- Institut National de la Santé et de la Recherche Médicale (INSERM) UMR 1186, Integrative Tumor Immunology and Immunotherapy, Gustave Roussy, Fac. de Médecine - Univ. Paris-Sud, Université Paris-Saclay, Villejuif, France
Tumor-infiltrating lymphocytes (TIL) often include a substantial subset of CD8+ tissue-resident memory T (TRM) cells enriched in tumor-specific T cells. These TRM cells play a major role in antitumor immune response. They are identified on the basis of their expression of the CD103 (αE(CD103)β7) and/or CD49a (α1(CD49a)β1) integrins, and the C-type lectin CD69, which are involved in tissue residency. TRM cells express several T-cell inhibitory receptors on their surface but they nevertheless react strongly to malignant cells, exerting a strong cytotoxic function, particularly in the context of blocking interactions of PD-1 with PD-L1 on target cells. These TRM cells form stable conjugates with autologous tumor cells and interact with dendritic cells and other T cells within the tumor microenvironment to orchestrate an optimal in situ T-cell response. There is growing evidence to indicate that TGF-β is essential for the formation and maintenance of TRM cells in the tumor, through the induction of CD103 expression on activated CD8+ T cells, and for the regulation of TRM effector functions through bidirectional integrin signaling. CD8+ TRM cells were initially described as a prognostic marker for survival in patients with various types of cancer, including ovarian, lung and breast cancers and melanoma. More recently, these tumor-resident CD8+ T cells have been shown to be a potent predictive biomarker of the response of cancer patients to immunotherapies, including therapeutic cancer vaccines and immune checkpoint blockade. In this review, we will highlight the major characteristics of tumor TRM cell populations and the possibilities for their exploitation in the design of more effective immunotherapy strategies for cancer.
1 Introduction
The memory T-cell population includes central memory T (TCM) cells, which reside in lymphoid organs and can be reactivated by secondary infection with the same pathogen, and effector memory T (TEM) cells, with cytotoxic properties for CD8+ T lymphocytes, that patrol lymphoid and non-lymphoid peripheral tissues. More recently, a third subset of memory T cells, tissue-resident memory T (TRM) cells, was identified in peripheral tissues and intestine grafts (1, 2). In mice, this population of memory T cells was characterized in grafts, but not in peripheral blood, and seemed to be tissue-restricted (1, 2). Tissue transplant and parabiosis experiments have shown that the principal property of TRM cells is their residence in peripheral tissues, with an inability to recirculate in the bloodstream (1–7). TRM cells have been described in diverse human and mouse peripheral tissues, including the tissues of the intestine (2, 8), brain (9), skin (1, 10) and lung (11), in which they can confer rapid and effective immune responses to reinfections (12). TRM cells are characterized principally by surface expression of the CD103 (αE(CD103)β7) and CD49a (VLA-1 or α1β1) integrins, and the C-type lectin CD69, although phenotype and integrin expression have been observed to differ between tissues (13, 14).
A subset of tumor-infiltrating lymphocytes (TIL) with several properties in common with TRM, including the expression of the CD103 integrin and CD69, has recently been identified in human tumors, including ovarian, lung and breast tumors, and melanoma (15–19). These CD103+CD8+ T cells accumulate in tumor regions, where they can interact with target cells to trigger their effector functions. An intra-epithelial location of CD103+CD8+ T cells was also observed in colorectal and bladder tumors with or without the expression of E-cadherin, the ligand of CD103, on cancer cells (20, 21). A high density of CD8+ TRM cells within the tumor was found to be associated with better patient survival in multiple cancers, including melanoma, glioma, non-small cell lung carcinoma (NSCLC), and ovarian, bladder and breast cancers (15, 16, 18, 20–25). Abundant tumor infiltration with CD8+ TRM cells is also associated with a stronger response of cancer patients to immunotherapies, including immune checkpoint blockade (ICB) and therapeutic cancer vaccines (17, 25, 26). In this review, we will summarize recent findings regarding the phenotypic and functional features of tumor TRM cells and their role in antitumor immunity and response in cancer immunotherapies.
2 Characterization of TRM cells in solid tumors
2.1 CD8+ TRM cells
CD8+ TRM cells can be identified on the basis of their surface expression of particular adhesion molecules and activation receptors. The receptors expressed on CD8+ TRM cells include the CD69 lectin, which is known to bind to S1PR1, inhibiting its function and, thus, the egress of T cells from the tissue; it therefore contributes to the residency of these cells in the tissue (27). CD69 is not a specific marker of TRM; it is expressed on activated T-cells (28, 29) and on other types of memory T cells (30, 31). CD69 expression alone is not, therefore, sufficient to define TRM cells. The expression of several integrins, particularly CD103 (αEβ7) and CD49a (α1β1), has been reported to identify TRM cells and to participate in the residency of these cells in peripheral tissues.
2.1.1 The CD103 integrin, a key molecule for TRM identification and functions
The CD103 integrin has two major functions on CD8+ TRM cells: it is involved in the retention of T cells within epithelial tissues and it acts as a T-cell receptor (TCR) costimulatory molecule. Indeed, the binding of CD103 to its ligand, E-cadherin, contributes to the accumulation and maintenance of TRM within tissues (9, 32–35). CD8+ TRM cells can also express E-cadherin, which contributes to their own retention within tissues (36). CD103 neutralization decreases T-cell infiltration into tumors and aggravates tumor progression (37–40). CD103 also provides CD8+ TRM cells with a TCR costimulation signal, and its interaction with E-cadherin strengthens adhesion to cancer cells and triggers the relocalization of cytotoxic granules to the immune synapse and target cell lysis (41–44). We have previously shown that the adhesion of CD103 to E-cadherin results in the binding of phosphorylated paxillin to the CD103 intracytoplasmic domain, initiating an outside-in signaling by CD103, thereby promoting CD8+ TRM migration toward tumor cells and triggering T-cell effector functions (45).
2.1.2 The CD49a integrin and other markers of CD8+ TRM cells
Much less is known about the contribution of the CD49a integrin to TRM cell functions. The binding of CD49a to collagen IV induces a relocalization of the integrin to focal adhesion points and increases T-cell anchorage to the extracellular matrix (ECM) (46). CD49a has been reported to promote T-cell retention in tissues, including those of the lungs (47), skin (14) and liver (48).
Another marker of CD8+ TRM cells, CXCR6, has been reported to promote T-cell homing to the tissues and to regulate TRM cell formation and maintenance (49, 50). Other chemokine receptors, such as CCR5 and CXCR3, are also expressed by tumor CD8+ TRM cells and are probably involved in the residency of these cells in the inflammatory tumor microenvironment (16–18, 51, 52).
2.1.3 Transcription factors involved in TRM cell differention and persistence
TRM cells undergo a specific differentiation program, distinct from that of memory T-cell lineage, such as by downregulating eomesodermin (EOMES) and T-cell factor 1 (TCF1) (53, 54). In mice, the expression of both Hobit and Blimp-1 transcription factors is required to generate and maintain TRM cells in tissues (55). Notch and Runx3 have been reported to play an important role in TRM cell differentiation in tumor microenvironments in both humans and mice, and in infectious contexts (35, 56). We have previously reported that the NFAT and SMAD signaling pathways triggered by the concomitant engagement of TCR and TGFBR, cooperate to induce CD103 expression in tumor-specific T lymphocytes and in the formation of CD8+ TRM cells in a TGF-β-rich tumor microenvironment (43, 44, 51). Human NSCLC CD8+ TRM cells display a particular molecular signature, with the expression of transcription factors specific to resident T cells, including NF-κB, BATF (basic leucine zipper transcription factor), Aiolos and AHR (aryl hydrocarbon receptor), which are probably involved in the maintenance and effector functions of these lymphocytes (17, 18). T-bet downregulation is required for the formation of memory T cells in mouse models, but residual T-bet is required to promote IL-15R signaling, thereby favoring the long-term survival of TRM cells in peripheral tissues (57).
2.2 CD4+ TRM cells
The identification of human CD4+ TRM cells has remained challenging, partly due to the presence of functionally different helper T-cell (TH) subpopulations and their plasticity, making it difficult to identify a common CD4+ T-cell memory precursor (58, 59). Nevertheless, CD4+ TRM cells have been characterized in both mouse and human lymph nodes, salivary glands, skin, gut, spleen, brain and lungs, mostly in infectious contexts (60–65). Human CD4+ TRM cells have several transcriptional and phenotypic features in common with CD8+ TRM. Indeed, CD4+ TRM cells express the CD69 lectin and have an effector-cell memory phenotype, with a CD45 RA- CCR7- and CD62L- profile at diverse anatomic sites, including the spleen, lymph nodes, lungs, tonsils, salivary glands and intestine (61, 64, 66, 67).
Experiments in various infectious models have shown that CD4+ TRM cells play a protective role. In this context, the adoptive transfer of lung CD4+ TRM cells provides better protection against influenza infection than CD4+ circulating memory T cells (68, 69). In Mycobacterium tuberculosis infection, CD4+ TRM cells migrate to the lung parenchyma more strongly than circulating memory T-cells, resulting in a greater reduction of bacterial load (70). In Leishmania major infections, CD4+ TRM cells also play an important role in improving the effector functions of innate and adaptive immune cells and recruiting these cell populations to infected sites (71).
2.2.1 Phenotype of CD4+ TRM cells
The phenotypic and functional characterization of CD4+ TRM cells was first described in infectious diseases. CD103 expression on CD4+ T cells differs between tissues, and CD103+CD4+ TRM cells are mostly found in the skin, gut and lungs (61). Human dermis contains CD4+ memory T cells that do not express CD103, but the CD4+ memory T cells in the epidermis are CD103+ (63). Other key molecules involved in the tissue residency of CD4+ T cells include the CD49a integrin, which is present on CD4+ TRM cells in normal brain tissues and lung tumors, and the CXCR6 chemokine receptor (61, 66, 67, 72). CD4+ TRM cells also strongly express the CXCR3 and CCR5 chemokine receptors, which promote T-cell recruitment to inflamed tissues (73, 74). During influenza virus infection, CCR5 accelerates the recruitment of memory CD8+ T cells to the lung airways, and CCR5 deficiency in infected mice results in a decrease in TRM cell recruitment and, thus, impaired control over viral replication during subsequent rechallenge (75). CD4+ TRM cells display heterogeneous phenotypes according to their tissue residency, but additional markers of this TRM-cell subset need to be identified.
CD103+CD4+ T lymphocytes infiltrating human lung tumors express multiple inhibitory receptors, such as PD-1, CTLA-4 and 2B4 (CD244), suggesting that they are chronically stimulated and potentially exhausted (72). However, upon stimulation with anti-CD3 and anti-CD28 antibodies, these cells are able to secrete IFN-γ, TNF-α and IL-2 more efficiently than other TIL populations. CD103+CD4+ TIL also display increases in the levels of transcripts encoding inflammatory chemokines, such as CCL3, CCL4 and CCL5, and cytotoxic molecules, including IFNγ, granzyme A and granzyme B. These data suggest that the response to antigenic stimulation is rapid, despite the strong expression of inhibitory receptors (72).
2.2.2 Cytokines and transcription factors involved in CD4+ TRM cell formation and maintenance
Following the transfer of antigen-primed T cells into influenza-infected mice, IL-2 neutralization greatly decreases CD4+ TRM cell formation, and a residual memory population is maintained independently of IL-2 signaling (68). Blockade with anti-IL-15 receptor antibodies results in a defect of memory cell recovery after infection, with no impact on the accumulation of effector T cells in the lungs, suggesting that the maintenance of CD4+ TRM cells requires IL-15 (68). Moreover, the administration of anti-TGF-β neutralizing antibodies has no effect on the generation of CD4+ TRM cells in the lungs. Overall, these studies suggest that the mechanisms by which CD4+ TRM cells differentiate are different from those of CD8+ TRM cells, particularly in terms of the need for TGF-β (68).
Transcriptional analyses investigating the transcription factors involved in CD4+ TRM cell differentiation revealed an upregulation of the ERG2, EPAS1, BATF and IRF4 genes in lung tumor CD4+ TRM cells (66, 72). Increases in PRDM1 (encoding the transcription factor Blimp-1) and ZNF683 (encoding Hobit) transcript levels were also observed in CD4+ TRM cells from lung tumors, with no increase in the level of Hobit protein (66, 72). The genes encoding the proteins of the Notch pathway are also upregulated in CD4+ TRM cells, with transcript levels highest for NOCTH1, RBPJ and JAG2 (66). However, the roles of these transcription factors in CD4+ TRM cell formation and maintenance remain to be elucidated. Further studies are required to improve our understanding of the regulatory pathway and mechanisms of differentiation of CD4+ TRM cells, particularly in tumor settings.
3 TGF-β, a master regulator for CD8+ TRM cell differentiation
It is now widely accepted that TGF-β is required for the differentiation of CD8+ TRM cells in infectious diseases and cancers (8, 35, 76–78). The TGF-β-dependent differentiation into CD8+ TRM cells of CD8+ T lymphocytes from various tissues, including those of the intestine, skin and lungs, has been described (8, 79, 80). This process involves a decrease in the levels of Eomes and Tbet, which is essential for the differentiation of TRM cells (57). TGF-β is known for its immunosuppressive effects and its role in T-cell exclusion from the tumor microenvironment. However, TGF-β is also a key regulator of CD103 expression in TCR-engaged CD8+ T cells and is involved in the activation of this integrin in CD8+ TRM cells (41, 43, 44). Indeed, CD103 is induced in tumor-reactive T lymphocytes following TCR engagement with specific peptide-major histocompatibility complex (MHC)-class I complexes on cancer cells in a tumor microenvironment containing mature TGF-β. TGF-β is also involved in CD103 activation through the induction of integrin-linked kinase (ILK) phosphorylation. This leads to AKT phosphorylation and the initiation of integrin inside-out signaling, resulting in an increase in the affinity of CD103 for its ligand, E-cadherin (81). The purinergic receptor P2RX7 is required for TRM cell sensitivity to TGF-β, and defects of P2RX7 in T cells result in a transient decrease in the expression of TGFBR2 and CD103, and weaker TGF-β signaling (77). The treatment of activated CD8+ T cells with a pharmacological agonist of P2RX7 leads to increases in Itgae and Tgfbr2 mRNA levels, suggesting that P2RX7 signaling improves the response of CD8+ T cells to TGF-β, thereby promoting CD8+ TRM cell differentiation (77).
TGF-β is secreted in a latent, inactive form bound to latency-associated protein (LAP) and its activation is mediated by the cleavage of LAP by metalloproteinases and αvβ6 and αvβ8 integrins. These integrins are expressed by keratinocytes, and defects of αvβ6 or αvβ8 decrease the amount of mature TGF-β, in turn leading to a decrease in CD103 expression and TRM density in the tissue (82). The specific inhibition of αvβ6 integrin after TRM establishment significantly decreases the number of these cells in epithelial tissues, with no consequences for their frequency in lymphoid organs and blood, providing further support for the conclusion that TGF-β is essential for the development and maintenance of TRM cells (82). Cancer cells also express αv integrins. They are therefore able to activate TGF-β and, thus, to promote in situ CD8+ TRM cell differentiation and CD103 induction in CD8 T cells (83). In a mouse model based on the tamoxifen-inducible ablation of Tgfbr2 in CD8+ T cells, only small numbers of CD8+ TRM cells were detected in the skin, demonstrating the dependence of epidermal TRM cells on an autocrine source of TGF-β (78). Moreover, virus-specific TRM cells are more likely to persist in the epidermis than bystander TRM cells when small amounts of active TGF-β are present in the skin (78). Overall, the differentiation of TRM cells is orchestrated by antigen-dependent TCR activation and the Smad signaling pathway mediated by TGF-β, which is also required for the long-term maintenance of TRM cells in tissues (Figure 1).
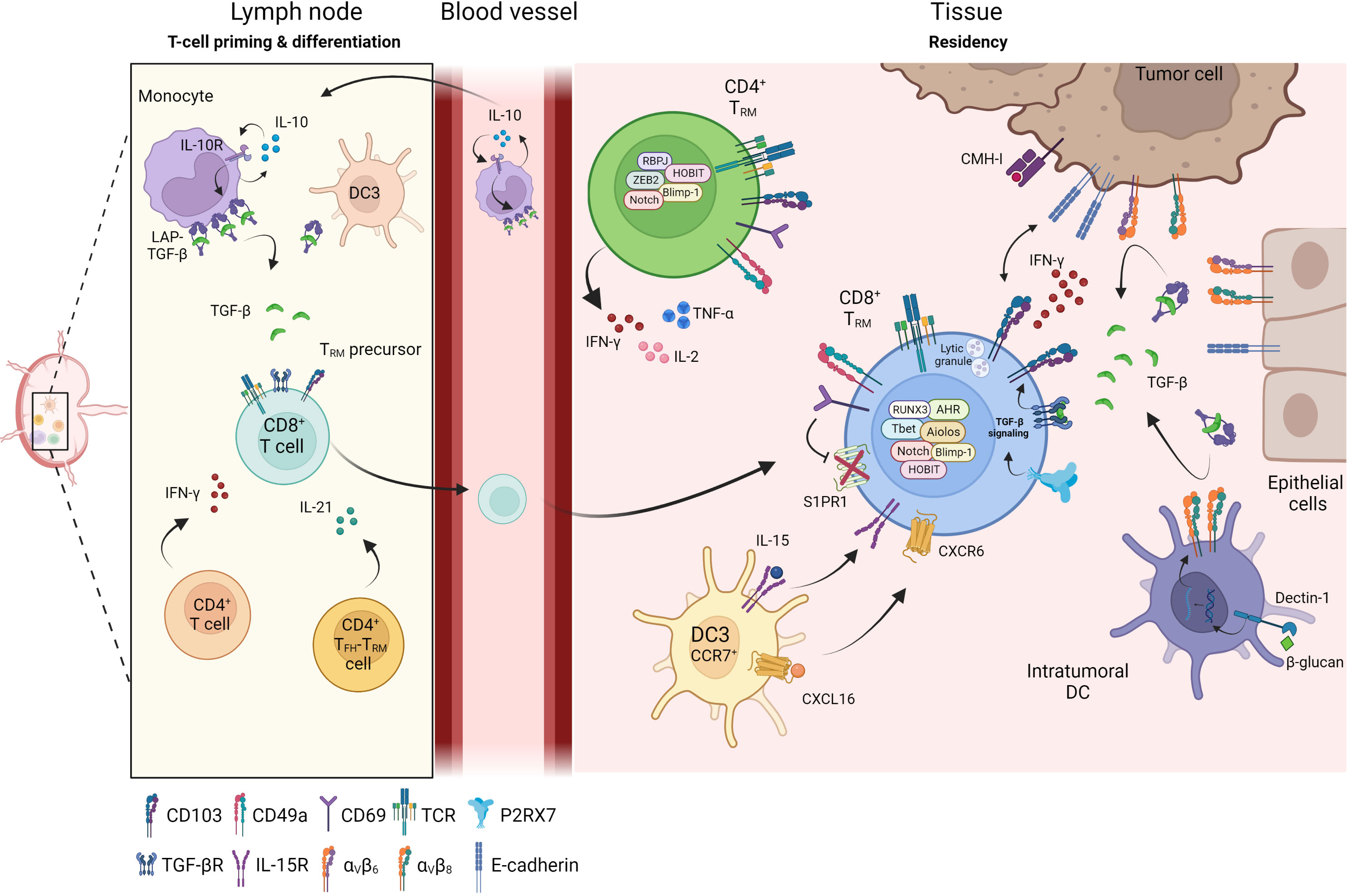
Figure 1 Differentiation of TRM in tissue. Priming and differentiation of naïve CD8+ T cells take place in the lymph node in contact with monocytes or dendritic cells (DC3) which promote the generation of CD8+ TRM precursors. Through cytokines secretion such as IFN-γ or IL-21, CD4+ T cells help CD8+ T cells to be fully functional and influence the TRM differentiation. Primed CD8+ TRM precursor cells reach the inflamed sites where they differentiate into CD8+ TRM. Within the tissue, several cellular components regulate and maintain TRM cells: DC3 participate in positioning CD8+ TRM cells in an immune niche and promote their survival, DC infiltrating tumors, tumor cells or epithelial cells express integrin αvβ6 and αvβ8 and transform LAP-TGF-β to active TGF-β. Active TGF-β maintains tissue residence of TRM cells and initiates CD103 signaling leading to functional and cytotoxic TRM cells. CD4+ TRM cells have recently been identified and are also present in peripheral tissues and solid tumors where they secrete inflammatory cytokines such as IFN-γ, TNF-α or IL-2.
4 The interactome of TRM cells
4.1 Interactome with tumor cells
In NSCLC, CD103+CD8+ TRM cells migrate toward epithelial tumor regions more rapidly than effector T cells, which are retained in the stroma (16, 17, 25, 81). Most of the CD103+ cells in the tumor microenvironment are CD8 T cells, whereas most of the CD8+ TIL located in the stroma do not express this integrin (16, 25). This capacity of TRM cells to migrate to the tumor islets is mediated by CD103 and enhanced by the presence of TGF-β (81). An accumulation of CD103+ TRM cells in tumor epithelial regions has also been observed in ovarian and urothelial cell carcinomas and in endometrial adenocarcinoma (15, 21, 84).
The recognition by the TCR of specific antigenic peptides presented by MHC-class I complexes on tumor cells is the first step in the engagement of a target-directed T-cell response. Following this first signal, a second signal is required for the full activation of CD8 T cells and to trigger cytotoxic functions. In CD8+ TRM cells, CD103 acts as a costimulatory molecule that binds to E-cadherin, initiating an outside-in signal that promotes T-cell effector functions (43, 45). Moreover, CD103 is a key molecule for triggering TRM cell cytotoxic activity following interaction with E-cadherin on target cells (41). CD103 is recruited to the immunological synapse formed between T cells and autologous tumor cells, where it promotes polarization and the exocytosis of cytotoxic granules, leading to the lysis of target cells (42) (Figure 1). Tumor CD8+ TRM cells are enriched in antigen-specific T cells, which are activated upon coculture with autologous tumor cells and can kill tumor targets (16, 17, 29). Cytotoxic activity toward malignant cells is mediated by the TCR and dependent on CD103 expression, as anti-CD103 neutralizing antibodies block CD103-E-cadherin interaction and inhibit target cell lysis (16, 17, 43). Remarkably, the loss of E-cadherin during epithelial-to-mesenchymal transition corresponds to a mechanism used by cancer stem-cell (CSC)-like cells to evade CD103+CD8+ TRM cell-mediated recognition and destruction (85).
4.2 Interactome with dendritic cells
Dendritic cells (DC) are important regulators of the immune response through their interaction with T lymphocytes and their orchestration of T-cell activation via the presentation of antigenic peptides and production of specific cytokines and chemokines. In lung tissues, CD103+ DC trigger the upregulation of CD103 on activated CD8+ T cells in a TGF-β-dependent manner (76). In a virus model, DNGR-1+ (Clec9a) DC-mediated cross-presentation is essential for the optimal priming of TRM cells through the production of cytokines such as IL-12 and IL-15 (86). Furthermore, mice in which αv integrin expression by DC is defective, have lower levels of CD8+CD103+CD69+ T cells in the epidermis (87). Migratory DC expressing αVβ8 have also been shown to be involved in TRM cell formation by supplying TGF-β to naïve CD8+ T cells in the lymph nodes (87). A population of circulating CD88-CD1c+CD163+ DC (called DC3), the precursors of inflammatory DC, was recently reported to drive the activation of naïve T cells and their differentiation into CD103+CD8+ T cells expressing TRM cell markers, such as the NUSAP1, DUSP4, CXCR6 and FASLG genes, in a TGF-β-dependent manner (88). In the context of HIV vaccination, IL-10-secreting monocytes potentiate TGF-β production, leading to the generation of CD8+ TRM cell precursors in non-human primates and mice (89).
A similar DC3-induced CD103+ T-cell gene signature has been identified in breast and lung cancers (19, 52, 61). In breast cancer, the intratumoral administration of the β-glucan curdlan, a ligand of dectin-1, reprograms tumor-infiltrating DC, favoring the generation of Th1 cells with a higher proportion of CD8+CD103+ T cells in tumors; this effect is abolished by the neutralization of TGF-β or inhibition of αvβ8 integrin (90). CXCR6 regulates the accumulation and persistence of effector CD8+ T cells by supporting their survival in the tumor tissue (91). This chemokine receptor can position CD8+ cytotoxic T lymphocytes (CTL) in perivascular niches in the tumor stroma, which is enriched in DC3 (CCR7+ DC) expressing the ligand of CXCR6, CXCL16. DC3 also trans-present IL-15 to tumor-resident CD8+ T cells to maintain their survival in intratumoral areas (91). In a mouse melanoma model, CXCR6 expression has been shown to result in the presence of tumor-specific CD8+ TRM cells within CXCL16+ DC clusters in the skin, ensuring the persistence and functioning of these cells (92). Several types of cells, including epithelial cells (such as keratinocytes), lung tumor cells (82, 93), and immune cells, such as DC (91, 92) and T cells (66), can secrete or present CXCL16 at their membrane. In head-and-neck tumors, intranasal immunization induces CXCL16 production in broncho-alveolar lavage (BAL) and pulmonary parenchyma, and is associated with the formation of tumor-specific TRM cells (93). CXCR6 deficiency in T cells decreases the efficacy of anti-cancer vaccines and recruitment of CD8+ TRM cells to tumors (50). The CXCR6-CXCL16 axis has also been shown to be crucial for the recruitment and maintenance of TRM cells in the airways (50). DC and tumor cells play important roles in TRM cell differentiation (Figure 1), but additional cellular and soluble factors promoting the formation, survival and localization of TRM remain to be identified.
4.3 Interactome with CD4+ T cells
CD4+ T cells play an important role in CD8+ T-cell priming through the licensing of DC and the generation of a chemokine gradient within the draining lymph node. In infectious diseases, CD4+ T cells are required for the formation and function of memory T cells, and to confer cytotoxic and migratory capacities in T cells (94, 95). In the influenza infection model, CD4 T-cell depletion has no impact on the expansion and migration of memory CD8+ T cells into the lung parenchyma, but it does impair the expression of CD103 and CD69 on these cells in the tissue (96). Un-helped memory CD8+ T cells with low levels of CD103 expression are associated with changes in the ability of T cells to localize to the airway epithelium. By contrast, CD8+ T cells with CD4 T-cell help are associated with the recruitment of larger numbers of virus-specific CD8+ T cells and the expression of granzyme B upon heterosubtypic rechallenge (96). CD4+ T cells control CD103+CD8+ TRM cell development and localization in the airway through IFN-γ signaling, and they also regulate the degree of exposure of CD8+ T cells to chemokines and cytokines, such as TGF-β, which regulate the expression of CD103.
The specific depletion of lung CD4+ follicular helper (TFH) TRM-like cells, which strongly express TFH-associated genes, including Il21, Tox2, and Pdcd1, results in a decrease in influenza virus-specific CD8+ TRM cells (97). Late depletion, at the memory stage, of CD4+ TRM cells decreases CD8+ TRM cell responses, suggesting that lung CD4+ TRM cell continuous help is required for the maintenance of CD8+ TRM cells. This help is mediated by IL-21, as the administration of anti-αIL-21R antibodies after infection decreases antigen-specific CD8+ TRM responses. Overall, these studies support the involvement of CD4+ T cells, and more specifically of CD4+ TRM cells, in the development of CD8+ TRM cells during infectious diseases. However, the help that these cells provide for tumoral CD8+ TRM cell formation and function requires further investigation.
5 Functions of TRM cells and their role in antitumor immune responses
TRM cells are highly heterogeneous, differing considerably between tissues and diseases, and either within tissues (98, 99). There is a tremendous diversity in the levels of CD69, CD49a and CD103 expression observed, which can affect TRM cell functions, including proliferation and the production of cytokines, such as TGF-β, type 1 interferon (IFN) and IL-12 (100–102). In lung tumors and the skin, a proportion of CD8+ TRM cells displaying strong CD49a and CD103 expression can produce Th1 cytokines, such as IFN-γ and TNF-α, and IL-17, suggesting a particular function in the control of tumor progression (17, 103). The binding of CD49a to collagen IV has been reported to increase IFN-γ and TNF-α production (104). The capacity of TRM cells to secrete IFN-γ and TNF-α provides evidence for a key role of these cells in antitumor immune responses and in the control of tumor progression in melanoma (105). In B16-Ova-engrafted mice, the blockade of VLA-1 (CD49a/CD29) or CD103 alters tumor growth control (38). CD49a and CD103 are important integrins for TRM cell activation, migration and cytotoxic function within tumors.
Tumor CD8+ TRM cells express a range of inhibitory receptors, such as PD-1, CTLA-4, and TIM-3, together with co-activation molecules, such as 4-1BB (16–19). The co-expression of PD-1 and 4-1BB by CD8+ TIL in lung tumors is associated with a higher density of TIL (18). Despite the expression of these inhibitory receptors, which suggests an overlapping phenotype with exhausted T cells, TRM cells upregulate TCR activation genes (NR4A1, TNFRSF9 [4-1BB], CD69), secrete more TNF-α and IFN-γ, and are more cytotoxic than other CD8+ TIL (16–19, 26). This gene signature is associated with higher levels of proliferation and inflammatory cytokine production by CD8+ TRM in early-stage NSCLC (106). In head-and-neck (29) and lung (17) cancers, CD8+CD103+ TIL also strongly express the CD39 ectonuclease. CD8+ TIL co-expressing CD103 and CD39 are tumor-reactive and have a resident memory profile (18, 29, 107), with a restricted TCR repertoire (17, 18, 29). CD103+CD8+ TIL produce large amounts of cytotoxic molecules, such as granzyme B, and can kill autologous tumor cells (16, 17, 19, 26). These TRM cell features may account for the better overall survival (OS) observed in patients with tumors displaying high levels of CD8+CD103+ TIL infiltration (Table 1). In tumors, TRM cells and exhausted T cells display similar phenotypes and anti-tumor effector functions following anti-PD-1 treatment (16, 17, 26). However, transcriptional and functional studies of tumor TRM cells revealed specific differentiation program and molecular features distinct from non-TRM cells (17).
6 Relevance of TRM cells for cancer immunotherapy
6.1 TRM cells as a biomarker of the response to therapeutic cancer vaccines
The role of TRM cells in immune responses to tumors makes them particularly relevant as potential biomarkers of responses to cancer immunotherapies. In melanoma patients treated with the Melan-A peptide vaccine, circulating VLA-1-expressing Melan-A-specific CD8+ T cell numbers are correlated with better OS (38). Intranasal STxB-E7 vaccination has a protective effect in a mouse orthotopic head-and-neck tumor model, and this effect is suppressed by the in vivo blockade of CD49a, through decreases in CD8+ TIL densities (39). Moreover, the in vivo blockade of TGF-β results in decreases in the number of tumoral CD8+ TRM cells and the benefits of vaccination (25). In a similar model, the combination of an intramuscular vaccine targeting HPV-E6 and -E7 with irradiation triggered the formation of larger numbers of CD103+ cells in TC1 tumors than either of these therapies used alone (110). In the mouse B16F10-Ova melanoma model, intradermal vaccination induces a stronger accumulation of CD8+CD69+CD103+ TRM cells than intraperitoneal immunization and results in stronger protective effects due to the local protective effect of TRM cells (121). Local mucosal vaccination therefore promotes the formation of TRM cells, which can be used as a biomarker of the response to the cancer vaccine and of an optimal immune response.
6.2 TRM cells as a biomarker of the response to immune checkpoint inhibitors
Inhibitory receptors have been reported to inhibit the effector functions of TRM cells during long-term exposure to the antigen (122, 123). Tumor TRM cells express a wide range of inhibitory receptors (16–18, 35, 124). Immune checkpoint inhibitors (ICI) would therefore be expected to restore, or boost the functional activities of TRM cells. In NSCLC and hepatocellular carcinoma (HCC), the inhibition of PD-1 on tumor CD103+CD8+ T cells stimulated with anti-CD3 plus anti-CD28 antibodies enhances IFN-γ production by these cells (115, 125) (Table 2). Blockade of the PD-1-PD-L1 interaction also increases the cytotoxicity of CD8+ TRM cells toward tumor cells (16). In a cohort of NSCLC patients treated with anti-PD-(L)-1, tumors with high levels of CD103+CD8+ TIL had the best responses to immunotherapy (17) (Table 2). The use of TRM cells as a predictive biomarker of the response to immunotherapies has been also documented in urothelial cancer and NSCLC patients harboring tumors with high levels of ITGAE transcripts treated with anti-PD-L1 (126). This finding supports the conclusion that CD8+ TRM cells can be used as a biomarker of the response to ICI. In addition, low levels of αv integrin in tumor cells are associated with higher levels of TRM cell infiltration into lung tumors and a better response to anti-PD-(L)1 (83).
Remarkably, for patients treated with immunotherapy classified as “responders”, the administration of ICI increases the infiltration of CD103+CD8+ T cells into the tumor in NSCLC and melanoma (17, 117). This finding suggests that PD-1 blockade has an impact on TRM cells, but it remains unclear if it directly affects intratumoral TRM cells or has a more indirect effect on the newly recruited T cells that differentiate into TRM cells within the tumor. In a PDX model of gastric adenocarcinoma, anti-PD-L1 therapy results in an increase in the proportion of TRM cells among total CD8+ T cells (112). In an esophageal squamous cell carcinoma mouse model, PD-1 blockade induces an increase in the size of both the CD8+ and CD103+ cell populations (111). The proliferation and differentiation of stem-like TCF1+PD-1med TIL are also important mechanisms involved in the response to ICI and therapeutic vaccination (128, 129). Deeper investigations of the role of TRM cells in the response to cancer immunotherapies will help to improve both treatments and the management of patients.
7 Conclusion
The differentiation and maintenance of CD8+ TRM cells across tissues are orchestrated by diverse cellular interactions, with immune cells, stromal cells or tumoral cells, and by soluble factors, including cytokines and chemokines, which are produced by these cells within the tumor microenvironment. In normal epithelial tissues and solid tumors, TGF-β is a key cytokine involved in the regulation of CD8+ TRM cell formation and function. However, it remains unclear whether other signals are required for TRM cell differentiation and persistence. Similar, many questions remain unanswered concerning the cytokines and signaling pathways regulating CD4+ TRM cell development and maintenance in the tumor. CD8+ TRM cells are emerging as a reliable biomarker of the response to cancer immunotherapies. However, even though TRM cells are enriched in tumor-specific T cells endowed with cytotoxic functions, additional features of TRM cells influencing the efficacy of cancer therapies remain to be characterized. Improvements in our understanding of the mechanisms involved in the differentiation and survival of TRM cells within the tumor microenvironment would lead to the identification of promising novel targets for optimizing the efficacy of current cancer immunotherapies.
Author contributions
ID, TT, FM-C and SC wrote, reviewed and/or revised the manuscript. All authors contributed to the article and approved the submitted version.
Funding
This work was supported by grants from the French “Institut National du Cancer” (INCa; Grant number 070), ARCPGA2020. SC and ID are supported by grants from “Association pour la Recherche sur le Cancer” (ARC, SIGN’IT20181007792). The funders did not play any role in the decision to write or the writing and finalization of the manuscript.
Conflict of interest
The authors declare that the research was conducted in the absence of any commercial or financial relationships that could be construed as a potential conflict of interest.
Publisher’s note
All claims expressed in this article are solely those of the authors and do not necessarily represent those of their affiliated organizations, or those of the publisher, the editors and the reviewers. Any product that may be evaluated in this article, or claim that may be made by its manufacturer, is not guaranteed or endorsed by the publisher.
Abbreviations
AHR, Aryl hydrocarbon receptor; BAL, broncho-alveolar lavage; CSC, cancer stem-cell; DC, dendritic cell; ECM, extracellular matrix; ICB, immune checkpoint blockade; IFN, interferon; ILK, integrin-linked kinase; LAP, latency-associated protein; MHC, major histocompatibility complex; NSCLC, non-small cell lung cancer; OS, overall survival; S1PR1, Sphingosine-1-phosphate receptor 1; TCM, Central memory T cells; TCR, T cell receptor; TEM, Effector memory T cells; TFH; follicular helper T cells; TGF-β, transforming growth factor-β; TIL, Tumor-infiltrating lymphocytes; TRM, resident memory T cells; VLA-1, very late antigen-1.
References
1. Gebhardt T, Wakim LM, Eidsmo L, Reading PC, Heath WR, Carbone FR. Memory T cells in nonlymphoid tissue that provide enhanced local immunity during infection with herpes simplex virus. Nat Immunol (2009) 10(5):524–30. doi: 10.1038/ni.1718
2. Masopust D, Choo D, Vezys V, Wherry EJ, Duraiswamy J, Akondy R, et al. Dynamic T cell migration program provides resident memory within intestinal epithelium. J Exp Med (2010) 207(3):553–64. doi: 10.1084/jem.20090858
3. Hogan RJ, Usherwood EJ, Zhong W, Roberts AA, Dutton RW, Harmsen AG, et al. Activated antigen-specific CD8+ T cells persist in the lungs following recovery from respiratory virus infections. J Immunol (2001) 166(3):1813–22. doi: 10.4049/jimmunol.166.3.1813
4. Wakim LM, Waithman J, van Rooijen N, Heath WR, Carbone FR. Dendritic cell-induced memory T cell activation in nonlymphoid tissues. Sci (New York NY). (2008) 319(5860):198–202. doi: 10.1126/science.1151869
5. Woodberry T, Suscovich TJ, Henry LM, August M, Waring MT, Kaur A, et al. Alpha e beta 7 (CD103) expression identifies a highly active, tonsil-resident effector-memory CTL population. J Immunol (2005) 175(7):4355–62. doi: 10.4049/jimmunol.175.7.4355
6. Clark RA, Watanabe R, Teague JE, Schlapbach C, Tawa MC, Adams N, et al. Skin effector memory T cells do not recirculate and provide immune protection in alemtuzumab-treated CTCL patients. Sci Transl Med (2012) 4(117):117ra7. doi: 10.1126/scitranslmed.3003008
7. Steinert EM, Schenkel JM, Fraser KA, Beura LK, Manlove LS, Igyártó BZ, et al. Quantifying memory CD8 T cells reveals regionalization of immunosurveillance. Cell (2015) 161(4):737–49. doi: 10.1016/j.cell.2015.03.031
8. Zhang N, Bevan MJ. Transforming growth factor-beta signaling controls the formation and maintenance of gut-resident memory T cells by regulating migration and retention. Immunity (2013) 39(4):687–96. doi: 10.1016/j.immuni.2013.08.019
9. Wakim LM, Woodward-Davis A, Bevan MJ. Memory T cells persisting within the brain after local infection show functional adaptations to their tissue of residence. Proc Natl Acad Sci USA (2010) 107(42):17872–9. doi: 10.1073/pnas.1010201107
10. Jiang X, Clark RA, Liu L, Wagers AJ, Fuhlbrigge RC, Kupper TS. Skin infection generates non-migratory memory CD8+ T(RM) cells providing global skin immunity. Nature (2012) 483(7388):227–31. doi: 10.1038/nature10851
11. Piet B, de Bree GJ, Smids-Dierdorp BS, van der Loos CM, Remmerswaal EB, von der Thusen JH, et al. CD8(+) T cells with an intraepithelial phenotype upregulate cytotoxic function upon influenza infection in human lung. J Clin Invest (2011) 121(6):2254–63. doi: 10.1172/JCI44675
12. Mackay LK, Wakim L, van Vliet CJ, Jones CM, Mueller SN, Bannard O, et al. Maintenance of T cell function in the face of chronic antigen stimulation and repeated reactivation for a latent virus infection. J Immunol (2012) 188(5):2173–8. doi: 10.4049/jimmunol.1102719
13. Bergsbaken T, Bevan MJ. Proinflammatory microenvironments within the intestine regulate the differentiation of tissue-resident CD8(+) T cells responding to infection. Nat Immunol (2015) 16(4):406–14. doi: 10.1038/ni.3108
14. Bromley SK, Akbaba H, Mani V, Mora-Buch R, Chasse AY, Sama A, et al. CD49a regulates cutaneous resident memory CD8(+) T cell persistence and response. Cell Rep (2020) 32(9):108085. doi: 10.1016/j.celrep.2020.108085
15. Webb JR, Milne K, Watson P, Deleeuw RJ, Nelson BH. Tumor-infiltrating lymphocytes expressing the tissue resident memory marker CD103 are associated with increased survival in high-grade serous ovarian cancer. Clin Cancer research: an Off J Am Assoc Cancer Res (2014) 20(2):434–44. doi: 10.1158/1078-0432.CCR-13-1877
16. Djenidi F, Adam J, Goubar A, Durgeau A, Meurice G, de Montpreville V, et al. CD8+CD103+ tumor-infiltrating lymphocytes are tumor-specific tissue-resident memory T cells and a prognostic factor for survival in lung cancer patients. J Immunol (2015) 194(7):3475–86. doi: 10.4049/jimmunol.1402711
17. Corgnac S, Malenica I, Mezquita L, Auclin E, Voilin E, Kacher J, et al. CD103(+)CD8(+) T(RM) cells accumulate in tumors of anti-PD-1-Responder lung cancer patients and are tumor-reactive lymphocytes enriched with Tc17. Cell Rep Med (2020) 1(7):100127. doi: 10.1016/j.xcrm.2020.100127
18. Ganesan AP, Clarke J, Wood O, Garrido-Martin EM, Chee SJ, Mellows T, et al. Tissue-resident memory features are linked to the magnitude of cytotoxic T cell responses in human lung cancer. Nat Immunol (2017) 18(8):940–50. doi: 10.1038/ni.3775
19. Savas P, Virassamy B, Ye C, Salim A, Mintoff CP, Caramia F, et al. Single-cell profiling of breast cancer T cells reveals a tissue-resident memory subset associated with improved prognosis. Nat Med (2018) 24(7):986–93. doi: 10.1038/s41591-018-0078-7
20. Quinn E, Hawkins N, Yip YL, Suter C, Ward R. CD103+ intraepithelial lymphocytes–a unique population in microsatellite unstable sporadic colorectal cancer. Eur J Cancer (Oxford England: 1990) (2003) 39(4):469–75. doi: 10.1016/S0959-8049(02)00633-0
21. Wang B, Wu S, Zeng H, Liu Z, Dong W, He W, et al. CD103+ tumor infiltrating lymphocytes predict a favorable prognosis in urothelial cell carcinoma of the bladder. J Urol (2015) 194(2):556–62. doi: 10.1016/j.juro.2015.02.2941
22. Koh J, Kim S, Kim MY, Go H, Jeon YK, Chung DH. Prognostic implications of intratumoral CD103+ tumor-infiltrating lymphocytes in pulmonary squamous cell carcinoma. Oncotarget (2017) 8(8):13762–9. doi: 10.18632/oncotarget.14632
23. Jouanneau E, Black KL, Veiga L, Cordner R, Goverdhana S, Zhai Y, et al. Intrinsically de-sialylated CD103(+) CD8 T cells mediate beneficial anti-glioma immune responses. Cancer Immunol Immunother (2014) 63(9):911–24. doi: 10.1007/s00262-014-1559-2
24. Webb JR, Milne K, Nelson BH. PD-1 and CD103 are widely coexpressed on prognostically favorable intraepithelial CD8 T cells in human ovarian cancer. Cancer Immunol Res (2015) 3(8):926–35. doi: 10.1158/2326-6066.CIR-14-0239
25. Nizard M, Roussel H, Diniz MO, Karaki S, Tran T, Voron T, et al. Induction of resident memory T cells enhances the efficacy of cancer vaccine. Nat Commun (2017) 8:15221. doi: 10.1038/ncomms15221
26. Clarke J, Panwar B, Madrigal A, Singh D, Gujar R, Wood O, et al. Single-cell transcriptomic analysis of tissue-resident memory T cells in human lung cancer. J Exp Med (2019) 216(9):2128–49. doi: 10.1084/jem.20190249
27. Mackay LK, Braun A, Macleod BL, Collins N, Tebartz C, Bedoui S, et al. Cutting edge: CD69 interference with sphingosine-1-phosphate receptor function regulates peripheral T cell retention. J Immunol (2015) 194(5):2059–63. doi: 10.4049/jimmunol.1402256
28. Beura LK, Anderson KG, Schenkel JM, Locquiao JJ, Fraser KA, Vezys V, et al. Lymphocytic choriomeningitis virus persistence promotes effector-like memory differentiation and enhances mucosal T cell distribution. J Leukocyte Biol (2015) 97(2):217–25. doi: 10.1189/jlb.1HI0314-154R
29. Duhen T, Duhen R, Montler R, Moses J, Moudgil T, de Miranda NF, et al. Co-Expression of CD39 and CD103 identifies tumor-reactive CD8 T cells in human solid tumors. Nat Commun (2018) 9(1):2724. doi: 10.1038/s41467-018-05072-0
30. Gonzalez-Amaro R, Cortes JR, Sanchez-Madrid F, Martin P. Is CD69 an effective brake to control inflammatory diseases? Trends Mol Med (2013) 19(10):625–32. doi: 10.1016/j.molmed.2013.07.006
31. Okhrimenko A, Grun JR, Westendorf K, Fang Z, Reinke S, von Roth P, et al. Human memory T cells from the bone marrow are resting and maintain long-lasting systemic memory. Proc Natl Acad Sci USA (2014) 111(25):9229–34. doi: 10.1073/pnas.1318731111
32. Sheridan BS, Pham QM, Lee YT, Cauley LS, Puddington L, Lefrancois L. Oral infection drives a distinct population of intestinal resident memory CD8(+) T cells with enhanced protective function. Immunity (2014) 40(5):747–57. doi: 10.1016/j.immuni.2014.03.007
33. Casey KA, Fraser KA, Schenkel JM, Moran A, Abt MC, Beura LK, et al. Antigen-independent differentiation and maintenance of effector-like resident memory T cells in tissues. J Immunol (2012) 188(10):4866–75. doi: 10.4049/jimmunol.1200402
34. Lee YT, Suarez-Ramirez JE, Wu T, Redman JM, Bouchard K, Hadley GA, et al. Environmental and antigen receptor-derived signals support sustained surveillance of the lungs by pathogen-specific cytotoxic T lymphocytes. J Virol (2011) 85(9):4085–94. doi: 10.1128/JVI.02493-10
35. Mackay LK, Rahimpour A, Ma JZ, Collins N, Stock AT, Hafon ML, et al. The developmental pathway for CD103(+)CD8+ tissue-resident memory T cells of skin. Nat Immunol (2013) 14(12):1294–301. doi: 10.1038/ni.2744
36. Hofmann M, Pircher H. E-cadherin promotes accumulation of a unique memory CD8 T-cell population in murine salivary glands. Proc Natl Acad Sci USA (2011) 108(40):16741–6. doi: 10.1073/pnas.1107200108
37. Malik BT, Byrne KT, Vella JL, Zhang P, Shabaneh TB, Steinberg SM, et al. Resident memory T cells in the skin mediate durable immunity to melanoma. Sci Immunol (2017) 2(10). doi: 10.1126/sciimmunol.aam6346
38. Murray T, Fuertes Marraco SA, Baumgaertner P, Bordry N, Cagnon L, Donda A, et al. Very late antigen-1 marks functional tumor-resident CD8 T cells and correlates with survival of melanoma patients. Front Immunol (2016) 7:573. doi: 10.3389/fimmu.2016.00573
39. Sandoval F, Terme M, Nizard M, Badoual C, Bureau MF, Freyburger L, et al. Mucosal imprinting of vaccine-induced CD8(+) T cells is crucial to inhibit the growth of mucosal tumors. Sci Transl Med (2013) 5(172):172ra20. doi: 10.1126/scitranslmed.3004888
40. Sun YY, Peng S, Han L, Qiu J, Song L, Tsai Y, et al. Local HPV recombinant vaccinia boost following priming with an HPV DNA vaccine enhances local HPV-specific CD8+ T-cell-Mediated tumor control in the genital tract. Clin Cancer research: an Off J Am Assoc Cancer Res (2016) 22(3):657–69. doi: 10.1158/1078-0432.CCR-15-0234
41. Le Floc’h A, Jalil A, Vergnon I, Le Maux Chansac B, Lazar V, Bismuth G, et al. Alpha e beta 7 integrin interaction with e-cadherin promotes antitumor CTL activity by triggering lytic granule polarization and exocytosis. J Exp Med (2007) 204(3):559–70. doi: 10.1084/jem.20061524
42. Le Floc’h A, Jalil A, Franciszkiewicz K, Validire P, Vergnon I, Mami-Chouaib F. Minimal engagement of CD103 on cytotoxic T lymphocytes with an e-cadherin-Fc molecule triggers lytic granule polarization via a phospholipase cgamma-dependent pathway. Cancer Res (2011) 71(2):328–38. doi: 10.1158/0008-5472.CAN-10-2457
43. Franciszkiewicz K, Le Floc’h A, Boutet M, Vergnon I, Schmitt A, Mami-Chouaib F. CD103 or LFA-1 engagement at the immune synapse between cytotoxic T cells and tumor cells promotes maturation and regulates T-cell effector functions. Cancer Res (2013) 73(2):617–28. doi: 10.1158/0008-5472.CAN-12-2569
44. Mokrani M, Klibi J, Bluteau D, Bismuth G, Mami-Chouaib F. Smad and NFAT pathways cooperate to induce CD103 expression in human CD8 T lymphocytes. J Immunol (2014) 192(5):2471–9. doi: 10.4049/jimmunol.1302192
45. Gauthier L, Corgnac S, Boutet M, Gros G, Validire P, Bismuth G, et al. Paxillin binding to the cytoplasmic domain of CD103 promotes cell adhesion and effector functions for CD8(+) resident memory T cells in tumors. Cancer Res (2017) 77(24):7072–82. doi: 10.1158/0008-5472.CAN-17-1487
46. Loster K, Vossmeyer D, Hofmann W, Reutter W, Danker K. alpha1 integrin cytoplasmic domain is involved in focal adhesion formation via association with intracellular proteins. Biochem J (2001) 356(Pt 1):233–40. doi: 10.1042/bj3560233
47. Ray SJ, Franki SN, Pierce RH, Dimitrova S, Koteliansky V, Sprague AG, et al. The collagen binding alpha1beta1 integrin VLA-1 regulates CD8 T cell-mediated immune protection against heterologous influenza infection. Immunity (2004) 20(2):167–79. doi: 10.1016/S1074-7613(04)00021-4
48. McNamara HA, Cai Y, Wagle MV, Sontani Y, Roots CM, Miosge LA, et al. Up-regulation of LFA-1 allows liver-resident memory T cells to patrol and remain in the hepatic sinusoids. Sci Immunol (2017) 2(9). doi: 10.1126/sciimmunol.aaj1996
49. Zaid A, Hor JL, Christo SN, Groom JR, Heath WR, Mackay LK, et al. Chemokine receptor-dependent control of skin tissue-resident memory T cell formation. J Immunol (2017) 199(7):2451–9. doi: 10.4049/jimmunol.1700571
50. Wein AN, McMaster SR, Takamura S, Dunbar PR, Cartwright EK, Hayward SL, et al. CXCR6 regulates localization of tissue-resident memory CD8 T cells to the airways. J Exp Med (2019) 216(12):2748–62. doi: 10.1084/jem.20181308
51. Franciszkiewicz K, Le Floc’h A, Jalil A, Vigant F, Robert T, Vergnon I, et al. Intratumoral induction of CD103 triggers tumor-specific CTL function and CCR5-dependent T-cell retention. Cancer Res (2009) 69(15):6249–55. doi: 10.1158/0008-5472.CAN-08-3571
52. Hombrink P, Helbig C, Backer RA, Piet B, Oja AE, Stark R, et al. Programs for the persistence, vigilance and control of human CD8(+) lung-resident memory T cells. Nat Immunol (2016) 17(12):1467–78. doi: 10.1038/ni.3589
53. Intlekofer AM, Takemoto N, Wherry EJ, Longworth SA, Northrup JT, Palanivel VR, et al. Effector and memory CD8+ T cell fate coupled by T-bet and eomesodermin. Nat Immunol (2005) 6(12):1236–44. doi: 10.1038/ni1268
54. Zhou X, Yu S, Zhao DM, Harty JT, Badovinac VP, Xue HH. Differentiation and persistence of memory CD8(+) T cells depend on T cell factor 1. Immunity (2010) 33(2):229–40. doi: 10.1016/j.immuni.2010.08.002
55. Mackay LK, Minnich M, Kragten NA, Liao Y, Nota B, Seillet C, et al. Hobit and Blimp1 instruct a universal transcriptional program of tissue residency in lymphocytes. Sci (New York NY) (2016) 352(6284):459–63. doi: 10.1126/science.aad2035
56. Milner JJ, Toma C, Yu B, Zhang K, Omilusik K, Phan AT, et al. Runx3 programs CD8(+) T cell residency in non-lymphoid tissues and tumours. Nature (2017) 552(7684):253–7. doi: 10.1038/nature24993
57. Mackay LK, Wynne-Jones E, Freestone D, Pellicci DG, Mielke LA, Newman DM, et al. T-Box transcription factors combine with the cytokines TGF-beta and IL-15 to control tissue-resident memory T cell fate. Immunity (2015) 43(6):1101–11. doi: 10.1016/j.immuni.2015.11.008
58. Zhou L, Chong MM, Littman DR. Plasticity of CD4+ T cell lineage differentiation. Immunity (2009) 30(5):646–55. doi: 10.1016/j.immuni.2009.05.001
59. Yamane H, Paul WE. Memory CD4+ T cells: fate determination, positive feedback and plasticity. Cell Mol Life Sci (2012) 69(10):1577–83. doi: 10.1007/s00018-012-0966-9
60. Sathaliyawala T, Kubota M, Yudanin N, Turner D, Camp P, Thome JJ, et al. Distribution and compartmentalization of human circulating and tissue-resident memory T cell subsets. Immunity (2013) 38(1):187–97. doi: 10.1016/j.immuni.2012.09.020
61. Kumar BV, Ma W, Miron M, Granot T, Guyer RS, Carpenter DJ, et al. Human tissue-resident memory T cells are defined by core transcriptional and functional signatures in lymphoid and mucosal sites. Cell Rep (2017) 20(12):2921–34. doi: 10.1016/j.celrep.2017.08.078
62. Collins N, Jiang X, Zaid A, Macleod BL, Li J, Park CO, et al. Skin CD4(+) memory T cells exhibit combined cluster-mediated retention and equilibration with the circulation. Nat Commun (2016) 7:11514. doi: 10.1038/ncomms11514
63. Watanabe R, Gehad A, Yang C, Scott LL, Teague JE, Schlapbach C, et al. Human skin is protected by four functionally and phenotypically discrete populations of resident and recirculating memory T cells. Sci Transl Med (2015) 7(279):279ra39. doi: 10.1126/scitranslmed.3010302
64. Klicznik MM, Morawski PA, Hollbacher B, Varkhande SR, Motley SJ, Kuri-Cervantes L, et al. Human CD4(+)CD103(+) cutaneous resident memory T cells are found in the circulation of healthy individuals. Sci Immunol (2019) 4(37). doi: 10.1126/sciimmunol.aav8995
65. Bartolome-Casado R, Landsverk OJB, Chauhan SK, Saetre F, Hagen KT, Yaqub S, et al. CD4(+) T cells persist for years in the human small intestine and display a T(H)1 cytokine profile. Mucosal Immunol (2021) 14(2):402–10. doi: 10.1038/s41385-020-0315-5
66. Oja AE, Piet B, van der Zwan D, Blaauwgeers H, Mensink M, de Kivit S, et al. Functional heterogeneity of CD4(+) tumor-infiltrating lymphocytes with a resident memory phenotype in NSCLC. Front Immunol (2018) 9:2654. doi: 10.3389/fimmu.2018.02654
67. Smolders J, Heutinck KM, Fransen NL, Remmerswaal EBM, Hombrink P, Ten Berge IJM, et al. Tissue-resident memory T cells populate the human brain. Nat Commun (2018) 9(1):4593. doi: 10.1038/s41467-018-07053-9
68. Strutt TM, Dhume K, Finn CM, Hwang JH, Castonguay C, Swain SL, et al. IL-15 supports the generation of protective lung-resident memory CD4 T cells. Mucosal Immunol (2018) 11(3):668–80. doi: 10.1038/mi.2017.101
69. Teijaro JR, Turner D, Pham Q, Wherry EJ, Lefrancois L, Farber DL. Cutting edge: tissue-retentive lung memory CD4 T cells mediate optimal protection to respiratory virus infection. J Immunol (2011) 187(11):5510–4. doi: 10.4049/jimmunol.1102243
70. Sakai S, Kauffman KD, Schenkel JM, McBerry CC, Mayer-Barber KD, Masopust D, et al. Cutting edge: control of mycobacterium tuberculosis infection by a subset of lung parenchyma-homing CD4 T cells. J Immunol (2014) 192(7):2965–9. doi: 10.4049/jimmunol.1400019
71. Glennie ND, Volk SW, Scott P. Skin-resident CD4+ T cells protect against leishmania major by recruiting and activating inflammatory monocytes. PloS Pathog (2017) 13(4):e1006349. doi: 10.1371/journal.ppat.1006349
72. Oja AE, Piet B, Helbig C, Stark R, van der Zwan D, Blaauwgeers H, et al. Trigger-happy resident memory CD4(+) T cells inhabit the human lungs. Mucosal Immunol (2018) 11(3):654–67. doi: 10.1038/mi.2017.94
73. Thomas SY, Banerji A, Medoff BD, Lilly CM, Luster AD. Multiple chemokine receptors, including CCR6 and CXCR3, regulate antigen-induced T cell homing to the human asthmatic airway. J Immunol (2007) 179(3):1901–12. doi: 10.4049/jimmunol.179.3.1901
74. Kohlmeier JE, Cookenham T, Miller SC, Roberts AD, Christensen JP, Thomsen AR, et al. CXCR3 directs antigen-specific effector CD4+ T cell migration to the lung during parainfluenza virus infection. J Immunol (2009) 183(7):4378–84. doi: 10.4049/jimmunol.0902022
75. Kohlmeier JE, Miller SC, Smith J, Lu B, Gerard C, Cookenham T, et al. The chemokine receptor CCR5 plays a key role in the early memory CD8+ T cell response to respiratory virus infections. Immunity (2008) 29(1):101–13. doi: 10.1016/j.immuni.2008.05.011
76. Wakim LM, Smith J, Caminschi I, Lahoud MH, Villadangos JA. Antibody-targeted vaccination to lung dendritic cells generates tissue-resident memory CD8 T cells that are highly protective against influenza virus infection. Mucosal Immunol (2015) 8(5):1060–71. doi: 10.1038/mi.2014.133
77. Borges da Silva H, Peng C, Wang H, Wanhainen KM, Ma C, Lopez S, et al. Sensing of ATP via the purinergic receptor P2RX7 promotes CD8(+) trm cell generation by enhancing their sensitivity to the cytokine TGF-beta. Immunity (2020) 53(1):158–71 e6. doi: 10.1016/j.immuni.2020.06.010
78. Hirai T, Yang Y, Zenke Y, Li H, Chaudhri VK, de la Cruz Diaz JS, et al. Competition for active TGFbeta cytokine allows for selective retention of antigen-specific tissue- resident memory T cells in the epidermal niche. Immunity (2021) 54(1):84–98.e5. doi: 10.1016/j.immuni.2020.10.022
79. Pauls K, Schon M, Kubitza RC, Homey B, Wiesenborn A, Lehmann P, et al. Role of integrin alphaE(CD103)beta7 for tissue-specific epidermal localization of CD8+ T lymphocytes. J Invest Dermatol (2001) 117(3):569–75. doi: 10.1046/j.0022-202x.2001.01481.x
80. Raphael I, Joern RR, Forsthuber TG. Memory CD4(+) T cells in immunity and autoimmune diseases. Cells (2020) 9(3). doi: 10.3390/cells9030531
81. Boutet M, Gauthier L, Leclerc M, Gros G, de Montpreville V, Theret N, et al. TGFbeta signaling intersects with CD103 integrin signaling to promote T-lymphocyte accumulation and antitumor activity in the lung tumor microenvironment. Cancer Res (2016) 76(7):1757–69. doi: 10.1158/0008-5472.CAN-15-1545
82. Mohammed J, Beura LK, Bobr A, Astry B, Chicoine B, Kashem SW, et al. Stromal cells control the epithelial residence of DCs and memory T cells by regulated activation of TGF-beta. Nat Immunol (2016) 17(4):414–21. doi: 10.1038/ni.3396
83. Malenica I, Adam J, Corgnac S, Mezquita L, Auclin E, Damei I, et al. Integrin-alpha(V)-mediated activation of TGF-beta regulates anti-tumour CD8 T cell immunity and response to PD-1 blockade. Nat Commun (2021) 12(1):5209. doi: 10.1038/s41467-021-25322-y
84. Workel HH, Komdeur FL, Wouters MC, Plat A, Klip HG, Eggink FA, et al. CD103 defines intraepithelial CD8+ PD1+ tumour-infiltrating lymphocytes of prognostic significance in endometrial adenocarcinoma. Eur J Cancer (Oxford England: 1990) (2016) 60:1–11. doi: 10.1016/j.ejca.2016.02.026
85. Corgnac S, Damei I, Gros G, Caidi A, Terry S, Chouaib S, et al. Cancer stem-like cells evade CD8(+)CD103(+) tumor-resident memory T (T(RM)) lymphocytes by initiating an epithelial-to-mesenchymal transition program in a human lung tumor model. J Immunother Cancer (2022) 10(4). doi: 10.1136/jitc-2022-004527
86. Iborra S, Martinez-Lopez M, Khouili SC, Enamorado M, Cueto FJ, Conde-Garrosa R, et al. Optimal generation of tissue-resident but not circulating memory T cells during viral infection requires crosspriming by DNGR-1(+) dendritic cells. Immunity (2016) 45(4):847–60. doi: 10.1016/j.immuni.2016.08.019
87. Mani V, Bromley SK, Aijo T, Mora-Buch R, Carrizosa E, Warner RD, et al. Migratory DCs activate TGF-beta to precondition naive CD8(+) T cells for tissue-resident memory fate. Science (2019) 366(6462). doi: 10.1126/science.aav5728
88. Bourdely P, Anselmi G, Vaivode K, Ramos RN, Missolo-Koussou Y, Hidalgo S, et al. Transcriptional and functional analysis of CD1c(+) human dendritic cells identifies a CD163(+) subset priming CD8(+)CD103(+) T cells. Immunity (2020) 53(2):335–52.e8. doi: 10.1016/j.immuni.2020.06.002
89. Thompson EA, Darrah PA, Foulds KE, Hoffer E, Caffrey-Carr A, Norenstedt S, et al. Monocytes acquire the ability to prime tissue-resident T cells via IL-10-Mediated TGF-beta release. Cell Rep (2019) 28(5):1127–35.e4. doi: 10.1016/j.celrep.2019.06.087
90. Wu TC, Xu K, Banchereau R, Marches F, Yu CI, Martinek J, et al. Reprogramming tumor-infiltrating dendritic cells for CD103+ CD8+ mucosal T-cell differentiation and breast cancer rejection. Cancer Immunol Res (2014) 2(5):487–500. doi: 10.1158/2326-6066.CIR-13-0217
91. Di Pilato M, Kfuri-Rubens R, Pruessmann JN, Ozga AJ, Messemaker M, Cadilha BL, et al. CXCR6 positions cytotoxic T cells to receive critical survival signals in the tumor microenvironment. Cell. (2021) 184(17):4512–30.e22. doi: 10.1016/j.cell.2021.07.015
92. Vella JL, Molodtsov A, Angeles CV, Branchini BR, Turk MJ, Huang YH. Dendritic cells maintain anti-tumor immunity by positioning CD8 skin-resident memory T cells. Life Sci Alliance (2021) 4(10). doi: 10.26508/lsa.202101056
93. Karaki S, Blanc C, Tran T, Galy-Fauroux I, Mougel A, Dransart E, et al. CXCR6 deficiency impairs cancer vaccine efficacy and CD8(+) resident memory T-cell recruitment in head and neck and lung tumors. J Immunother Cancer (2021) 9(3). doi: 10.1136/jitc-2020-001948
94. Sun JC, Bevan MJ. Defective CD8 T cell memory following acute infection without CD4 T cell help. Sci (New York NY) (2003) 300(5617):339–42. doi: 10.1126/science.1083317
95. Ahrends T, Spanjaard A, Pilzecker B, Babala N, Bovens A, Xiao Y, et al. CD4(+) T cell help confers a cytotoxic T cell effector program including coinhibitory receptor downregulation and increased tissue invasiveness. Immunity (2017) 47(5):848–61 e5. doi: 10.1016/j.immuni.2017.10.009
96. Laidlaw BJ, Zhang N, Marshall HD, Staron MM, Guan T, Hu Y, et al. CD4+ T cell help guides formation of CD103+ lung-resident memory CD8+ T cells during influenza viral infection. Immunity (2014) 41(4):633–45. doi: 10.1016/j.immuni.2014.09.007
97. Son YM, Cheon IS, Wu Y, Li C, Wang Z, Gao X, et al. Tissue-resident CD4(+) T helper cells assist the development of protective respiratory b and CD8(+) T cell memory responses. Sci Immunol (2021) 6(55). doi: 10.1126/sciimmunol.abb6852
98. Kurd NS, He Z, Louis TL, Milner JJ, Omilusik KD, Jin W, et al. Early precursors and molecular determinants of tissue-resident memory CD8(+) T lymphocytes revealed by single-cell RNA sequencing. Sci Immunol (2020) 5(47). doi: 10.1126/sciimmunol.aaz6894
99. Milner JJ, Toma C, He Z, Kurd NS, Nguyen QP, McDonald B, et al. Heterogenous populations of tissue-resident CD8(+) T cells are generated in response to infection and malignancy. Immunity (2020) 52(5):808–24 e7. doi: 10.1016/j.immuni.2020.04.007
100. Bartolome-Casado R, Landsverk OJB, Chauhan SK, Richter L, Phung D, Greiff V, et al. Resident memory CD8 T cells persist for years in human small intestine. J Exp Med (2019) 216(10):2412–26. doi: 10.1084/jem.20190414
101. Bergsbaken T, Bevan MJ, Fink PJ. Local inflammatory cues regulate differentiation and persistence of CD8(+) tissue-resident memory T cells. Cell Rep (2017) 19(1):114–24. doi: 10.1016/j.celrep.2017.03.031
102. Wakim LM, Woodward-Davis A, Liu R, Hu Y, Villadangos J, Smyth G, et al. The molecular signature of tissue resident memory CD8 T cells isolated from the brain. J Immunol (2012) 189(7):3462–71. doi: 10.4049/jimmunol.1201305
103. Cheuk S, Schlums H, Gallais Serezal I, Martini E, Chiang SC, Marquardt N, et al. CD49a expression defines tissue-resident CD8(+) T cells poised for cytotoxic function in human skin. Immunity (2017) 46(2):287–300. doi: 10.1016/j.immuni.2017.01.009
104. Tanaka T, Ohtsuka Y, Yagita H, Shiratori Y, Omata M, Okumura K. Involvement of alpha 1 and alpha 4 integrins in gut mucosal injury of graft-versus-host disease. Int Immunol (1995) 7(8):1183–9. doi: 10.1093/intimm/7.8.1183
105. Park SL, Buzzai A, Rautela J, Hor JL, Hochheiser K, Effern M, et al. Tissue-resident memory CD8(+) T cells promote melanoma-immune equilibrium in skin. Nature (2019) 565(7739):366–71. doi: 10.1038/s41586-018-0812-9
106. O’Brien SM, Klampatsa A, Thompson JC, Martinez MC, Hwang WT, Rao AS, et al. Function of human tumor-infiltrating lymphocytes in early-stage non-small cell lung cancer. Cancer Immunol Res (2019) 7(6):896–909. doi: 10.1158/2326-6066.CIR-18-0713
107. Simoni Y, Becht E, Fehlings M, Loh CY, Koo SL, Teng KWW, et al. Bystander CD8(+) T cells are abundant and phenotypically distinct in human tumour infiltrates. Nature (2018) 557(7706):575–9. doi: 10.1038/s41586-018-0130-2
108. Wang ZQ, Milne K, Derocher H, Webb JR, Nelson BH, Watson PH. CD103 and intratumoral immune response in breast cancer. Clin Cancer research: an Off J Am Assoc Cancer Res (2016) 22(24):6290–7. doi: 10.1158/1078-0432.CCR-16-0732
109. Egelston CA, Avalos C, Tu TY, Rosario A, Wang R, Solomon S, et al. Resident memory CD8+ T cells within cancer islands mediate survival in breast cancer patients. JCI Insight (2019) 4(19). doi: 10.1172/jci.insight.130000
110. Komdeur FL, Prins TM, van de Wall S, Plat A, Wisman GBA, Hollema H, et al. CD103+ tumor-infiltrating lymphocytes are tumor-reactive intraepithelial CD8+ T cells associated with prognostic benefit and therapy response in cervical cancer. Oncoimmunology (2017) 6(9):e1338230. doi: 10.1080/2162402X.2017.1338230
111. Han L, Gao QL, Zhou XM, Shi C, Chen GY, Song YP, et al. Characterization of CD103(+) CD8(+) tissue-resident T cells in esophageal squamous cell carcinoma: may be tumor reactive and resurrected by anti-PD-1 blockade. Cancer Immunol Immunother (2020) 69(8):1493–504. doi: 10.1007/s00262-020-02562-3
112. Lin R, Zhang H, Yuan Y, He Q, Zhou J, Li S, et al. Fatty acid oxidation controls CD8(+) tissue-resident memory T-cell survival in gastric adenocarcinoma. Cancer Immunol Res (2020) 8(4):479–92. doi: 10.1158/2326-6066.CIR-19-0702
113. Li R, Liu H, Cao Y, Wang J, Chen Y, Qi Y, et al. Identification and validation of an immunogenic subtype of gastric cancer with abundant intratumoural CD103(+)CD8(+) T cells conferring favourable prognosis. Br J Cancer (2020) 122(10):1525–34. doi: 10.1038/s41416-020-0813-y
114. Shen Y, Li XL, Li YX, Shan ZG, Zhao YL, Cheng P, et al. Distribution, phenotype, functional and clinical relevance of CD8(+)CD103(+) tissue-resident memory T cells in human gastric cancer. Cancer Immunol Immunother (2022) 71(7):1645–54. doi: 10.1007/s00262-021-03105-0
115. Barsch M, Salie H, Schlaak AE, Zhang Z, Hess M, Mayer LS, et al. T-Cell exhaustion and residency dynamics inform clinical outcomes in hepatocellular carcinoma. J Hepatol (2022) 77(2):397–409. doi: 10.1016/j.jhep.2022.02.032
116. Cheng Y, Gunasegaran B, Singh HD, Dutertre CA, Loh CY, Lim JQ, et al. Non-terminally exhausted tumor-resident memory HBV-specific T cell responses correlate with relapse-free survival in hepatocellular carcinoma. Immunity (2021) 54(8):1825–40.e7. doi: 10.1016/j.immuni.2021.06.013
117. Edwards J, Wilmott JS, Madore J, Gide TN, Quek C, Tasker A, et al. CD103(+) tumor-resident CD8(+) T cells are associated with improved survival in immunotherapy-naive melanoma patients and expand significantly during anti-PD-1 treatment. Clin Cancer Research: an Off J Am Assoc Cancer Res (2018) 24(13):3036–45. doi: 10.1158/1078-0432.CCR-17-2257
118. Solomon B, Young RJ, Bressel M, Cernelc J, Savas P, Liu H, et al. Identification of an excellent prognosis subset of human papillomavirus-associated oropharyngeal cancer patients by quantification of intratumoral CD103+ immune cell abundance. Ann Oncology: Off J Eur Soc Med Oncol (2019) 30(10):1638–46. doi: 10.1093/annonc/mdz271
119. Bosmuller HC, Wagner P, Peper JK, Schuster H, Pham DL, Greif K, et al. Combined immunoscore of CD103 and CD3 identifies long-term survivors in high-grade serous ovarian cancer. Int J gynecological cancer: Off J Int Gynecological Cancer Society (2016) 26(4):671–9. doi: 10.1097/IGC.0000000000000672
120. Lohneis P, Sinn M, Bischoff S, Juhling A, Pelzer U, Wislocka L, et al. Cytotoxic tumour-infiltrating T lymphocytes influence outcome in resected pancreatic ductal adenocarcinoma. Eur J Cancer (Oxford England: 1990) (2017) 83:290–301. doi: 10.1016/j.ejca.2017.06.016
121. Galvez-Cancino F, Lopez E, Menares E, Diaz X, Flores C, Caceres P, et al. Vaccination-induced skin-resident memory CD8(+) T cells mediate strong protection against cutaneous melanoma. Oncoimmunology (2018) 7(7):e1442163. doi: 10.1080/2162402X.2018.1442163
122. Gamradt P, Laoubi L, Nosbaum A, Mutez V, Lenief V, Grande S, et al. Inhibitory checkpoint receptors control CD8(+) resident memory T cells to prevent skin allergy. J Allergy Clin Immunol (2019) 143(6):2147–57.e9. doi: 10.1016/j.jaci.2018.11.048
123. Wang Z, Wang S, Goplen NP, Li C, Cheon IS, Dai Q, et al. PD-1(hi) CD8(+) resident memory T cells balance immunity and fibrotic sequelae. Sci Immunol (2019) 4(36). doi: 10.1126/sciimmunol.aaw1217
124. Park SL, Zaid A, Hor JL, Christo SN, Prier JE, Davies B, et al. Local proliferation maintains a stable pool of tissue-resident memory T cells after antiviral recall responses. Nat Immunol (2018) 19(2):183–91. doi: 10.1038/s41590-017-0027-5
125. Wang P, Huang B, Gao Y, Yang J, Liang Z, Zhang N, et al. CD103(+)CD8(+) T lymphocytes in non-small cell lung cancer are phenotypically and functionally primed to respond to PD-1 blockade. Cell Immunol (2018) 325:48–55. doi: 10.1016/j.cellimm.2018.02.002
126. Banchereau R, Chitre AS, Scherl A, Wu TD, Patil NS, de Almeida P, et al. Intratumoral CD103+ CD8+ T cells predict response to PD-L1 blockade. J Immunother Cancer (2021) 9(4). doi: 10.1136/jitc-2020-002231
127. Enamorado M, Iborra S, Priego E, Cueto FJ, Quintana JA, Martinez-Cano S, et al. Enhanced anti-tumour immunity requires the interplay between resident and circulating memory CD8(+) T cells. Nat Commun (2017) 8:16073. doi: 10.1038/ncomms16073
128. Kurtulus S, Madi A, Escobar G, Klapholz M, Nyman J, Christian E, et al. Checkpoint blockade immunotherapy induces dynamic changes in PD-1(-)CD8(+) tumor-infiltrating T cells. Immunity (2019) 50(1):181–94 e6. doi: 10.1016/j.immuni.2018.11.014
129. Siddiqui I, Schaeuble K, Chennupati V, Fuertes Marraco SA, Calderon-Copete S, Pais Ferreira D, et al. Intratumoral Tcf1(+)PD-1(+)CD8(+) T cells with stem-like properties promote tumor control in response to vaccination and checkpoint blockade immunotherapy. Immunity (2019) 50(1):195–211.e10. doi: 10.1016/j.immuni.2018.12.021
Keywords: tumor-resident memory T (TRM) cells, CD103 integrin, tumor microenvironment, cancer immunotherapy, biomarker
Citation: Damei I, Trickovic T, Mami-Chouaib F and Corgnac S (2023) Tumor-resident memory T cells as a biomarker of the response to cancer immunotherapy. Front. Immunol. 14:1205984. doi: 10.3389/fimmu.2023.1205984
Received: 14 April 2023; Accepted: 03 July 2023;
Published: 20 July 2023.
Edited by:
Jianghong Man, National Center of Biomedical Analysis (NCBA), ChinaReviewed by:
Colt Egelston, City of Hope National Medical Center, United StatesInge Jedema, Leiden University Medical Center (LUMC), Netherlands
Copyright © 2023 Damei, Trickovic, Mami-Chouaib and Corgnac. This is an open-access article distributed under the terms of the Creative Commons Attribution License (CC BY). The use, distribution or reproduction in other forums is permitted, provided the original author(s) and the copyright owner(s) are credited and that the original publication in this journal is cited, in accordance with accepted academic practice. No use, distribution or reproduction is permitted which does not comply with these terms.
*Correspondence: Stéphanie Corgnac, stephanie.corgnac@gustaveroussy.fr
†These authors have contributed equally to this work and share first authorship