- 1Department of Radiation Oncology, Institut De Cancérologie De L’Ouest (ICO), Saint-Herblain, France
- 2Department of Medical Oncology, Institut De Cancérologie De L’Ouest, Saint-Herblain, France
- 3Department of Medical Oncology, CHRU Morvan, University Hospital, Brest, France
- 4Radiation Oncology Department, University Hospital, Brest, France
- 5LaTIM, INSERM, UMR 1101, Univ Brest, Brest, France
Despite human papillomavirus vaccination and screening, in about 5% of cases, cervical cancer (CC) is discovered at an initial metastatic stage. Moreover, nearly one-third of patients with locally advanced CC (LACC) will have a recurrence of their disease during follow-up. At the stage of recurrent or metastatic CC, there are very few treatment options. They are considered incurable with a very poor prognosis. For many years, the standard of care was the combination of platinum-based drug and paclitaxel with the possible addition of bevacizumab. The most recent years have seen the development of the use of immune checkpoint inhibitors (ICIs) (pembrolizumab, cemiplimab and others) in patients with CC. They have shown long term responses with improved overall survival of patients in 1st line (in addition to chemotherapy) or 2nd line (as monotherapy) treatment. Another emerging drug is tisotumab vedotin, an antibody-drug conjugate targeting tissue factor. Radiation therapy (RT) often has a limited palliative indication in metastatic cancers. However, it has been observed that RT can induce tumor shrinkage both in distant metastatic tumors beyond the radiation field and in primary irradiated tumors. This is a rarely observed phenomenon, called abscopal effect, which is thought to be related to the immune system and allows a tumor response throughout the body. It would be the activation of the immune system induced by the irradiation of cancer cells that would lead to a specific type of apoptosis, the immunogenic cell death. Today, there is a growing consensus that combining RT with ICIs may boost abscopal response or cure rates for various cancers. Here we will review the potential abscopal effect of immune-radiation therapy in metastatic cervical cancer.
1 Introduction
Although considerable progress has been made in the prevention and treatment of this challenging disease, large populations in more rural and underserved regions continue to have much lower rates of vaccination. Thus, According to GLOBOCAN 2020, cervical cancer is one of the most common cancers among women worldwide, after breast, colon, and lung cancer, respectively. It is most common in developing countries, accounting for more than 85% of diagnosed cases (1). In developing countries where routine screening is not available, more than 70% of CC are diagnosed in advanced or metastatic stages (2, 3). In developed countries, in about 5% of cases, cervical cancer (CC) is discovered at an initial metastatic stage. Moreover, the number of new cases of locally advanced cervical cancer (LACC), also known as International Federation of Gynecology and Obstetrics (FIGO) 2018 stage IB3 and IIA2-IVA, is stable due to lack of widespread vaccination and incomplete compliance with screening. Concurrent chemoradiation therapy (CCRT) is the standard of care for patients with LACC. Despite considerable progress and optimization of treatments over the past 20 years, nearly one-third of patients will experience a recurrence of their disease despite the initial optimal management (4). Most patients with recurrent or metastatic disease will be treated with systemic therapy including chemotherapy (CT) plus or minus an angiogenesis inhibitor. However, HPV DNA is detected in over 99% of cervical cancer biopsies with the majority being of types 16 and 18. The E6 and E7 oncogenes are primarily responsible for the transformation of HPV-infected cervical keratinocytes. HPV-associated tumors always express these viral antigens which are attractive targets for immunotherapy (IT) (5).
Currently, the role of radiation therapy (RT) in the management of CC is mainly limited to the locally advanced stage. Cisplatin-based chemoradiation therapy (CRT) followed by Image Guided Adaptative Brachytherapy (IGABT) is the gold standard for FIGO 2018 stage IB3–IVA, regardless of histological subtype (6). However, stereotactic body radiotherapy (SBRT), for curative treatment of oligometastatic patients or palliative treatment in polymetastatic disease, has developed quickly in recent years. Its potential immunosensitizing effect, including the abscopal effect, raises the question of its association with IT in metastatic stages (7, 8).
In this review, we discuss the current status of IT for patients with primary metastatic or recurrent CC and the relevance of combining RT for its immunomodulatory effect.
2 Systemic treatment for recurrent or metastatic cervical cancer
2.1 Standard of care before immunotherapy
Since the results of the phase II trial Gynecologic Oncology Group (GOG)-26 the standard of care for recurrent disseminated CC has been cisplatin-based CT (9). Cisplatin combined with either paclitaxel, gemcitabine, topotecan, or vinorelbine was studied in the GOG-204 trial. Although the cisplatin-paclitaxel combination tended to have better results, no statistically significant difference was found between the different doublets. Despite these negative results, this combination was considered as the standard of care, particularly in women who had not received prior cisplatin-based therapy (10). In a non-inferiority study, 253 patients with recurrent stage IVB CC were randomized to paclitaxel plus either carboplatin or cisplatin. The combination of paclitaxel and carboplatin was non-inferior to the doublet paclitaxel and cisplatin in terms of PFS and OS, and showed a significant reduction in toxicity. However, in the subgroup of patients who had not previously received cisplatin the cisplatin-based doublet was superior (11).
Bevacizumab, a humanized monoclonal antibody targeting VEGF, is the leading antiangiogenic therapy studied in patients managed for CC (12). A randomized phase III trial showed an improvement in both PFS [8.2 versus 5.9 months; hazard ratio (HR) 0.67, 95% confidence interval (CI) 0.54-0.82] and OS (17.0 versus 13.3 months; HR 0.71, 95% CI 0.54-0.95) with the combination of CT (paclitaxel and cisplatin or topotecan) and bevacizumab (13, 14). As such, these combinations have been approved by the Food and Drug Administration (FDA) and the European Medicines Agency (EMA) for the treatment of patients with metastatic or recurrent CC.
2.2 Immunotherapy in metastatic cervical cancer
In recent years, several studies have focused on the use of IT for the treatment of CC because of evidence of an immune system response related to interactions with HPV infection (15).
2.2.1 Rationale
HPV induces an immunosuppressive tumor microenvironment (TME) and deficient immunosurveillance by upregulating TGF-β via regulatory T (Treg) cells and changing the cytokine profile from a T helper 1 (Th1) profile to a Th2 profile (16). Moreover, some immune system inhibitory molecules such as Programmed cell death-1 (PD-1)/Programmed cell death ligand1 (PD-L1), are expressed by CC cells whereas they are not in healthy cervical tissue. However, PD-L1 expression seems to be more frequent in SCC (19% to 88%) than in adenocarcinoma (14%) (17, 18). Other immunomodulatory molecules such as cytokines (TGF-β, IL-10), cytotoxic T-lymphocyte antigen 4 (CTLA-4) and surface receptors (TIM3) have also been identified (19, 20). It was also shown that CD8+, CD4+ and Treg cells were more frequently found in the TME in CC than in normal cervical tissue with a significant decrease in survival (21, 22). Finally, studies have shown an increase in the total mutational burden (TMB) rate in CC (approximately 5-6 mutations per megabase) (23, 24). Thus, immune checkpoints inhibitors (ICIs) including inhibitors of PD-1 and PD-L1, as well as inhibitors of CTLA-4 have been evaluated in several CC trials (5, 25).
2.2.2 Monotherapy
Monotherapy showed a lack of significant single-agent activity.
2.2.3 PD-1 inhibitors
Pembrolizumab, balstilimab and nivolumab were evaluated in phase II studies for recurrent or metastastic CC in the second line-treatment. They found objective response rates (ORRs) of 12%, 15%, 26% respectively (26–30).
Cemiplimab was evaluated in a phase III study where 608 patients with recurrent or metastatic CC after platinum-based CT were randomized between cemiplimab or CT of investigator’s choice (31). Cemiplimab showed a significant improvement in overall survival, 12.0 versus 8.5 months, HR= 0.69 (0.56-0.84), p<0.0001.
2.2.4 Anti CTLA-4
Forty-two patients with a recurrent or metastatic CC have received ipilimumab in a phase I-II study. The mPFS and mOS were 2.5 and 8.5 months, respectively (32).
2.2.5 PD-L1 inhibitors
Socazolimab was evaluated in a phase I and showed ORRs similar between PD-L1 positive and negative patients (16.7% and 17.9%, respectively) (33).
2.2.6 Bispecific antibody
Cadonilimab (a PD-1/CTLA-4 bi-specific antibody) and Bintrafusp alfa (an innovative bifunctional fusion protein consisting of the extracellular domain of transforming growth factor-β receptor II (TGF-βRII) fused to human IgG1 mAb of PD-L1) were evaluated in phase II studies and showed ORRs of 33% and 31%, respectively (34, 35).
The unsuccessful results of ICI monotherapies have led to studies evaluating such as ICI combination therapy, ICI and targeted therapy, antibody-drug conjugate (ADC), adoptive cell therapy, and therapeutic vaccines.
2.2.7 Combination of ICI and anti-VEGF
Treatment with PD-1 mAb (Sintilimab/Camrelizumab) in combination with or without antiangiogenic drugs (Apatinib) was evaluated in 102 patients with recurrent or metastatic CC in a retrospective study and showed an ORR of 51.0%, a DCR of 66.7%, and a median PFS of 11.0 months (36).
Atezolizumab and cadonilimab (AK104) in combination with bevacizumab were tested in 2 phase II studies and showed ORRS of 0%, 73.3% (11/15), 68.8% (11/16), and 92.3% (12/13) for atezolizumab + bevacizumab, AK104 15 mg/kg, AK104 10 mg/kg and AK104 10 mg/kg + bevacizumab 15 mg/kg, respectively (37, 38).
Camrelizumab (anti PD-1 antibody) + apatinib (anti VEGFR2 antibody), Sintilimab (anti-PD-1) + anlotinib (a small molecule VEGFR-2 selective inhibitor), nivolumab + lucitanib [a tyrosinase inhibitor with multiple targets (VEGFR1-3, FGFR1-3, and PDGFRa/b)] and serplulimab (anti-PD-1) + albumin-bound paclitaxel showed in phase II studies, ORRs of 55.6% (95% CI, 40.0% to 70.4%), 59.0%, 23.5% and 57.1% and a median PFS 8.8 months (95% CI 5.6 months not reached), 9.4 months, not reported and 5.7 months, respectively (39–42).
The double-blind, randomized, phase III KEYNOTE-826, in which 617 patients received carboplatin + paclitaxel with or without bevacizumab randomized between pembrolizumab and placebo, showed improved PFS [12.4 months vs 8.2 months, HR =0.65 (95% CI: 0.53-0.79)] as well as OS (24.4 months vs 16.3 months; HR= (0.67 (95% CI: 0.54 -0.84)). The subgroup analysis does not find any benefit in patients with a CPS score <1 and those who are metastatic from the outset (43).
2.2.8 ICI combination therapy
Balstilimab + zalifrelimab (anti-CTLA-4), pembrolizumab + tisotumab (ADC that targets tissue factors and releases a microtubule-disrupting agent, monomethyl auristatin E) and pembrolizumab + GX-188E (Tirvalimogene teraplasmid is an HPV-16 and HPV18 E6 and E7 therapeutic DNA vaccine) showed in phase II studies ORRs of 25.6%, 41% and 33.3% and median PFS of not reported, 5.3 months, not reported (44–46).
Nivolumab + tumor-infiltrating lymphocytes (TILs) showed for patients with low microsatellite instability (MSI) expression, PD-L1 negative metastatic CC an ORR of 25%, a median PFS of 6.1 months, and a median OS of 11.3 months (47).
2.3 Synthetic
Among patients with recurrent or metastatic CC, two large randomized controlled phase III trials have shown improved outcomes. The GOG-3016 trial reported improved overall survival in patients treated with ICI compared with CT alone. The KEYNOTE-826 study showed an almost one-year improvement in overall survival when combining an ICI with CT, with or without anti-angiogenic therapy, compared with CT alone. However, some studies have failed to achieve such encouraging results, probably due to the suppression of the tumour microenvironment (32, 47). RT, and especially the high-dose fractionated RT, may induce a strong antitumor immune effect. Thus, the combination of RT and ICI could lead to an improvement of the efficacy of ICI.
3 Abscopal effect
3.1 Rational of optimizing local efficiency towards remote efficiency
Since the first use of RT to treat in the early 1900s, local efficacy with local toxicity has been observed. However, in some cases, lesions located at a distance from the irradiated lesion have shown stability and even a response (48). This phenomenon was called the abscopal effect (“ab” meaning position away from, and “scopus” meaning “target”), i.e. at a distance from the target. At that time, this explanation for this effect remained unresolved. Today, we have preclinical and clinical arguments to affirm that there are interactions between irradiation and the immune system and we can exploit them.
Immunity has been identified to be a factor in response to RT. In a mouse model, tumors were implanted subcutaneously in immunodeficient (nude) and immunocompetent mice and then irradiated. Measurement of the tumor growth curves showed that the response to irradiation is totally different between an immunodeficient and an immunocompetent mouse. Indeed, the immunodeficient mouse showed no response to irradiation while the immunocompetent mouse responded. Thus, immunity could have an impact on the response to irradiation (49).
The immune mechanism is explained by the fact that a dead tumor cell will release a cascade of signals and ligands into the microenvironment and express receptors on its surface that will activate immunity. Indeed, we will observe an increase in the expression rate of the major histocompatibility complex after irradiation. Then, this process results in the release of cytokines, cell death factors and cell damage factors which increase immunogenicity and lead to interactions with dendritic cells and T cells (50).
When a tumor is irradiated, there are 2 main mechanisms. The first is direct cell death linked to apoptosis, mitotic catastrophe (particularly in the case of a p53 mutation and for tumor cells), and other types of cell death including oncosis. The second is indirect cell death linked to the activation of T lymphocytes that will destroy the surviving tumor cells (51). Thus, tumor cells that survive irradiation can be eliminated by immune cells.
Increased immunogenicity may be enabled by anti CTLA-4 and anti PD-1, PD-L1 monoclonal antibodies (52). In combination with RT, a synergy has been pre-clinically demonstrated in implanted breast cancer models in mice with an increase in local efficacy but also an effect on the non-irradiated tumor (53). Thus, the combination of RT with an immunomodulator can induce an action on non-irradiated sites at a distance, this is the abscopal effect. In fact, a tumor irradiated with a high dose will release antigens in the microenvironment which will be taken up by dendritic cells and which will migrate into the lymph nodes and lead to an effector immune response with T and B lymphocytes, which will proliferate in the lymph nodes and will destroy the tumor cells which would have survived the irradiation locally or those which are at a distance (50).
For the moment the abscopal effect is very uncommon because the tumors are well developed with immune escape mechanisms. Thus, it is difficult for RT alone to create an effective immune response, hence the interest in combining RT with ICI (54). The current goal is to generalize this rare phenomenon given its potential impact on efficacy, particularly on overall survival and progression-free survival (55).
In CC, there are very few results on the combination of ICI and radiotherapy.
3.2 Preclinical clinical studies
One preclinical study evaluated anti-CD40 therapy to boost the abscopal effect (56). CD40 is a costimulatory protein that binds to CD154 (CD40L) on T helper cells allowing activation of APCs and a cascade of immune effects. Studies have shown the absence of CD40 overexpression in normal cervical epithelium, whereas it was overexpressed in human papillomavirus infection and in SCC of the cervix (57, 58). Ligation of CD40 results in inhibition of tumor growth through strong apoptotic signals to cancer cells. This could be a candidate therapeutic target that would result in the production of antigens to enhance the abscopal effect (58).
In this study, TC-1 cells were injected subcutaneously into both dorsolateral flanks of each mouse to mimic metastatic cervical cancer models. Tumor growth was monitored to a treatable size of 3-4 mm in diameter. Two main results were highlighted in this study. The first one concerned the optimal irradiation dose in combination with a single dose of anti-CD40 to obtain an abscopal effect, and only the lowest dose studied, 6 Gy, showed a significant abscopal response compared to higher doses of 10 and 15 Gy. The second result concerns the number of anti-CD40 injections, the greatest abscopal effect was in the group receiving a single dose of anti-CD-40 and not in the group receiving 3 doses.
A study assessed intratumoral changes induced by radioimmunotherapy by performing single‐cell RNA‐sequencing on a pair of cervical squamous cell carcinoma samples before and during radioimmunotherapy (59). They showed that radioimmunotherapy induced changes in the tumor and immune microenvironment including transformation of epithelial cell subclusters from a malignant to a normal phenotype with some residual malignant cells, an increase in apolipoprotein E+ macrophages with high levels of M2 features, and a decrease in inhibition scores of residual exhausted CD8+ T and regulatory T cells.
3.3 Clinical results
A case report about a patient with recurrent cervical cancer metastatic to the liver demonstrated the benefits of combining radioembolization with pembrolizumab, the patient having achieved a complete response at 8 months. Like SBRT, radioembolization enables very high doses to be delivered in a short space of time (60).
A phase 1 study evaluated the association of RT with granulocyte-macrophage colony-stimulating factor and found in some patients treated for metastatic solid tumors objective abscopal responses but only one patient had a CC and no abscopal response was observed (61).
A phase 1 study assessed the safety of combination of cemiplimab and RT (3x9 Gy) in metastatic or recurrent CC patients who were resistant to or intolerant of platinum and taxane CT (62). The results showed that adding RT to cemiplimab did not increase grade ≥3 AE but did not improve the response rate versus single-agent cemiplimab (one PR in ten patients [10%] in both arms).
A multi-center open-label, non-randomized phase 2 study (PRIMMO), in patients with metastatic or recurrent CC, endometrial carcinoma, or uterine sarcoma, evaluated the combination of PD-1 blockade (pembrolizumab), radiation (3x8 Gy in 48 h-intervals) and repurposed compounds (i.e. drugs approved for another indication) with (immune) modulating properties (vitamin D, aspirin, lansoprazole, a proton-pump inhibitor, cyclophosphamide and curcumin) aimed at targeting TME and promoting anti-tumor immunity (63). This study found an ORR of 11.1% (depending on the criteria) of patients in at least 2nd line treatment. The primary objective of this study was an ORR with the lower bound of the 90% CI of > 10% in either cohort. Unfortunately, it did not meet its primary objective with results even lower than those found in studies testing other associations with ICIs and the rate of grade ≥3 toxicity was 55.6% (10 patients).
A phase 1 study (GOG-9929) included 21 patients with node-positive LACC to evaluate the safety of ipilimumab administration after CT. The authors reported a safety grade ≥ 3 rate of 10% (64). Moreover, the authors found that CRT alone resulted in an increase of the activation markers ICOS and PD-1 on T-cell subsets. The combination of ipilimumab and CRT led to a significant increase of both central and effector memory T-cell populations.
Unfortunely, the CALLA trial which evaluated the addition of durvalumab to standard CRT is negative on its main endpoint which is progression-free survival (HR 0.84, 95%CI 0.65-1.08, p=0.174), which also raises the question of how to optimize the association of RT with IT in this type of tumor.
4 Ongoing studies
As mentioned above, few clinical results are available at this time. However, based on the potential benefit of combining RT and ICIs, trials of concomitant RT with IT are currently underway for LACC but also for metastatic or recurrent CC (Tables 1, 2).
5 Perspectives
In CC, the responder rate to IT monotherapy is lower than in melanoma or in lung cancers for example. Furthermore, no objective abscopal effect was found in advanced or recurrent CC when combining RT and ICIs. Prospective randomized trials evaluating the combination of RT and ICIs are needed. However, it is also essential that studies be conducted to improve the therapeutic index of this promising association.
5.1 Improvement radiation therapy in combination with immunotherapy
RT alone allows a kind of reprogramming of the tumor microenvironment with the increase in pro immunogenic actors, in particular pro immunogenic myeloid populations, effector CD8 Ts, but on the other hand it also increases regulatory Ts (65, 66). Thus the current objective is to tilt the immune balance by reducing the negative effects of RT or by increasing its positive effects (67). Today, it is not a question of using IT to increase the response to RT but rather the opposite, to use this local treatment that is RT and to optimize it in order to combine it with IT and potentiate its effects.
The parameters that can be optimized in RT are i) the irradiation fields, in particular the lymph node areas, ii) the total dose and the dose per fraction, iii) the identification of new organs at risk such as the blood, iv) the therapeutic sequence with ICI v) and the irradiation of several sites simultaneously.
i) Irradiation fields and lymph node sparing
In a preclinical study using immunocompetent mouse model with tongue cancer treated by an inhibitor of CTLA-4, the authors compared efficacy between a local treatment of lymph nodes to no local treatment (68). They found that the anti-CTLA-4 allowed a tumor control which is abrogated in case of local treatment of the lymph node areas by surgery or RT. Thus, by irradiating the drainage lymph nodes of the tumor at 18 Gy, the effect of anti-CTLA-4 was abolished. Furthermore, there were significant differences in the tumor-infiltrating immune population depending on whether or not lymph node treatment was performed after anti CTLA-4 therapy. In the group without local lymph node treatment, there was a large infiltrate of CD4 and CD8 cells and few immunosuppressive myeloid cells, whereas the opposite was in case of local lymph node treatment.
In a phase II trial (69), early-stage non-small cell lung cancers (NSCLC) evaluated a neoadjuvant treatment combining durvalumab and SBRT (3x8 Gy) of the primary tumor only without including draining nodes versus durvalumab one before surgery. The combination was safe and was associated with an increase of histopathological response (53.3% versus 6.7% (p<0.001)). Interestingly, on the off-screen response, 13 patients had pre-treatment positive lymph nodes detected on PET/CT.
In the JAVELIN trial (70), there is irradiation of the primitive site and of the lymph node drainage areas at high dose (70 Gy in 35 fractions) with Avelumab. This study did not meet the primary objective (PFS) and one of the explanatory hypotheses for this failure is the lack of modification of the irradiation field to spare the lymph node drainage areas.
Thus, the question arises in the context of association with IT to exclude the first lymph node relay from RT fields.
In LACC, a randomized phase II trial evaluated the value of adding induction CT before conventional CCRT (71). Results showed a decrease in 3-year PFS (40.9% vs. 60.4%) and 3-year OS (60.7% vs. 86.8%) in the induction CT group compared with the immediate CCRT group. Two hypotheses were suggested by the authors to explain these disappointing results. First, there may be a risk of local disease progression during induction CT because of the absence of local treatment during this phase. Second, the administration of induction CT could lead to side effects that would limit the administration of CT concomitant with RT. SBRT alone on the pathological nodes combined with RT on the primary tumor with CT and IT could be an alternative (Figure 1).
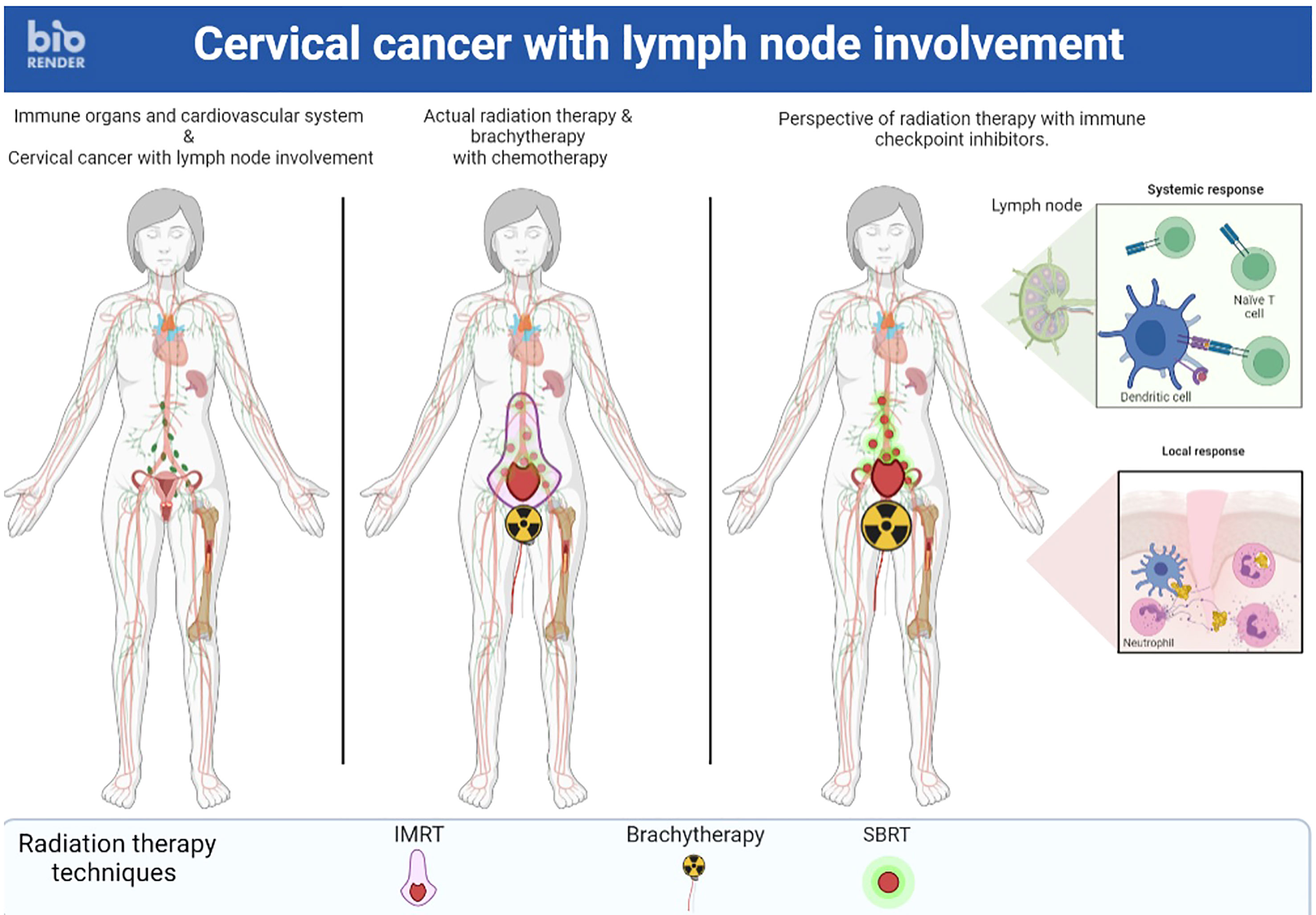
Figure 1 A locally advanced cervical cancer where stereotactic body radiation therapy alone on the pathological nodes combined with radiation therapy on the primary tumor with chemotherapy and immunotherapy could be an alternative.
ii) Total dose, dose by fractions and heavy particles
An important role of RT dose is to increase recruitment of the innate immune system and initiate gene transcription of the interferon that will be released in the TME, which is key to activating the immune response. It will enable the recruitment of immune cells into the TME and of dendritic cells for cross-presentation (72, 73).
In vitro and in vivo models have shown that there is more interferon response when using doses of 8 Gy than when using higher doses of 20 Gy, for example. Thus, it would seem that 3x8 Gy is the optimal scheme (74). Recently, a classification of RT regimens has been proposed according to their potential to enhance immune responses (75).
A preclinical study investigated the combination of brachytherapy (3x8 Gy) with IT (anti-PD1, anti-CD137, and/or their respective isotype controls) in mice with subcutaneous colorectal cancer at 2 sites. To evaluate a possible abscopal effect, only one tumor was irradiated. They demonstrated a response on the non-irradiated tumor, and thus an abscopal effect, only in mice receiving the BT and ICI combination (76). The clinical application of this approach could potentially provide a treatment alternative for patients being treated for CC where brachytherapy is a routinely used treatment.
One study reported the results of a combination of high-dose and low-dose RT versus high-dose RT alone in patients with IT-resistant metastatic cancer (77). This combination appeared to improve lesion-specific response in patients with immune-resistant solid tumors by promoting the infiltration of effector immune cells into the TME.
Heavy particles, such as carbon ions and protons, seem to have immune effects that could be of interest in association with ICIs (78). A multicenter Phase II trial to assess the feasibility and clinical activity of adding carbon ion radiotherapy to immune checkpoint inhibitors in cancer patients who have achieved stable disease with pembrolizumab administered as standard therapy has started (78).
iii) Identification of new organs at risk such as blood
Studies have shown that RT could induce lymphopenia resulting in poorer overall survival for many types of cancer (79). The mechanism of this lymphopenia can be direct in case of a large percentage of irradiated circulating cells making the treatment less effective. For example, in the case of an 8 cm lung cancer treated with 60 Gy in 30 fractions, a 2 Gy fraction gives 0.5 Gy to 5% of the circulating cells and 3 fractions give more than 0.5 Gy to 99% of the circulating cells (80). This suggests the value of hypofractionation and reduction of radiation fields and low doses. These results are in agreement with the recommendations that have been proposed recently (81).
The mechanisms of CRT-induced lymphopenia are being explored since it could also be caused by treatment-induced circulating immunosuppressive myeloid cells (82).
Moreover, the response of a tumor to irradiation has been shown to be impacted by neutrophilia. So hyperleukocytosis is a pejorative factor in the response to irradiation and we see a clear, linear response between the severity of the different cancers and the proportion of patients who have baseline hyperleukocytosis, which suggests the link between neutrophil count and response to irradiation (83). In the case of CC, intense spinal fixation could be seen on PET scan. Having bone marrow hyperactivation in non-metastatic cervical cancer patients is highly significantly correlated with the probability of survival. This hyperactivation is related to hyperleukocytosis and correlates with poor response, but also with tumor infiltration by myeloid-suppressive cells. This suggests a deleterious role of the myeloid compartment in the response to irradiation (84).
iv) Therapeutic sequence
It is a challenge to find the optimal therapeutic sequence in which RT and IT are administered. Anti-PD-1 administration before irradiation sensitizes T cells or more γH2ax breaks and later apoptosis are observed (85). In mice, this results in improved survival and preferential induction of the abscopal effect if IT is given after RT.
v) A final approach to promote the abscopal effect would be to abandon the idea of irradiating a single metastatic site but rather to irradiate several sites simultaneously (86) (Figures 2, 3). First, this RT targeting different tumor lesions could avoid T cell exhaustion by decreasing the antigenic load (87). Moreover, ICI seems to be more effective with lower volume disease (88). This multi-site RT could lead to a larger and more diversified release of tumor antigens. Finally, this RT is now feasible in the light of the many technological advances that make it increasingly accurate (89). Unfortunately, this RT is not widely available in countries where cervical cancer is most common.
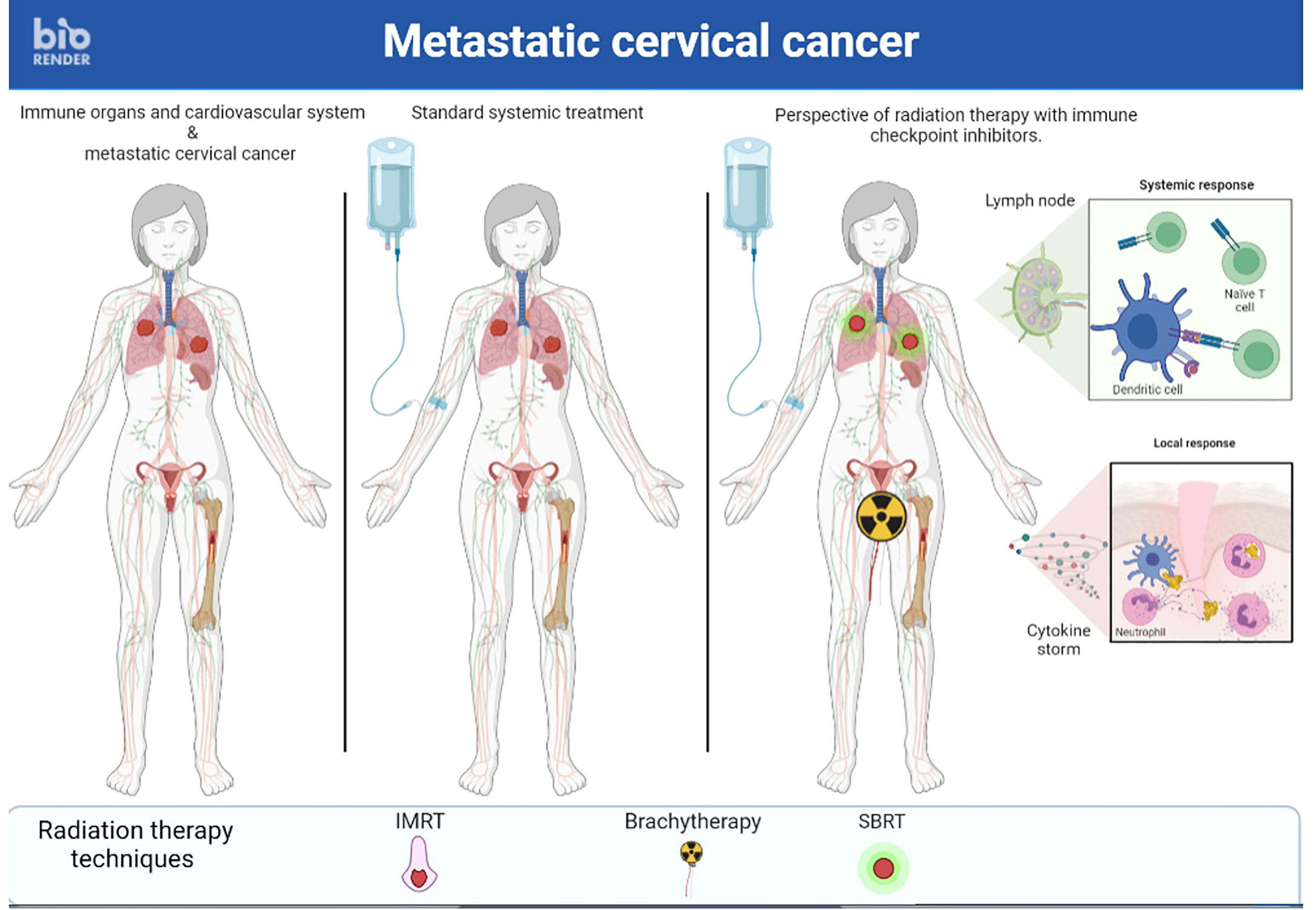
Figure 2 A case of metastastic cervical cancer treated with stereotactic body radiation Brachytherapy therapy multiple metastastic sites and radiation therapy followed by brachytherapy on primary tumor and immunotherapy simultaneously to enhance immune response.
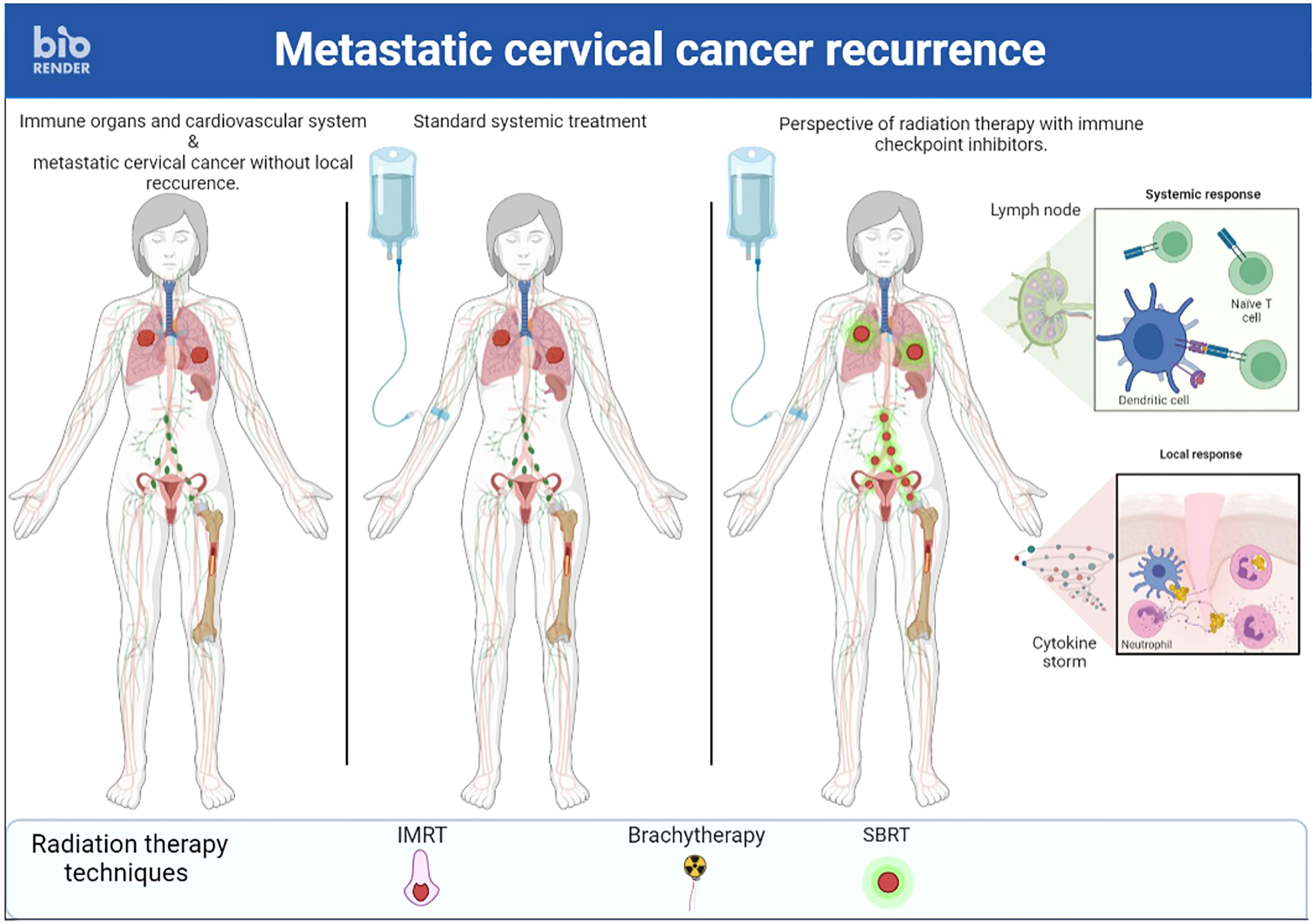
Figure 3 A case of recurrent cervical cancer treated with stereotactic body radiation therapy on multiple metastastic sites and immunotherapy simultaneously to enhance immune response.
5.2 Management of the tumor microenvironment to enhance the immunogenic side of radiation therapy
Another avenue of research to improve the therapeutic index of RT and ICI combinations would be to manipulate the TME to improve the immunogenicity of RT and reduce its immunosuppressive effect.
Potential TME targets have already been identified, such as tumor-associated macrophage (combination with granulocyte colony-stimulating factor or with a colony-stimulating factor 1 receptor antagonist monoclonal antibody), 4-1BB, a transmembrane glycoprotein presents on activated effector T cells (combination with a 4-1BB agonist monoclonal antibodies) and TGF-β (combination with a monoclonal antibody targeting TGF-β or with a bispecific antibodies targeting both PD-1 and TGF-β) (90).
PRIMMO study tried to modulate the TME in an inexpensive manner with repurposed compounds (i.e. drugs approved for another indication) with (immune) modulating properties (63). However, this study failed to improve ORR. Thus, despite the promising preliminary evidence and biological potential of these compounds, these results emphasize that additional studies are needed to obtain clinical evidence of their benefit.
The result of a preclinical study showed that cisplatin CT can enhance the abscopal effects induced by RT in association with ICI (91). This study highlights that efficiently attracting induced T cells to unirradiated tumors is an interesting alternative to obtain an abscopal effect in addition to the induction of tumor-specific T cells. This finding is particularly interesting given that cisplatin is the most widely used CT for CC.
Pre-clinical results have shown that modulated electro-hyperthermia (mEHT) associated with IT would be able to induce an immune mediated response (92). A phase III randomized controlled trial has evaluated the combination of mEHT with CRT in LACC (93). The rate of complete metabolic response (CMR) was higher in the mEHT group than in the control group 24.1% vs. 5.6% (p = 0.013), both within and outside the radiation fields. Thus, obtaining a CMR from an unirradiated tumor shows that combining mEHT with ICI and RT treatment would increase the abscopal effect. It can be assumed that RT and mEHT have a synergistic action that could be used in the treatment of metastatic disease treated with ICIs.
5.3 Biomarkers
To improve the ratio of ICI + RT, it is essential to identify the subpopulation of patients who benefit the most. Various grading systems and thresholds have been identified to assess PD-L1 expression by immunohistochemistry. However, these biomarkers have only been used as biomarkers in clinical trials of ICIs in CC and not in the RT + ICI combination. They include the ratio of PD-L1-stained tumor cells (TCs) to the total number of viable TCs, the combined positive score (CPS) which is defined as the total number of PD-L1-stained cells (including TCs, lymphocytes, and macrophages) divided by the number of all viable TCs, then multiplied by 100 (94).
Moreover, studies have shown that it is possible to identify patients at risk of polymetastatic progression from patients who will have oligometastatic progression using molecular biomarkers (95). Thus, one could avoid proposing RT to patients at risk of polymetastatic progression. In agreement with this concept, a study of colorectal cancer liver metastases suggested the use of a molecular classification to identify patients at risk of progression after metastasectomy (96). This could be a relevant tool to select patients with oligometastatic tumors eligible for curative treatment, i.e. who could benefit from the combination of RT and ICIs.
Finally, an ongoing study (NCT04574635) collects blood samples to assess if the DNA of HPV that causes cervical cancer can be detected in patients with CC that is new (primary), recurrent, or metastatic and are undergoing treatment with surgery, RT, CT, and/or ICI. The main objective is to predict response of the cervical cancer to treatment and detect recurrent cancer sooner.
6 Conclusion
Currently, there is no clinical proof of abscopal effect in advanced, recurrent or metastatic CC with the combination of RT and ICI. Thus, the results of the ongoing prospective randomized trials combining RT and ICIs will be very informative. Research avenues concern new strategies to improve the therapeutic index of this combination including manipulation of the tumor microenvironment, by combining new drugs such as 4-1BB agonist monoclonal antibodies or bispecific antibodies targeting both PD-1 and TGF-β, and reflection on the type of RT to be combined with ICIs, mainly techniques enabling high doses to be delivered per fraction such as SBRT or brachytherapy.
Author contributions
Conceptualization, FL and LO; methodology, FL and LO; investigation, FL and LO; data curation, FL, CM, ER, ED and LO; writing—original draft preparation, LO and FL; writing—review and editing, FL, CM, ER, ED and LO. All authors have read and agreed to the published version of the manuscript.
Funding
The authors declare that no financial support was received for the research, authorship, and/or publication of this article.
Conflict of interest
The authors declare that the research was conducted in the absence of any commercial or financial relationships that could be construed as a potential conflict of interest.
Publisher’s note
All claims expressed in this article are solely those of the authors and do not necessarily represent those of their affiliated organizations, or those of the publisher, the editors and the reviewers. Any product that may be evaluated in this article, or claim that may be made by its manufacturer, is not guaranteed or endorsed by the publisher.
References
1. Sung H, Ferlay J, Siegel RL, Laversanne M, Soerjomataram I, Jemal A, et al. Global cancer statistics 2020: GLOBOCAN estimates of incidence and mortality worldwide for 36 cancers in 185 countries. CA Cancer J Clin (2021) 71:209–49. doi: 10.3322/caac.21660
2. Ibrahim A, Rasch V, Pukkala E, Aro AR. Predictors of cervical cancer being at an advanced stage at diagnosis in Sudan. Int J Womens Health (2011) 3:385–9. doi: 10.2147/IJWH.S21063
3. Liu Y, Wu L, Tong R, Yang F, Yin L, Li M, et al. PD-1/PD-L1 inhibitors in cervical cancer. Front Pharmacol (2019) 10:65. doi: 10.3389/fphar.2019.00065
4. Gennigens C, De Cuypere M, Hermesse J, Kridelka F, Jerusalem G. Optimal treatment in locally advanced cervical cancer. Expert Rev Anticancer Ther (2021) 21:657–71. doi: 10.1080/14737140.2021.1879646
5. Gennigens C, Jerusalem G, Lapaille L, De Cuypere M, Streel S, Kridelka F, et al. Recurrent or primary metastatic cervical cancer: current and future treatments. ESMO Open (2022) 7:100579. doi: 10.1016/j.esmoop.2022.100579
6. Cibula D, Pötter R, Planchamp F, Avall-Lundqvist E, Fischerova D, Haie Meder C, et al. The European society of gynaecological Oncology/European society for radiotherapy and Oncology/European society of pathology guidelines for the management of patients with cervical cancer. Radiother Oncol (2018) 127:404–16. doi: 10.1016/j.radonc.2018.03.003
7. Palma DA, Olson R, Harrow S, Gaede S, Louie AV, Haasbeek C, et al. Stereotactic ablative radiotherapy for the comprehensive treatment of oligometastatic cancers: long-term results of the SABR-COMET phase II randomized trial. J Clin Oncol (2020) 38:2830–8. doi: 10.1200/JCO.20.00818
8. Palma DA, Olson R, Harrow S, Gaede S, Louie AV, Haasbeek C, et al. Stereotactic ablative radiotherapy versus standard of care palliative treatment in patients with oligometastatic cancers (SABR-COMET): a randomised, phase 2, open-label trial. Lancet. (2019) 393:2051–8. doi: 10.1016/S0140-6736(18)32487-5
9. Thigpen T, Shingleton H, Homesley H, Lagasse L, Blessing J. Cis-platinum in treatment of advanced or recurrent squamous cell carcinoma of the cervix: a phase II study of the gynecologic oncology group. Cancer. (1981) 48:899–903. doi: 10.1002/1097-0142(19810815)48:4<899::AID-CNCR2820480406>3.0.CO;2-6
10. Monk BJ, Sill MW, McMeekin DS, Cohn DE, Ramondetta LM, Boardman CH, et al. Phase III trial of four cisplatin-containing doublet combinations in stage IVB, recurrent, or persistent cervical carcinoma: a gynecologic oncology group study. J Clin Oncol (2009) 27:4649–55. doi: 10.1200/JCO.2009.21.8909
11. Kitagawa R, Katsumata N, Shibata T, Kamura T, Kasamatsu T, Nakanishi T, et al. Paclitaxel plus carboplatin versus paclitaxel plus cisplatin in metastatic or recurrent cervical cancer: the open-label randomized phase III trial JCOG0505. J Clin Oncol (2015) 33:2129–35. doi: 10.1200/JCO.2014.58.4391
12. Minion LE, Tewari KS. Cervical cancer - state of the science: from angiogenesis blockade to checkpoint inhibition. Gynecol Oncol (2018) 148:609–21. doi: 10.1016/j.ygyno.2018.01.009
13. Tewari KS, Sill MW, Penson RT, Huang H, Ramondetta LM, Landrum LM, et al. Bevacizumab for advanced cervical cancer: final overall survival and adverse event analysis of a randomised, controlled, open-label, phase 3 trial (Gynecologic oncology group 240). Lancet. (2017) 390:1654–63. doi: 10.1016/S0140-6736(17)31607-0
14. Tewari KS, Sill MW, Long HJ, Penson RT, Huang H, Ramondetta LM, et al. Improved survival with bevacizumab in advanced cervical cancer. N Engl J Med (2014) 370:734–43. doi: 10.1056/NEJMoa1309748
15. Lee S-M, Lee S, Cho H-W, Min K-J, Hong J-H, Song J-Y, et al. Application of immune checkpoint inhibitors in gynecological cancers: what do gynecologists need to know before using immune checkpoint inhibitors? Int J Mol Sci (2023) 24:974. doi: 10.3390/ijms24020974
16. Wakabayashi R, Nakahama Y, Nguyen V, Espinoza JL. The host-microbe interplay in human papillomavirus-induced carcinogenesis. Microorganisms. (2019) 7:199. doi: 10.3390/microorganisms7070199
17. Heeren AM, Punt S, Bleeker MC, Gaarenstroom KN, van der Velden J, Kenter GG, et al. Prognostic effect of different PD-L1 expression patterns in squamous cell carcinoma and adenocarcinoma of the cervix. Mod Pathol (2016) 29:753–63. doi: 10.1038/modpathol.2016.64
18. Mezache L, Paniccia B, Nyinawabera A, Nuovo GJ. Enhanced expression of PD L1 in cervical intraepithelial neoplasia and cervical cancers. Mod Pathol (2015) 28:1594–602. doi: 10.1038/modpathol.2015.108
19. Kosmaczewska A, Bocko D, Ciszak L, Wlodarska-Polinska I, Kornafel J, Szteblich A, et al. Dysregulated expression of both the costimulatory CD28 and inhibitory CTLA-4 molecules in PB T cells of advanced cervical cancer patients suggests systemic immunosuppression related to disease progression. Pathol Oncol Res (2012) 18:479–89. doi: 10.1007/s12253-011-9471-y
20. Cao Y, Zhou X, Huang X, Li Q, Gao L, Jiang L, et al. Tim-3 expression in cervical cancer promotes tumor metastasis. PloS One (2013) 8:e53834. doi: 10.1371/journal.pone.0053834
21. Shah W, Yan X, Jing L, Zhou Y, Chen H, Wang Y. A reversed CD4/CD8 ratio of tumor-infiltrating lymphocytes and a high percentage of CD4(+)FOXP3(+) regulatory T cells are significantly associated with clinical outcome in squamous cell carcinoma of the cervix. Cell Mol Immunol (2011) 8:59–66. doi: 10.1038/cmi.2010.56
22. Piersma SJ, Jordanova ES, van Poelgeest MIE, Kwappenberg KMC, van der Hulst JM, Drijfhout JW, et al. High number of intraepithelial CD8+ tumor-infiltrating lymphocytes is associated with the absence of lymph node metastases in patients with large early-stage cervical cancer. Cancer Res (2007) 67:354–61. doi: 10.1158/0008-5472.CAN-06-3388
23. Chalmers ZR, Connelly CF, Fabrizio D, Gay L, Ali SM, Ennis R, et al. Analysis of 100,000 human cancer genomes reveals the landscape of tumor mutational burden. Genome Med (2017) 9:34. doi: 10.1186/s13073-017-0424-2
24. Alexandrov LB, Nik-Zainal S, Wedge DC, Aparicio SAJR, Behjati S, Biankin AV, et al. Signatures of mutational processes in human cancer. Nature. (2013) 500:415–21. doi: 10.1038/nature12477
25. Song Z, Zou K, Zou L. Immune checkpoint blockade for locally advanced or recurrent/metastatic cervical cancer: an update on clinical data. Front Oncol (2022) 12:1045481. doi: 10.3389/fonc.2022.1045481
26. O’Malley DM, Oaknin A, Monk BJ, Selle F, Rojas C, Gladieff L, et al. Phase II study of the safety and efficacy of the anti-PD-1 antibody balstilimab in patients with recurrent and/or metastatic cervical cancer. Gynecol Oncol (2021) 163:274–80. doi: 10.1016/j.ygyno.2021.08.018
27. Chung HC, Ros W, Delord J-P, Perets R, Italiano A, Shapira-Frommer R, et al. Efficacy and safety of pembrolizumab in previously treated advanced cervical cancer: results from the phase II KEYNOTE-158 study. J Clin Oncol (2019) 37:1470–8. doi: 10.1200/JCO.18.01265
28. Naumann RW, Hollebecque A, Meyer T, Devlin M-J, Oaknin A, Kerger J, et al. Safety and efficacy of nivolumab monotherapy in recurrent or metastatic cervical, vaginal, or vulvar carcinoma: results from the phase I/II CheckMate 358 trial. J Clin Oncol (2019) 37:2825–34. doi: 10.1200/JCO.19.00739
29. Tamura K, Hasegawa K, Katsumata N, Matsumoto K, Mukai H, Takahashi S, et al. Efficacy and safety of nivolumab in Japanese patients with uterine cervical cancer, uterine corpus cancer, or soft tissue sarcoma: multicenter, open-label phase 2 trial. Cancer Sci (2019) 110:2894–904. doi: 10.1111/cas.14148
30. Santin AD, Deng W, Frumovitz M, Buza N, Bellone S, Huh W, et al. Phase II evaluation of nivolumab in the treatment of persistent or recurrent cervical cancer (NCT02257528/NRG-GY002). Gynecol Oncol (2020) 157:161–6. doi: 10.1016/j.ygyno.2019.12.034
31. Tewari KS, Monk BJ, Vergote I, Miller A, de Melo AC, Kim H-S, et al. Survival with cemiplimab in recurrent cervical cancer. N Engl J Med (2022) 386:544–55. doi: 10.1056/NEJMoa2112187
32. Lheureux S, Butler MO, Clarke B, Cristea MC, Martin LP, Tonkin K, et al. Association of ipilimumab with safety and antitumor activity in women with metastatic or recurrent human papillomavirus-related cervical carcinoma. JAMA Oncol (2018) 4:e173776. doi: 10.1001/jamaoncol.2017.3776
33. An J, Tang J, Li BX, Xiong H, Qiu H, Luo L, et al. Efficacy and safety of the anti-PD-L1 mAb socazolimab for recurrent or metastatic cervical cancer: a phase I dose-escalation and expansion study. Clin Cancer Res (2022) 28:5098–106. doi: 10.1158/1078-0432.CCR-22-1280
34. Strauss J, Gatti-Mays ME, Cho BC, Hill A, Salas S, McClay E, et al. Bintrafusp alfa, a bifunctional fusion protein targeting TGF-β and PD-L1, in patients with human papillomavirus-associated malignancies. J Immunother Cancer. (2020) 8:e001395. doi: 10.1136/jitc-2020-001395
35. Wu X, Ji J, Lou H, Li Y, Feng M, Xu N, et al. Efficacy and safety of cadonilimab, an anti-PD-1/CTLA4 bi-specific antibody, in previously treated recurrent or metastatic (R/M) cervical cancer: a multicenter, open-label, single-arm, phase II trial (075). Gynecologic Oncol (2022) 166:S47–8. doi: 10.1016/S0090-8258(22)01293-8
36. Cheng M, Wang H, Zhao Y, Li G. Efficacy and prognostic factors for response to PD-1 inhibitors in advanced cervical carcinoma: a retrospective study. Drug Des Devel Ther (2022) 16:887–97. doi: 10.2147/DDDT.S358302
37. Wang J, Lou H, Cai H-B, Huang X, Li G, Wang L, et al. A study of AK104 (an anti-PD1 and anti-CTLA4 bispecific antibody) combined with standard therapy for the first-line treatment of persistent, recurrent, or metastatic cervical cancer (R/M CC). JCO (2022) 40 16_suppl:106–6. doi: 10.1200/JCO.2022.40.16_suppl.106
38. Friedman CF, Snyder Charen A, Zhou Q, Carducci MA, Buckley De Meritens A, Corr BR, et al. Phase II study of atezolizumab in combination with bevacizumab in patients with advanced cervical cancer. J Immunother Cancer. (2020) 8:e001126. doi: 10.1136/jitc-2020-001126
39. An J, Wu L, Li X, Wang J, Hou X, Wang Q, et al. Efficacy and safety of serplulimab (an anti-PD-1 antibody) combined with albumin-bound paclitaxel in patients with advanced cervical cancer who have progressive disease or intolerable toxicity after first-line standard chemotherapy (074). Gynecologic Oncol (2022) 166:S47. doi: 10.1016/S0090-8258(22)01292-6
40. Lan C, Shen J, Wang Y, Li J, Liu Z, He M, et al. Camrelizumab plus apatinib in patients with advanced cervical cancer (CLAP): a multicenter, open-label, single-arm, phase II trial. J Clin Oncol (2020) 38:4095–106. doi: 10.1200/JCO.20.01920
41. Xu Q, Wang J, Sun Y, Lin Y, Liu J, Zhuo Y, et al. Efficacy and safety of sintilimab plus anlotinib for PD-L1-Positive recurrent or metastatic cervical cancer: a multicenter, single-arm, prospective phase II trial. J Clin Oncol (2022) 40:1795–805. doi: 10.1200/JCO.21.02091
42. Oaknin A, Backes F, Nieuwenhuysen EV, Eskander R, González-Martín A, Makker V, et al. LIO-1: initial phase 2 experience of lucitanib + nivolumab in patients with metastatic or recurrent cervical cancer (NCT04042116; ENGOT-GYN3/AGO/LIO) (034). Gynecologic Oncol (2022) 166:S24. doi: 10.1016/S0090-8258(22)01252-5
43. Colombo N, Dubot C, Lorusso D, Caceres MV, Hasegawa K, Shapira-Frommer R, et al. Pembrolizumab for persistent, recurrent, or metastatic cervical cancer. N Engl J Med (2021) 385:1856–67. doi: 10.1056/NEJMoa2112435
44. Park JS, Hur S-Y, Lim MC, Kim YM, No JH, Kim B-G, et al. Efficacy and safety results of GX-188E, a therapeutic DNA vaccine, combined with pembrolizumab administration in patients with HPV 16- and/or 18- positive advanced cervical cancer: phase II interim analysis results (KEYNOTE-567). JCO (2021) 39 15_suppl:5511–1. doi: 10.1200/JCO.2021.39.15_suppl.5511
45. O’Malley DM, Neffa M, Monk BJ, Melkadze T, Huang M, Kryzhanivska A, et al. Dual PD-1 and CTLA-4 checkpoint blockade using balstilimab and zalifrelimab combination as second-line treatment for advanced cervical cancer: an open-label phase II study. J Clin Oncol (2022) 40:762–71. doi: 10.1200/JCO.21.02067
46. Lorusso D, Vergote I, O’Cearbhaill RE, Westermann AM, Banerjee SN, Van Nieuwenhuysen E, et al. Tisotumab vedotin (TV) + pembrolizumab (pembro) in first-line (1L) recurrent or metastatic cervical cancer (r/mCC): interim results of ENGOT Cx8/GOG 3024/innovaTV 205. JCO (2022) 40 16_suppl:5507–7. doi: 10.1200/JCO.2022.40.16_suppl.5507
47. Yin H, Guo W, Sun X, Li R, Feng C, Tan Y. TILs and anti-PD1 therapy: an alternative combination therapy for PDL1 negative metastatic cervical cancer. J Immunol Res (2020) 2020:8345235. doi: 10.1155/2020/8345235
48. Mole RH. Whole body irradiation; radiobiology or medicine? Br J Radiol (1953) 26:234–41. doi: 10.1259/0007-1285-26-305-234
49. Apetoh L, Tesniere A, Ghiringhelli F, Kroemer G, Zitvogel L. Molecular interactions between dying tumor cells and the innate immune system determine the efficacy of conventional anticancer therapies. Cancer Res (2008) 68:4026–30. doi: 10.1158/0008-5472.CAN-08-0427
50. Sharabi AB, Lim M, DeWeese TL, Drake CG. Radiation and checkpoint blockade immunotherapy: radiosensitisation and potential mechanisms of synergy. Lancet Oncol (2015) 16:e498–509. doi: 10.1016/S1470-2045(15)00007-8
51. Boissonnas A, Fetler L, Zeelenberg IS, Hugues S, Amigorena S. In vivo imaging of cytotoxic T cell infiltration and elimination of a solid tumor. J Exp Med (2007) 204:345–56. doi: 10.1084/jem.20061890
52. Ribas A. Tumor immunotherapy directed at PD-1. N Engl J Med (2012) 366:2517–9. doi: 10.1056/NEJMe1205943
53. Deng L, Liang H, Burnette B, Beckett M, Darga T, Weichselbaum RR, et al. Irradiation and anti-PD-L1 treatment synergistically promote antitumor immunity in mice. J Clin Invest. (2014) 124:687–95. doi: 10.1172/JCI67313
54. Demaria S, Golden EB, Formenti SC. Role of local radiation therapy in cancer immunotherapy. JAMA Oncol (2015) 1:1325–32. doi: 10.1001/jamaoncol.2015.2756
55. Ollivier L, Orione C, Bore P, Misery L, Legoupil D, Leclere J-C, et al. Abscopal response in metastatic melanoma: real-world data of a retrospective, multicenter study. Cancers (Basel). (2022) 14:4213. doi: 10.3390/cancers14174213
56. Wood J, Yasmin-Karim S, Mueller R, Viswanathan AN, Ngwa W. Single radiotherapy fraction with local anti-CD40 therapy generates effective abscopal responses in mouse models of cervical cancer. Cancers (Basel). (2020) 12:1026. doi: 10.3390/cancers12041026
57. Beatty GL, Li Y, Long KB. Cancer immunotherapy: activating innate and adaptive immunity through CD40 agonists. Expert Rev Anticancer Ther (2017) 17:175–86. doi: 10.1080/14737140.2017.1270208
58. Hill SC, Youde SJ, Man S, Teale GR, Baxendale AJ, Hislop A, et al. Activation of CD40 in cervical carcinoma cells facilitates CTL responses and augments chemotherapy-induced apoptosis. J Immunol (2005) 174:41–50. doi: 10.4049/jimmunol.174.1.41
59. Liu C, Yu H, Huang R, Lei T, Li X, Liu M, et al. Radioimmunotherapy-induced intratumoral changes in cervical squamous cell carcinoma at single-cell resolution. Cancer Commun (Lond). (2022) 42:1407–11. doi: 10.1002/cac2.12342
60. Nance ME, Biedermann GB, Bhat AP, Davis RM. Chemorefractory liver metastasis from cervical cancer successfully treated with a combination of yttrium-90 and immunotherapy. Radiol Case Rep (2020) 15:1359–65. doi: 10.1016/j.radcr.2020.06.010
61. Golden EB, Chhabra A, Chachoua A, Adams S, Donach M, Fenton-Kerimian M, et al. Local radiotherapy and granulocyte-macrophage colony-stimulating factor to generate abscopal responses in patients with metastatic solid tumours: a proof-of-principle trial. Lancet Oncol (2015) 16:795–803. doi: 10.1016/S1470-2045(15)00054-6
62. Papadopoulos KP, Johnson ML, Lockhart AC, Moore K, Falchook GS, Formenti SC, et al. First-In-Human study of cemiplimab alone or in combination with radiotherapy and/or low-dose cyclophosphamide in patients with advanced malignancies. Clin Cancer Res (2020) 26:1025–33. doi: 10.1158/1078-0432.CCR-19-2609
63. De Jaeghere EA, Tuyaerts S, Van Nuffel AMT, Belmans A, Bogaerts K, Baiden-Amissah R, et al. Pembrolizumab, radiotherapy, and an immunomodulatory five-drug cocktail in pretreated patients with persistent, recurrent, or metastatic cervical or endometrial carcinoma: results of the phase II PRIMMO study. Cancer Immunol Immunother. (2023) 72:475–91. doi: 10.1007/s00262-022-03253-x
64. Da Silva DM, Enserro DM, Mayadev JS, Skeate JG, Matsuo K, Pham HQ, et al. Immune activation in patients with locally advanced cervical cancer treated with ipilimumab following definitive chemoradiation (GOG-9929). Clin Cancer Res (2020) 26:5621–30. doi: 10.1158/1078-0432.CCR-20-0776
65. Buqué A, Bloy N, Perez-Lanzón M, Iribarren K, Humeau J, Pol JG, et al. Immunoprophylactic and immunotherapeutic control of hormone receptor-positive breast cancer. Nat Commun (2020) 11:3819. doi: 10.1038/s41467-020-17644-0
66. Colton M, Cheadle EJ, Honeychurch J, Illidge TM. Reprogramming the tumour microenvironment by radiotherapy: implications for radiotherapy and immunotherapy combinations. Radiat Oncol (2020) 15:254. doi: 10.1186/s13014-020-01678-1
67. Formenti SC, Demaria S. Combining radiotherapy and cancer immunotherapy: a paradigm shift. J Natl Cancer Inst (2013) 105:256–65. doi: 10.1093/jnci/djs629
68. Saddawi-Konefka R, O’Farrell A, Faraji F, Clubb L, Allevato MM, Jensen SM, et al. Lymphatic-preserving treatment sequencing with immune checkpoint inhibition unleashes cDC1-dependent antitumor immunity in HNSCC. Nat Commun (2022) 13:4298. doi: 10.1038/s41467-022-31941-w
69. Altorki NK, McGraw TE, Borczuk AC, Saxena A, Port JL, Stiles BM, et al. Neoadjuvant durvalumab with or without stereotactic body radiotherapy in patients with early-stage non-small-cell lung cancer: a single-centre, randomised phase 2 trial. Lancet Oncol (2021) 22:824–35. doi: 10.1016/S1470-2045(21)00149-2
70. Lee NY, Ferris RL, Psyrri A, Haddad RI, Tahara M, Bourhis J, et al. Avelumab plus standard-of-care chemoradiotherapy versus chemoradiotherapy alone in patients with locally advanced squamous cell carcinoma of the head and neck: a randomised, double-blind, placebo-controlled, multicentre, phase 3 trial. Lancet Oncol (2021) 22:450–62. doi: 10.1016/S1470-2045(20)30737-3
71. da Costa SCS, Bonadio RC, Gabrielli FCG, Aranha AS, Dias Genta MLN, Miranda VC, et al. Neoadjuvant chemotherapy with cisplatin and gemcitabine followed by chemoradiation versus chemoradiation for locally advanced cervical cancer: a randomized phase II trial. J Clin Oncol (2019) 37:3124–31. doi: 10.1200/JCO.19.00674
72. Zhao X, Shao C. Radiotherapy-mediated immunomodulation and anti-tumor abscopal effect combining immune checkpoint blockade. Cancers (Basel). (2020) 12:2762. doi: 10.3390/cancers12102762
73. Yamazaki T, Kirchmair A, Sato A, Buqué A, Rybstein M, Petroni G, et al. Mitochondrial DNA drives abscopal responses to radiation that are inhibited by autophagy. Nat Immunol (2020) 21:1160–71. doi: 10.1038/s41590-020-0751-0
74. Vanpouille-Box C, Alard A, Aryankalayil MJ, Sarfraz Y, Diamond JM, Schneider RJ, et al. DNA Exonuclease Trex1 regulates radiotherapy-induced tumour immunogenicity. Nat Commun (2017) 8:15618. doi: 10.1038/ncomms15618
75. Demaria S, Guha C, Schoenfeld J, Morris Z, Monjazeb A, Sikora A, et al. Radiation dose and fraction in immunotherapy: one-size regimen does not fit all settings, so how does one choose? J Immunother Cancer (2021) 9:e002038. doi: 10.1136/jitc-2020-002038
76. Rodriguez-Ruiz ME, Rodriguez I, Barbes B, Mayorga L, Sanchez-Paulete AR, Ponz-Sarvise M, et al. Brachytherapy attains abscopal effects when combined with immunostimulatory monoclonal antibodies. Brachytherapy. (2017) 16:1246–51. doi: 10.1016/j.brachy.2017.06.012
77. Patel RR, He K, Barsoumian HB, Chang JY, Tang C, Verma V, et al. High-dose irradiation in combination with non-ablative low-dose radiation to treat metastatic disease after progression on immunotherapy: results of a phase II trial. Radiother Oncol (2021) 162:60–7. doi: 10.1016/j.radonc.2021.06.037
78. Cavalieri S, Vitolo V, Barcellini A, Ronchi S, Facoetti A, Campo C, et al. Immune checkpoint inhibitors and carbon iON radiotherapy in solid cancers with stable disease (ICONIC). Future Oncol (2023) 19:193–203. doi: 10.2217/fon-2022-0503
79. Grassberger C, Ellsworth SG, Wilks MQ, Keane FK, Loeffler JS. Assessing the interactions between radiotherapy and antitumour immunity. Nat Rev Clin Oncol (2019) 16:729–45. doi: 10.1038/s41571-019-0238-9
80. Yovino S, Kleinberg L, Grossman SA, Narayanan M, Ford E. The etiology of treatment-related lymphopenia in patients with malignant gliomas: modeling radiation dose to circulating lymphocytes explains clinical observations and suggests methods of modifying the impact of radiation on immune cells. Cancer Invest. (2013) 31:140–4. doi: 10.3109/07357907.2012.762780
81. Venkatesulu B, Giridhar P, Pujari L, Chou B, Lee JH, Block AM, et al. Lymphocyte sparing normal tissue effects in the clinic (LymphoTEC): a systematic review of dose constraint considerations to mitigate radiation-related lymphopenia in the era of immunotherapy. Radiother Oncol (2022) 177:81–94. doi: 10.1016/j.radonc.2022.10.019
82. Ghosh S, Huang J, Inkman M, Zhang J, Thotala S, Tikhonova E, et al. Radiation-induced circulating myeloid-derived suppressor cells induce systemic lymphopenia after chemoradiotherapy in patients with glioblastoma. Sci Transl Med (2023) 15:eabn6758. doi: 10.1126/scitranslmed.abn6758
83. Schernberg A, Blanchard P, Chargari C, Deutsch E. Neutrophils, a candidate biomarker and target for radiation therapy? Acta Oncol (2017) 56:1522–30. doi: 10.1080/0284186X.2017.1348623
84. Shimura T, Sharma P, Sharma GG, Banwait JK, Goel A. Enhanced anti-cancer activity of andrographis with oligomeric proanthocyanidins through activation of metabolic and ferroptosis pathways in colorectal cancer. Sci Rep (2021) 11:7548. doi: 10.1038/s41598-021-87283-y
85. Wei J, Montalvo-Ortiz W, Yu L, Krasco A, Ebstein S, Cortez C, et al. Sequence of αPD-1 relative to local tumor irradiation determines the induction of abscopal antitumor immune responses. Sci Immunol (2021) 6:eabg0117. doi: 10.1126/sciimmunol.abg0117
86. Brooks ED, Chang JY. Time to abandon single-site irradiation for inducing abscopal effects. Nat Rev Clin Oncol (2019) 16:123–35. doi: 10.1038/s41571-018-0119-7
87. Blackburn SD, Shin H, Haining WN, Zou T, Workman CJ, Polley A, et al. Coregulation of CD8+ T cell exhaustion by multiple inhibitory receptors during chronic viral infection. Nat Immunol (2009) 10:29–37. doi: 10.1038/ni.1679
88. Predina JD, Judy B, Aliperti LA, Fridlender ZG, Blouin A, Kapoor V, et al. Neoadjuvant in situ gene-mediated cytotoxic immunotherapy improves postoperative outcomes in novel syngeneic esophageal carcinoma models. Cancer Gene Ther (2011) 18:871–83. doi: 10.1038/cgt.2011.56
89. Xiao A, Luke JJ, Partouche J, Karrison T, Chmura SJ, Al-Hallaq HA. Evaluation of dose distribution to organs-at-Risk in a prospective phase 1 trial of pembrolizumab and multisite stereotactic body radiation therapy (SBRT). Pract Radiat Oncol (2022) 12:68–77. doi: 10.1016/j.prro.2021.09.005
90. Lucia F, Geier M, Schick U, Bourbonne V. Narrative review of synergistics effects of combining immunotherapy and stereotactic radiation therapy. Biomedicines. (2022) 10:1414. doi: 10.3390/biomedicines10061414
91. Luo R, Firat E, Gaedicke S, Guffart E, Watanabe T, Niedermann G. Cisplatin facilitates radiation-induced abscopal effects in conjunction with PD-1 checkpoint blockade through CXCR3/CXCL10-mediated T-cell recruitment. Clin Cancer Res (2019) 25:7243–55. doi: 10.1158/1078-0432.CCR-19-1344
92. Tsang Y-W, Huang C-C, Yang K-L, Chi M-S, Chiang H-C, Wang Y-S, et al. Improving immunological tumor microenvironment using electro-hyperthermia followed by dendritic cell immunotherapy. BMC Cancer. (2015) 15:708. doi: 10.1186/s12885-015-1690-2
93. Minnaar CA, Kotzen JA, Ayeni OA, Vangu M-D-T, Baeyens A. Potentiation of the abscopal effect by modulated electro-hyperthermia in locally advanced cervical cancer patients. Front Oncol (2020) 10:376. doi: 10.3389/fonc.2020.00376
94. Omenai SA, Ajani MA, Okolo CA. Programme death ligand 1 expressions as a surrogate for determining immunotherapy in cervical carcinoma patients. PloS One (2022) 17:e0263615. doi: 10.1371/journal.pone.0263615
95. Lussier YA, Khodarev NN, Regan K, Corbin K, Li H, Ganai S, et al. Oligo- and polymetastatic progression in lung metastasis(es) patients is associated with specific microRNAs. PloS One (2012) 7:e50141. doi: 10.1371/journal.pone.0050141
Keywords: abscopal effect, immunotherapy, radiation therapy, cervical cancer, immune checkpoint inhibitors, metastastic cancer
Citation: Ollivier L, Moreau Bachelard C, Renaud E, Dhamelincourt E and Lucia F (2023) The abscopal effect of immune-radiation therapy in recurrent and metastatic cervical cancer: a narrative review. Front. Immunol. 14:1201675. doi: 10.3389/fimmu.2023.1201675
Received: 06 April 2023; Accepted: 30 June 2023;
Published: 19 July 2023.
Edited by:
Yan Feng, Memorial Sloan Kettering Cancer Center, United StatesReviewed by:
Yona Keisari, Tel Aviv University, IsraelCarrie Anne Minnaar, Wits University Donald Gordon Medical Centre, South Africa
Copyright © 2023 Ollivier, Moreau Bachelard, Renaud, Dhamelincourt and Lucia. This is an open-access article distributed under the terms of the Creative Commons Attribution License (CC BY). The use, distribution or reproduction in other forums is permitted, provided the original author(s) and the copyright owner(s) are credited and that the original publication in this journal is cited, in accordance with accepted academic practice. No use, distribution or reproduction is permitted which does not comply with these terms.
*Correspondence: Francois Lucia, francois.lucia@gmail.com