histidine-rich protein 2 (HRP2). Assay plates were read on MAGPIX™ machines (Luminex Corporation, Austin, TX), and with a target of 50 beads/region, the median fluorescence intensity (MFI) was generated for each analyte. For each assay plate, the buffer background was subtracted for each target’s MFI signal to provide an MFI-bg assay signal for analysis. To determine the assay signal threshold for antigen positivity, a finite mixture model (FMM) approach was employed to estimate the ‘antigen negative’ population within these data (using data from specimens from all children <15 years of age from 36 states and FCT plus a sample of 9,191 women of reproductive age with completed antigen testing) and then establishing a cutoff using the mean + two standard deviations from this distribution (21).
The IgG-detecting multiplex bead assay (MBA) was performed as described previously (19). The four Plasmodium merozoite surface protein 1, 19kD (MSP1) antigens from P. falciparum, P. malariae, P. ovale, and P. vivax were utilized to assess IgG levels to different Plasmodium spp. Additionally, the following P. falciparum antigens were also included: apical membrane antigen-1 (AMA1), circumsporozoite protein (CSP), liver-stage antigen (LSA1), glutamate-rich protein R0 fragment (GLURP-R0), Schistosoma japonicum glutathione-S-transferase (GST) served as a control antigen (22). Further details for each antigen used in this multiplex IgG detection assay are included in Supplementary Table 1. From the DBS elution described above, an approximate serum dilution of 1:400 was used for the assay. Any DBS with GST MFI-bg reads above 500 were excluded from the analysis for quality assurance purposes to remove samples with potential non-specific IgG binding (23). To dichotomize seropositivity to each antigen, a 2-component FMM of the log10-transformed MFI-bg signal was fit for each antigen’s IgG response (22), and the positivity cutoff was determined by the lognormal mean of the first component (presumed seronegative) plus three standard deviations (21). Due to high levels of P. falciparum exposure and seropositivity, a 6-component FMM was created for PfMSP1 and AMA1 to best define the first, putative seronegative, component (24). Due to the transition between passively-transferred IgG in child serum, and actively acquired serum antibodies through natural exposure, different seropositivity cutoffs were generated for the population of children under 2, and the population of children from 2 to 4 years of age.
FMM plots were generated in SAS version 9.4 (SAS Institute, Cary, NC). All remaining analyses were conducted in R version 4.1.1 (R Foundation for Statistical Computing, Vienna, Austria). Locally estimated scatterplot smoothing (LOESS) regression was used to show the proportion of children seropositive by age in months up until 4 years of age. Age in months was only available for children under 2 years, and seropositivity was plotted at the midpoint of the age group above 2 years (e.g., 30 months for 2 years of age). We also analyzed the proportion of children seropositive by age group stratified by relative lower and higher transmission areas of Nigeria. As an objective measure of P. falciparum transmission in the population through antimalarial antibody carriage, the definition for a higher transmission area of Nigeria was inclusive of any state at or above the median of seropositivity to PfMSP1 among the children < 5 years of age. As a proxy for previous P. falciparum exposure, this metric was utilized only to classify Nigerian states into relatively higher or lower transmission of P. falciparum. Demographics and malaria positivity estimates were adjusted considering the complex sample design using the R survey package (25). Mother and child IgG levels were assessed for available pairs for several age groups (children under 1 month, 1 month, 2 months, 6 months, etc.) fitting a linear regression line on a scatterplot. Kendall’s rank correlation coefficient (τ) was used to determine the correlation between paired maternal and very young infants’ IgG levels. Any IgG MFI-bg levels < 1.0 were assigned a value of 1.0 to be included in the analysis when log-transforming the MFI-bg values.
A total of 9,487 DBS samples from children under 60 months of age were available for testing by the multiplex assay. Of these, 44 (0.5%) had a GST assay signal above 500 and were excluded from the analysis for quality assurance (23), leaving a total of 9,443 samples available for analysis. Table 1 presents the demographic characteristics of the children for whom samples are included in this analysis. Compared with the other Nigerian zones, a higher percentage of children were from the North West Zone (27.9%), though the number of children from any one zone was over 1,000 (range: 1,241-2,630). The number of children with serology data available for analysis by state in Nigeria is shown in Supplemental Table 2 (median: 220; range: 74-563). Representation by sex was even: 50.9% male vs. 49.1% female.
Table 1 Demographic characteristics of the study population with serological data collected: Nigeria, 2018.
The youngest children (less than 2 months of age) had the highest median IgG levels to all five P. falciparum antigens utilized in this panel (Figure 1A), as well as the three non-falciparum MSP1 antigens (Supplementary Figure 1A). As infants aged in this population, decreases were observed in median IgG levels, with the longest monotonic decreases in median IgG levels observed for 11 months (for AMA1) and the shortest monotonic decreases for the non-falciparum MSP1 antigens (all 3 months or less). Upon reaching the lowest point for IgG levels to any Plasmodium antigen, population median IgG levels up to 24 months of age were highly variable for the AMA1 and PfMSP1 antigens but variation was generally low for all other IgG targets (Figure 1A). Between 0 and 6 months of age, significant reductions in IgG levels were observed for all eight IgG targets, with the greatest reduction in CSP IgG (by 52.0%) and the smallest reduction in PvMSP1 IgG (by 15.1%) (Table 2). All P. falciparum antigens saw a minimum 32.2% decrease in the population IgG levels for infants as they aged from 0 to 6 months.
Figure 1 Anti-Plasmodium falciparum antibody dynamics in Nigerian children under the age of five years. (A) IgG levels for children 0-23 months of age. Boxes display interquartile range (IQR) and whiskers extend 1.5x IQR above and below. Markers indicate observations outside of 1.5x IQR. Medians are displayed by horizontal lines. (B) IgG seropositivity by age to P. falciparum antigens for 0-59 month-old-children with smoothed LOESS regression curve (solid line) and 95% confidence intervals (shading).
For all Plasmodium antigens, IgG seropositivity among <1 month-old neonates (essentially at birth) ranged from 9.1% to 90.9% (Table 2). P. falciparum IgG targets reached a seropositivity low point between 10-18 months of age, but then continually increased up to the oldest ages assessed here: 59 months (Figure 1B). The low point for seropositivity for the P. falciparum AMA1 and PfMSP1 targets remained steady at approximately 40-60% up to 24 months of age, but by five years of age, 78.1% and 81.1% of the children had a positive IgG response to AMA1 and PfMSP1, respectively. At the population level, increases in seropositivity were also observed for the GLURP-R0, CSP, and LSA1 IgG targets as children approached the age of 5 years, though not at the same rate as the AMA1 and PfMSP1 antigens. Between 24 months and 5 years of age, steady increases in seropositivity were also seen for the PmMSP1, PoMSP1, and PvMSP1 antigens (Supplemental Figure 1B).
When comparing populations of children living in areas of relatively different P. falciparum transmission intensity (as defined in Methods, and displayed in Supplemental Table 2 and Supplemental Figure 2), the proportion of neonates (<1 month old) seropositive to different Plasmodium targets between higher and lower transmission areas was similar among all targets (Figure 2) with no statistically significant differences found in IgG levels to any of the P. falciparum targets (Supplementary Table 3). However, as children in the population aged from 0 to 10 months, sharper decreases were noted in loss of IgG seropositivity (seroreversion) in populations of children residing in relatively lower transmission areas versus the higher transmission group, and this trend was most pronounced for the AMA1, PfMSP1, and LSA1 antigens. For all P. falciparum IgG targets, the LOESS curves for child seropositivity rates were separated with children in the higher transmission settings consistently showing higher seropositivity. As stratified by the P. falciparum transmission setting, seropositivity to the non-falciparum targets was generally higher in higher P. falciparum areas, but was more variable due to overall lower seropositivity to non-falciparum antigens (Supplemental Figure 3).
Figure 2 Seropositivity to Plasmodium falciparum antigens by relative transmission intensity. Smoothed LOESS regression curves indicate estimates by lower transmission (blue hashed line) and higher transmission (red solid line) settings in Nigeria. Grey shading around LOESS curves indicates a 95% confidence interval.
HRP2 antigenemia during the first 2 months of life could be a factor of congenital malaria infection (26), placental malaria in utero (27), or P. falciparum infection acquired after birth. In this Nigerian population, young children were found to be more likely to carry HRP2 antigen as they aged. HRP2 positivity among all 0- to 5-month-old children was 18.3% which increased to 22.8% in 6- to 11-month-olds, 29.9% in 12- to 17-month-olds, and 27.0% in 18- to 23-month-olds. In comparing HRP2 antigenemia among infants less than 6 months of age to the paired maternal samples, the association for frequency of antigenemia was not found to be significant (Fisher’s exact p-value = 0.15, Supplementary Table 4). Differences in IgG seropositivity among young children were assessed by HRP2 antigenemia status. Though HRP2-positive infants typically had higher seropositivity rates for Plasmodium antigens versus HRP2-negative infants, no substantial differences in seropositivity were observed for all five P. falciparum antigens and the three non-falciparum MSP1 antigens (Supplementary Table 5).
A total of 41 pairs of DBS samples had IgG results available for infants less than 2 months old and their mothers. Overall, there was a strong correlation between these infants’ and their mothers’ IgG levels for all Plasmodium antigens (median Kendall’s τ= 0.45) (Table 3, Figure 3), with the highest correlation for PfMSP1 (τ= 0.65) and lowest for LSA1 and PvMSP1 (τ= 0.29). Except for PmMSP1 IgG levels, median IgG levels to all other Plasmodium antigens were consistently higher in mothers compared to their corresponding infants (Supplementary Figure 4). As children age, the correlation between infant and mothers’ IgG levels is essentially lost after approximately 6 months of age as shown in Supplementary Figure 5. HRP2 antigenemia among children under 2 years of age was compared by mother’s IgG levels as stratified by tertiles and categorized by different child age groups (Supplementary Table 6, Supplementary Figure 6). For all P. falciparum IgG targets assessed, mothers with higher IgG levels generally had a higher proportion of children with HRP2 antigenemia compared with mothers with lower IgG levels to the P. falciparum targets.
Figure 3 Scatterplots of paired maternal and very young infants’ anti-malarial IgG levels against P. falciparum antigens. A solid line shows linear regression fitting for the data with shading indicating a 95% confidence interval.
Neonates are born with a variety of different serum and immunological factors which offer a level of protection against the establishment of Plasmodium spp. infection and development of severe malaria. One of the most abundant factors in terms of serum concentration is IgG antibodies which are transferred across the fetal placenta in utero (8, 28, 29). For neonates and young infants across Nigeria, this current study found IgG levels against P. falciparum sporozoite, liver-stage, and blood-stage antigens were all very high during the first 3 months of life and quickly waned as infants aged through their first year of life. Median IgG levels against the AMA1 and PfMSP1 antigens decreased to their lowest level in infants around 11 months of age but reached a low point around 5-7 months of age for CSP, GLURP-R0, and LSA1. IgG levels for these latter three targets remained low throughout the first 2 years of life, but high variability in AMA1 and PfMSP1 antigens as children aged showed P. falciparum exposure and adaptive immune responses to these immunogenic antigens. Maternal transfer of non-falciparum IgG was also noted in this neonatal study population (24) and showed similar temporal dynamics to the P. falciparum IgG targets. Similar trends of waning maternally-derived IgG levels have been found in previous studies, with antimalarial IgG levels decreasing to their lowest between 6-9 months of age in other high-transmission settings and the lowest proportion of seropositive infants was seen at approximately 10 months for AMA1 and PfMSP1 (30, 31). These previous findings, in conjunction with the results presented here, suggest that even in very high transmission and holoendemic settings, infants have lost all passively-transferred maternal IgG by their first birthday.
Several studies have shown that maternal antibodies in cord blood provide protection against severe malaria in infants (32, 33). One longitudinal study found that infants with high anti-PfSEA-1 antibody levels in cord blood had 51% fewer cases of severe malaria compared to those with lower antibody levels over the 12-month follow-up period (33). Some previous studies have observed a protective effect of maternal antibodies against any malaria infections in infants while others have not (8, 34). Branch and colleagues found that infants with high IgG and IgM levels to MSP-1-19kD antigen experienced a longer time to first infection (113 vs. 69 days) in a Kenyan cohort (35). Importantly, all participants from this current Nigerian survey were enrolled in their households, so any P. falciparum infections identified here would most likely be described as low-density and asymptomatic, or at least not exhibiting treatment-seeking behavior at the time of sampling. This current study utilized a relatively small panel of five P. falciparum antigen targets for IgG detection and was only able to identify the presence of P. falciparum by HRP2 antigenemia. Taking these points into consideration, higher levels of maternal IgG to these P. falciparum antigens did not have a protective effect in reducing rates of P. falciparum in the corresponding children. In fact, mothers with higher IgG levels consistently had children with higher rates of HRP2 antigenemia. This association is most likely due to the fact that the higher IgG levels of mothers are a reliable proxy for sustained P. falciparum transmission in their area of residence, so their children residing in the same area are also subjected to these higher levels of P. falciparum exposure (30).
It is documented that it takes many occurrences of natural exposure to P. falciparum to develop an immune response capable of preventing clinical malaria (36). Even in higher transmission settings, resistance through adaptive immune responses to the most severe forms of malaria is obtained through multiple natural exposures over 5 years, and resistance to any clinical malaria begins to be attained by late childhood or early adolescence (37). The findings presented here show that from 3 to 23 months of age, there was a wide interquartile range in IgG levels against the blood-stage antigens. This likely indicates that there are many factors that influence antibody levels in the first 2 years of life, including the amount of malaria exposure, behavioral factors, and protective measures that are utilized. Passively transferred maternal anti-malarial IgG was consistently highest within the first 2 months of life. Along with IgG to other pathogen-specific antigens, IgG antibodies against malaria parasites are transferred via the placenta during fetal development (2). In this study, it was observed that mother and infant IgG levels are highly correlated for infants less than 2 months old, with strong mother/child correlation in the quantitative levels of AMA1, PfMSP1, CSP, and GLURP-R0 antigens. A previous study in Kenya testing placental cord blood and found that 97% of samples were positive for AMA1 IgG antibodies and 87% tested positive for CSP IgG antibodies (30). Other studies have detected maternal IgG antibodies against P. falciparum AMA1, CSP, GLURP-R0, and MSP1-19 antigens in cord blood (29, 32, 38, 39). This current study found neonatal antimalarial IgG levels were generally lower compared to their mothers’ levels, and this is consistent with other studies conducted in different P. falciparum transmission settings (8, 28, 40, 41).
When assessing children living in the lower malaria transmission areas of Nigeria, a more rapid decline in seropositivity was observed for the P. falciparum antigens compared to the children in higher transmission areas. This is most likely due to lower P. falciparum exposure in lower transmission areas compared to the higher transmission settings. Between 5-24 months of age, most children in lower transmission areas were seronegative to the AMA1 and PfMSP1 antigens, while seropositivity never fell below 50% for the children in higher transmission areas. One study that followed children in Kenya during a year of decreasing malaria transmission identified that the average antibody levels against AMA1 and MSP2 significantly declined in all age groups between the start and end of the year (42).
This study is subject to several limitations. Primarily, the cross-sectional design does not allow for longitudinal assessment of an individual child’s IgG levels over time, so the assumption by the analyses is that infants sampled at each age category offer a proxy for children as they age from month to month in Nigeria. Though nearly 10,000 samples were available from children less than 5 years of age in this study, an overall limited number of infant samples paired with maternal samples were available for assessing mother/child correlation (n= 66 for HRP2 antigenemia among children under 6 months and n= 41 for IgG data among children under 2 months). Additionally, in assessing HRP2 antigenemia as a measure of active infection, recent clinical history was unavailable for these persons, and HRP2 transfer across the placental barrier is poorly understood. Only eight Plasmodium antigens were utilized for IgG capture, though numerous Plasmodium antigens have been identified to date. Functional assays were not performed, nor was sub-typing of IgG antibodies, so total IgG binding to antigens was the only antibody data collected in this study. No data on symptomatic status nor clinical history (previous or post-survey) for these children was collected, so the assessment of P. falciparum infection was only considering if the child had evidence of infection at the time of sampling, and protection from clinical disease was unable to be ascertained. Infection or exposure to many other infectious agents endemic to Nigeria was not ascertained in this study, so potential IgG cross-reactivity could not be assessed here.
Understanding the transition from passively transferred maternal antibodies present in infant serum to the active adaptive immune responses of the host can assist in determining the risk of severe disease for infants in different malaria transmission settings. This work additionally found that antibodies to non-falciparum species-specific antigens showed similar patterns in waning immunity and the correlation with paired mother’s IgG levels was similar to P. falciparum antigens. Further studies should evaluate both maternal and neonatal factors associated with protective immunity in infants.
The original contributions presented in the study are included in the article/Supplementary Material, further inquiries can be directed to the corresponding author.
The studies involving human participants were reviewed and approved by National Health Research Ethics Committee of Nigeria. Written informed consent to participate in this study was provided by the participants’ legal guardian/next of kin.
Project coordination: PU, AA, AO, NM, SG, MO, NI, CI, LS, and ER; Data collection: AA and ER; Formal analysis: CL; Manuscript drafting: CL and ER. All authors contributed to the article and approved the submitted version.
Oluwaseun O. Akinmulero, Andrew Thomas, Ayuba Dawurung, Mudiaga Eseikpe, Abubakar Bichi Iliyasu, Nengi Israel, Erasogie Evbuomwan, Chimaoge Achugbu, Mary Okoli, Nnaemeka Ndodo.
The NMS4 project was financed by contributions from the President’s Emergency Plan for AIDS Relief (PEPFAR), the US President’s Malaria Initiative, the Global Immunization Division of the US Centers for Disease Control and Prevention, and The Global Fund to Fight AIDS, Tuberculosis, and Malaria, and the Bill and Melinda Gates Foundation through CDC Foundation.
The authors would like to acknowledge the field teams of the NAIIS survey staff, Nigerian survey participants, and NMS4 technical working group. The authors acknowledge the contribution of valuable specimens by the NAIIS Group, including the Federal Ministry of Health (FMoH), National Agency for the Control of AIDS (NACA), National Population Commission (NPopC), National Bureau of Statistics (NBS), U.S. Centers for Disease Control and Prevention (CDC), The Global Funds to Fight AIDS, Tuberculosis, and Malaria, University of Maryland Baltimore (UMB), ICF International, African Field Epidemiology Network (AFENET), University of Washington (UW), the Joint United Nations Programme on HIV and AIDS (UNAIDS), World Health Organization (WHO), and United Nations Children’s Fund (UNICEF).
The authors declare that the research was conducted in the absence of any commercial or financial relationships that could be construed as a potential conflict of interest.
All claims expressed in this article are solely those of the authors and do not necessarily represent those of their affiliated organizations, or those of the publisher, the editors and the reviewers. Any product that may be evaluated in this article, or claim that may be made by its manufacturer, is not guaranteed or endorsed by the publisher.
The findings and conclusions in this report are those of the authors and do not necessarily represent the official position of the US Centers for Disease Control and Prevention, NAIIS group, or Nigeria Centre for Disease Control.
The Supplementary Material for this article can be found online at: https://www.frontiersin.org/articles/10.3389/fimmu.2023.1208822/full#supplementary-material
1. Liu L, Oza S, Hogan D, Chu Y, Perin J, Zhu J, et al. Global, regional, and national causes of under-5 mortality in 2000-15: an updated systematic analysis with implications for the Sustainable Development Goals. Lancet (2016) 388(10063):3027–35. doi: 10.1016/S0140-6736(16)31593-8
2. Williams AI, McFarlane H. Distribution of malarial antibody in maternal and cord sera. Arch Dis Child (1969) 44(236):511–4. doi: 10.1136/adc.44.236.511
3. Leke RG, Ndansi R, Southerland NJ, Quakyi IA, Taylor DW. Identification of anti-Plasmodium falciparum antibodies in human breast milk. Scand J Immunol Suppl (1992) 11:17–22. doi: 10.1111/j.1365-3083.1992.tb01612.x
4. Snow RW, Nahlen B, Palmer A, Donnelly CA, Gupta S, Marsh K. Risk of severe malaria among African infants: direct evidence of clinical protection during early infancy. J Infect Dis (1998) 177(3):819–22. doi: 10.1086/517818
5. Falade C, Mokuolu O, Okafor H, Orogade A, Falade A, Adedoyin O, et al. Epidemiology of congenital malaria in Nigeria: a multi-centre study. Trop Med Int Health (2007) 12(11):1279–87. doi: 10.1111/j.1365-3156.2007.01931.x
6. Riley EM, Wagner GE, Akanmori BD, Koram KA. Do maternally acquired antibodies protect infants from malaria infection? Parasite Immunol (2001) 23(2):51–9. doi: 10.1046/j.1365-3024.2001.00364.x
7. Brabin B. An analysis of malaria parasite rates in infants: 40 years after Macdonald. Trop Dis Bull (1990) 87(10):20.
8. Riley EM, Wagner GE, Ofori MF, Wheeler JG, Akanmori BD, Tetteh K, et al. Lack of association between maternal antibody and protection of African infants from malaria infection. Infect Immun (2000) 68(10):5856–63. doi: 10.1128/IAI.68.10.5856-5863.2000
9. Kangoye DT, Mensah VA, Murungi LM, Nkumama I, Nebie I, Marsh K, et al. Dynamics and role of antibodies to Plasmodium falciparum merozoite antigens in children living in two settings with differing malaria transmission intensity. Vaccine (2016) 34(1):160–6. doi: 10.1016/j.vaccine.2015.10.058
10. Kassim O, Ako-Anai K, Torimiro S, Hollowell G, Okoye V, Martin S. Inhibitory factors in breastmilk, maternal and infant sera against in vitro growth of Plasmodium falciparum malaria parasite. J Trop Pediatr (2000) 46(2):92–6. doi: 10.1093/tropej/46.2.92
11. Amaratunga C, Lopera-Mesa TM, Brittain NJ, Cholera R, Arie T, Fujioka H, et al. A role for fetal hemoglobin and maternal immune IgG in infant resistance to Plasmodium falciparum malaria. PloS One (2011) 6(4):e14798. doi: 10.1371/journal.pone.0014798
12. Kitua AY, Urassa H, Wechsler M, Smith T, Vounatsou P, Weiss NA, et al. Antibodies against Plasmodium falciparum vaccine candidates in infants in an area of intense and perennial transmission: relationships with clinical malaria and with entomological inoculation rates. Parasite Immunol (1999) 21(6):307–17. doi: 10.1046/j.1365-3024.1999.00230.x
13. McGuinness D, Koram K, Bennett S, Wagner G, Nkrumah F, Riley E. Clinical case definitions for malaria: clinical malaria associated with very low parasite densities in African infants. Trans R Soc Trop Med Hyg (1998) 92(5):527–31. doi: 10.1016/S0035-9203(98)90902-6
14. Macdonald G. The analysis of malaria parasite rates in infants. Trop Dis Bull (1950) 47(10):915–38.
15. Goncalves BP, Kapulu MC, Sawa P, Guelbéogo WM, Tiono AB, Grignard L, et al. Examining the human infectious reservoir for Plasmodium falciparum malaria in areas of differing transmission intensity. Nat Commun (2017) 8(1):1133. doi: 10.1038/s41467-017-01270-4
16. Muirhead-Thomson RC. The distribution of anopheline mosquito bites among different age groups; a new factor in malaria epidemiology. Br Med J (1951) 1(4715):1114–7. doi: 10.1136/bmj.1.4715.1114
17. Reynaldi A, Dent AE, Schlub TE, Ogolla S, Rochford R, Davenport MP. Interaction between maternally derived antibodies and heterogeneity in exposure combined to determine time-to-first Plasmodium falciparum infection in Kenyan infants. Malar J (2019) 18(1):19. doi: 10.1186/s12936-019-2657-6
18. Federal Ministry of Health N. Nigeria HIV/AIDS Indicator and Impact Survey (NAIIS) 2018: Technical Report. (Abuja, Nigeria: Nigeria Federal Ministry of Health) (2019).
19. Arzika AM, Mindo-Panusis D, Abdou A, Kadri B, Nassirou B, Maliki R. Effect of biannual azithromycin distribution on antibody responses to malaria, bacterial, and protozoan pathogens in Niger. Nat Commun (2022) 13(1):976. doi: 10.1038/s41467-022-28565-5
20. Alvarado R, van den Hoogen LL, Iriemenam NC, Akinmulero OO, Thomas AN, Tamunonengiyeofori I, et al. Considerations for quality assurance of multiplex malaria antigen detection assays with large sample sets. Sci Rep (2021) 11(1):13248. doi: 10.1038/s41598-021-92723-w
21. Rogier E, Wiegand R, Moss D, Priest J, Angov E, Dutta S, et al. Multiple comparisons analysis of serological data from an area of low Plasmodium falciparum transmission. Malar J (2015) 14:436. doi: 10.1186/s12936-015-0955-1
22. Priest JW, Plucinski MM, Huber CS, Rogier E, Mao B, Gregory CJ, et al. Specificity of the IgG antibody response to Plasmodium falciparum, Plasmodium vivax, Plasmodium malariae, and Plasmodium ovale MSP1(19) subunit proteins in multiplexed serologic assays. Malar J (2018) 17(1):417. doi: 10.1186/s12936-018-2566-0
23. van den Hoogen LL, Presume J, Romilus I, Mondélus G, Elismé T, Sepúlveda N, et al. Quality control of multiplex antibody detection in samples from large-scale surveys: the example of malaria in Haiti. Sci Rep (2020) 10(1):1135. doi: 10.1038/s41598-020-57876-0
24. Herman C, Leonard CM, Uhomoibhi P, Maire M, Moss D, Inyang U, et al. Non-falciparum malaria infection and IgG seroprevalence among children under 15 years in Nigeria, 2018. Nat Commun (2023) 14(1):1360. doi: 10.1038/s41467-023-37010-0
25. Lumley T. Analysis of complex survey samples. J Stat Softw (2004) 9(1):1–19. doi: 10.18637/jss.v009.i08
26. Kajoba D, Ivan Egesa W, Jean Petit H, Omar Matan M, Laker G, Mugowa Waibi W, et al. Congenital malaria in a 2-day-old neonate: A case report and literature review. Case Rep Infect Dis (2021) 2021:9960006. doi: 10.1155/2021/9960006
27. Leke RF, Djokam RR, Mbu R, Leke RJ, Fogako J, Megnekou R, et al. Detection of the Plasmodium falciparum antigen histidine-rich protein 2 in blood of pregnant women: implications for diagnosing placental malaria. J Clin Microbiol (1999) 37(9):2992–6. doi: 10.1128/JCM.37.9.2992-2996.1999
28. Desowitz RS, Elm J, Alpers MP. Plasmodium falciparum-specific immunoglobulin G (IgG), IgM, and IgE antibodies in paired maternal-cord sera from east Sepik Province, Papua New Guinea. Infect Immun (1993) 61(3):988–93. doi: 10.1128/iai.61.3.988-993.1993
29. Duah NO, Miles DJ, Whittle HC, Conway DJ. Acquisition of antibody isotypes against Plasmodium falciparum blood stage antigens in a birth cohort. Parasite Immunol (2010) 32(2):125–34. doi: 10.1111/j.1365-3024.2009.01165.x
30. Dent AE, Malhotra I, Wang X, Babineau D, Yeo KT, Anderson T, et al. Contrasting patterns of serologic and functional antibody dynamics to plasmodium falciparum antigens in a Kenyan birth cohort. Clin Vaccine Immunol (2016) 23(2):104–16. doi: 10.1128/CVI.00452-15
31. Tassi Yunga S, Siriwardhana C, Fouda GG, Bobbili N, Sama G, Chen JJ, et al. Characterization of the primary antibody response to Plasmodium falciparum antigens in infants living in a malaria-endemic area. Malar J (2022) 21(1):346. doi: 10.21203/rs.3.rs-1865608/v1
32. Stephens JK, Kyei-Baafour E, Dickson EK, Ofori JK, Ofori MF, Wilson ML, et al. Effect of IPTp on Plasmodium falciparum antibody levels among pregnant women and their babies in a sub-urban coastal area in Ghana. Malar J (2017) 16(1):224. doi: 10.1186/s12936-017-1857-1
33. Kurtis JD, Raj DK, Michelow IC, Park S, Nixon CE, McDonald EA, et al. Maternally-derived antibodies to schizont egress antigen-1 and protection of infants from severe malaria. Clin Infect Dis (2019) 68(10):1718–24. doi: 10.1093/cid/ciy728
34. Dobbs KR, Dent AE. Plasmodium malaria and antimalarial antibodies in the first year of life. Parasitology (2016) 143(2):129–38. doi: 10.1017/S0031182015001626
35. Branch OH, Udhayakumar V, Hightower AW, Oloo AJ, Hawley WA, Nahlen BL, et al. A longitudinal investigation of IgG and IgM antibody responses to the merozoite surface protein-1 19-kiloDalton domain of Plasmodium falciparum in pregnant women and infants: associations with febrile illness, parasitemia, and anemia. Am J Trop Med Hyg (1998) 58(2):211–9. doi: 10.4269/ajtmh.1998.58.211
36. Langhorne J, Ndungu FM, Sponaas AM, Marsh K. Immunity to malaria: more questions than answers. Nat Immunol (2008) 9(7):725–32. doi: 10.1038/ni.f.205
37. Marsh K, Kinyanjui S. Immune effector mechanisms in malaria. Parasite Immunol (2006) 28(1-2):51–60. doi: 10.1111/j.1365-3024.2006.00808.x
38. Nhabomba AJ, Guinovart C, Jimenez A, Manaca MN, Quintó L, Cisteró P, et al. Impact of age of first exposure to Plasmodium falciparum on antibody responses to malaria in children: a randomized, controlled trial in Mozambique. Malar J (2014) 13:121. doi: 10.1186/1475-2875-13-121
39. Achidi EA, Perlmann H, Salimonu LS, Perlmann P, Walker O, Asuzu MC. A longitudinal study of seroreactivities to Plasmodium falciparum antigens in Nigerian infants during their first year of life. Acta Trop (1995) 59(2):173–83. doi: 10.1016/0001-706X(95)00076-Q
40. Charnaud SC, McGready R, Herten-Crabb A, Powell R, Guy A, Langer C, et al. Maternal-foetal transfer of Plasmodium falciparum and Plasmodium vivax antibodies in a low transmission setting. Sci Rep (2016) 6:20859. doi: 10.1038/srep20859
41. Deloron P, Dubois B, Le Hesran JY, Riche D, Fievet N, Cornet M, et al. Isotypic analysis of maternally transmitted Plasmodium falciparum-specific antibodies in Cameroon, and relationship with risk of P. falciparum Infection Clin Exp Immunol (1997) 110(2):212–8. doi: 10.1111/j.1365-2249.1997.tb08319.x
Keywords: malaria, antigens, maternal IgG, passive antibodies, humoral immunity
Citation: Leonard CM, Uhomoibhi P, Abubakar A, Ogunniyi A, Mba N, Greby SM, Okoye MI, Iriemenam NC, Ihekweazu C, Steinhardt L, Rogier E and NMS4 Technical Working Group (2023) Dynamics of IgG antibody response against Plasmodium antigens among Nigerian infants and young children. Front. Immunol. 14:1208822. doi: 10.3389/fimmu.2023.1208822
Received: 19 April 2023; Accepted: 24 July 2023;
Published: 24 August 2023.
Edited by:
Abhay Satoskar, The Ohio State University, United StatesReviewed by:
Adrian John Frederick Luty, Institut de Recherche Pour le Développement (IRD), FranceCopyright © 2023 Leonard, Uhomoibhi, Abubakar, Ogunniyi, Mba, Greby, Okoye, Iriemenam, Ihekweazu, Steinhardt, Rogier and NMS4 Technical Working Group. This is an open-access article distributed under the terms of the Creative Commons Attribution License (CC BY). The use, distribution or reproduction in other forums is permitted, provided the original author(s) and the copyright owner(s) are credited and that the original publication in this journal is cited, in accordance with accepted academic practice. No use, distribution or reproduction is permitted which does not comply with these terms.
*Correspondence: Eric Rogier, ZXJvZ2llckBjZGMuZ292
Disclaimer: All claims expressed in this article are solely those of the authors and do not necessarily represent those of their affiliated organizations, or those of the publisher, the editors and the reviewers. Any product that may be evaluated in this article or claim that may be made by its manufacturer is not guaranteed or endorsed by the publisher.
Research integrity at Frontiers
Learn more about the work of our research integrity team to safeguard the quality of each article we publish.