- Pulmonary Oncology Research Team (New York University (NYU) PORT), Section of Interventional Pulmonology, Division of Pulmonary, Critical Care and Sleep Medicine, NYU Grossman School of Medicine, NYU Langone Health, New York, NY, United States
Despite remarkable advances in tumor response and patient survival in the past decade, systemic immunotherapies for lung cancer result in an objective response in only around half of patients treated. On the basis of this limitation, combination strategies are being investigated to improve response rates. Cryoablation has been proposed as one such technique to induce immunogenic cell death and synergize with systemic immunotherapies, including immune checkpoint inhibitors. Cryoablation has been traditionally delivered percutaneously with imaging guidance although recent technological advances allow for bronchoscopic delivery. Herein, we review the pre-clinical and clinical evidence for the use of cryoablation in non-small cell lung cancer and potential induction of anti-tumor immunity. We highlight ongoing studies involving this approach and propose areas of future investigation.
1 Introduction
Immune checkpoint inhibitors, including antibodies to programmed death cell death protein 1 (PD-1) and its ligand PD-L1 have revolutionized the treatment of patients with non-small cell lung cancer over the past decade. However, response rates to these therapies remain suboptimal even when the tumor demonstrates significant PD-L1 expression. Thus, combination immunotherapy approaches which use immune checkpoint inhibitors in combination with other therapeutic interventions have been proposed with the goal of improving overall clinical responses.
The cancer-immunity cycle has been proposed as a framework of seven steps required for the killing of cancer cells by the immune system (1). Specifically, the steps include: 1) release of tumor associated antigens and immunomodulatory danger signals, (2) cancer antigen presentation, (3) priming and activation of immune cells ,(4) trafficking and (5) infiltration of T cells into tumors, (6) recognition of cancer cells by T cells, and (7) immune-mediated cell death leading to further tumor antigen and danger signal release.. Each of these steps has been targeted by investigators for its potential to combine with current immunotherapies to improve response rates.
Release of cancer cell antigens is pivotal to generation of an anti-tumor immune response and may be augmented in several ways. For example, local treatments (tumor ablation, intratumoral injection, radiotherapy) and systemic therapies have been proposed as strategies to increase tumor antigen release and presentation to the immune system. Additionally, an increasing body of evidence suggests that immunogenic cell death may sensitize patients to PD-1/PD-L1 blockade (2). One particular local therapy, cryoablation, has been studied for its ability to generate an anti-tumor immune response and may have advantages over several of the other proposed methods.
In this review, we will discuss the proposed immunologic mechanisms of cryoablation and highlight pre-clinical and clinical investigations using cryoablation as part of a combination immunotherapy strategy.
2 Mechanisms of cryoablation
Cryoablation is a thermal ablation technique in which cold temperature is used to induce tissue injury. Although the therapeutic properties of cold have been utilized for centuries, the modern era of cryosurgery began in the 1960s when the neurosurgeon Dr. Irving Cooper developed a modern cryosurgical system using a probe for treatment of brain tumors and movement disorders (3). Since then, cryoablation has been utilized for local treatment of several malignancies including liver, kidney, breast, prostate, lung and bone (4).
To achieve low temperatures at the tip of the probe, a compressed gas (i.e., cryogen) is passed through a valve where it rapidly expands, resulting in cooling via the Joule-Thomson effect. A variety of cryogens are utilized clinically including liquid nitrogen, argon, and carbon dioxide, which produce a range of cold temperatures at the tip of the probe within seconds of application (5). By direct application of this temperature to target tissues, tissue injury and cell death are locally induced. It is postulated that this tissue injury in turn leads to antigen presentation and the development of an antitumor immune response both locally and at distant sites (7). This will be further discussed in Section 6, Immune Response to Cryoablation.
Cryoablation results in cell death via an immediate direct cell injury, as well as delayed effects due to vascular thrombosis and potentially immune-mediated pathways (5). First, the direct application of cold damages target tissues and causes crystallization of extracellular water, leading to a hyperosmotic extracellular space resulting in osmotic injury to cells. With application of colder temperatures, direct intracellular crystallization impairs function of membranes and organelles, leading to cellular dysfunction and death. Following this direct effect, microvascular stasis occurs resulting in thrombosis, local ischemia and additional cell injury. While cells not subjected to lethal injury may be able to recover, temperatures of -20°C to -50°C may induce complete necrosis, which notably depends on the nature of the frozen tissue (6). For example, neoplastic cells may be more resistant to lethality from cold temperature than benign tissues. The effects of cold injury depend on many factors, including the tissue frozen, rapidity and duration of freeze and thaw cycles, number of freeze-thaw cycles (4, 5).
The optimal “ablative dose” is not known and many have investigated different protocols to optimize cryosurgical techniques (8). Thus, several authors have proposed a target temperature of -40 C for at least one minute in order to achieve a lethal effect in neoplastic tissues (4). In general, a rapid cooling phase, followed by a slow thaw phase, and repeated freeze-thaw cycles increase the amount of cell death with cryoablation (4). In a model of pulmonary cryoablation, three freeze-thaw cycles were suggested to be superior to two cycles for overall efficiency and visualization of the cryoablation zone. In particular, the first freeze-thaw cycle may improve thermal conductivity of lung tissue and tumor to amplify the freeze effect during subsequent cycles (9). Further investigation is needed to determine optimal ablation protocols.
3 Cryoablation versus other thermal ablative modalities
In comparison with other modalities of image-guided thermal ablation such as radiofrequency ablation (RFA) and microwave ablation (MWA), cryoablation offers several possible advantages as part of an immune stimulating strategy. Certain tissues have an intrinsic cryo-resistance due to low water content (including cartilage, a component of the bronchial wall) which may minimize damage to normal tissues surrounding a tumor (10). Thus, cryoablation is the preferred image-guided ablation technique for tumors near the large airways, diaphragm or pleura (11). A meta-analysis comparing thermal ablative therapies for lung cancer reported no statistically significant differences in adverse reactions between cryoablation, radiofrequency ablation (RFA), and microwave ablation (MWA) (12). Additionally, cryoablation may be less painful that other thermal modalities as cold temperatures have an analgesic effect on tissues (13). Finally, cryoablation differs from RFA and MWA in that it does not produce extreme heat which is known to denature tumor antigens, and therefore may better preserve tumor antigens for processing by the immune system.
4 Current uses of cryoablation in thoracic malignancies
4.1 Percutaneous cryoablation
Percutaneous cryoablation is an image-guided thermal ablation technique which is used for the treatment of early-stage peripheral lung cancer, multiple primary lung cancers, or metastatic disease to the lung, typically in medically inoperable patients. Additionally, image-guided ablation has been used for recurrent disease or symptomatic tumor invasion of the chest wall. The major benefits over surgery include sparing of lung parenchyma, with pulmonary function testing post tumor cryoablation revealing minimal to no sustained reduction in FEV1 or DLCO (14). Several societal guidelines support its use as an alternative to radiotherapy in patients who are “high risk,” defined as medically inoperable or borderline operable (14, 15).
A number of studies over the past decade have revealed safety and efficacy of percutaneous cryoablation (16–23). Pneumothorax is the most common complication, requiring chest tube placement in around 20% of patients (11, 24). The incidence of severe adverse events (defined as grade 3 or higher according to the National Cancer Institute Common Terminology Criteria for Adverse Events) is low, occurring in around 6% of patients (18, 20, 21). Rarely, bronchopleural fistula or hemorrhage may occur.
4.2 Bronchoscopic cryoablation
Bronchoscopic cryoablation is a technique used for destruction of tissue within the airways. Most commonly, it is applied for palliative treatment of malignant airway obstruction (25, 26). Bronchoscopic cryoablation has traditionally been applied by direct application of a cryoprobe via several freeze-thaw cycles. Recently, spray cryotherapy has been investigated as another delivery method (27). Bronchoscopic cryoablation has also been utilized for the curative-intent treatment of endobronchial malignancy in patients not eligible for surgical resection (27). This technique has primarily been utilized in superficial malignancies involving the central airways including carcinoma in situ and carcinoid tumors with demonstrated safety and clinical efficacy (28–30).
Transbronchial cryoablation for therapeutic intervention with curative intent of inoperable peripheral lung tumors is being investigated, but remains limited to research settings only (31–34). The techniques of transbronchial cryobiopsy and cryo-recanalization are not discussed here as the technique does not leave sufficient frozen tissue in situ for processing by the immune system and is thus postulated not to have the same immunomodulatory effect.
5 Delivery approaches for cryoablation as a combination immunotherapy
5.1 Percutaneous
There is accumulating clinical evidence for the use of percutaneous cryoablation for non-small cell lung cancer, although the procedure is currently performed only at specialized centers in the United States. For thoracic indications, imaging guidance is typically performed with computed tomography allowing precise lesion targeting. Percutaneous probes commonly use argon for cooling and helium for rewarming, giving them the ability to reach temperatures of as low as -140°C at the probe tip (35). Commonly used probe sizes for lung ablation range from 1.5 to 2.5 mm in diameter, and a single probe has the capacity to create an ablation zone (defined by a measured -20°C isotherm) of up to 40 mm in largest dimension depending on probe size (13). Cryoablation has the benefit of improved visualization of the ablation zone when compared to other thermal ablative methods (17). This allows for placement of several cryoprobes to increase the cryoablation zone to completely encapsulate a lesion.
Negative aspects of the percutaneous approach include breach of the visceral pleura, which is associated with a risk of complications. In fact, pneumothorax may occur in up to 50% of percutaneous cryoablation procedures, requiring chest tube placement in about 20% (11, 24). Some have suggested that tract embolization with gelatin sponge slurry may reduce the incidence of pneumothorax after radiofrequency ablation of lung tumors (36), but the quality of evidence is limited and we could not find any reports of its use with percutaneous cryoablation. Thus, alternative approaches to cryoablation of lung tumors have been sought which do not cross the visceral pleura.
5.2 Bronchoscopic
Bronchoscopic cryoablation has been postulated as a potential way to reduce risks of cryoablation for lung tumors as the cryoprobe can be directed within the airways and does not cross the visceral pleura. With improving bronchoscopic navigation technologies and confirmation of probe positioning with cone-beam CT, bronchoscopic cryoablation may soon be feasible. Bronchoscopic cryoablation may also allow for access to multiple intrathoracic thoracic lesions, including the future potential for treatment of nodal metastasis.
A disadvantage of bronchoscopic cryoablation is the challenge of encapsulating the entire lesion within the ablation zone, as cryoprobes delivered through an airway are not as easily positioned as percutaneous probes under CT guidance. Additionally, currently available flexible cryoprobes have inferior ablation zones compared to rigid percutaneous probes. Rigid probes may allow for shortening of the thawing phase, therefore shortening procedure time, by a mechanism that prevents decompressed gas from returning into the cryoprobe. On the other hand, longer thawing time may assist in cellular injury, which could be enhanced with the flexible cryoprobe (27). Although rigid cryoprobes can be used through a rigid bronchoscope, these are solely for endoluminal lesions and the reach of these probes is limited mostly to central airways and proximal lower lobes.
Preclinical studies using a cryoprobe for bronchoscopic delivery of cryoablation have shown feasibility. Kohno et al. demonstrated the feasibility of transbronchial cryoablation in a porcine model using a rigid cryoprobe (31). Temperature measurements during the cryoablation and histologic evaluation of the ablation zone after animal sacrifice were performed. Although the rigid cryoprobe is unable to reach many peripheral lung lesions, its technical feasibility and lack of serious adverse effects in the ablation zone were demonstrated.
Subsequently, in an ex vivo model utilizing an explanted porcine lung, Zheng et al. used a prototype 2.3 mm flexible cryoprobe through a bronchoscope to evaluate feasibility of transbronchial cryoablation (37). This specially designed cryoprobe reaches a temperature of nearly -160 C at the tip using liquid nitrogen as the cryogen. In this preclinical study, the probe tip created an ice ball of up to 3.5 cm in diameter in porcine lung and the target temperature was reached close to the cryoprobe. Specifically, a threshold temperature of -20°C or less was reached within 12 mm from the cryoprobe. A further study by this group demonstrated the feasibility of transbronchial cryoablation in an in vivo porcine model (33). Another research group used a commercially available 2.4 mm flexible bronchoscopic cryoprobe (ERBECRYO2; Erbe Elektromedizin GmbH, Tubingen, Germany) to ablate a gelatin model of lung tissue (34). Although the pre-specified ablation temperature (-20°C) was reached after 15 minutes with a double freeze protocol, the ablation zone was small due to the limited ablation effect of this carbon dioxide-based probe, which can only reach temperatures as low as -79°C. Thus, further work is needed to develop bronchoscopic devices which may provide an enhanced ablative effect.
An additional potential benefit of the bronchoscopic cryoablation is the ability to combine with standard of care diagnostic or therapeutic bronchoscopy procedures. Our institution is currently recruiting for a safety and feasibility trial of bronchoscopic cryo-immunotherapy (BCI) for non-small cell lung cancer (ClinicalTrials.gov identifier NCT04049474). This phase I study is designed to evaluate the safety, feasibility and preliminary systemic immune response to dose-escalated cryoablation of a peripheral lung tumor delivered at the time of diagnostic or therapeutic bronchoscopy for known or suspected advanced non-small cell lung cancer. Dosing in this trial is determined by freeze time with standardization of the optimal freeze temperature achievable with standard flexible cryoprobes. This study utilizes a radial ultrasound probe (UM-S20-17S; Olympus, Tokyo, Japan) via a guide sheath to confirm as close as possible to a concentric view of the peripheral lesion prior to insertion of the cryoprobe under fluoroscopic guidance. The maximal freezing time in this current study design is 30 seconds per centimeter of tumor, which is a far shorter duration than was achieved in the porcine studies. It is possible that longer freeze durations will be necessary for optimal tumor cell killing and antigen presentation. Additionally, the protocol allows for usage of robotic bronchoscopy for localization of the peripheral lesion and to facilitate cryoablation of target tumors using the 1.1 mm diameter flexible cryoprobe (ERBECRYO2; Erbe Elektromedizin GmbH, Tubingen, Germany). The robotic bronchoscope’s working channel allows for passage of the 1.1 mm probe without the need for insertion of a guide sheath. The shape-sensing technology implemented in the robotic bronchoscope (Ion Endoluminal System; Intuitive Surgical, Sunnyvale, California), allows for stable positioning of the cryoprobe within the lesion despite the device exchanges of the biospy forceps and the radial ultrasound probe beforehand.
There have been several studies published in the medical literature of the use of robotic bronchoscopy for cryobiopsy of peripheral lung lesions (38, 39), but no published studies have demonstrated the use of the 1.1 mm flexible cryoprobe for therapeutic purposes in patients with lung cancer. In addition, the use of advanced fluoroscopy and/or cone-beam computed tomography allows for confirmation of the presence of the cryoprobe within the target lesion to optimize the cryoablation procedure. In the current protocol, the immune response to BCI is determined solely by assessment of peripheral blood samples taken just prior to the study procedure and at 7 and 14 days post-procedure. In future iterations of BCI, we aim to obtain repeat tumor biopsies and endobronchial ultrasound (EBUS)-guided nodal aspirates after several weeks to be able to better discern the effects of BCI on the tumor and draining lymph node immune microenvironments.
Recently, several studies have investigated the use of the EBUS-guided cryobiopsy of mediastinal and hilar lymph nodes to obtain larger biopsy specimens for improved diagnosis of disorders such as lymphoma and sarcoidosis (40, 41). The procedure involves first accessing the target node using the linear EBUS bronchoscope and large biopsy needle or needle-knife. Subsequently, the 1.1 mm cryoprobe is advanced through the working channel and into the lymph node under EBUS guidance with freezing and cryoadhesion of nodal tissue. In the future, there may be an immunologic role for therapeutic cryoablation of tumor-involved lymph nodes. Induction of cell necrosis via cryoablation within the nodal environment may facilitate enhanced tumor antigen presentation to naïve CD8 T cells and increased production of tumor-directed cytotoxic T cells which can then enter the systemic circulation. Furthermore, the potential for nodal cryoablation to work in synergy with immune checkpoint inhibitors and combat immune checkpoint inhibitor resistance was explored in a published case of aortocaval lymph nodal metastasis from immunotherapy-resistant non-small cell lung cancer which was treated successfully with percutaneous cryoablation while continuing the immune checkpoint inhibitor (42). It remains to be studied whether such effects are reproducible, and whether they can be extrapolated to nodal ablation via EBUS.
6 Immune response to cryoablation
Studies dating back to the 1960s have suggested a potential immune response to cryoablation. Specifically, it was initially recognized that cryoablation caused formation of auto-antibodies against the frozen tissues (43). Characterization of the immune response was limited by the laboratory techniques at the time. However, there were several case reports in the 1970s of regression of distant lesions (“abscopal” effect) following cryoablation of prostate tissue (44, 45). Since then, technologies for assessment of the immune response have improved and allowed for further investigation into potential mechanisms of immune response to cryoablation, although the understanding remains incomplete.
Much of the data on immune response to cryoablation are based on pre-clinical models. Briefly, after cryoablation there is an influx of acute inflammatory cells to the cryoablation zone followed by antigen presenting cells. Dying cells release a variety of pro-inflammatory cytokines (e.g., IL-12, IFN-gamma and TNF-alpha) from necrotic tissues at the center of the ablation zone, which serve as “danger signals” to alert the immune system of cellular injury. Antigen presenting cells take up cellular debris including key tumor antigens and then migrate to regional lymph nodes where they interact with T and B-lymphocytes to initiate a cellular and/or humoral immune response (Figure 1). It is important to note that cells at the periphery of the ablation zone, where temperatures are not sufficient to induce necrosis, may undergo apoptosis, potentially resulting in release of immune suppressive cytokines (e.g., IL-10, TGF-beta) (7).
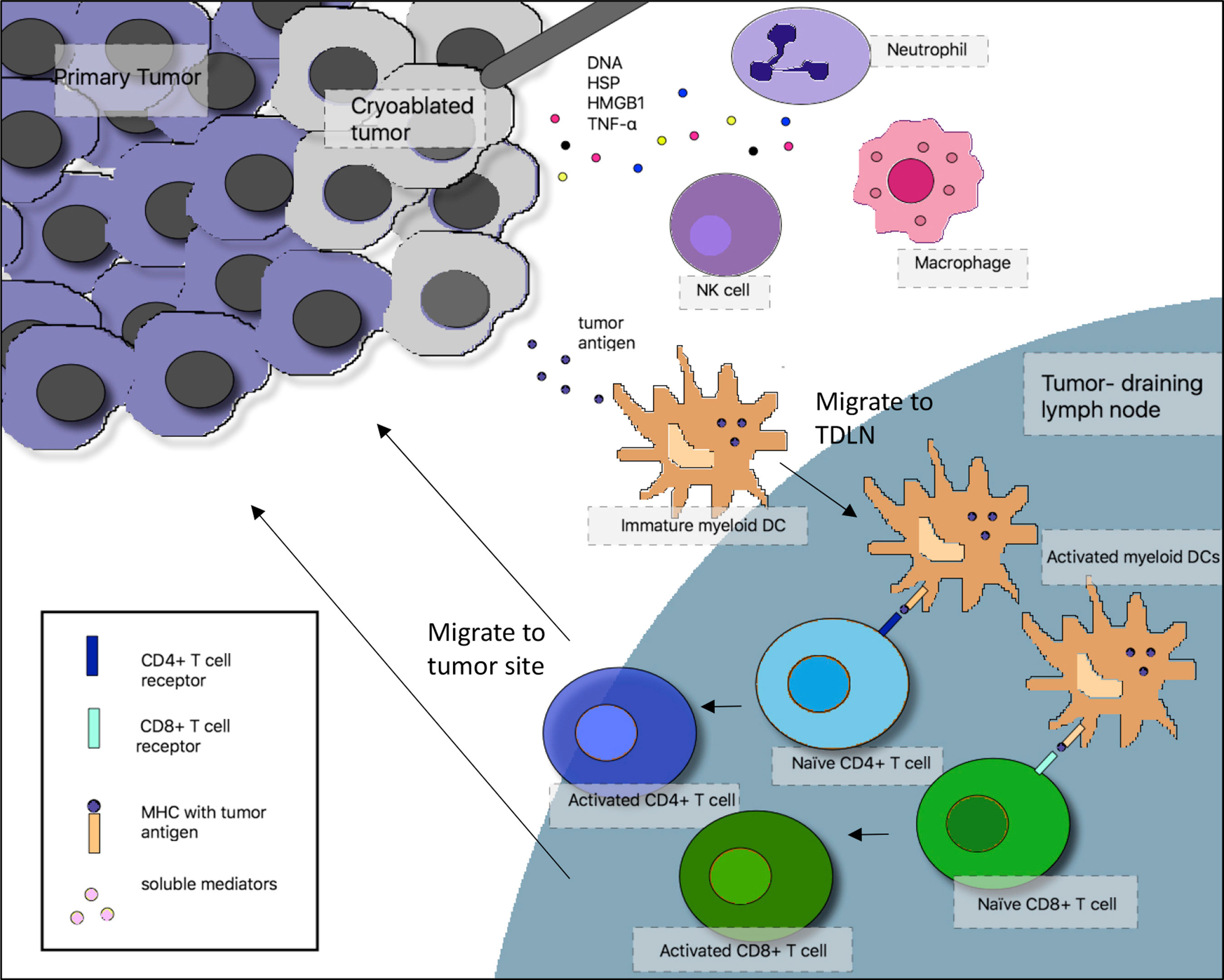
Figure 1 Interactions between the cryoablated tumor and the adaptive and innate immune system. In the proposed mechanism of cryoimmunotherapy, cryoablated tumor cells release tumor antigens, which are taken up by immature myeloid dendritic cells (DCs). In the context of necrotic cell death and generation of inflammatory cytokines after cryoablation, DCs mature and migrate to tumor-draining lymph nodes (TDLN), where they activate tumor-specific T cells, which migrate back to the tumor site. At the same time, dying tumor cells release “danger signals” that recruit macrophages, neutrophils, and natural killer (NK) cells to the tumor site. NK cells can play a direct role in tumor cell lysis. HSP, heat shock protein, HMGB1, high mobility group box 1; MHC, major histocompatibility complex; TNF-α, tumor necrosis factor-α.
Subsequent to cryoablation, anti-tumor antibodies may be formed against ablated tissues, as demonstrated in several pre-clinical cancer models (46–48). It is thought that these antibodies are a marker of an anti-tumor immune response, due to their specificity to tumor tissues. Additionally, murine models demonstrated resistance to re-challenge by the same tumor (but not different tumor lines) after cryoablation which may be due to both humoral- and cell-mediated immunity. Furthermore, the resistance to tumor challenge was demonstrated to be tumor specific (49). Further details of pre-clinical studies of the immunological effects of tumor cryoablation has been covered in detail in previously published manuscripts (7). It is not known how some of these mechanisms may interact with immunotherapies, including immune checkpoint inhibitors.
Notably, not all studies have demonstrated an anti-tumor immune response to cryoablation. In fact, some investigations have suggested an immunosuppressive effect of cryoablation (50–52). Sabel and colleagues proffered that the immune response to cryoablation is dependent upon the cytokine profile triggered by cryoablation, the availability of antigens that can be processed by antigen presenting cells, the mechanism of cell death (specifically necrosis versus apoptosis), and the subsets of phagocytes that process ablated cells (i.e., dendritic cells versus macrophages) (7). Further investigation is needed to understand mechanisms of immune response following cryoablation.
7 Studies of cryoablation and immunity in non-small cell lung cancer
Multiple pre-clinical and clinical studies in non-small cell lung cancer have suggested positive outcomes with cryoablation. Several of these studies are have been reported in a previously published review by our group (53). A list of published studies involving cryoablation for the induction of immune response (i.e., cryo-immunotherapy) are included in Table 1.
Additionally, more recently Xu et al. studied the clinical outcomes and immune response of patients with lung cancer who received systemic therapies with or without bronchoscopic cryoablation for endobronchial disease (60). Bronchoscopic cryotherapy was applied via cryoprobe via a single freeze-thaw cycle lasting 3 to 4 minutes. Improved response rate was noted in the cryoablation arm and there was suggestion of decreased serum tumor markers (e.g., carcinoembryonic antigen) and changes in CD4 and CD8 T-cell subsets in the peripheral blood following cryotherapy. It is important to note that the methodology in this study was limited by absence of standardization of systemic therapy, and that patients in this study received systemic chemotherapy or molecular targeted therapy rather than immunotherapy. However, the concept of combining cryoablation, in this study delivered bronchoscopically, with systemic therapies – particularly immune checkpoint inhibitors – is an approach that warrants further investigation.
8 Cryoablation in combination with immune-checkpoint inhibitors
There are theoretical benefits of combining cryoablation with immune checkpoint inhibitors, as they work on different elements of anti-tumor immunity. Based on a proposed mechanism, releasing tumor antigens while the immune system is under the influence of immune checkpoint blockade may prevent T-cell exhaustion or anergy and reinstate anti-tumor immunity (Figure 2). At least one case report has demonstrated a robust clinical response due to the combination of bronchoscopic cryoablation and camrelizumab, a humanized monoclonal antibody against programmed cell death protein 1 (PD-1), in a patient with advanced sarcomatoid carcinoma of the lung (32).
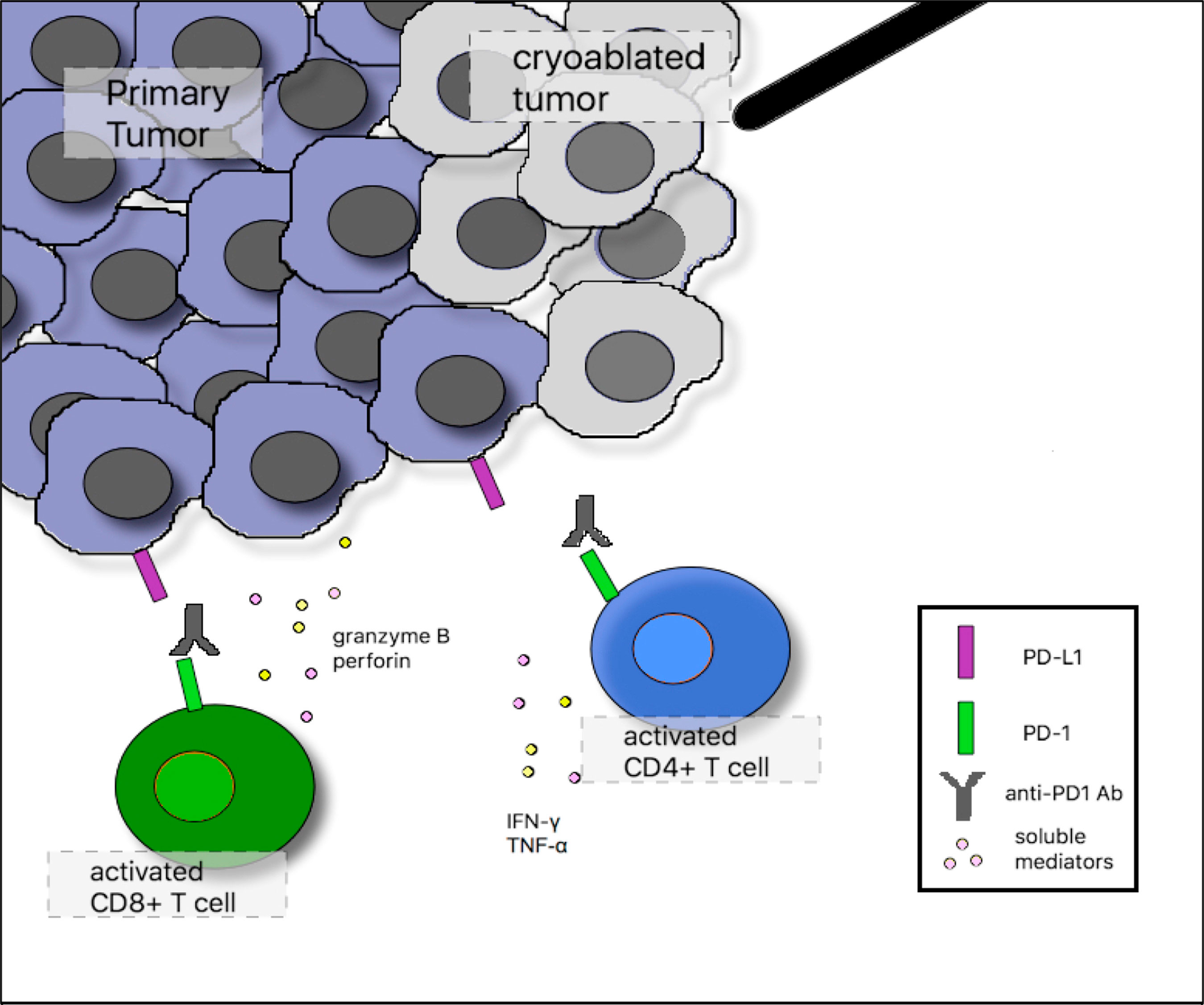
Figure 2 Proposed mechanism of synergy between cryoablation and anti–programmed cell death 1 (PD-1) monoclonal antibodies. In the tumor microenvironment after cryoablation, activated CD4-positive T cells augment immune responses through release of the proinflammatory cytokines interferon gamma (IFN-γ) and tumor necrosis factor-α (TNF-α). Activated CD8-positive T cells induce tumor cell lysis through release of granzyme B and perforin. Tumor cells can inhibit infiltrating tumor-specific T cells by up-regulating programmed death ligand 1 (PD-L1) surface expression, which binds PD-1 on the surface of activated T cells. Anti–PD-1 monoclonal antibodies block this inhibitory interaction and sustain T-cell activation.
Leppelman et al. performed a single-center retrospective cohort study evaluating the safety of locoregional therapies in patients receiving systemic immune checkpoint inhibitors for several malignancies including non-small cell lung cancer (12 of 65 patients) (58). Notably, locoregional therapies included thermal ablation, embolization, or selective internal radiation therapy, with cryoablation accounting for 28% of procedures. Immune-related adverse events occurred in 10.7% of patients, all less than or equal to grade 3, with none occurring in those with non-small cell lung cancer. It is important to recognize the potential for adverse effects from combining systemic and locoregional therapies. At least in this experience, there were no safety signals for combination therapies noted with cryoablation.
Feng et al. investigated the safety and efficacy of percutaneous cryoablation with or without nivolumab in patients with stage IIIB or IV non-small cell lung cancer (59). The combination of cryoablation with nivolumab versus nivolumab alone, was associated with an increase in the number of immune effector cells such as total CD4+ and CD8+ T cells and natural killer (NK) cells and the serum levels of inflammatory cytokines (i.e., IL-2, TNF-beta, IFN-gamma) in the cryotherapy plus nivolumab group. Adverse events were all less than or equal to grade 3 and without significant differences among the two groups. The combination of nivolumab with cryoablation resulted in significantly reduced circulating tumor cells and decreases in the tumor markers CYFRA21-1 and neuron-specific enolase (NSE). This study was limited by its retrospective nature and unconventional treatment strategies.
Further data regarding the safety and efficacy of the combination of cryoablation with immune-checkpoint inhibitors is needed. Several studies are currently investigating cryoablation in combination with systemic immunotherapies in non-small cell lung cancer (see Table 2). Additionally, studies which characterize both the tumor microenvironment and peripheral immune cells before and after cryoablation will be crucial to further understanding potential mechanisms of synergy.
9 Discussion
Historically, cryoablation has been a means of local tumor control in patients who are unfit for surgery. However, there is growing interest in cryoablation as a means to induce an immunologic effect which is specific to the ablated tumor. With recent development of immunotherapies for lung cancer, cryoablation has entered a new era, with the potential to synergize with systemic checkpoint inhibitors. Several important questions arise regarding the role of cryoablation in non-small cell lung cancer based on this review of recent literature.
First, what is the optimal ablative dose and number of lesions to target? Despite increasing knowledge and experience with various cryoablation protocols, knowledge of the best strategy remains limited. As multiple studies have suggested that immune response is variable after cryoablation (61, 62), determining the most effective dose for immune stimulation (which may be different from achieving maximal cell death) is of utmost importance.
Second, is there any role for cryoablation of tumor-draining lymph nodes? Although at present in limited capacity, some have postulated nodal cryoablation as a means to combat immune checkpoint inhibitor resistance. Thoracic lymph nodes in non-small cell lung cancer may be readily accessible for EBUS-mediated bronchoscopic cryoablation, and may be therefore present an opportunity for future investigation into this topic.
Additionally, is there any potential neoadjuvant role of cryoablation and immunotherapy prior to resection of early stage non-small cell lung cancer? Impressive pathological responses have been observed with neoadjuvant checkpoint inhibitors prior to resection (63), and the addition of cryoablation to such a regimen at the time of bronchoscopy could theoretically further enhance this effect, particularly in those lung cancers with “cold” baseline immune microenvironments (low PD-L1 expression and absence of infiltrating T cells).
Accumulating evidence suggests that cryoablation may generate an anti-tumor immune response at the cellular level by potentiating the cancer-immunity cycle, especially when used in combination with other immunotherapies. At the clinical level, limited data suggest a potential improvement in disease regression and progression-free survival when combination of therapy and cryoablation is used. More clinical studies are needed to further determine how cryoimmunotherapy translates to clinical outcomes.
Due to technical limitations, cryoablation has largely been performed via percutaneous approach, although recent progress in the field of interventional pulmonology may allow for routine bronchoscopic cryoablation in the near future (Figure 3). Additionally, further research into discovering optimal dose and mechanisms of cryoablation will be essential to maximize this potential therapy.
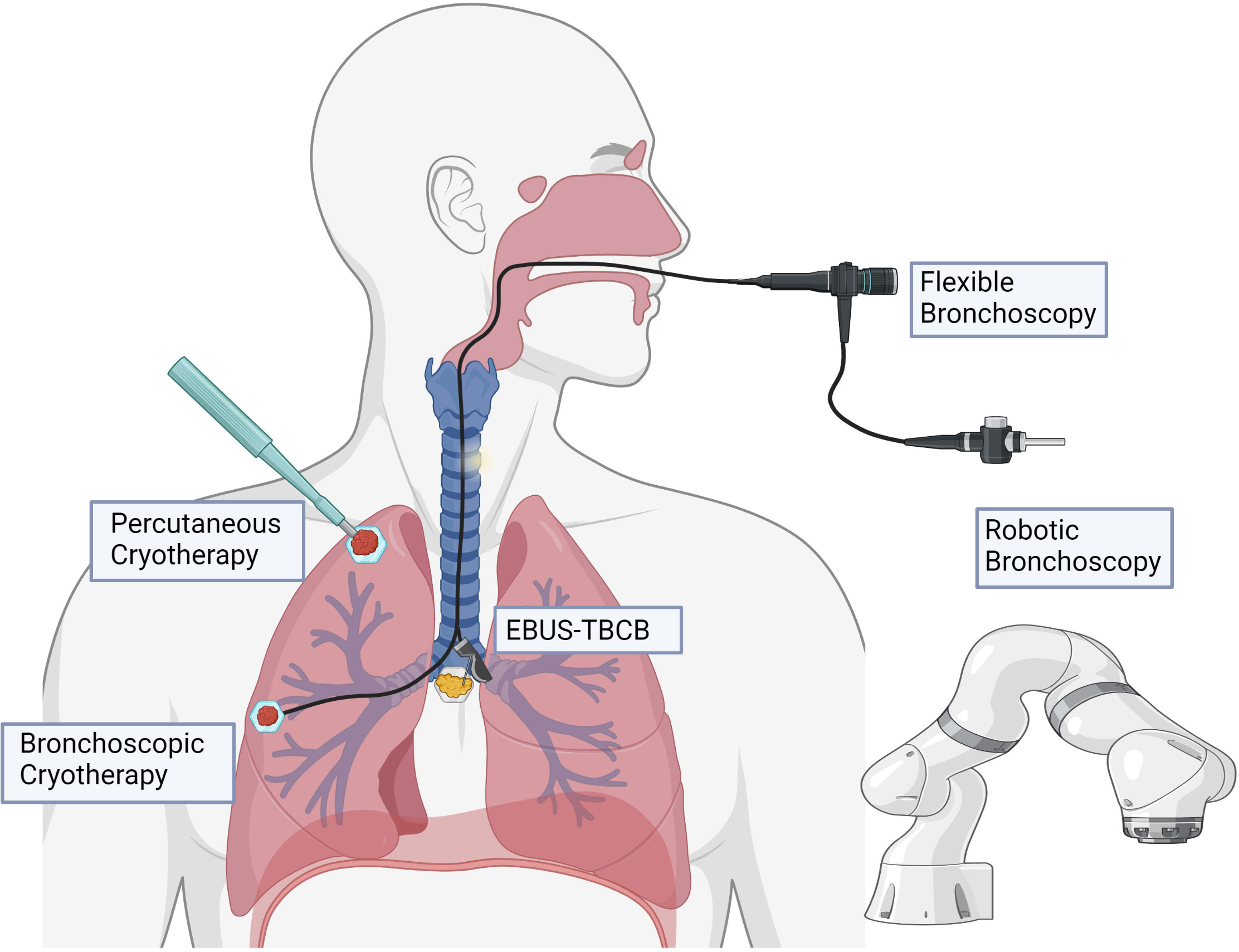
Figure 3 Potential modalities for delivery of cryotherapy. Percutaneous cryoablation is typically delivered with image guidance (e.g., computed tomography). Bronchoscopic cryotherapy can be delivered to endoluminal lesions in proximal and segmental airways under direct visualization, as well as to more peripheral lesions with the assistance of robotic bronchoscopy. Endobronchial ultrasound guidance may allow for potential to target tumor-draining lymph nodes in the mediastinum.
Author contributions
AV, AD, and DS designed this study. AV performed the initial literature search and wrote the first draft of the manuscript. AD and DS revised the manuscript and provided additional sources. All authors contributed to the article and approved the submitted version.
Funding
Publication fees for this article were supplied by a National Institute of Health (NIH) Exploratory/Developmental Research Grant (R21CA229714-01A1). The funding for this manuscript was provided by the Pulmonary, Critical Care and Sleep Medicine Division at NYU Grossman School of Medicine.
Acknowledgments
We offer special thanks to Dr. Leopoldo Segal for his assistance with the development of Figure 3 of this manuscript.
Conflict of interest
The authors declare that the research was conducted in the absence of any commercial or financial relationships that could be construed as a potential conflict of interest.
Publisher’s note
All claims expressed in this article are solely those of the authors and do not necessarily represent those of their affiliated organizations, or those of the publisher, the editors and the reviewers. Any product that may be evaluated in this article, or claim that may be made by its manufacturer, is not guaranteed or endorsed by the publisher.
References
1. Chen DS, Mellman I. Oncology meets immunology: the cancer-immunity cycle. Immunity (2013) 39(1):1–10. doi: 10.1016/j.immuni.2013.07.012
2. Kepp O, Zitvogel L, Kroemer G. Clinical evidence that immunogenic cell death sensitizes to PD-1/PD-L1 blockade. Oncoimmunology (2019) 8(10):e1637188. doi: 10.1080/2162402X.2019.1637188
3. Cooper IS, Lee AS. Cryostatic congelation: a system for producing a limited, controlled region of cooling or freezing of biologic tissues. J Nerv Ment Dis (1961) 133:259–63. doi: 10.1097/00005053-196109000-00013
4. Baust JG, Gage AA, Bjerklund Johansen TE, Baust JM. Mechanisms of cryoablation: clinical consequences on Malignant tumors. Cryobiology (2014) 68(1):1–11. doi: 10.1016/j.cryobiol.2013.11.001
5. Gage AA, Baust J. Mechanisms of tissue injury in cryosurgery. Cryobiology (1998) 37(3):171–86. doi: 10.1006/cryo.1998.2115
6. Rubinsky B. Cryosurgery. Annu Rev BioMed Eng (2000) 2:157–87. doi: 10.1146/annurev.bioeng.2.1.157
7. Sabel MS. Cryo-immunology: a review of the literature and proposed mechanisms for stimulatory versus suppressive immune responses. Cryobiology (2009) 58(1):1–11. doi: 10.1016/j.cryobiol.2008.10.126
8. Baust JG, Gage AA. Progress toward optimization of cryosurgery. Technol Cancer Res Treat (2004) 3(2):95–101. doi: 10.1177/153303460400300202
9. Hinshaw JL, Littrup PJ, Durick N, Leung W, Lee FT Jr., Sampson L, et al. Optimizing the protocol for pulmonary cryoablation: a comparison of a dual- and triple-freeze protocol. Cardiovasc Intervent Radiol (2010) 33(6):1180–5. doi: 10.1007/s00270-010-9868-0
10. Zhao ZR, Lau RWH, Ng CSH. Catheter-based alternative treatment for early-stage lung cancer with a high-risk for morbidity. J Thorac Dis (2018) 10(Suppl 16):S1864–S70. doi: 10.21037/jtd.2018.03.151
11. Murphy MC, Wrobel MM, Fisher DA, Cahalane AM, Fintelmann FJ. Update on image-guided thermal lung ablation: society guidelines, therapeutic alternatives, and postablation imaging findings. AJR Am J Roentgenol (2022) 219(3):471–85. doi: 10.2214/AJR.21.27099
12. Jiang B, McClure MA, Chen T, Chen S. Efficacy and safety of thermal ablation of lung Malignancies: A Network meta-analysis. Ann Thorac Med (2018) 13(4):243–50. doi: 10.4103/atm.ATM_392_17
13. Erinjeri JP, Clark TW. Cryoablation: mechanism of action and devices. J Vasc Interv Radiol (2010) 21(8 Suppl):S187–91. doi: 10.1016/j.jvir.2009.12.403
14. Genshaft SJ, Suh RD, Abtin F, Baerlocher MO, Chang AJ, Dariushnia SR, et al. Society of interventional radiology multidisciplinary position statement on percutaneous ablation of non-small cell lung cancer and metastatic disease to the lungs: endorsed by the canadian association for interventional radiology, the cardiovascular and interventional radiological society of europe, and the society of interventional oncology. J Vasc Interv Radiol (2021) 32(8):1241.e1–e12. doi: 10.1016/j.jvir.2021.04.024
15. Ettinger DS, Wood DE, Aisner DL, Akerley W, Bauman JR, Bharat A, et al. National Comprehensive Cancer Network Clinical Practice Guidelines in Oncology. In: Non-Small Cell Lung Cancer (Version 2.2023).
16. Yamauchi Y, Izumi Y, Kawamura M, Nakatsuka S, Yashiro H, Tsukada N, et al. Percutaneous cryoablation of pulmonary metastases from colorectal cancer. PloS One (2011) 6(11):e27086. doi: 10.1371/journal.pone.0027086
17. Inoue M, Nakatsuka S, Yashiro H, Ito N, Izumi Y, Yamauchi Y, et al. Percutaneous cryoablation of lung tumors: feasibility and safety. J Vasc Interv Radiol (2012) 23(3):295–302. doi: 10.1016/j.jvir.2011.11.019
18. Moore W, Talati R, Bhattacharji P, Bilfinger T. Five-year survival after cryoablation of stage I non-small cell lung cancer in medically inoperable patients. J Vasc Interv Radiol (2015) 26(3):312–9. doi: 10.1016/j.jvir.2014.12.006
19. McDevitt JL, Mouli SK, Nemcek AA, Lewandowski RJ, Salem R, Sato KT. Percutaneous cryoablation for the treatment of primary and metastatic lung tumors: identification of risk factors for recurrence and major complications. J Vasc Interv Radiol (2016) 27(9):1371–9. doi: 10.1016/j.jvir.2016.04.005
20. de Baere T, Tselikas L, Woodrum D, Abtin F, Littrup P, Deschamps F, et al. Evaluating cryoablation of metastatic lung tumors in patients–safety and efficacy: the ECLIPSE trial–interim analysis at 1 year. J Thorac Oncol (2015) 10(10):1468–74. doi: 10.1097/JTO.0000000000000632
21. Callstrom MR, Woodrum DA, Nichols FC, Palussiere J, Buy X, Suh RD, et al. Multicenter study of metastatic lung tumors targeted by interventional cryoablation evaluation (SOLSTICE). J Thorac Oncol (2020) 15(7):1200–9. doi: 10.1016/j.jtho.2020.02.022
22. de Baere T, Woodrum D, Tselikas L, Abtin F, Littrup P, Deschamps F, et al. The ECLIPSE study: efficacy of cryoablation on metastatic lung tumors with a 5-year follow-up. J Thorac Oncol (2021) 16(11):1840–9. doi: 10.1016/j.jtho.2021.07.021
23. Kawamura M, Izumi Y, Tsukada N, Asakura K, Sugiura H, Yashiro H, et al. Percutaneous cryoablation of small pulmonary Malignant tumors under computed tomographic guidance with local anesthesia for nonsurgical candidates. J Thorac Cardiovasc Surg (2006) 131(5):1007–13. doi: 10.1016/j.jtcvs.2005.12.051
24. Genshaft SJ, Suh RD, Abtin F, Baerlocher MO, Dariushnia SR, Devane AM, et al. Society of interventional radiology quality improvement standards on percutaneous ablation of non-small cell lung cancer and metastatic disease to the lungs. J Vasc Interv Radiol (2021) 32(8):1242.e1–e10. doi: 10.1016/j.jvir.2021.04.027
25. Maiwand MO, Asimakopoulos G. Cryosurgery for lung cancer: clinical results and technical aspects. Technol Cancer Res Treat (2004) 3(2):143–50. doi: 10.1177/153303460400300207
26. Asimakopoulos G, Beeson J, Evans J, Maiwand MO. Cryosurgery for Malignant endobronchial tumors: analysis of outcome. Chest (2005) 127(6):2007–14. doi: 10.1378/chest.127.6.2007
27. DiBardino DM, Lanfranco AR, Haas AR. Bronchoscopic cryotherapy. Clinical applications of the cryoprobe, cryospray, and cryoadhesion. Ann Am Thorac Soc (2016) 13(8):1405–15. doi: 10.1513/AnnalsATS.201601-062FR
28. Noppen M, Meysman M, Van Herreweghe R, Lamote J, D'Haese J, Vincken W. Bronchoscopic cryotherapy: preliminary experience. Acta Clin Belg (2001) 56(2):73–7. doi: 10.1179/acb.2001.013
29. Deygas N, Froudarakis M, Ozenne G, Vergnon JM. Cryotherapy in early superficial bronchogenic carcinoma. Chest (2001) 120(1):26–31. doi: 10.1378/chest.120.1.26
30. Bertoletti L, Elleuch R, Kaczmarek D, Jean-Francois R, Vergnon JM. Bronchoscopic cryotherapy treatment of isolated endoluminal typical carcinoid tumor. Chest (2006) 130(5):1405–11. doi: 10.1378/chest.130.5.1405
31. Kohno M, Hashimoto R, Oiwa K, Yashiro H, Nakatsuka S, Kawamura M, et al. Initial experience with transbronchial cryoablation as a novel local treatment for Malignant peripheral lung lesions. BMJ Open Respir Res (2018) 5(1):e000315. doi: 10.1136/bmjresp-2018-000315
32. Nian J, Zhu Y, Fu Q, Yang G, Wang X. Significant response of pulmonary sarcomatoid carcinoma with obstructive atelectasis to treatment with the PD-1 inhibitor camrelizumab combined with transbronchial cryoablation: A case report and literature review. Front Oncol (2022) 12:1013047. doi: 10.3389/fonc.2022.1013047
33. Zheng X, Yuan H, Gu C, Yang C, Xie F, Zhang X, et al. Transbronchial lung parenchyma cryoablation with a novel flexible cryoprobe in an in vivo porcine model. Diagn Interv Imaging (2022) 103(1):49–57. doi: 10.1016/j.diii.2021.08.005
34. Hammer D, Budi L, Nagy A, Varga R, Horvath P. Evaluation of a transbronchial cryoprobe for the ablation of pulmonary nodules: an in vitro pilot study. BMC Pulm Med (2023) 23(1):71. doi: 10.1186/s12890-023-02358-y
35. Niu L, Xu K, Mu F. Cryosurgery for lung cancer. J Thorac Dis (2012) 4(4):408–19. doi: 10.1142/9789814329668_0021
36. Dassa M, Izaaryene J, Daidj N, Piana G. Efficacy of tract embolization after percutaneous pulmonary radiofrequency ablation. Cardiovasc Intervent Radiol (2021) 44(6):903–10. doi: 10.1007/s00270-020-02745-6
37. Zheng X, Yang C, Zhang X, Yuan H, Xie F, Li Y, et al. The Cryoablation for Peripheral Pulmonary Lesions Using a Novel Flexible Bronchoscopic Cryoprobe in the ex vivo Pig Lung and Liver. Respiration (2019) 97(5):457–62. doi: 10.1159/000494142
38. Oberg CL, Lau RP, Folch EE, He T, Ronaghi R, Susanto I, et al. Novel robotic-assisted cryobiopsy for peripheral pulmonary lesions. Lung (2022) 200(6):737–45. doi: 10.1007/s00408-022-00578-3
39. Kim SH, Mok J, Jo EJ, Kim MH, Lee K, Kim KU, et al. The additive impact of transbronchial cryobiopsy using a 1.1-mm diameter cryoprobe on conventional biopsy for peripheral lung nodules. Cancer Res Treat (2023) 55(2):506–12. doi: 10.1016/j.rmed.2023.107338
40. Zhang J, Guo JR, Huang ZS, Fu WL, Wu XL, Wu N, et al. Transbronchial mediastinal cryobiopsy in the diagnosis of mediastinal lesions: a randomised trial. Eur Respir J (2021) 58(6):2100055. doi: 10.1183/13993003.00055-2021
41. Fan Y, Zhang AM, Wu XL, Huang ZS, Kontogianni K, Sun K, et al. Transbronchial needle aspiration combined with cryobiopsy in the diagnosis of mediastinal diseases: a multicentre, open-label, randomised trial. Lancet Respir Med (2023) 11(3):256–64. doi: 10.1016/S2213-2600(22)00392-7
42. Adam LC, Raja J, Ludwig JM, AdenIran A, Gettinger SN, Kim HS. Cryotherapy for nodal metastasis in NSCLC with acquired resistance to immunotherapy. J Immunother Cancer (2018) 6(1):147. doi: 10.1186/s40425-018-0468-x
43. Shulman S, Yantorno C, Bronson P. Cryo-immunology: a method of immunization to autologous tissue. Proc Soc Exp Biol Med (1967) 124(2):658–61. doi: 10.3181/00379727-124-31817
44. Soanes WA, Ablin RJ, Gonder MJ. Remission of metastatic lesions following cryosurgery in prostatic cancer: immunologic considerations. J Urol (1970) 104(1):154–9. doi: 10.1016/S0022-5347(17)61690-2
45. Ablin RJ, Soanes WA, Bronson PM, Gonder MJ. Serum proteins in patients with benign and Malignant diseases of the prostate. Neoplasma (1971) 18(3):271–6.
46. Riera CM, Brandt EJ, Shulman S. Studies in cryo-immunology. IV. Antibody development in rabbits after iso-immunization followed by freezing. Immunology (1968) 15(6):779–87.
47. Shulman S, Brandt EJ, Yantorno C. Studies in cryo-immunology. II. Tissue and species specificity of the autoantibody response and comparison with iso-immunization. Immunology (1968) 14(2):149–58.
48. Reddy KP, Ablin RJ. Immunologic and morphologic effects of cryosurgery of the monkey (macaque) prostate. Res Exp Med (Berl) (1979) 175(2):123–38. doi: 10.1007/BF01851819
49. Sabel MS, Nehs MA, Su G, Lowler KP, Ferrara JL, Chang AE. Immunologic response to cryoablation of breast cancer. Breast Cancer Res Treat (2005) 90(1):97–104. doi: 10.1007/s10549-004-3289-1
50. Yamashita T, Hayakawa K, Hosokawa M, Kodama T, Inoue N, Tomita K, et al. Enhanced tumor metastases in rats following cryosurgery of primary tumor. Gan (1982) 73(2):222–8.
51. Shibata T, Yamashita T, Suzuki K, Takeichi N, Micallef M, Hosokawa M, et al. Enhancement of experimental pulmonary metastasis and inhibition of subcutaneously transplanted tumor growth following cryosurgery. Anticancer Res (1998) 18(6A):4443–8.
52. Miya K, Saji S, Morita T, Niwa H, Sakata K. Experimental study on mechanism of absorption of cryonecrotized tumor antigens. Cryobiology (1987) 24(2):135–9. doi: 10.1016/0011-2240(87)90015-0
53. Katzman D, Wu S, Sterman DH. Immunological aspects of cryoablation of non-small cell lung cancer: A comprehensive review. J Thorac Oncol (2018) 13(5):624–35. doi: 10.1016/j.jtho.2018.01.017
54. Gu XY, Jiang Z, Fang W. Cryoablation combined with molecular target therapy improves the curative effect in patients with advanced non-small cell lung cancer. J Int Med Res (2011) 39(5):1736–43. doi: 10.1177/147323001103900516
55. Yuanying Y, Lizhi N, Feng M, Xiaohua W, Jianying Z, Fei Y, et al. Therapeutic outcomes of combining cryotherapy, chemotherapy and DC-CIK immunotherapy in the treatment of metastatic non-small cell lung cancer. Cryobiology (2013) 67(2):235–40. doi: 10.1016/j.cryobiol.2013.08.001
56. Lin M, Liang SZ, Wang XH, Liang YQ, Zhang MJ, Niu LZ, et al. Clinical efficacy of percutaneous cryoablation combined with allogenic NK cell immunotherapy for advanced non-small cell lung cancer. Immunol Res (2017) 65(4):880–7. doi: 10.1007/s12026-017-8927-x
57. Takaki H, Imai N, Thomas CT, Yamakado K, Yarmohammadi H, Ziv E, et al. Changes in peripheral blood T-cell balance after percutaneous tumor ablation. Minim Invasive Ther Allied Technol (2017) 26(6):331–7. doi: 10.1080/13645706.2017.1310737
58. Leppelmann KS, Mooradian MJ, Ganguli S, Uppot RN, Yamada K, Irani Z, et al. Thermal ablation, embolization, and selective internal radiation therapy combined with checkpoint inhibitor cancer immunotherapy: safety analysis. J Vasc Interv Radiol (2021) 32(2):187–95. doi: 10.1016/j.jvir.2020.09.014
59. Feng J, Guiyu D, Xiongwen W. The clinical efficacy of argon-helium knife cryoablation combined with nivolumab in the treatment of advanced non-small cell lung cancer. Cryobiology (2021) 102:92–6. doi: 10.1016/j.cryobiol.2021.07.007
60. Xu F, Song J, Xu B, Wang J, Mao J, Liu H, et al. Clinical study of systemic chemotherapy combined with bronchoscopic interventional cryotherapy in the treatment of lung cancer. BMC Cancer (2020) 20(1):1089. doi: 10.1186/s12885-020-07444-6
61. Sabel MS, Su G, Griffith KA, Chang AE. Rate of freeze alters the immunologic response after cryoablation of breast cancer. Ann Surg Oncol (2010) 17(4):1187–93. doi: 10.1245/s10434-009-0846-1
62. Yakkala C, Dagher J, Sempoux C, Chiang CL, Denys A, Kandalaft LE, et al. Rate of freeze impacts the survival and immune responses post cryoablation of melanoma. Front Immunol (2021) 12:695150. doi: 10.3389/fimmu.2021.695150
Keywords: cryoablation, cryotherapy, lung cancer, immune response, bronchoscopy
Citation: Velez A, DeMaio A and Sterman D (2023) Cryoablation and immunity in non-small cell lung cancer: a new era of cryo-immunotherapy. Front. Immunol. 14:1203539. doi: 10.3389/fimmu.2023.1203539
Received: 10 April 2023; Accepted: 31 July 2023;
Published: 21 August 2023.
Edited by:
Ming Kuang, The First Affiliated Hospital of Sun Yat-sen University, ChinaReviewed by:
Israel Barken, University of California, San Diego, United StatesCalvin Sze Hang Ng, The Chinese University of Hong Kong, China
Copyright © 2023 Velez, DeMaio and Sterman. This is an open-access article distributed under the terms of the Creative Commons Attribution License (CC BY). The use, distribution or reproduction in other forums is permitted, provided the original author(s) and the copyright owner(s) are credited and that the original publication in this journal is cited, in accordance with accepted academic practice. No use, distribution or reproduction is permitted which does not comply with these terms.
*Correspondence: Antonio Velez, QW50b25pby52ZWxlekBueXVsYW5nb25lLm9yZw==