- 1Division of Hospital Medicine, Department of Internal Medicine, Sharp Rees-Stealy Medical Group, Sharp Healthcare, San Diego, CA, United States
- 2Department of Pathology and Laboratory Medicine, David Geffen School of Medicine at UCLA, Los Angeles, CA, United States
- 3Department of Biostatistics, Fielding School of Public Health, University of California Los Angeles, Los Angeles, CA, United States
- 4Division of Infectious Diseases, Department of Pediatrics, David Geffen School of Medicine at UCLA, Los Angeles, CA, United States
- 5Department of Epidemiology, Fielding School of Public Health, University of California Los Angeles, Los Angeles, CA, United States
- 6Division of Infectious Diseases, Department of Medicine, David Geffen School of Medicine at UCLA, Los Angeles, CA, United States
- 7Infectious Diseases Section, Veterans Affairs (VA) Greater Los Angeles Healthcare System, Los Angeles, CA, United States
Introduction: Severe COVID-19 illness is characterized by an overwhelming immune hyperactivation. Autoantibodies against vascular, tissue, and cytokine antigens have been detected across the spectrum of COVID-19. How these autoantibodies correlate with COVID-19 severity is not fully defined.
Methods: We performed an exploratory study to investigate the expression of vascular and non-HLA autoantibodies in 110 hospitalized patients with COVID-19 ranging from moderate to critically ill. Relationships between autoantibodies and COVID- 19 severity and clinical risk factors were examined using logistic regression analysis.
Results: There were no absolute differences in levels of expression of autoantibodies against angiotensin II receptor type 1 (AT1R) or endothelial cell proteins between COVID-19 severity groups. AT1R autoantibody expression also did not differ by age, sex, or diabetes status. Using a multiplex panel of 60 non- HLA autoantigens we did identify seven autoantibodies that differed by COVID-19 severity including myosin (myosin; p=0.02), SHC-transforming protein 3 (shc3; p=0.07), peroxisome proliferator-activated receptor gamma coactivator 1-beta (perc; p=0.05), glial-cell derived neurotrophic factor (gdnf; p=0.07), enolase 1 (eno1; p=0.08), latrophilin-1 (lphn1; p=0.08), and collagen VI (coll6; p=0.05) with greater breadth and higher expression levels seen in less severe COVID-19.
Discussion: Overall, we found that patients hospitalized with COVID-19 demonstrate evidence of auto-reactive antibodies targeting endothelial cells, angiotensin II receptors, and numerous structural proteins including collagens. Phenotypic severity did not correlate with specific autoantibodies. This exploratory study underscores the importance of better understanding of the role of autoimmunity in COVID-19 disease and sequelae.
1 Introduction
While SARS-CoV-2 infection causes mild illness in most persons, a minority develop severe COVID-19 that can progress to acute respiratory distress syndrome, multiorgan failure, and death. Severe COVID-19 is characterized by an overwhelming immune response with elevated pro-inflammatory cytokines and innate immune hyperactivation (1, 2). Current pharmacotherapy for COVID-19 targets these immune mechanisms via the routine use immunomodulatory therapy (glucocorticoids, IL6 inhibitors and JAK inhibitors in severe to critical cases of COVID-19) (3). In the case of severe COVID-19, the wide spectrum of illness and multiorgan involvement may also be related to the presence and severity of thrombovasculitic disease. Indeed, two post-mortem studies demonstrated that microthrombotic angiopathy and endothelialitis in major organs are the predominant pathologic findings of patients who die due to overwhelming COVID-19 (4, 5).
Autoimmunity against the renin-angiotensin system, which regulates vascular tone, is a well-established pathology in vasculitic disease in humans. Auto-antibodies against angiotensin converting enzyme 2 (ACE2) and endothelial cell proteins are known to correlate with both the presence and severity of vasculitis diseases including systemic lupus erythematosus, anti-phospholipid syndrome, rheumatoid arthritis, systemic sclerosis, and Kawasaki disease (6–9). Angiotensin II receptor type 1 auto-antibodies (AT1R-Ab) represent another source of immune pathology in humans. Organ transplant patients who develop HLA-negative antibody mediated rejection typically do so as a result of circulating AT1R-Ab against the allograft tissue (10–15). These antibodies appear to trigger vascular inflammation in transplanted organs that is accompanied by the production of inflammatory cytokines with associated graft vasculopathy and allograft dysfunction (16, 17). Auto-immunity against endothelial cell proteins may also be a factor in such cases, as the presence of AT1R-Ab also correlates strongly with level of anti-endothelial cell antibodies (AECA) as measured by the endothelial cell flow cytometric crossmatch (ECXM) assay (12, 18). Perhaps it comes as little surprise that patients with COVID-19 also express these autoimmune markers. Among patients with severe or unfavorable courses with COVID-19, AT1R-Ab and anti-endothelial antibodies were higher than those with mild COVID-19 and/or matched controls (19, 20). However, other studies found that patients with mild COVID-19 had higher AT1R compared to patients with more severe COVID-19 and/or healthy controls (21, 22). Severe COVID-19 can also induce anti-angiotensin II antibodies which correlated with poor oxygenation and blood pressure dysregulation in these patients (23).
Understanding the breadth and magnitude of autoantibody responses across COVID-19 will be important to better understand pathophysiology of this disease and its sequelae. In addition to vascular antigens, autoantibodies against tissue and cytokine antigens develop during SARS-CoV-2 infection in hospitalized patients (24, 25), and track with the onset of SARS-CoV-2 immune responses (25). Persistent autoantibody responses have also been found in recovered patients following mild or asymptomatic SARS-CoV-2 infection (26). While specific autoantibodies against vascular and cytokine antigens have been correlated with COVID-19 clinical severity (24, 25, 27, 28), the relationships between autoantibody breadth and clinical outcomes have not been established. Here we investigated not only the prevalence of vascular autoimmune responses, but also further examined the breadth of the autoantibody response including other tissue antigens as correlates of phenotypic severity in patients hospitalized with COVID-19. We hypothesized that greater autoimmune responses would correlate with more severe COVID-19 disease.
2 Materials and methods
2.1 Ethics statement
The study was approved by the UCLA Institutional Review Board (#20-000473) and the Sharp Healthcare Institutional Review Board (#2012806). Informed consent was obtained from all study participants.
2.2 Study participants
Patients were recruited at two large healthcare organizations in Southern California. Inclusion criteria included hospitalization for COVID-19, age greater than 18 years, and confirmed positive SARS-CoV-2 RT-PCR test results within 72 hours of admission.
UCLA Health is a large academic healthcare organization in Los Angeles, California. Patients with confirmed positive SARS-CoV-2 RT-PCR nasopharyngeal swab were enrolled in an observational cohort study within 72 hours of admission. Exclusion criteria included pregnancy, hemoglobin < 8g/dL, or inability to provide informed consent. Blood specimens, nasopharyngeal swab, and saliva were collected throughout hospitalization up to 6 weeks. A total of 80 patients from UCLA Health were included in this study from April 2020 until February 2021. Samples from the first study visit after admission (within 72 hours) were used in this analysis.
Sharp HealthCare is a regional healthcare organization in San Diego, California. Patients with confirmed positive SARS-CoV-2 RT-PCR nasopharyngeal swab within 72 hours of admission or the seven days preceding admission for hospital transfer were enrolled in this observational study. Exclusion criteria included: prior vaccination against SARS-CoV-2, treatment with anti-CD20 or anti-CD19 monoclonal antibodies, COVID convalescent plasma (CCP) or fresh frozen plasma, IVIG, plasmapheresis, anti-neoplastic chemotherapy, or other biologic or immunosuppressive agents (aside from corticosteroids, IL6 receptor inhibitors, or baricitinib given for treatment of COVID-19) in the three months prior to enrollment, history of organ transplant, or clinically active autoimmune disease. A total of 32 patients from Sharp HealthCare were included in this study from February 2021 until August 2021. Samples from the first study visit after admission (within 72 hours) were used in this analysis. Two enrolled patients were diagnosed with multisystem inflammatory syndrome in adults (MIS-A) and excluded from this analysis, but clinical data for these cases is included in Supplementary Table 1.
Demographic and clinical data, including laboratory results and therapeutics, were collected from the electronic medical records. Clinical severity was scored using the NIAID 8-point ordinal scale (29): 1, not hospitalized and no limitations; 2, not hospitalized but with limitations; 3, hospitalized no supplemental oxygen or ongoing medical care; 4, hospitalized no supplemental oxygen but with ongoing medical care; 5, hospitalized with supplemental oxygen; 6, hospitalized with non-invasive ventilation or high-flow oxygen; 7, hospitalized with invasive mechanical ventilation or ECMO; 8, death.
2.3 AT1R-Ab quantification
Plasma samples at the first study visit after admission were used for all assays. AT1R-Ab were quantified by ELISA (Cell Trend, Germany) as previously described (17). Briefly, sera were diluted 1:100, tested in duplicate, and AT1R-Ab concentrations were determined by a standard curve. AT1R-Abs exceeding the limit of the standard curve were further diluted and re-tested to determine concentration. AT1R-Ab IgG >10 units/ml was considered at risk for endothelial dysfunction based on laboratory validated manufacturer’s recommended cutoff value.
2.4 Endothelial cell crossmatch
Anti-endothelial cell antibodies were quantified using endothelial cell crossmatch by flow cytometry as previously described (30). Briefly, primary human aortic endothelial cells were isolated from aortic rings of explanted donor hearts or obtained from commercial sources. The cells were cultured and passages 7 to 8 were frozen and used in the endothelial cell crossmatch. A total of 2x105 cells were incubated with 100 μl patient serum on ice for 30 minutes, washed and incubated with F(ab′)2 fragment Goat Anti-Human IgG Fcγ fragment (Jackson ImmunoResearch Laboratories). Cell fluorescence was analyzed on a FACSCalibur flow cytometer using CellQuest Software (BD Biosciences) with a minimum of 10,000 gated events acquired. The positive endothelial cell crossmatch threshold was set at two standard deviations (50 Median Channel Shift) above the mean of negative control serum tests
2.5 Autoantibody quantification by luminex
A custom Luminex panel was used to quantify 60 different autoantibodies as previously described (31). The non-HLA multiplex bead panel was purchased from Immucor, Inc, and included 60 non-HLA antigens conjugated to polystyrene beads (LIFECODES Non-HLA Antibody Kit; cat # 265500R). Forty µL of antigen-coated beads were incubated with 10 µL of serum for 30 minutes. After washing beads were stained with 50 µL of phycoerythrin (PE) conjugated goat anti-human IgG diluted 1:10 in buffer and incubated in the dark on a shaking platform for 30 minutes. Antibody binding was reported as the median fluorescence intensity (MFI) of IgG binding on the Luminex 100 (Luminex). Antibodies with mean MFI >500 in either group were selected for further analysis based on manufacturer’s provided average background expression in healthy control populations resulting in n=36 antibodies. From there a stringent MFI cutoff of 1000 was used to determined positive/negative based on overall assay variance.
2.6 Statistical analysis
Autoantibody MFI levels were log-transformed to reduce positive skew of the raw data. Comparisons of mean and median log-MFI estimates were performed between COVID-19 severity groups (moderate/severe vs critical/deceased) using t-tests and Wilcoxon rank sum tests. Comparisons of positive autoantibodies by severity groups were performed using t-tests and Fisher’s exact tests. Associations with COVID-19 risk factors were analyzed using multiple logistic regression. In this exploratory analysis an unadjusted p-value < 0.1 was considered significant. Statistical analyses were conducted using STATA version 17.0 and R version 4.2.1.
3 Results
3.1 Study population
This study included a total of 110 patients with n=80 patients from the Los Angeles site and n=30 patients from the San Diego site. This was a diverse cohort with 52% Hispanic, 10% Asian/Non-Hispanic, 7% Black/Non-Hispanic, and 20% White/Non-Hispanic. Consistent with COVID-19 hospitalization trends, there were more men (59%) than women. The average age across the study population was 52.5 years. Patients were grouped based on maximum clinical severity during hospitalization as classified by the NIAID 8-point ordinal scale (29), and further combined into moderate to severe (ordinal scale groups 4-5) group or critical to decreased (ordinal scale groups 6-8) group. Patients with moderate to severe disease were on average younger (mean age 49 years) than those with critical to fatal disease (mean age 56 years) (Table 1). Comorbidities included cardiovascular disease (57%) and diabetes (68%) in the majority of patients, with greater prevalence of diabetes in the moderate to severe group (78% vs 56%). In addition, this study included 21 patients (19%) with solid organ transplant at the time of study entry. COVID-directed therapies varied based on standard of care at each site and a stage of the pandemic.
3.2 Prevalence of AT1R-Ab and endothelial cross-reactivity
To assess the prevalence of ATR1-Ab in COVID-19 patients we used ELISA to quantify anti-AT1R IgG in plasma samples collected within 72 hours of hospital admission. The median concentration of AT1R-Ab was similar across both severity groups (Figure 1A). However, when looking at the proportion of patients with ATR1-Ab levels above the threshold for endothelial dysfunction risk (>10 U/ml), the majority of patients in both severity groups had elevated ATR1-Ab with the critically ill group having slightly greater proportion (Figure 1B).
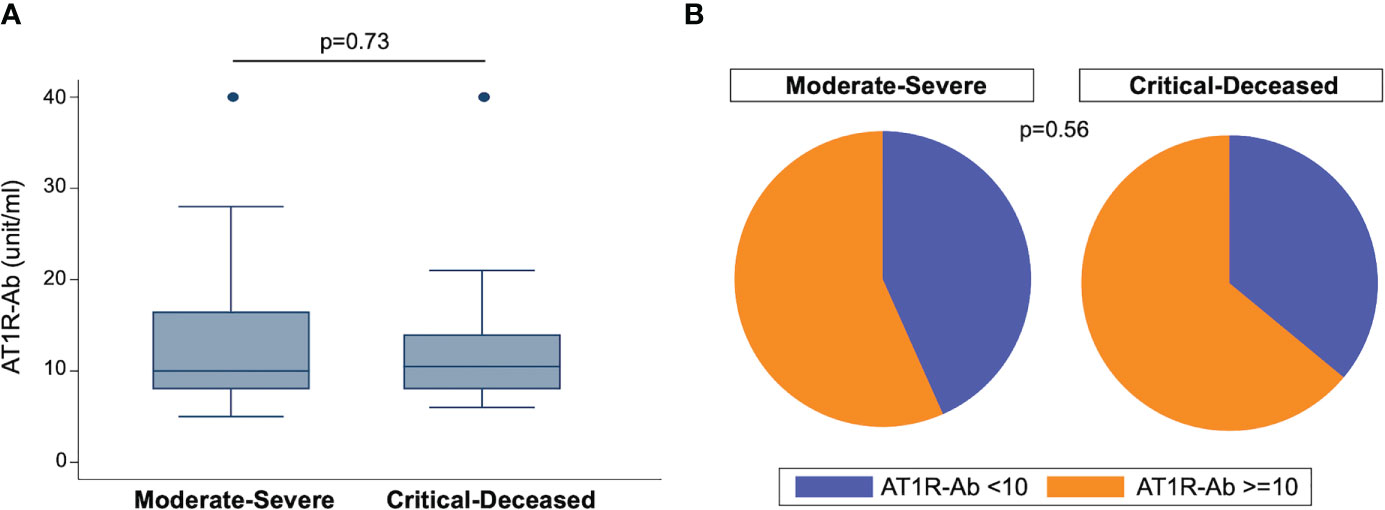
Figure 1 Angiotensin II receptor type 1 antibody (AT1R-Ab) quantification by COVID-19 severity. (A) Amount of AT1R-Ab measured by ELISA in plasma collected within 72 hours of hospital admission from patients with COVID-19 grouped by severity. Boxes show the interquartile range (IQR) with the line at the median and whiskers extend to 1.5 times the IQR. Data points outside the range (outliers) are shown with dots. P-value calculated using Wilcoxon rank-sum test. (B) Proportion of patients at risk for endothelial cell dysfunction (AT1R-Ab >=10) grouped by COVID-19 severity. P-value calculated using Fisher’s exact test.
We also examined endothelial cell cross-reactivity using a flow cytometry-based method to examine presence of cross-reactive antibodies against human aortic endothelial cells (ECXM) (30). The median ECXM scores were higher in the critically ill group but did not meet statistical significance (Figure 2A). When looking at the proportion of patients in each clinical group with ECXM greater than 50 median channel shift, again the majority of patients in both groups had evidence of anti-endothelial cell antibodies with the critically ill having the greatest proportion (Figure 2B; p=0.19).
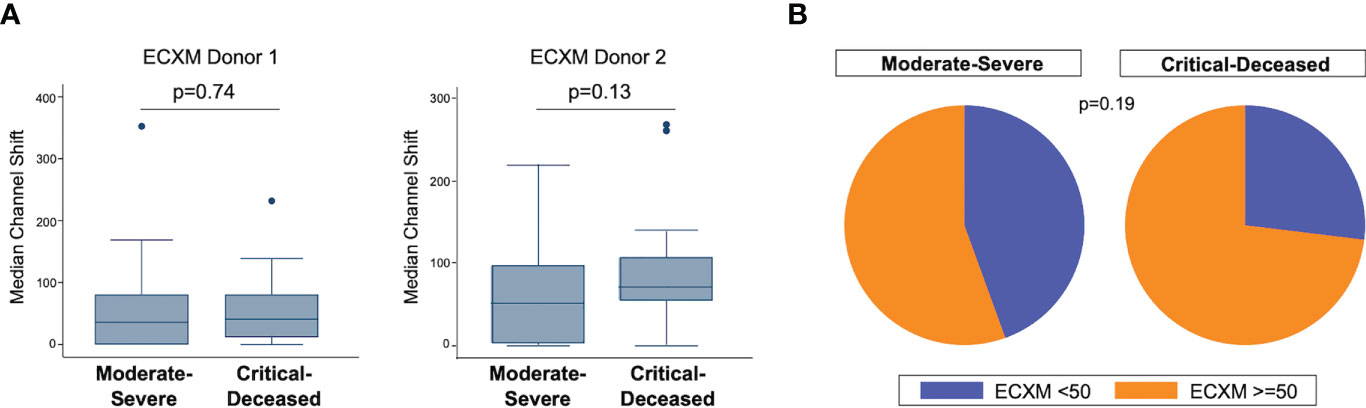
Figure 2 Anti-endothelial cell antibodies by COVID-19 severity. (A) Anti-endothelial cell antibodies were measured in plasma collected within 72 hours of hospital admission by endothelial cell crossmatch using two separate donors shown as median channel shift above negative control serum. Boxes show the interquartile range (IQR) with the line at the median and whiskers extend to 1.5 times the IQR. Data points outside the range (outliers) are shown with dots. P-values calculated using Wilcoxon rank-sum tests. (B) Proportion of patients with positive endothelial cell crossmatch (EXCM >=50 based on two standard deviations above negative control serum) grouped by COVID-19 severity. P-value calculated using Fisher’s exact test.
We next used regression analysis to further examine associations between AT1R-Ab and COVID-19 severity. There was no significant association between AT1R-Ab and COVID-19 severity in univariate analysis (OR 1.02, 95% CI [0.97, 1.07], p=0.45). We still found no significant associations with AT1R-Ab using multiple logistic regression including age, sex, ethnicity, race, BMI, diabetes, cardiovascular disease, pulmonary disease, kidney disease, or transplant status. We again used regression analysis to further examine associations between ECXM and COVID-19 severity, but found no significant association between ECXM and COVID-19 severity in univariate analysis (OR 2.17, 95% CI [0.73, 6.44], p=0.16) or multivariate analysis.
3.3 Correlation of vascular autoantibodies and COVID-19 risk factors
We next examined the correlations between AT1R autoantibodies and known COVID-19 risk factors. As the presence of autoantibodies can increase with age (32), we hypothesized that the presence of autoantibodies may be a mediating factor in the association between COVID-19 severity and age. However, we found no correlation between age and ATR1-Ab (Figure 3A). We also looked at comparisons of autoantibodies by sex, but again found no specific difference of AT1R-Ab by sex (Figure 3B). Since diabetes is also a significant risk factor for severe COVID-19 we compared autoantibodies by diabetes status and found no significant difference in AT1R-Ab in those with or without diabetes (Figure 3C).
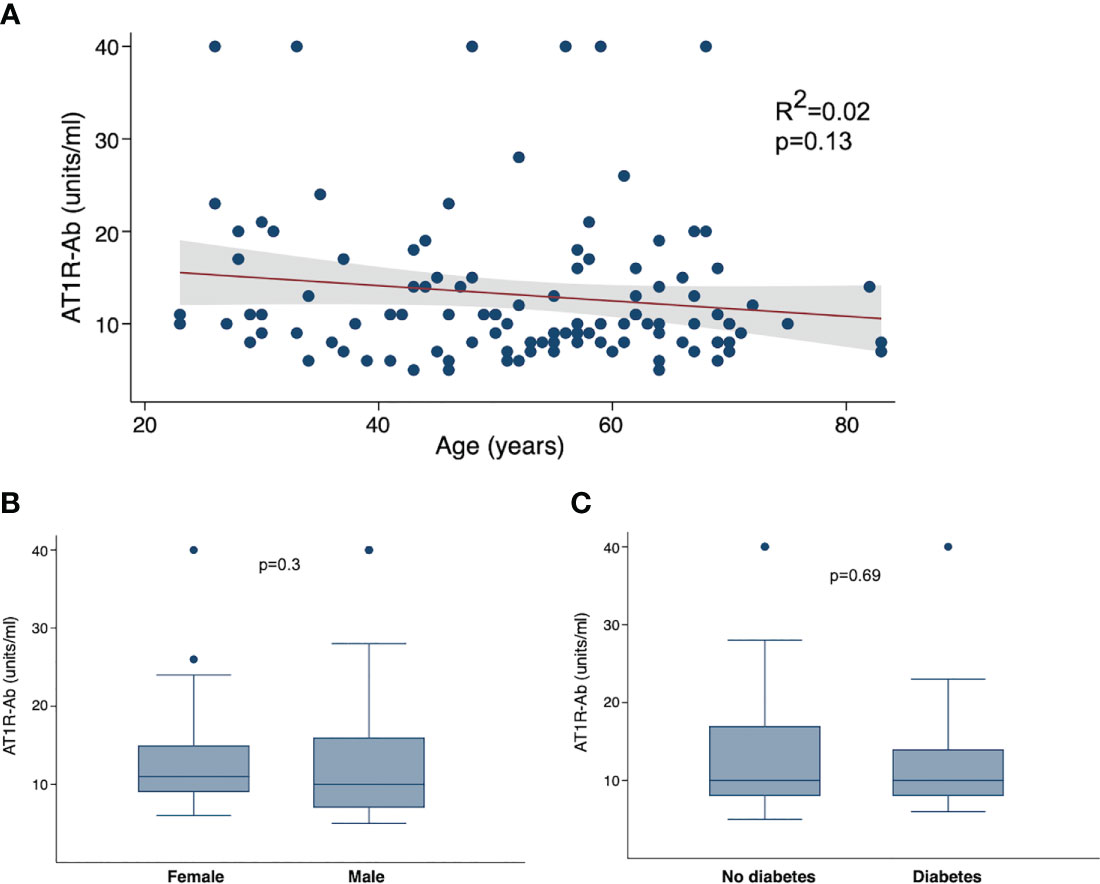
Figure 3 Angiotensin II receptor type 1 antibody (AT1R-Ab) by COVID-19 clinical characteristics. (A) AT1R-Ab quantification plotted by age in years. P-value and R2 calculated using linear regression. AT1R-Ab quantification by (B) sex and (C) diabetes status. Boxes show the interquartile range (IQR) with the line at the median and whiskers extend to 1.5 times the IQR. Data points outside the range (outliers) are shown with dots. P-values calculated using Wilcoxon rank sum tests.
3.4 Expression of non-HLA self-reactive antibodies in COVID-19
To assess for other targets of autoimmunity, we used a multiplex panel to quantify self-reactive antibodies against 60 collagen-vascular antigens. We used unsupervised hierarchical clustering to visualize differences in these autoantibodies by COVID-19 severity. Comparing each severity group there does appear to be overall higher expression of autoantibodies in the moderate-severe compared to the critical-deceased group (Figure 4A), but the number of positive autoantibodies did not significantly differ by severity groups (p=0.18; Figure 4B). The autoantibodies with the most significant difference in positivity between severity groups included myosin (myosin; p=0.02), SHC-transforming protein 3 (shc3; p=0.07), peroxisome proliferator-activated receptor gamma coactivator 1-beta (perc; p=0.05), glial-cell derived neurotrophic factor (gdnf; p=0.07), enolase 1 (eno1; p=0.08), latrophilin-1 (lphn1; p=0.08), and collagen VI (coll6; p=0.05). With the exception of enolase 1, expression of these autoantibodies was higher in the moderate-severe group compared to the critical-deceased (Supplementary Table 2 and Figure 4C).
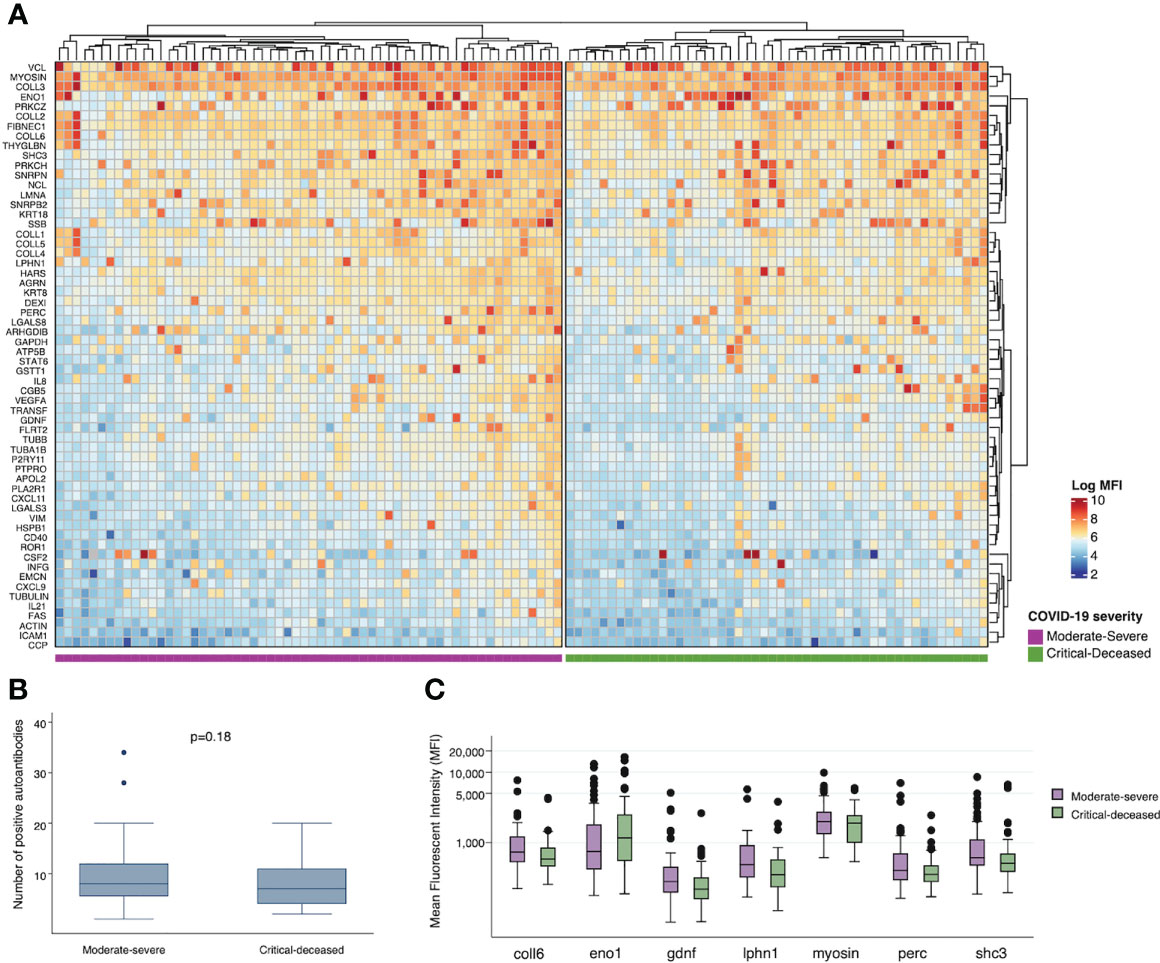
Figure 4 Plasma autoantibodies by COVID-19 severity. (A) Heatmap showing relative expression of autoantibodies in plasma collected within 72 hours of hospital admission among moderate-severe (left) or critical-deceased (right) COVID-19 patients. (B) Comparison of number of positive autoantibodies by COVID-19 severity. P-value calculated using t-test. (C) Relative expression of plasma autoantibodies compared by COVID-19 severity (orange, moderate-severe; blue, critical-deceased). Only antibodies with p<=0.1 by Fisher’s exact tests are shown. Boxes show the interquartile range (IQR) with the line at the median and whiskers extend to 1.5 times the IQR. Data points outside the range (outliers) are shown with dots.
4 Discussion
Mounting evidence implicates a dysregulated host immune response in the pathophysiology of COVID-19. Severe disease typically presents as a rapid progression of worsening dyspnea and hypoxemia around the second week following infection, a time course is also coincident with the early humoral immune response (33–35). The presence of autoantibodies has been described in several clinical studies resulting in a need to better understand the potential role of autoantibodies in contributing to COVID-19 disease. This exploratory study sought to expand this understanding, specifically examining the presence of autoantibodies against endothelial cells and collagen-vascular tissue. We did not find an association with AT1R-Ab or AECA and COVID-19 severity. We did identify the presence of other collagen-vascular autoantibodies in hospitalized patients with COVID-19, and those with less severe COVID-19 actually had higher autoantibody levels.
Our data are consistent with prior studies detailing the presence of autoantibodies in COVID-19. While others showed associations between AT1R and COVID-19 severity (19, 20), we did not find similar associations. At least one other study also found no difference in AT1R-Ab when comparing ICU patients and non-ICU hospitalized patients (22). Several factors may contribute to these differences. The timing of sampling during disease course may differ between studies which could affect the ability to detect autoantibodies. Though several studies, including ours, controlled for comorbid conditions or other confounding factors, there is still heterogeneity between study populations as well as differences in COVID-directed therapeutics that may affect results. The totality of these studies suggest that these autoantibodies may play a role in COVID-19, and further investigation with larger longitudinal analysis will be necessary to improve understanding.
Virus-triggered immune dysregulation contributes not only to the acute presentation, but also to post-infection sequelae. Well known pathogens such as enteroviruses and Parvovirus B19 are associated with and potentially causative of autoimmune diseases including rheumatoid arthritis and type 1 diabetes mellitus (36, 37). More recently, prior infection with Epstein-Barr virus was shown to increase odds of later developing multiple sclerosis (38). Many of these same autoimmune epitopes observed in our study are also implicated in the development and progression of autoimmune disease. Anti-collagen antibodies have been observed in the early phase response of rheumatoid arthritis (39) and anti-enolase in a multitude of autoimmune diseases (40). Similar collagen autoantibodies have also been implicated in transplant allograft rejection (41). It is possible that the severe immune activation that characterizes COVID-19 leads to either epitope spread or bystander activation resulting in loss of self-tolerance and autoantibody production. Indeed, there have been reports describing increased incidence of autoimmune disease following COVID-19 (42–44). Interestingly, there have been reports of higher risk of new onset autoimmune disease following mild to moderate COVID-19 (43); we also observed greater autoantibody levels in the moderate group compared to critical. Longitudinal studies will be important to determine if COVID-19 severity and/or extent of autoantibody responses during COVID-19 are risk factors for subsequent autoimmune disease.
Our study has several important limitations to consider when interpreting the data. We did not have non-COVID-19 control samples available for analysis, thus can only draw conclusions within our COVID-19 severity groups. While we can compare our results to historical controls and published studies from before SARS-CoV-2, the lack of a within study control group is a limitation. Our study population also had heterogeneity in terms of treatments received and comorbidities, including solid organ transplantation. We accounted for these differences in our analyses as able, but these may still confound our results. Finally, our study is cross-sectional which limits any conclusions regarding causality between autoantibody presence and COVID-19 outcomes or future disease risk.
Patients hospitalized with COVID-19 demonstrated evidence of auto-reactive antibodies targeting endothelial cells, angiotensin II receptors, and numerous structural proteins including collagens. In this exploratory study, phenotypic severity did not correlate with specific autoantibodies. Further research is required to understand the mechanisms and clinical implications of autoimmunity following infection with SARS-CoV-2.
Data availability statement
The original contributions presented in the study are included in the article/Supplementary Material. Further inquiries can be directed to the corresponding author.
Ethics statement
The studies involving human participants were reviewed and approved by UCLA Institutional Review Board (#20-000473) and Sharp Healthcare Institutional Review Board (#2012806). The patients/participants provided their written informed consent to participate in this study.
Author contributions
BL and JF conceived of the study and BL, JF, and ER designed the study. KF, AR, OY, GA contributed to overall cohort design, sample processing, and funding support. JS contributed to study recruitment and implementation. YZ, DG, and JF performed analyses. BL, JF, and ER wrote the manuscript. All authors contributed to the article and approved the submitted version.
Funding
This work was supported by the UCLA David Geffen School of Medicine – Eli and Edythe Broad Center of Regenerative Medicine and Stem Cell Research Award to JF and Sharp HealthCare Foundation to BL. JF is also supported in part by the Doris Duke Foundation award #2019086.
Acknowledgments
We are grateful to the study participants for their willingness to participate in this research. We would also like to thank MacKenzie Habib (Sharp HealthCare), Claudia Perdomo (UCLA), and Adreanne Rivera (UCLA) for study coordination.
Conflict of interest
The authors declare that the research was conducted in the absence of any commercial or financial relationships that could be construed as a potential conflict of interest.
Publisher’s note
All claims expressed in this article are solely those of the authors and do not necessarily represent those of their affiliated organizations, or those of the publisher, the editors and the reviewers. Any product that may be evaluated in this article, or claim that may be made by its manufacturer, is not guaranteed or endorsed by the publisher.
Supplementary material
The Supplementary Material for this article can be found online at: https://www.frontiersin.org/articles/10.3389/fimmu.2023.1197326/full#supplementary-material
References
1. Huang C, Wang Y, Li X, Ren L, Zhao J, Hu Y, et al. Clinical features of patients infected with 2019 novel coronavirus in wuhan, China. Lancet (2020) 395(10223):497–506. doi: 10.1016/S0140-6736(20)30183-5
2. Wilk AJ, Lee MJ, Wei B, Parks B, Pi R, Martinez-Colon GJ, et al. Multi-omic profiling reveals widespread dysregulation of innate immunity and hematopoiesis in COVID-19. J Exp Med (2021) 218(8):e20210582. doi: 10.1101/2020.12.18.423363
3. Bhimraj A, Morgan RL, Shumaker AH, Lavergne V, Baden L, Cheng VC, et al. Infectious diseases society of America guidelines on the treatment and management of patients with COVID-19. Infectious Diseases Society of America (2023); Version 10.1.0. Available at https://www.idsociety.org/practice-guideline/covid-19-guideline-treatment-and-management/. Accessed 3 November 2022.
4. Ackermann M, Verleden SE, Kuehnel M, Haverich A, Welte T, Laenger F, et al. Pulmonary vascular endothelialitis, thrombosis, and angiogenesis in covid-19. N Engl J Med (2020) 383(2):120–8. doi: 10.1056/NEJMoa2015432
5. Bryce C, Grimes Z, Pujadas E, Ahuja S, Beasley MB, Albrecht R, et al. Pathophysiology of SARS-CoV-2: the mount Sinai COVID-19 autopsy experience. Mod Pathol (2021) 34(8):1456–67. doi: 10.1038/s41379-021-00793-y
6. Chappell MC. Angiotensin-converting enzyme 2 autoantibodies: further evidence for a role of the renin-angiotensin system in inflammation. Arthritis Res Ther (2010) 12(3):128. doi: 10.1186/ar3052
7. Takahashi Y, Haga S, Ishizaka Y, Mimori A. Autoantibodies to angiotensin-converting enzyme 2 in patients with connective tissue diseases. Arthritis Res Ther (2010) 12(3):R85. doi: 10.1186/ar3012
8. Fujieda M, Oishi N, Kurashige T. Antibodies to endothelial cells in Kawasaki disease lyse endothelial cells without cytokine pretreatment. Clin Exp Immunol (1997) 107(1):120–6. doi: 10.1046/j.1365-2249.1997.d01-894.x
9. Grunebaum E, Blank M, Cohen S, Afek A, Kopolovic J, Meroni PL, et al. The role of anti-endothelial cell antibodies in Kawasaki disease - in vitro and in vivo studies. Clin Exp Immunol (2002) 130(2):233–40. doi: 10.1046/j.1365-2249.2002.02000.x
10. Dragun D, Muller DN, Brasen JH, Fritsche L, Nieminen-Kelha M, Dechend R, et al. Angiotensin II type 1-receptor activating antibodies in renal-allograft rejection. N Engl J Med (2005) 352(6):558–69. doi: 10.1056/NEJMoa035717
11. Jobert A, Rao N, Deayton S, Bennett GD, Brealey J, Nolan J, et al. Angiotensin II type 1 receptor antibody precipitating acute vascular rejection in kidney transplantation. Nephrol (Carlton) (2015) 20 Suppl 1:10–2. doi: 10.1111/nep.12421
12. Philogene MC, Bagnasco S, Kraus ES, Montgomery RA, Dragun D, Leffell MS, et al. Anti-angiotensin II type 1 receptor and anti-endothelial cell antibodies: a cross-sectional analysis of pathological findings in allograft biopsies. Transplantation (2017) 101(3):608–15. doi: 10.1097/TP.0000000000001231
13. Crespo M, Llinas-Mallol L, Redondo-Pachon D, Butler C, Gimeno J, Perez-Saez MJ, et al. Non-HLA antibodies and epitope mismatches in kidney transplant recipients with histological antibody-mediated rejection. Front Immunol (2021) 12:703457. doi: 10.3389/fimmu.2021.703457
14. Chan AP, Guerra MR, Rossetti M, Hickey MJ, Venick RS, Marcus EA, et al. Non-HLA AT1R antibodies are highly prevalent after pediatric intestinal transplantation. Pediatr Transplant (2021) 25(3):e13987. doi: 10.1111/petr.13987
15. Wozniak LJ, Hickey MJ, Chan AP, Venick RS, Farmer DG, Busuttil RW, et al. Angiotensin II type-1 receptor antibodies are associated with active allograft dysfunction following pediatric liver transplantation. Transplantation (2020) 104(12):2547–56. doi: 10.1097/TP.0000000000003206
16. Pearl MH, Grotts J, Rossetti M, Zhang Q, Gjertson DW, Weng P, et al. Cytokine profiles associated with angiotensin II type 1 receptor antibodies. Kidney Int Rep (2019) 4(4):541–50. doi: 10.1016/j.ekir.2018.12.011
17. Pearl MH, Zhang Q, Palma Diaz MF, Grotts J, Rossetti M, Elashoff D, et al. Angiotensin II type 1 receptor antibodies are associated with inflammatory cytokines and poor clinical outcomes in pediatric kidney transplantation. Kidney Int (2018) 93(1):260–9. doi: 10.1016/j.kint.2017.06.034
18. Pearl MH, Chen L, ElChaki R, Elashoff D, Gjertson DW, Rossetti M, et al. Endothelin type a receptor antibodies are associated with angiotensin II type 1 receptor antibodies, vascular inflammation, and decline in renal function in pediatric kidney transplantation. Kidney Int Rep (2020) 5(11):1925–36. doi: 10.1016/j.ekir.2020.09.004
19. Miedema J, Schreurs M, van der Sar-van der Brugge S, Paats M, Baart S, Bakker M, et al. Antibodies against angiotensin II receptor type 1 and endothelin a receptor are associated with an unfavorable COVID19 disease course. Front Immunol (2021) 12:84142. doi: 10.3389/fimmu.2021.684142
20. Rodriguez-Perez AI, Labandeira CM, Pedrosa MA, Valenzuela R, Suarez-Quintanilla JA, Cortes-Ayaso M, et al. Autoantibodies against ACE2 and angiotensin type-1 receptors increase severity of COVID-19. J Autoimmun (2021) 122:102683. doi: 10.1016/j.jaut.2021.102683
21. Jiang Y, Duffy F, Hadlock J, Raappana A, Styrchak S, Beck I, et al. Angiotensin II receptor I auto-antibodies following SARS-CoV-2 infection. PloS One (2021) 16(11):e0259902. doi: 10.1371/journal.pone.0259902
22. Papola F, Biancofiore V, Angeletti C, Grimaldi A, Carucci AC, Cofini V, et al. Anti-AT1R autoantibodies and prediction of the severity of covid-19. Hum Immunol (2022) 83(2):130–3. doi: 10.1016/j.humimm.2021.10.006
23. Briquez PS, Rouhani SJ, Yu J, Pyzer AR, Trujillo J, Dugan HL, et al. Severe COVID-19 induces autoantibodies against angiotensin II that correlate with blood pressure dysregulation and disease severity. Sci Adv (2022) 8(40):eabn3777. doi: 10.1126/sciadv.abn3777
24. Wang EY, Mao T, Klein J, Dai Y, Huck JD, Jaycox JR, et al. Diverse functional autoantibodies in patients with COVID-19. Nature (2021) 595(7866):283–8. doi: 10.1038/s41586-021-03631-y
25. Chang SE, Feng A, Meng W, Apostolidis SA, Mack E, Artandi M, et al. New-onset IgG autoantibodies in hospitalized patients with COVID-19. Nat Commun (2021) 12(1):5417. doi: 10.1038/s41467-021-25509-3
26. Liu Y, Ebinger JE, Mostafa R, Budde P, Gajewski J, Walker B, et al. Paradoxical sex-specific patterns of autoantibody response to SARS-CoV-2 infection. J Transl Med (2021) 19(1):524. doi: 10.1186/s12967-021-03184-8
27. Zuo Y, Yalavarthi S, Navaz SA, Hoy CK, Harbaugh A, Gockman K, et al. Autoantibodies stabilize neutrophil extracellular traps in COVID-19. JCI Insight (2021) 6(15):e150111. doi: 10.1172/jci.insight.150111
28. Bastard P, Rosen LB, Zhang Q, Michailidis E, Hoffmann HH, Zhang Y, et al. Autoantibodies against type I IFNs in patients with life-threatening COVID-19. Science (2020) 370(6515):eabd4585. doi: 10.1126/science.abd4585
29. Beigel JH, Tomashek KM, Dodd LE, Mehta AK, Zingman BS, Kalil AC, et al. Remdesivir for the treatment of covid-19 - final report. N Engl J Med (2020) 383(19):1813–26. doi: 10.1056/NEJMoa2007764
30. Zhang Q, Cecka JM, Gjertson DW, Ge P, Rose ML, Patel JK, et al. HLA and MICA: targets of antibody-mediated rejection in heart transplantation. Transplantation (2011) 91(10):1153–8. doi: 10.1097/TP.0b013e3182157d60
31. Butler CL, Hickey MJ, Jiang N, Zheng Y, Gjertson D, Zhang Q, et al. Discovery of non-HLA antibodies associated with cardiac allograft rejection and development and validation of a non-HLA antigen multiplex panel: from bench to bedside. Am J Transplant (2020) 20(10):2768–80. doi: 10.1111/ajt.15863
32. Tomer Y, Shoenfeld Y. Ageing and autoantibodies. Autoimmunity (1988) 1(2):141–9. doi: 10.3109/08916938809001927
33. Guo L, Ren L, Yang S, Xiao M, Chang D, Yang F, et al. Profiling early humoral response to diagnose novel coronavirus disease (COVID-19). Clin Infect Dis (2020) 71(15):778–85. doi: 10.1093/cid/ciaa310
34. Zhao J, Yuan Q, Wang H, Liu W, Liao X, Su Y, et al. Antibody responses to SARS-CoV-2 in patients with novel coronavirus disease 2019. Clin Infect Dis (2020) 71(16):2027–34. doi: 10.1093/cid/ciaa344
35. Long QX, Liu BZ, Deng HJ, Wu GC, Deng K, Chen YK, et al. Antibody responses to SARS-CoV-2 in patients with COVID-19. Nat Med (2020) 26(6):845–8. doi: 10.1038/s41591-020-0897-1
36. Kerr JR. The role of parvovirus B19 in the pathogenesis of autoimmunity and autoimmune disease. J Clin Pathol (2016) 69(4):279–91. doi: 10.1136/jclinpath-2015-203455
37. Yeung WC, Rawlinson WD, Craig ME. Enterovirus infection and type 1 diabetes mellitus: systematic review and meta-analysis of observational molecular studies. BMJ (2011) 342:d35. doi: 10.1136/bmj.d35
38. Bjornevik K, Cortese M, Healy BC, Kuhle J, Mina MJ, Leng Y, et al. Longitudinal analysis reveals high prevalence of Epstein-Barr virus associated with multiple sclerosis. Science (2022) 375(6578):296–301. doi: 10.1126/science.abj8222
39. Raza K, Mullazehi M, Salmon M, Buckley CD, Rönnelid J. Anti-collagen type II antibodies in patients with very early synovitis. Ann Rheum Dis (2008) 67(9):1354–5. doi: 10.1136/ard.2007.084277
40. Terrier B, Degand N, Guilpain P, Servettaz A, Guillevin L, Mouthon L. Alpha-enolase: a target of antibodies in infectious and autoimmune diseases. Autoimmun Rev (2007) 6(3):176–82. doi: 10.1016/j.autrev.2006.10.004
41. Kardol-Hoefnagel T, Otten HG. A comprehensive overview of the clinical relevance and treatment options for antibody-mediated rejection associated with non-HLA antibodies. Transplantation (2021) 105(7):1459–70. doi: 10.1097/TP.0000000000003551
42. Kendall EK, Olaker VR, Kaelber DC, Xu R, Davis PB. Association of SARS-CoV-2 infection with new-onset type 1 diabetes among pediatric patients from 2020 to 2021. JAMA Netw Open (2022) 5(9):e2233014. doi: 10.1001/jamanetworkopen.2022.33014
43. Chang R, Yen-Ting Chen T, Wang SI, Hung YM, Chen HY, Wei CJ. Risk of autoimmune diseases in patients with COVID-19: a retrospective cohort study. EClinicalMedicine (2023) 56:101783. doi: 10.1016/j.eclinm.2022.101783
Keywords: COVID-19, autoantibody, angiotensin II receptor type 1 (AT1R), Non-HLA antigens, Anti-endothelial antibodies
Citation: Lichtenstein B, Zheng Y, Gjertson D, Ferbas KG, Rimoin AW, Yang OO, Aldrovandi GM, Schaenman JM, Reed EF and Fulcher JA (2023) Vascular and Non-HLA autoantibody profiles in hospitalized patients with COVID-19. Front. Immunol. 14:1197326. doi: 10.3389/fimmu.2023.1197326
Received: 30 March 2023; Accepted: 23 May 2023;
Published: 15 June 2023.
Edited by:
Pier Paolo Sainaghi, University of Eastern Piedmont, ItalyReviewed by:
Carsten Grötzinger, Charité University Medicine Berlin, GermanyRobert Markewitz, Institute of Clinical Chemistry, Germany
Copyright © 2023 Lichtenstein, Zheng, Gjertson, Ferbas, Rimoin, Yang, Aldrovandi, Schaenman, Reed and Fulcher. This is an open-access article distributed under the terms of the Creative Commons Attribution License (CC BY). The use, distribution or reproduction in other forums is permitted, provided the original author(s) and the copyright owner(s) are credited and that the original publication in this journal is cited, in accordance with accepted academic practice. No use, distribution or reproduction is permitted which does not comply with these terms.
*Correspondence: Jennifer A. Fulcher, jfulcher@mednet.ucla.edu