- 1Department of Trauma Surgery, Emergency Surgery & Surgical Critical, Tongji Trauma Center, Wuhan, China
- 2Department of Emergency and Critical Care Medicine, Tongji Hospital, Tongji Medical College, Huazhong University of Science and Technology, Wuhan, China
Nowadays, people have relaxed their vigilance against COVID-19 due to its declining infection numbers and attenuated virulence. However, COVID-19 still needs to be concern due to its emerging variants, the relaxation of restrictions as well as breakthrough infections. During the period of the COVID-19 infection, the imbalanced and hyper-responsive immune system plays a critical role in its pathogenesis. Macrophage Activation Syndrome (MAS) is a fatal complication of immune system disease, which is caused by the excessive activation and proliferation of macrophages and cytotoxic T cells (CTL). COVID-19-related hyperinflammation shares common clinical features with the above MAS symptoms, such as hypercytokinemia, hyperferritinemia, and coagulopathy. In MAS, immune exhaustion or defective anti-viral responses leads to the inadequate cytolytic capacity of CTL which contributes to prolonged interaction between CTL, APCs and macrophages. It is possible that the same process also occurred in COVID-19 patients, and further led to a cytokine storm confined to the lungs. It is associated with the poor prognosis of severe patients such as multiple organ failure and even death. The main difference of cytokine storm is that in COVID-19 pneumonia is mainly the specific damage of the lung, while in MAS is easy to develop into a systemic. The attractive therapeutic approach to prevent MAS in COVID-19 mainly includes antiviral, antibiotics, convalescent plasma (CP) therapy and hemadsorption, extensive immunosuppressive agents, and cytokine-targeted therapies. Here, we discuss the role of the therapeutic approaches mentioned above in the two diseases. And we found that the treatment effect of the same therapeutic approach is different.
1 Introduction
Since December 2019, a new β-coronavirus named severe acute respiratory syndrome coronavirus 2 (SARS-CoV-2) has led to an outbreak around the world, which has posed a global challenge (1–3). According to the World Health Organization (WHO) Dashboard (https://covid19.who.int/), up to 24 May 2023, there have been 766,895,075 confirmed cases of COVID-19, including 6,935,889 deaths. Even now that the number of COVID-19 vaccinations has reached 13355264024, there are still two currently circulating variants of interest (VOI), XBB.1.5 and XBB.1.16,and seven currently circulating variants under monitoring (VUMs) (Tracking SARS-CoV-2 variants (who.int)). These variants have been associated with an increase in the transmission or mortality of COVID-19 (4–9), or may escape immunity when compared to the original strain or D614G variant (9–14). Despite the current trend of COVID-19 being in decline, the harm of COVID-19 cannot be underestimated due to the great possibility of its resurgence.
The imbalanced and hyper-responsive immune system plays a critical role in COVID-19 pathogenesis. The immune response of SARS-CoV-2 infection is characterized by the differentiation and proliferation of various types of immune cells and the release of immune mediators (15, 16). Early evidence suggests that the clinical and laboratory features of the patients severely infected with COVID-19 are similar to the clinical phenotypes of “Cytokine Storm Syndromes” (CSS) (17–19).In general, the patterns and levels of inflammation and immune dysregulation observed in the periphery and lungs of COVID-19 patients are characteristic of “Cytokine Storm Syndromes”. Acute respiratory distress syndrome (ARDS) and Multiple organ dysfunction syndrome (MODS) are the main causes of mortality in patients with COVID-19, while CSS will lead to ARDS and MODS (20–25). Therefore, CSS plays a very critical role in patients with severe COVID-19.
CSS refers to a diverse set of conditions that collectively manifest a clinical phenotype of hyperinflammation, hyperferritinemia, and multiorgan failure. The spectrum of cytokine storm syndromes spans wide-ranging conditions, one of which is called Macrophage Activation Syndrome (MAS) (26). MAS is a prototypic form of CSS that develops in many rheumatic diseases, such as the Still’s disease spectrum (systemic juvenile idiopathic arthritis [sJIA] and adult-onset Still’s disease) (27), systemic lupus erythematosus (28) and Kawasaki disease (29). Considerable studies have been performed to elucidate that MAS is involved in COVID-19 associated with worse disease severity and poorer prognosis (30). Overzealous immune responses associated with MAS, may be driving COVID-19 related ARDS (21). There has been research shows that treatment of MAS, such as the use of tocilizumab and the combination of tocilizumab and steroids, significantly reduced the intubation or death of severe patients (31, 32).
While the new case of COVID-19 infections is decreasing, it is premature for ceasing the research on its treatment when there is so much uncertainty about how SARS-CoV-2 will behave, the path of its mutations, as well as its many possible long-term effects. Standard treatment for MAS includes several immunosuppressive drugs (33). Furthermore, many cytokines targeted therapeutic agents have achieved good results in the treatment of MAS, such as Anakinra, Tocilizumab, etc. al (30, 34–36). However, further research into SARS-CoV-2 pathogenesis has found some differences between MAS and COVID-19, which makes the therapeutic effect uncertain. More recently, randomized trials of anti-IL-1 and IL-6 agents have found little evidence of overall benefit (37–39). It is obvious that the effect of therapeutic interventions for MAS in COVID-19 is controversial. To address this issue, in this review, we systematically summarize the advances in attractive therapeutic approaches to treat MAS for COVID-19.
2 The MAS of COVID-19
MAS is a fatal complication of immune system disease, which is caused by the excessive activation and proliferation of macrophages and cytotoxic T cells (CTL). MAS can lead to a cytokine storm and a persistent inflammatory state (40, 41). This disease is caused by the combination of the following three conditions (1): the inability of the cytolytic function of NK cells and CTL cells (2); the high sensitivity of macrophages caused by the high response of macrophage pattern recognition receptors (PRR) to pathogen-associated molecular patterns (PAMP) and the underlying inflammatory symptoms of the body;(3) trigger factors (42). Under the above conditions, the end product is escalating production of cytokines. The main diagnostic criteria that contribute to the development of a diagnostic score for MAS include: Fever, hepatosplenomegaly, hyperferritinemia, hepatopathy, coagulopathy, thrombocytopenia, hypertriglyceridemia, decrease in erythrocyte sedimentation rate and bone marrow hemophagocytosis (30, 43).
COVID-19 related hyperinflammation shares common clinical features with the above MAS symptoms, such as hypercytokinemia, hyperferritinemia, coagulopathy (2, 18, 19, 21, 44) (17). Some scholars proposed some classification framework for identifying COVID-19 cytokine storms according to diagnostic and classification criteria of MAS, such as cHIS (COVID-19 associated hyperinflammatory syndrome) and COVID hyperinflammation (COV-HI) (45, 46).
The entry of SARS-CoV-2 into cells depends on the binding of S protein on the surface of virus particles to the ACE2 receptor of cells, and the activation of S protein by the host membrane serine protease TMPRSS2 (47, 48). SARS CoV-2 virus was found in alveolar macrophages in autopsy of SARS CoV-2 pneumonia patients, but the macrophages do not express ACE2 and TMPRSS2 (49). Therefore, the entrance of virus into macrophages should be achieved by other means (35, 50).This leads to abnormal activation of macrophages to release cytokines including IL-6, which is one of the driving factors of MAS (51–53). In MAS, immune exhaustion or defective anti-viral responses leads to inadequate cytolytic capacity of CTL which contributes to prolonged interaction between CTL, APCs and macrophages (54). It is possible that the same process also occurred in COVID-19 patients, and further led to a cytokine storm confined to the lungs (55)(Figure 1).In deed, in addition to macrophages, there are many other cells, which will release a large number of cytokines and chemokines, thus leading to the inflammatory cascade reaction to further expand the cytokine storm (56).And then, the local inflammation induced by SARS-CoV-2 infection spread rapidly to the entire lung (57). Accumulation of immune cells accelerates the progression of lung inflammation into ARDS, which is associated with the poor prognosis of severe patients such as multiple organ failure and even death (24, 35).
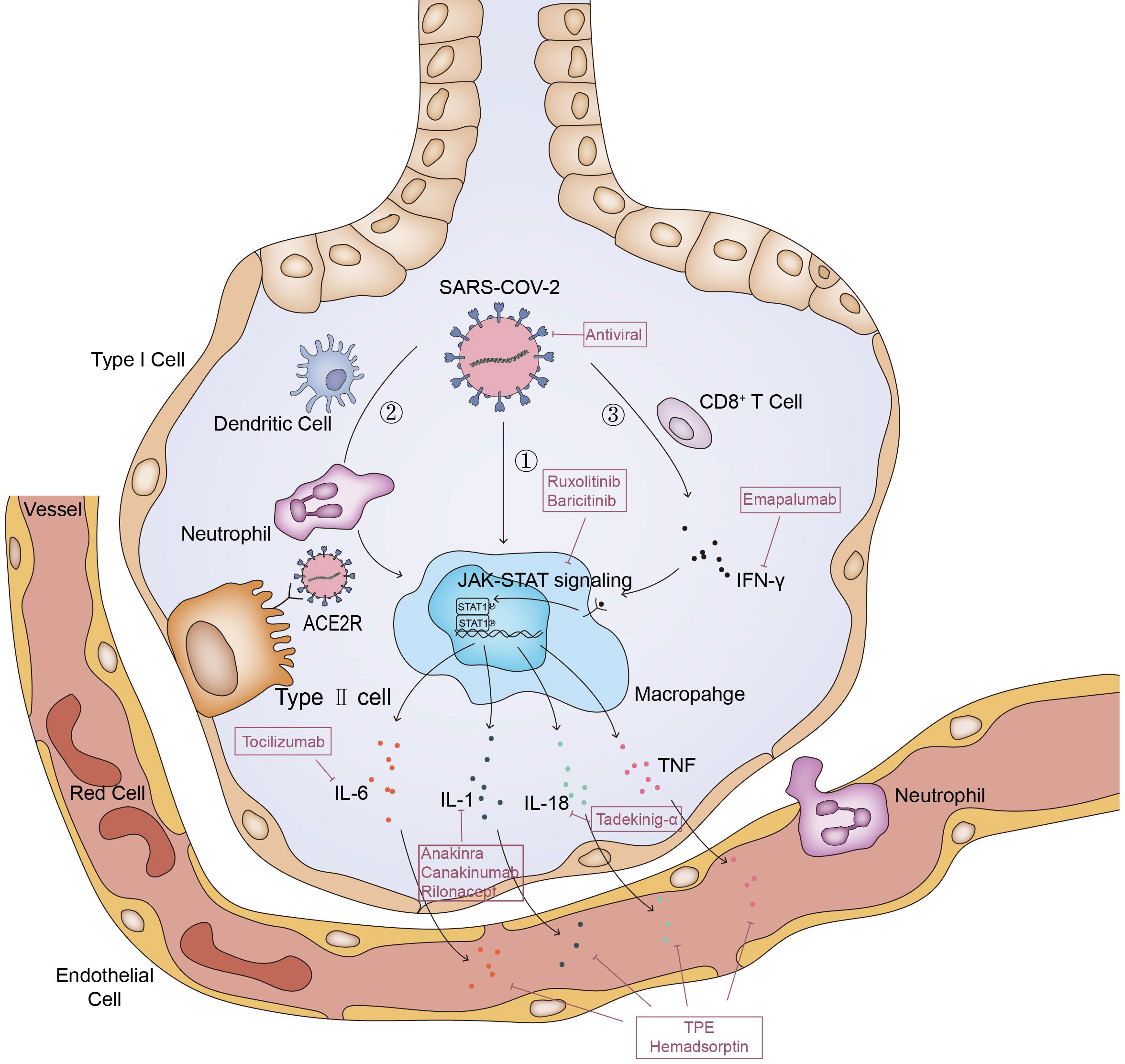
Figure 1 Pathways of MAS in COVID-19. The entry of SARS-CoV-2 into cells depends on the binding of S protein on the surface of virus particles to the ACE2 receptor of cells; ①. Macrophage ingests viral RNA through phagocytosis, phagocytosis of alveolar epithelial cells infected by SARS-CoV-2 viruses or phagocytosis of viruses through Fc receptor mediated endocytosis. This leads to abnormal activation of macrophages to release cytokines; ②. Dendritic cells and neutrophils will also be recruited here. On the one hand, neutrophils and dendritic cells will produce a variety of cytokines under the stimulation of SARS-CoV-2, on the other hand, they will also transmit activation signals to macrophages, making them release cytokines and chemokines; ③. SARS-CoV-2 stimulates anti-viral immune pathways with CD8+ T cell expansion. Expanded CD8+ T cells produce interferon-γ (IFN-γ), IFN-γ binds the IFN-γ receptor and further stimulate macrophage activation to produce multiple anti-inflammatory cytokines through the JAK-STAT pathway. And some of these cytokines signal via the JAK-STAT pathway, including IL-6 and IFN (but not IL-1 and TNF), to regulate cell homeostasis, proliferation and differentiation as well as control the immune system and inflammatory response. Inadequate cytolytic capacity due to immune exhaustion and/or defective antiviral responses leads to prolonged cross-talk between CD8+ T cell, Antigen-presenting cells (APCs) and alveolar macrophages, contributing to the further release of many proinflammatory cytokines.
Although the process of macrophage over activation is very similar to that of MAS, there are still many differences between them, such as clinical manifestations, laboratory tests and specific forms of cytokine storm (2, 58, 59). In severe COVID-19, many studies have found the expansion of alveolar macrophages and the obvious increase of pulmonary inflammatory factors (IL-6, IL-8, IL-1β) (60–62). And David A. Dorward et al. have observed that although SARS-CoV-2 RNA exists in many organs, such as the gastrointestinal tract, liver and kidney, these extrapulmonary sites with evidence of viral transcription, did not have substantial local inflammation (63).The evidence above supports a model of a lung-centric, self-sustaining inflammatory loop leading to cytokine storm (55). However, in MAS, cell mediated cytotoxicity fails due to various reasons such as genetic or viral infection, which leads to the prolonged cell-to-cell interactions, the cascade expansion of proinflammatory cytokines, and then the generation of systemic cytokine storms (Figure 2). Cytokine storm will further lead to systemic macrophage activation, hemophagocytosis and multiple organ dysfunction (54). To sum up, it is easy to see that in terms of the specific form of cytokines storm, the main differences between them is the cytokines storm in COVID-19 pneumonia is mainly the specific damage of the lung, while in MAS is easy to develop into systemic (58, 59).
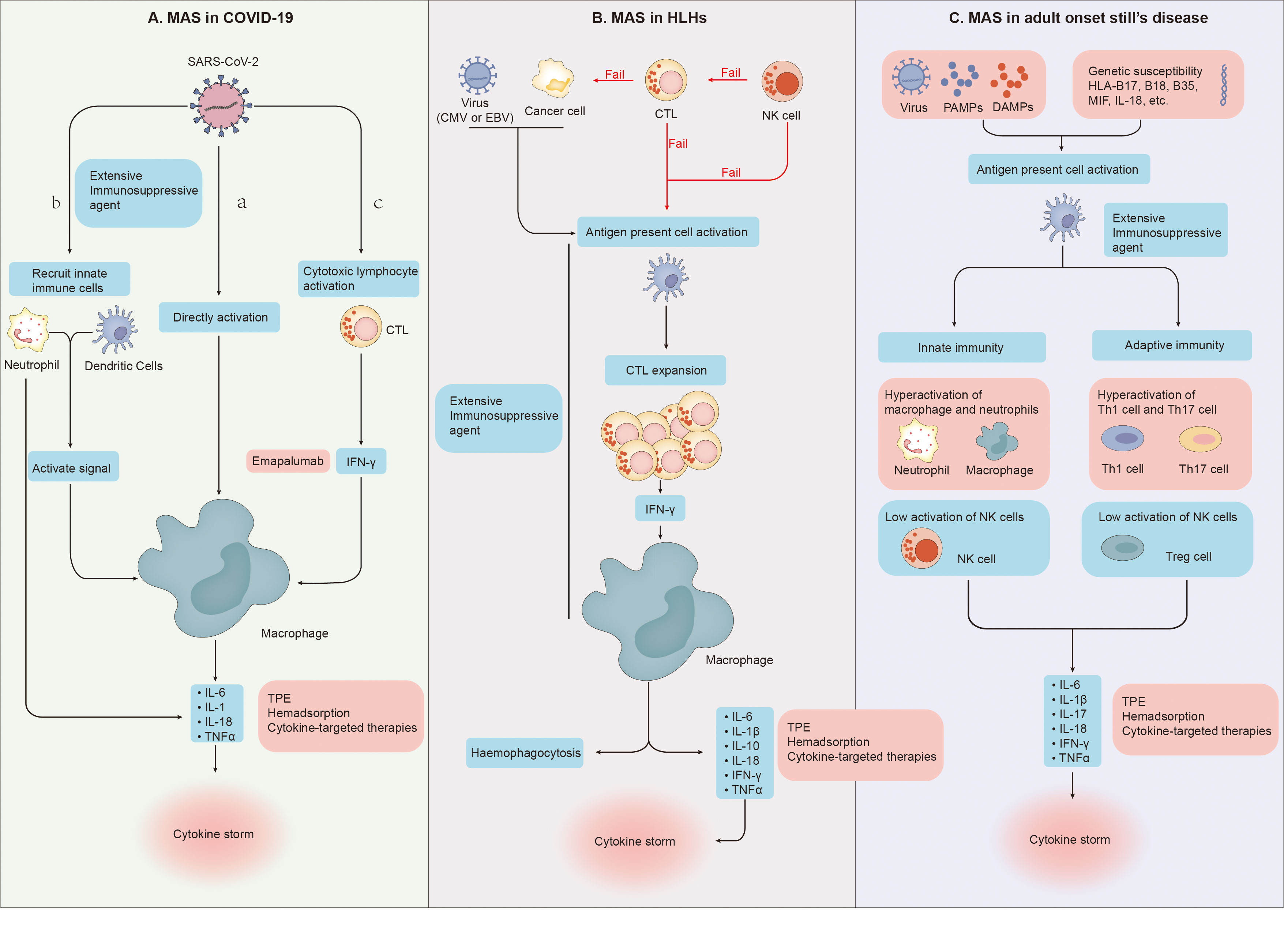
Figure 2 (A) (a) SARS-CoV-2 directly leads to abnormal activation of macrophages to release cytokines. (b) Dendritic cells and neutrophils will also be recruited. On the one hand, neutrophils and dendritic cells will produce a variety of cytokines under the stimulation of SARS-CoV-2, on the other hand, they will also transmit activation signals to macrophages, making them release cytokines and chemokines. (c) SARS-CoV-2 stimulates anti-viral immune pathways with CTL expansion. Expanded CTL produce interferon-γ(IFN-γ), IFN-γ binds the IFN-γ receptor and further stimulate macrophage activation to produce multiple anti-inflammatory cytokines. (B) Cytotoxic function of NK cells fails to clear tumor or infected cells and cytotoxic T cells. Cytotoxic function of CTLs fail to clear tumor cells and APCs. And then Proliferation of the population of activated CTLs induce activation and proliferation of tissue macrophages (histiocytes). Further haemophagocytosis and cytokine storms occur. (C) Under the dual effects of non-infectious factors, bacterial or viral attacks, and the patient’s own genetic susceptibility, both the adaptive and innate immune systems are activated. In the innate immune system, the activity of macrophages and neutrophils is abnormally elevated, and the activity of NK cells is impaired. In the adaptive immune system, Th1 and Th17 activities increase while Treg activity decreases. Under the combined action of these immune cells, the body produces cytokine storms.
Precisely because of the similarity between over-activated alveolar macrophages in COVID-19 and MAS, the therapeutic approach of MAS might be able to analogize to COVID-19 patients, but the effect is still not so clear (55). Although some scholars believe that the concept of MAS in COVID-19 is relatively abstract (64), and many of the supporting evidence for drugs and treatments we have found are only case reports, with relatively limited reference value. However, the treatment of MAS like inflammation in COVID-19 could be improved by summarizing some treatment targets and interventions of MAS and we may be able to propose some potential treatment methods, so as to improve the prognosis of COVID-19.
3 The attractive therapeutic approach to prevent MAS in COVID-19
3.1 Early identification and active interventions
Early active intervention is necessary to improve the prognosis of MAS in COVID-19 patients, so early identification of MAS in COVID-19 patients is very important. However, in the research of Caricchio and colleagues, we found that some patients suspected of COVID-19 cytokine storm rarely met the diagnostic criteria of HLH/MAS, whether HLH-2004 or 2016 MAS Criteria (55, 65). Even HScore, a score recommended by Puja Mehta and colleagues to detect the inflammatory states of COVID-19 patients (21), was considered to lack sensitivity in managing hyperinflammatory states in patients by David L Leverenz in May 2020 and therefore not recommended for use (66).Therefore, some authors have developed new methods to identified the MAS in COVID-19. Webb and colleagues developed a classification framework called cHIS (COVID-19 associated hyperinflammatory syndrome) with 6 core features of CSS including: fever, macrophage activation, liver inflammation, hematologic dysfunction, coagulopathy, and hypercytokinemia (45). Besides, Manson et al. classified patients as having COVID hyperinflammation (COV-HI) with ferritin>1500 mg/L, or CRP >15 mg/dL or doubling in 24h (46). These scores and classifications have a very significant relationship with the prognosis of patients, and can identify the time for intervention earlier. It has also been applied in subsequent studies and clinical practice (67–69).
3.1.1 Antiviral
Antiviral drugs have been widely concerned since the beginning of the epidemic. Remdesivir, a nucleotide analog, is the first direct antiviral drug. According to the data, early use of this drugs after diagnosis can reduce the symptoms and hospitalization rate of patients (70). However, the drug is expensive and must be injected intravenously (71–73). Recently, oral antiviral drugs for COVID-19 have achieved great progress. The United States (US) Food and Drug Administration (FDA) issued an EUA for the use of the molnupiravir and nirmatrelvir-ritonavir (Paxlovid) (74). In a recent meta-analysis, the existing data showed that these new oral antiviral drugs can effectively reduce the mortality and hospitalization rate of patients with COVID-19, and will not increase the incidence of adverse events (75). As mentioned earlier, MAS often occurs in patients with systemic inflammatory reaction in the late stage of infection, so the early application of these antiviral drugs may also have a very expectant effect on the prevention of MAS.
3.1.2 Convalescent plasma (CP) therapy
Antibodies in convalescent plasma can kill or prevent further replication of the virus (76), and then further inhibit the inflammatory reaction and reduce the risk of excessive immune response (77). It has been proved that early administration of CP (within 9 days after the onset of symptoms) can significantly reduce the risk of hospitalization due to disease progression (78, 79). In a systematic review and meta-analysis of CP, there was a clear association between the use of CP and the mortality benefit of hospitalized patients with COVID-19 (80). In addition, inflammatory markers such as IL-6 and CRP are also less expressed in COVID-19 patients receiving CP blood transfusion (81). And IL-6 plays a key role in the pathogenesis of MAS (82). Therefore, the early use of CP may have active effect on the prevention of MAS in COVID-19.
However, the application value of CP in patients who require invasive mechanical ventilation is limited (83). Moreover, there are currently some issues with the collection of CCPs. Because most blood services do not actively collect CP, people can only use old stocks of CCPs. The effect of the old CP on the current epidemic strains may be limited, due to differences between variants of COVID-19 (84). It is impractical to conduct new experiments every time a new strain of virus appears, and collecting CCP again, so the application prospects of this treatment method are still relatively limited.
3.2 Therapeutic plasma exchange (TPE) and hemadsorption
Although the treatment of the virus itself is certainly desired, hypercytokinemia will occur in COVID-19 associated hyperinflammation, which will lead to sever cytokine storm. Therefore, it is also a necessary treatment to eliminate inflammatory cytokines through Therapeutic Plasma exchange (TPE) and Hemadsorption.
With the background therapy of glucocorticoids and immunosuppressive agents, Plasma exchange is an effective approach to rapidly clear inflammatory cytokines and reduce mortality of autoimmune inflammatory rheumatic diseases (AIIRD)-associated MAS (85). A prospective randomized controlled trial of Yuan YH et al. showed that incorporation of plasma exchange could reduce the levels of CRP, procalcitonin, alanine transaminase and total bilirubin, reduce the stay time in ICU and increase the response rate to other treatments (86). Satoshi Sato et al. also reported a case recently, after using standard treatment and immunosuppressions, the patient still did not achieve clinical remission. However, after initiation of PE, the patient’s symptoms improved significantly (87). The evidences above provide a practical basis for applying this method to COVID-19.
As early as 2020, Philip Keith et al. have proposed the possibility of applying TPE to fulminant COVID-19 (88). In fact, a lot of evidence has emerged to prove that TPE can significantly reduce the mortality rates of COVID-19 since then (89–97). In a recent meta-analysis conducted by JinlvQin et al., it was proposed that TPE significantly reduced the mortality of hospitalized patients with moderate risk COVID-19 (98).What is more remarkable is that TPE can significantly reduce the levels of IL-6, ferritin and CRP in COVID-19 in these studies. As mentioned earlier, these laboratory indicators are markers of MAS. Therefore, we have reason to believe that TPE has therapeutic and even preventive effects on MAS, and has obvious benefits for the prognosis of patients.
Hemadsorption can also significantly reduce the high level of cytokines in the patient’s circulation (99). But unlike PE, hemadsorption does not require plasma separation and allows for simultaneous fluid removal (100)(Figure 3). Recently, many reports have found that this treatment can rapidly reduce the level of IL-6 and IL-10, significantly improve hemodynamics, and without relevant associated adverse effects (101, 102). Therefore, hemadsorption can be used as an effective and safe rescue treatment for patients with MAS and multiple organ dysfunctions, and complementary to standard protocol treatments (103, 104). In a randomized controlled trial, it was clearly proved that Hemadsorption can early relieve organ dysfunction in critically ill patients with COVID-19 and stabilize the clinical symptoms, although it has no significant impact on inflammatory indicators and 28 day mortality (105). Besides, in a case report by Rajib Paul et al, they proposed that Hemadsorption was effective in providing hemodynamic stability, improving organ dysfunction, and modulating the cytokine storm (106).
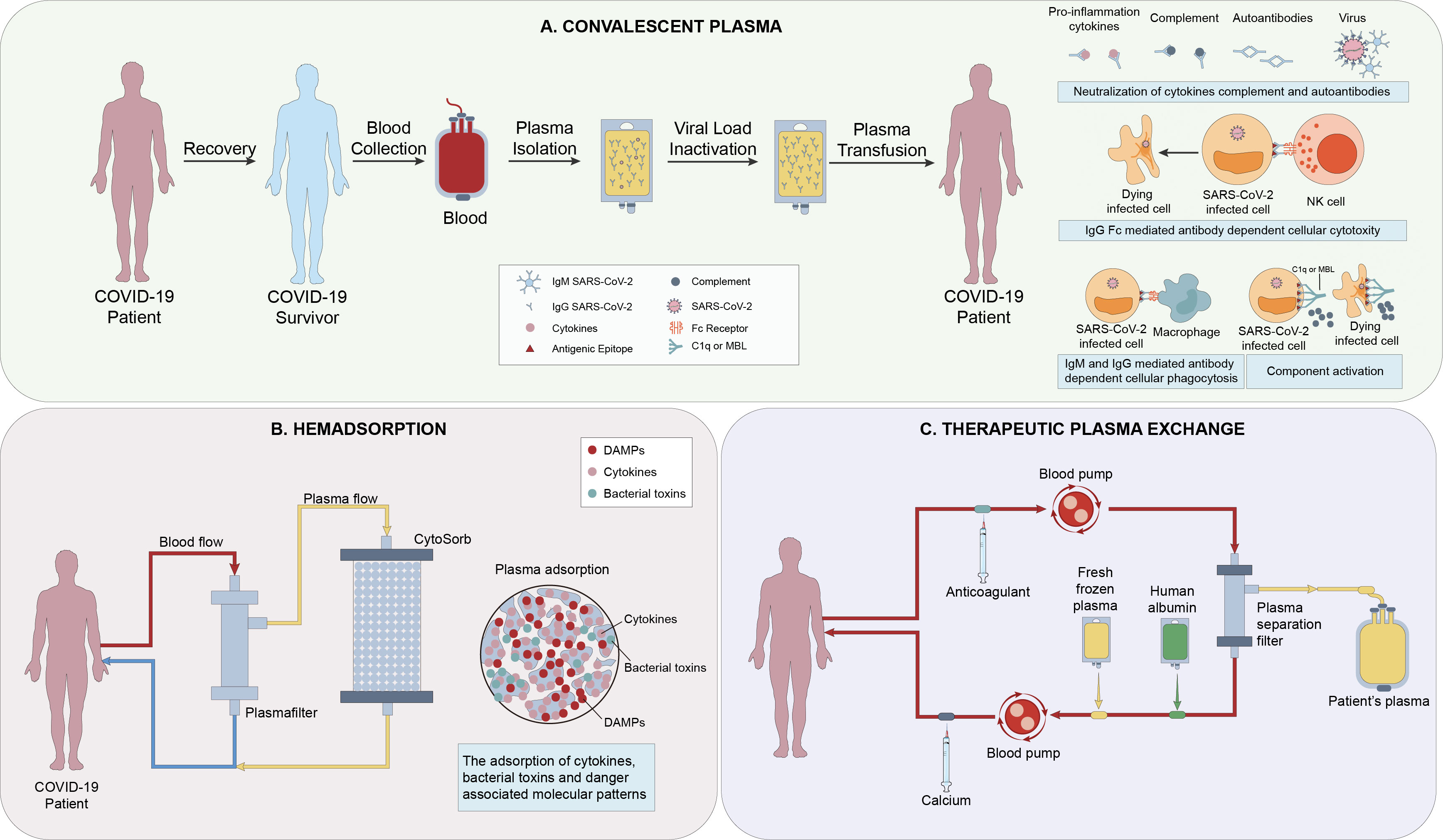
Figure 3 (A) The mechanisms of action of Convalescent Plasma(CP) include direct antiviral effects (eg, viral neutralization), viral clearance via immunoglobulin (Ig M and IgA-mediated neutralization, non-neutralizing IgG Fc-mediated functions (eg, antibody-dependent cellular cytotoxicity, phagocytosis and complement activation), and immunomodulation (1). (B) Hemadsorption is a technique in which a sorbent is placed in direct contact with blood in an extracorporeal circuit. CytoSorb is a highly adsorptive and biocompatible polymer able to eliminate various mediators (e.g., IL-1/6/8/10), bacterial toxins, and danger-associated molecular patterns (DAMPS) from the bloodstream (2). (C) Plasma exchange involves separating and discarding the plasma and blood cells in a patient’s body, and replacing them with an equal amount of fresh frozen plasma or human albumin exchange solution. There are metabolic toxins and various inflammatory mediators of all sizes present in the discarded plasma (3).
To sum up, TPE has therapeutic and even preventive effects on MAS of COVID-19 patients, and can reduce the mortality of patients. But hemadsorption may not have a direct impact on the mortality, but it can also stabilize the patient’s general condition to provide time for further treatment.
3.3 Extensive immunosuppressive agent
3.3.1 Steroids
According to the Consensus-Based Guidelines for the Recognition, Diagnosis, and Management of Hemophagocytic Lymphohistiocytosis in Critically Ill Children and Adults, corticosteroids are the backbone of the therapy of MAS (107). Moreover, the efficacy of corticosteroids as a basic treatment for MAS has been proven in many studies and clinical practices (108–110). Sathish Loganathan et al. reported two cases using tapering dose regimen of intravenous methylprednisolone (IVMP), and they achieved good results (111). In addition, early treatment of steroids can help stabilize the disease and buy time for further diagnosis and treatment (107).
But in the initial expert opinion, steroids are discouraged for use in viral lung infections, including COVID-19 (112). They believe that the immunosuppressive effect of steroids will lead to prolonged viremia and increased risk of bacterial coinfection. And based on some past research experience in the treatment of SARS-CoV-1 (113) and Middle East respiratory syndrome (MERS)-CoV (114), they believe that the use of steroid drugs should be very cautious.
However, in February 2021, Peter Horby et al. reported for the first time that dexamethasone (6 mg once daily for up to 10 days) reduced the 28-day mortality of hospitalized patients with COVID-19 through a randomized controlled trial (115). In a previous prospective meta-analysis of clinical trials in 257 critically ill patients with COVID-19, the use of systemic corticosteroids was associated with lower 28-day 258 all-cause mortality (116). In a recent meta-analysis conducted by Manisha Thakur et al., it further explained that the steroids play a significant role in the decrease of demises of hospitalized COVID-19 patients (117).
Frank L. van de Veerdunk et al. believes that the observation that the beneficial effects of steroids are significant in sicker patients could be explained by the pleiotropic effects of steroids (118). However, they also suggested that excessive use of corticosteroids, especially in the early stage of disease, may be harmful. In addition, some experts suggested that glucocorticoids should be used, but only at the right time (119). In other words, glucocorticoid can only be prescribed in the inflammatory phase of COVID-19 (120, 121). This is different from the therapeutic backbone role of steroids in MAS. Steroid is a systemic and widespread immunosuppressive drug, so it is easier to benefit from the treatment of MAS, while the use of early COVID-19 patients should be cautious, and it is easier to benefit from severe COVID-19 patients.
3.3.2 Calcineurin inhibitors (CNIs)
The two most common CNIs drugs are cyclosporine A (Cys) and tacrolimus (TAC), which are most commonly used in solid organ transplantation(SOT) (122) and systemic rheumatic diseases (123). When MAS develops to moderate or response to steroids is not evident, early initiation of cyclosporine a rapid and effective treatment option (124–126). Besides, later studies showed that anti thymocyte globulin (ATG) may be a safer choice for patients who failed to respond to the combined treatment of steroids and cyclosporine A (127–129).
As early as July 2020, Ren é Hage et al. found that a few of the patients with SOT who used tacrolimus reported severe COVID-19, and proposed that this type of drugs might prevent or even treat the excessive inflammation caused by SARS CoV-2 infection (130). This therapeutic drug may be superior to steroids. On the one hand, cyclosporine A can protect the upstream of cytokines in patients with COVID-19, rather than just targeting proinflammatory cytokines (131).On another hand, Long term and chronic use of glucocorticoids may lead to severe COVID-19, complications, etc., while chronic CNIs treatment has no effect on mortality (132). In addition to its immunosuppressive effect, CNIs can also prevent the occurrence of MAS from the upstream of cytokine storm through its antiviral activity shown in many RNA viruses (133, 134) and the ability to prevent mitochondrial failure (135, 136).
However, like the application of other extensive immunosuppressive drugs in viral infections, CNIs may lead to uncontrolled initial viral replication, viral immune evasion, and higher mortality rates (137–139). Moreover, there is still a lack of prospective and randomized clinical trials to demonstrate the specific effects of CNIs in the treatment of COVID-19.
3.4 Etoposide
The etoposide (VP-16)-based regimens, a kind of treatment approach for MAS, include HLH-94, HLH-04 and the modified Doxorubicin−Etoposide-Methylprednisolone (DEP) regimen (140).
In the HLH-94 and HLH-2004 regimens, the incorporation of etoposide and dexamethasone has been proposed as the treatment pillar. Karin Palmblad et al. found that the use of etoposide can lead to clinical improvement coinciding with a decline of systemic HMGB1, IL-18, IFN-γ, and ferritin levels (141). Although there is some evidence that this drug may aggravate the infection or make it easier to develop new infections, its benefits are obviously greater (140).
Modified Doxorubicin−Etoposide−Methylprednisolone (DEP) regimen has attracted much attention since it was proposed. Doxorubicin can quickly eliminate the overactivated macrophages and T cells (142). Besides, the dose of the glucocorticoid in the regimen was reduced to avoid the adverse effects in patients who failed to achieve remission after glucocorticoid pulse therapy. Recently, the research hold by He, L. et al. have demonstrated that modified DEP regimen is a promising alternative therapy for adults with R/R MAS owing to the high response rate, rapid action, and satisfactory tolerance (143).
As early as 2020, some people put forward the idea of using etoposide to treat severe patients with COVID-19 accompanied by systemic inflammation (144–146). In a mouse model study, the combination of low-dose etoposide and prednisolone improved the survival rate of fatal ARDS model mice, which all suffered from hypercytokinemia and MAS (147). They also found that this treatment combination can inhibit the recruitment and activation of macrophages, T cells, NK cells and neutrophils in the lung. And it has been proved that early administration of etoposide is a treatment option for EBV-related MAS patients (148). So similarly, low-dose etoposide may play a therapeutic role in treating MAS in COVID-19. Akiyoshi Takami believes that low-dose etoposide can update the CTL that has lost its function so that the abnormally activated macrophages and SARS-CoV-2 infected cells are eliminated, thus recovering the abnormal immune regulation associated with SARS-CoV-2 infection (146). A clinical trial lasting from May 8, 2020 to the present will likely contribute to further evaluate the safety and efficacy of etoposide in COVID-19 patients (ClinicalTrials.gov Identifier: NCT04356690).
3.5 Cytokine-targeted therapies
As mentioned earlier, many cytokines play a key role in the MAS caused by individual MAS and COVID-19, so the treatment methods targeting these cytokines are also the focus of current research.
3.5.1 IL-1
IL-1 blockers mainly include anakinra, canakinumab, rilonacept, gevokizumab and bermekimab.
Enough clinical trials have proved that anakinra can achieve obvious benefit in the treatment of macrophage activation syndrome (MAS), sepsis with MAS, and severe acute respiratory syndrome coronavirus 2–associated cytokine storm (149, 150).Recently, a retrospective analysis of 218 COVID-19 patients with MAS conducted by S. Amikishiyev et al. suggests that starting anakinra earlier in hospitalized patients may provide better results (151). Another retrospective analysis involving 16 patients showed that the patients had good tolerance to anakinra compared with the above etoposide-based therapy anakinra (152).Besides, there is a case report and a single-center, retrospective experience highlight that anakinra use in treating MAS is effective (153, 154). Therefore, there is no doubt that Anakinra has a positive effect on the treatment of MAS. In fact, this drug has also been included in the Consensus-Based Guidelines for the Recognition, Diagnosis, and Management of Hemophagocytic Lymphohistiocytosis in Critically Ill Children and Adults (107).
However, anakinra’s treatment effect on patients with COVID-19 is still controversial so far. A cohort study recorded a clear benefit from the use of this drug in severe forms of COVID-19 (155). They put forward that anakinra reduced both need for invasive mechanical ventilation in the ICU and mortality among patients with severe forms of COVID-19, without serious side-effects. Similarly, this view was put forward by two other cohort studies in the same period (156, 157). However, in a later randomized controlled experiment, totally different results were obtained (38). The experimental results showed that Anakinra could not effectively reduce the need for mechanical ventilation and mortality in patients with mild to moderate COVID-19. So, up to now, there are still several related studies on this drug in progress (ClinicalTrials.gov Identifier: NCT04357366 NCT04362111). Elnaz Khani et al. summarized the existing evidence on the use of Anakinra in COVID-19 (158). They believed that the benefits of Anakinra in patients with high inflammation were highly related to the duration of treatment, drug dose, route and time of administration. This is very similar to the treatment of anakinra in MAS. In the early stage of inflammation, around the first week of symptom onset, when patients need to supplement oxygen and do not undergo invasive mechanical ventilation within about 10 days, high dose of anakinra (>100 mg) may be effective and improve the results.
In addition to anakinra, canakinumab are also being tried for MAS treatment. Some studies have shown that canakinumab administered at doses of 4 mg/kg monthly does not have a major effect on the risk of developing MAS or on its clinical features (159). Some scholars believe that this is related to the dose. The monthly dose does not exceed 4 mg/kg, which may not be sufficient to neutralize the excessive IL- 1β in MAS activity. Kostik et al. recently described eight patients with sJIA treated with canakinumab. Several patients had the dose of canakinumab increased and they experienced the resolution of MAS. And there were no notable short-term adverse events (160). In a case-based review of Deniz Gezgin Yıldırım et al., a case that did not respond to anakinra but well responded to canakinumab was reported (161). Although this drug is more used in sJIA patients. But this may provide a new possibility for the management of MAS in COVID-19, especially for patients who do not respond to anakinra.
In August 2020, Ucciferri et al. described for the first time through a retrospective analysis that subcutaneous injection of canakinumab 300mg can rapidly reduce systemic inflammatory reaction and improve the oxygenation of COVID-19 patients (162). Lorenza Landi et al. also proved that this drug can reduce the inflammatory markers of patients with COVID-19 and improve the survival rate of patients (163). Furthermore, Guangyu Ao et al. infer that canakinumab could mitigate and even prevent immune-mediated tissue damage and organ dysfunction by limiting the level of acute inflammation and propensity for the activation of a cytokine storm (164–166). However, some people believe that the treatment of severe COVID-19 with canakinumab may increase the incidence rate of severe infection (167). And severe infection will induce macrophage activation syndrome. So, more trials are needed to prove the safety and efficacy of canakinumab injection in prevent MAS in COVID-19.
Besides, rilonacept is approved for the treatment of IL-1-mediated diseases too. Although rilonacept can improve some inflammatory diseases such as sJIA (168), familial cold autoinflammatory syndrome (FCAS) and Muckle-Wells syndrome (MWS) (169). However, rilonacept does not have much evidence and research to prove its therapeutic effect in COVID-19, just as it is in the treatment of MAS.
Overall, Anakinra seems to be superior to the other two drugs in the actual treatment process, which may be due to its better safty, rapid effect, short half-life and its ability to block both IL-1α and IL-1β. The increased dosage of canakinumab may provide a new possibility for the treatment of patients who do not respond to anakinra. The treatment of Rilonacept in MAS is relatively rare. It is easy to find that the effect of cytokines targeting drugs against IL-1 in preventing MAS in COVID-19 is different from that in patients with MAS alone, which may be related to the difference between MAS and MAS in COVID-19 mentioned above. Therefore, more research is needed to ensure the efficacy of these drugs.
3.5.2 IL-6
Tocilizumab is a recombinant humanized IL-6 receptor monoclonal antibody. Although it has been reported previously that tocilizumab can reduce the clinical symptoms of MAS (170).A recent single-center observational study found that up to 6 of the 20 patients with adult-onset Still’s disease (AOSD) under tocilizumab treatment developed clinically diagnosed MAS (171). In a case report, a patient successfully treated AOSD with tocilizumab at the beginning, but when it relapsed, it quickly developed symptoms of MAS after using tocilizumab again (172).However, in another case report, the combination of anakinra and tocilizumab successfully produced significant clinical improvement in a critically unwell patient (173). This may be because the selective inhibition of IL-6 by tocilizumab alone enhances the production of other proinflammatory cytokines such as TNF-α, IL-1, IL-18, etc. in AOSD patients prone to MAS (172).
In view of the efficacy of tocilizumab in MAS and the key role of IL-6 in COVID-19, Bingwen Liu et al. proposed to use this drug for COVID-19-induced CRS (20). This view has been gradually confirmed and improved. In a recent systematic review of a randomized clinical trial, Driton Vela et al. proposed that the use of tocilizumab in patients with moderate and severe COVID-19 could reduce all-cause mortality without increasing the number of serious adverse events (174). In another recent systematic review, they believed that immunosuppressants can significantly reduce mortality and have no effect on the increase of the risk of secondary infection (175). In terms of secondary infection, an umbrella review in August 2022 also put forward same views (176, 177). Their research shows that the treatment of steroids or steroids plus tocilizumab did not confer a higher risk of bacterial infections and improved survival rates. As mentioned above, overlapping infection is one of the inducements of MAS (42). Tocilizumab will not increase the risk of overlapping infection when reducing the inflammatory indicators of patients and blocking the cytokine storm, so it has an optimistic prospect for the management of MAS in patients with COVID-19.
However, the population and time of application of this drug are very critical. In a randomized controlled trial, they found that among patients with severe or critical COVID-19, tocilizumab did not benefit, and even increased mortality (178). The reason for this phenomenon was later explained in a review, in the early stage of COVID-19, cytokines may have a protective effect, while in the late stage, IL-6 will be cis phagocytic regulation, promoting cell growth and survival (179). Therefore, intervention measures should be taken in the second week of symptom onset (or within 7 days after hospitalization) to be benefit to patients, which could only disrupt the presentation and trans-signaling of pro-inflammatory IL-6 (180).
Therefore, the efficacy and safety of IL-6 monoclonal antibodies in the treatment of MAS in COVID-19 and in the treatment of COVID-19 itself need to be more fully evaluated through further prospective and well-designed clinical studies with larger sample sizes and long-term follow-up.
3.5.3 IL-18
There is evidence that the level of IL-18 in COVID-19 patients with MAS and ARDS is significantly higher than that in patients without MAS and ARDS, and high levels of IL-18 and IL-1Ra also seem to be related to the mortality of patients (181).
Tadekinig-α is a recombinant human IL-18-binding protein (rhIL-18BP). So far, there have been many results that can prove a favorable response toward tadekinig-α in MAS (ClinicalTrials.gov Identifier: NCT02398435, NCT03113760, NCT03512314). In an open-label, multicenter, dose-escalating phase II clinical trial, the use of tadekinig-α led to clinical improvement in 50% of patients with AOSD, and although there are some adverse events such as upper airway infections and arthralgia, most of them were mild and resolved after drug discontinuation (182). In addition, a recent case report described that MAS associated with XIAP deficiency was successfully controlled by using tadekinig-α (183).
At present, no relevant research has been found to prove the role of tadekinig- α in COVID-19. However, it still provides a new thought to prevent the occurrence of MAS and ARDS in patients with COVID-19 and improve the survival rate of patients.
3.5.4 IFN-γ
Emapalumab is a fully human monoclonal anti-IFN-γ antibody (184). In November 2018, emapalumab (Gamifant) obtained an FDA indication for the treatment of children and adults with relapsed/refractory (not newly diagnosed) HLH. Recently, Michael Ryan et al. report a case of CAR T therapy-associated MAS/HLH that was successfully treated with emapalumab in combination with anakinra and corticosteroids (185). After using Anakinra, tocilizumab, and dexamethasone, the patient’s clinical symptoms did not improve. However, after the addition of emapalumab, the patient became much more stable, and achieve rapid defervescence. In an open label, single arm, phase 2 study, a total of 14 patients were enrolled, of which 13 patients achieved clinical remission (186).
Erkan Cure et al. believed that emapalumab can be life saving for cytokine storm caused by COVID-19, which is resistant to anakinra, tocilizumab, and JAK inhibitors (187). In a recent case report, this is the first case of a pediatric patient with COVID-19 associated sHLH successfully treated with emapalumab (188). The patient in this case had symptoms relapse after using etoposide and dexamethasone, so she received HCT after using emapalumab, and she has been in remission after treatment. Although this shows that the application of this drug is promising, the case report cannot fully prove its therapeutic effect. Clinical trials with a large sample size are needed to scrutinize the efficacy of emapalumab in MAS caused by COVID-19.
Moreover, like the previous drugs, the time of application of Emapalumab is also very important, because at some stages, cytokines are crucial to eliminate SARS-CoV-2, and blocking the cytokine pathway may lead to more serious consequences (189).
3.5.5 JAKs pathway
Janus kinase inhibitor (JAKi) agents mainly include ruxolitinib, upadacitinib, baricitinib, tofacitinib, fedratinib, filgotinib, delgocitinib, oclacitinib and peficitinib, which act on different targets (190). Among them, ruxolitinib is the most widely used in the treatment of MAS, with a large proportion exhibiting favorable responses (191).
Compared with targeting any cytokine alone, ruxolitinib may produce more extensive anti-inflammatory activity, because these cytokines, such as IL-2, IL-6, IL-7, IL-10, IFN-γ, G-CSF, and GM-CSF, signals signal through the JAKs pathway (192). Ofer Levy et al. described a nearly fatal case of a young patient, which has been refractory to corticosteroids (CS), anakinra, tocilizumab, cyclosporine A (CSA), and etoposide, but eventually responded miraculously to salvage therapy with ruxolitinib (193). Josée-AnneJolyMSc et al. found that a therapy combining inhibition of JAK-dependent cytokines using ruxolitinib in clinically relevant doses with low doses of cytokine-specific antibodies against IFN-γ work in concert to temper the inflammatory response and control the overt activation of antigen-specific T cells, leading to a rapid and complete resolution of HLH in mice (194). Triebwasser et al. also described a patient with chronic active EBV-associated HLH who was refractory to HLH-2004 and anakinra but responded to a combination of emapalumab and ruxolitinib therapy (195).
Therefore, the potential of ruxolitinib for the treatment of severe COVID-19 has been concerned for a long time (192, 196, 197). However, in a recent randomized phase 3 trial (198) and a randomized, double-blind, placebo-controlled phase 3 trial (199), they have proved that ruxolitinib has no significance in the clinical improvement of patients and the reduction of 28-day mortality. Compared with the therapeutic effect of this drug in MAS, this is very surprising.
However, some scholars have come up with different results. After the combination with remdesivir, another JAK1/2 inhibitor, Baricitinib, can reduce recovery time and accelerate improvement in clinical status among patients with Covid-19 (200). In addition, in another randomized controlled trial, the combination of tofacitinib and glucocorticoid significantly reduced the 28-day mortality and the risk of respiratory failure in patients (201).
In summary, the treatment effect of the same treatment method in MAS alone and MAS caused by COVID-19 is different. This may be caused by different immune biological mechanisms. Some cytokines play a very important role in clearing the virus at the early stage of virus infection, before the appearance of MAS, and after the body is in a high inflammatory state, these cytokines will lead to the occurrence of MAS and ARDS (189). Therefore, the time of intervention, the way of use and the target population of different treatment methods are extremely important. A large number of clinical trials with more careful clinical design are needed to determine when and which treatment approaches is the most beneficial.
4 Conclusion
Although variants of SARS-CoV-2 are now becoming less virulent and transmissible, we still don’t know whether new variants will emerge and whether such variants will again pose a threat to public health. Therefore, the importance of further in-depth research on COVID-19 treatment cannot be made light of. This research aimed at MAS, which is involved in COVID-19 associated with worse disease severity and poorer prognosis. We summarized and analyzed the role of various therapeutic approaches in the two diseases. And found that the treatment effect of the same therapeutic approach is different (Table 1). This may be caused by different immune biological mechanisms between MAS alone and MAS caused by COVID-19. To deal this problem, further clinical trials and research are needed.
Author contributions
Z-H.T. and LMD supervised the study. TDC, SYC and LMD designed the structure. SYC search the paper and wrote the manuscript. TDC, SYC and DC drew the illustration. CZ analyzed references and design the table. All authors contributed to the article and approved the submitted version.
Funding
National Natural Science Foundation of China 81873870 (Z-HT).
Conflict of interest
The authors declare that the research was conducted in the absence of any commercial or financial relationships that could be construed as a potential conflict of interest.
Publisher’s note
All claims expressed in this article are solely those of the authors and do not necessarily represent those of their affiliated organizations, or those of the publisher, the editors and the reviewers. Any product that may be evaluated in this article, or claim that may be made by its manufacturer, is not guaranteed or endorsed by the publisher.
References
1. Guan WJ, Ni ZY, Hu Y, Liang WH, Ou CQ, He JX, et al. Clinical characteristics of coronavirus disease 2019 in China. N Engl J Med (2020) 382(18):1708–20. doi: 10.1056/NEJMoa2002032
2. Huang C, Wang Y, Li X, Ren L, Zhao J, Hu Y, et al. Clinical features of patients infected with 2019 novel coronavirus in wuhan, China. Lancet (2020) 395(10223):497–506. doi: 10.1016/S0140-6736(20)30183-5
3. Pons S, Fodil S, Azoulay E, Zafrani L. The vascular endothelium: the cornerstone of organ dysfunction in severe SARS-CoV-2 infection. Crit Care (2020) 24(1):353. doi: 10.1186/s13054-020-03062-7
4. Volz E, Mishra S, Chand M, Barrett JC, Johnson R, Geidelberg L, et al. Assessing transmissibility of SARS-CoV-2 lineage B.1.1.7 in England. Nature (2021) 593(7858):266–9. doi: 10.1038/s41586-021-03470-x
5. Coutinho RM, Marquitti FMD, Ferreira LS, Borges ME, da Silva RLP, Canton O, et al. Model-based estimation of transmissibility and reinfection of SARS-CoV-2 P.1 variant. Commun Med (Lond) (2021) 1:48. doi: 10.1038/s43856-021-00048-6
6. Davies NG, Jarvis CI, Edmunds WJ, Jewell NP, Diaz-Ordaz K, Keogh RH. Increased mortality in community-tested cases of SARS-CoV-2 lineage B.1.1.7. Nature (2021) 593(7858):270–4. doi: 10.1038/s41586-021-03426-1
7. Challen R, Brooks-Pollock E, Read JM, Dyson L, Tsaneva-Atanasova K, Danon L. Risk of mortality in patients infected with SARS-CoV-2 variant of concern 202012/1: matched cohort study. Bmj (2021) 372:n579. doi: 10.1136/bmj.n579
8. Faria NR, Mellan TA, Whittaker C, Claro IM, Candido DDS, Mishra S, et al. Genomics and epidemiology of the P.1 SARS-CoV-2 lineage in manaus, Brazil. Science (2021) 372(6544):815–21. doi: 10.1126/science.abh2644
9. Kannan S, Shaik Syed Ali P, Sheeza A. Omicron (B.1.1.529) - variant of concern - molecular profile and epidemiology: a mini review. Eur Rev Med Pharmacol Sci (2021) 25(24):8019–22. doi: 10.26355/eurrev_202112_27653
10. Planas D, Bruel T, Grzelak L, Guivel-Benhassine F, Staropoli I, Porrot F, et al. Sensitivity of infectious SARS-CoV-2 B.1.1.7 and B.1.351 variants to neutralizing antibodies. Nat Med (2021) 27(5):917–24. doi: 10.1038/s41591-021-01318-5
11. Edara VV, Hudson WH, Xie X, Ahmed R, Suthar MS. Neutralizing antibodies against SARS-CoV-2 variants after infection and vaccination. Jama (2021) 325(18):1896–8. doi: 10.1001/jama.2021.4388
12. Wang P, Nair MS, Liu L, Iketani S, Luo Y, Guo Y, et al. Antibody resistance of SARS-CoV-2 variants B.1.351 and B.1.1.7. Nature (2021) 593(7857):130–5. doi: 10.1038/s41586-021-03398-2
13. Cele S, Gazy I, Jackson L, Hwa SH, Tegally H, Lustig G, et al. Escape of SARS-CoV-2 501Y.V2 from neutralization by convalescent plasma. Nature (2021) 593(7857):142–6. doi: 10.1038/s41586-021-03471-w
14. Wibmer CK, Ayres F, Hermanus T, Madzivhandila M, Kgagudi P, Oosthuysen B, et al. SARS-CoV-2 501Y.V2 escapes neutralization by south African COVID-19 donor plasma. Nat Med (2021) 27(4):622–5. doi: 10.1038/s41591-021-01285-x
15. Allegra A, Di Gioacchino M, Tonacci A, Musolino C, Gangemi S. Immunopathology of SARS-CoV-2 infection: immune cells and mediators, prognostic factors, and immune-therapeutic implications. Int J Mol Sci (2020) 21(13):4782. doi: 10.3390/ijms21134782
16. Deng H, Tang TX, Chen D, Tang LS, Yang XP, Tang ZH. Endothelial dysfunction and SARS-CoV-2 infection: association and therapeutic strategies. Pathogens (2021) 10(5):582. doi: 10.3390/pathogens10050582
17. Zhou F, Yu T, Du R, Fan G, Liu Y, Liu Z, et al. Clinical course and risk factors for mortality of adult inpatients with COVID-19 in wuhan, China: a retrospective cohort study. Lancet (2020) 395(10229):1054–62. doi: 10.1016/S0140-6736(20)30566-3
18. Wu C, Chen X, Cai Y, Xia J, Zhou X, Xu S, et al. Risk factors associated with acute respiratory distress syndrome and death in patients with coronavirus disease 2019 pneumonia in wuhan, China. JAMA Intern Med (2020) 180(7):934–43. doi: 10.1001/jamainternmed.2020.0994
19. Chen G, Wu D, Guo W, Cao Y, Huang D, Wang H, et al. Clinical and immunological features of severe and moderate coronavirus disease 2019. J Clin Invest. (2020) 130(5):2620–9. doi: 10.1172/JCI137244
20. Liu B, Li M, Zhou Z, Guan X, Xiang Y. Can we use interleukin-6 (IL-6) blockade for coronavirus disease 2019 (COVID-19)-induced cytokine release syndrome (CRS)? J Autoimmun (2020) 111:102452. doi: 10.1016/j.jaut.2020.102452
21. Mehta P, McAuley DF, Brown M, Sanchez E, Tattersall RS, Manson JJ. COVID-19: consider cytokine storm syndromes and immunosuppression. Lancet (2020) 395(10229):1033–4. doi: 10.1016/S0140-6736(20)30628-0
22. Moore JB, June CH. Cytokine release syndrome in severe COVID-19. Science (2020) 368(6490):473–4. doi: 10.1126/science.abb8925
23. Qin C, Zhou L, Hu Z, Zhang S, Yang S, Tao Y, et al. Dysregulation of immune response in patients with coronavirus 2019 (COVID-19) in wuhan, China. Clin Infect Dis (2020) 71(15):762–8. doi: 10.1093/cid/ciaa248
24. Ye Q, Wang B, Mao J. The pathogenesis and treatment of the `Cytokine storm’ in COVID-19. J Infect (2020) 80(6):607–13. doi: 10.1016/j.jinf.2020.03.037
25. Chousterman BG, Swirski FK, Weber GF. Cytokine storm and sepsis disease pathogenesis. Semin Immunopathol (2017) 39(5):517–28. doi: 10.1007/s00281-017-0639-8
26. Yildiz H, Bailly S, Van Den Neste E, Yombi JC. Clinical management of Relapsed/Refractory hemophagocytic lymphohistiocytosis in adult patients: a review of current strategies and emerging therapies. Ther Clin Risk Manage (2021) 17:293–304. doi: 10.2147/TCRM.S195538
27. Javaux C, El-Jammal T, Neau PA, Fournier N, Gerfaud-Valentin M, Perard L, et al. Detection and prediction of macrophage activation syndrome in still’s disease. J Clin Med (2021) 11(1):206. doi: 10.3390/jcm11010206
28. Abdirakhmanova A, Sazonov V, Mukusheva Z, Assylbekova M, Abdukhakimova D, Poddighe D. Macrophage activation syndrome in pediatric systemic lupus erythematosus: a systematic review of the diagnostic aspects. Front Med (Lausanne). (2021) 8:681875. doi: 10.3389/fmed.2021.681875
29. Natoli V, Rosina S, Ravelli A. Is macrophage activation syndrome in Kawasaki disease underrecognized? J Rheumatol (2021) 48(2):162–4. doi: 10.3899/jrheum.200361
30. McGonagle D, Sharif K, O’Regan A, Bridgewood C. The role of cytokines including interleukin-6 in COVID-19 induced pneumonia and macrophage activation syndrome-like disease. Autoimmun Rev (2020) 19(6):102537. doi: 10.1016/j.autrev.2020.102537
31. Mert A, Vahaboğlu H, Arslan F, Batirel A, Saraçoğlu KT, Bastug A, et al. Tocilizumab treatment in severe COVID-19: a multicenter retrospective study with matched controls. Rheumatol Int (2022) 42(3):457–67. doi: 10.1007/s00296-021-04965-6
32. Ruiz-Antorán B, Sancho-López A, Torres F, Moreno-Torres V, de Pablo-López I, García-López P, et al. Combination of tocilizumab and steroids to improve mortality in patients with severe COVID-19 infection: a Spanish, multicenter, cohort study. Infect Dis Ther (2021) 10(1):347–62. doi: 10.1007/s40121-020-00373-8
33. Schulert GS, Grom AA. Macrophage activation syndrome and cytokine-directed therapies. Best Pract Res Clin Rheumatol (2014) 28(2):277–92. doi: 10.1016/j.berh.2014.03.002
34. Shimizu M, Mizuta M, Okamoto N, Yasumi T, Iwata N, Umebayashi H, et al. Tocilizumab modifies clinical and laboratory features of macrophage activation syndrome complicating systemic juvenile idiopathic arthritis. Pediatr Rheumatol Online J (2020) 18(1):2. doi: 10.1186/s12969-020-0399-1
35. Otsuka R, Seino KI. Macrophage activation syndrome and COVID-19. Inflammation Regen. (2020) 40:19. doi: 10.1186/s41232-020-00131-w
36. Sönmez HE, Demir S, Bilginer Y, Özen S. Anakinra treatment in macrophage activation syndrome: a single center experience and systemic review of literature. Clin Rheumatol (2018) 37(12):3329–35. doi: 10.1007/s10067-018-4095-1
37. Stone JH, Frigault MJ, Serling-Boyd NJ, Fernandes AD, Harvey L, Foulkes AS, et al. Efficacy of tocilizumab in patients hospitalized with covid-19. N Engl J Med (2020) 383(24):2333–44. doi: 10.1056/NEJMoa2028836
38. CORIMUNO-19 Collaborative group Effect of anakinra versus usual care in adults in hospital with COVID-19 and mild-to-moderate pneumonia (CORIMUNO-ANA-1): a randomised controlled trial. Lancet Respir Med (2021) 9(3):295–304. doi: 10.1016/S2213-2600(20)30556-7
39. Salvarani C, Dolci G, Massari M, Merlo DF, Cavuto S, Savoldi L, et al. Effect of tocilizumab vs standard care on clinical worsening in patients hospitalized with COVID-19 pneumonia: a randomized clinical trial. JAMA Intern Med (2021) 181(1):24–31. doi: 10.1001/jamainternmed.2020.6615
40. Schulert GS, Pickering AV, Do T, Dhakal S, Fall N, Schnell D, et al. Monocyte and bone marrow macrophage transcriptional phenotypes in systemic juvenile idiopathic arthritis reveal TRIM8 as a mediator of IFN-γ hyper-responsiveness and risk for macrophage activation syndrome. Ann Rheum Dis (2021) 80(5):617–25. doi: 10.1136/annrheumdis-2020-217470
41. Ponnatt TS, Lilley CM, Mirza KM. Hemophagocytic lymphohistiocytosis. Arch Pathol Lab Med (2022) 146(4):507–19. doi: 10.5858/arpa.2020-0802-RA
42. Strippoli R, Caiello I, De Benedetti F. Reaching the threshold: a multilayer pathogenesis of macrophage activation syndrome. J Rheumatol (2013) 40(6):761–7. doi: 10.3899/jrheum.121233
43. Bojan A, Parvu A, Zsoldos IA, Torok T, Farcas AD. Macrophage activation syndrome: a diagnostic challenge (Review). Exp Ther Med (2021) 22(2):904. doi: 10.3892/etm.2021.10336
44. Tay MZ, Poh CM, Rénia L, MacAry PA, Ng LFP. The trinity of COVID-19: immunity, inflammation and intervention. Nat Rev Immunol (2020) 20(6):363–74. doi: 10.1038/s41577-020-0311-8
45. Webb BJ, Peltan ID, Jensen P, Hoda D, Hunter B, Silver A, et al. Clinical criteria for COVID-19-associated hyperinflammatory syndrome: a cohort study. Lancet Rheumatol (2020) 2(12):e754–e63. doi: 10.1016/S2665-9913(20)30343-X
46. Manson JJ, Crooks C, Naja M, Ledlie A, Goulden B, Liddle T, et al. COVID-19-associated hyperinflammation and escalation of patient care: a retrospective longitudinal cohort study. Lancet Rheumatol (2020) 2(10):e594–602. doi: 10.1016/S2665-9913(20)30275-7
47. Hoffmann M, Kleine-Weber H, Schroeder S, Krüger N, Herrler T, Erichsen S, et al. SARS-CoV-2 cell entry depends on ACE2 and TMPRSS2 and is blocked by a clinically proven protease inhibitor. Cell (2020) 181(2):271–80.e8. doi: 10.1016/j.cell.2020.02.052
48. Zhou P, Yang XL, Wang XG, Hu B, Zhang L, Zhang W, et al. A pneumonia outbreak associated with a new coronavirus of probable bat origin. Nature (2020) 579(7798):270–3. doi: 10.1038/s41586-020-2012-7
49. Martines RB, Ritter JM, Matkovic E, Gary J, Bollweg BC, Bullock H, et al. Pathology and pathogenesis of SARS-CoV-2 associated with fatal coronavirus disease, united states. Emerg Infect Dis (2020) 26(9):2005–15. doi: 10.3201/eid2609.202095
50. Grant RA, Morales-Nebreda L, Markov NS, Swaminathan S, Querrey M, Guzman ER, et al. Circuits between infected macrophages and T cells in SARS-CoV-2 pneumonia. Nature (2021) 590(7847):635–41. doi: 10.1038/s41586-020-03148-w
51. Wang C, Xie J, Zhao L, Fei X, Zhang H, Tan Y, et al. Alveolar macrophage dysfunction and cytokine storm in the pathogenesis of two severe COVID-19 patients. EBioMedicine (2020) 57:102833. doi: 10.1016/j.ebiom.2020.102833
52. Gao YM, Xu G, Wang B, Liu BC. Cytokine storm syndrome in coronavirus disease 2019: a narrative review. J Intern Med (2021) 289(2):147–61. doi: 10.1111/joim.13144
53. Billiau AD, Roskams T, Van Damme-Lombaerts R, Matthys P, Wouters C. Macrophage activation syndrome: characteristic findings on liver biopsy illustrating the key role of activated, IFN-gamma-producing lymphocytes and IL-6- and TNF-alpha-producing macrophages. Blood (2005) 105(4):1648–51. doi: 10.1182/blood-2004-08-2997
54. Crayne CB, Albeituni S, Nichols KE, Cron RQ. The immunology of macrophage activation syndrome. Frontiers in Immunology (2019) 10:119. doi: 10.3389/fimmu.2019.00119
55. Ombrello MJ, Schulert GS. COVID-19 and cytokine storm syndrome: are there lessons from macrophage activation syndrome? Transl Res (2021) 232:1–12. doi: 10.1016/j.trsl.2021.03.002
56. Kaur S, Bansal R, Kollimuttathuillam S, Gowda AM, Singh B, Mehta D, et al. The looming storm: blood and cytokines in COVID-19. Blood Rev (2021) 46:100743. doi: 10.1016/j.blre.2020.100743
57. Bar-On YM, Flamholz A, Phillips R, Milo R. SARS-CoV-2 (COVID-19) by the numbers. Elife (2020) 9:e57309. doi: 10.7554/eLife.57309
58. Sinha P, Matthay MA, Calfee CS. Is a “Cytokine storm” relevant to COVID-19? JAMA Intern Med (2020) 180(9):1152–4. doi: 10.1001/jamainternmed.2020.3313
59. McGonagle D, Ramanan AV, Bridgewood C. Immune cartography of macrophage activation syndrome in the COVID-19 era. Nat Rev Rheumatol (2021) 17(3):145–57. doi: 10.1038/s41584-020-00571-1
60. Pandolfi L, Fossali T, Frangipane V, Bozzini S, Morosini M, D’Amato M, et al. Broncho-alveolar inflammation in COVID-19 patients: a correlation with clinical outcome. BMC Pulmonary Med (2020) 20(1):301. doi: 10.1186/s12890-020-01343-z
61. Liao M, Liu Y, Yuan J, Wen Y, Xu G, Zhao J, et al. Single-cell landscape of bronchoalveolar immune cells in patients with COVID-19. Nat Med (2020) 26(6):842–4. doi: 10.1038/s41591-020-0901-9
62. Grant RA, Morales-Nebreda L, Markov NS, Swaminathan S, Guzman ER, Abbott DA, et al. Alveolitis in severe SARS-CoV-2 pneumonia is driven by self-sustaining circuits between infected alveolar macrophages and T cells. bioRxiv (2020) 590(7847):635–41. doi: 10.1101/2020.08.05.238188
63. Dorward DA, Russell CD, Um IH, Elshani M, Armstrong SD, Penrice-Randal R, et al. Tissue-specific immunopathology in fatal COVID-19. Am J Respir Crit Care Med (2021) 203(2):192–201. doi: 10.1164/rccm.202008-3265OC
64. Leisman DE, Ronner L, Pinotti R, Taylor MD, Sinha P, Calfee CS, et al. Cytokine elevation in severe and critical COVID-19: a rapid systematic review, meta-analysis, and comparison with other inflammatory syndromes. Lancet Respir Med (2020) 8(12):1233–44. doi: 10.1016/S2213-2600(20)30404-5
65. Caricchio R, Gallucci M, Dass C, Zhang X, Gallucci S, Fleece D, et al. Preliminary predictive criteria for COVID-19 cytokine storm. Ann Rheum Dis (2021) 80(1):88–95. doi: 10.1136/annrheumdis-2020-218323
66. Leverenz DL, Tarrant TK. Is the HScore useful in COVID-19? Lancet (2020) 395(10236):e83. doi: 10.1016/S0140-6736(20)31057-6
67. Kyriazopoulou E, Poulakou G, Milionis H, Metallidis S, Adamis G, Tsiakos K, et al. Early treatment of COVID-19 with anakinra guided by soluble urokinase plasminogen receptor plasma levels: a double-blind, randomized controlled phase 3 trial. Nat Med (2021) 27(10):1752–60. doi: 10.1038/s41591-021-01499-z
68. Hoenigl M, Seidel D, Sprute R, Cunha C, Oliverio M, Goldman GH, et al. COVID-19-associated fungal infections. Nat Microbiol (2022) 7(8):1127–40. doi: 10.1038/s41564-022-01172-2
69. Sapir T, Averch Z, Lerman B, Bodzin A, Fishman Y, Maitra R. COVID-19 and the immune response: a multi-phasic approach to the treatment of COVID-19. Int J Mol Sci (2022) 23(15):8606. doi: 10.3390/ijms23158606
70. Tran A, Witek TJ Jr.. The emergency use authorization of pharmaceuticals: history and utility during the COVID-19 pandemic. Pharmaceut Med (2021) 35(4):203–13. doi: 10.1007/s40290-021-00397-6
71. Vitiello A, Troiano V, La Porta R. What will be the role of molnupiravir in the treatment of COVID-19 infection? Drugs Ther Perspect (2021) 37(12):579–80. doi: 10.1007/s40267-021-00879-2
73. Vitiello A, Ferrara F, Porta R. Remdesivir and COVID-19 infection, therapeutic benefits or unnecessary risks? Ir J Med Sci (2021) 190(4):1637–8. doi: 10.1007/s11845-020-02482-2
74. Saravolatz LD, Depcinski S, Sharma M. Molnupiravir and nirmatrelvir-ritonavir: oral coronavirus disease 2019 antiviral drugs. Clin Infect Diseases. (2023) 76(1):165–71. doi: 10.1093/cid/ciac180
75. Wen W, Chen C, Tang J, Wang C, Zhou M, Cheng Y, et al. Efficacy and safety of three new oral antiviral treatment (molnupiravir, fluvoxamine and paxlovid) for COVID-19:a meta-analysis. Ann Med (2022) 54(1):516–23. doi: 10.1080/07853890.2022.2034936
76. Kloypan C, Saesong M, Sangsuemoon J, Chantharit P, Mongkhon P. CONVALESCENT plasma for COVID-19: a meta-analysis of clinical trials and real-world evidence. Eur J Clin Invest. (2021) 51(11):e13663. doi: 10.1111/eci.13663
77. Klassen SA, Senefeld JW, Johnson PW, Carter RE, Wiggins CC, Shoham S, et al. The effect of convalescent plasma therapy on mortality among patients with COVID-19: systematic review and meta-analysis. Mayo Clin Proc (2021) 96(5):1262–75. doi: 10.1016/j.mayocp.2021.02.008
78. Sullivan DJ, Gebo KA, Shoham S, Bloch EM, Lau B, Shenoy AG, et al. Early outpatient treatment for covid-19 with convalescent plasma. N Engl J Med (2022) 386(18):1700–11. doi: 10.1056/NEJMoa2119657
79. Aryana I, Daniella D, Paulus IB, Rini SS, Setiati S. Convalescent plasma in older adults with COVID-19: a systematic review and meta-analysis. Ann Geriatr Med Res (2022) 26(3):208–14. doi: 10.4235/agmr.22.0045
80. Senefeld JW, Franchini M, Mengoli C, Cruciani M, Zani M, Gorman EK, et al. COVID-19 convalescent plasma for the treatment of immunocompromised patients: a systematic review and meta-analysis. JAMA Netw Open (2023) 6(1):e2250647. doi: 10.1001/jamanetworkopen.2022.50647
81. Tremblay D, Seah C, Schneider T, Bhalla S, Feld J, Naymagon L, et al. Convalescent plasma for the treatment of severe COVID-19 infection in cancer patients. Cancer Med (2020) 9(22):8571–8. doi: 10.1002/cam4.3457
82. Crayne CB, Albeituni S, Nichols KE, Cron RQ. The immunology of macrophage activation syndrome. Front Immunol (2019) 10:119. doi: 10.3389/fimmu.2019.00119
83. Valk SJ, Piechotta V, Chai KL, Doree C, Monsef I, Wood EM, et al. Convalescent plasma or hyperimmune immunoglobulin for people with COVID-19: a rapid review. Cochrane Database Syst Rev (2020) 5(5):Cd013600. doi: 10.1002/14651858.CD013600
84. Kunze KL, Johnson PW, van Helmond N, Senefeld JW, Petersen MM, Klassen SA, et al. Mortality in individuals treated with COVID-19 convalescent plasma varies with the geographic provenance of donors. Nat Commun (2021) 12(1):4864. doi: 10.1038/s41467-021-25113-5
85. Chen Y, Hu Z, Cai S, Shen G, Zhong J, Dong L. Efficacy of plasma exchange on top of standard immunosuppressive therapy in adult autoimmune inflammatory rheumatic diseases-associated macrophage activation syndrome, a single center real-world analysis. Semin Arthritis Rheumatism. (2022) 55:152043. doi: 10.1016/j.semarthrit.2022.152043
86. Yuan YH, Zhang H, Xiao ZH, Zhang XP, Lu XL, Xu ZY, et al. Efficacy of plasma exchange in children with severe hemophagocytic syndrome: a prospective randomized controlled trial. Zhongguo Dang Dai Er Ke Za Zhi. (2022) 24(3):249–54. doi: 10.7499/j.issn.1008-8830.2110111
87. Sato S, Hosokawa T, Kawashima H. Successful treatment of plasma exchange for refractory systemic juvenile idiopathic arthritis complicated with macrophage activation syndrome and severe lung disease. Ann Rheumatic Diseases. (2022) 81(4):e61. doi: 10.1136/annrheumdis-2020-217390
88. Keith P, Day M, Perkins L, Moyer L, Hewitt K, Wells A. A novel treatment approach to the novel coronavirus: an argument for the use of therapeutic plasma exchange for fulminant COVID-19. Crit Care (2020) 24(1):128. doi: 10.1186/s13054-020-2836-4
89. Faqihi F, Alharthy A, Abdulaziz S, Balhamar A, Alomari A, AlAseri Z, et al. Therapeutic plasma exchange in patients with life-threatening COVID-19: a randomised controlled clinical trial. Int J Antimicrobial Agents. (2021) 57(5):106334. doi: 10.1016/j.ijantimicag.2021.106334
90. Arulkumaran N, Thomas M, Brealey D, Alwan F, Singh D, Lunn M, et al. Plasma exchange for COVID-19 thrombo-inflammatory disease. eJHaem (2021) 2(1):26–32. doi: 10.1002/jha2.140
91. Gucyetmez B, Atalan HK, Sertdemir I, Cakir U, Telci L, Ogan A, et al. Therapeutic plasma exchange in patients with COVID-19 pneumonia in intensive care unit: a retrospective study. Crit Care (2020) 24(1):492. doi: 10.1186/s13054-020-03215-8
92. Khamis F, Al-Zakwani I, Al Hashmi S, Al Dowaiki S, Al Bahrani M, Pandak N, et al. Therapeutic plasma exchange in adults with severe COVID-19 infection. Int J Infect Diseases. (2020) 99:214–8. doi: 10.1016/j.ijid.2020.06.064
93. Faqihi F, Alharthy A, Alodat M, Kutsogiannis DJ, Brindley PG, Karakitsos D. Therapeutic plasma exchange in adult critically ill patients with life-threatening SARS-CoV-2 disease: a pilot study. J Crit Care (2020) 60:328–33. doi: 10.1016/j.jcrc.2020.07.001
94. Hashemian SM, Shafigh N, Afzal G, Jamaati H, Tabarsi P, Marjani M, et al. Plasmapheresis reduces cytokine and immune cell levels in COVID-19 patients with acute respiratory distress syndrome (ARDS). Pulmonology (2021) 27(6):486–92. doi: 10.1016/j.pulmoe.2020.10.017
95. Jaiswal V, Nasa P, Raouf M, Gupta M, Dewedar H, Mohammad H, et al. Therapeutic plasma exchange followed by convalescent plasma transfusion in critical COVID-19–an exploratory study. Int J Infect Diseases. (2021) 102:332–4. doi: 10.1016/j.ijid.2020.10.085
96. Morath C, Weigand MA, Zeier M, Speer C, Tiwari-Heckler S, Merle U. Plasma exchange in critically ill COVID-19 patients. Crit Care (2020) 24(1):481. doi: 10.1186/s13054-020-03171-3
97. Fernandez J, Gratacos-Ginès J, Olivas P, Costa M, Nieto S, Mateo D, et al. Plasma exchange: an effective rescue therapy in critically ill patients with coronavirus disease 2019 infection. Crit Care Med (2020) 48(12):e1350–e5. doi: 10.1097/CCM.0000000000004613
98. Qin J, Wang G, Han D. Benefits of plasma exchange on mortality in patients with COVID-19: a systematic review and meta-analysis. Int J Infect Diseases. (2022) 122:332–6. doi: 10.1016/j.ijid.2022.06.014
99. Köhler T, Schwier E, Praxenthaler J, Kirchner C, Henzler D, Eickmeyer C. Therapeutic modulation of the host defense by hemoadsorption with CytoSorb®—Basics, indications and Perspectives—A scoping review. Int J Mol Sci (2021) 22(23):12786. doi: 10.3390/ijms222312786
100. Jesudas R, Nichols KE. Recent advances in the treatment of hemophagocytic lymphohistiocytosis and macrophage activation syndrome. Curr Opin Allergy Clin Immunol (2022) 22(6):364–70. doi: 10.1097/ACI.0000000000000865
101. Rademacher JG, Wulf G, Koziolek MJ, Zeisberg M, Wallbach M. Cytokine adsorption therapy in lymphoma-associated hemophagocytic lymphohistiocytosis and allogeneic stem cell transplantation. J Artif Organs. (2021) 24(3):402–6. doi: 10.1007/s10047-020-01244-2
102. Bottari G, Murciano M, Merli P, Bracaglia C, Guzzo I, Stoppa F, et al. Hemoperfusion with CytoSorb to manage multiorgan dysfunction in the spectrum of hemophagocytic lymphohistiocytosis syndrome in critically ill children. Blood Purif. (2022) 51(5):417–24. doi: 10.1159/000517471
103. Ruiz-Rodríguez JC, Chiscano-Camón L, Ruiz-Sanmartin A, Palmada C, Bajaña I, Iacoboni G, et al. Case report: cytokine hemoadsorption in a case of hemophagocytic lymphohistiocytosis secondary to extranodal NK/T-cell lymphoma. Front Med (Lausanne). (2022) 9:925751. doi: 10.3389/fmed.2022.925751
104. Ceruti S, Glotta A, Adamson H, Mauri R, Molnar Z. Hemoadsorption treatment with CytoSorb® in probable hemophagocytic lymphohistiocytosis: a role as adjunctive therapy? Case Rep Hematol (2021) 2021:5539126. doi: 10.1155/2021/5539126
105. Jarczak D, Roedl K, Fischer M, de Heer G, Burdelski C, Frings DP, et al. Effect of hemadsorption therapy in critically ill patients with COVID-19 (CYTOCOV-19): a prospective randomized controlled pilot trial. Blood Purification (2022) 52(2):183–92. doi: 10.21203/rs.3.rs-704552/v1
106. Paul R, Aleem M, Gorla N. CytoSorb® in the treatment of severely-ill patient with post-COVID-19 complications: a case report. Clin Case Rep Open Access. (2022) 5(4):238. doi: 10.46527/2582-5038.238
107. Hines MR, von Bahr Greenwood T, Beutel G, Beutel K, Hays JA, Horne A, et al. Consensus-based guidelines for the recognition, diagnosis, and management of hemophagocytic lymphohistiocytosis in critically ill children and adults. Crit Care Med (2022) 50(5):860–72. doi: 10.1097/CCM.0000000000005361
108. Bossi G, Codazzi AC, Vinci F, Clerici E, Regalbuto C, Crapanzano C, et al. Efficacy of anakinra on multiple coronary arteries aneurysms in an infant with recurrent Kawasaki disease, complicated by macrophage activation syndrome. Children (2022) 9(5):672. doi: 10.3390/children9050672
109. Yi G, Huang Z, Huang Z, Wang Y, Deng W, Zheng S, et al. Case report: baricitinib as an alternative in the maintenance therapy for macrophage activation syndrome secondary to nodular panniculitis. Front Immunol (2022) 13:914265. doi: 10.3389/fimmu.2022.914265
110. Wang R, Li T, Ye S, Lv L, Chen S, Wang X, et al. Short-term, low-dose etoposide in refractory adult-onset still’s disease-associated macrophage activation syndrome. Clin Rheumatol (2022) 41(9):2817–23. doi: 10.1007/s10067-022-06184-1
111. Loganathan S, Banday A, Jindal AK, Sudhakar M, Patra N, Pulipaka S, et al. Tapering doses of methylprednisolone pulse in the treatment of macrophage activation syndrome associated with systemic juvenile idiopathic arthritis. Indian J Pediatrics. (2021) 88(10):1056–. doi: 10.1007/s12098-021-03831-4
112. Mattos-Silva P, Felix NS, Silva PL, Robba C, Battaglini D, Pelosi P, et al. Pros and cons of corticosteroid therapy for COVID-19 patients. Respir Physiol Neurobiology. (2020) 280:103492. doi: 10.1016/j.resp.2020.103492
113. Stockman LJ, Bellamy R, Garner P. SARS: systematic review of treatment effects. PloS Med (2006) 3(9):e343. doi: 10.1371/journal.pmed.0030343
114. Arabi YM, Mandourah Y, Al-Hameed F, Sindi AA, Almekhlafi GA, Hussein MA, et al. Corticosteroid therapy for critically ill patients with middle East respiratory syndrome. Am J Respir Crit Care Med (2018) 197(6):757–67. doi: 10.1164/rccm.201706-1172OC
115. Horby P, Lim WS, Emberson JR, Mafham M, Bell JL, Linsell L, et al. Dexamethasone in hospitalized patients with covid-19. N Engl J Med (2021) 384(8):693–704. doi: 10.1056/NEJMoa2021436
116. Sterne JAC, Murthy S, Diaz JV, Slutsky AS, Villar J, Angus DC, et al. Association between administration of systemic corticosteroids and mortality among critically ill patients with COVID-19: a meta-analysis. Jama (2020) 324(13):1330–41. doi: 10.1001/jama.2020.17023
117. Thakur M, Datusalia AK, Kumar A. Use of steroids in COVID-19 patients: a meta-analysis. Eur J Pharmacol (2022) 914:174579. doi: 10.1016/j.ejphar.2021.174579
118. van de Veerdonk FL, Giamarellos-Bourboulis E, Pickkers P, Derde L, Leavis H, van Crevel R, et al. A guide to immunotherapy for COVID-19. Nat Med (2022) 28(1):39–50. doi: 10.1038/s41591-021-01643-9
119. Fernández-Cruz A, Ruiz-Antorán B, Múñez-Rubio E, Sancho-López A, Callejas-Díaz A, Avendaño-Solá C, et al. The right time for steroids in COVID-19. Clin Infect Dis (2021) 72(8):1486–7. doi: 10.1093/cid/ciaa865
120. Griffin DO, Brennan-Rieder D, Ngo B, Kory P, Confalonieri M, Shapiro L, et al. The importance of understanding the stages of COVID-19 in treatment and trials. AIDS Rev (2021) 23(1):40–7. doi: 10.24875/AIDSRev.200001261
121. Ngo BT, Marik P, Kory P, Shapiro L, Thomadsen R, Iglesias J, et al. The time to offer treatments for COVID-19. Expert Opin Investig Drugs (2021) 30(5):505–18. doi: 10.1080/13543784.2021.1901883
122. Kuypers DRJ. Intrapatient variability of tacrolimus exposure in solid organ transplantation: a novel marker for clinical outcome. Clin Pharmacol Ther (2020) 107(2):347–58. doi: 10.1002/cpt.1618
123. Cavagna L, Caporali R, Abdì-Alì L, Dore R, Meloni F, Montecucco C. Cyclosporine in anti-Jo1-positive patients with corticosteroid-refractory interstitial lung disease. J Rheumatol (2013) 40(4):484–92. doi: 10.3899/jrheum.121026
124. La Rosée P, Horne A, Hines M, von Bahr Greenwood T, Machowicz R, Berliner N, et al. Recommendations for the management of hemophagocytic lymphohistiocytosis in adults. Blood (2019) 133(23):2465–77. doi: 10.1182/blood.2018894618
125. Zhou S, Qiao J, Bai J, Wu Y, Fang H. Biological therapy of traditional therapy-resistant adult-onset still’s disease: an evidence-based review. Ther Clin Risk Manage (2018) 14:167–71. doi: 10.2147/TCRM.S155488
126. Rajagopala L, Jagoda J, Gunatilaka ML. P163 The cytokine horror: Kawasaki disease shock syndrome complicated with macrophage activation syndrome. Rheumatology (2022) 61(Supplement_1):keac133.162. doi: 10.1093/rheumatology/keac133.162
127. Coca A, Bundy KW, Marston B, Huggins J, Looney RJ. Macrophage activation syndrome: serological markers and treatment with anti-thymocyte globulin. Clin Immunol (2009) 132(1):10–8. doi: 10.1016/j.clim.2009.02.005
128. Mahlaoui N, Ouachée-Chardin M, de Saint Basile G, Neven B, Picard C, Blanche S, et al. Immunotherapy of familial hemophagocytic lymphohistiocytosis with antithymocyte globulins: a single-center retrospective report of 38 patients. Pediatrics (2007) 120(3):e622–8. doi: 10.1542/peds.2006-3164
129. Ponnatt TS, Lilley CM, Mirza KM. Hemophagocytic lymphohistiocytosis. Arch Pathol Lab Med (2021) 146(4):507–19. doi: 10.5858/arpa.2020-0802-RA
130. Hage R, Steinack C, Schuurmans MM. Calcineurin inhibitors revisited: a new paradigm for COVID-19? Braz J Infect Diseases. (2020) 24:365–7. doi: 10.1016/j.bjid.2020.06.005
131. Sanchez-Pernaute O, Romero-Bueno FI, Selva-O’Callaghan A. Why choose cyclosporin a as first-line therapy in COVID-19 pneumonia. Reumatol Clin (Engl Ed). (2021) 17(9):556–7. doi: 10.1016/j.reumae.2020.03.005
132. Calderón-Parra J, Cuervas-Mons V, Moreno-Torres V, Rubio-Rivas M, PA-d B, Pinilla-Llorente B, et al. Influence of chronic use of corticosteroids and calcineurin inhibitors on COVID-19 clinical outcomes: analysis of a nationwide registry. Int J Infect Diseases. (2022) 116:51–8. doi: 10.1016/j.ijid.2021.12.327
133. Pfefferle S, Schöpf J, Kögl M, Friedel CC, Müller MA, Carbajo-Lozoya J, et al. The SARS-coronavirus-host interactome: identification of cyclophilins as target for pan-coronavirus inhibitors. PloS Pathog (2011) 7(10):e1002331. doi: 10.1371/journal.ppat.1002331
134. de Wilde AH, Raj VS, Oudshoorn D, Bestebroer TM, van Nieuwkoop S, Limpens R, et al. MERS-coronavirus replication induces severe in vitro cytopathology and is strongly inhibited by cyclosporin a or interferon-α treatment. J Gen Virol (2013) 94(Pt 8):1749–60. doi: 10.1099/vir.0.052910-0
135. Lebedev I, Nemajerova A, Foda ZH, Kornaj M, Tong M, Moll UM, et al. A novel In vitro CypD-mediated p53 aggregation assay suggests a model for mitochondrial permeability transition by chaperone systems. J Mol Biol (2016) 428(20):4154–67. doi: 10.1016/j.jmb.2016.08.001
136. Baines CP, Kaiser RA, Purcell NH, Blair NS, Osinska H, Hambleton MA, et al. Loss of cyclophilin d reveals a critical role for mitochondrial permeability transition in cell death. Nature (2005) 434(7033):658–62. doi: 10.1038/nature03434
137. Belli LS, Fondevila C, Cortesi PA, Conti S, Karam V, Adam R, et al. Protective role of tacrolimus, deleterious role of age and comorbidities in liver transplant recipients with covid-19: results from the ELITA/ELTR multi-center European study. Gastroenterology (2021) 160(4):1151–63.e3. doi: 10.1053/j.gastro.2020.11.045
138. Urra JM, Cabrera CM, Porras L, Ródenas I. Selective CD8 cell reduction by SARS-CoV-2 is associated with a worse prognosis and systemic inflammation in COVID-19 patients. Clin Immunol (2020) 217:108486. doi: 10.1016/j.clim.2020.108486
139. Belsky JA, Tullius BP, Lamb MG, Sayegh R, Stanek JR, Auletta JJ. COVID-19 in immunocompromised patients: a systematic review of cancer, hematopoietic cell and solid organ transplant patients. J Infect (2021) 82(3):329–38. doi: 10.1016/j.jinf.2021.01.022
140. He L, Yao S, Zhang R, Liu M, Hua Z, Zou H, et al. Macrophage activation syndrome in adults: characteristics, outcomes, and therapeutic effectiveness of etoposide-based regimen. Front Immunol (2022) 13:955523. doi: 10.3389/fimmu.2022.955523
141. Palmblad K, Schierbeck H, Sundberg E, Horne A-C, Erlandsson Harris H, Henter J-I, et al. Therapeutic administration of etoposide coincides with reduced systemic HMGB1 levels in macrophage activation syndrome. Mol Med (2021) 27(1):48. doi: 10.1186/s10020-021-00308-0
142. Peter S, Alven S, Maseko RB, Aderibigbe BA. Doxorubicin-based hybrid compounds as potential anticancer agents: a review. Molecules (2022) 27(14):4478. doi: 10.3390/molecules27144478
143. He L, Jin Z, Liu M, Zhang R, Wang Z, Wang Y. Effectiveness of a modified doxorubicin-etoposide-methylprednisolone regimen for the treatment of refractory or relapsed macrophage activation syndrome in adults. Pol Arch Intern Med (2022) 132(3):16226. doi: 10.20452/pamw.16226
144. Hamizi K, Aouidane S, Belaaloui G. Etoposide-based therapy for severe forms of COVID-19. Med Hypotheses. (2020) 142:109826. doi: 10.1016/j.mehy.2020.109826
145. MB M, L B, I A, P D, E I, F C, et al. Etoposide treatment adjunctive to immunosuppressants for critically ill COVID-19 patients. J Infect (2020) 81(3):452–82. doi: 10.1016/j.jinf.2020.06.006
146. Takami A. Possible role of low-dose etoposide therapy for hemophagocytic lymphohistiocytosis by COVID-19. Int J Hematol (2020) 112(1):122–4. doi: 10.1007/s12185-020-02888-9
147. Aoyagi T, Sato Y, Toyama M, Oshima K, Kawakami K, Kaku M. Etoposide and corticosteroid combination therapy improves acute respiratory distress syndrome in mice. Shock (2019) 52(1):83–91. doi: 10.1097/SHK.0000000000001231
148. Imashuku S, Kuriyama K, Teramura T, Ishii E, Kinugawa N, Kato M, et al. Requirement for etoposide in the treatment of Epstein-Barr virus-associated hemophagocytic lymphohistiocytosis. J Clin Oncol (2001) 19(10):2665–73. doi: 10.1200/JCO.2001.19.10.2665
149. Diorio C, Vatsayan A, Talleur AC, Annesley C, Jaroscak JJ, Shalabi H, et al. Anakinra utilization in refractory pediatric CAR T-cell associated toxicities. Blood Advances. (2022) 6(11):3398–403. doi: 10.1182/bloodadvances.2022006983
150. Cavalli G, Dinarello CA. Anakinra therapy for non-cancer inflammatory diseases. Front Pharmacol (2018) 9. doi: 10.3389/fphar.2018.01157
151. Amikishiyev S, Deniz R, Gunver MG, Aghamuradov S, Koca N, Ince B, et al. Pos1216 potential predictors of outcome for anakinra treatment in covid-19 patients with macrophage activation syndrome. Ann Rheumatic Diseases. (2022) 81(Suppl 1):937. doi: 10.1136/annrheumdis-2022-eular.1637
152. Naymagon L. Anakinra for the treatment of adult secondary HLH: a retrospective experience. Int J Hematol (2022) 116(6):947–55. doi: 10.1007/s12185-022-03430-9
153. AlNouwaiser DN, AlMutairi SS, AlRowailey AS. Management of hepatitis a-induced macrophage activation syndrome with anakinra in systemic juvenile idiopathic arthritis: a case report. Cureus (2022) 14(1):e21430. doi: 10.7759/cureus.21430
154. Demir F, Gürler E, Sözeri B. Efficacy of anakinra treatment in pediatric rheumatic diseases: our single-center experience. Arch Rheumatol (2022) 37(3):435–43. doi: 10.46497/ArchRheumatol.2022.8998
155. Huet T, Beaussier H, Voisin O, Jouveshomme S, Dauriat G, Lazareth I, et al. Anakinra for severe forms of COVID-19: a cohort study. Lancet Rheumatol (2020) 2(7):e393–400. doi: 10.1016/S2665-9913(20)30164-8
156. Cavalli G, De Luca G, Campochiaro C, Della-Torre E, Ripa M, Canetti D, et al. Interleukin-1 blockade with high-dose anakinra in patients with COVID-19, acute respiratory distress syndrome, and hyperinflammation: a retrospective cohort study. Lancet Rheumatol (2020) 2(6):e325–e31. doi: 10.1016/S2665-9913(20)30127-2
157. Aouba A, Baldolli A, Geffray L, Verdon R, Bergot E, Martin-Silva N, et al. Targeting the inflammatory cascade with anakinra in moderate to severe COVID-19 pneumonia: case series. Ann Rheum Dis (2020) 79(10):1381–2. doi: 10.1136/annrheumdis-2020-217706
158. Khani E, Shahrabi M, Rezaei H, Pourkarim F, Afsharirad H, Solduzian M. Current evidence on the use of anakinra in COVID-19. Int Immunopharmacol. (2022) 111:109075. doi: 10.1016/j.intimp.2022.109075
159. Ruperto N, Brunner HI, Quartier P, Constantin T, Wulffraat N, Horneff G, et al. Two randomized trials of canakinumab in systemic juvenile idiopathic arthritis. N Engl J Med (2012) 367(25):2396–406. doi: 10.1056/NEJMoa1205099
160. Kostik MM, Isupova EA, Belozerov K, Likhacheva TS, Suspitsin EN, Raupov R, et al. Standard and increased canakinumab dosing to quiet macrophage activation syndrome in children with systemic juvenile idiopathic arthritis. Front Pediatr (2022) 10:894846. doi: 10.3389/fped.2022.894846
161. Gezgin Yıldırım D, Yıldız Yıldırım Ç, Karaçayır N, Esmeray Şenol P, Sunar Yayla EN, Bakkaloğlu SA. Recurrent macrophage activation syndrome due to hyperimmunoglobulin d syndrome: a case-based review. Clin Rheumatol (2022) 42(1):277–83. doi: 10.1007/s10067-022-06384-9
162. Ucciferri C, Auricchio A, Di Nicola M, Potere N, Abbate A, Cipollone F, et al. Canakinumab in a subgroup of patients with COVID-19. Lancet Rheumatol (2020) 2(8):e457–ee8. doi: 10.1016/S2665-9913(20)30167-3
163. Landi L, Ravaglia C, Russo E, Cataleta P, Fusari M, Boschi A, et al. Blockage of interleukin-1β with canakinumab in patients with covid-19. Sci Rep (2020) 10(1):21775. doi: 10.1038/s41598-020-78492-y
164. Koritala T, Pattan V, Tirupathi R, Rabaan AA, Al Mutair A, Alhumaid S, et al. Infection risk with the use of interleukin inhibitors in hospitalized patients with COVID-19: a narrative review. Infez Med (2021) 29(4):495–503. doi: 10.53854/liim-2904-1
165. Sheng CC, Sahoo D, Dugar S, Prada RA, Wang TKM, Abou Hassan OK, et al. Canakinumab to reduce deterioration of cardiac and respiratory function in SARS-CoV-2 associated myocardial injury with heightened inflammation (canakinumab in covid-19 cardiac injury: the three c study). Clin Cardiol (2020) 43(10):1055–63. doi: 10.1002/clc.23451
166. Ao G, Wang Y, Li A, Tran C, Yang Q. The effect of canakinumab on clinical outcomes in patients with COVID-19: a meta-analysis. J Infect (2022) 84(6):834–72. doi: 10.1016/j.jinf.2022.03.011
167. Geng J, Wang F, Huang Z, Chen X, Wang Y. Perspectives on anti-IL-1 inhibitors as potential therapeutic interventions for severe COVID-19. Cytokine (2021) 143:155544. doi: 10.1016/j.cyto.2021.155544
168. Ilowite NT, Prather K, Lokhnygina Y, Schanberg LE, Elder M, Milojevic D, et al. Randomized, double-blind, placebo-controlled trial of the efficacy and safety of rilonacept in the treatment of systemic juvenile idiopathic arthritis. Arthritis Rheumatol (2014) 66(9):2570–9. doi: 10.1002/art.38699
169. Hoffman HM, Throne ML, Amar NJ, Sebai M, Kivitz AJ, Kavanaugh A, et al. Efficacy and safety of rilonacept (interleukin-1 trap) in patients with cryopyrin-associated periodic syndromes: results from two sequential placebo-controlled studies. Arthritis Rheumatol (2008) 58(8):2443–52. doi: 10.1002/art.23687
170. Grom AA, Horne A, De Benedetti F. Macrophage activation syndrome in the era of biologic therapy. Nat Rev Rheumatol (2016) 12(5):259–68. doi: 10.1038/nrrheum.2015.179
171. Yamabe T, Ohmura SI, Uehara K, Naniwa T. Macrophage activation syndrome in patients with adult-onset still’s disease under tocilizumab treatment: a single-center observational study. Mod Rheumatol (2022) 32(1):169–76. doi: 10.1080/14397595.2021.1899565
172. Naniwa T, Uehara K, Yamabe T, Ohmura SI. Reintroduction of tocilizumab elicited macrophage activation syndrome in a patient with adult-onset still’s disease with a previous successful tocilizumab treatment. Mod Rheumatol Case Rep (2021) 5(2):360–4. doi: 10.1080/24725625.2020.1818961
173. Alveyn E, Rutherford A. Op0194 combination anakinra and tocilizumab to treat refractory macrophage activation syndrome triggered by adult-onset still’s disease. Ann Rheumatic Diseases. (2022) 81(Suppl 1):128. doi: 10.1136/annrheumdis-2022-eular.2275
174. Vela D, Vela-Gaxha Z, Rexhepi M, Olloni R, Hyseni V, Nallbani R. Efficacy and safety of tocilizumab versus standard care/placebo in patients with COVID-19; a systematic review and meta-analysis of randomized clinical trials. Br J Clin Pharmacol (2022) 88(5):1955–63. doi: 10.1111/bcp.15124
175. Peng J, Fu M, Mei H, Zheng H, Liang G, She X, et al. Efficacy and secondary infection risk of tocilizumab, sarilumab and anakinra in COVID-19 patients: a systematic review and meta-analysis. Rev Med Virol (2022) 32(3):e2295. doi: 10.1002/rmv.2295
176. Rezaei Tolzali MM, Noori M, Shokri P, Rahmani S, Khanzadeh S, Nejadghaderi SA, et al. Efficacy of tocilizumab in the treatment of COVID-19: an umbrella review. Rev Med Virol (2022) 32(6):e2388. doi: 10.1002/rmv.2388
177. Moreno-Torres V, de Mendoza C, de la Fuente S, Sánchez E, Martínez-Urbistondo M, Herráiz J, et al. Bacterial infections in patients hospitalized with COVID-19. Intern Emerg Med (2022) 17(2):431–8. doi: 10.1007/s11739-021-02824-7
178. Veiga VC, Prats J, Farias DLC, Rosa RG, Dourado LK, Zampieri FG, et al. Effect of tocilizumab on clinical outcomes at 15 days in patients with severe or critical coronavirus disease 2019: randomised controlled trial. Bmj (2021) 372:n84. doi: 10.1136/bmj.n84
179. Marino A, Munafò A, Augello E, Bellanca CM, Bonomo C, Ceccarelli M, et al. Sarilumab administration in COVID-19 patients: literature review and considerations. Infect Dis Rep (2022) 14(3):360–71. doi: 10.3390/idr14030040
180. Zizzo G, Tamburello A, Castelnovo L, Laria A, Mumoli N, Faggioli PM, et al. Immunotherapy of COVID-19: inside and beyond IL-6 signalling. Front Immunol (2022) 13:795315. doi: 10.3389/fimmu.2022.795315
181. Kerget B, Kerget F, Aksakal A, Aşkın S, Sağlam L, Akgün M. Evaluation of alpha defensin, IL-1 receptor antagonist, and IL-18 levels in COVID-19 patients with macrophage activation syndrome and acute respiratory distress syndrome. J Med Virol (2021) 93(4):2090–8. doi: 10.1002/jmv.26589
182. Gabay C, Fautrel B, Rech J, Spertini F, Feist E, Kötter I, et al. Open-label, multicentre, dose-escalating phase II clinical trial on the safety and efficacy of tadekinig alfa (IL-18BP) in adult-onset still’s disease. Ann Rheumatic Diseases. (2018) 77(6):840. doi: 10.1136/annrheumdis-2017-212608
183. Geerlinks AV, Dvorak AM. A case of XIAP deficiency successfully managed with tadekinig Alfa (rhIL-18BP). J Clin Immunol (2022) 42(4):901–3. doi: 10.1007/s10875-022-01236-2
184. Hatterer E, Richard F, Malinge P, Sergé A, Startchick S, Kosco-Vilbois M, et al. P156 investigating the novel mechanism of action for NI-0501, a human interferon gamma monoclonal antibody. Cytokine (2012) 59(3):570. doi: 10.1016/j.cyto.2012.06.257
185. Rainone M, Ngo D, Baird JH, Budde LE, Htut M, Aldoss I, et al. Interferon-γ blockade in CART-cell therapy-associated macrophage activation syndrome/hemophagocytic lymphohistiocytosis. Blood Adv (2022) 7(4):533–6. doi: 10.1182/bloodadvances.2022008256
186. De Benedetti F, Grom A, Brogan P, Bracaglia C, Pardeo M, Marucci G, et al. Op0193 efficacy and safety of emapalumab, an anti-interferon gamma monoclonal antibody, in patients with macrophage activation syndrome (Mas) in systemic juvenile idiopathic arthritis (Sjia) who had failed high-dose glucocorticoids. Ann Rheumatic Diseases. (2022) 81(Suppl 1):128. doi: 10.1136/annrheumdis-2022-eular.803
187. Cure E, Kucuk A, Cure MC. Can emapalumab be life saving for refractory, recurrent, and progressive cytokine storm caused by COVID-19, which is resistant to anakinra, tocilizumab, and janus kinase inhibitors. Indian J Pharmacol (2021) 53(3):226–8. doi: 10.4103/ijp.IJP_615_20
188. Greenmyer JR, Wyatt KD, Milanovich S, Kohorst MA, Ferdjallah A. COVID-19-associated secondary hemophagocytic lymphohistiocytosis requiring hematopoietic cell transplant. EJHaem (2022) 3(3):1025–8. doi: 10.1002/jha2.456
189. Liu JM, Chi J. Is COVID-19-associated cytokine storm distinct from non-COVID-19 secondary hemophagocytic lymphohistiocytosis? Exp Biol Med (Maywood) (2022) 247(4):330–7. doi: 10.1177/15353702211068840
190. Spinelli FR, Meylan F, O’Shea JJ, Gadina M. JAK inhibitors: ten years after. Eur J Immunol (2021) 51(7):1615–27. doi: 10.1002/eji.202048922
191. Keenan C, Nichols KE, Albeituni S. Use of the JAK inhibitor ruxolitinib in the treatment of hemophagocytic lymphohistiocytosis. Front Immunol (2021) 12:614704. doi: 10.3389/fimmu.2021.614704
192. Yeleswaram S, Smith P, Burn T, Covington M, Juvekar A, Li Y, et al. Inhibition of cytokine signaling by ruxolitinib and implications for COVID-19 treatment. Clin Immunol (2020) 218:108517. doi: 10.1016/j.clim.2020.108517
193. Levy O, Apel A, Alhdor H, Mizrachi A, Agmon-Levin N, Koren-Michowitz M, et al. Ruxolitinib for refractory macrophage activation syndrome complicating adult-onset still’s disease. Eur J Rheumatol (2022) 9(4):217–20. doi: 10.5152/eurjrheum.2022.21064
194. Joly J-A, Vallée A, Bourdin B, Bourbonnais S, Patey N, Gaboury L, et al. Combined IFN-γ and JAK inhibition to treat hemophagocytic lymphohistiocytosis in mice. J Allergy Clin Immunol (2022) 151(1):247–59.e7. doi: 10.1016/j.jaci.2022.07.026
195. Triebwasser MP, Barrett DM, Bassiri H, Bunin N, Elgarten C, Freedman J, et al. Combined use of emapalumab and ruxolitinib in a patient with refractory hemophagocytic lymphohistiocytosis was safe and effective. Pediatr Blood Cancer. (2021) 68(7):e29026. doi: 10.1002/pbc.29026
196. Goker Bagca B, Biray Avci C. The potential of JAK/STAT pathway inhibition by ruxolitinib in the treatment of COVID-19. Cytokine Growth Factor Rev (2020) 54:51–61. doi: 10.1016/j.cytogfr.2020.06.013
197. La Rosée F, Bremer HC, Gehrke I, Kehr A, Hochhaus A, Birndt S, et al. The janus kinase 1/2 inhibitor ruxolitinib in COVID-19 with severe systemic hyperinflammation. Leukemia (2020) 34(7):1805–15. doi: 10.1038/s41375-020-0891-0
198. Rein L, Calero K, Shah R, Ojielo C, Hudock KM, Lodhi S, et al. Randomized phase 3 trial of ruxolitinib for COVID-19-Associated acute respiratory distress syndrome. Crit Care Med (2022) 50(12):1701–13. doi: 10.1097/CCM.0000000000005682
199. Han MK, Antila M, Ficker JH, Gordeev I, Guerreros A, Bernus AL, et al. Ruxolitinib in addition to standard of care for the treatment of patients admitted to hospital with COVID-19 (RUXCOVID): a randomised, double-blind, placebo-controlled, phase 3 trial. Lancet Rheumatol (2022) 4(5):e351–e61. doi: 10.1016/S2665-9913(22)00044-3
200. Kalil AC, Patterson TF, Mehta AK, Tomashek KM, Wolfe CR, Ghazaryan V, et al. Baricitinib plus remdesivir for hospitalized adults with covid-19. N Engl J Med (2021) 384(9):795–807. doi: 10.1056/NEJMoa2031994
Keywords: macrophage activation syndrome (MAS), COVID-19, SARS-CoV-2, therapy, cytokine storm syndrome (CSS)s
Citation: Chen S, Zhang C, Chen D, Dong L, Chang T and Tang Z-H (2023) Advances in attractive therapeutic approach for macrophage activation syndrome in COVID-19. Front. Immunol. 14:1200289. doi: 10.3389/fimmu.2023.1200289
Received: 04 April 2023; Accepted: 12 June 2023;
Published: 06 July 2023.
Edited by:
Oksana Boyarchuk, Ternopil State Medical University, UkraineReviewed by:
Halil Yildiz, Cliniques Universitaires Saint-Luc, BelgiumVíctor Moreno-Torres, Hospital Universitario Puerta de Hierro Majadahonda, Spain
Copyright © 2023 Chen, Zhang, Chen, Dong, Chang and Tang. This is an open-access article distributed under the terms of the Creative Commons Attribution License (CC BY). The use, distribution or reproduction in other forums is permitted, provided the original author(s) and the copyright owner(s) are credited and that the original publication in this journal is cited, in accordance with accepted academic practice. No use, distribution or reproduction is permitted which does not comply with these terms.
*Correspondence: Liming Dong, lmdong@tjh.tjmu.edu.cn; Teding Chang, changtd@tjh.tjmu.edu.cn; Zhao-Hui Tang, tangzh@tjh.tjmu.edu.cn