Corrigendum: Fetal-maternal interactions during pregnancy: a ‘three-in-one’ perspective
- 1Center for Reproductive Medicine and Obstetrics and Gynecology, Nanjing Drum Tower Hospital, The Affiliated Hospital of Nanjing University Medical School, Nanjing, China
- 2Reproduction Center, The Third Affiliated Hospital of ZhengZhou University, ZhengZhou, China
A successful human pregnancy requires the maternal immune system to recognize and tolerate the semi-allogeneic fetus, allowing for appropriate trophoblasts invasion and protecting the fetus from invading pathogens. Therefore, maternal immunity is critical for the establishment and maintenance of pregnancy, especially at the maternal-fetal interface. Anatomically, the maternal-fetal interface has both maternally- and fetally- derived cells, including fetal originated trophoblasts and maternal derived immune cells and stromal cells. Besides, a commensal microbiota in the uterus was supposed to aid the unique immunity in pregnancy. The appropriate crosstalk between fetal derived and maternal originated cells and uterine microbiota are critical for normal pregnancy. Dysfunctional maternal-fetal interactions might be associated with the development of pregnancy complications. This review elaborates the latest knowledge on the interactions between trophoblasts and decidual immune cells, highlighting their critical roles in maternal-fetal tolerance and pregnancy development. We also characterize the role of commensal bacteria in promoting pregnancy progression. Furthermore, this review may provide new thought on future basic research and the development of clinical applications for pregnancy complications.
Introduction
A successful human pregnancy requires an intricate balance of maternal tolerance towards the fetus with paternal antigens while providing protection against pathogen invasion (1). This balance is achieved via delicate modulations in the maternal immune system. The placenta is a key mediator that regulates the capabilities of the maternal immune system to maintain the local homeostasis at the implantation site (2). The maternal-fetal interface is considered a complex environment constituted by cells of multiple origins, including fetal originated trophoblasts, and maternal derived decidual stromal cells and decidual immune cells (DICs). The decidual immune cellular part includes several immune cells, including decidual natural killer (dNK) cells, decidual macrophages (DMs), dendritic cells (DCs), and T cells (3). From implantation to delivery, the invading trophoblasts are in close communication with the DICs. Thus, there is an active process of adaptation and modulation from both the maternal and fetal sides, especially at the implantation site. The appropriate communication between the fetal trophoblasts and the maternal DICs contribute to the success of pregnancy.
There is increasing evidence for the presence and importance of ‘commensal’ microbiota in both the pregnant and non-pregnant uterus, although still controversial and not well defined (4, 5). With patten recognition receptors, trophoblasts have the potential to sense and respond to bacterial products (6). The downstream signals may help to induce the tolerogenic immune system, thus allowing embryo implantation and fetus development (7). Furthermore, the interactions between normal bacteria and trophoblasts induce basal expression of type I interferon-β (IFN-β) and downstream interferon stimulating genes (ISGs) in the regulation of maternal immune homeostasis and protection against viral infection (2). Therefore, evidence for a trophoblast-dominated three-way interaction among trophoblasts, decidual immune system, and uterine microbiota is emerging, especially in humans. Exploring the crosstalk at the maternal-fetal interface will help us further understand the development of normal pregnancy and pregnancy complications. Since both human and mice are characterized with highly invasive hemochorial placenta, mice become an important model for investigating human pregnancy mechanism. In the current review, we discuss the crosstalk among trophoblasts, DICs, and commensal microbiota at the maternal-fetal interface in human and mice in order to provide an overview of communication network that promoting normal pregnancy.
The characteristic and functions (including the modulation on trophoblasts) of decidual immune cells
Decidualization is one of the critical events for a successful pregnancy in humans (8, 9). The decidua provides the embryo with adequate nutritional supply and an immunologically privileged site before placentation (2, 10). Apart from the decidualized stromal cells, the decidua is enriched with multiple immune cells, such as NK cells, macrophages and T cells. DICs jointly contribute to the remodeling of decidua immune homeostasis, subsequently promoting the establishment and maintenance of successful pregnancy. However, dysfunction of DICs is closely associated with pregnancy complications, including implantation failure, recurrent miscarriage (RM), pre-eclampsia (PE), and intrauterine fetal growth restriction (FGR). Therefore, maternal immune homeostasis is the key component for placenta homeostasis (2).
Decidual NK cells
NK cells, which make up around 70% of immune cells in the uterine endometrium during the secretory phase and early pregnancy, express a variety of activating and inhibitory receptors and cytokines. Human dNK cells are characterized by a unique CD56brightCD16- phenotype and encoded several unusual receptors, such as lectin-like receptors, integrin subunits and various killer cell Ig-like receptors (11). Single cell RNA sequence analysis revealed the heterogeneity of dNK cells, namely, dNK1, dNK2 and dNK3 subsets (12). In comparison with the other two subsets, dNK1 cells exhibit higher expression of killer cell immunoglobulin-like receptors (KIRs), leukocyte immunoglobulin-like receptor B1 and human leukocyte antigen (HLA) receptors. Furthermore, the dNK1 population contain enzymes involved in glycolysis and cytoplasmic granule and perforin which protect from pathogen infection. The activating killer cell lectin-like receptors C2 (NKG2C), NKG2E and NKG2A are observed on the dNK2 and dNK1 cells, while dNK2 cells show high expression of X-C motif chemokine ligand 1, which suggests a role in mediating recruitment of other immune cells and trophoblasts at the placenta site (13–15). The smallest proportion, dNK3 cells exhibit high expression of chemokine ligand 5 which suggests a role in modulating invasion of trophoblasts. Although the importance of dNK cells in early pregnancy has been widely acknowledged, it remains an important question whether the dNK phenotypic proportions remain constant throughout pregnancy.
Accumulating evidence indicates that dNK cells benefit early pregnancy via several methods (Figure 1). Firstly, several studies indicates that dNK cells promote spiral artery (SA) remodeling by producing angiogenic growth factors in early pregnancy, such as interleukin 8 (IL-8), angiopoietin-1/2, and vascular endothelial growth factor (VEGF), initiating SA transformation (16–18). Besides, dNK cells express a wide range of matrix metalloproteinases (MMPs), which can initiate breakdown of the extracellular matrix (19). To prevent excess extravillous trophoblasts (EVT) invasion, dNK cells can also produce cytokines, such as transforming growth factor β (TGF-β), tumor necrosis factor α (TNF-α), and interferon γ (IFN-γ), which inhibit excessive trophoblast invasion in later stages (20, 21). The critical role of uNK cells on SA remodeling was further confirmed in pregnancy complications (18). Reduced dNK numbers have been found in women with FGR and PE, both of which are pathologically characterized by poor SA remodeling and shallow trophoblast invasion (18). The capacity of dNK cells to promote fetal growth and development has been reported. Specifically, CD49a+dNK cells have been identified as having the ability to promote fetal development by producing growth-promoting factors, such as pleiotrophin and osteoglycin, before placental establishment (22). Secondly, dNK cells play a role as immunomodulators at the maternal-fetal interface by suppressing inflammatory Th17 cells via IFN-γ to promote maternal immune tolerance (23). Thirdly, dNK cells fight against invading pathogens at the placenta, such as Listeria monocytogenes and Zika virus (ZIKV), via expressing the antimicrobial peptide granulysin (24). Thus, dNK cells play a crucial role in modulating placental vascular remodeling and contribute to the immunomodulatory micro-environment facilitating fetal growth during early pregnancy.
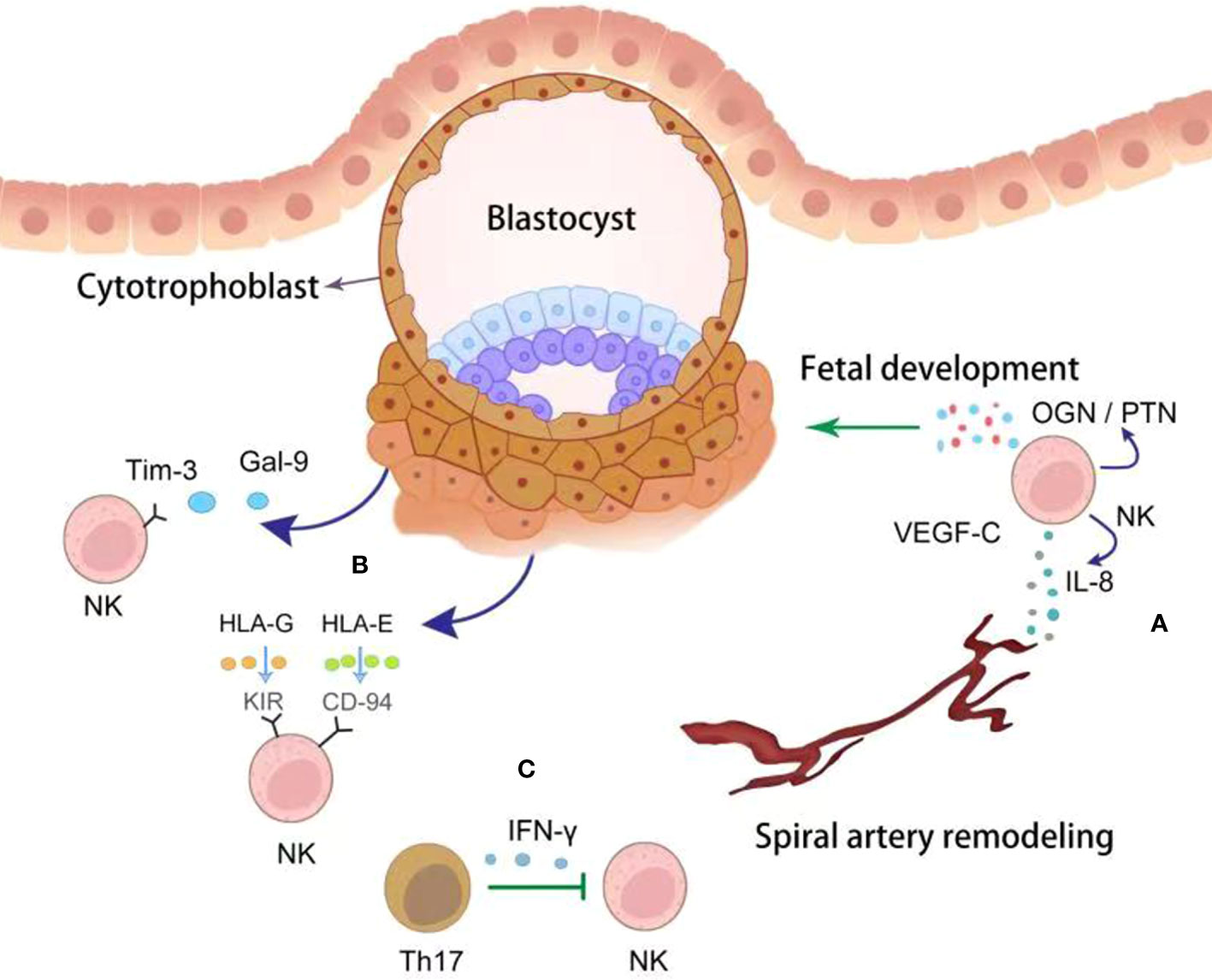
Figure 1 The crosstalk between trophoblasts and dNK cells. NK cells derived cytokines, including VEGF-C, IL-8 and OGN/PTN, promote spiral artery remodeling and fetal development (green point arrow) (A). Correspondingly, trophoblasts educate dNK cells with tolerant phenotype by producing Gal-9 and HLAs (dark blue point arrow) (B). dNK cells were inhibited (green block arrow) by Th17 cells via secreting IFN-γ (C).
Decidual macrophages
During reproduction, decidual macrophages (DMs) are present in the decidua, accounting for 20-30% of the DICs. Based on their surface antigens, cytokine profile and functions, DMs could be classified into classically activated (M1, CD14+CD86+) and alternatively activated (M2, CD14+CD206+) subtypes, although this was still controversial (25). In order to adapt to different stages of fetal development, the phenotype of DM changes dynamically with the change of microenvironment at different gestational ages. During the embryo implantation window, DMs exhibit M1 phenotype (26). With the implantation and invasion of trophoblasts into the endometrium, DMs change into M1/M2 mixed type, which persists until the first trimester and early stage of the second trimester (26). In the second trimester, DMs polarize towards the M2 phenotype with maternal immune tolerance and promote fetal growth until delivery (27). During delivery, the DMs exhibit M1 phenotype (28). Although accumulating studies has proved the role of DMs, their classification and phenotype remain controversial.
DMs play numerous important roles during pregnancy (Figure 2). Firstly, DMs are proposed to perform regulatory/homeostatic functions. Through phagocytosis, DMs perform ‘clean up’ apoptotic trophoblasts, preventing activation of pro-inflammatory pathways (29). They also secrete indoleamine 2,3-dioxygenase (IDO) which catabolizes tryptophan and further inhibits T helper cell activation (30). Secondly, DMs perform an antimicrobial role to protect the fetus against infections via expression of pattern recognition receptors, such as CD206, CD209 and CD163 (31). Thirdly, DMs enhance SAs remodeling and trophoblast invasion via producing MMP9 and VEGF, promoting tissue remodeling and angiogenesis (32). It has also been reported that DMs regulate vascular remodeling by secreting placental growth factor and their receptor, FMS-like tyrosine kinase (33, 34). Compared with M1 subsets, M2 macrophages have higher angiogenic potential. M2 macrophages produce a higher level of granulocyte colony stimulating factor (G-CSF) and were able to increase the expression of G-CSFR (35). Macrophage-derived G-CSF promotes the epithelial-to-mesenchymal transition, migration and invasion of trophoblasts via activating PI3K/Akt/Erk1/2 signaling pathway (36). The role of M2 macrophages in implantation was further proved using M2 deleted mice by targeting CD206. In the pregnant M2 deleted mice, lower implantation number and accelerated epithelial cell proliferation, and reduced leukemic inhibitory factor were observed. M2 depleted pregnant mice showed upregulated uterine Wnt/β-catenin signals. Thus, CD206+ M2-like macrophages may be essential for embryo implantation through the regulation of endometrial proliferation via Wnt/β-catenin signaling (37). Furthermore, activated DM-derived IL-1β facilitates trophoblast invasion by degrading the extracellular matrix (38, 39). In addition, DM promoted pregnancy via modulating other immune cells. For example, DMs with expression of T cell immunoglobulin domain and mucin domain-3 (Tim-3) induced CD4+T cells towards T helper 2 (Th2) and regulatory T (Treg) bias via CD132 (40).
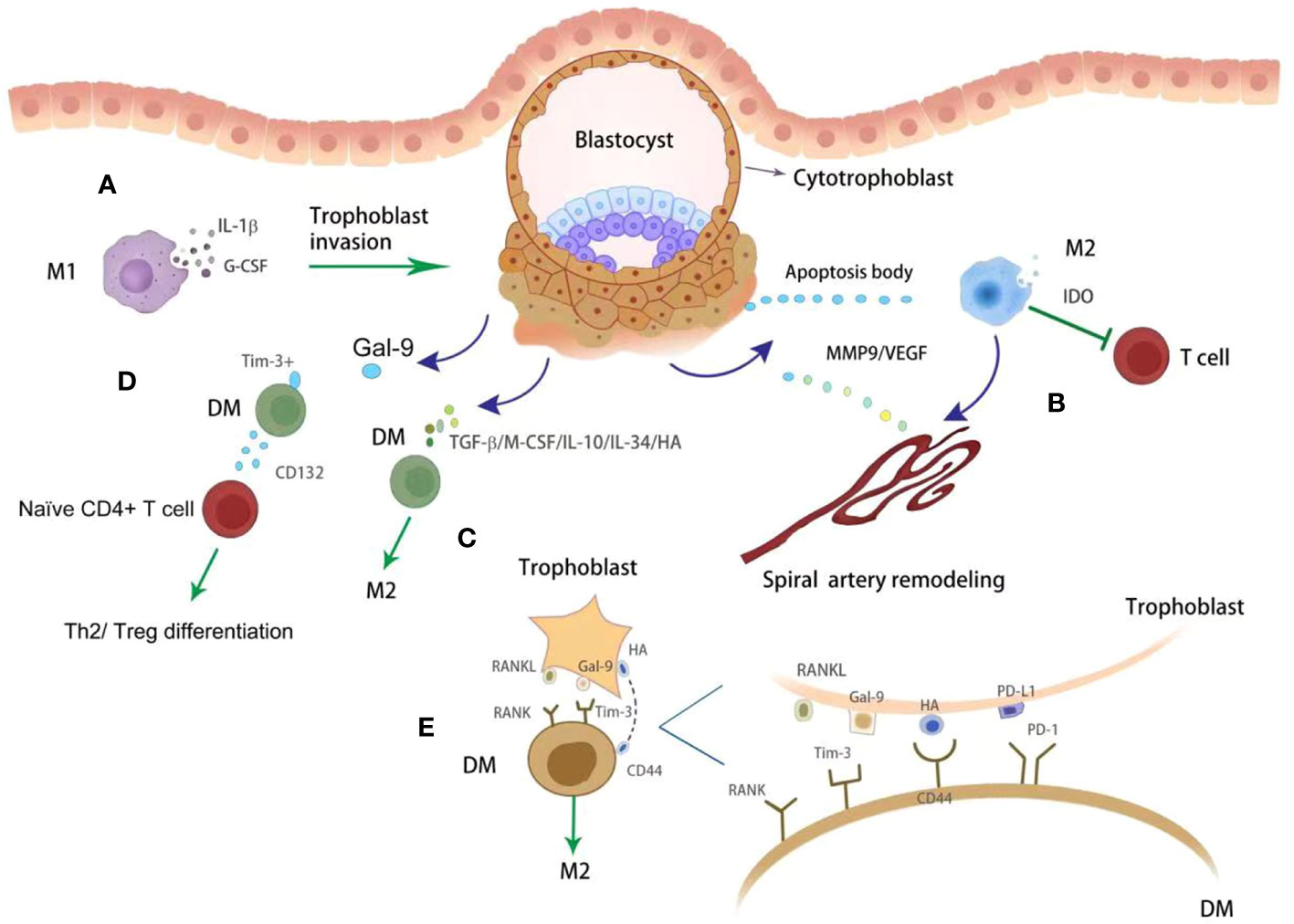
Figure 2 The crosstalk between trophoblasts and DMs. DMs with M1 phenotypes promote trophoblasts invasion via releasing IL-1β and G-CSF (A). With phagocytosing apoptotic bodies from placenta, DMs show M2 phenotype which further inhibit T cell activity via producing IDO (green block arrow) (B). Apart from immune modulation, DMs also promote artery remodeling by producing MMP9 and VEGF (dark blue point arrow) (B). In addition, DMs were regulated by trophoblasts derived various cytokines (dark blue point arrow), such as TGF-β, M-CSF, IL-10, IL-34 and HA, toward M2 phenotype (green point arrow) (C). The immune checkpoints were also observed functional on modulating DMs immunity. Tim3+ DMs promote Th2 and Treg differentiation (green point arrow) via producing CD132 (D). Gal-9/PD-L1/RANKL/HA were observed on trophoblasts, which further promote DMs polarization toward M2 phenotype (green point arrow) by binding with specific receptors (E).
Decidual T cells
Compared with dNK and DMs, T cells constitute a smaller proportion (10-20%) of DICs, but are important for implantation and placentation. Although multiple adaptive immune cell subsets play a role throughout pregnancy, the key cell mediators are Treg cells and Th cells. At the maternal-fetal interface, approximately 10-30% of CD4+T cells are Treg cells, which show immunomodulatory effects on immunity through their signature transcription factor Forkhead box protein 3 (Foxp3) (41, 42). However, effector T (Teff) cells could be classified into different subsets based on their functional phenotypes and cytokine production. For example, Th1 and Th17 cells are pro-inflammatory subsets while Th2 cells are anti-inflammatory during pregnancy (43). Decidual Th1 cells are moderately enriched at the maternal-fetal interface, while the proportions of Th2 and Th17 cells are similar. This signifies the presence of a Th1/Th2/Th17/Treg paradigm in the decidua. Recently, more attention has been paid to the Treg/Th17 balance in the field of reproductive immunity. CD4+CD25+Foxp3+ Treg cells are essential for the anti-inflammatory transition accompanying implantation and placentation. Without the presence of Treg cells, the allogeneic fetuses were uniformly rejected, which was most vulnerable in the pre- and peri-implantation phase (44, 45). Pregnant Rag1(-/-) mice with T cell-deficient are more susceptible to LPS induced PTD, which could be alleviated by adoptive transplantation of Foxp3+ cells (46).
Treg cells facilitate implantation and placental development via at least three mechanisms (Figure 3). Firstly, Treg cells have a profoundly inhibitory effects on effector CD4+T and CD8+T cells (47, 48). Uncontrolled Teff cells had adverse effects on placental development in an antigen-independent manner, possibly through the release of inflammatory cytokine and antigen-dependent trophoblast cytotoxicity (49). Decidual Treg cells secret TGF-β and IL-10 and express CD25, cytotoxic T lymphocyte-associated antigen-4 (CTLA-4), and programmed cell death ligand 1 (PD-L1), all of which are signature mediators of Treg inhibition and may contribute to Teff cell constraint in early pregnancy (43, 50–52). Secondly, Treg cells regulate other immune cells in the decidual environment, facilitating anti-inflammatory and tolerogenic phenotypes in M2 macrophages and tolerogenic DCs via TGF-β and IL-10, and CTLA-4. Treg cells may be important modulators for the phenotype and function of NK cells during implantation by controlling the release of IL-15 release from DCs and inhibiting NK cytolytic activity (53). These coordinated interactions enable Treg cells to control the inflammatory response and oxidative stress associated with trophoblast invasion. Thirdly, Treg cells are a critical modulator of SA remodeling, which is essential for normal placental development. Treg-deficient pregnant mice exhibit consistent damages in uterine SA modification, decreased placental blood flow and FGR (54, 55). Acute depletion of Foxp3+ Treg cells in first trimester of pregnancy leads to uterine artery dysfunction in later pregnancy, which is closely associated with increased production of vasoconstrictor endothelin-1 (55). Poor trophoblast invasion and SA transformation failure were also seen in mice after depletion of Treg cells (56). Therefore, Treg action is indispensable for successful pregnancy.
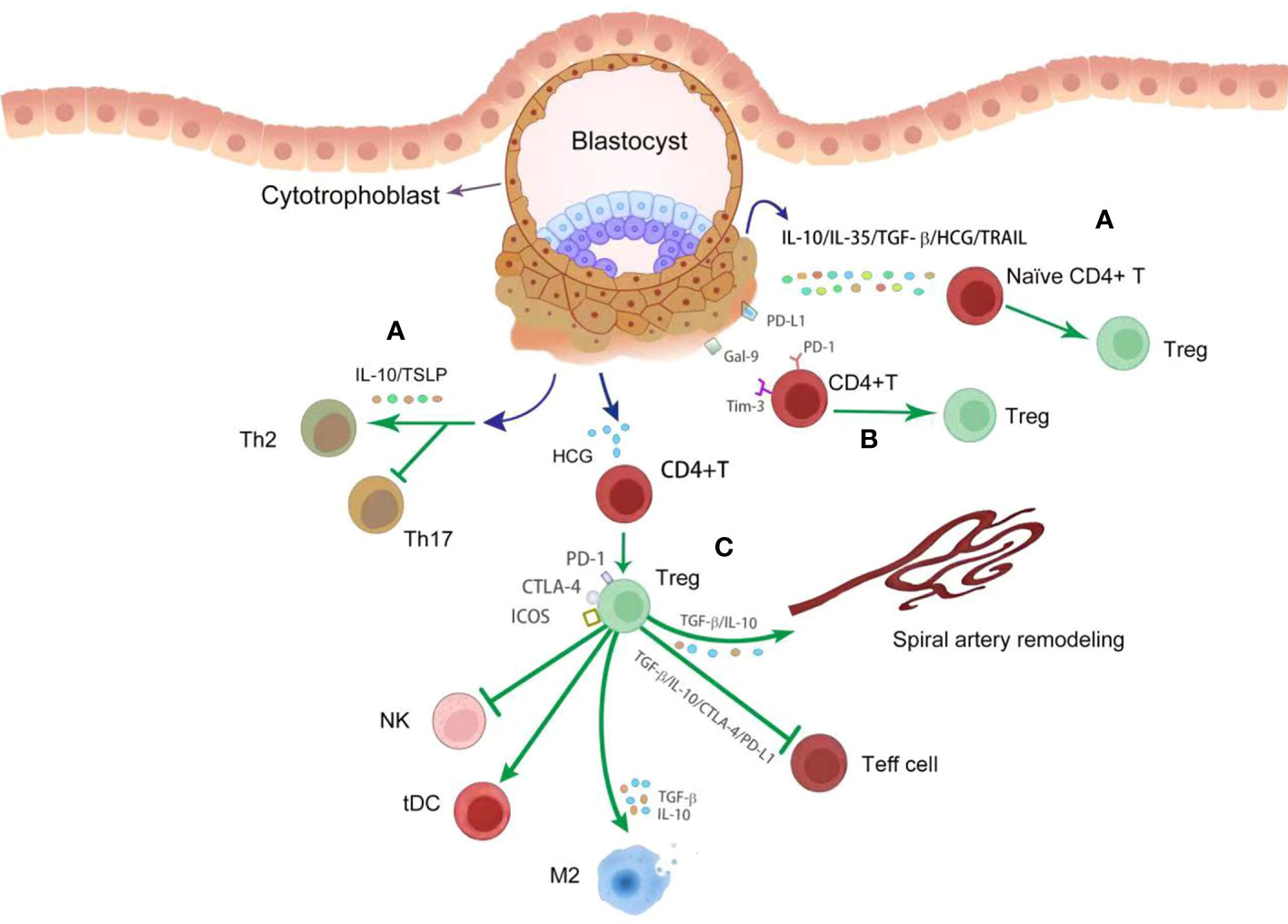
Figure 3 The crosstalk between trophoblasts and decidual T cells. During placentation, trophoblasts derived various cytokines (dark bule point arrow) promote Treg differentiation (green point arrow) and inhibit Th17 response to control the inflammatory response (A). These cytokines include IL-10, IL-35, TGF-β, HCG, TSLP and TRAIL. With engagement with Gal-9 and PD-L1 from trophoblasts, Treg differentiation (green point arrow) was enhanced (B). By producing anti-inflammatory cytokines and immune modulatory proteins, such as TGF-β, IL-10, PD-L1 and CTLA-4, Treg cells profoundly inhibit Teff cell response and NK activation (green block arrow), and promote the differentiation of tDC and M2 macrophages (green point arrow) (C). In addition, Treg cells promote spiral artery remodeling (green point arrow) via TGF-β and IL-10 (C).
Apart from modulatory effects, Treg cells show significant phenotypic plasticity, as they can transdifferentiate into potentially deleterous Th17 cells in response to certain environmental signals. This was also observed in pre-eclamptic women in the third trimester (51). The ability of Treg cells to transition between pro- and anti-inflammatory cell types provides a therapeutic opportunity for inflammatory pregnancy complications. PD-L1 was one of the regulators promoting Th17 cells or Foxp3+IL-17+ cells transdifferentiated into Treg cells, providing evidence supporting the hypothesis that PD-1 might be an ideal therapeutic target in pregnancy complications (51).
The commensal microbiota
Although still controversial and not clearly defined, there are a growing number of studies proving the evidence of the presence and importance of a commensal microbiome in both the pregnant and non-pregnant uterus (4). Human placenta has a unique microbial niche, which is composed of nonpathogenic symbiotic microbiota from the Firmicutes, Tenericutes, Proteobacteria, Bacteroidetes, and Fusobacteria phyla (5). Overall, the placental microbiome is most similar with the human oral microbiome (5, 57). In vivo studies have shown that the normal maternal microbiota may help induce immune tolerance, allowing receptivity and preventing rejection of the fetal-placental unit (7). However, abnormal maternal microbiota might be associated with pregnancy loss. The increase in Ureaplasma species in uterine endometrium microbiota of women with recurrent pregnancy loss, were risks of miscarriage and preterm delivery in subsequent pregnancies with normal chromosome karyotype (58). During peri-implantation phase, the presence of Lactobacillus-dominated endometrium microbiota (>90% Lactobacillus spp.) was associated with significantly increased implantation, pregnancy, and live birth rates compared with those with non-Lactobacillus-dominated microbiota (<90% Lactobacillus spp. with >10% of other bacteria) in endometrium (59). Pathogenic bacteria, such as Streptococcus, Staphylococcus, Neisseria, and Klebsiella were most frequently observed in the endometrium of patients with repeated implantation failure (60). Thus, the maternal endometrium microbiota is closely associated with pregnancy development. Keeping endometrium microbiota homeostasis might contribute to embryo implantation and pregnancy development.
Immune cells and trophoblasts interactions
Trophoblast invasion and vascular remodeling are the two most crucial events during placental development. During early pregnancy, decidual immune cells showing pro-inflammatory profile were closely associated with placentation, by releasing soluble cytokines and cell-to-cell dependent interactions to facilitate trophoblast invasion and vascular remodeling (61). Reduced trophoblast invasion and vascular transformation can result in poor placental perfusion, potentially leading to pregnancy disorders, such as recurrent miscarriage (RM), FGR and PE. To accept the semi-allogenic fetus, the maternal immune system will establish an immunological tolerance pre-dominated state, while preserving immune response against infection. Interestingly, the shift of immune condition from pro-inflammatory to immune tolerance along with implantation and placentation is modulated by trophoblasts of fetal origin. Therefore, the fetus might be not passively accepted by his mother, but actively educates maternal immune cells switch to anti-inflammatory profile. However, excessive immune response is considered the leading cause of the unexplained pregnancy loss (62). Since the modulation of DICs on trophoblasts have been showed in the former section, we only review the modulatory effects of trophoblasts on DICs.
The modulation of trophoblasts on dNK cells
Trophoblast is the only cell type at the maternal-fetal interface that carries paternal antigens and express a unique repertoire of MHC ligands. dNK cells are modulated by trophoblasts (Figure 1). HLA-C, -E, and -G are MHC ligands expressed by EVT, being the targets of dNK cells. As we have mentioned, dNK cells display high level of the inhibitory receptors KIRs, which engage with HLA-G to inhibit NK cytotoxicity (63). Another non-classical HLA class I molecule, HLA-E, is also expressed by trophoblasts and regulate dNK cytotoxicity through direct interaction with the inhibitory receptors CD94/NKG2A (64, 65). Asymmetric growth restriction and abnormal brain development were observed in NKG2A-deficient mice (65). The non-functional HLA-B→HLA-E→NKG2A pathway exposes women to greater pre-eclampsia risk (65). Additionally, HLA-C attenuates NK cell cytotoxicity by interacting with KIRs expressed on NK cells (66). At the maternal-fetal interface, the trophoblast-derived key metabolic enzyme, IDO, degrades tryptophan and has been shown to reduce the cytotoxicity of dNK-cells by downregulating expression of NKp46 and NKG2D, as well as the cytotoxicity of peripheral NK cells (67). These findings demonstrate the important role of IDO in the maintenance of a normal pregnancy (67). Trophoblasts autophagy inhibits the killing capacity of NK cell through insulin growth factor (IGF)-2 (68). Low level of trophoblastic autophagy leads to the increased production of IGF-2, which in turn leads to the high cytotoxicity of NK cells attacking the normal cells in RM patients (68). As a recently defined immune checkpoint, Tim-3 exhibits modulatory effects on T cell immunity (67). Interestingly, Tim-3 was also detected on dNK cells (69). By engagement with Tim-3, galectin-9 (Gal-9) produced by human trophoblast induce the transformation of peripheral NK cells into a dNK like phenotype (70). Furthermore, after ligation with Gal-9 on trophoblasts, Tim-3+dNK cells exhibited inhibited cytotoxicity due to decreased production of perforin in comparison with Tim-3- dNK cells (70). Therefore, further exploration on the mechanisms that modulate the functions and phenotype of dNK cells during pregnancy may contribute to the development of novel treatments to prevent pregnancy failure.
The crosstalk between trophoblasts and DM in shifting towards M2 phenotype
DMs play an active role in several events throughout pregnancy, including embryo implantation, placentation, fetus development, and parturition. CD14+ DM were observed closely to the EVTs. Correspondingly, DMs are also regulated by trophoblasts (Figure 2). Trophoblasts secreted soluble factors, such as TGF-β, macrophage colony stimulating factor and IL-10 (71). These factors induce the differentiation of monocytes into M2-like macrophages, which are characterized by the expression of CD163, CD206 and CD209, and are known for their enhanced phagocytic capacity (72, 73). Furthermore, trophoblasts derived IL-34 polarizes macrophages into a decidual-like phenotype in vitro (74). Pregnancy-specific glycoprotein (PSG) are released by the placenta and are the most abundant fetal proteins in the maternal blood at the end of pregnancy (75). The expression of VEGF was upregulated by PSGs both in RAW 264.7 cell line and in human peripheral monocytes derived macrophages (76). These findings suggest that placenta derived PSGs may play important roles in vascular modification by regulating macrophages. In addition to cytokines and chemokines, trophoblasts secret hyaluronic acid, the most abundant component of the extracellular matrix in all mammalian tissues (77), which can induce M2 polarization of macrophages at the maternal-fetal interface by interacting with CD44 and activating the downstream PI3K/AKT-STAT-3/STAT-6 signal pathway (77).
Tim-3 is constitutively expressed on macrophages (78), and Gal-9, was observed on trophoblasts during pregnancy (79). The ligation of Tim-3 and Gal-9 has been shown to modulate macrophage function at the maternal-fetal interface (80). Blocking Tim-3 signaling by Tim-3 blocking antibodies in pregnant mice inhibits the phagocytic potential of uterine macrophages resulting in a building up of apoptotic bodies at the uteroplacental interface that elicits a local immune response (81). Another immune checkpoint PD-1/PD-L1 axis also plays a crucial role in modulating immune cell homeostasis. PD-L1 was observed on the trophoblasts, while decreased expression was confirmed in women with pregnancy complications such as RM (25). The engagement of PD-L1 and PD-1 promoted macrophages polarization towards M2 phenotype (82). HLA-G was highly expressed in EVTs and its soluble form can reduce the expression of CD86 and increase the expression of CD163 in macrophages (83). Soluble HLA-G5 polarized macrophages increased the production of IL-6 and C-X-C motif ligand 1, which further enhance trophoblast invasion (83). Receptor activator of nuclear factor κB (NF-κB) ligand (RANKL) and its TNF-family receptor RANK are involved in multiple activities. With the expression and secretion of RANKL, trophoblasts could promote macrophage different into M2 subtype via AKT/STAT6-Jmjd3/IRF4 signaling pathway (84). However, low levels of RANKL in trophoblasts and RANK on DMs was observed in women with miscarriage (84). This was further demonstrated by using RANKL-/- mice, which exhibited macrophage dysfunction and increased fetal loss (84). However, adoptive transfer of RANK+ macrophages alleviate the fetal loss induced by macrophage depletion in mice (84). Metabolic immunity is a hot topic in tumor macrophage immunity. The research on DM and metabolism is emerging. Lactic acid (LA) is produced from glucose through glycolysis. Gao et al. (85) found that trophoblast-derived LA orchestrates DM polarization through SRC/LDHA signaling in human early pregnancy. Under normoxic and anoxic conditions, M2 or M1 macrophage polarization is triggered by LA via oxidative phosphorylation and glycolysis, respectively. Blocking the intake of LA could improve pregnancy outcome in the animal model, indicating that it is a potential therapeutic target for pregnancy loss in the future (85). However, the association between amino acid and lipid metabolism and DM polarization is still unknown.
In recent years, extracellular vesicles (EVs) have been shown to be one critical medium in maternal-fetal communication (86). EVs include several types, such as micro-vesicles, exosomes and apoptotic bodies, which could be released by trophoblasts and macrophages (86, 87). The concentration of EVs in peripheral blood is approximately 5-fold higher in pregnant women compared with the non-pregnant women (88). The exosomes produced by placental trophoblasts modulate monocytes to produce G-CSF, IL-1β, IL-6 and TNF-α, which further contribute to embryo implantation, stromal remodeling and angiogenesis (89). DM-derived EVs deliver miR-153-3p, miR-146a-5p and mi-R146b-5p inhibiting proliferation, migration and invasion of trophoblasts, associated with the development of unexplained RM (90, 91). However, more studies are required to further clarify the role of EVs during human pregnancy.
The crosstalk between trophoblasts and T cells in promoting Treg function
At the implantation site, placental trophoblasts are considered to be ‘foreign cells’ with paternal antigen (92) invading deeply and actively modulating T cell homeostasis (93). Emerging evidence proves that trophoblasts can induce Treg tolerance by producing various immune mediators, including chemokines and cytokines, hormones, EVs and immune checkpoint ligands (Figure 3). Trophoblast cells also promote T cell immunity toward Th2 bias and inhibit Th17 cells through producing cytokines such as IL-10 and thymic stromal lymphopoietins (94). Shifting Th1/Th2/Th17/Treg paradigm toward Treg dominance at the implantation site is critical for establishing a tolerant microenvironment (95). Several studies found that trophoblast-derived cytokines, such as IL-10, IL-35, TGF-β, HCG and TNF-related apoptosis-inducing ligand, enhanced Foxp3 expression in naive CD4+T cells and increased the production of Treg specific cytokines (73, 96–99). Furthermore, trophoblasts derived HCG promoted Treg differentiation and the expression of immune checkpoints, including PD-1 and CTLA-4, and transdifferentiate Th17 cells into Th subsets with an anti-inflammatory profile (99). Immune checkpoint molecules are coinhibitory receptors observed on surface of T cells, like PD-1, Tim-3 and CTLA-4 (79). One of the most widely explored is the PD-1/PD-L1 axis in human pregnancy (79). PD-L1 is present in the placenta throughout gestation (79). The engagement of PD-1 and PD-L1 leads to decreased production of proinflammatory cytokines from activated CD4+T cells when cocultured with trophoblasts (100). PD-1 was an important mediator in Treg-induced fetal protection in the CBA/J×DBA/2 murine model (101). Blocking PD-L1 via PD-L1 mAb administration in animal model significantly increases fetal loss, which were associated with Treg deficiency at the maternal-fetal interface (102). Furthermore, trophoblasts originated IL-27 and Gal-9 activate the Tim-3 signaling pathway in CD4+T cells and Treg cells, thus promoting accumulation of Tim-3+Treg cells (103). The abnormal expression of IL-27 and Gal-9 is associated with decreased Treg proportion and impaired immune tolerance in pregnancy loss patients (103). The findings on immune checkpoint molecules modulating T cell immunity during pregnancy indicate their potential for immunotherapy.
Commensal bacteria and trophoblasts interactions
Trophoblasts constitutively express pattern recognition receptors, including toll like receptor (TLRs) and NOD-like receptors, which can recognize damage associated molecular patterns that are produced by damaged tissues and dying cells (104, 105), and pathogen-associated molecular pattens from bacteria, viruses and other microorganisms (106–109). Instead of inducing the classical NF-κB mediated inflammatory response, lipopolysaccharide (LPS) engagement with TLR4 in trophoblast cells resulted in the production of type 1 IFNs via the MyD88-independent pathway (110). The interaction of LPS and TLR4 leads to the phosphorylation of tank-binding kinase (TBK) and interferon regulatory factor 3 (IRF3), inducing IFN-β expression in trophoblasts (110). Therefore, these findings highlight the potential role of endometrium normal microbiota on maintaining constitutive expression of IFN-β via the TLR4/TBK3/IRF3 signaling in trophoblasts.
As one of the IFN family, IFN-β is capable of inducing an anti-microbial response, modulating innate immunity, and inducing the activation of the adaptive immune system (111). By binding with IFNARs, basal IFN-β promotes the expression of ISGs with immune modulatory activation, as well as provide immune protection against invading pathogens (2). Therefore, the basal IFN-β from trophoblasts is critical for immune homeostasis and preventing virus towards the fetus. However, the effective of IFN-β is dose dependent. Excessive IFN-β responses have harmful effects and facilitate the development of autoimmune diseases (112). Therefore, proper regulation of the expression and activation of type I IFNs is essential for tissue homeostasis (2).
During early pregnancy, trophoblasts produce multiple ISGs in the presence of IFN-β (110). These ISGs regulate immune cell functions and promote immune homeostasis at the implantation site by inducing tolerance to paternal antigens and protecting against pathogen infections. One of the key roles of the placenta is to prevent vertical transmission of the virus, which may be fetal to the fetus and may also lead to serious developmental problems (2). With the infection of ZIKV, trophoblasts can strongly induce IFN-β signaling, which in turn results in the production of several ISGs against virus (110), such as ISG20. The recent publication proved that ISG20 is critical for protecting placenta against ZIKV replication (113). The essential role of IFN-β and ISGs in virus defense was further demonstrated by ifnar-/- pregnant mice, which showed increased viral titers in ifnar-/- placenta and fetus when challenged by the viruses (such as ZIKV, MHV68 and HSV-2) (114). When challenged with LPS, wild-type mice exhibited normal pregnancy outcomes. In contrast, IFN receptor-deficient mice were more sensitive to LPS and experienced preterm birth within 24 h. IFN receptor deficiency may lead to increased production of pro-inflammatory cytokines, such as TNF-α, IL-6 and IL-8, and the development of preterm birth (115). Thus, the deficiency of IFN-β signaling resulted in uncontrolled placental-fetal viral infection and maternal mortality.
During pregnancy, ISGs are critical not only on antiviral infection, but also on modulating immune cell function (116). Trophoblasts modulate macrophage polarization by producing ISGs, such as PD-L1 (82). Trophoblast-derived PD-L1, which is modulated by IFN-β, contributes to macrophage polarization towards M2 phenotype (56). Nicotinamide phosphoribosyltransferase, another trophoblast-derived ISGs, is known as a critical modulator of macrophages polarization and migration (117). Despite previous research, the functions of ISGs on modulating immune cell functions during pregnancy remains unclear and requires further exploration.
Commensal microbiota and DICs interactions
The crosstalk between DICs and commensal microbiota has rarely been reported. Commensal bacteria might contribute to homeostasis by modulating the innate and adaptive immune system in the endometrium (118). Since classical antigen presenting cells (APCs) are abundantly in the endometrium, such as macrophages and DCs, these cells should have close interactions with the commensal bacteria. Their communications might subsequently promote pregnancy development by two methods: a, macrophages and DCs promote commensal microbiota homeostasis; b, endometrium bacteria could be recognized by macrophages and DCs and further induce adaptive immune tolerance towards the fetus. Further, the downstream effects of triggering the immune system at the maternal-fetal interface by bacteria is the activation of uterine NK cells and the development of specific subsets of T-cells, characterized by high number of Treg cells and lower number of Th17 cells (118). How the microbiota-immune cells interactions affect the maternal immune response during implantation and pregnancy is not clearly understood.
Immunotherapy of RM
The harmonious crosstalk among fetal derived trophoblasts, maternal derived DICs, and the commensal microbiota contribute to the maternal-fetal immune tolerance. Although the interactions between DICs and commensal microbiota has rarely been reported. Their crosstalk should contribute to the establishment of maternal tolerance and pregnancy development. Abnormal dialog between the mother and fetus can lead to a deficiency of maternal tolerance and subsequently result in pregnancy failure. Notably, RM is one of the big challenges in reproductive medicine and placed a heavy burden on patients and their families (119). Therefore, effective therapies are urgently required.
The maternal-fetal interactions during pregnancy have significant clinical implications for the therapy of RM. In the past decades, various immunotherapies have emerged, including lymphocyte immunotherapy, intravenous immunoglobulin therapy, intralipid and TNF-α inhibitor (120–123). However, the effectiveness of the current immunotherapies is still controversial. All the novel therapies should be processed based on clear evidence of pregnancy development mechanisms. Until now, great efforts had been done trying to characterize the alloimmune immunity at the maternal-fetal interface. And some new targets had been proposed, such as PD-1, Tim-3 and IFN-β. PD-1 and Tim-3 are immune checkpoint inhibitors (ICIs), the effective therapeutic strategy to restore antitumor immunity by targeting immune checkpoint molecules (124). ICI strategies have shown remarkable success in clinical studies of several malignant tumors (125, 126). Administration of agonists to these ICIs might prevent the development and improve the outcome of pregnancy complications. However, the reproductive safety must be carefully considered. Fundamentally, it is critical to focus on the interactions among trophoblasts, DICs, and microbiota during pregnancy to reveal the mechanisms of normal pregnancy and pave the way for the promising immunotherapy of RM.
Conclusion and perspectives
The crosstalk among DICs, trophoblasts, and commensal microbiota are critical to ensuring maternal immune tolerance to the semi-allogenic fetus and effective immune protection against pathogens, ultimately safeguarding the smooth progression of pregnancy until successful delivery, especially in the highly invasive hemochorial placenta as seen in humans and mice. However, abnormal conversation among trophoblasts, DICs and microbiota might directly lead to the development of pregnancy complications, such as RM. Therefore, further exploration on the three-way interaction among trophoblasts, decidual immune system, and uterine microbiota will provide fundamental scientific basis for the development of immunotherapies for pregnancy complications. Although efforts had been done, numerous questions remain: (і) Since trophoblasts, DICs, and endometrium microbiota are communicated with each other, which one plays the dominant role? (ii) As pregnancy is characterized by multiple stages, which crosstalk plays a fundamental role at specific stages? (iii) Can microbiota be developed to modulate maternal-fetal tolerance?
Author contributions
YZ wrote and revised the manuscript. ZL wrote and designed the figures. HS revised the manuscript. All authors contributed to the article and approved the submitted version.
Funding
This study was supported by the National Nature Science Foundation of China (No. 82001637), Natural Science Foundation of Jiangsu Province (No. BK20200130) and Medical Science and Technology project of Henan Province (LHGJ20210468).
Conflict of interest
The authors declare that the research was conducted in the absence of any commercial or financial relationships that could be construed as a potential conflict of interest.
Publisher’s note
All claims expressed in this article are solely those of the authors and do not necessarily represent those of their affiliated organizations, or those of the publisher, the editors and the reviewers. Any product that may be evaluated in this article, or claim that may be made by its manufacturer, is not guaranteed or endorsed by the publisher.
References
1. Mor G, Aldo P, Alvero AB. The unique immunological and microbial aspects of pregnancy. Nat Rev Immunol (2017) 17:469–82. doi: 10.1038/nri.2017.64
2. Ding J, Maxwell A, Adzibolosu N, Hu A, You Y, Liao A, et al. Mechanisms of immune regulation by the placenta: role of type I interferon and interferon-stimulated genes signaling during pregnancy. Immunol Rev (2022) 308:9–24. doi: 10.1111/imr.13077
3. Gomez-Lopez N, Guilbert LJ, Olson DM. Invasion of the leukocytes into the fetal-maternal interface during pregnancy. J Leukoc Biol (2010) 88:625–33. doi: 10.1189/jlb.1209796
4. Cao B, Mysorekar IU. Intracellular bacteria in placental basal plate localize to extravillous trophoblasts. Placenta (2014) 35:139–42. doi: 10.1016/j.placenta.2013.12.007
5. Aagaard K, Ma J, Antony KM, Ganu R, Petrosino J, Versalovic J. The placenta harbors a unique microbiome. Sci Transl Med (2014) 6:237ra265. doi: 10.1126/scitranslmed.3008599
6. Abrahams VM, Mor G. Toll-like receptors and their role in the trophoblast. Placenta (2005) 26:540–7. doi: 10.1016/j.placenta.2004.08.010
7. Adams Waldorf KM, Rubens CE, Gravett MG. Use of nonhuman primate models to investigate mechanisms of infection-associated preterm birth. BJOG (2011) 118:136–44. doi: 10.1111/j.1471-0528.2010.02728.x
8. Coulam C. What about superfertility, decidualization, and natural selection? J Assit Reprod Genet (2016) 33:577–80. doi: 10.1007/s10815-016-0658-8
9. Schatz F, Guzeloglu-Kayisli O, Arlier S, Kayisli UA, Lockwood CJ. The role of decidual cells in uterine hemostasis, menstruation, inflammation, adverse pregnancy outcomes and abnormal uterine bleeding. Hum Reprod Update (2016) 22:497–515. doi: 10.1093/humupd/dmw004
10. Chi RA, Wang T, Adams N, Wu SP, Young SL, Spencer TE, et al. Human endometrial transcriptome and progesterone receptor cistrome reveal important pathways and epithelial regulators. J Clin Endocrinol Metab (2020) 105:1419–39. doi: 10.1210/clinem/dgz117
11. Koopman LA, Kopcow HD, Rybalov B, Boyson JL, Orange JS, Schatz F, et al. Human decidual natural killer clels are a unique NK clel subset with immunomodulatory potential. J Exp Med (2003) 198:1201–12. doi: 10.1084/jem.20030305
12. Vento-Tormo R, Efremova M, Botting RA, Turco MY, Vento-Tormo M, Meyer KB, et al. Single-cell reconstruction of the early maternal-fetal interface in humans. Nature (2018) 563:347–53. doi: 10.1038/s41586-018-0698-6
13. Morandi F, Pistoia V. Interactions between HLA-G and HLA-e in physiological and pathological conditions. Front Immunol (2014) 5:394. doi: 10.3389/fimmu.2014.00394
14. Böttcher JP, Bonavita E, Chakravarty P, Blees H, Cabeza-Cabrerizo M, Sammicheli S, et al. NK cells stimulate recruitment of cdc 1 into the tumor microenvironment promoting cancer immune control. Cell (2018) 172:1022–37.e14. doi: 10.1016/j.cell.2018.01.004
15. Sato Y, Higuchi T, Yoshioka S, Tatsumi K, Fujiwara H, Fujii S. Trophoblasts acquire a chemokine receptor, CCR1, as they differentiate towards invasive phenotype. Development (2003) 130:5519–32. doi: 10.1242/dev.00729
16. Lash GE, Schiessl B, Kirkley M, Innes BA, Cooper A, Searle RF, et al. Expression of angiogenic growth factors by uterine natural killer cells during early pregnancy. J Leukoc Biol (2006) 80:572–80. doi: 10.1189/jlb.0406250
17. Schiessl B, Innes BA, Bulmer JN, Otun HA, Chadwick TJ, Robson SC, et al. Localization of angiogenic growth factors and their receptors in the human placental bed throughout normal human pregnancy. Placenta (2009) 30:79–87. doi: 10.1016/j.placenta.2008.10.004
18. Wallace AE, Fraser R, Cartwright JE. Extravillous trophoblast and decidual natural killer cells: a remodelling partnership. Hum Reprod Update (2012) 18:458–71. doi: 10.1093/humupd/dms015
19. Choudhury RH, Dunk CE, Lye SJ, Harris LK, Aplin JD, Jones RL. Decidual leucocytes infiltrating human spiral arterioles are rich source of matrix metalloproteinases and degrade extracellular matrix in vitro and in situ. Am J Reprod Immunol (2019) 81:e13054. doi: 10.1111/aji.13054
20. Lash GE, Otun HA, Innes BA, Bulmer JN, Searle RF, Robson SC. Inhibition of trophoblast cell invasion by TGFB1, 2, and 3 is associated with a decrease in active proteases. Biol Reprod (2005) 73:374–81. doi: 10.1095/biolreprod.105.040337
21. Lash GE, Otun HA, Innes BA, Kirkley M, De Oliveira L, Searle RF, et al. Interferon-gamma inhibits extravillous trophoblast cell invasion by a mechanism that involves both changes in apoptosis and protease levels. FASEB J (2006) 20:2512–8. doi: 10.1096/fj.06-6616com
22. Fu B, Zhou Y, Ni X, Tong X, Xu X, Dong Z, et al. Natural killer cells promote fetal development through the secretion of growth-promoting factors. Immunity (2017) 47:1100–1113 e1106. doi: 10.1016/j.immuni.2017.11.018
23. Fu B, Li X, Sun R, Tong X, Ling B, Tian Z, et al. Natural killer cells promote immune tolerance by regulating inflammatory TH17 cells at the human maternal-fetal interface. Proc Natl Acad Sci USA (2013) 110:E231–240. doi: 10.1073/pnas.1206322110
24. Crespo AC, Mulik S, Dotiwala F, Ansara JA, Sen Santara S, Ingersoll K, et al. Decidual NK cells transfer granulysin to selectively kill bacteria in trophoblasts. Cell (2020) 182:1125–1139 e1118. doi: 10.1016/j.cell.2020.07.019
25. Zhang Y, Ma L, Hu X, Ji J, Mor G, Liao A. The role of the PD-1/PD-L1 axis in macrophage differentiation and function during pregnancy. Hum Reprod (2019) 34:25–36. doi: 10.1093/humrep/dey347
26. Jaiswal MK, Mallers TM, Larsen B, Kwak-Kim J, Chaouat G, Gilman-Sachs A, et al. V-ATPase upregulation during early pregnancy: a possible link to establishment of an inflammatory response during preimplantation period of pregnancy. Reproduction (2012) 143:713–25. doi: 10.1530/REP12-0036
27. Zhang YH, He M, Wang Y, Liao AH. Modulators of the balance between M1 and M2 macrophages during pregnancy. Front Immunol (2017) 8:120.eCollection2017. doi: 10.3389/fimmu.2017.00120.eCollection2017
28. Hamilton S, Oomomian Y, Stephen G, Shynlova O, Tower CL, Garrod D, et al. Macrophages infiltrate the human and rat decidua during term and preterm labor: evidence that decidual inflammation precedes labor. Biol Reprod (2012) 86:39. doi: 10.1095/biolreprod.111.095505
29. Mor G, Straszewski-Chavez SL, Abrahams VM. Macrophage trophoblast interactions. Methods Mol Med (2006) 122:149–63. doi: 10.1385/1-59259-989-3:149
30. Munn DH, Shafizadeh E, Attwood JT, Bondarev I, Pashine A, Mellor AL. Inhibiton of T cell proliferation by macrophage tryptophan catabolism. J Exp Med (1999) 189:1363–72. doi: 10.1084/jem.189.9.1363
31. Tang MX, Hu XH, Liu ZZ, Kwak-Kim J, Liao AH. What are the roles of macrophages and monocytes in human pregnancy? J Reprod Immunol (2015) 112:73–80. doi: 10.1016/j.jri.2015.08.001
32. Engert S, Rieger L, Kapp M, Becker JC, Dietl J, Kammerer U. Profiling chemokines, cytokines and growth factors in human early pregnancy decidua by protein array. Am J Reprod Immunol (2007) 58:129–37. doi: 10.1111/j.1600-0897.2007.00498.x
33. Clark DE, Smith SK, Licence D, Evans AL, Charnock-Jones DS. Comparison of expression patterns for placenta growth factor, vascular endothelial growth factor (VEGF), VEGF-b and VEGF-c in the human placenta throughout gestation. J Endocrinol (1998) 159:459–67. doi: 10.1677/joe.0.1590459
34. Kumazaki K, Nakayama M, Suehara N, Wada Y. Expression of vascular endothelial growth factor, placental growth factor, and their receptors flt-1 and KDR in human placenta under pathologic conditions. Hum Pathol (2002) 33:1069–77. doi: 10.1053/hupa.2002.129420
35. Che JH, Zheng ZM, Li MQ, Yao XY. Macrophage polarization in placenta accreta and macrophage-trophoblast interactions. Am J Reprod Immunol (2022) 88:e13611. doi: 10.1111/aji.13611
36. Ding J, Yang C, Zhang Y, Wang J, Zhang S, Guo D, et al. M2 macrophage-derived G-CSF promotes trophoblasts EMT, invasion and migration via activating PI3K/Akt/Erk1/2 pathway to mediate normal pregnancy. J Cell Mol Med (2021) 25:2136–47. doi: 10.1111/jcmm.16191
37. Ono Y, Yoshino O, Hiraoka T, Sato E, Fukui Y, Ushijima A, et al. CD206+ M2-like macrophages are essential for successful implantation. Front Immunol (2020) 11:557184. doi: 10.3389/fimmu.2020.557184
38. Sharma S, Godbole G, Modi D. Decidual control of trophoblast invasion. Am J Reprod Immunol (2016) 75:341–50. doi: 10.1111/aji.12466
39. Fontana VA, Sanchez M, Cebral E, Calvo JC. Interleukin-1β regulates metalloproteinase activity and leptin secretion in a cytotrophoblast model. Biocell (2010) 34:37–43. doi: 10.32604/biocell.2010.34.037
40. Li M, Sun F, Xu Y, Chen L, Chen C, Cui L, et al. Tim-3(+) decidual mphis induced Th2 and treg bias in decidual CD4(+)T cells and promoted pregnancy maintenance via CD132. Cell Death Dis (2022) 13:454. doi: 10.1038/s41419-022-04899-2
41. Dimova T, Nagaeva O, Stenqvist AC, Hedlund M, Kjellberg L, Strand M, et al. Maternal Foxp3 expressing CD4+CD25+ and CD4+CD25- regulatory T-cell populations are enriched in human early normal pregnancy decidua: a phenotypic study of paired decidual and peripheral blood samples. Am J Reprod Immunol (2011) 66:44–56. doi: 10.1111/j.1600-0897.2011.01046.x
42. Tilburgs T, Roelen DL, van der Mast BJ, de Groot-Swings GM, Kleijburg C, Scherjon SA, et al. Evidence for a selective migration of fetus-specific CD4+CD25bright regulatory T cells from the peripheral blood to the decidua in human pregnancy. J Immunol (2008) 180:5737–45. doi: 10.4049/jimmunol.180.8.5737
43. Mjosberg J, Berg G, Jenmalm MC, Ernerudh J. FOXP3+ regulatory T cells and T helper 1, T helper 2, and T helper 17 cells in human early pregnancy decidua. Biol Reprod (2010) 82:698–705. doi: 10.1095/biolreprod.109.081208
44. Shima T, Inada K, Nakashima A, Ushijima A, Ito M, Yoshino O, et al. Paternal antigen-specific proliferating regulatory T cells are increased in uterine-draining lymph nodes just before implantation and in pregnant uterus just after implantation by seminal plasma-priming in allogeneic mouse pregnancy. J Reprod Immunol (2015) 108:72–82. doi: 10.1016/j.jri.2015.02.005
45. Guerin LR, Moldenhauer LM, Prins JR, Bromfield JJ, Hayball JD, Robertson SA. Seminal fluid regulates accumulation of FOXP3+ regulatory T cells in the preimplantation mouse uterus through expanding the FOXP3+ cell pool and CCL19-mediated recruitment. Biol Reprod (2011) 85:397–408. doi: 10.1095/biolreprod.110.088591
46. Bizargity P, Del Rio R, Phillippe M, Teuscher C, Bonney EA. Resistance to lipopolysaccharide-induced preterm delivery mediated by regulatory T cell function in mice. Biol Reprod (2009) 80:874–81. doi: 10.1095/biolreprod.108.074294
47. Xin L, Ertelt JM, Rowe JH, Jiang TT, Kinder JM, Chaturvedi V, et al. Cutting edge: committed Th1 CD4+ T cell differentiation blocks pregnancy-induced Foxp3 expression with antigen-specific fetal loss. J Immunol (2014) 192:2970–4. doi: 10.4049/jimmunol.1302678
48. Moldenhauer LM, Hayball JD, Robertson SA. Utilising T cell receptor transgenic mice to define mechanisms of maternal T cell tolerance in pregnancy. J Reprod Immunol (2010) 87:1–13. doi: 10.1016/j.jri.2010.05.007
49. Moldenhauer LM, Diener KR, Hayball JD, Robertson SA. An immunogenic phenotype in paternal antigen-specific CD8(+) T cells at embryo implantation elicits later fetal loss in mice. Immunol Cell Biol (2017) 95:705–15. doi: 10.1038/icb.2017.41
50. Li L, Tu J, Jiang Y, Zhou J, Schust DJ. Regulatory T cells decrease invariant natural killer T cell-mediated pregnancy loss in mice. Mucosal Immunol (2017) 10:613–23. doi: 10.1038/mi.2016.84
51. Zhang Y, Liu Z, Tian M, Hu X, Wang L, Ji J, et al. The altered PD-1/PD-L1 pathway delivers the 'one-two punch' effects to promote the Treg/Th17 imbalance in pre-eclampsia. Cell Mol Immunol (2017) 15:710–23. doi: 10.1038/cmi.2017.70
52. Blois SM, Ilarregui JM, Tometten M, Garcia M, Orsal AS, Cordo-Russo R, et al. A pivotal role for galectin-1 in fetomaternal tolerance. Nat Med (2007) 13:1450–7. doi: 10.1038/nm1680
53. Terme M, Chaput N, Combadiere B, Ma A, Ohteki T, Zitvogel L. Regulatory T cells control dendritic cell/NK cell cross-talk in lymph nodes at the steady state by inhibiting CD4+ self-reactive T cells. J Immunol (2008) 180:4679–86. doi: 10.4049/jimmunol.180.7.4679
54. Samstein RM, Josefowicz SZ, Arvey A, Treuting PM, Rudensky AY. Extrathymic generation of regulatory T cells in placental mammals mitigates maternal-fetal conflict. Cell (2012) 150:29–38. doi: 10.1016/j.cell.2012.05.031
55. Care AS, Bourque SL, Morton JS, Hjartarson EP, Robertson SA, Davidge ST. Reduction in regulatory T cells in early pregnancy causes uterine artery dysfunction in mice. Hypertension (2018) 72:177–87. doi: 10.1161/HYPERTENSIONAHA.118.10858
56. Nadkarni S, Smith J, Sferruzzi-Perri AN, Ledwozyw A, Kishore M, Haas R, et al. Neutrophils induce proangiogenic T cells with a regulatory phenotype in pregnancy. Proc Natl Acad Sci USA (2016) 113:E8415–24. doi: 10.1073/pnas.1611944114
57. Prince AL, Antony KM, Ma J, Aagaard KM. The microbiome and development: a mother's perspective. Semin Reprod Med (2014) 32:14–22. doi: 10.1055/s-0033-1361818
58. Shi Y, Yamada H, Sasagawa Y, Tanimura K, Deguchi M. Uterine endometrium microbiota and pregnancy outcome in women with recurrent pregnancy loss. J Reprod Immunol (2022) 152:103653. doi: 10.1016/j.jri.2022.103653
59. Moreno I, Codoñer FM, Vilella F, Valbuena D, Martinez-Blanch JF, Jimenez-Almazán J, et al. Evidence that the endometrial microbiota has an effect on implantation success or failure. Am J Obstet Gynecol (2016) 215:684–703. doi: 10.1016/j.ajog.2016.09.075
60. Zou Y, Liu X, Chen P, Wang Y, Li W, Huang R. The endometrial microbiota profile influenced pregnancy outcomes in patients with repeated implantation failure: a retrospective study. J Reprod Immunol (2023) 155:103782. doi: 10.1016/j.jri.2022.103782
61. Kwak-Kim J, Bao S, Lee SK, Kim JW, Gilman-Sachs A. Immunological modes of pregnancy loss: inflammation, immune effectors, and stress. Am J Reprod Immunol (2014) 72:129–40. doi: 10.1111/aji.12234
62. Lee SK, Kim JY, Hur SE, Kim CJ, Na BJ, Lee M, et al. An imbalance in interleukin-17-producing T and Foxp3+ regulatory T cells in women with idiopathic recurrent pregnancy loss. Hum Reprod (2011) 26:2964–71. doi: 10.1093/humrep/der301
63. Kopcow HD, Allan David SJ, Chen X, Rybalov B, Andzelm MM, Ge BX, et al. Human decidual NK cells from immature activating synapses and are not cytotoxic. Proc Natl Acad Sci USA (2005) 102:15563–8. doi: 10.1073/pnas.0507835102
64. King A, Allan DSJ, Bowen M, Powis SJ, Joseph S, Verma S, et al. HLA-e is expressed on trophoblast and interacts with CD94 / NKG2 receptors on decidual NK cells. Eur J Immunol (2000) 30:1623–31. doi: 10.1002/1521-4141(200006)30:6<1623
65. Shreeve N, Depierreux D, Hawkes D, Traherne JA, Sovio U, Huhn O, et al. The CD94/NKG2A inhibitory receptor educates uterine NK cells to optimize pregnancy outcomes in humans and mice. Immunity (2021) 54:1231–1244 e1234. doi: 10.1016/j.immuni.2021.03.021
66. Colonna M, Borsellino G, Falco M, Ferrara GB, Strominger JL. HLA-c is the inhibitory ligand that determines dominant resistance to lysis by NK1- and NK2-specific natural killer cells. Proc Natl Acad Sci USA (1993) 90:12000–4. doi: 10.1073/pnas.90.24.12000
67. Ban Y, Zhao Y, Liu F, Dong B, Kong B, Qu X. Effect of indoleamine 2,3-dioxygenase expressed in HTR-8/SVneo cells on decidual NK cell cytotoxicity. Am J Reprod Immunol (2016) 75:519–28. doi: 10.1111/aji.12481
68. Tan HX, Yang SL, Li MQ, Wang HY. Autophagy suppression of trophoblast cells induces pregnancy loss by activating decidual NK cytotoxicity and inhibiting trophoblast invasion. Cell Commun Signal (2020) 18:73. doi: 10.1186/s12964-020-00579-w
69. Ndhlovu LC, Lopez-Vergès S, Barbour JD, Jones RB, Jha AR, Long BR, et al. Tim-3 marks human natural killer cell maturation and suppresses cell-mediated cytotoxicity. Blood (2012) 119:3734–43. doi: 10.1182/blood-2011-11-392951
70. Li YH, Zhou WH, Tao Y, Wang SC, Jiang YL, Zhang D, et al. The galectin-9/Tim-3 pathway is involved in the regulation of NK cell function at the maternal-fetal interface in early pregnancy. Cell Mol Immunol (2016) 13:73–81. doi: 10.1038/cmi.2014.126
71. Abrahams VM, Bole-Aldo P, Kim YM, Straszewski-Chavez SL, Chaiworapongsa T, Romero R, et al. Divergent trophoblast responses to bacterial products mediated by TLRs. J Immunol (2004) 173:4286–96. doi: 10.4049/jimmunol.173.7.4286
72. Aldo PB, Racicot K, Craviero V, Guller S, Romero R, Mor G. Trophoblast induces monocyte differentiation into CD14+/CD16+ macrophages. Am J Reprod Immunol (2014) 72:270–84. doi: 10.1111/aji.12288
73. Svensson-Arvelund J, Mehta RB, Lindau R, Mirrasekhian E, Rodriguez-Martinez H, Berg G, et al. The human fetal placenta promotes tolerance against the semiallogeneic fetus by inducing regulatory T cells and homeostatic M2 macrophages. J Immunol (2015) 194:1534–44. doi: 10.4049/jimmunol.1401536
74. Lindau R, Mehta RB, Lash GE, Papapavlou G, Boij R, Berg G, et al. Interleukin-34 is present at the fetal-maternal interface and induces immunoregulatory macrophages of a decidual phenotype. vitro. Hum Reprod (2018) 33:588–99. doi: 10.1093/humrep/dey037
75. Lin TM, Halbert SP, Spellacy WN. Measurement of pregnancy-associated plasma proteins during human gestation. J Clin Invest (1974) 54:576–82. doi: 10.1172/JCI107794
76. Wessells J, Wessner D, Parsells R, White K, Finkenzeller D, Zimmermann W, et al. Pregnancy specific glycoprotein 18 induces IL-10 expression in murine macrophages. Eur J Immunol (2000) 30:1830–40. doi: 10.1002/1521-4141(200007)30:7<1830
77. Wang S, Sun F, Han M, Liu Y, Zou Q, Wang F, et al. Trophoblast-derived hyaluronan promotes the regulatory phenotype of decidual macrophages. Reproduction (2019) 157:189–98. doi: 10.1530/REP-18-0450
78. DeKruyff RH, Bu X, Ballesteros A, Santiago C, Chim YL, Lee HH, et al. T Cell/transmembrane, ig, and mucin-3 allelic variants differentially recognize phosphatidylserine and mediate phagocytosis of apoptotic cells. J Immunol (2010) 184:1918–30. doi: 10.4049/jimmunol.0903059
79. Xu YY, Wang SC, Li DJ, Du MR. Co-Signaling molecules in maternal-fetal immunity. Trends Mol Med (2017) 23:46–58. doi: 10.1016/j.molmed.2016.11.001
80. Li ZH, Wang LL, Liu H, Muyayalo KP, Huang XB, Mor G, et al. Galectin-9 alleviates LPS-induced preeclampsia-like impairment in rats via switching decidual macrophage polarization to M2 subtype. Front Immunol (2019) 9:3142. doi: 10.3389/fimmu.2018.03142
81. Chabtini L, Mfarrej B, Mounayar M, Zhu B, Batal I, Dakle PJ, et al. TIM-3 regulates innate immune cells to induce fetomaternal tolerance. J Immunol (2013) 190:88–96. doi: 10.4049/jimmunol.1202176
82. Zhang YH, Aldo P, You Y, Ding J, Kaislasuo J, Petersen JF, et al. Trophoblast-secreted soluble-PD-L1 modulates macrophage polarization and function. J Leukoc Biol (2020) 108:983–98. doi: 10.1002/JLB.1A0420-012RR
83. Lee CL, Guo Y, So KH, Vijayan M, Guo Y, Wong VH, et al. Soluble human leukocyte antigen G5 polarizes differentiation of macrophages toward a decidual macrophage-like phenotype. Hum Reprod (2015) 30:2263–74. doi: 10.1093/humrep/dev196
84. Meng YH, Zhou WJ, Jin LP, Liu LB, Chang KK, Mei J, et al. RANKL-mediated harmonious dialogue between fetus and mother guarantees smooth gestation by inducing decidual M2 macrophage polarization. Cell Death Dis (2017) 8:e3105. doi: 10.1038/cddis.2017.505
85. Gao L, Xu QH, Ma LN, Luo J, Muyayalo KP, Wang LL, et al. Trophoblast-derived lactic acid orchestrates decidual macrophage differentiation via SRC/LDHA signaling in early pregnancy. Int J Biol Sci (2022) 18:599–616. doi: 10.7150/ijbs.67816
86. Tannetta D, Masliukaite I, Vatish M, Redman C, Sargent I. Update of syncytiotrophoblast derived extracellular vesicles in normal pregnancy and preeclampsia. J Reprod Immunol (2017) 119:98–106. doi: 10.1016/j.jri.2016.08.008
87. Holder B, Jones T, Sancho Shimizu V, Rice TF, Donaldson B, Bouqueau M, et al. Macrophage exosomes induce placental inflammatory cytokines: a novel mode of maternal-placental messaging. Traffic (2016) 17:168–78. doi: 10.1111/tra.12352
88. Salomon C, Torres MJ, Kobayashi M, Scholz-Romero K, Sobrevia L, Dobierzewska A, et al. A gestational profile of placental exosomes in maternal plasma and their effects on endothelial cell migration. PloS One (2014) 9:e98667. doi: 10.1371/journal.pone.0098667
89. Atay S, Gercel-Taylor C, Suttles J, Mor G, Taylor DD. Trophoblast-derived exosomes mediate monocyte recruitment and differentiation. Am J Reprod Immunol (2011) 65(1):65–77. doi: 10.1111/j.1600-0897.2010.00880.x
90. Ying X, Jin X, Zhu Y, Liang M, Chang X, Zheng L. Exosomes released from decidual macrophages deliver miR-153-3p, which inhibits trophoblastic biological behavior in unexplained recurrent spontaneous abortion. Int Immunopharmacol (2020) 88:106981. doi: 10.1016/j.intimp.2020.106981
91. Ding J, Zhang Y, Cai X, Zhang Y, Yan S, Wang J, et al. Extracellular vesicles derived from M1 macrophages deliver miR-146a-5p and miR-146b-5p to suppress trophoblast migration and invasion by targeting TRAF6 in recurrent spontaneous abortion. Theranostics (2021) 11:5813–30. doi: 10.7150/thno.58731
92. Rendell V, Bath NM, Brennan TV. Medawar's paradox and immune mechanisms of fetomaternal tolerance. OBM Transplant (2020) 4:26. doi: 10.21926/obm.transplant.2001104
93. Wang W, Sung N, Gilman-Sachs A, Kwak-Kim J. T Helper (Th) cell profiles in pregnancy and recurrent pregnancy losses: Th1/Th2/Th9/Th17/Th22/Tfh cells. Front Immunol (2020) 11 doi: 10.3389/fimmu
94. Guo PF, Du MR, Wu HX, Lin Y, Jin LP, Li DJ. Thymic stromal lymphopoietin from trophoblasts induces dendritic cell-mediated regulatory TH2 bias in the decidua during early gestation in humans. Blood (2010) 116:2061–9. doi: 10.1182/blood-2009-11-252940
95. Figueiredo AS, Schumacher A. The T helper type 17/regulatory T cell paradigm in pregnancy. Immunology (2016) 148:13–21. doi: 10.1111/imm.12595
96. Sun Y, Wu S, Zhou Q, Li X. Trophoblast-derived interleukin 9 mediates immune cell conversion and contributes to maternal-fetal tolerance. J Reprod Immunol (2021) 148:103379. doi: 10.1016/j.jri.2021.103379
97. Ramhorst R, Fraccaroli L, Aldo P, Alvero AB, Cardenas I, Leiros CP, et al. Modulation and recruitment of inducible regulatory T cells by first trimester trophoblast cells. Am J Reprod Immunol (2012) 67:17–27. doi: 10.1111/j.1600-0897.2011.01056.x
98. Liu J, Hao S, Chen X, Zhao H, Du L, Ren H, et al. Human placental trophoblast cells contribute to maternal-fetal tolerance through expressing IL-35 and mediating iT(R)35 conversion. Nat Commun (2019) 10:4601. doi: 10.1038/s41467-019-12484-z
99. Lentz LS, Stutz AJ, Meyer N, Schubert K, Karkossa I, von Bergen M, et al. Human chorionic gonadotropin promotes murine treg cells and restricts pregnancy-harmful proinflammatory Th17 responses. Front Immunol (2022) 13:989247. doi: 10.3389/fimmu.2022.989247
100. Wang SC, Li YH, Piao HL, Hong XW, Zhang D, Xu YY, et al. PD-1 and Tim-3 pathways are associated with regulatory CD8+ T-cell function in decidua and maintenance of normal pregnancy. Cell Death Dis (2015) 6:e1738. doi: 10.1038/cddis.2015.112
101. Wafula PO, Teles A, Schumacher A, Pohl K, Yagita H, Volk HD, et al. PD-1 but not CTLA-4 blockage abrogates the protective effect of regulatory T cells in a pregnancy murine model. Am J Reprod Immunol (2009) 62:283–92. doi: 10.1111/j.1600-0897.2009.00737.x
102. Wang S, Zhu X, Xu Y, Zhang D, Li Y, Tao Y, et al. Programmed cell death-1 (PD-1) and T-cell immunoglobulin mucin-3 (Tim-3) regulate CD4+ T cells to induce type 2 helper T cell (Th2) bias at the maternal-fetal interface. Hum Reprod (2016) 31:700–11. doi: 10.1093/humrep/dew019
103. Hu X, Zhu Q, Wang Y, Wang L, Li Z, Mor G, et al. Newly characterized decidual Tim-3+ treg cells are abundant during early pregnancy and driven by IL-27 coordinately with gal-9 from trophoblasts. Hum Reprod (2020) 35:2454–66. doi: 10.1093/humrep/deaa223
104. Janeway CA Jr., Medzhitov R. Innate immune recognition. Annu Rev Immunol (2002) 20:197–216. doi: 10.1146/annurev.immunol.20.083001.084359
105. Medzhitov R, Janeway C Jr. Innate immunity. N Engl J Med (2000) 343:338–44. doi: 10.1056/NEJM200008033430506
106. Abrahams VM, Visintin I, Aldo PB, Guller S, Romero R, Mor G. A role for TLRs in the regulation of immune cell migration by first trimester trophoblast cells. J Immunol (2005) 175:8096–104. doi: 10.4049/jimmunol.175.12.8096
107. Abrahams VM, Schaefer TM, Fahey JV, Visintin I, Wright JA, Aldo PB, et al. Expression and secretion of antiviral factors by trophoblast cells following stimulation by the TLR-3 agonist, Poly(I : c). Hum Reprod (2006) 21:2432–9. doi: 10.1093/humrep/del178
108. Mor G, Romero R, Aldo PB, Abrahams VM. Is the trophoblast an immune regulator? the role of toll-like receptor during prengnacy. Crit Rev Immunol (2005) 25:375–88. doi: 10.1615/critrevimmunol.v25.i5.30
109. Costello MJ, Joyce SK, Abrahams VM. NOD protein expression and function in first trimester trophoblast cells. Am J Reprod Immunol (2007) 57:67–80. doi: 10.1111/j.1600-0897.2006.00447.x
110. Kwon JY, Aldo P, You Y, Ding J, Racicot K, Dong X, et al. Relevance of placental type I interferon beta regulation for pregnancy success. Cell Mol Immunol (2018) 15:1010–26. doi: 10.1038/s41423-018-0050-y
111. Ivashkiv LB, Donlin LT. Regulation of type I interferon responses. Nat Rev Immunol (2014) 14:36–49. doi: 10.1038/nri3581
112. Li C, Wang Y, Xing Y, Han J, Zhang Y, Zhang A, et al. Regulation of microglia phagocytosis and potential involvement of exercise. Front Cell Neurosci (2022) 16:953534. doi: 10.3389/fncel.2022.953534
113. Ding J, Aldo P, Roberts CM, Stabach P, Liu H, You Y, et al. Placenta-derived interferon-stimulated gene 20 controls ZIKA virus infection. EMBO Rep (2021) 22:e52450. doi: 10.15252/embr.202152450
114. Racicot K, Aldo P, El-Guindy A, Kwon JY, Romero R, Mor G. Cutting edge: Fetal/Placental type I IFN can affect maternal survival and fetal viral load during viral infection. J Immunol (2017) 198:3029–32. doi: 10.4049/jimmunol.1601824
115. Racicot K, Kwon JY, Aldo P, Abrahams V, El-Guindy A, Romero R, et al. Type I interferon regulates the placental inflammatory response to bacteria and is targeted by virus: mechanism of polymicrobial infection-induced preterm birth. Am J Reprod Immunol (2016) 75:451–60. doi: 10.1111/aji.12501
116. Spencer TE, Bazer FW. Conceptus signals for establishment and maintenance of pregnancy. Reprod Biol Endocrinol (2004) 2:49. doi: 10.1186/1477-7827-2-49
117. Travelli C, Colombo G, Mola S, Genazzani AA, Porta C. NAMPT: a pleiotropic modulator of monocytes and macrophages. Pharmacol Res (2018) 135:25–36. doi: 10.1016/j.phrs.2018.06.022
118. Al-Nasiry S, Ambrosino E, Schlaepfer M, Morré SA, Wieten L, Voncken JW, et al. The interplay between reproductive tract microbiota and immunological system in human reproduction. Front Immunol (2020) 11:378. doi: 10.3389/fimmu.2020.00378
119. Coomarasamy A, Dhillon-Smith RK, Papadopoulou A, Al-Memar M, Brewin J, Abrahams VM, et al. Recurrent miscarriage: evidence to accelerate action. Lancet (2021) 397:1675–82. doi: 10.1016/S0140-6736(21)00681-4
120. Shi Y, Tan D, Hao B, Zhang X, Geng W, Wang Y, et al. Efficacy of intravenous immunoglobulin in the treatment of recurrent spontaneous abortion: a systematic review and meta-analysis. Am J Reprod Immunol (2022) 88:e13615. doi: 10.1111/aji.13615
121. Khonina NA, Broitman EV, Shevela EY, Pasman NM, Chernykh ER. Mixed lymphocyte reaction blocking factors (MLR-bf) as potential biomarker for indication and efficacy of paternal lymphocyte immunization in recurrent spontaneous abortion. Arch Gynecol Obstet (2013) 288:933–7. doi: 10.1007/s00404-013-2832-x
122. Dakhly DM, Bayoumi YA, Sharkawy M, Gad Allah SH, Hassan MA, Gouda HM, et al. Intralipid supplementation in women with recurrent spontaneous abortion and elevated levels of natural killer cells. Int J Gynaecol Obstet (2016) 135:324–7. doi: 10.1016/j.ijgo.2016.06.026
123. Wu H, You Q, Jiang Y, Mu F. Tumor necrosis factor inhibitors as therapeutic agents for recurrent spontaneous abortion. Mol Med Rep (2021) 24:847. doi: 10.3892/mmr.2021.12487
124. Tsai HF, Hsu PN. Cancer immunotherapy by targeting immune checkpoints: mechanism of T cell dysfunction in cancer immunity and new therapeutic targets. J BioMed Sci (2017) 24:35. doi: 10.1186/s12929-017-0341-0
125. Ansell SM, Lesokhin AM, Borrello I, Halwani A, Scott EC, Gutierrez M, et al. PD-1 blockade with nivolumab in relapsed or refractory hodgkin's lymphoma. N Engl J Med (2015) 372:311–9. doi: 10.1056/NEJMoa1411087
Keywords: trophoblast, NK, macrophage, Treg, commensal microbiota, interactions
Citation: Zhang Y, Liu Z and Sun H (2023) Fetal-maternal interactions during pregnancy: a ‘three-in-one’ perspective. Front. Immunol. 14:1198430. doi: 10.3389/fimmu.2023.1198430
Received: 01 April 2023; Accepted: 24 May 2023;
Published: 07 June 2023.
Edited by:
Robert David Miller, University of New Mexico, United StatesReviewed by:
Dongyao Wang, University of Science and Technology of China, ChinaVictoria Hansen, University of Rochester Medical Center, United States
Copyright © 2023 Zhang, Liu and Sun. This is an open-access article distributed under the terms of the Creative Commons Attribution License (CC BY). The use, distribution or reproduction in other forums is permitted, provided the original author(s) and the copyright owner(s) are credited and that the original publication in this journal is cited, in accordance with accepted academic practice. No use, distribution or reproduction is permitted which does not comply with these terms.
*Correspondence: Yonghong Zhang, eWh6aGFuZ2xzQDE2My5jb20=
†These authors have contributed equally to this work