- 1Adagene Inc., San Diego, CA, United States
- 2Adagene (Suzhou) Limited., Suzhou, China
T cells play a critical role in antitumor immunity, where T cell activation is regulated by both inhibitory and costimulatory receptor signaling that fine-tune T cell activity during different stages of T cell immune responses. Currently, cancer immunotherapy by targeting inhibitory receptors such as CTLA-4 and PD-1/L1, and their combination by antagonist antibodies, has been well established. However, developing agonist antibodies that target costimulatory receptors such as CD28 and CD137/4-1BB has faced considerable challenges, including highly publicized adverse events. Intracellular costimulatory domains of CD28 and/or CD137/4-1BB are essential for the clinical benefits of FDA-approved chimeric antigen receptor T cell (CAR-T) therapies. The major challenge is how to decouple efficacy from toxicity by systemic immune activation. This review focuses on anti-CD137 agonist monoclonal antibodies with different IgG isotypes in clinical development. It discusses CD137 biology in the context of anti-CD137 agonist drug discovery, including the binding epitope selected for anti-CD137 agonist antibody in competition or not with CD137 ligand (CD137L), the IgG isotype of antibodies selected with an impact on crosslinking by Fc gamma receptors, and the conditional activation of anti-CD137 antibodies for safe and potent engagement with CD137 in the tumor microenvironment (TME). We discuss and compare the potential mechanisms/effects of different CD137 targeting strategies and agents under development and how rational combinations could enhance antitumor activities without amplifying the toxicity of these agonist antibodies.
Introduction
T cell-mediated immunity is crucial for the host antitumor response (1). Under physiological conditions, T cell activation requires two signals: signal 1 involves TCR activation triggered by the major histocompatibility complex (MHC) presented antigenic peptide, and signal 2, a costimulatory signal, amplifies the antigen-specific signal 1 (2). CD137 or 4-1BB, a member of the tumor necrosis factor receptor superfamily (TNFRSF), also known as TNFRSF9, is one of the key costimulatory receptors identified that has shown promise as a therapeutic target for boosting antitumor immune responses in both preclinical and clinical studies over the past two decades (3). CD137 is induced upon activation in T cells, B cells, and natural killer (NK) cells (4). Ligation of CD137 by its natural ligand, CD137L or 4-1BBL, recruits TNFR-associated factor (TRAF) 1 and TRAF2 and induces signaling through the master transcription factor NF-kB and MAPKs (5) (6), which coactivates CD8+ T cells and natural killer cells, resulting in enhanced cellular proliferation and survival, increased proinflammatory cytokine secretion, cytolytic function, and antibody-dependent cell-mediated cytotoxicity (7). As most tumors are killed by CTLs in an antigen-specific manner, agents that propel CD8+ T-cell activation and impart strong cytolytic, inflammatory, and immune-regulating properties in an antigen-specific manner are ideal candidates for enhancing antitumor immunity. Agonistic anti-CD137 mAb immunotherapy targeting CD8+ T cells meets these requisites. However, clinical development of the first fully human anti-CD137 IgG4 agonistic antibody, urelumab, was put on hold due to dose-dependent liver toxicity, including grade 3 and higher liver-related toxicities and two fatalities, despite demonstrating monotherapy efficacy in melanoma patients (3) (8). By contrast, the second clinical development of a fully human IgG2 anti-CD137 agonist antibody, utomilumab, has shown excellent safety and tolerability following a lengthy dose escalation schedule, presumably, due to the clinical safety issues for urelumab, the first agonist anti-CD137 antibody in clinics. Only modest or marginal efficacy in utomilumab monotherapy is reported for a few patients in immune-responsive Merkel cell carcinoma and for checkpoint-experienced melanoma and non-small cell lung cancer patients (9). These two extreme cases highlight the challenges in developing costimulatory receptor antibody therapies in general and anti-CD137 agonist antibodies in particular, where the preclinical observation is yet to translate into clinical reality. New generations of CD137 agonists with different targeting strategies are under development that could get around these challenges and realize the full potential of CD137 targeted immunotherapy for cancer treatment.
CD137 binding epitopes, complex conformations and IgG isotypes
The binding epitope on CD137 of an anti-CD137 antibody could have direct impact on its agonistic activity. Figure 1A illustrates that the trimeric CD137 ligand in gray binds to CRD2 and 3 on CD137 to cluster the receptor, whereas the binding epitopes of different anti-CD137 agonist antibodies can vary (10). For example, Urelumab binds to the N-terminal portion of CRD-1, utomilumab binds at the junction of CRD-3 and CRD-4 (10), and ADG106, a fully human anti-CD137 IgG4 agonist antibody developed by us, binds at the junction of CRD-2 and CRD-3, which overlaps with the CD137L binding site at CRD-2 and CRD-3 (Figure 1B) (11). Such differences in binding epitopes among different agonistic anti-CD137 antibodies explain their ligand-blocking versus non-blocking properties. Urelumab does not block CD137L interaction with CD137, whereas ADG106 strongly blocks CD137 binding to its ligand.
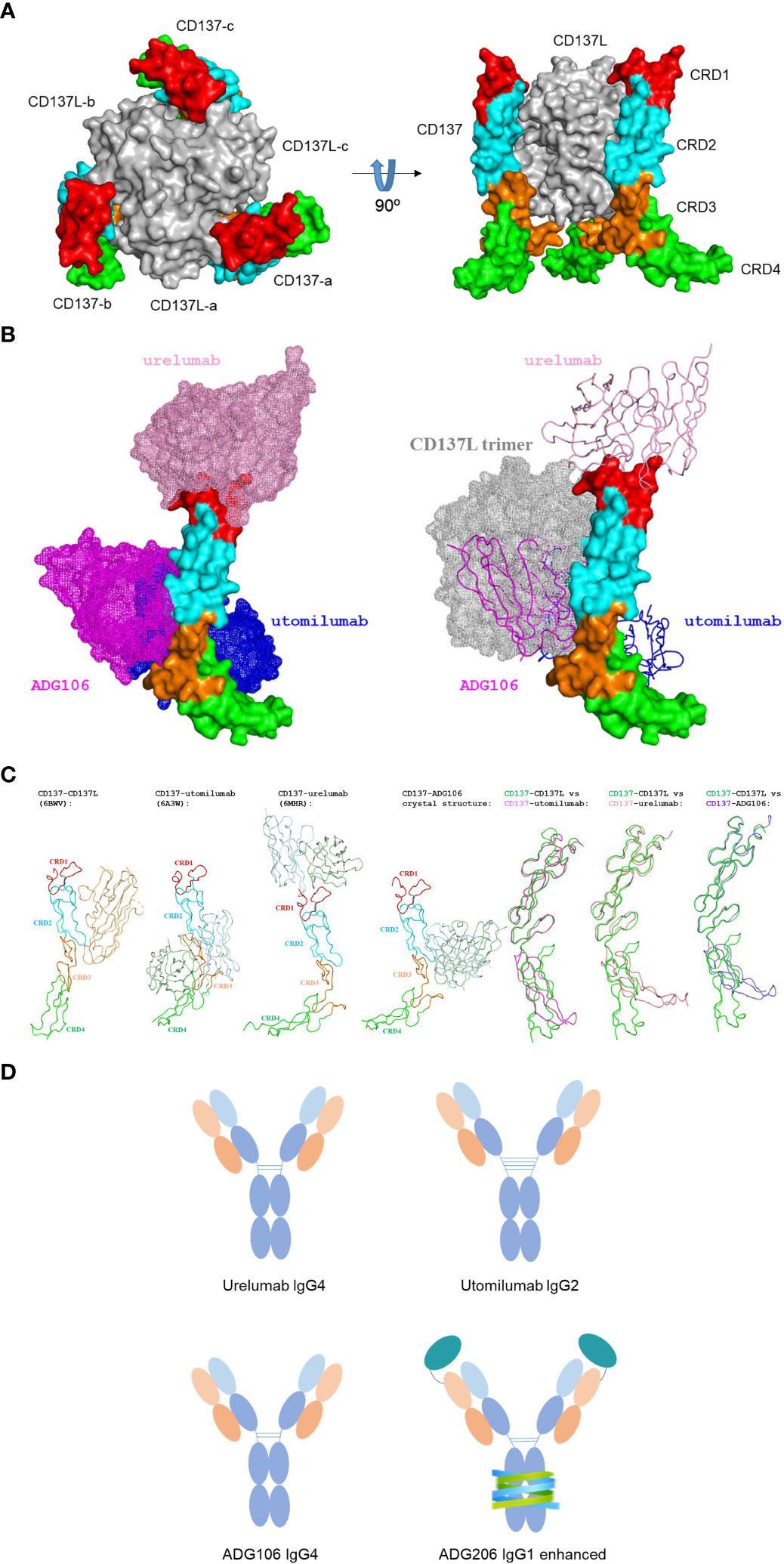
Figure 1 (A) The constructed structure of trimeric CD137 in complex with trimeric CD137L; (B) Structure of CD137 in complex with anti-CD137 agonistic mAbs with only one CD137 structure shown to illustrate the difference in CD137 binding epitopes by 3 anti-CD137 agonistic mAbs, together to show the complete overlap between ADG106 with CD137L trimers in gray; (C) The induced conformational changes of CD137 upon binding to CD137 ligand, three agonist antibodies and their pairwise comparison of the induced conformational change of CD137 with reference to its conformation upon binding to CD137 ligand; (D) the diagram illustrating the difference of IgG isotypes for urelumab, utomilumab, ADG106 and ADG206.
It should be noted that the conformation of the CD137 complex also depends on the binding epitope of the agonist antibodies in comparison with the CD137 ligand-induced complex conformation, which may play a role in CD137-mediated T cell signaling. CD137 conformations in complex with different agonist antibodies, including urelumab, utomilumab, and ADG106, in comparison with CD137 ligand seem to follow a trend that mimics their functional activities.
The CD137 binding epitope may also affect the antibody’s Fc interactions with FcγRs. Such variations in epitope-oriented FcγRs engagement could further contribute to differences in the levels of agonist activity for different anti-CD137 agonistic mAbs. In addition, the difference in IgG isotypes, because of their importance in FcγRs, especially FcγRIIB-mediated crosslinking for agonistic activities, is also shown in Figure 1D, for urelumab in IgG4, utomilumab in IgG2, ADG106 in IgG4, and ADG206, a conditionally activatable ADG106 in Fc-enhanced IgG1 (more details in the following sections).
Moreover, the interactions between CD137 and CD137L not only trigger CD137-mediated costimulatory signaling, but a reverse signaling through CD137L may also be activated to regulate immune responses (12). Previous studies have indicated that blockade of CD137L reverse signaling promotes intra-tumoral differentiation of IFNγ-producing cytotoxic T cells, IL12-producing CD103+ DC, and type 1 tumor-associated macrophages to suppress tumor growth (13). Thus, the ligand-blocking anti-CD137 antibody could have additional pharmacological activities through inhibiting the CD137L-mediated reverse signaling. The ligand-blocking versus non-blocking properties of the agonist antibodies can also impact their activity in the presence and absence of CD137 ligand. For example, as a ligand non-blocking agonist, urelumab was demonstrated to be capable of inducing strong ligand-dependent CD137 clustering through cross-linking receptors trimerized by the ligand, whereas the ligand-blocking utomilumab failed to induce ligand-dependent clustering (10). Thus, the influence on ligand/receptor interaction could be a noteworthy property of an anti-CD137 agonist antibody when comprehensively evaluating its pharmacological activity.
Finally, we noted that CRD4 is tilted by Utomilumab-induced conformational change, while CRD4 is much more tilted by urelumab and ADG106, respectively, in comparison with the reference state by CD137L. This becomes quite obvious once we overlap their CD137 structures in complex with different agonist antibodies with that of CD137 (in green) in complex with CD137L based on the CRD1 and CRD2 as shown in Figure 1C.
Crosslinking by FcγR
In physiological conditions, CD137 costimulatory signaling activation requires clustering of the receptor through its natural ligand to form trimeric or larger lattice-shaped structures (14). Similarly, agonistic antibodies activate CD137 receptor signaling by inducing cluster formation at high receptor density (15). However, the crystal structure of utomilumab in complex with CD137 indicates that utomilumab binds to monomeric or dimeric CD137 to induce limited crosslinking of the receptor (16). This explains why the Fab binding to CD137 alone by many anti-CD137 monoclonal antibodies (mAbs) is not sufficient to induce CD137 receptor activation. Most anti-CD137 agonistic antibodies require engagement of FcγRs, particularly FcγRIIb, to mediate clustering and activation of CD137. Upon Fc binding to FcγRIIb, higher order CD137 receptor clusters can be achieved, which activates CD137-mediated downstream signaling (Figure 2). Certain antibodies, such as urelumab, can directly activate CD137 receptor independent of FcγR engagement. A study comparing the agonist activity of urelumab, utomilumab, and AD106 in the CD137 Jurkat NFκB reporter cell signaling assay in the presence and absence of FcγRIIb-expressing cells demonstrated that, in the presence of FcγRIIb-expressing cells, all three antibodies stimulate CD137 and downstream signaling, with urelumab showing more potent activity than utomilumab and ADG106. However, in the absence of FcγRIIb-expressing cells, both utomilumab and ADG106 are inactive, whereas urelumab is still capable of stimulating CD137 receptor activation (11). These results suggest that the binding epitope of urelumab may allow this antibody to cluster CD137 monomers or dimers more efficiently through bivalent binding than other anti-CD137 agonists. Nonetheless, engagement of FcγRs, particularly FcγRIIb, by Fc could further enhance the clustering effect (17) (18). FcγRIIb is expressed on many types of immune cells, including B cells, dendritic cells (DCs), monocytes/macrophages, mast cells, and basophils (19). Notably, FcγRIIb is expressed on Kupffer cells, the resident liver macrophages, and liver sinusoidal endothelial cells in the liver, where it plays an important role in immune complex clearance (20). This may significantly contribute to the super-clustering and hyperactivation of CD137 by anti-CD137 agonists on immune cells in the liver, leading to hepatic inflammation and liver toxicity, as exemplified by urelumab (8).
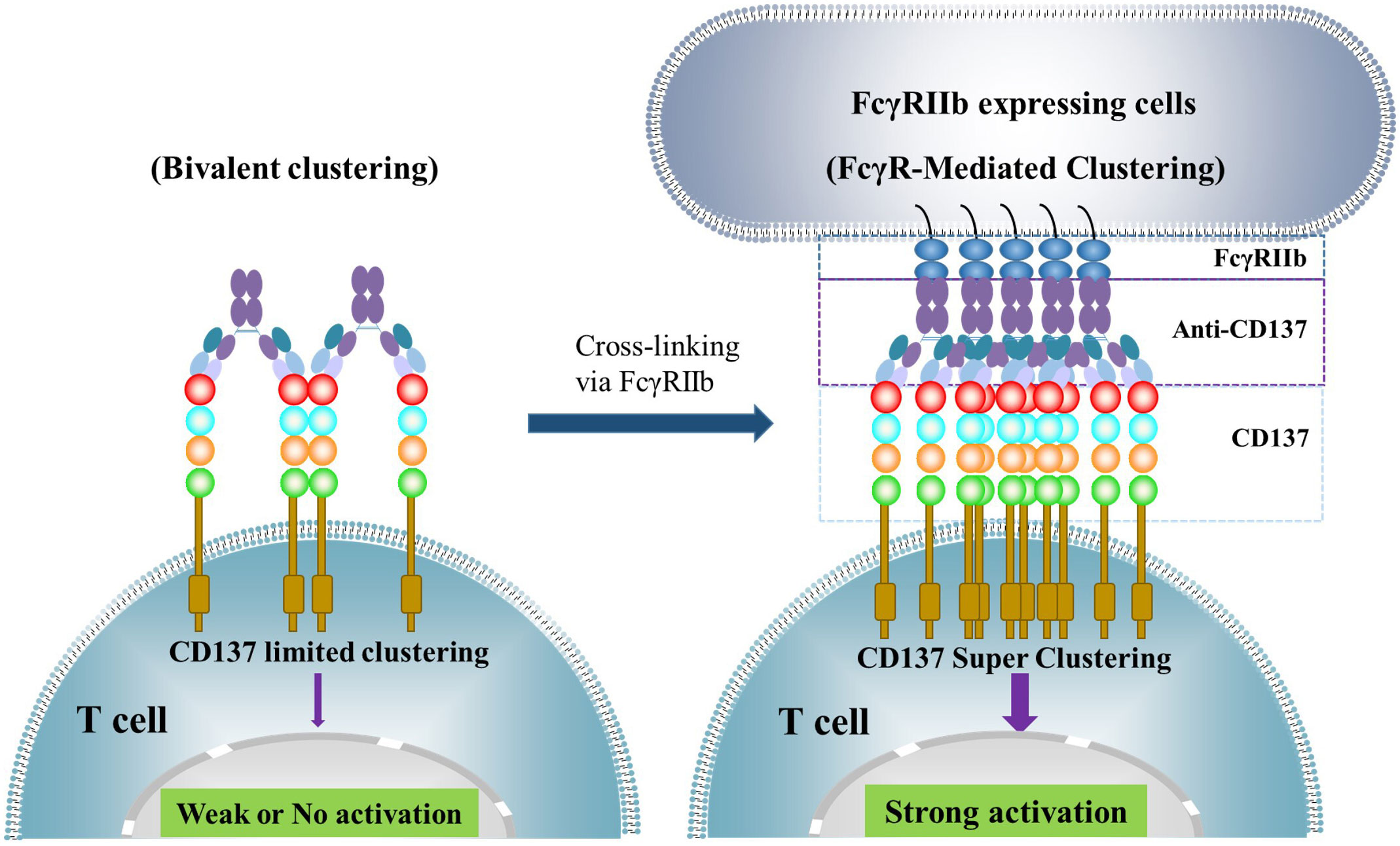
Figure 2 illustrates how the activation of CD137 receptor signaling is achieved through anti-CD137 agonistic monoclonal antibodies (mAbs). These antibodies bind to CD137 through their Fab region, inducing limited clustering of CD137 receptors and weak or no activation of downstream signaling. However, when the Fc region binds to FcγRs, particularly FcγRIIb, expressed on cells such as dendritic cells, macrophages, and B cells, they can induce CD137 receptor super-clustering and strong downstream signaling activation.
It has been shown that the non-blocking ligand urelumab increases the clustering of CD137 receptors on cells in a ligand-dependent manner (10). This may explain how urelumab stimulates CD137 receptor activation in the absence of FcγR engagement and cause severe toxicity, particularly liver damage, in patients. Conversely, the mild agonistic activity of utomilumab may account for its weak clinical efficacy in patients.
To strengthen the activity of CD137 agonist antibodies, enhancing the clustering of CD137 receptors has been a strategy, especially for those anti-CD137 mAbs with weaker agonist activity. Fc engineering can achieve this by enhancing crosslinking by FcγRIIb. Examples include LVGN6051 (21) in an IgG4 backbone and ADG206 (22) in an IgG1 backbone. ADG206 has shown four-fold higher cross-linking potency than urelumab in the functional assay, where urelumab is the most potent anti-CD137 agonist shown clinically.
Notably, CD137 is not only expressed in activated effector T cells but has also been identified as an activation marker for antigen-specific T regulatory (Treg) cells with immunosuppressive functions (23). CD137+ cells form a major part of functional tumor Tregs (24). In one preclinical study using mouse tumor models, two mechanisms were shown to promote robust tumor rejection: tumor Treg depletion and effector T cell agonism by anti-CD137 mAbs, which, however, are competitive and dependent on antibody isotype and FcγR availability. Administration of anti-CD137 mouse IgG2a, which preferentially depletes Treg cells, followed by either agonistic anti-CD137 mouse IgG1 or anti-PD-1 mAb augmented anti-tumor responses. An antibody engineered to optimize both FcγR-dependent Treg cell depleting capacity and FcγR-independent agonism delivered enhanced anti-tumor therapy (25). Although none of the current clinical stage anti-CD137 agonist antibodies developed so far demonstrated Treg depleting capacity, such mechanism can be provided by a Treg depleting antibody, such as anti-CTLA-4 mAb (26) (27), in a combination setting.
Binding affinity and agonism
High affinity is often the desired characteristic for therapeutic antibodies, particularly for antagonistic antibodies that can neutralize or inhibit target functions. However, for agonistic antibodies, this is not always the case. Epitope and Fc-mediated crosslinking, rather than high affinity, are critical for the antitumor activity of CD137 agonist antibodies with reduced liver toxicity (28). A recent study examined the relationship between affinity and function of immunomodulatory antibodies and found that reducing affinity can be a strategy to enhance immunomodulatory antibody agonism (29). Low rather than high affinity delivers greater activity through increased receptor clustering, independent of FcγRs. The study suggests that a faster dissociation rate, or higher off-rate rather than on-rate of the monoclonal antibody, is responsible for the increased agonistic activity of low-affinity variants. Additionally, an inert anti-CD137 mAb, utomilumab, can be transformed into an agonist. Low-affinity variants of the clinical antagonistic anti-PD-1 mAb, nivolumab, also mediated more potent signaling and affected T cell activation. Notably, low-affinity targeting by antibody binding is conducive to receptor activation but detrimental to Fc-mediated effector function, which could limit further enhanced receptor clustering through antibody engagement of FcγRs. Nonetheless, these findings provide a new avenue for agonistic antibody engineering (29).
Conditional activation
The specificity of T cell immune responses to an antigen comes from the recognition of the antigen peptide MHC complex by TCR, and not from costimulatory signaling. However, the use of CD137 agonists for cancer immunotherapy can potentially boost T cell responses triggered by any existing antigen MHC complex, including those involved in mediating autoimmune responses. This creates a dilemma between antitumor efficacy and autoimmune toxicity for costimulatory agonists with systemic agonistic activity, such as anti-CD137 agonist antibody. To address this issue, various strategies have been developed to create next-generation anti-CD137 agonists that target the agonistic activity more specifically to the tumor site while limiting the agonistic activity in normal tissues to reduce immune-toxicity (30). The primary solution is to exploit the differences between tumors and normal tissues to target tumors more specifically. Tumor-associated antigens (TAAs) are overexpressed in tumors compared to most normal tissues, and this can be leveraged to direct the antibody primarily to tumor tissues (Figure 3). Several TAAs, such as HER2 (31), FAP (32), EGFR (33) (34), Claudin18.2 (35), PSMA (36), Nectin-4 (37), Mesothelin (38), and B7-H3 (39), have been selected to construct bi- or tri-specific CD137 agonistic antibodies. These antibodies directly target the tumor-associated antigens through TAA-targeting arms and provide a costimulatory signal to enhance antitumor immune responses. The agonist activity of these constructs is dependent on TAA for crosslinking, thereby limiting immune activation primarily in tumor tissues with high levels of TAA expression. A comprehensive summary of the status of development and available clinical results of these TAA×CD137 constructs has been provided in recent reviews (40) (41).
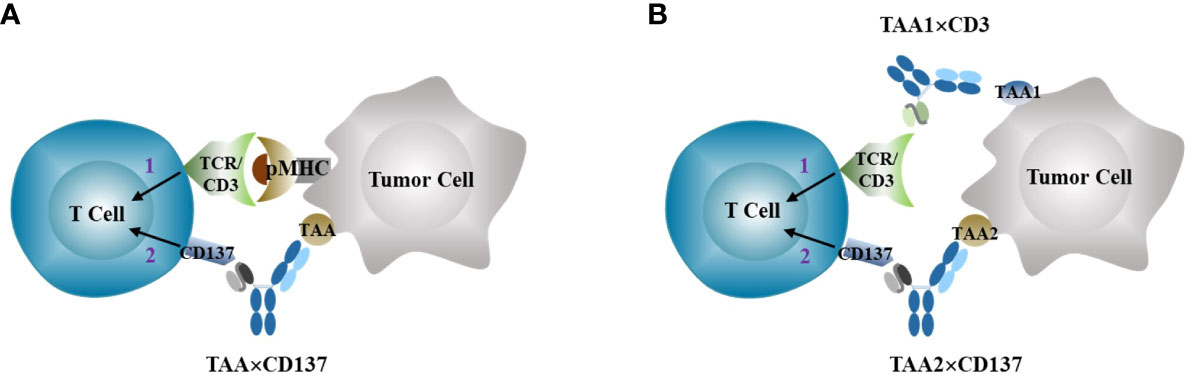
Figure 3 Anti-CD137 agonists (TAA×CD137 as example here) stimulates costimulatory signal 2 to enhance tumor-specific T cell activation primed by signal 1 through either neoantigen stimulated TCR activation (A), or bispecific TAA×CD3 T cell engagers (B).
Another major difference between tumors and normal tissues is that protease activity is generally up-regulated in tumor microenvironment through upregulation of protease expression, activation of zymogen, down-modulation of inhibitor expression, or a combination of these effects (42). Multiple extracellular proteases such as matrix metalloproteinases (MMPs), urokinase-type plasminogen activator (uPA), matriptase, etc. are widely overexpressed in different tumors but with limited expression in normal tissues. These proteases play important roles in many aspects of tumor biologic processes involved in tumor signaling, angiogenesis, tumor growth and metastasis (43). To take advantage of the rich protease activity in tumors, one strategy is to design a conditionally activatable agonist by blocking the antigen binding site of the antibody with a mask that is covalently attached to the antibody through a protease cleavable linker. In such antibody configuration, the masked agonist remains largely inactive in circulation and normal tissues. However, when in tumor microenvironment (TME), the masked antibody can be enriched by binding with upregulated antigen and then permanently activated through removal of masking peptides by rich proteases in the TME to expose the binding site of the antibody. This allows activation of the agonist preferentially in tumor (Figure 4). Such strategy has been tested successfully in both preclinical and clinical settings for anti-CTLA-4 antagonist antibody ADG126 (42). It has been shown, for example, a masked anti-murine CD137 agonist (Pb-Tx 1D8) has been generated with antitumor efficacy and reduced toxicity in mouse models (44). Recently, ADG206, a protease-activatable masked species cross-reactive anti-CD137 with enhanced Fc crosslinking (22), has entered clinical investigation (NCT05614258), which would provide direct assessment of the masking strategy in the costimulatory agonist therapeutics. Other approaches taking advantage of the differences between tumors and normal tissues to achieve preferential tumor activation have also been explored. For example, a conditional anti-CD137 agonistic antibody STA551 that binds and crosslink CD137 in ATP-rich condition in tumor microenvironment has been generated with improved safety in human CD137 knock-in mouse models (45).
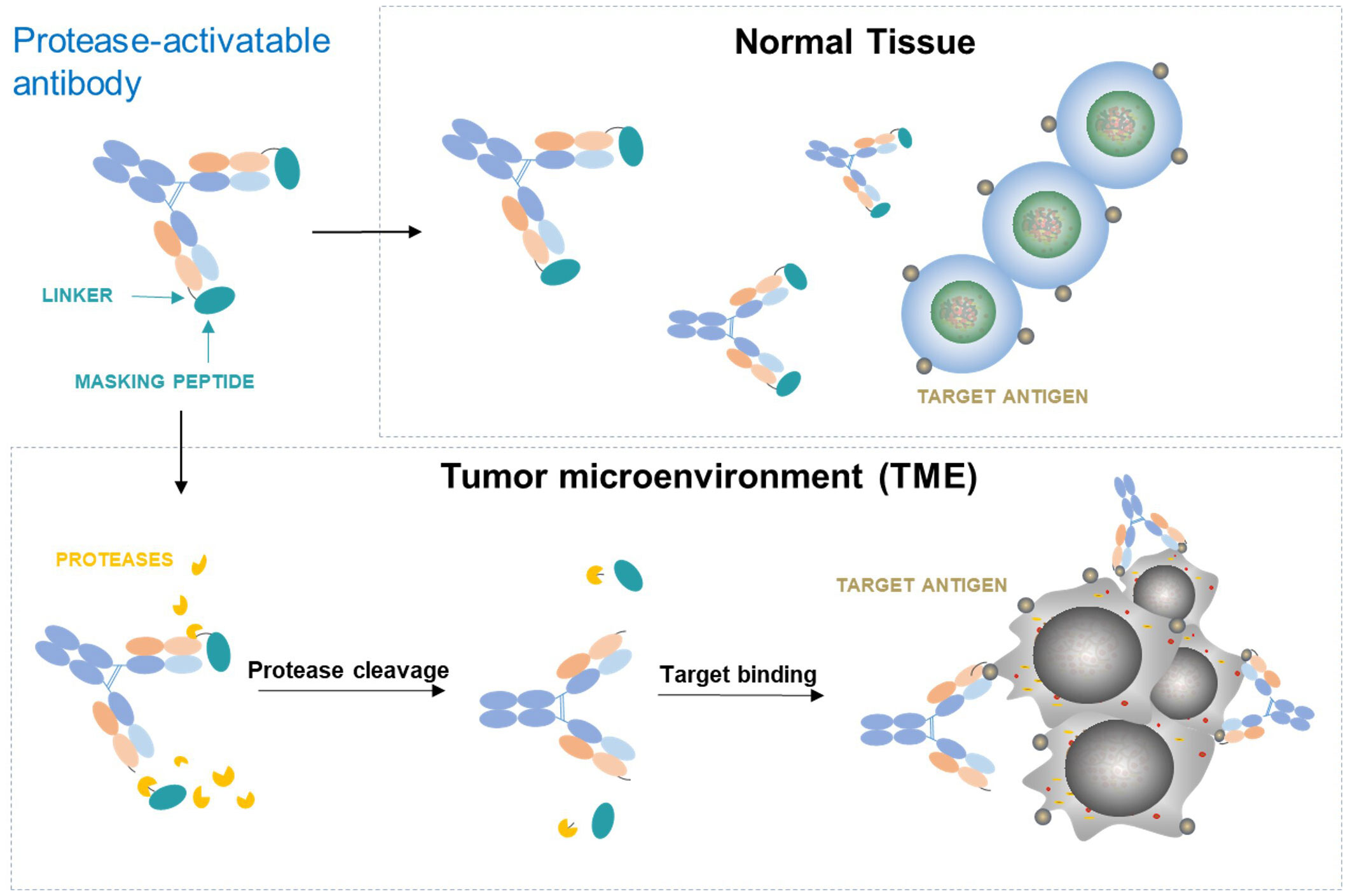
Figure 4 Conditionally activatable agonists controlled by antibody masking and protease-cleavable linker.
Combinations
To date, more than 20 different CD137-targeted agonistic antibodies have been developed and entered in clinical trials (40) (41). Although these trials have demonstrated various clinical responses, the overall activity in general is modest by monotherapy with these agonists. Combinations with other therapeutics are under testing, and anti-PD-1/L1 is the most common partner with the anti-CD137 agonists in the clinical investigations and improved therapeutic outcomes have been observed (3). In addition to the traditional drug-drug combinations, one other simple strategy for combination is to construct bispecific or multi-specific therapeutics, which sometimes may achieve greater activity than that of the individual drug-drug combinations. These bi- or multi-specifics targeting both CD137 and other immune-related pathways are under development and early clinical testing, such as CD137 with OX-40 (46), CD40 (47), PD-1 (48), PD-L1 (49–51), CD47 (52). Of note, various PD-L1 × CD137 bispecific antibodies have been developed. PD-L1 was used both to target PD-1 checkpoint pathway and agonistically crosslink CD137 in TME. Clinical results from the bispecific PD-L1 × CD137 antibody GEN1046 have shown signs of T cell activation and monotherapy antitumor activity in patients refractory to anti-PD-1 therapy, with 20% patients developing controllable abnormalities in liver function test (53).
The potential of the anti-CD137 and anti-CTLA-4 therapies to synergistically treat tumors has been well-established in preclinical models and confirmed by clinical trials using cross-reactive therapeutic antibodies against both targets. Combining these therapies is not only biologically compelling for their anti-tumor efficacy but also to reduce the toxicity of each monotherapy, making the anti-CD137/anti-CTLA-4 combination the top choice for clinical translation. Anti-CD137 agonists such as ADG106/206 and anti-CTLA-4 antagonists such as ADG116/ADG126, which are related to the CD137 and CTLA-4 pathways, have shown promise in preclinical studies and are being evaluated in clinical trials (11, 22, 54, 55).
Other therapeutics that can take advantage of the T cell costimulatory activity of CD137 agonists include vaccines, certain chemotherapies, and/or radiotherapy. Costimulatory signals are required for the generation of a robust and long-term T cell response, but the target specificity of this response is determined by the TCR/pMHC recognition, also known as the priming signal or Signal 1. Combining a cancer vaccine that can initiate the tumor-specific priming signal with the costimulatory agonists is a conceptually appealing approach to enhance the targeted antitumor immune response. This approach has been evaluated in mouse tumor models with promising results, showing that CD137 agonist antibodies have unique potential to promote durable regression of HPV+ tumors when combined with an E6/E7 peptide vaccine (56). Multiple personalized or neoantigen cancer vaccines have entered clinical investigations in combination with checkpoint inhibitors, mostly anti-PD-(L)1, to overcome resistance to immune checkpoint inhibitors (57, 58). RNA-based tumor vaccines in combination with checkpoint inhibitors have also demonstrated durable objective responses in anti-PD1 experienced patients with unresectable melanoma, accompanied by the induction of strong CD4+ and CD8+ T cell immunity against the vaccine antigens (59). It is yet to be seen but worth exploring the combination of cancer vaccines and anti-CD137 agonists in a clinical setting.
CD3 bispecific T cell engagers (TCE) can directly trigger Signal 1 and combining them with the anti-CD137 agonist as Signal 2 can overcome resistance to bispecific TCE treatment in T cell-cold solid tumors in preclinical animal tumor models (60), as conceptually illustrated in Figure 3B.
Translational research
Since Melero et al. first reported that agonist anti-CD137 monoclonal antibodies can eradicate transplanted mouse tumors through enhanced CD8+ T-cell antitumor immunity over two decades ago (61), extensive studies have been conducted to evaluate the antitumor activity, toxicity, and mechanisms of anti-CD137 agonists and their combinations with other agents using syngeneic mouse tumor models, which greatly facilitated our understanding of CD137-targeted immunotherapies (55). These studies either involved mouse CD137 surrogate antibodies in wild-type mouse background or human CD137 agonists but in human CD137 knock-in mouse background due to the lack of mouse cross-reactivity of many human CD137 agonist antibodies. One caveat is that the human CD137 knock-in mice may not fully recapitulate CD137 biology, as the mouse CD137 ligand does not bind to human CD137 (62). Interestingly, ADG106, as well as its conditionally activatable, Fc-enhanced form ADG206, bind to a conserved epitope of CD137 from human, monkey, and rodents, allowing direct assessment of their pharmacological/toxicological properties in wild-type mice, which may better mimic clinical conditions. In preclinical studies, ADG106 induces robust single-agent antitumor responses in multiple syngeneic tumor models and synergizes with anti-PD-(L)1 or anti-CTLA-4 checkpoint inhibitors, without showing significant toxicity (11) (22), which translated to patient studies. In clinical trials, ADG106 stimulates CD4+ and CD8+ T-cell and NK cell proliferation and proinflammatory interferon-gamma (IFN-γ) release while displaying a low risk for adverse immune responses. Notably, ADG106 treatment alone induces significant dose-dependent increases of soluble CD137, which is further enhanced when combined with anti-PD-1 antibody (63). Similar results were observed in other anti-CD137 studies and demonstrated that soluble CD137 can be a dynamic biomarker to monitor agonist CD137 immunotherapies (9) (64).
Concluding remarks
Current checkpoint blockade therapies have shown impressive benefits in cancer treatment, but only for a minority of patients. However, recent years have seen a shift towards exploring immuno-oncology agents beyond PD1/PDL1 inhibitors in clinical trials (65). There is a pressing need for agents that can further enhance T cell immunity for cancer treatment while also having improved safety profiles. Targeting CD137, a potent T cell costimulatory receptor, with agonist antibodies shows promise for cancer immunotherapy beyond its successful application in CAR T cells (66). It is encouraging to see that multiple strategies have been employed to develop the next generation of anti-CD137 agonists that have strong agonistic activity, increased tumor specificity, and reduced agonism in normal tissues to minimize immunotoxicity. Furthermore, the success of costimulatory CD137 agonists depends on their synergistic and rational combinations with other therapeutics or CD137-based bi- or multi-specific antibodies. With many of these new agents entering clinical investigations, the next few years hold great promise for CD137-targeted immunotherapies.
Author contributions
All authors listed have made a substantial, direct and intellectual contribution to the work, and approved it for publication.
Funding
This review is supported by Adagene internal funds. The funder was not involved in the study design, collection, analysis, interpretation of data, the writing of this article, or the decision to submit it for publication.
Acknowledgments
We want to thank Felix Du and Yan Li for the research in CD137 agonist antibody discovery, Zhining Wan for the help on making the figures and Ruby Dai for proof-reading the manuscript.
Conflict of interest
Both authors are employed by Adagene Inc. and have an ownership interest in Adagene.
Publisher’s note
All claims expressed in this article are solely those of the authors and do not necessarily represent those of their affiliated organizations, or those of the publisher, the editors and the reviewers. Any product that may be evaluated in this article, or claim that may be made by its manufacturer, is not guaranteed or endorsed by the publisher.
References
1. Chen DS, Mellman I. Oncology meets immunology: the cancer-immunity cycle. Immunity (2013) 39(1):1–10. doi: 10.1016/j.immuni.2013.07.012
2. Bretscher PA, two-step A. Two-signal model for the primary activation of precursor helper T cells. Proc Natl Acad Sci U.S.A. (1999) 96(1):185–90. doi: 10.1073/pnas.96.1.185
3. Chester C, Sanmamed MF, Wang J, Melero I. Immunotherapy targeting 4-1BB: mechanistic rationale, clinical results, and future strategies. Blood (2018) 131(1):49–57. doi: 10.1182/blood-2017-06-741041
4. Schwarz H, Valbracht J, Tuckwell J, von Kempis J, Lotz M. ILA, the human 4-1BB homologue, is inducible in lymphoid and other cell lineages. Blood (1995) 85(4):1043–52. doi: 10.1182/blood.V85.4.1043.bloodjournal8541043
5. Vinay DS, Kwon BS. Role of 4-1BB in immune responses. Semin Immunol (1998) 10(6):481–9. doi: 10.1006/smim.1998.0157
6. Lee DY, Choi BK, Lee DG, Kim YH, Kim CH, Lee SJ, et al. 4-1BB signaling activates the t cell factor 1 effector/beta-catenin pathway with delayed kinetics via ERK signaling and delayed PI3K/AKT activation to promote the proliferation of CD8+ T cells. PloS One (2013) 8(7):e69677. doi: 10.1371/journal.pone.0069677
7. Croft M. The role of TNF superfamily members in T-cell function and diseases. Nat Rev Immunol (2009) 9(4):271–85. doi: 10.1038/nri2526
8. Segal NH, Logan TF, Hodi FS, McDermott D, Melero I, Hamid O, et al. Results from an integrated safety analysis of urelumab, an agonist anti-CD137 monoclonal antibody. Clin Cancer Res (2017) 23(8):1929–36. doi: 10.1158/1078-0432.CCR-16-1272
9. Segal NH, He AR, Doi T, Levy R, Bhatia S, Pishvaian MJ, et al. Phase I study of single-agent utomilumab (PF-05082566), a 4-1BB/CD137 agonist, in patients with advanced cancer. Clin Cancer Res (2018) 24(8):1816–23. doi: 10.1158/1078-0432.CCR-17-1922
10. Chin SM, Kimberlin CR, Roe-Zurz Z, Zhang P, Xu A, Liao-Chan S, et al. Structure of the 4-1BB/4-1BBL complex and distinct binding and functional properties of utomilumab and urelumab. Nat Commun (2018) 9(1):4679. doi: 10.1038/s41467-018-07136-7
11. Liu G, Du F, She X, Zhu Y, Tolcher AW, Luo P. A safe and potent agonist ADG106 targeting a unique epitope of CD137 with novel mechanism of actions. Cancer Res (2020) 80(16_Supplement):4538. doi: 10.1158/1538-7445.AM2020-4538
12. Shao Z, Schwarz H. CD137 ligand, a member of the tumor necrosis factor family, regulates immune responses via reverse signal transduction. J Leukoc Biol (2011) 89(1):21–9. doi: 10.1189/jlb.0510315
13. Kang SW, Lee SC, Park SH, Kim J, Kim HH, Lee HW, et al. Anti-CD137 suppresses tumor growth by blocking reverse signaling by CD137 ligand. Cancer Res (2017) 77(21):5989–6000. doi: 10.1158/0008-5472.CAN-17-0610
14. Zapata JM, Lefebvre S, Reed JC. Targeting TRAfs for therapeutic intervention. Adv Exp Med Biol (2007) 597:188–201. doi: 10.1007/978-0-387-70630-6_15
15. Yu X, James S, Felce JH, Kellermayer B, Johnston DA, Chan HTC, et al. TNF receptor agonists induce distinct receptor clusters to mediate differential agonistic activity. Commun Biol (2021) 4(1):772. doi: 10.1038/s42003-021-02309-5
16. Li Y, Tan S, Zhang C, Chai Y, He M, Zhang CW, et al. Limited cross-linking of 4-1BB by 4-1BB ligand and the agonist monoclonal antibody utomilumab. Cell Rep (2018) 25(4):909–920 e4. doi: 10.1016/j.celrep.2018.09.073
17. White AL, Chan HT, French RR, Beers SA, Cragg MS, Johnson PW, et al. FcgammaRIotaIotaB controls the potency of agonistic anti-TNFR mAbs. Cancer Immunol Immunother (2013) 62(5):941–8. doi: 10.1007/s00262-013-1398-6
18. Li F, Ravetch JV. Antitumor activities of agonistic anti-TNFR antibodies require differential FcgammaRIIB coengagement in vivo. Proc Natl Acad Sci U.S.A. (2013) 110(48):19501–6. doi: 10.1073/pnas.1319502110
19. Smith KG, Clatworthy MR. FcgammaRIIB in autoimmunity and infection: evolutionary and therapeutic implications. Nat Rev Immunol (2010) 10(5):328–43. doi: 10.1038/nri2762
20. Ganesan LP, Kim J, Wu Y, Mohanty S, Phillips GS, Birmingham DJ, et al. FcgammaRIIb on liver sinusoidal endothelium clears small immune complexes. J Immunol (2012) 189(10):4981–8. doi: 10.4049/jimmunol.1202017
21. Qi X, Li F, Wu Y, Cheng C, Han P, Wang J, et al. Optimization of 4-1BB antibody for cancer immunotherapy by balancing agonistic strength with FcgammaR affinity. Nat Commun (2019) 10(1):2141. doi: 10.1038/s41467-019-10088-1
22. Liu G, Dai Z, Qu J, Shi J, Zhao Q, Qu L, et al. ADG206, an anti-CD137 agonistic POWERbody with tailor-made efficacy and safety profiles by strong crosslinking and tumor selective activation for single and combinational cancer immunotherapy. Cancer Res (2022) 82(12_Supplement):2868. doi: 10.1158/1538-7445.AM2022-2868
23. Bacher P, Heinrich F, Stervbo U, Nienen M, Vahldieck M, Iwert C, et al. Regulatory T cell specificity directs tolerance versus allergy against aeroantigens in humans. Cell (2016) 167(4):1067–1078 e16. doi: 10.1016/j.cell.2016.09.050
24. Guo X, Zhang Y, Zheng L, Zheng C, Song J, Zhang Q, et al. Global characterization of T cells in non-small-cell lung cancer by single-cell sequencing. Nat Med (2018) 24(7):978–85. doi: 10.1038/s41591-018-0045-3
25. Buchan SL, Dou L, Remer M, Booth SG, Dunn SN, Lai C, et al. Antibodies to costimulatory receptor 4-1BB enhance anti-tumor immunity via T regulatory cell depletion and promotion of CD8 T cell effector function. Immunity (2018) 49(5):958–970 e7. doi: 10.1016/j.immuni.2018.09.014
26. Arce Vargas F, Furness AJS, Litchfield K, Joshi K, Rosenthal R, Ghorani E, et al. Fc effector function contributes to the activity of human anti-CTLA-4 antibodies. Cancer Cell (2018) 33(4):649–663 e4. doi: 10.1016/j.ccell.2018.02.010
27. Ha D, Tanaka A, Kibayashi T, Tanemura A, Sugiyama D, Wing JB, et al. Differential control of human treg and effector T cells in tumor immunity by fc-engineered anti-CTLA-4 antibody. Proc Natl Acad Sci U.S.A. (2019) 116(2):609–18. doi: 10.1073/pnas.1812186116
28. Ho SK, Xu Z, Thakur A, Fox M, Tan SS, DiGiammarino E, et al. Epitope and fc-mediated cross-linking, but not high affinity, are critical for antitumor activity of CD137 agonist antibody with reduced liver toxicity. Mol Cancer Ther (2020) 19(4):1040–51. doi: 10.1158/1535-7163.MCT-19-0608
29. Yu X, Orr CM, Chan HTC, James S, Penfold CA, Kim J, et al. Reducing affinity as a strategy to boost immunomodulatory antibody agonism. Nature (2023) 614(7948):539–47. doi: 10.1038/s41586-022-05673-2
30. Su TT, Gao X, Wang J. A tumor-localized approach to bypass anti-4-1BB immuno-toxicity. Clin Cancer Res (2019) 25(19):5732–4. doi: 10.1158/1078-0432.CCR-19-1799
31. Hinner MJ, Aiba RSB, Jaquin TJ, Berger S, Durr MC, Schlosser C, et al. Tumor-localized costimulatory T-cell engagement by the 4-1BB/HER2 bispecific antibody-anticalin fusion PRS-343. Clin Cancer Res (2019) 25(19):5878–89. doi: 10.1158/1078-0432.CCR-18-3654
32. Claus C, Ferrara-Koller C, Klein C. Tumor-targeted 4-1BB agonists for combination with T cell bispecific antibodies as off-the-shelf therapy. Sci Transl Med (2019) 11(496):eaav5989. doi: 10.1126/scitranslmed.aav5989
33. Compte M, Harwood SL, Munoz IG, Navarro R, Zonca M, Perez-Chacon G, et al. A tumor-targeted trimeric 4-1BB-agonistic antibody induces potent anti-tumor immunity without systemic toxicity. Nat Commun (2018) 9(1):4809. doi: 10.1038/s41467-018-07195-w
34. Compte M, Harwood SL, Erce-LIamazares A, Tapia-Galisteo A, Romero E, Ferrer I, et al. An fc-free EGFR-specific 4-1BB-agonistic trimerbody displays broad antitumor activity in humanized murine cancer models without toxicity. Clin Cancer Res (2021) 27(11):3167–77. doi: 10.1158/1078-0432.CCR-20-4625
35. Jiang W, Wang Z, Sheng Z, Jung J, Guo T. TJ-CD4B (ABL111), a Claudin18.2-targeted 4-1BB tumor engager induces potent tumor-dependent immune response without dose-limiting toxicity in preclinical studies. J Immunother Cancer (2021) 9(Suppl 2):702. doi: 10.1136/jitc-2021-SITC2021.702
36. Legg JW. Tumor dependent co-stimulation of CD137/4-1BB in PSMA positive tumors: preclinical characterization of CB307, a half-life extended PSMAxCD137 bispecific humabody therapeutic. Cancer Res (2020) 80:3352. doi: 10.1158/1538-7445.AM2020-3352
37. Hurov K, Lahdenranta J, Upadhyaya P, Haines E, Cohen H, Repash E, et al. BT7480, a novel fully synthetic bicycle tumor-targeted immune cell agonist (Bicycle TICA) induces tumor localized CD137 agonism. J Immunother Cancer (2021) 9(11):e002883. doi: 10.1136/jitc-2021-002883
38. Xu C, Rabinovich B, Deshpande A, Zhou X, Pipp FC, Schweickhardt R, et al. M9657, a novel tumor-targeted conditional anti-CD137 agonist display MSLN-dependent anti-tumor immunity. J Immunother Cancer (2021) 9(Suppl 2):757. doi: 10.1136/jitc-2021-SITC2021.757
39. You G, Lee Y, Kang YW, Park HW, Park K, Kim H, et al. B7-H3x4-1BB bispecific antibody augments antitumor immunity by enhancing terminally differentiated CD8(+) tumor-infiltrating lymphocytes. Sci Adv (2021) 7(3):eaax3160. doi: 10.1126/sciadv.aax3160
40. Claus C, Ferrara-Koller C, Klein C. The emerging landscape of novel 4-1BB (CD137) agonistic drugs for cancer immunotherapy. MAbs (2023) 15(1):2167189. doi: 10.1080/19420862.2023.2167189
41. Melero I, Sanmamed MF, Glez-Vaz J, Luri-Rey C, Wang J, Chen L. CD137 (4-1BB)-Based cancer immunotherapy on its 25th anniversary. Cancer Discovery (2023) 13(3):552–69. doi: 10.1158/2159-8290.CD-22-1029
42. van Kempen LC, de Visser KE, Coussens LM. Inflammation, proteases and cancer. Eur J Cancer (2006) 42(6):728–34. doi: 10.1016/j.ejca.2006.01.004
43. Mason SD, Joyce JA. Proteolytic networks in cancer. Trends Cell Biol (2011) 21(4):228–37. doi: 10.1016/j.tcb.2010.12.002
44. Etxeberria I, Bolanos E, Teijeira A, Garasa S, Yanguas A, Azpilikueta A, et al. Antitumor efficacy and reduced toxicity using an anti-CD137 probody therapeutic. Proc Natl Acad Sci U.S.A. (2021) 118(26):e2025930118. doi: 10.1073/pnas.2025930118
45. Kamata-Sakurai M, Narita Y, Hori Y, Nemoto T, Uchikawa R, Honda M, et al. Antibody to CD137 activated by extracellular adenosine triphosphate is tumor selective and broadly effective In vivo without systemic immune activation. Cancer Discovery (2021) 11(1):158–75. doi: 10.1158/2159-8290.CD-20-0328
46. Gaspar M, Pravin J, Rodrigues L, Uhlenbroich S, Everett KL, Wollerton F, et al. CD137/OX40 bispecific antibody induces potent antitumor activity that is dependent on target coengagement. Cancer Immunol Res (2020) 8(6):781–93. doi: 10.1158/2326-6066.CIR-19-0798
47. Muik A, Adams HC 3rd, Gieseke F, Altintas I, Schoedel KB, Blum JM, et al. DuoBody-CD40x4-1BB induces dendritic-cell maturation and enhances T-cell activation through conditional CD40 and 4-1BB agonist activity. J Immunother Cancer (2022) 10(6):e004322. doi: 10.1136/jitc-2021-004322
48. Qiao Y, Qiu Y, Ding J, Luo N, Wang H, Ling X, et al. Cancer immune therapy with PD-1-dependent CD137 co-stimulation provides localized tumour killing without systemic toxicity. Nat Commun (2021) 12(1):6360. doi: 10.1038/s41467-021-26645-6
49. Lakins MA, Koers A, Giambalvo R, Munoz-Olaya J, Hughes R, Goodman E, et al. FS222, a CD137/PD-L1 tetravalent bispecific antibody, exhibits low toxicity and antitumor activity in colorectal cancer models. Clin Cancer Res (2020) 26(15):4154–67. doi: 10.1158/1078-0432.CCR-19-2958
50. Geuijen C, Tacken P, Wang LC, Klooster R, van Loo PF, Zhou J, et al. A human CD137xPD-L1 bispecific antibody promotes anti-tumor immunity via context-dependent T cell costimulation and checkpoint blockade. Nat Commun (2021) 12(1):4445. doi: 10.1038/s41467-021-24767-5
51. Peper-Gabriel JK, Pavlidou M, Pattarini L, Morales-Kastresana A, Jaquin TJ, Gallou C, et al. The PD-L1/4-1BB bispecific antibody-anticalin fusion protein PRS-344/S095012 elicits strong T-cell stimulation in a tumor-localized manner. Clin Cancer Res (2022) 28(15):3387–99. doi: 10.1158/1078-0432.CCR-21-2762
52. Cendrowicz E, Jacob L, Greenwald S, Tamir A, Pecker I, Tabakman R, et al. DSP107 combines inhibition of CD47/SIRPalpha axis with activation of 4-1BB to trigger anticancer immunity. J Exp Clin Cancer Res (2022) 41(1):97. doi: 10.1186/s13046-022-02256-x
53. Muik A, Garralda E, Altintas I, Gieseke F, Geva R, Ben-Ami E, et al. Preclinical characterization and phase I trial results of a bispecific antibody targeting PD-L1 and 4-1BB (GEN1046) in patients with advanced refractory solid tumors. Cancer Discovery (2022) 12(5):1248–65. doi: 10.1158/2159-8290.CD-21-1345
54. Kocak E, Lute K, Chang X, May KF, Exten KR, Zhang H, et al. Combination therapy with anti-CTL antigen-4 and anti-4-1BB antibodies enhances cancer immunity and reduces autoimmunity. Cancer Res (2006) 66(14):7276–84. doi: 10.1158/0008-5472.CAN-05-2128
55. Bartkowiak T, Curran MA. 4-1BB agonists: multi-potent potentiators of tumor immunity. Front Oncol (2015) 5:117. doi: 10.3389/fonc.2015.00117
56. Bartkowiak T, Singh S, Yang G, Galvan G, Haria D, Ai M, et al. Unique potential of 4-1BB agonist antibody to promote durable regression of HPV+ tumors when combined with an E6/E7 peptide vaccine. Proc Natl Acad Sci U.S.A. (2015) 112(38):E5290–9. doi: 10.1073/pnas.1514418112
57. Curran MA, Glisson BS. New hope for therapeutic cancer vaccines in the era of immune checkpoint modulation. Annu Rev Med (2019) 70:409–24. doi: 10.1146/annurev-med-050217-121900
58. Liao JY, Zhang S. Safety and efficacy of personalized cancer vaccines in combination with immune checkpoint inhibitors in cancer treatment. Front Oncol (2021) 11:663264. doi: 10.3389/fonc.2021.663264
59. Sahin U, Oehm P, Derhovanessian E, Jabulowsky RA, Vormehr M, Gold M, et al. An RNA vaccine drives immunity in checkpoint-inhibitor-treated melanoma. Nature (2020) 585(7823):107–12. doi: 10.1038/s41586-020-2537-9
60. Belmontes B, Sawant DV, Zhong W, Tan H, Kaul A, Aeffner F, et al. Immunotherapy combinations overcome resistance to bispecific T cell engager treatment in T cell-cold solid tumors. Sci Transl Med (2021) 13(608):eabd1524. doi: 10.1126/scitranslmed.abd1524
61. Melero I, Shuford WW, Newby SA, Aruffo A, Ledbetter JA, Hellstrom KE, et al. Monoclonal antibodies against the 4-1BB T-cell activation molecule eradicate established tumors. Nat Med (1997) 3(6):682–5. doi: 10.1038/nm0697-682
62. Yi L, Zhao Y, Wang X, Dai M, Hellstrom KE, Hellstrom I, et al. Human and mouse CD137 have predominantly different binding CRDs to their respective ligands. PloS One (2014) 9(1):e86337. doi: 10.1371/journal.pone.0086337
63. Zhao H, Zheng S, Liu G, Ma Y, She X, Chen M, et al. Assessment of biomarker kinetics for ADG106 (anti-CD137 agonist) as monotherapy or combined with toripalimab. Ann Oncol (2021) 32:S1389–90. doi: 10.1016/j.annonc.2021.10.059
64. Glez-Vaz J, Azpilikueta A, Olivera I, Cirella A, Teijeira A, Ochoa MC, et al. Soluble CD137 as a dynamic biomarker to monitor agonist CD137 immunotherapies. J Immunother Cancer (2022) 10(3):e003532. doi: 10.1136/jitc-2021-003532
65. Saez-Ibanez AR, Upadhaya S, Campbell J. Immuno-oncology clinical trials take a turn beyond PD1/PDL1 inhibitors. Nat Rev Drug Discovery (2023) 22. doi: 10.1038/d41573-023-00066-0
Keywords: TNFR agonist, CD137/4-1BB, FcγR mediated cross-linking, conditional activation, cancer immunotherapy, costimulatory receptor
Citation: Liu G and Luo P (2023) Targeting CD137 (4-1BB) towards improved safety and efficacy for cancer immunotherapy. Front. Immunol. 14:1208788. doi: 10.3389/fimmu.2023.1208788
Received: 19 April 2023; Accepted: 22 May 2023;
Published: 02 June 2023.
Edited by:
Harald Wajant, University Hospital Würzburg, GermanyReviewed by:
Shahram Salek-Ardakani, Pfizer, United StatesSandra Stephanie Diebold, King’s College London, United Kingdom
Copyright © 2023 Liu and Luo. This is an open-access article distributed under the terms of the Creative Commons Attribution License (CC BY). The use, distribution or reproduction in other forums is permitted, provided the original author(s) and the copyright owner(s) are credited and that the original publication in this journal is cited, in accordance with accepted academic practice. No use, distribution or reproduction is permitted which does not comply with these terms.
*Correspondence: Peter Luo, Peter_luo@adagene.com