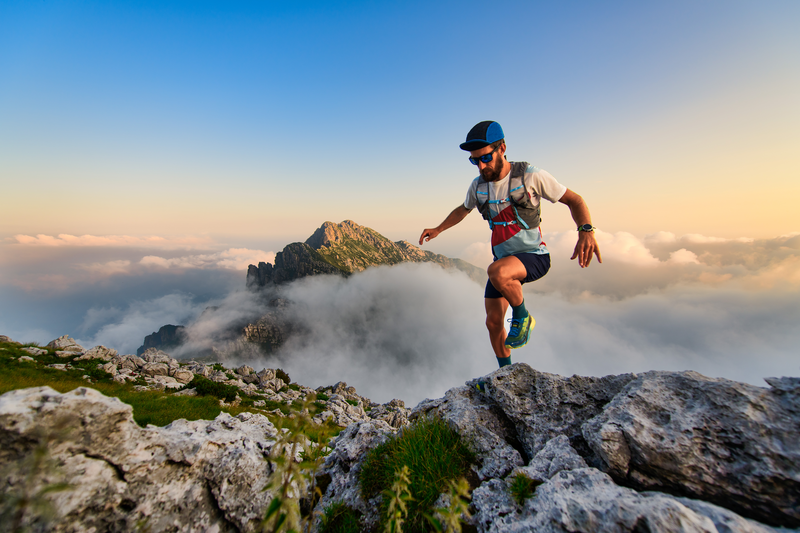
95% of researchers rate our articles as excellent or good
Learn more about the work of our research integrity team to safeguard the quality of each article we publish.
Find out more
SYSTEMATIC REVIEW article
Front. Immunol. , 12 September 2023
Sec. Vaccines and Molecular Therapeutics
Volume 14 - 2023 | https://doi.org/10.3389/fimmu.2023.1204831
This article is part of the Research Topic Therapeutic management of COVID-19 in immunocompromised patients and interaction with the immune response: from preventive to curative strategies View all 9 articles
Introduction: Despite representing only 3% of the US population, immunocompromised (IC) individuals account for nearly half of the COVID-19 breakthrough hospitalizations. IC individuals generate a lower immune response after vaccination in general, and the US CDC recommended a third dose of either mRNA-1273 or BNT162b2 COVID-19 vaccines as part of their primary series. Influenza vaccine trials have shown that increasing dosage could improve effectiveness in IC populations. The objective of this systematic literature review and pairwise meta-analysis was to evaluate the clinical effectiveness of mRNA-1273 (50 or 100 mcg/dose) vs BNT162b2 (30 mcg/dose) in IC populations using the GRADE framework.
Methods: The systematic literature search was conducted in the World Health Organization COVID-19 Research Database. Studies were included in the pairwise meta-analysis if they reported comparisons of mRNA-1273 and BNT162b2 in IC individuals ≥18 years of age; outcomes of interest were symptomatic, laboratory-confirmed SARS-CoV-2 infection, SARS-CoV-2 infection, severe SARS-CoV-2 infection, hospitalization due to COVID-19, and mortality due to COVID-19. Risk ratios (RR) were pooled across studies using random-effects meta-analysis models. Outcomes were also analyzed in subgroups of patients with cancer, autoimmune disease, and solid organ transplant. Risk of bias was assessed using the Newcastle-Ottawa Scale for observational studies. Evidence was evaluated using the GRADE framework.
Results: Overall, 17 studies were included in the pairwise meta-analysis. Compared with BNT162b2, mRNA-1273 was associated with significantly reduced risk of SARS-CoV-2 infection (RR, 0.85 [95% CI, 0.75–0.97]; P=0.0151; I2 = 67.7%), severe SARS-CoV-2 infection (RR, 0.85 [95% CI, 0.77–0.93]; P=0.0009; I2 = 0%), COVID-19–associated hospitalization (RR, 0.88 [95% CI, 0.79–0.97]; P<0.0001; I2 = 0%), and COVID-19–associated mortality (RR, 0.63 [95% CI, 0.44–0.90]; P=0.0119; I2 = 0%) in IC populations. Results were consistent across subgroups. Because of sample size limitations, relative effectiveness of COVID-19 mRNA vaccines in IC populations cannot be studied in randomized trials. Based on nonrandomized studies, evidence certainty among comparisons was type 3 (low) and 4 (very low), reflecting potential biases in observational studies.
Conclusion: This GRADE meta-analysis based on a large number of consistent observational studies showed that the mRNA-1273 COVID-19 vaccine is associated with improved clinical effectiveness in IC populations compared with BNT162b2.
The global coronavirus disease 2019 (COVID-19) pandemic caused by severe acute respiratory syndrome coronavirus 2 (SARS-CoV-2) has resulted in 103 million reported infections and 1.1 million deaths to date in the United States (1). In response to the pandemic, mRNA-1273 (Spikevax®, Moderna, Inc., Cambridge, MA, USA) (2) and BNT162b2 (Comirnaty®, Pfizer/BioNTech, New York, NY, USA/Mainz, Germany) (3), each employing novel messenger ribonucleic acid (mRNA) technology, were developed and approved for the prevention of COVID-19 (4). Global phase 2/3 studies demonstrated that both mRNA vaccines given in a 2-dose series were highly efficacious at reducing symptomatic infections and hospitalizations in the immunocompetent population (5, 6).
Although immunocompromised (IC) individuals comprise only approximately 3% of the US population (7), they account for nearly half of the breakthrough COVID-19 hospitalizations (8). While there is a range of severity across conditions at the population level, adults considered immunodeficient had 2.68-fold greater adjusted odds of being hospitalized with COVID-19 compared with immunocompetent individuals due both to the underlying IC condition and therapies used for treatment (9, 10). In 1 study, use of immunosuppression in patients with autoimmune disease resulted in 1.35-fold (95% CI, 1.29–1.40) greater odds of developing life-threatening COVID-19 (11).
Despite being at increased risk of COVID-19–related morbidity and mortality (10, 12–14), IC individuals and patients receiving immunosuppressive medications were excluded from participating in pivotal trials of mRNA-1273 and BNT162b2 (5, 6). Real-world COVID-19 data indicate that vaccine immune responses are generally impaired in IC populations (9, 15–17) and that vaccine effectiveness, estimated as the odds of obtaining a positive SARS-CoV-2 test result using multivariate logistic regression models, is lower in IC versus immunocompetent individuals (18). In addition to severe COVID-19, IC populations are at higher risk of prolonged SARS-CoV-2 infection (19–26) and viral evolution (19–22, 24, 27, 28) due to poor humoral responses. These risks are exacerbated by even lower antibody responses to SARS-CoV-2 variants (29–35). High vaccine effectiveness is therefore critically important for this population, and the US Centers for Disease Control and Prevention (CDC) recommended a third dose of either mRNA-1273 or BNT162b2 COVID-19 vaccines as part of their primary series.
Influenza vaccine trials demonstrated that high-dose vaccines led to improved immune responses in IC individuals compared with standard dose vaccines and suggested that a high-dose vaccine offers greater effectiveness for IC populations (36–41). Although both mRNA-1273 and BNT162b2 employ the mRNA mode of action, the composition of each vaccine is different. For instance, the mRNA dosage and type of lipid nanoparticles used in the delivery system differs between vaccines. The mRNA-1273 primary series contains 100 mcg of mRNA and 50 mcg for the booster (2, 42), whereas BNT162b2 contains 30 mcg of mRNA for each primary and booster dose (3, 43). Observational studies have consistently shown differences between the 2 mRNA COVID-19 vaccines, both in terms of immune response (15) and clinical effectiveness (44–46) in IC populations.
As SARS-CoV-2 transitions from a pandemic to an endemic state, countries are transferring vaccination programs from central government purchasing to their respective national healthcare systems, which is triggering in-depth health technology assessments to recommend the best use of available vaccines in specific populations. Several national immunization technical advisory groups, including the Advisory Committee on Immunization Practices in the United States, use the GRADE (Grading of Recommendations, Assessment, Development and Evaluations) framework for identifying questions relevant to healthcare, selecting outcomes of interest and assessing their importance, evaluating the available evidence, and synthesizing evidence to develop recommendations consistent with considerations of values and preferences of patients and the society in which they live (47, 48).
This present analysis follows the GRADE framework to address the following healthcare question: Is the mRNA-1273 COVID-19 vaccine (50 or 100 mcg/dose) more clinically effective in IC populations compared with the BNT162b2 COVID-19 vaccine (30 mcg/dose)? Accordingly, we performed a systematic literature review and pairwise meta-analysis to compare COVID-19 vaccine effectiveness outcomes among IC individuals given either mRNA-1273 or BNT162b2.
We performed a systematic literature review in accordance with the Preferred Reporting Items for Systematic Reviews and Meta-Analyses 2020 framework (49). The main search was conducted in the World Health Organization COVID-19 Research Database on April 14, 2022, and updated on December 19, 2022. Databases searched were MEDLINE, International Clinical Trials Registry Platform, Embase, EuropePMC, medRxiv, Web of Science, ProQuest Central, Academic Search Complete, Scopus, and COVIDWHO. The search strategy is presented in Table S1.
Clinical trials, observational studies, or any real-world evidence published as manuscripts, letters, commentaries, abstracts, or posters were included if they reported efficacy or clinical effectiveness outcomes in IC individuals ≥18 years of age vaccinated with mRNA-1273 or BNT162b2 within the same study. IC individuals were defined as people with immunocompromising conditions considered to be in the clinically extremely vulnerable (CEV) groups 1 or 2, which includes solid organ transplant, solid and hematologic cancers, hemodialysis, poorly controlled HIV infection, and autoimmune conditions requiring immunosuppressive therapy (50). Outcomes of interest were vaccine efficacy or effectiveness against symptomatic, laboratory-confirmed SARS-CoV-2 infection, SARS-CoV-2 infection, severe SARS-CoV-2 infection (defined as patients with infection and hospitalization or death, or as reported by each study), COVID-19–associated hospitalization, and COVID-19–associated death. Recently published systematic literature reviews on the same topic were cross-checked to ensure relevant articles were included. Studies reporting outcomes in pregnant women, current or former smokers, physically inactive people, or those with a heterologous vaccination schedule (ie, mix of mRNA-1273 and BNT162b2), only safety data, or study protocols or economic models were excluded. The population, exposure, comparison, and outcomes used in the systematic literature review are summarized in Table S2. Two independent reviewers selected studies following a 2-level approach; a third reviewer arbitrated conflicts. Titles and abstracts were screened against inclusion criteria in level 1, followed by full-text appraisal of articles not excluded at level 1 against selection criteria in level 2.
Publication details, study and participant characteristics, vaccine type and vaccination status, at-risk condition, and clinical outcomes were extracted. Risk of bias (RoB) was assessed in accordance with Cochrane review guidelines (51) using the Newcastle-Ottawa Scale (52) for observational studies. Evidence was evaluated based on GRADE criteria (47, 48).
Random-effects meta-analysis models were used to pool risk ratios (RR) and calculate absolute effects as risk difference (RD) per 100,000 individuals across studies. Inverse variance weights were calculated for individual studies with the DerSimonian-Laird method (53). Chi-square testing to evaluate heterogeneity across studies was performed (54). The I2 statistic was estimated (0%–100%, 0% meaning no evidence of heterogeneity). Subgroup analysis was performed for patients with cancer, autoimmune disease, and solid organ transplant to account for differences between the underlying health conditions in the overall IC population.
Of 5745 unique items retrieved, 34 studies were identified reporting COVID-19 clinical efficacy or effectiveness outcomes in IC individuals ≥18 years of age who received mRNA-1273 or BNT162b2 in the same study (Figure 1). Seventeen articles were excluded because the population did not meet the inclusion criteria (ie, participants had IC conditions not included in CEV groups 1 or 2), 1-dose vaccine regimen data were reported, or the outcome of interest data were not reported in sufficient detail for analysis. Characteristics of all studies (n=17) included in the pairwise meta-analysis are shown in Table 1. Overall, 178,298 and 170,760 patients received mRNA-1273 and BNT162b2, respectively. Studies included mostly US populations (n=12) (18, 44, 46, 55–59, 61, 64, 65, 67), with the remaining trials reporting data on patients from Spain (n=2) (45, 62), Italy (n=1) (63), Singapore (n=1) (66), and multiple countries (n=1) (60). Specific at-risk and IC conditions included solid organ transplant (n=5) (44, 45, 55, 61, 67), cancer (n=5) (18, 44, 58, 63, 64), hemodialysis (n=2) (56, 65), rheumatologic disease (n=3) (18, 46, 60), multiple sclerosis or other neurologic autoimmune disease (n=2) (57, 66), inflammatory bowel disease (n=1) (59), and hematologic disorders (n=1) (62). Individuals received ≥2 doses of mRNA-1273 or BNT162b2. Data on 2-dose regimens were considered if reported (n=13) (18, 44, 45, 55–57, 59–62, 64, 65, 67); otherwise, data from 3- or 4-dose regimens (n=4) (46, 58, 63, 66) were used. Outcomes were assessed ≥14 days after the second dose (n=13) (18, 44, 45, 55, 56, 58, 60–64, 66, 67), other timepoints (n=2) (46, 65), and ≥7 days after the second dose (n=1) (59). Timing of outcome assessment relative to the second dose was not specified in 1 study (57). Variants of concern were delta (n=6) (18, 44, 45, 62, 64, 67), delta and omicron (n=2) (58, 66), delta and beta (n=1) (56), and pre-omicron variants (n=1) (46). Seven studies did not directly specify the variant assessed (55, 57, 59–61, 63, 65).
Figure 1 PRISMA Flow Diagram. Searches were first performed on April 14, 2022 followed by an update on December 19, 2022. *Databases searched include ICTRP, Embase, EuropePMC, medRxiv, Web of Science, ProQuest Central, Academic Search Complete, Scopus, and COVIDWHO. **Includes internal documents from Moderna and recently published SLRs. COVID-19, coronavirus disease 2019; PRISMA, Preferred Reporting Items for Systematic Reviews and Meta-Analyses; SLR, systematic literature review; WHO, World Health Organization.
Risk-of-bias assessment found no serious RoB for 13 studies and serious RoB in 4 nonrandomized studies, primarily because of the lack of description of comparability between cohorts or adjustment for confounding factors (Table S3).
Of the 4 studies reporting symptomatic, laboratory-confirmed SARS-CoV-2 infection, mRNA COVID-19 vaccine type was not associated with a statistically significant risk of infection (RR, 0.88 [95% CI, 0.65–1.19]; Table 2). The RD (95% CI) of mRNA-1273 compared with BNT162b2 was estimated to be 633 fewer infections (792 fewer to 473 fewer). Heterogeneity between studies analyzed for this outcome may be considered substantial (I2 = 60.3%). Evidence certainty was rated as type 3 (low) due to the inclusion of nonrandomized studies and because of imprecision (Table 2).
Subgroup analysis was performed in 2 studies of solid organ transplant recipients (55, 67) and in 1 study of patients with autoimmune disease (66). No association between mRNA COVID-19 vaccine type and risk of symptomatic, laboratory-confirmed SARS-CoV-2 infection was observed in either the solid organ transplant recipient subgroup (RR, 1.11 [95% CI, 0.86–1.43]; RD, −202 [95% CI, −1059 to 655]) or the autoimmune disease subgroup (RR, 0.48 [95% CI, 0.07–3.48; RD, −2873 [95% CI, −8531 to 2785]; Table 3).
Meta-analysis of the 15 studies reporting SARS-CoV-2 infection in IC populations showed that mRNA-1273 was associated with a statistically significant reduction in the risk of SARS-CoV-2 infection compared with BNT162b2 (RR, 0.85 [95% CI, 0.75–0.97]; P=0.0151). The RD (95% CI) of mRNA-1273 versus BNT162b2 was estimated to be 375 fewer SARS-CoV-2 infections (from 626 fewer to 124 fewer; P=0.0034). Heterogeneity between studies may be considered substantial (I2 = 67.7%). The certainty of evidence was graded as type 4 (very low) for imprecision and indirectness due to varying outcome definitions (Table 2).
Analysis of 5 studies reporting SARS-CoV-2 infection in patients with cancer (18, 44, 62–64) found that mRNA-1273 was associated with significantly reduced risk of infection compared with BNT162b2 (RR, 0.68 [95% CI, 0.55–0.83]; P=0.0002; RD, −437 [95% CI, −699 to −175]; P=0.0011). Similar findings were observed in 5 studies assessing patients with autoimmune diseases (RR, 0.77 [95% CI, 0.62–0.96]; P=0.0187; RD, −363 [95% CI, −956 to 231]) (18, 46, 57, 59, 66). No association between mRNA vaccine type and risk of infection was found for the 4 studies reporting SARS-CoV-2 infection in solid organ transplant recipients (RR, 1.05 [95% CI, 0.87–1.26]; RD, −93 [95% CI, −573 to 386]) (44, 55, 61, 67). No evidence of heterogeneity was observed between any of the studies (I2 = 0% for all subgroups). As in the overall meta-analysis of SARS-CoV-2 infection, the certainty of evidence was graded as type 4 (very low; Table 3).
Compared with BNT162b2, mRNA-1273 was associated with a statistically significant reduction in the risk of severe SARS-CoV-2 infection among IC patients in 12 studies (RR, 0.85 [95% CI, 0.77–0.93]; P=0.0009). The RD (95% CI) of mRNA-1273 versus BNT162b2 was 25 fewer severe SARS-CoV-2 infections (from 82 fewer to 32 more). No evidence of heterogeneity was observed between studies (I2 = 0%). The certainty of evidence for this outcome was type 4 (very low) because of imprecision and indirectness due to varying outcome definitions (Table 2).
In 2 studies reporting severe SARS-CoV-2 infection in patients with cancer (18, 44), mRNA-1273 was associated with a statistically significant reduction in the risk of severe infection compared with BNT162b2 (RR, 0.54 [95% CI, 0.37–0.79]; P=0.0013; RD, −585 [95% CI, −1655 to 485]). No evidence of heterogeneity was observed between studies (I2 = 0%). The certainty of evidence for severe SARS-CoV-2 infection in this subgroup was type 4 (very low) due to limited evidence and indirectness due to varying outcome definitions. mRNA COVID-19 vaccine type was not associated with statistically significant differences in the risk of severe SARS-CoV-2 infection in solid organ transplant (44, 45, 55, 61, 67) (RR, 0.91 [95% CI, 0.79–1.06]; RD, −16 [95% CI, −275 to 244]) and autoimmune disease subgroups (18, 57, 59, 60, 66) (RR, 0.98 [95% CI, 0.71–1.36]; RD, 24 [95% CI, −82 to 130]; Table 3).
mRNA-1273 was associated with a significantly lower risk of COVID-19–associated hospitalization versus BNT162b2 in the 9 studies included in the overall analysis (RR, 0.88 [95% CI, 0.79–0.97]; P<0.0001). The RD (95% CI) of mRNA-1273 compared with BNT162b2 was estimated to be 14 fewer hospitalizations due to COVID-19 (from 78 fewer to 51 more). No evidence of heterogeneity was observed between studies (I2 = 0%). The certainty of evidence for this outcome was type 3 (low) because of inclusion of nonrandomized studies and imprecision (Table 2).
In 2 studies reporting hospitalization in patients with cancer (18, 44), mRNA-1273 was associated with a significantly reduced risk of hospitalization compared with BNT162b2 (RR, 0.54 [95% CI, 0.37–0.79]; P=0.0013; RD, −585 [95% CI, −1655 to 485]). No association between mRNA vaccine type and COVID-19–associated hospitalization was found for the 4 studies each reporting hospitalization in the subgroups of patients with autoimmune diseases (RR, 0.98 [95% CI, 0.70–1.35]; RD, −16 [95% CI, −1139 to 1107]) (18, 57, 60, 66) or solid organ transplant (RR, 0.91 [95% CI, 0.79–1.06]; RD, −31 [95% CI, −330 to 268]) (44, 45, 61). No evidence of heterogeneity was observed between any of the studies for the subgroup analysis (I2 = 0%). The certainty of evidence in the cancer subgroup was graded as type 3 (low; Table 3).
Of the 7 studies reporting COVID-19–associated mortality (45, 55, 57, 60, 61, 65, 67), mRNA-1273 was associated with a significantly reduced risk of death compared with BNT162b2 (RR, 0.63 [95% CI, 0.44–0.90]; P=0.0119). Vaccination with mRNA-1273 was estimated to result in 15 fewer deaths associated with COVID-19 (from 307 fewer to 227 more) compared with BNT162b2. No evidence of heterogeneity was observed between the studies (I2 = 0%). The certainty of evidence was rated as type 3 (low) due to inclusion of nonrandomized studies (Table 2). Grading was reduced for imprecision.
COVID-19–associated death was assessed only in the subgroup of solid organ transplant recipients (n=4) (45, 55, 61, 67) and autoimmune disease (n=2) (57, 60).mRNA-1273 was associated with a significantly reduced risk of death compared with BNT162b2 in solid organ transplant recipients (RR, 0. 57 [95% CI, 0.38–0.85]; P=0.0056; RD, −180 [95% CI, −1443 to 1083]). No evidence of heterogeneity was observed between any of the studies in this subgroup (I2 = 0%). The certainty of evidence in the solid organ transplant subgroup was type 3 (low) because of inclusion of nonrandomized studies. Lower grading due to imprecision was offset by no concerns with indirectness and a strong association. mRNA COVID-19 vaccine type was not associated with statistically significant reduction in COVID-19–associated mortality in patients with autoimmune disease (RR, 0.81 [95% CI, 0.12–5.53]; RD, 40 [95% CI, −1563 to 1642]; Table 3).
In this systematic review and pairwise meta-analysis of 17 studies, we found that mRNA-1273 was associated with a significantly lower risk of SARS-CoV-2 infection, severe SARS-CoV-2 infection, hospitalization due to COVID-19, and COVID-19–associated mortality compared with BNT162b2 in adults with a broad spectrum of severe IC conditions. A summary of the RR of each outcome for mRNA-1273 versus BNT162b2 is presented in Figure 2. The certainty of this evidence was type 4 (very low) for the SARS-CoV-2 infection and severe SARS-CoV-2 infection outcomes and type 3 (low) for the COVID-19–associated hospitalization and death outcomes (Table 4). Because all included studies were pairwise comparisons between mRNA-1273 and BNT162b2, the research question was not biased by differences in period assessed, population, or viral variants within each study. When outcomes were assessed in subgroups, mRNA-1273 was associated with significantly lower risk of SARS-CoV-2 infection, severe SARS-CoV-2 infection, and COVID-19–associated hospitalization versus BNT162b2 in patients with cancer. Compared with BNT162b2, mRNA-1273 was also associated with a significantly reduced risk of SARS-CoV-2 infection in patients with autoimmune diseases and COVID-19–associated death in solid organ transplant recipients.
Figure 2 Summary of the Clinical Effectiveness Meta-Analysis. A graphical summary of the relative risks of clinical effectiveness outcomes analyzed for mRNA-1273 vs BNT162b2 COVID-19 vaccines in IC populations is shown. COVID-19, coronavirus disease 2019; IC, immunocompromised; SARS-CoV-2, severe acute respiratory syndrome coronavirus 2.
Immunocompromised individuals have a high burden of COVID-19 due to characteristics of their underlying disease or immunosuppressive treatments that affect their ability to mount productive immune responses and increased susceptibility to severe COVID-19 (30). Physicians may seek to optimize COVID-19 vaccine type, timing, and number of doses to improve outcomes in IC patients (32). RCTs are ranked highly in the hierarchy of evidence; however, studying comparative efficacy with adequate power would require enrolling a prohibitive number of IC patients. Given the rapid spread of SARS-CoV-2 globally, there was also insufficient time following the development of mRNA COVID-19 vaccines to conduct a larger number of RCTs in IC populations. Therefore, the research question can only be assessed using large real-world databases where individual medical and pharmacy information is available and real-world studies evaluating vaccine effectiveness in IC patients. Given the risk of severe COVID-19 in IC patients, our approach may be justified given the absence of RCT data in this vulnerable population.
Limitations of this systematic literature review were that non-English studies were excluded, and publication bias was not assessed in the meta-analysis. Inherent to the GRADE framework, evidence certainty is initially set to either high if the included studies are randomized studies or low if they are observational studies. Because all 17 studies included in the pairwise meta-analysis were nonrandomized, the maximum certainty of evidence achievable in this meta-analysis was low despite the high number of observational studies and consistency of results. The pairwise meta-analysis was also limited by inconsistent outcome definitions across studies and differences in covariates between studies. For example, the vaccination scheme (2 vs 3 doses; booster) differed between studies, with a mix of primary series (100 mcg vs 30 mcg) and booster (50 mcg vs 30 mcg) pairwise comparisons included in the meta-analysis. Variants of concern changed over time, with risks of hospitalization and death (68) and vaccine effectiveness differing by variant (69). Vaccine effectiveness of 2-dose regimens could only be shown for the ancestral strain through the delta variants, whereas the omicron variant required a 3-dose schedule. Other sources of bias, such as prescribing differences by risk of severe COVID-19, ability of patients to choose the mRNA vaccine type, and differing risks of infection or COVID-19–associated morbidity and mortality among patients with different IC conditions, could not be accounted for in this meta-analysis. In addition to differences in mRNA dosage between mRNA-1273 and BNT162b2, other differences such as the lipid nanoparticle delivery system and mRNA translation efficiency may also have impacted clinical effectiveness between vaccines.
Our meta-analysis of observational studies showed that mRNA-1273 (50 or 100 mcg/dose) was associated with a significantly reduced risk of SARS-CoV-2 infection, severe SARS-CoV-2 infection, COVID-19–associated hospitalization, and death due to COVID-19 when compared with BNT162b2 (30 mcg/dose) in IC populations. Based on these findings, vaccinating IC individuals in the United States with mRNA-1273 instead of BNT162b2 would prevent an additional 14 and 15 hospitalizations and deaths per 100,000 individuals, respectively. Although the numerical differences in estimated prevented COVID-19–associated hospitalizations and deaths are small, the implications for care are considerable in IC patients, who are at increased risk of severe COVID-19. Considering the limited availability of data from RCTs and to provide needed clinical decision-making guidance, our results showed that mRNA-1273 offers better clinical outcomes compared with BNT162b2 in vulnerable IC populations. A video summary linked to this article can be found at Video S1.
The original contributions presented in the study are included in the article/Supplementary Material. Further inquiries can be directed to the corresponding author.
XW and KH designed and performed the systematic literature review and meta-analysis and critically evaluated the manuscript. AS, LP, AR, and AK designed and performed the systematic literature review and critically evaluated the manuscript. PS and SV collected data and critically evaluated the manuscript. MB-J and NV conceptualized the article and provided oversight and critical evaluation of the manuscript. All authors contributed to the article and approved the submitted version.
This study was funded by Moderna, Inc. Authors employed by Moderna, Inc. were involved in the study design, analysis and interpretation of data, the writing of the manuscript, and the decision to submit the manuscript for publication.
This manuscript has been posted on the preprint server medRxiv (70). Writing assistance was provided by Erin McClure, PhD, an employee of ICON (Blue Bell, PA, USA) in accordance with Good Publication Practice (GPP3) guidelines, funded by Moderna, Inc., and under the direction of the authors.
XW, KH, PS, AK, and SV are employees of ICON plc, a clinical research organization paid by Moderna, Inc., to conduct the study. AS is an independent epidemiology consultant/director of Data Health Ltd, which provides health data consultancy services, and was paid by Moderna, Inc., to conduct aspects of this study. LP is an employee and owner of Data-Driven LLC and AR is a contractor of Data-Driven LLC, a research organization paid by Moderna, Inc. to conduct aspects of this study. MB-J and NV are employees of Moderna, Inc., and hold stock/stock options in the company.
This study was funded by Moderna, Inc. Authors employed by Moderna, Inc. were involved in the study design, analysis and interpretation of data, the writing of the manuscript, and the decision to submit the manuscript for publication.
All claims expressed in this article are solely those of the authors and do not necessarily represent those of their affiliated organizations, or those of the publisher, the editors and the reviewers. Any product that may be evaluated in this article, or claim that may be made by its manufacturer, is not guaranteed or endorsed by the publisher.
The Supplementary Material for this article can be found online at: https://www.frontiersin.org/articles/10.3389/fimmu.2023.1204831/full#supplementary-material
1. National Center for Immunization and Respiratory Diseases (NCIRD) Division of Viral Diseases. COVID data tracker weekly review: interpretive summary for February 10, 2023 (2023). Centers for Disease Control and Prevention. Available at: https://www.cdc.gov/coronavirus/2019-ncov/covid-data/covidview/index.html (Accessed April 4, 2023).
4. Chaudhary N, Weissman D, Whitehead KA. mRNA vaccines for infectious diseases: principles, delivery and clinical translation. Nat Rev Drug Discov (2021) 20:817–38. doi: 10.1038/s41573-021-00283-5
5. Polack FP, Thomas SJ, Kitchin N, Absalon J, Gurtman A, Lockhart S, et al. Safety and efficacy of the BNT162b2 mRNA Covid-19 vaccine. N Engl J Med (2020) 383:2603–15. doi: 10.1056/NEJMoa2034577
6. Baden LR, El Sahly HM, Essink B, Kotloff K, Frey S, Novak R, et al. Efficacy and safety of the mRNA-1273 SARS-CoV-2 vaccine. N Engl J Med (2021) 384:403–16. doi: 10.1056/NEJMoa2035389
7. Harpaz R, Dahl RM, Dooling KL. Prevalence of immunosuppression among US adults, 2013. JAMA (2016) 316:2547–8. doi: 10.1001/jama.2016.16477
8. Tenforde MW, Patel MM, Ginde AA, Douin DJ, Talbot HK, Casey JD, et al. Effectiveness of severe acute respiratory syndrome coronavirus 2 messenger RNA vaccines for preventing coronavirus disease 2019 hospitalizations in the United States. Clin Infect Dis (2022) 74:1515–24. doi: 10.1093/cid/ciab687
9. Ridgway JP, Tideman S, French T, Wright B, Parsons G, Diaz G, et al. Odds of hospitalization for COVID-19 after 3 vs 2 doses of mRNA COVID-19 vaccine by time since booster dose. JAMA (2022) 328:1559–61. doi: 10.1001/jama.2022.17811
10. DeWolf S, Laracy JC, Perales MA, Kamboj M, van den Brink MRM, Vardhana S. SARS-CoV-2 in immunocompromised individuals. Immunity (2022) 55:1779–98. doi: 10.1016/j.immuni.2022.09.006
11. Yadaw AS, Afzali B, Hotaling N, Sidky H, Pfaff ER, Sahner DK, et al. Pre-existing autoimmunity is associated with increased severity of COVID-19: a retrospective cohort study using data from the National COVID Cohort Collaborative (N3C). medRxiv. doi: 10.1101/2023.02.02.23285353. Preprint posted online Feb 4, 2023.
12. National Center for Immunization and Respiratory Diseases (NCIRD) Division of Viral Diseases. People with certain medical conditions (2023). Centers for Disease Control and Prevention. Available at: https://www.cdc.gov/coronavirus/2019-ncov/need-extra-precautions/people-with-medical-conditions.html (Accessed February 16, 2023).
13. Singson JRC, Kirley PD, Pham H, Rothrock G, Armistead I, Meek J, et al. Factors associated with severe outcomes among immunocompromised adults hospitalized for COVID-19 - COVID-NET, 10 states, March 2020-February 2022. MMWR Morb. Mortal. Wkly. Rep (2022) 71:878–84. doi: 10.15585/mmwr.mm7127a3
14. Dandachi D, Geiger G, Montgomery MW, Karmen-Tuohy S, Golzy M, Antar AAR, et al. Characteristics, comorbidities, and outcomes in a multicenter registry of patients with human immunodeficiency virus and coronavirus disease 2019. Clin Infect Dis (2021) 73:e1964–72. doi: 10.1093/cid/ciaa1339
15. Stumpf J, Siepmann T, Lindner T, Karger C, Schwobel J, Anders L, et al. Humoral and cellular immunity to SARS-CoV-2 vaccination in renal transplant versus dialysis patients: a prospective, multicenter observational study using mRNA-1273 or BNT162b2 mRNA vaccine. Lancet Reg Health Eur (2021) 9:100178. doi: 10.1016/j.lanepe.2021.100178
16. Shen C, Risk M, Schiopu E, Hayek SS, Xie T, Holevinski L, et al. Efficacy of COVID-19 vaccines in patients taking immunosuppressants. Ann Rheum Dis (2022) 81:875–80. doi: 10.1136/annrheumdis-2021-222045
17. Jena A, Mishra S, Deepak P, Kumar MP, Sharma A, Patel YI, et al. Response to SARS-CoV-2 vaccination in immune mediated inflammatory diseases: systematic review and meta-analysis. Autoimmun Rev (2022) 21:102927. doi: 10.1016/j.autrev.2021.102927
18. Embi PJ, Levy ME, Naleway AL, Patel P, Gaglani M, Natarajan K, et al. Effectiveness of 2-dose vaccination with mRNA COVID-19 vaccines against COVID-19–associated hospitalizations among immunocompromised adults—nine states, January–September 2021. MMWR Morb. Mortal. Wkly. Rep (2021) 70:1553. doi: 10.15585/mmwr.mm7044e3
19. Truong TT, Ryutov A, Pandey U, Yee R, Goldberg L, Bhojwani D, et al. Increased viral variants in children and young adults with impaired humoral immunity and persistent SARS-CoV-2 infection: a consecutive case series. EBioMedicine (2021) 67:103355. doi: 10.1016/j.ebiom.2021.103355
20. Hensley MK, Bain WG, Jacobs J, Nambulli S, Parikh U, Cillo A, et al. Intractable coronavirus disease 2019 (COVID-19) and prolonged severe acute respiratory syndrome coronavirus 2 (SARS-CoV-2) replication in a chimeric antigen receptor-modified T-cell therapy recipient: a case study. Clin Infect Dis (2021) 73:e815–21. doi: 10.1093/cid/ciab072
21. Baang JH, Smith C, Mirabelli C, Valesano AL, Manthei DM, Bachman MA, et al. Prolonged severe acute respiratory syndrome coronavirus 2 replication in an immunocompromised patient. J Infect Dis (2021) 223:23–7. doi: 10.1093/infdis/jiaa666
22. Choi B, Choudhary MC, Regan J, Sparks JA, Padera RF, Qiu X, et al. Persistence and evolution of SARS-CoV-2 in an immunocompromised host. N Engl J Med (2020) 383:2291–3. doi: 10.1056/NEJMc2031364
23. Helleberg M, Niemann CU, Moestrup KS, Kirk O, Lebech AM, Lane C, et al. Persistent COVID-19 in an immunocompromised patient temporarily responsive to two courses of remdesivir therapy. J Infect Dis (2020) 222:1103–7. doi: 10.1093/infdis/jiaa446
24. Khatamzas E, Rehn A, Muenchhoff M, Hellmuth J, Gaitzsch E, Weiglein T, et al. Emergence of multiple SARS-CoV-2 mutations in an immunocompromised host. medRxiv. Preprint posted online April 7, 2023. doi: 10.1101/2021.01.10.20248871
25. Avanzato VA, Matson MJ, Seifert SN, Pryce R, Williamson BN, Anzick SL, et al. Case study: prolonged infectious SARS-CoV-2 shedding from an asymptomatic immunocompromised individual with cancer. Cell (2020) 183:1901–12.e9. doi: 10.1016/j.cell.2020.10.049
26. Nakajima Y, Ogai A, Furukawa K, Arai R, Anan R, Nakano Y, et al. Prolonged viral shedding of SARS-CoV-2 in an immunocompromised patient. J Infect Chemother (2021) 27:387–9. doi: 10.1016/j.jiac.2020.12.001
27. Clark SA, Clark LE, Pan J, Coscia A, McKay LGA, Shankar S, et al. SARS-CoV-2 evolution in an immunocompromised host reveals shared neutralization escape mechanisms. Cell (2021) 184:2605–17.e18. doi: 10.1016/j.cell.2021.03.027
28. Kemp SA, Collier DA, Datir RP, Ferreira I, Gayed S, Jahun A, et al. SARS-CoV-2 evolution during treatment of chronic infection. Nature (2021) 592:277–82. doi: 10.1038/s41586-021-03291-y
29. Kwon JH, Tenforde MW, Gaglani M, Talbot HK, Ginde AA, McNeal T, et al. mRNA vaccine effectiveness against coronavirus disease 2019 hospitalization among solid organ transplant recipients. J Infect Dis (2022) 226:797–807. doi: 10.1093/infdis/jiac118
30. Parker EPK, Desai S, Marti M, Nohynek H, Kaslow DC, Kochhar S, et al. Response to additional COVID-19 vaccine doses in people who are immunocompromised: a rapid review. Lancet Glob Health (2022) 10:e326–8. doi: 10.1016/S2214-109X(21)00593-3
31. Patyna S, Eckes T, Koch BF, Sudowe S, Oftring A, Kohmer N, et al. Impact of Moderna mRNA-1273 booster vaccine on fully vaccinated high-risk chronic dialysis patients after loss of humoral response. Vaccines (Basel) (2022) 10:585. doi: 10.3390/vaccines10040585
32. Quiroga B, Soler MJ, Ortiz A, Jaravaca Mantecon CJ, Nava Perez N, Serra Martin M, et al. Anti-spike antibodies 3 months after SARS-CoV-2 mRNA vaccine booster dose in patients on hemodialysis: the prospective SENCOVAC study. Clin Kidney J (2022) 15:1856–64. doi: 10.1093/ckj/sfac169
33. Risk M, Hayek SS, Schiopu E, Yuan L, Shen C, Shi X, et al. COVID-19 vaccine effectiveness against omicron (B.1.1.529) variant infection and hospitalisation in patients taking immunosuppressive medications: a retrospective cohort study. Lancet Rheumatol (2022) 4:e775–84. doi: 10.1016/s2665-9913(22)00216-8
34. Wieske L, van Dam KPJ, Steenhuis M, Stalman EW, Kummer LYL, van Kempen ZLE, et al. Humoral responses after second and third SARS-CoV-2 vaccination in patients with immune-mediated inflammatory disorders on immunosuppressants: a cohort study. Lancet Rheumatol (2022) 4:e338–50. doi: 10.1016/S2665-9913(22)00034-0
35. Yang LM, Costales C, Ramanathan M, Bulterys PL, Murugesan K, Schroers-Martin J, et al. Cellular and humoral immune response to SARS-CoV-2 vaccination and booster dose in immunosuppressed patients: an observational cohort study. J Clin Virol (2022) 153:105217. doi: 10.1016/j.jcv.2022.105217
36. Caldera F, Hillman L, Saha S, Wald A, Grimes I, Zhang Y, et al. Immunogenicity of high dose influenza vaccine for patients with inflammatory bowel disease on anti-TNF monotherapy: a randomized clinical trial. Inflamm Bowel Dis (2020) 26:593–602. doi: 10.1093/ibd/izz164
37. Colmegna I, Useche ML, Rodriguez K, McCormack D, Alfonso G, Patel A, et al. Immunogenicity and safety of high-dose versus standard-dose inactivated influenza vaccine in rheumatoid arthritis patients: a randomised, double-blind, active-comparator trial. Lancet Rheumatol (2020) 2:e14–23. doi: 10.1016/S2665-9913(19)30094-3
38. Hakim H, Allison KJ, Van de Velde LA, Tang L, Sun Y, Flynn PM, et al. Immunogenicity and safety of high-dose trivalent inactivated influenza vaccine compared to standard-dose vaccine in children and young adults with cancer or HIV infection. Vaccine (2016) 34:3141–8. doi: 10.1016/j.vaccine.2016.04.053
39. Halasa NB, Savani BN, Asokan I, Kassim A, Simons R, Summers C, et al. Randomized double-blind study of the safety and immunogenicity of standard-dose trivalent inactivated influenza vaccine versus high-dose trivalent inactivated influenza vaccine in adult hematopoietic stem cell transplantation patients. Biol Blood Marrow Transplant. (2016) 22:528–35. doi: 10.1016/j.bbmt.2015.12.003
40. McKittrick N, Frank I, Jacobson JM, White CJ, Kim D, Kappes R, et al. Improved immunogenicity with high-dose seasonal influenza vaccine in HIV-infected persons: a single-center, parallel, randomized trial. Ann Intern Med (2013) 158:19–26. doi: 10.7326/0003-4819-158-1-201301010-00005
41. Natori Y, Shiotsuka M, Slomovic J, Hoschler K, Ferreira V, Ashton P, et al. A double-blind, randomized trial of high-dose vs standard-dose influenza vaccine in adult solid-organ transplant recipients. Clin Infect Dis (2018) 66:1698–704. doi: 10.1093/cid/cix1082
42. Moderna Inc. Fact sheet for healthcare providers administering vaccine: emergency use authorization of Moderna Covid-19 vaccine, bivalent (original and omicron ba.4/ba.5). Cambridge, MA, USA: Moderna, Inc. Available at: https://eua.modernatx.com/covid19vaccine-eua/eua-fact-sheet-providers.pdf (Accessed August 25, 2023).
43. Pfizer/BioNTech. Fact sheet for healthcare providers administering vaccine: emergency use authorization (EUA): Pfizer-BioNTech COVID-19 vaccine, bivalent (original and omicron BA.4/BA.5) and booster dose for 12 years of age and older (2022). New York, NY, USA: Pfizer/BioNTech. Available at: https://www.fda.gov/media/167211/download (Accessed March 7, 2023).
44. Mues KE, Kirk B, Patel DA, Gelman A, Chavers S, Talarico C, et al. Real-world comparative effectiveness of mRNA-1273 and BNT162b2 vaccines among immunocompromised adults identified in adminstrative claims data in the United States. Vaccine (2022) 40:6730–9. doi: 10.1101/2022.05.13.22274960
45. Mazuecos A, Villanego F, Zarraga S, López V, Oppenheimer F, Llinàs-Mallol L, et al. Breakthrough infections following mRNA SARS-CoV-2 vaccination in kidney transplant recipients. Transplantation (2022) 106:1430–9. doi: 10.1097/tp.0000000000004119
46. Patel NJ, Wang X, Fu X, Kawano Y, Cook C, Vanni KMM, et al. Factors associated with COVID-19 breakthrough infection among vaccinated patients with rheumatic diseases: a cohort study. Semin Arthritis Rheumatol (2022) 58:152108. doi: 10.1016/j.semarthrit.2022.152108
47. Schünemann H, Brożek J, Guyatt G, Oxman A eds. Handbook for grading the quality of evidence and the strength of recommendations using the GRADE approach. The GRADE Working Group (2013).
48. Ahmed F, Temte JL, Campos-Outcalt D, Schünemann HJ. Methods for developing evidence-based recommendations by the Advisory Committee on Immunization Practices (ACIP) of the U.S. Centers for Disease Control and Prevention (CDC). Vaccine (2011) 29:9171–6. doi: 10.1016/j.vaccine.2011.08.005
49. Page MJ, McKenzie JE, Bossuyt PM, Boutron I, Hoffmann TC, Mulrow CD, et al. The PRISMA 2020 statement: an updated guideline for reporting systematic reviews. BMJ (2021) 372:n71. doi: 10.1136/bmj.n71
50. BC COVID Therapeutics Committee. Practice tool 2 - definitions of CEV/immunosuppressed (2022). British Columbia, Canada: BC Centre for Disease Control. Available at: http://www.bccdc.ca/Health-Professionals-Site/Documents/COVID-treatment/PracticeTool2_CEVCriteria.pdf (Accessed February 16, 2023).
51. Higgins JPT, Savović J, Page MJ, Elbers RG, Sterne JAC. Chapter 8: Assessing risk of bias in a randomized trial. In: . Cochrane (2022).
52. Wells GA, Shea B, O'Connell D, Peterson J, Welch V, Losos M, et al. The Newcastle-Ottawa Scale (NOS) for assessing the quality of nonrandomised studies in meta-analyses. Available at: https://www.ohri.ca/programs/clinical_epidemiology/oxford.asp (Accessed February 16, 2023).
53. DerSimonian R, Laird N. Meta-analysis in clinical trials. Control. Clin Trials (1986) 7:177–88. doi: 10.1016/0197-2456(86)90046-2
54. Higgins JPT, Thomas J, Chandler J, Cumpston M, Li T, Page MJ, et al. editors. Cochrane Handbook for Systematic Reviews of Interventions version 6.4 (updated August 2023). Cochrane (2023). Available from www.training.cochrane.org/handbook.
55. Aslam S, Adler E, Mekeel K, Little SJ. Clinical effectiveness of COVID-19 vaccination in solid organ transplant recipients. Transpl. Infect Dis (2021) 23:e13705. doi: 10.1111/tid.13705
56. Butt AA, Talisa VB, Yan P, Shaikh OS, Omer SB, Mayr FB. Real-world effectiveness of the severe acute respiratory syndrome coronavirus 2 (SARS-CoV-2) mRNA vaccines in preventing confirmed infection in patients on chronic hemodialysis. Clin Infect Dis (2022) 75:e617–22. doi: 10.1093/cid/ciac118
57. Holroyd KB, Healy BC, Conway S, Houtchens M, Bakshi R, Bhattacharyya S, et al. Humoral response to COVID-19 vaccination in MS patients on disease modifying therapy: immune profiles and clinical outcomes. Mult Scler Relat Disord (2022) 67:104079. doi: 10.1016/j.msard.2022.104079
58. Kelly JD, Leonard S, Hoggatt KJ, Boscardin WJ, Lum EN, Moss-Vazquez TA, et al. Incidence of severe COVID-19 illness following vaccination and booster with BNT162b2, mRNA-1273, and Ad26.COV2.S vaccines. JAMA (2022) 328:1427–37. doi: 10.1001/jama.2022.17985
59. Khan N, Mahmud N. Effectiveness of SARS-CoV-2 vaccination in a Veterans Affairs cohort of patients with inflammatory bowel disease with diverse exposure to immunosuppressive medications. Gastroenterology (2021) 161:827–36. doi: 10.1053/j.gastro.2021.05.044
60. Liew J, GianFrancesco M, Harrison C, Izadi Z, Rush S, Lawson-Tovey S, et al. SARS-CoV-2 breakthrough infections among vaccinated individuals with rheumatic disease: results from the COVID-19 Global Rheumatology Alliance provider registry. RMD Open (2022) 8:e002187. doi: 10.1136/rmdopen-2021-002187
61. Malinis M, Cohen E, Azar MM. Effectiveness of SARS-CoV-2 vaccination in fully vaccinated solid organ transplant recipients. Am J Transplant. (2021) 21:2916–8. doi: 10.1111/ajt.16713
62. Pinana JL, Lopez-Corral L, Martino R, Vazquez L, Perez A, Martin-Martin G, et al. SARS-CoV-2 vaccine response and rate of breakthrough infection in patients with hematological disorders. J Hematol Oncol (2022) 15:54. doi: 10.1186/s13045-022-01275-7
63. Pino MS, Cheli S, Perna M, Fabbroni V, Giordano C, Martella F, et al. The national COVID-19 vaccination campaign targeting the extremely vulnerable: the Florence Medical Oncology Unit experience in patients with cancer. Eur J Cancer (2022) 170:149–57. doi: 10.1016/j.ejca.2022.04.008
64. Rooney A, Bivona C, Liu B, Streeter D, Gong H, Khan Q. Risk of SARS-CoV-2 breakthrough infection in vaccinated cancer patients: a retrospective cohort study. J Hematol Oncol (2022) 15:67. doi: 10.1186/s13045-022-01290-8
65. Sibbel S, McKeon K, Luo J, Wendt K, Walker AG, Kelley T, et al. Real-world effectiveness and immunogenicity of BNT162b2 and mRNA-1273 SARS-CoV-2 vaccines in patients on hemodialysis. J Am Soc Nephrol. (2022) 33:49–57. doi: 10.1681/ASN.2021060778
66. Yeo T, Quek AML, Yong KP, Tye JSN, Ratnagopal P, Soon DTL, et al. COVID-19 infection after two doses of SARS-CoV-2 mRNA vaccine in multiple sclerosis, AQP4-antibody NMOSD and MOGAD. Mult Scler Relat Disord (2022) 65:104003. doi: 10.1016/j.msard.2022.104003
67. Yetmar ZA, Bhaimia E, Bierle DM, Ganesh R, Razonable RR. Breakthrough COVID-19 after SARS-CoV-2 vaccination in solid organ transplant recipients: an analysis of symptomatic cases and monoclonal antibody therapy. Transpl. Infect Dis (2022) 24:e13779. doi: 10.1111/tid.13779
68. Sigal A, Milo R, Jassat W. Estimating disease severity of Omicron and Delta SARS-CoV-2 infections. Nat Rev Immunol (2022) 22:267–9. doi: 10.1038/s41577-022-00720-5
69. Zeng B, Gao L, Zhou Q, Yu K, Sun F. Effectiveness of COVID-19 vaccines against SARS-CoV-2 variants of concern: a systematic review and meta-analysis. BMC Med (2022) 20:200. doi: 10.1186/s12916-022-02397-y
70. Wang X, Haeussler K, Spellman A, Phillips LE, Ramiller A, Bausch-Jurken MT, et al. Comparative effectiveness of mRNA-1273 and BNT162b2 COVID-19 vaccines in immunocompromised individuals: a systematic review and meta-analysis using the GRADE framework. medRxiv (2023). doi: 10.1101/2023.04.05.23288195. Preprint posted online April 6.
Keywords: severe acute respiratory syndrome coronavirus 2, SARS-CoV-2, COVID-19, mRNA vaccine, mRNA-1273, BNT162b2, immunocompromised, effectiveness
Citation: Wang X, Haeussler K, Spellman A, Phillips LE, Ramiller A, Bausch-Jurken MT, Sharma P, Krivelyova A, Vats S and Van de Velde N (2023) Comparative effectiveness of mRNA-1273 and BNT162b2 COVID-19 vaccines in immunocompromised individuals: a systematic review and meta-analysis using the GRADE framework. Front. Immunol. 14:1204831. doi: 10.3389/fimmu.2023.1204831
Received: 12 April 2023; Accepted: 16 August 2023;
Published: 12 September 2023.
Edited by:
Morgane Solis, Hôpitaux Universitaires de Strasbourg, FranceReviewed by:
Dominique Bertrand, Centre Hospitalier Universitaire (CHU) de Rouen, FranceCopyright © 2023 Wang, Haeussler, Spellman, Phillips, Ramiller, Bausch-Jurken, Sharma, Krivelyova, Vats and Van de Velde. This is an open-access article distributed under the terms of the Creative Commons Attribution License (CC BY). The use, distribution or reproduction in other forums is permitted, provided the original author(s) and the copyright owner(s) are credited and that the original publication in this journal is cited, in accordance with accepted academic practice. No use, distribution or reproduction is permitted which does not comply with these terms.
*Correspondence: Nicolas Van de Velde, Tmljb2xhcy5WYW5kZVZlbGRlQG1vZGVybmF0eC5jb20=
Disclaimer: All claims expressed in this article are solely those of the authors and do not necessarily represent those of their affiliated organizations, or those of the publisher, the editors and the reviewers. Any product that may be evaluated in this article or claim that may be made by its manufacturer is not guaranteed or endorsed by the publisher.
Research integrity at Frontiers
Learn more about the work of our research integrity team to safeguard the quality of each article we publish.