- 1Wal-yan Respiratory Research Centre, Telethon Kids Institute, University of Western Australia, Nedlands, WA, Australia
- 2Medical School, University of Western Australia, Nedlands, WA, Australia
- 3Wesfarmers Centre of Vaccines and Infectious Diseases, Telethon Kids Institute, Nedlands, WA, Australia
- 4Department of Infectious Diseases, Perth Children’s Hospital, Nedlands, WA, Australia
- 5Menzies School of Health Research, Charles Darwin University, Darwin, NT, Australia
- 6School of Biomedical Sciences, University of Western Australia, Nedlands, WA, Australia
- 7Telethon Kids Institute, University of Western Australia, Nedlands, WA, Australia
- 8Department of Pediatrics, University of British Columbia, Vancouver, BC, Canada
- 9Department of Respiratory and Sleep Medicine, Perth Children’s Hospital, Perth, WA, Australia
- 10Centre for Cell Therapy and Regenerative Medicine, School of Medicine and Pharmacology, University of Western Australia and Harry Perkins Institute of Medical Research, Nedlands, WA, Australia
- 11School of Population Health, Curtin University, Perth, WA, Australia
The global population has been severely affected by the coronavirus disease 2019 (COVID-19) pandemic, however, with older age identified as a risk factor, children have been underprioritized. This article discusses the factors contributing to the less severe response observed in children following infection with severe acute respiratory syndrome coronavirus 2 (SARS-CoV-2), including, differing viral entry receptor expression and immune responses. It also discusses how emerging and future variants could present a higher risk to children, including those with underlying comorbidities, in developing severe disease. Furthermore, this perspective discusses the differential inflammatory markers between critical and non-critical cases, as well as discussing the types of variants that may be more pathogenic to children. Importantly, this article highlights where more research is urgently required, in order to protect the most vulnerable of our children.
1 Introduction
The current coronavirus disease 2019 (COVID-19) pandemic has become common knowledge to most, including the general community, and is caused by the severe acute respiratory syndrome coronavirus 2 (SARS-CoV-2). Studies have identified that ‘older age’ and being ‘male’ remain primary risk factors for infection (1, 2). These along with underlying comorbidities or respiratory illness have been suggested to possibly increase susceptibility to infection and severe disease (2, 3). Furthermore, children <14 years appeared to have significantly lower susceptibility to infection, with seroprevalence of SARS-CoV-2 increasing with age (4). However, a recent systematic review did note variability in the reporting of age-specific infection rates with most estimated attack rates (AR) in pediatric groups now being comparable to adults (5). If true, it raises the question as to why children appear less susceptible to SARS-CoV-2 infection and associated disease?
The currently known clinical spectrum of COVID-19 in adults is broad with about 80% of presentations being mild, 15% needing hospitalization, and 5% requiring intensive care (6). These rates still appear far lower in children (cumulative hospitalization incidence was 49.7 per 100,000 children) as the pandemic continues (7). The disease presents predominantly as a respiratory illness (Figure 1), but presentation can range from asymptomatic infection to extrapulmonary manifestations (3). A common subsequent complication includes acute respiratory distress syndrome (ARDS), but infection with SARS-CoV-2 may also lead to other types of organ failure and death (2, 3). Most children do not present with respiratory illness, but higher proportion of fever, vomiting, and diarrhea are observed upon admission to hospital (Figure 1) (2, 8). They are also at risk of a rare complication with non-specific symptoms and organ dysfunction termed COVID-19 associated multisystem inflammatory syndrome in children (MIS-C), also termed pediatric inflammatory multisystem syndrome temporally associated with SARS‐CoV‐2 (PIMS‐TS) (9–11). These differences suggest a possible deviation in the underlying immune response of adults and children to the virus.
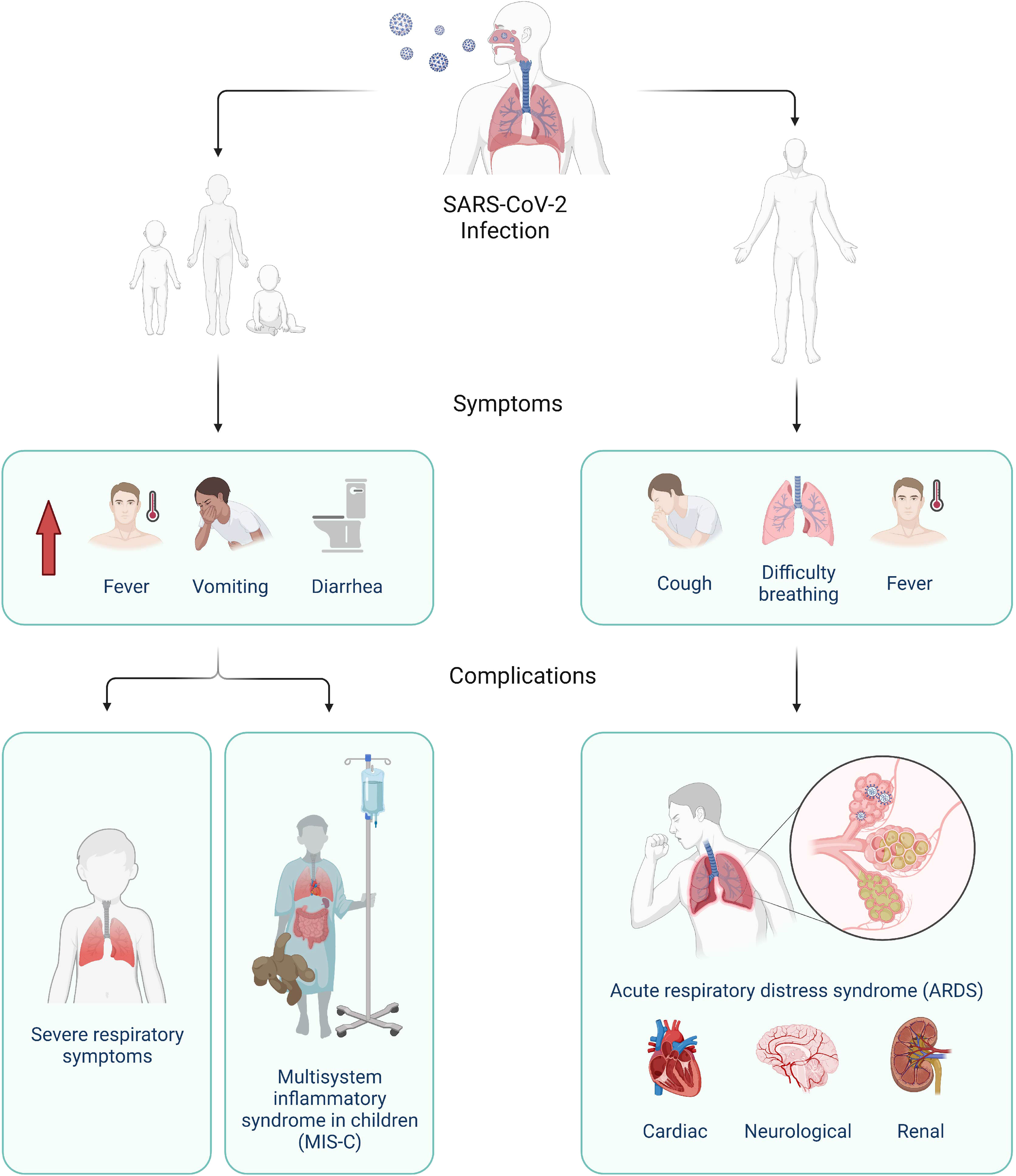
Figure 1 Clinical progression of SARS-CoV-2 infection in children (left) and adults (right). In children, higher proportion of fever, vomiting, and diarrhea were reported compared to adults and severe disease can manifest as severe respiratory symptoms or multisystem inflammatory syndrome (MIS-C), Adults typically present with common respiratory illness symptoms, which can lead to severe complications such as acute respiratory distress syndrome (ARDS) and as well as cardiac, neurological, and renal complications. Created with BioRender.com.
2 Immune responses
Viral pathogens typically bind to host receptors, which then stimulate appropriate immune responses to control infection. However, since these receptors interact with the virus, they can also facilitate viral entry into cells. Consequently, one of the main factors thought to affect SARS-CoV-2 infection susceptibility is the expression of its receptors and relevant host factors (enzymes), namely angiotensin converting enzyme 2 (ACE2) and transmembrane protease serine 2 (TMPRSS2), in the airways (12, 13). Interindividual variations of distribution and expression of these receptors in the upper airway have been suggested to influence the infectivity of SARS-CoV-2 (12). This has been supported by several other studies where higher receptor expression is associated with increased age and the male sex (Supplementary Table 1).
Collectively, these observations suggest that the lack of respiratory symptoms in children might be due to a distinct infection course resulting from the difference in receptor expression. The lower expression of these host receptors in children could account for reduced viral entry and hence a less severe course of infection. However, it should be noted, that several studies have reported no correlation between age or sex with ACE2 or TMPRSS2 (14–16) (Supplementary Table 1). How “children” have been defined as part of study inclusion criteria may have also contributed towards these conflicting observations. Some have used the term to broadly encompass children and adolescents between 0-17 years of age (15–17), whereas others have utilized a restricted age range, i.e. <10 years (13, 18). This is a likely factor for the inconsistency seen for ACE2 expression levels where younger children (<10 years) appear to have significantly lower ACE2 expression expression compared to their older counterparts (10-17 years) (19). Thus, a more standardized approach in the terminology used for age cohorts or in the study design regarding the inclusion criteria will assist in interpreting results generated in future studies. Multiple other host receptors and factors with affinity to SARS-CoV-2 have also started to be implicated in the infection process by providing alternative entry pathways and enhancing viral entry (Supplementary Table 1). As this is an extensive list of potential receptors, there is still a lack of corroborated information on the relationship between their expression levels, especially in children and the potential effects they might have on susceptibility to SARS-CoV-2 infection.
Studies have also investigated the initial innate immune responses of children compared to those of adults which have led to the discovery of multiple differences, both prior to and following SARS-CoV-2 infection (Supplementary Table 2 and Figure 2). Amongst these, children appear to be capable of eliciting a more immediate and stronger anti-viral response when infected as shown by significantly higher levels of IFNG and CCL5 expression in the nasal epithelium (15) (Figure 2). This stronger anti-viral response would allow for better control of SARS-CoV-2 replication in the airway and thereby, prevent the development of COVID-19-associated symptoms. This is in line with pediatric nasal epithelial cells being found to be less permissive to SARS-CoV-2 replication and mount a heightened antiviral response resulting in reduced viral replication following infection (20). Furthermore, lower viral loads were also found in upper respiratory tracts of asymptomatic children (<17 years) compared to children who present with symptoms (21). Interestingly, a very recent study by Koch and colleagues (16) did, however, report similar interferon gene responses in the nasal mucosa of children and adults infected with SARS-CoV-2.
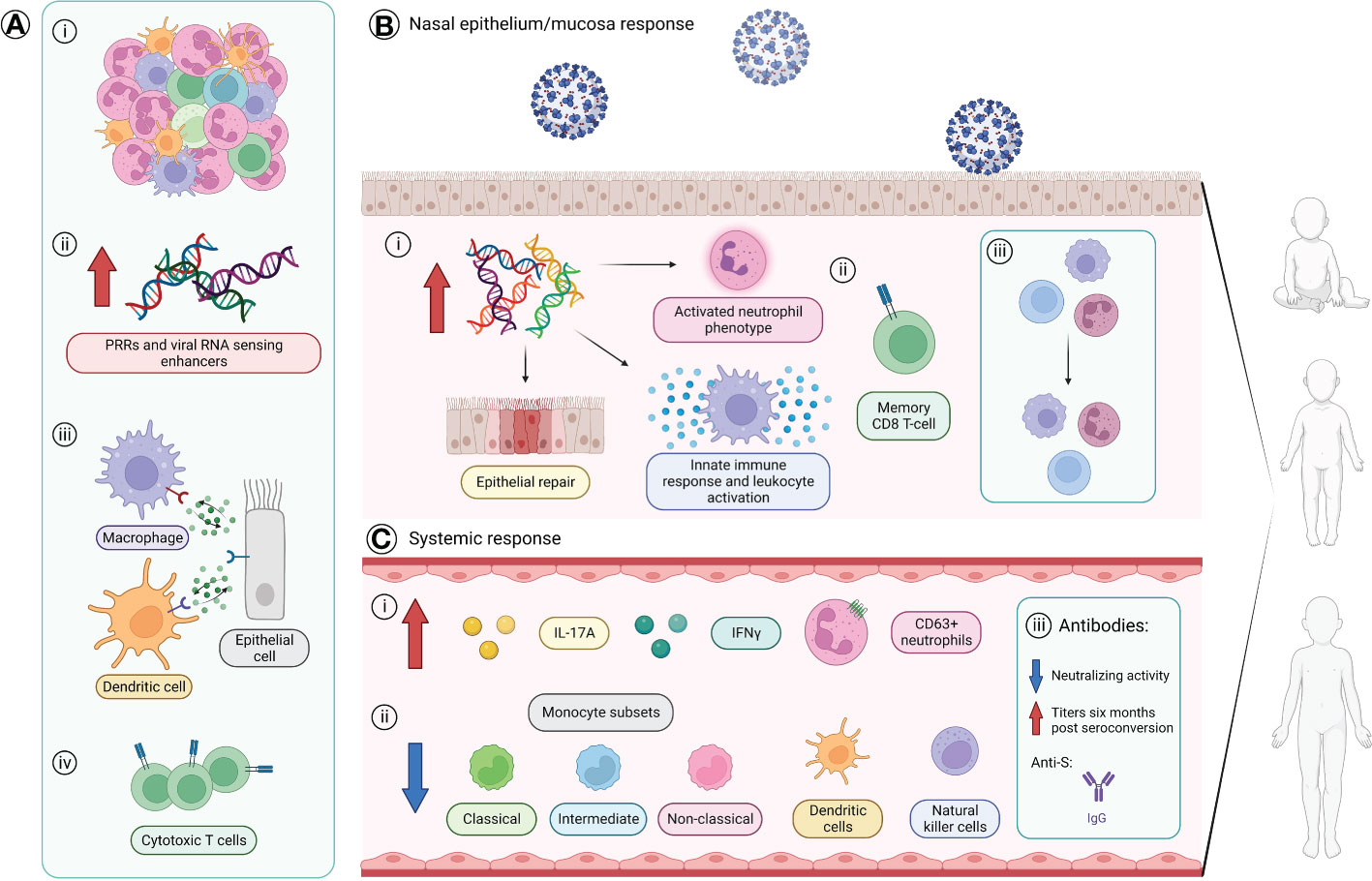
Figure 2 The age-specific innate and adaptive immune responses of children. (A) Baseline variances found in children compared to adults prior viral infection. (i) Greater number and diversity of immune cells with a neutrophil predominance. (ii) Higher levels of genes coding for SARS-CoV-2 pattern recognition receptors (PRRs) and viral RNA sensing enhancers. (iii) Stronger immune-epithelial cell cross-talk. (iv) Presence of a subpopulation of cytotoxic T-cells that allows preservation of virus-specific CD8+ T cell response without apoptosis. (B) Differences found in the nasal epithelium/mucosa immune response of children compared to adults following infection of SARS-CoV-2. Children are suggested to mount a stronger anti-viral response with: (i) increased gene expression levels involved with an activated neutrophil phenotype, epithelial repair, and innate immune responses such as response to TNF, leukocyte activation, and IFNG and CCL5 expression, and (ii) presence of a distinct CD8+ T cell population with a memory phenotype. (iii) Stable maintenance of the proportion of immune and epithelial cells in nasal epithelium of children. (C) Systemic immune differences found in the blood of children compared to adults. (i) Higher levels of serum IL-17A and IFNγ and circulating CD63+ neutrophils. (ii) Reduced proportions of circulating monocytes subsets (classical, intermediate, non-classical), dendritic cells, and natural killer cells. (iii) Antibody response in children primarily consists of anti-S protein IgG antibodies with an overall lower neutralizing activity, but children maintain higher antibody titers against the S protein and RBD six months after seroconversion. Created with BioRender.com. IFN, interferon; IL, interleukin; N, nucleocapsid; RBD, receptor-binding domain; S, spike; TNF, tumor necrosis factor.
SARS-CoV-2 infection in children also appears to reduce proportions of many immune cell types in the blood (Supplementary Table 2) (22–24). However, the observed higher serum IL-17 and IFNγ levels seen suggests that other cell types may be driving their production (22, 25). One such source may be CD8+IL-2-TNF+IFN-γ+ T-cells which have been found via flow cytometry to drive the SARS-CoV-2 T-cell response (26). Antibody responses also appear to differ between adults and children where, in children, the antibody response appears to be largely limited to anti-S IgG antibodies (Figure 2), unlike the broader response seen in adults (1). Furthermore, antibodies generated in children appear to have overall lower levels of neutralizing activity (1) (Figure 2). This appear in contrast to a recent study that reports similar antibody responses in seropositive children and adults to SARS-CoV-2 proteins (26). Distinct antibody profiles between children and adults are also seen with increased disease severity, where severely ill children with MIS-C show a broad non-specific IgG-driven monocyte-activating response, while adults with severe acute COVID-19 have enhanced IgA-related responses linked to neutrophil activation (27). As for the longevity of the humoral immunity, children have been reported to maintain higher antibody titers against the S protein and RBD compared to adults at least six months after seroconversion and these levels are only slightly reduced 12 months post-seroconversion (26). These results indicate the dire need for further studies to form an established understanding of the effects of age on the response to SARS-CoV-2 infection.
3 Multisystem inflammatory syndrome
In addition to severe COVID-19 typically seen in adults, SARS-CoV-2 infection may also drive a multisystem inflammatory response in children termed MIS-C or PIMS-TS (10, 22, 28, 29). Children who develop MIS-C are more likely to require intensive care with invasive ventilation, intravenous corticosteroids, vasoactive infusions, and inotropic support (11). They also typically present with leukocytosis with lymphopenia as well as high levels of systemic inflammation markers (24, 28). Work conducted has also identified lower lymphocyte counts in those with MIS-C compared to their non-MIS-C counterparts with acute presentations (25). Higher serum concentrations of IL‐1β, IL‐8, IL-10, TNFα, and IFNγ as well as more monocyte/antibody‐dependent cellular phagocytosis (ADCP) activity has also been associated with MIS-C (24, 25, 27, 29). Moreover, elevated pro-inflammatory cytokines (IL-6 and IL-17A) as well as chemokines (CXCL1, CXCL5, CXCL6, and CXCL11) including those involved with lymphocytic and myeloid chemotaxis and activation (CCL3 and CCL4, CCL19, and CXCL10) and mucosal chemotaxis (CCL20) were able to distinguish children with and without MIS-C (22). Variance in specific lymphocyte populations, namely lower CD56lo NK cells and CD4− T cells (mostly CD8+ T cells), have also been shown in MIS-C cohorts relative to infected non-MIS-C cohorts (10, 22).
Interestingly, similar antibody profiles have been exhibited in pediatric cohorts regardless of disease severity (with or without MIS-C) (1). This similarity may imply that the humoral immune response to the virus is not associated with MIS-C pathogenesis. However, Yonker et al. (9) did observe elevated IgM and IgG responses to the SARS-CoV-2 RBD and Bartsch et al. (27), observed higher SARS-CoV-2 S protein–specific IgM, IgG1 and IgA1 titers in children with severe MIS-C compared with mild MIS-C. Children with MIS-C were also found to have higher anti-S and anti-RBD IgG as well as anti-S IgA titers with higher neutralization activity compared with those that did not meet the criteria for MIS-C but had severe respiratory symptoms and required increased positive pressure support above their baseline (severe COVID-19) (30). Although differences in antibodies were not seen between the MIS-C and mild COVID-19 group (30) another study found higher titers of anti-S and anti-RBD IgG in children with MIS-C compared to children with COVID-19 (31). Variable levels of neutralizing antibodies were also found with the mild group (30). This suggests that children with MIS-C have greater capacity to more effectively neutralize SARS-CoV-2 compared to children with severe COVID-19 (30). Another distinction between the MIS-C and severe COVID-19 groups is higher TNFα and IL-10 proportions in the former (29). In addition, as autoreactive antibodies (with possible targets broadly found across tissues) have recently been observed with MIS-C, anomalous immune responses may also be promoted leading to systemic inflammation and multi-organ involvement (10, 22). These findings show that there are certainly specific mechanisms following SARS-CoV-2 infection that are involved with the divergence from the standard COVID-19 progression into the development of MIS-C, but there is still more to be investigated and their significance still needs to be validated.
4 Pediatric responses to SARS-CoV-2 variants
The combination of SARS-CoV-2 accumulating mutations in 1-2 nucleotides every month and the lack of effective containment strategies have given rise to the most recent issue of the pandemic, the emergence of new variants (Supplementary Table 3) (32). Work by Salleh, Derrick, and Deris (33) have performed an in-depth review of some of the earlier variants of concern (VoCs) which have all been suggested to have higher binding affinity to the human ACE2 receptor (except B.1.1.529 [Omicron]) and increased transmissibility (33, 34) (Supplementary Table 3); suggesting increasing adaptation of viral infectivity to humans but does not necessarily mean greater pathogenicity. Infection with B.1.617.2 (Delta) in primary Air Liquid Interface (ALI) cultures however, has revealed extensive nuclear damage and syncytial formation with increased replicative capacity compared to an ancestral Australian clinical strain suggesting possible increased pathogenicity (35). This is consistent with increased pathogenicity observed with Delta compared to a D614G-bearing isolate in a hamster model study (36). Aside from higher viral entry efficacies, B.1.1.7 (Alpha), B.1.351 (Beta), P.1 (Gamma), and Delta were also more resistant to the neutralizing activity of monoclonal antibodies and convalescent sera from recovered COVID-19 patients compared to the wildtype strain in vitro (33, 37, 38). Omicron has also been reported to escape clinically approved monoclonal antibodies, even in a combination which was highly potent against Delta (39). With multiple other variants identified since the original strain, the virus appears to be evolving, raising the potential for new variants to emerge in the future (32). Nonetheless, as the specific antigenicity of these variants have yet to be established, it also gives rise to the question of how each variant would differ in pathogenicity and respond to any vaccine or therapeutic approach.
Due to the sudden increase in incidence of SARS-CoV-2 infection in children with the emergence of new variants, there is still a current lack of evidence surrounding the response of the pediatric airway to them. Consequently, there is still some reliance on pre-prints for information specifically for the more recent variants, Delta and Omicron (40, 41). These studies compared variants and their respective effects on transmissibility and/or pathogenicity in children (Supplementary Table 3). The Delta variant, for instance, has been associated with higher incidence and increased hospitalization rates in children (7, 42, 43) (Supplementary Table 3). However, in a study conducted in UK comparing clinical outcomes in children infected during either an Alpha predominance or a Delta predominance, both variants were associated with similar median illness duration and symptoms experienced where most symptoms were short-lived and resolved within five days (44). Odds ratios (ORs) for some symptoms as well as the median symptom burden were only slightly higher with Delta compared to Alpha (44). This study suggested that these variants cause similar illness and symptoms and short duration. Consistent with this, another study reported that infection with Alpha or Delta in children ≤18 years was not associated with increased disease severity compared to non-VoCs (45). Findings implied that the higher hospitalization rates seen with the emergence of Delta were not a result of increased pathogenicity of the variant but simply due to the increased prevalence of infection. However, a case series in Tunisia reported that Delta causes critical outcomes in infants (<2 years) without any underlying conditions whose mothers were unvaccinated, where over half of the patients had pediatric ARDS and 20% ended with death (46). While this is not an overarching outcome of all pediatric SARS-CoV-2 infections, it emphasizes the potential risk new variants could bring.
Other studies on the Omicron variant have reported that vaccine effectiveness against Omicron infection is severely reduced compared to Delta and previous VoCs (39, 47). Interestingly, there was a difference in the preferred entry pathway whereby Omicron predominantly infects cells via endosomal fusion activated by cathepsin L (CTSL) and B (CTSB), whereas D614, Alpha, and Delta variants rely on cell surface fusion via TMPRSS2 proteolysis of the spike (39, 41, 47). Furthermore, Omicron does not heavily rely on the spike-mediated fusion via TMPRSS2, and so does not induce fusion with adjacent cells resulting in syncytia (39, 41, 47). Syncytia formation in pneumocytes has been associated with severe COVID-19, where this feature was found in majority of post-mortem samples from patients who died of COVID-19 (48). The lack of syncytia formation with Omicron’s etiology could possibly mean lower virulence compared to previous VoCs, which is in line with the milder outcomes and lower risk for severe illness observed in children following infection with Omicron (40, 49, 50) (Supplementary Table 3). Collectively, these results do imply that Omicron appears to be more transmissible compared to previous VoCs due to the overall higher expression of CTSL and CTSB in airway epithelial cells (12, 41).
With regards to possible cross-reactivity between variants, Dowell et al. (26) reports that children previously infected by the original strain of the virus, produced SARS-CoV-2 specific antibodies that were also cross-reactive to Alpha, Beta, and Gamma. Levels of these cross-reactive antibodies in children six months post-infection were also higher than that of adults (26). However, it should also be noted, that antibodies in all individuals showed reduced capacity to neutralize infection by live virus (26). Another study on a relatively small sample size reported higher lymphocyte and white blood cell counts but lower IL-6 levels in younger children <12 years old infected with Delta compared to those ≥12 years (51). Age cohorts also differed in antibody levels with lower anti-SARS-CoV-2 IgG and IgM found on admission, and lower IgG but higher IgM in in younger children at convalescence (51). Younger children were also associated with a higher asymptomatic rate and milder illness with lower incidence of severe cases, pneumonia, and respiratory failure reported (51). With new studies being performed, clinical impacts of these new variants on the pediatric population are becoming more evident, however, additional studies are still required to elucidate the potential cellular and molecular differences these variants would incite in children.
5 Impact of comorbidities
With certain comorbidities highlighted to increase the risk of susceptibility to the effects of SARS-CoV-2 infection in adults, it is important to know if children living with underlying health conditions are also at higher risk. Overall, children (and young adults <21 years) living with comorbidities have been found to be more likely to develop severe COVID-19 requiring critical care and COVID-19-associated death than those without, and this risk increases with the number of comorbidities they have (11, 52). Further insights can be made from a recent cross-sectional study conducted by Kompaniyets et al. (53), who examined data from 43,465 patients ≤18 years of age who presented to hospital with COVID-19. Results showed that 28.7% of children had an underlying medical condition, and this increased to 62.9% in those who were hospitalized. Observed comorbidities included asthma, neurodevelopmental disorders, anxiety and depressive disorders, and obesity (53). Although asthma was the most common risk factor observed, its adjusted risk ratio (aRR) for hospitalization was not amongst the highest at 1.23. Instead, type 1 diabetes was reported to have the highest aRR (4.6), followed by obesity (3.07), and congenital cardiovascular anomalies (2.12) (53). Children with type 1 diabetes and congenital cardiovascular anomalies were also reported to have the highest risk of developing severe COVID-19 (53). Other studies have also associated asthma, neurological, cardiovascular, and immunological/hematological disorders (immunocompromised), gastrointestinal disease, respiratory comorbidities, prematurity, obesity, and diabetes in children with higher risk of requiring hospital admission (11, 28, 52). This is also observed during the period where Delta was predominant, with underlying respiratory and endocrine disorders found more common in adolescents who were hospitalized with COVID-19 compared to age-matched controls (54). Age-stratification showed that chronic lung disease, neurologic disorders, cardiovascular disease, prematurity, and airway abnormality were risk factors for severe COVID-19 in infants and toddlers under 2 years (53, 55). For older aged children, diabetes mellitus and obesity were the predominant risk factors associated with severe COVID-19 (53, 55). As of writing this review, the underlying mechanisms as to why and how these specific comorbidities increase the risk of developing severe disease in children remain unknown, however, several theories are now emerging including the potential for the accumulation of viral mutations during a period of prolonged infectivity (21, 56).
6 Conclusions
In summary, we present a current overview on pediatric COVID-19 literature, particularly the differences suggested to contribute to the lower severity seen in children infected with SARS-CoV-2. Despite progress being made in identifying age-related immune response differences to infection, little is still known as to how and why, in some children, the disease progression leads to MIS-C and varies from what is typically seen with adults. With vaccines being made available for the younger population, it is encouraging that unexposed children will have vaccine-derived immunity, however many of our youngest remain vulnerable. Consequently, the priority now should include understanding how emerging variants will affect these individuals as well as identifying complementary therapeutics that can be adapted and tailored to the relevant variant at the time. With continued scientific advances, we will be able to fully comprehend the underlying biology of all SARS-CoV-2 variants and hopefully identify therapies for the youngest of our population where none currently exist, to keep our children safe.
Author contributions
RH and AK conceived the review topic and initial structure. RH conducted literature review and drafted the manuscript. AK and AB provided extensive structural and editorial revisions and approved the final version for publication. CB, AI, TK and SS contributed to the structure and content, provided critical review, and approved the final version to be published. All authors contributed to the article and approved the submitted version.
Funding
This work is supported by a NHMRC MRFF grant (2016238). RH is supported the University of Western Australia International Fee Scholarship, University Postgraduate Award, Stan and Jean Perron Top-Up Scholarship, and Wesfarmers Centre of Vaccines and Infectious Diseases and Wal-Yan Respiratory Centre Top Up Scholarship. AK is a Rothwell Family Fellow. SS, CB and AB are all supported by NHMRC investigator awards (2021/GR000216, GNT1173163, and GNT1175509).
Acknowledgments
The authors would like to thank the participants and their families in the Western Australian Epithelial Research Program (WAERP) for their contribution to this study. Current members of WAERP include: Anthony Kicic, Stephen M. Stick, Elizabeth Kicic-Starcevich, Amy Greenly, Angela Fuery, Luke W. Garratt, Erika N. Sutanto, Kevin Looi, Thomas Iosifidis, Craig Schofield, Samantha McLean, Luke Berry, Samuel T. Montgomery, Kak-Ming Ling, Renee Ng, Andrew Vaitekenas, Daniel Laucirica, Matthew Poh, Reanne Ho, Joshua Iszatt, Katherine Landwehr, Denby Evans, Rebecca Watkinson, Patricia Agudelo-Romero, Jose Caparros-Martin, Rohan Flint, Jack Canning, Sourav Shyam, Jane Choi, Shyan Vijayasekaran, George Sim, Mairead Heaney, Tom Rawlings, Neil Chambers, Christopher Johnson, Eugene Roscioli, Alexander Larcombe, Tim Barnett, Rael, Rivers, and Kate McGee.
Conflict of interest
The authors declare that the research was conducted in the absence of any commercial or financial relationships that could be construed as a potential conflict of interest.
Publisher’s note
All claims expressed in this article are solely those of the authors and do not necessarily represent those of their affiliated organizations, or those of the publisher, the editors and the reviewers. Any product that may be evaluated in this article, or claim that may be made by its manufacturer, is not guaranteed or endorsed by the publisher.
Supplementary material
The Supplementary Material for this article can be found online at: https://www.frontiersin.org/articles/10.3389/fimmu.2023.1200456/full#supplementary-material
References
1. Weisberg SP, Connors TJ, Zhu Y, Baldwin MR, Lin WH, Wontakal S, et al. Distinct antibody responses to SARS-CoV-2 in children and adults across the COVID-19 clinical spectrum. Nat Immunol (2021) 22(1):25–31. doi: 10.1038/s41590-020-00826-9
2. Zhou F, Yu T, Du R, Fan G, Liu Y, Liu Z, et al. Clinical course and risk factors for mortality of adult inpatients with COVID-19 in wuhan, China: a retrospective cohort study. Lancet (2020) 395(10229):1054–62. doi: 10.1016/S0140-6736(20)30566-3
3. Huang C, Wang Y, Li X, Ren L, Zhao J, Hu Y, et al. Clinical features of patients infected with 2019 novel coronavirus in wuhan, China. Lancet (2020) 395(10223):497–506. doi: 10.1016/S0140-6736(20)30183-5
4. Viner RM, Mytton OT, Bonell C, Melendez-Torres GJ, Ward J, Hudson L, et al. Susceptibility to SARS-CoV-2 infection among children and adolescents compared with adults: a systematic review and meta-analysis. JAMA Pediatr (2021) 175(2):143–56. doi: 10.1001/jamapediatrics.2020.4573
5. Gaythorpe KAM, Bhatia S, Mangal T, Unwin HJT, Imai N, Cuomo-Dannenburg G, et al. Children’s role in the COVID-19 pandemic: a systematic review of early surveillance data on susceptibility, severity, and transmissibility. Sci Rep (2021) 11(1):1–14. doi: 10.1038/s41598-021-92500-9
6. Wu Z, McGoogan JM. Characteristics of and important lessons from the coronavirus disease 2019 (COVID-19) outbreak in China: summary of a report of 72314 cases from the Chinese center for disease control and prevention. JAMA - J Am Med Assoc (2020) 323(13):1239–42. doi: 10.1001/jama.2020.2648
7. Delahoy MJ, Ujamaa D, Whitaker M, O’Halloran A, Anglin O, Burns E, et al. Hospitalizations associated with COVID-19 among children and adolescents — COVID-NET, 14 states, march 1, 2020–august 14, 2021. Morb Mortal Wkly Rep (2021) 70(36):1255. doi: 10.15585/mmwr.mm7036e2
8. Zhang C, Gu J, Chen Q, Deng N, Li J, Huang L, et al. Clinical and epidemiological characteristics of pediatric SARS-CoV-2 infections in China: a multicenter case series. PloS Med (2020) 17(6):e1003130. doi: 10.1371/journal.pmed.1003130
9. Yonker LM, Neilan AM, Bartsch Y, Patel AB, Regan J, Arya P, et al. Pediatric severe acute respiratory syndrome coronavirus 2 (SARS-CoV-2): clinical presentation, infectivity, and immune responses. J Pediatr (2020) 227:45–52.e5. doi: 10.1016/j.jpeds.2020.08.037
10. Consiglio CR, Cotugno N, Sardh F, Pou C, Amodio D, Rodriguez L, et al. The immunology of multisystem inflammatory syndrome in children with COVID-19. Cell (2020) 183(4):968–981.e7. doi: 10.1016/j.cell.2020.09.016
11. Swann OV, Holden KA, Turtle L, Pollock L, Fairfield CJ, Drake TM, et al. Clinical characteristics of children and young people admitted to hospital with covid-19 in united kingdom: prospective multicentre observational cohort study. BMJ (2020) 370:5. doi: 10.1136/bmj.m3249
12. Sungnak W, Huang N, Bécavin C, Berg M, Queen R, Litvinukova M, et al. SARS-CoV-2 entry factors are highly expressed in nasal epithelial cells together with innate immune genes. Nat Med (2020) 26(5):681–7. doi: 10.1038/s41591-020-0868-6
13. Wark PAB, Pathinayake PS, Kaiko G, Nichol K, Ali A, Chen L, et al. ACE2 expression is elevated in airway epithelial cells from older and male healthy individuals but reduced in asthma. Respirology (2021) 26(5):442–51. doi: 10.1101/2020.07.26.20162248
14. Aguiar JA, Tremblay BJM, Mansfield MJ, Woody O, Lobb B, Banerjee A, et al. Gene expression and in situ protein profiling of candidate SARS-CoV-2 receptors in human airway epithelial cells and lung tissue. Eur Respir J (2020) 56(3):2001123. doi: 10.1183/13993003.01123-2020
15. Loske J, Röhmel J, Lukassen S, Stricker S, Magalhães VG, Liebig J, et al. Pre-activated antiviral innate immunity in the upper airways controls early SARS-CoV-2 infection in children. Nat Biotechnol (2022) 40(3):319–24. doi: 10.1038/s41587-021-01037-9
16. Koch CM, Prigge AD, Anekalla KR, Shukla A, Do Umehara HC, Setar L, et al. Age-related differences in the nasal mucosal immune response to SARS-CoV-2. Am J Respir Cell Mol Biol (2022) 66(2):206–22. doi: 10.1165/rcmb.2021-0292OC
17. Plaas M, Seppa K, Gaur N, Kasenõmm P, Plaas M. Age- and airway disease related gene expression patterns of key SARS-CoV-2 entry factors in human nasal epithelia. Virol (2021) 561:65. doi: 10.1016/J.VIROL.2021.05.012
18. Muus C, Luecken MD, Eraslan G, Sikkema L, Waghray A, Heimberg G, et al. Single-cell meta-analysis of SARS-CoV-2 entry genes across tissues and demographics. Nat Med (2021) 27(3):546–59. doi: 10.1038/s41591-020-01227-z
19. Bunyavanich S, Do A, Vicencio A. Nasal gene expression of angiotensin-converting enzyme 2 in children and adults. JAMA - J Am Med Assoc (2020) 323(23):2427–9. doi: 10.1001/jama.2020.8707
20. Zhu Y, Chew KY, Wu M, Karawita AC, McCallum G, Steele LE, et al. Ancestral SARS-CoV-2, but not omicron, replicates less efficiently in primary pediatric nasal epithelial cells. Sugden B, editor. PloS Biol (2022) 20(8):e3001728. doi: 10.1371/journal.pbio.3001728
21. Kociolek LK, Muller WJ, Yee R, Bard JD, Brown CA, Revell PA, et al. Comparison of upper respiratory viral load distributions in asymptomatic and symptomatic children diagnosed with SARS-CoV-2 infection in pediatric hospital testing programs. Hayden R, editor. J Clin Microbiol (2020) 59(1):e02593–20. doi: 10.1128/JCM.02593-20
22. Gruber CN, Patel RS, Trachtman R, Lepow L, Amanat F, Krammer F, et al. Mapping systemic inflammation and antibody responses in multisystem inflammatory syndrome in children (MIS-c). Cell (2020) 183(4):982–995.e14. doi: 10.1016/j.cell.2020.09.034
23. Neeland MR, Bannister S, Clifford V, Dohle K, Mulholland K, Sutton P, et al. Innate cell profiles during the acute and convalescent phase of SARS-CoV-2 infection in children. Nat Commun (2021) 12(1):1084. doi: 10.1038/s41467-021-21414-x
24. Carter MJ, Fish M, Jennings A, Doores KJ, Wellman P, Seow J, et al. Peripheral immunophenotypes in children with multisystem inflammatory syndrome associated with SARS-CoV-2 infection. Nat Med (2020) 26(11):1701–7. doi: 10.1038/s41591-020-1054-6
25. Pierce CA, Preston-Hurlburt P, Dai Y, Aschner CB, Cheshenko N, Galen B, et al. Immune responses to SARS-CoV-2 infection in hospitalized pediatric and adult patients. Sci Transl Med (2020) 12(564):eabd5487. doi: 10.1126/scitranslmed.abd5487
26. Dowell AC, Butler MS, Jinks E, Tut G, Lancaster T, Sylla P, et al. Children develop robust and sustained cross-reactive spike-specific immune responses to SARS-CoV-2 infection. Nat Immunol (2021) 23(1):40–9. doi: 10.1038/s41590-021-01089-8
27. Bartsch YC, Wang C, Zohar T, Fischinger S, Atyeo C, Burke JS, et al. Humoral signatures of protective and pathological SARS-CoV-2 infection in children. Nat Med (2021) 27(3):454–62. doi: 10.1038/s41591-021-01263-3
28. Fernandes DM, Oliveira CR, Guerguis S, Eisenberg R, Choi J, Kim M, et al. Severe acute respiratory syndrome coronavirus 2 clinical syndromes and predictors of disease severity in hospitalized children and youth. J Pediatr (2021) 230:23–31.e10. doi: 10.1016/j.jpeds.2020.11.016
29. Diorio C, Henrickson SE, Vella LA, McNerney KO, Chase J, Burudpakdee C, et al. Multisystem inflammatory syndrome in children and COVID-19 are distinct presentations of SARS–CoV-2. J Clin Invest (2020) 130(11):5967. doi: 10.1172/JCI140970
30. Anderson EM, Diorio C, Goodwin EC, Mcnerney KO, Weirick ME, Gouma S, et al. Severe acute respiratory syndrome-Coronavirus-2 (SARS-CoV-2) antibody responses in children with multisystem inflammatory syndrome in children (MIS-c) and mild and severe coronavirus disease 2019 (COVID-19). J Pediatr Infect Dis Soc (2021) 10(5):669–73. doi: 10.1093/JPIDS/PIAA161
31. Rostad CA, Chahroudi A, Mantus G, Lapp SA, Teherani M, Macoy L, et al. Quantitative SARS-CoV-2 serology in children with multisystem inflammatory syndrome (MIS-c). Pediatrics (2020) 146(6). doi: 10.1542/peds.2020-018242
32. Hadfield J, Megill C, Bell SM, Huddleston J, Potter B, Callender C, et al Nextstrain: real-time tracking of pathogen evolution. Kelso J. (eds). Bioninformatics (2018) 34(23):4121–3. doi: 10.1093/bioinformatics/bty407
33. Salleh MZ, Derrick JP, Deris ZZ. Structural evaluation of the spike glycoprotein variants on SARS-CoV-2 transmission and immune evasion. Int J Mol Sci (2021) 22(14):7425. doi: 10.3390/ijms22147425
34. Ito K, Piantham C, Nishiura H. Relative instantaneous reproduction number of omicron SARS-CoV-2 variant with respect to the delta variant in Denmark. J Med Virol (2022) 94(5):2265–8. doi: 10.1002/jmv.27560
35. Tran BM, Grimley SL, McAuley JL, Hachani A, Earnest L, Wong SL, et al. Air-Liquid-Interface differentiated human nose epithelium: a robust primary tissue culture model of SARS-CoV-2 infection. Int J Mol Sci (2022) 23(2):835. doi: 10.3390/IJMS23020835/S1
36. Saito A, Irie T, Suzuki R, Maemura T, Nasser H, Uriu K, et al. Enhanced fusogenicity and pathogenicity of SARS-CoV-2 delta P681R mutation. Nat (2021) 22(11):1–7. doi: 10.1038/s41586-021-04266-9
37. Wang P, Nair MS, Liu L, Iketani S, Luo Y, Guo Y, et al. Antibody resistance of SARS-CoV-2 variants B.1.351 and B.1.1.7. Nature (2021) 593(7857):130–5. doi: 10.1038/s41586-021-03398-2
38. Wang P, Casner RG, Nair MS, Wang M, Yu J, Cerutti G, et al. Increased resistance of SARS-CoV-2 variant P.1 to antibody neutralization. Cell Host Microbe (2021) 29(5):747-751.e4. doi: 10.1016/j.chom.2021.04.007
39. Meng B, Abdullahi A, Ferreira IATM, Goonawardane N, Saito A, Kimura I, et al. Altered TMPRSS2 usage by SARS-CoV-2 omicron impacts infectivity and fusogenicity. Nature (2022) 603(7902):706–14. doi: 10.1038/s41586-022-04474-x
40. Wang L, Berger NA, Kaelber DC, Davis PB, Volkow ND, Xu R. Comparison of outcomes from COVID infection in pediatric and adult patients before and after the emergence of omicron. medRxiv (2022), 2021.12.31.474653. doi: 10.1101/2021.12.31.474653
41. Peacock TP, Brown JC, Zhou J, Thakur N, Sukhova K, Newman J, et al. The altered entry pathway and antigenic distance of the SARS-CoV-2 omicron variant map to separate domains of spike protein. bioRxiv (2022) , 2021.12.31.474653. doi: 10.1101/2021.12.31.474653
42. Siegel DA, Reses HE, Cool AJ, Shapiro CN, Hsu J, Boehmer TK, et al. Trends in COVID-19 cases, emergency department visits, and hospital admissions among children and adolescents aged 0–17 years — united states, august 2020–august 2021. MMWR Morb Mortal Wkly Rep (2021) 70(36):1249–54. doi: 10.15585/mmwr.mm7036e1
43. Elliott P, Haw D, Wang H, Eales O, Walters CE, Ainslie KEC, et al. Exponential growth, high prevalence of SARS-CoV-2, and vaccine effectiveness associated with the delta variant. Science (2021) 374(6574). doi: 10.1126/science.abl9551
44. Molteni E, Sudre CH, Canas LDS, Bhopal SS, Hughes RC, Chen L, et al. Illness characteristics of COVID-19 in children infected with the SARS-CoV-2 delta variant. Children (2022) 9(5):652. doi: 10.3390/children9050652
45. Edward PR, Lorenzo-Redondo R, Reyna ME, Simons LM, Hultquist JF, Patel AB, et al. Severity of illness caused by severe acute respiratory syndrome coronavirus 2 variants of concern in children: a single-center retrospective cohort study. J Pediatr Infect Dis Soc (2022) 11(10):440–7. doi: 10.1093/jpids/piac068
46. Borgi A, Louati A, Miraoui A, Lahmar L, Mefteh K, Hajji A, et al. Severe and critical SARS-COV-2 delta variant infection in infants without underlying medical conditions. Respirol Case Rep (2022) 10(9):e01012. doi: 10.1002/rcr2.1012
47. Willett BJ, Grove J, MacLean OA, Wilkie C, De Lorenzo G, Furnon W, et al. SARS-CoV-2 omicron is an immune escape variant with an altered cell entry pathway. Nat Microbiol (2022) 7(8):1161–79. doi: 10.1038/s41564-022-01143-7
48. Bussani R, Schneider E, Zentilin L, Collesi C, Ali H, Braga L, et al. Persistence of viral RNA, pneumocyte syncytia and thrombosis are hallmarks of advanced COVID-19 pathology. EBioMedicine (2020) 61:103104. doi: 10.1016/j.ebiom.2020.103104.
49. Bahl A, Mielke N, Johnson S, Desai A, Qu L. Severe COVID-19 outcomes in pediatrics: an observational cohort analysis comparing alpha, delta, and omicron variants. Lancet Reg Heal - Am (2023) 18:100405. doi: 10.1016/j.lana.2022.100405
50. Wang L, Berger NA, Kaelber DC, Davis PB, Volkow ND, Xu R. Incidence rates and clinical outcomes of SARS-CoV-2 infection with the omicron and delta variants in children younger than 5 years in the US. JAMA Pediatr (2022) 176(8):811–3. doi: 10.1001/jamapediatrics.2022.0945
51. Li H, Lin H, Chen X, Li H, Li H, Lin S, et al. Unvaccinated children are an important link in the transmission of SARS-CoV-2 delta variant (B1.617.2): comparative clinical evidence from a recent community surge. Front Cell Infect Microbiol (2022) 12:1. doi: 10.3389/fcimb.2022.814782
52. Graff K, Smith C, Silveira L, Jung S, Curran-Hays S, Jarjour J, et al. Risk factors for severe COVID-19 in children. Pediatr Infect Dis J (2021) 40(4):e137–45. doi: 10.1097/INF.0000000000003043
53. Kompaniyets L, Agathis NT, Nelson JM, Preston LE, Ko JY, Belay B, et al. Underlying medical conditions associated with severe COVID-19 illness among children. JAMA Netw Open (2021) 4(6):e2111182–e2111182. doi: 10.1001/jamanetworkopen.2021.11182
54. Olson SM, Newhams MM, Halasa NB, Price AM, Boom JA, Sahni LC, et al. Effectiveness of BNT162b2 vaccine against critical covid-19 in adolescents. N Engl J Med (2022) 386(8):713–23. doi: 10.1056/NEJMoa2117995
55. Woodruff RC, Campbell AP, Taylor CA, Chai SJ, Kawasaki B, Meek J, et al. Risk factors for severe COVID-19 in children. Pediatrics (2022) 149(1):2021053418. doi: 10.1542/peds.2021-053418
Keywords: COVID-19, SARS-CoV-2, pediatric, children, emerging variants, molecular biology, cellular biology, age-specific response
Citation: Ho RM, Bowen AC, Blyth CC, Imrie A, Kollmann TR, Stick SM and Kicic A (2023) Defining the pediatric response to SARS-CoV-2 variants. Front. Immunol. 14:1200456. doi: 10.3389/fimmu.2023.1200456
Received: 05 April 2023; Accepted: 05 May 2023;
Published: 25 May 2023.
Edited by:
Adi Idris, Queensland University of Technology, AustraliaReviewed by:
Zi Wei Chang, A*STAR Infectious Disease Labs, SingaporeCopyright © 2023 Ho, Bowen, Blyth, Imrie, Kollmann, Stick and Kicic. This is an open-access article distributed under the terms of the Creative Commons Attribution License (CC BY). The use, distribution or reproduction in other forums is permitted, provided the original author(s) and the copyright owner(s) are credited and that the original publication in this journal is cited, in accordance with accepted academic practice. No use, distribution or reproduction is permitted which does not comply with these terms.
*Correspondence: Anthony Kicic, Anthony.Kicic@telethonkids.org.au