- 1Department of Obstetrics and Fetal Medicine, University Medical Center Hamburg-Eppendorf, Hamburg, Germany
- 2Department of Immunobiology, Yale School of Medicine, New Haven, CT, United States
- 3Department of Obstetrics, Gynecology, and Reproductive Sciences, Yale School of Medicine, New Haven, CT, United States
- 4Gene Therapy Laboratory, Instituto de Investigaciones en Medicina Traslacional, Consejo Nacional de Investigaciones Científicas y Técnicas (CONICET) - Universidad Austral, Buenos Aires, Argentina
- 5Section of Infectious Diseases, Department of Internal Medicine, Yale School of Medicine, Yale University, New Haven, CT, United States
- 6Department of Pathology, Uniformed Services University of the Health Sciences, Bethesda, MD, United States
The severe acute respiratory syndrome coronavirus 2 (SARS-CoV-2) pandemic imposed a risk of infection and disease in pregnant women and neonates. Successful pregnancy requires a fine-tuned regulation of the maternal immune system to accommodate the growing fetus and to protect the mother from infection. Galectins, a family of β-galactoside–binding proteins, modulate immune and inflammatory processes and have been recognized as critical factors in reproductive orchestration, including maternal immune adaptation in pregnancy. Pregnancy-specific glycoprotein 1 (PSG1) is a recently identified gal-1 ligand at the maternal–fetal interface, which may facilitate a successful pregnancy. Several studies suggest that galectins are involved in the immune response in SARS-CoV-2–infected patients. However, the galectins and PSG1 signature upon SARS-CoV-2 infection and vaccination during pregnancy remain unclear. In the present study, we examined the maternal circulating levels of galectins (gal-1, gal-3, gal-7, and gal-9) and PSG1 in pregnant women infected with SARS-CoV-2 before vaccination or uninfected women who were vaccinated against SARS-CoV-2 and correlated their expression with different pregnancy parameters. SARS-CoV-2 infection or vaccination during pregnancy provoked an increase in maternal gal-1 circulating levels. On the other hand, levels of PSG1 were only augmented upon SARS-CoV-2 infection. A healthy pregnancy is associated with a positive correlation between gal-1 concentrations and gal-3 or gal-9; however, no correlation was observed between these lectins during SARS-CoV-2 infection. Transcriptome analysis of the placenta showed that gal-1, gal-3, and several PSG and glycoenzymes responsible for the synthesis of gal-1-binding glycotopes (such as linkage-specific N-acetyl-glucosaminyltransferases (MGATs)) are upregulated in pregnant women infected with SARS-CoV-2. Collectively, our findings identify a dynamically regulated “galectin-specific signature” that accompanies the SARS-CoV-2 infection and vaccination in pregnancy, and they highlight a potentially significant role for gal-1 as a key pregnancy protective alarmin during virus infection.
1 Introduction
Severe acute respiratory syndrome coronavirus 2 (SARS-CoV-2) infection poses a particular risk to pregnant women and their neonates, in which pregnant women have higher rates of severe coronavirus disease 2019 (COVID-19) disease than non-pregnant adults (1). Pregnant women experience immunologic and physiologic changes, such as swelling of the respiratory tract and restricted lung expansion, that make them less tolerant to viral respiratory infections, especially in the last trimester (2, 3). Maternal SARS-CoV-2 infection affects pregnancy outcomes, with increased incidence rates of intensive care admission, invasive ventilation, maternal death, iatrogenic preterm birth, and stillbirth (4–12). Furthermore, comorbidities such as obesity, diabetes, heart disease, advanced maternal age, or a lack of vaccination increase the risk of severe COVID-19 symptoms (3, 11). A recent study revealed no association between the gestational age at infection and COVID-19 morbidity and mortality, suggesting that a previously reported increase in morbidity and mortality in the third trimester may be attributable to other gestational age–affected variables for which adjustment was made in this study (4, 13). Therefore, there is a continuing challenge for clinicians to manage the SARS-CoV-2 infection during pregnancy (1, 9). In this regard, SARS-CoV-2 vaccines have shown efficacy in preventing symptomatic maternal illness and are considered safe for both the mother and infant (8, 9, 14–16). SARS-CoV-2 antibodies have been documented in umbilical cord blood and breast milk after maternal vaccination, suggesting protection for the fetus (9, 14, 17–23). On the basis of the growing evidence that supports the safety and efficacy of COVID-19 vaccination in pregnancy (8, 9, 15–25), most countries recommend full COVID-19 vaccination, regardless of pregnancy trimester.
Galectins are a family of endogenous carbohydrate-binding proteins characterized by a unique sequence motif in their carbohydrate recognition domain (CRD), with the ability to bind β-galactosidase residues (6, 26–28). Galectins are classified into three major types: prototype, which contain one CRD and form homodimers (e.g., gal-1 and gal-7); chimera containing a C-terminal CRD and a proline- and glycine-rich N terminal tail that mediate their oligomerization (gal-3); and tandem repeats that have two different CRDs in tandem connected by a linker of up to 70 amino acids (e.g., gal-9). Galectins contribute to healthy gestation by modulating multiple immune and inflammatory processes (6). In normal pregnancy, circulating maternal gal-1 levels increase from the first trimester, peaking during the second trimester, and remain similar until term (28). Being less abundant, upregulation of gal-3, gal-7, and gal-9 levels in maternal circulation occurs mainly in the second trimester (26, 27). In the extracellular compartment, galectins bind to the glycans decorating glycoproteins. One identified gal-1 ligand at the maternal–fetal interface is pregnancy-specific glycoprotein 1 (PSG1); glycans in the N- and A2 domains of PSG1 mediate the interaction between these two molecules (29). PSGs belong to the carcinoembryonic antigen family within the immunoglobulin (Ig) superfamily and are secreted to the maternal circulation by trophoblast cells (30, 31). PSG1 is one of 10 PSGs and is considered one of the most abundant trophoblastic proteins in maternal circulation during the third trimester of pregnancy (31). PSG1 interacts with soluble and membrane-bound ligands and participates in processes required for successful pregnancy (29). Functional studies with recombinant PSG1 showed that this protein has pro-angiogenic activity; inhibits the interaction of fibrinogen with platelets; and regulates extravillous trophoblast adhesion, migration, and invasion (32–34). In addition, PSG1 activates the latent form of the anti-inflammatory cytokines Transforming growth factor β (TGF-β1) and (TGF-β2) by binding to the respective latent associated peptides contributing to the establishment of maternal immune tolerance (30, 35, 36). Dynamic changes in PSG1 maternal circulation levels during virus infection remain unexplored.
A growing body of clinical data suggests that the cytokine release syndrome is one of the main reasons for the high mortality observed in COVID-19 patients (37). Changes in the cytokine profile of pregnant women correlate with the clinical severity of patients with COVID-19 (10, 38). Recent studies revealed that circulating gal-1, gal-3, and gal-9 levels are increased in non-pregnant patients with COVID-19 (39–43). Levels of gal-3 and gal-9 were reported to be upregulated only in patients with severe disease (40, 41), raising the possibility that circulating gal-3 or gal-9 can be valuable biomarkers for severe pneumonia in patients with COVID-19 (44, 45). Interestingly, exogenous gal-9 administration during acute SARS-CoV-2 infection has been reported to increase the survival rate inducing a robust innate and adaptive immune response in mice (46). More recently, an inhaled gal-3 inhibitor has been tested as a potential therapy for COVID-19 pneumonitis (47). However, despite considerable progress in dissecting the functions of individual members of the galectin family, there is no comprehensive study of the galectin signature in the maternal circulation and placenta following SARS-CoV-2 infection and vaccination during pregnancy.
Therefore, we aimed to determine the galectin fingerprint in the maternal and placental compartment during COVID-19 disease and SARS-CoV-2 vaccination in pregnancy. Our findings indicate that gal-1 is uniquely upregulated at the maternal circulation during SARS-CoV-2 infection and following vaccination. These results underscore the importance of gal-1 as a key pregnancy protective alarmin during SARS-CoV-2 infection. In addition, we observed that the concentration of PSG1, a gal-1 ligand, was increased following infection, providing the first indication of potential regulation of this trophoblast-derived protein in response to insults to maternal health.
2 Material and methods
2.1 Participants and data collection: human subjects for the study of SARS-CoV-2 infection and vaccination during pregnancy
For the current study, we used two pregnancy cohorts as follows.
2.1.1 PRINCE cohort
The PRINCE study is a longitudinal prospective cohort of pregnant women and their children located at the University Medical Center Hamburg-Eppendorf. This cohort aims to identify prenatal factors influencing maternal and children’s future immune development and health. Women were included if they were at least 18 years old, were not expecting twins, and were between 12th and 14th ± 6 weeks of pregnancy with regular checking up by a specialized obstetrician. They were excluded if the pregnancy was conceived medically assisted, an autoimmune disorder was diagnosed, or fetal pathologies were observed. The PRINCE COVID cohort was established in March 2020 and recruited pregnant women infected with SARS-CoV-2 at any point during pregnancy. The third cohort, PRINCE VACCINE, included SARS-CoV-2–negative women vaccinated twice within an interval of 6 weeks with 30 μg of BNT162b2 messenger RNA COVID-19 vaccine during pregnancy. All study subjects signed informed consent forms, and the ethics committee of the Hamburg Chamber of Physicians (Ärztekammer Hamburg) approved the study protocol under the registration numbers PV3694 (PRINCE), PV 7312-4710 (PRINCE COVID), and 2021-10647-BO-ff (PRINCE VACCINE).
2.1.2 Yale IMPACT cohort
In the Yale study, women who were in labor at Yale New Haven Hospital from 27 March 2020 to 1 June 2020 and tested positive for SARS-CoV-2 by nasopharyngeal (NP) swab Quantitative reverse transcription polymerase chain reaction (RT-qPCR) were recruited to the Yale IMPACT study. These participants provided informed consent for research studies of donated placental tissue and blood. The placenta and blood of the control group were selected from SARS-CoV-2–uninfected women (as determined by negative RT-qPCR testing of NP swab) at Yale New Haven Hospital with matching maternal age, gestational age, and maternal comorbidities to the COVID-19 placental cases, recruited during the same months as the SARS-CoV-2–infected participants. They also provided informed consent to serve as uninfected controls for transcriptomic studies. The study was approved by the Yale Institutional Review Board (protocol #2000027690).
2.2 Determination of circulating galectins and PSG1 levels
For both PRINCE and Yale IMPACT studies, blood was collected in serum separator tubes, and serum was aliquoted and stored immediately at −80°C for further analysis. Human galectins and PSG1 levels were measured in the serum by enzyme-linked immunosorbent assay (ELISA) as previously described (26, 28). Briefly, Corning® 96-Well High-Binding (Fisher Scientific) was coated overnight with either polyclonal anti-human gal-1, gal-3, gal-7, gal-9, or anti-PSG1 antibodies (500-P210; PeproTech, AF 1154, 842118, AF 2045, and DY6799-05; R&D Systems, USA, respectively) and washed with washing buffer. Plates were blocked with 1%–2% Bovine serum albumin (BSA) in Phosphate-buffered saline (PBS). Individual wells were incubated with serial dilutions of galectins or PSG1 standards or serum samples for 1–2 h at room temperature (RT). Wells were washed and incubated with biotinylated polyclonal anti-human galectins or PSG1 antibodies (500-P210; PeproTech, AF 1154, 842118, AF 2045, and DY6799-05; R&D Systems, USA, respectively). Plates were washed three to six times and incubated with horseradish peroxidase–conjugated streptavidin (189733; Calbiochem, USA). After three to eight additional washes, a colorimetric reaction was developed with the 3,3,5,5′-tetramethyl benzidine. The reaction was stopped by adding one volume of 4 N H2SO4, and absorbance at 450 nm was recorded.
2.3 RNA sequencing data analysis
Bulk RNA sequencing analysis was performed on placental RNA sequencing data previously described (48). For the present study, FASTQ files were normalized with Kallisto v0.46.188 using the “-b 100 and -t 20” options to obtain transcript abundances in transcript per million (TPM). Expression data for our selected genes were downloaded, and differential expression analysis was performed using the Welch’s t-test. Genes were considered differentially expressed if P-value < 0.05 (Supplementary Table 1). The fold change in gene expression was represented with Z-score after being calculated from TPM values and visualized as a heatmap.
2.4 Statistical analyses
GraphPad PRISM version 9 and R Statistical Software were utilized for statistical analysis. Correlations between the serum galectin levels and the clinical parameters were conducted using Spearman’s correlation analysis. Statistical parameters, including sample sizes and dispersion, are reported in the figures and their legends. Statistical difference between two groups was determined by an unpaired, two tailed t-test. One-way analysis of variance with Bonferroni or Dunn’s multiple comparisons was used to compare different groups. If data did not meet test prerequisites, equivalent non-parametric tests were utilized. Data were considered to be statistically significant if P ≤ 0.05.
3 Results
3.1 Clinical characteristics of patients in the PRINCE COVID cohort
To explore the impact of SARS-CoV-2 infection or vaccination in the maternal galectin profile, women from the PRINCE COVID cohort were grouped as follows: pregnant women who were tested positive for SARS-CoV-2 by NP swab qRT-PCR any time during pregnancy, matched control pregnant women tested negative for SARS-CoV-2 during the whole gestation, pregnant women who received two doses of vaccination, and matched control vaccinated non-pregnant women. Most of SARS-CoV-2 infections (75%, 15 of 20) were diagnosed during the second trimester of pregnancy, and all these patients had a negative viral test (NP swab qRT-PCR) at the time of delivery. Of the 30 patients, 24 (80%) received their first vaccination dose in the second trimester. Maternal and neonatal characteristics are summarized in Table 1. No severe COVID-19 disease (intensive care unit stay or administration of supplemental oxygen required) was observed in the PRINCE COVID cohort. All pregnancies included in the cohort resulted in live births, with no complications related to SARS-CoV-2 infection or vaccination. There were no significant differences among healthy pregnant women, pregnant women with COVID-19, and pregnant women who were vaccinated in terms of maternal age, body mass index (BMI), gestational age, mode of delivery, neonatal outcomes, or comorbidities.
3.2 Maternal circulating gal-1 levels increase with SARS-CoV-2 infection or vaccination in pregnant women
We first measured the circulating gal-1, gal-3, gal-7, and gal-9 levels of SARS-CoV-2–infected pregnant women, uninfected and unvaccinated healthy pregnant women, pregnant women who were vaccinated against SARS-CoV-2, and healthy vaccinated women who were not pregnant in the PRINCE cohort. Our results showed that circulating gal-1 levels were increased in pregnant women with antecedent SARS-CoV-2 infection (P-value = 0.0185) and those who were vaccinated against SARS-CoV-2 (P-value = 0.0054) compared with control healthy unvaccinated pregnant patients (Figure 1A). We further observed that vaccinated women displayed higher levels of circulating gal-1 (P-value = 0.0313) if they were pregnant (Figure 1A), whereas they had similar levels of gal-3 in all the compared groups (Figure 1B). In addition, no differences were observed in the circulating levels of gal-7 and gal-9 between healthy pregnant women and the SARS-CoV-2 previously infected pregnant women (Figures 1C, D). Increased gal-9 levels in circulation were noticed in vaccinated pregnant women compared with the ones who were not vaccinated (P-value = 0.0243, Figure 1D). Furthermore, pregnant vaccinated women presented higher serum levels of gal-7 (P-value < 0.0001) and lower concentrations of gal-9 (P-value = 0.0015) compared with non-pregnant vaccinated group (Figures 1C, D).
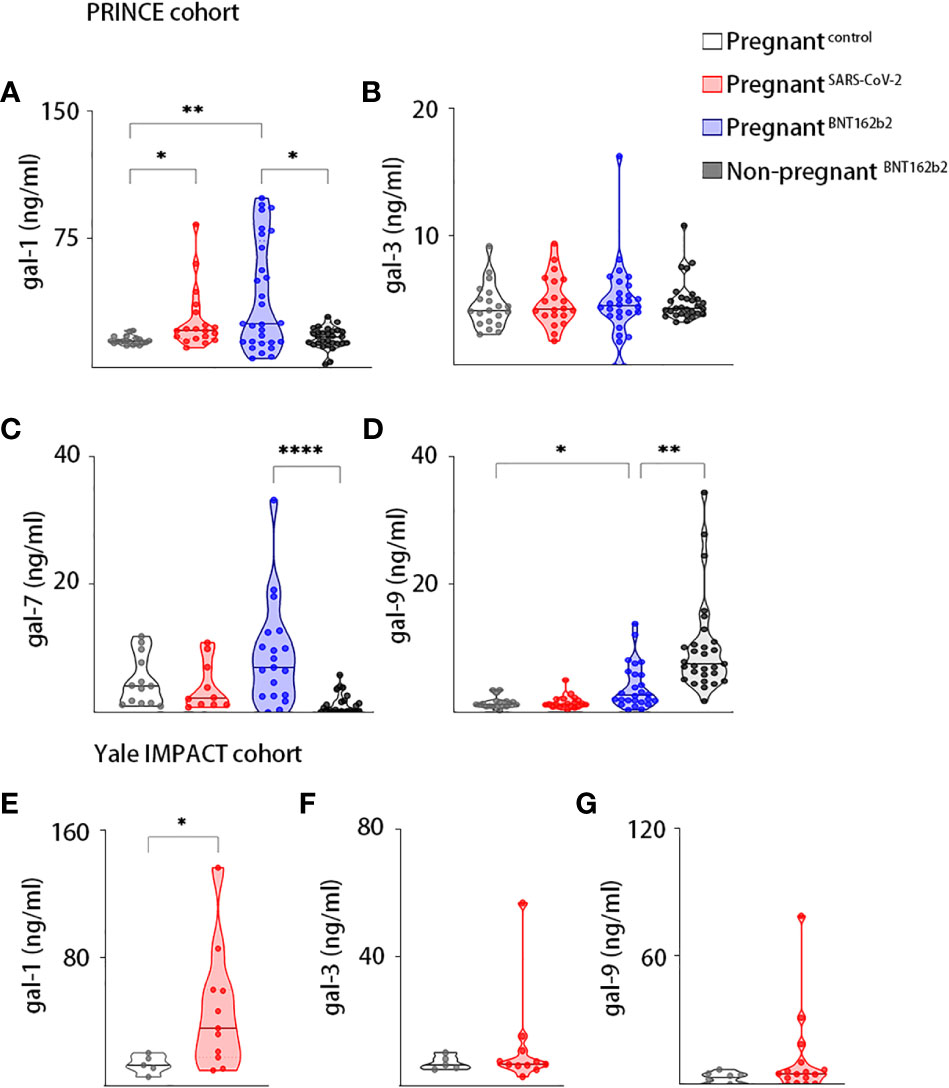
Figure 1 Galectins dynamics upon SARS-Cov-2 infection or vaccination during pregnancy. Maternal circulating levels of gal-1 (A), gal-3 (B), gal-7 (C), and gal-9 (D) in the PRINCE cohort analyzed by ELISA in healthy pregnant women (pregnantcontrol, n = 20), pregnant women infected by SARS-CoV-2 (pregnantSARS-CoV-2, n = 20), pregnant women vaccinated against SARS-CoV-2 (pregnantBNT162b2, n = 30), and non-pregnant vaccinated women (non-pregnantBNT162b2, n = 30). Circulating levels of gal-1 (E), gal-3 (F), and gal-9 (G) in the Yale IMPACT cohort analyzed by ELISA in healthy pregnant women (pregnantcontrol, n = 5) or pregnant women infected by SARS-CoV-2 (pregnantSARS-CoV-2, n = 11). *P < 0.05, **P < 0.01, and ****P < 0.0001 as analyzed by Kruskal–Wallis test or Welch’s t-test. In all figures, circulating levels of galectins and PSG1 were determined in triplicate for each serum sample.
3.3 Yale IMPACT cohort studies
We next sought to assess the galectins/PSG1 signature in acute SARS-CoV-2 infection during pregnancy. Table 2 describes the clinical features of the Yale IMPACT cohort, which were previously described (48). In addition, SARS-CoV-2–uninfected women (as determined by negative RT-qPCR testing of NP swab) were recruited as control. Among SARS-CoV-2–infected women, 45.5% (5 of 11) had symptomatic COVID-19, and two cases of severe COVID-19 disease required supplemental oxygen administration. All pregnancies resulted in live births, with a median Apgar score of 9 (range, 4–9). No significant differences existed between cases and matched controls for maternal age, gestational age, demographics, and neonatal outcomes. However, the groups differed in rates of gestational hypertension (45.5% in infected versus 0% in uninfected), preeclampsia (36.4% versus 0%), and diabetes (18.2% versus 0%).
In the Yale IMPACT cohort, we analyzed the circulating levels of gal-1, gal-3, and gal-9 in SARS-CoV-2–infected pregnant women and healthy pregnant controls. We found significantly higher levels of gal-1 in the serum of patients with COVID-19 compared with healthy pregnant controls (P-value = 0.0156, Figure 1E). However, no significant differences were observed in the circulating levels of gal-3 and gal-9 among both groups (Figures 1F, G).
3.4 Correlation of maternal gal-1, gal-3, and gal-9 levels is altered following SARS-CoV-2 infection
We performed correlation analysis to explore the dynamics of galectin levels at the maternal circulation. During a healthy pregnancy, we found a significant positive correlation between gal-1 and gal-3 [P-value = 0.0331, Spearman correlation coefficient (ρ) = 0.478; Figure 2A] and between gal-1 and gal-9 (P-value = 0.0083, ρ = 0.571; Figure 2B). SARS-CoV-2 infection resulted in the loss of the observed correlation of gal-1 with both gal-3 and gal-9 (Figures 2A, B). On the other hand, vaccination only compromised the correlation between gal-1 and gal-9 (Figure 2B), whereas the correlation between gal-1 and gal-3 was maintained (P-value = 0.0433, ρ = 0.468; Figure 2A). No significant correlation was found between gal-1 and gal-7 in any of the analyzed groups (Figure 2C). Our results also demonstrated that gal-3 was positively associated with the levels of gal-9 in healthy pregnant women (P-value = 0.0103, ρ = 0.562; Figure 2D) and that this correlation was still present in the SARS-CoV-2–infected (P-value = 0.0010, ρ = 0.666; Figure 2D) and the SARS-CoV-2 vaccinated individuals (P-value = 0.0013, ρ = 0.704; Figure 2D).
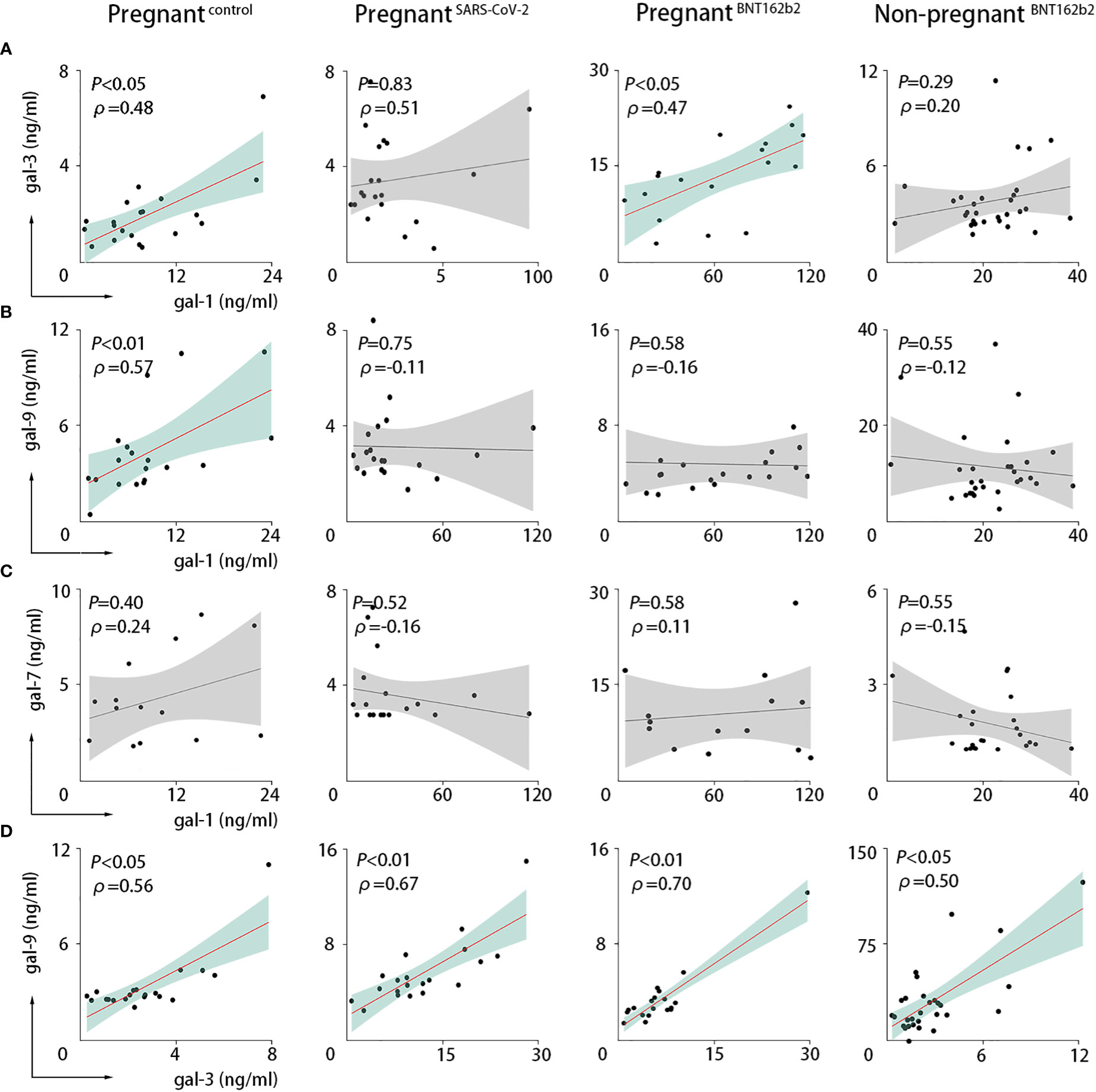
Figure 2 SARS-CoV-2 infection or vaccination altered the maternal circulating levels. Correlations in PRINCE cohort between serum values of gal-1 and gal-3 (A), gal-1 and gal-9 (B), gal-1 and gal-7 (C), or gal-3 and gal-9 (D). Pregnantcontrol, healthy pregnant women (n = 20); pregnantSARS-CoV-2, pregnant women infected by SARS-CoV-2 (n = 20); pregnantBNT162b2, pregnant women vaccinated against SARS-CoV-2 (n = 30); non-pregnantBNT162b2, non-pregnant vaccinated women (n = 30). The Spearman correlation coefficient (ρ) is shown. P < 0.05 was considered statistically significant (green) and P > 0.05 not significant (gray) as analyzed with the Spearman statistical test.
Further correlation studies between galectins and clinical features in the PRINCE cohort were performed by analyzing general clinical parameters, including BMI, age, parity, gestational week of delivery, and the birth weight and height of the neonate. In addition, for each experimental group, we considered the time of infection (gestational week and trimester) and the gestational week in which women received the first and second doses of vaccination. Our results showed that the BMI of the patients was positively associated with gal-1 levels in maternal circulation (P < 0.0001, ρ = 0.439; Table 3) and gal-3 levels (P = 0.0241, ρ = 0.240; Table 3), and the age of the patients inversely correlated with gal-9 levels (P = 0.0141, ρ = −0.267; Table 3). In addition, when we analyzed the correlation between galectins and clinical parameters in the Yale IMPACT cohort, our results showed that gal-1 was negatively associated with the parity of the pregnant women (P = 0.0029, ρ = −0.844; Table 4).
3.5 COVID-19 infection during pregnancy influences the PSG1 levels in maternal circulation
Because PSG1 is one of the most abundant trophoblastic proteins in maternal serum in the third trimester and binds gal-1 (29), we further examined the PSG1 levels in maternal circulation. Our results in the PRINCE cohort showed that pregnant women infected with SARS-CoV-2 displayed increased levels of PSG1 compared with non-infected pregnant women. A non-significant increase was observed in the vaccinated pregnant women (Figure 3A). However, we did not find any correlation between PSG1 and gal-1 (Figures 3C–E). The measurement of PSG1 levels in the Yale IMPACT cohort demonstrated similar circulating levels of PSG1 between control pregnant women and pregnant women with SARS-CoV-2 infection (Figure 3B).
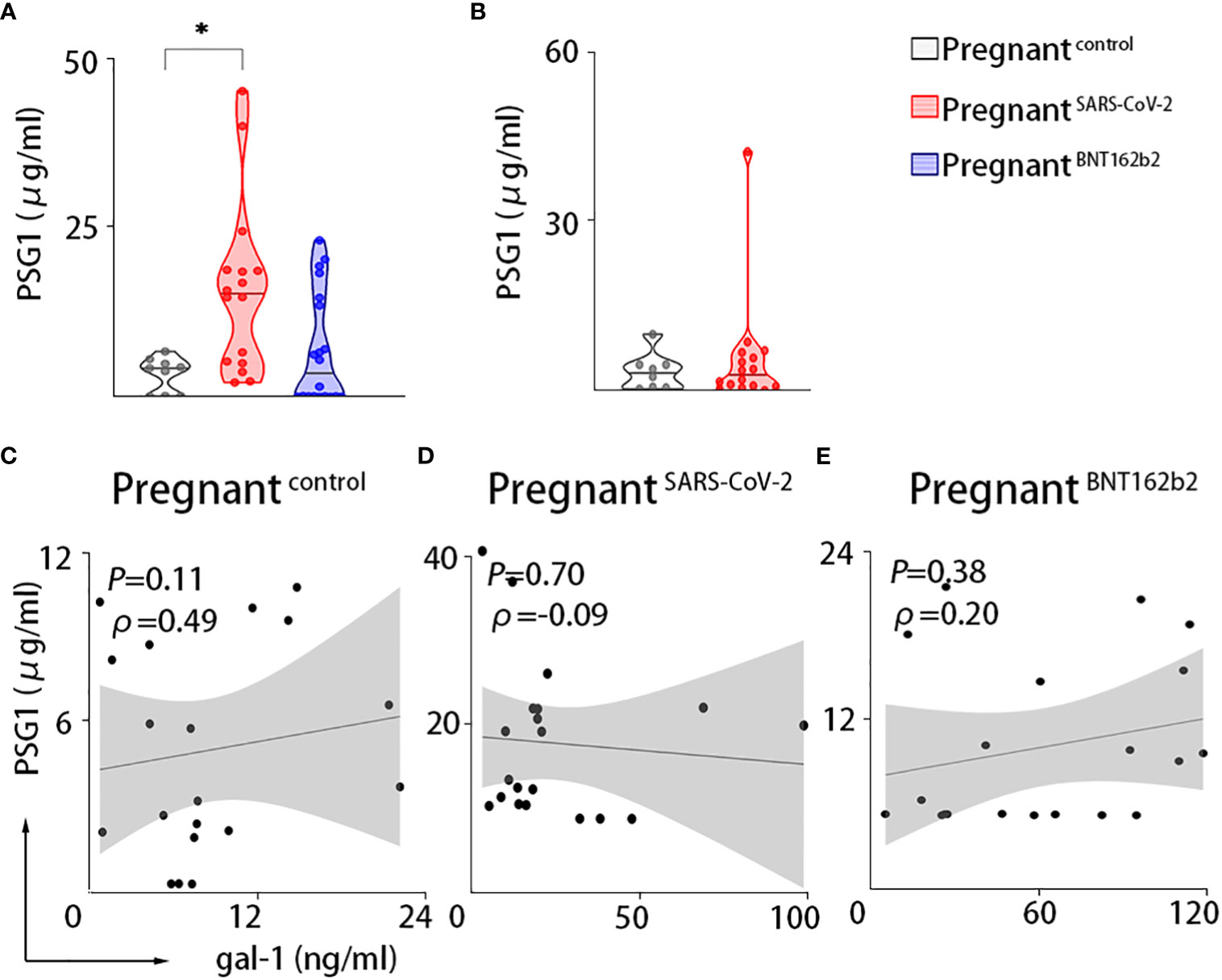
Figure 3 Maternal circulation of PSG1 is only upregulated upon SARS-CoV-2 infection during pregnancy. PSG1. (A, B) Maternal circulating levels of PSG1 in the PRINCE cohort (A) or the Yale IMPACT cohort (B). (C–E) Correlations between serum values of gal-1 and PSG1 in the PRINCE cohort. Pregnantcontrol, healthy pregnant women; pregnantSARS-CoV-2, pregnant women infected by SARS-CoV-2; pregnantBNT162b2, pregnant women vaccinated against SARS-CoV-2. *P < 0.05 as analyzed by Kruskal–Wallis test or the Welch’s t-test.
3.6 Transcriptional placental changes of galectins, PSGs, and glycosylation pathways during maternal SARS-CoV-2 infection
To further explore the impact of SARS-CoV-2 infection on the placenta compartment, we analyzed RNA sequencing data (GSE171995) of placental villi from the Yale IMPACT cohort. The transcriptomic placental analysis of galectins and PSGs in pregnant women with SARS-CoV-2 infection and matched healthy controls indicated that, consistent with the serum protein measurements, the expression of gal-1 (LGALS1, P = 0.0077) and gal-3 genes (LGALS3, P = 0.0152) were increased in the placentas of pregnant women with SARS-CoV-2 infection compared with controls (Figure 4A). Moreover, several PSG genes were differentially expressed in the placentas: increased expression of PSG1 (P = 0.0410), PSG3 (P = 0.0456), PSG5 (P = 0.0259), PSG6 (P = 0.0482), PSG8 (P = 0.0355), PSG9 (P = 0.0209), and PSG11 (P = 0.0462) were found in the SARS-CoV-2–infected individuals compared with controls (Figure 4A).
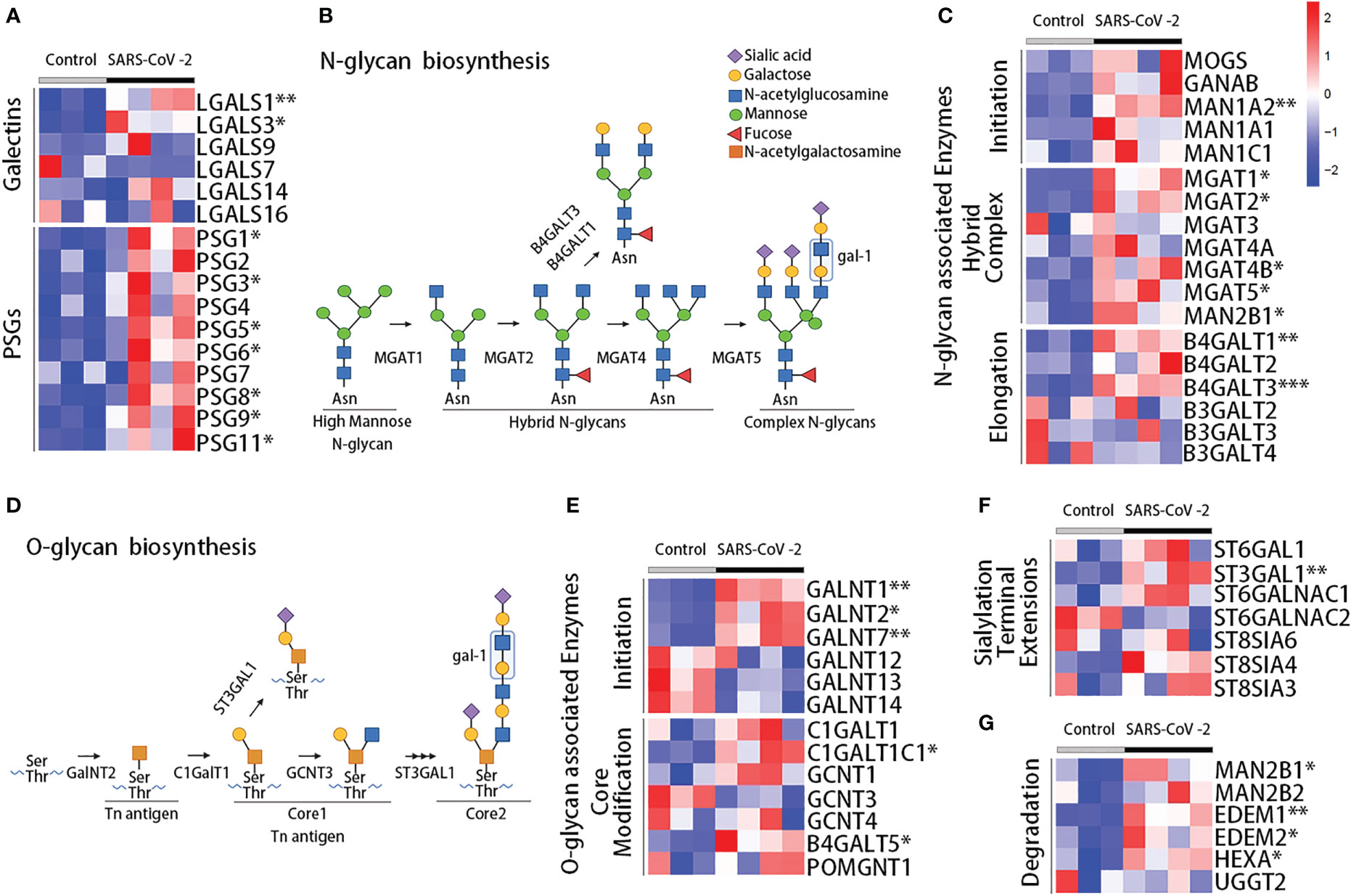
Figure 4 Transcriptome analysis of placenta during acute SARS-CoV-2 infection. (A) Heatmap showing the scaled expression of galectins and PSG genes from RNA sequencing data in the placenta of the Yale IMPACT cohort. Enzymes involved in the synthesis of N-glycans (B) and O-glycans (D), showing the preferred ligand for gal-1. Heatmaps for the expression of the enzymes involved in the synthesis of N-glycans (C) and O-glycans (E), involved in terminal extension (F) or glycan degradation (G). Control, healthy pregnant women; SARS-CoV-2, pregnant women infected by SARS-CoV-2. *P < 0.05, **P < 0.01, and ***P < 0.001 as analyzed with the Welch’s t-test.
Extracellular biological functions of galectins rely on their capacity to bind specific glycans present in proteins on the cell membrane and in the extracellular matrix. Indeed, gal-1 can recognize N- or O-linked glycans containing multiple LacNAc units, which are generated by the enzymes N-acetylglucosaminyltransferases (MGAT genes) or β1,6-N-acetylglucosaminyltransferases (GCNT genes), respectively (Figures 4B–D) (6, 49, 50). Poly-LacNAc is also reported as a preferred ligand for gal-3 (51); meanwhile, gal-9 is considered to preferentially bind to internal LacNAc residues of a poly-LacNAc chain (52). We performed differential gene expression analysis to compare the transcriptomic profile of 84 glycoenzymes and generated a hierarchical clustering scheme. A total of 18 glycoenzymes were differentially expressed. Among them, eight are involved in the synthesis and modification of N-linked glycans (Figure 4C), five in the synthesis and processing of O-linked glycans (Figures 4D–E), and five in terminal extensions of sialylation and its degradation (Figures 4F, G). Overall our results showed upregulated expression of glycoenzymes in the placentas of SARS-CoV-2–infected individuals compared with healthy controls, which included the enzymes that take part in the initiation (MAN1A2, P = 0.0011), branched complex (MGAT1, P = 0.0131; MGAT2, P = 0.0142; MGAT4B, P = 0.0260; MGAT5, P = 0.0143; MAN2B1, P = 0.0226), and elongation (B4GALT1, P = 0.0013; B4GALT3, P = 0.0004) of N-linked glycans (Figure 4C). We also observed an upregulation of the expression of glycoenzymes that take part in the initiation (GALNT1, P = 0.0015; GALNT2, P = 0.0172; GALNT7, P = 0.0023) and core modification (B4GALT5, P = 0.0302; C1GALTC1, P = 0.0171) of O-linked glycans (Figure 4E) and enzymes linked to terminal extensions of sialylation and degradation including ST3GAL1 (P = 0.0034), MAN2B1 (P = 0.0226), EDEM1 (P = 0.0062), EDEM2 (P = 0.0331), and HEXA (P = 0.0349) (Figures 4F, G). These results suggest an enrichment of N-glycan– and O-glycan–associated enzymes compatible with the gal-1 binding in SARS-CoV-2–infected placentas.
4 Discussion
SARS-CoV-2 infection leads to a higher risk of severe disease in pregnant than in non-pregnant women. Considering that women of reproductive age make up more than 20% of the global population, studying the effects of SARS-CoV-2 infection and vaccination in this population is of great importance. We measured the concentration of several galectins and PSG1 in the circulation of pregnant women infected with SARS-CoV-2 or vaccinated against SARS-CoV-2 and also explored their placental expression (Figure 5). Our results demonstrated a dominant role of gal-1 within the galectin signature in pregnant women upon SARS-CoV-2 infection in both the PRINCE and Yale IMPACT cohorts. Our findings align with previous reports in non-pregnant individuals, indicating that SARS-CoV-2 infection increased gal-1 levels (41, 42). Moreover, one study showed a positive correlation between gal-1; levels of pro-inflammatory cytokines such as Interleukin-1 beta (IL-1β), IL-6, and IL-23; and COVID-19 severity, suggesting that gal-1 acts as an alarmin (42). In non-pregnant patients, gal-3 and gal-9 were found to be increased and correlated with COVID-19 severity (45, 53). However, we did not find increased levels of gal-3 or gal-9 in pregnant women with SARS-CoV-2 infection. This difference in results may be explained, at least, in part, by the fact that most of our cohort patients had mild or non-symptomatic COVID-19 disease. Furthermore, our results showed positive correlations between gal-1 and gal-3 or gal-9 levels only in healthy pregnancies. This positive correlation was expected because successful pregnancy is associated with a rise in maternal circulating levels of these three galectins from the first to the third trimester (28, 54, 55). The observation that only gal-1 was increased as a result of SARS-CoV-2 infection is likely responsible for the observed loss of correlation with the other two galectins in this group of patients.
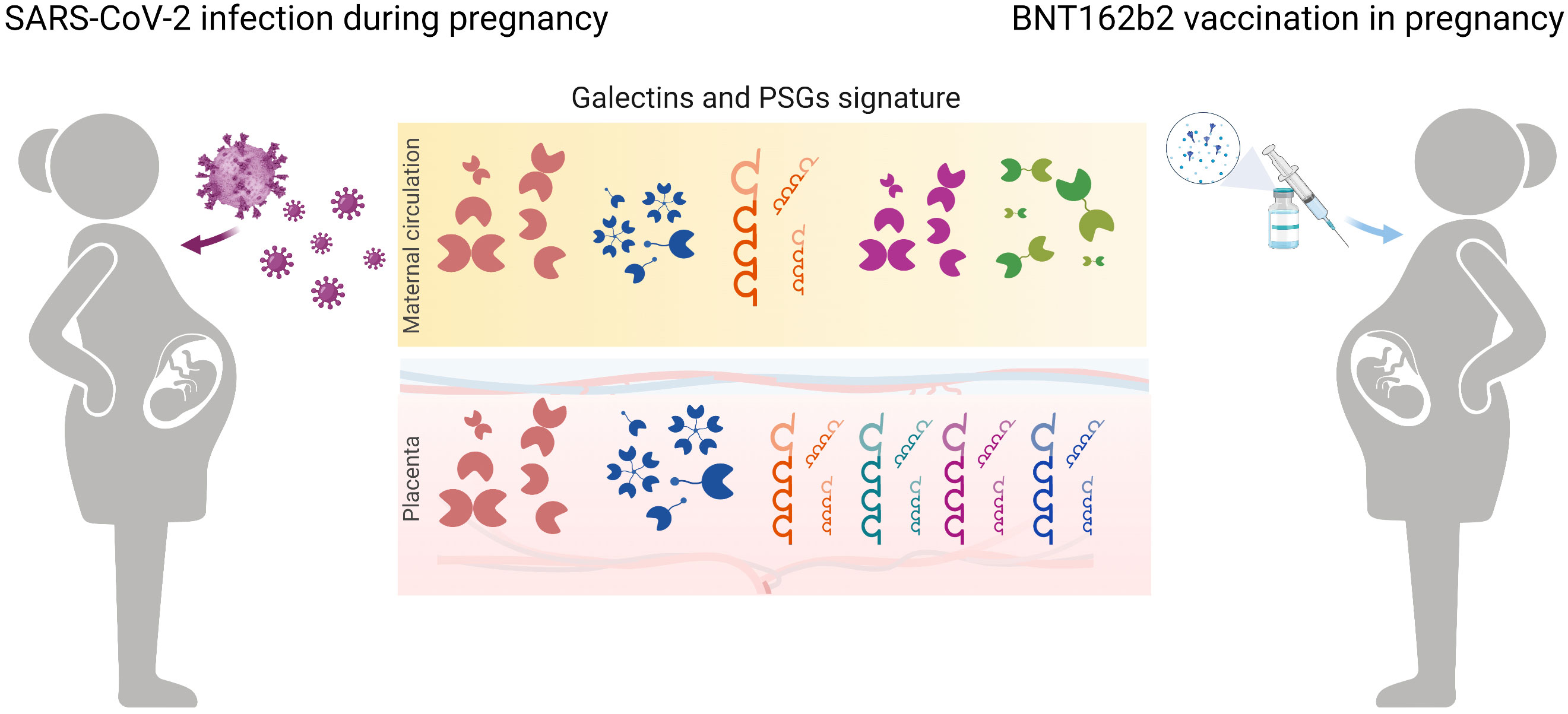
Figure 5 Schematic diagram highlighting galectin and PSGs fingerprints in pregnancy upon SARS-CoV-2 infection and in women subjected to vaccination. In maternal circulation, levels of gal-1 and PSG1 increased during COVID-19 disease in pregnancy. Vaccination with BNT162b2 caused an upregulation of gal-1 circulating levels mimicking the viral infection. During SARS-CoV-2 infection, placental transcription regulation of gal-1 and gal-3 dominates the galectin signature creating a protective microenvironment. In addition, upregulation of PSGs by the trophoblast cells contributes to immune modulation during COVID-19 disease.
The immune system plays a key role in mediating a successful pregnancy because a fine balance is necessary to promote maternal tolerance to the allogeneic fetus while protecting both the mother and the fetus from pathogens (56, 57). Several studies demonstrated that the maternal immune response tend to restrain inflammation through regulatory and anti-inflammatory mediators (58, 59), and galectins, especially gal-1, are involved in this process (6). Moreover, gal-1 has been described not only as a damage-associated molecular pattern molecule, amplifying the immune response, but also as a member of a diverse group of mediators collectively referred to as resolution-associated molecular pattern molecules with the capacity to resolve an acute inflammation process by counteracting the synthesis of pro-inflammatory cytokines (60, 61). The cytokine storm in the systemic circulation is responsible for the pathophysiology of SARS-CoV-2 infection and can lead to multi-organ damage (7, 62). Both systemic and inflammatory changes at the maternal–fetal interface were previously described during SARS-CoV-2 infection in pregnancy (48, 63). The analysis of bulk RNA sequencing of placental villi from the Yale IMPACT cohort demonstrated that COVID-19 cases presented increased expression of genes associated with an immune response, indicating a robust response at the maternal–fetal interface upon SARS-CoV-2 infection, even in the absence of localized placental infection (48). Another study evaluated the maternal systemic immune response, finding increased IL-8, IL-10, and IL-15 levels as well as a reduction in T-cell subsets, particularly of T helper cell 1 (Th1) and a subset of CD8+ cells characterized by the production of IL-17 (Tc17)-like cells in pregnant women infected with SARS-CoV-2 (63). Gal-1 controls the fate of Th-1 and Th-17 cells through the glycan repertoire expressed by these cells that allow gal-1 binding (64). Therefore, the increase of gal-1 in maternal circulation during COVID-19 could be associated with the observed systemic decrease in Th-1 and Tc17-like cells. Gal-1 also induces apoptosis of activated CD8+ T cells (65), which could explain the upregulation of gal-1 in SARS-CoV-2 infection. Thus, the increase of gal-1 observed in pregnant patients with COVID-19 could be related to its anti-inflammatory activity and its potential participation in the control of inflammation and tissue damage resulting from SARS-CoV-2 infection.
In our study, healthy pregnant women exposed to SARS-CoV-2 infection often exhibit simultaneously increased serum concentrations of gal-1 and PSG1. We have previously demonstrated that PSG1 binds to gal-1 and postulated that PSG1 protects gal-1 from inactivation by oxidation in the extracellular environment. Therefore, an increase in both PSG1 and gal-1 may extend the gal-1 activity once it is released from cells into the circulation (29). However, the correlation between these two proteins disappeared in infected pregnant women, likely due to the modest increase of PSG1 relative to gal-1. Unfortunately, we were unable to determine whether the concentration of other PSG family members, which can also interact with gal-1, is also increased in the circulation of SARS-CoV-2–infected pregnant women as suggested by the RNA sequencing data because specific validated ELISAs are only available to measure the concentration of PSG1. Future studies should be performed to determine the mechanism by which syncytiotrophoblast cells, the major cell type that secretes PSG1 into the maternal circulation, regulate PSG1 secretion following maternal SARS-CoV-2 infection. In contrast, PSG1 post-vaccination levels remain unchanged, suggesting that modulation of PSG1 secretion by the syncytiotrophoblast cells requires maternal SARS-CoV-2 infection. In the future, it would be interesting to determine whether an increase in PSG1 concentration is also observed following infection with other viruses that do not infect the PSG1-producing cells and whether this increase in PSG levels may contribute to the establishment of an anti-inflammatory environment.
The extracellular activity of gal-1 relies on its capacity to bind specific glycans. To further investigate the possible role of the gal-1 upregulation during the course of COVID-19, the expression of enzymes involved in relevant N- and O-glycosylation was analyzed in bulk placentas by RNA sequencing. Our results showed that genes involved in the initiation, hybrid complex formation, and elongation of N-glycans and in initiation and core modification of O-glycans were upregulated in patients with COVID-19. Gal-1 and gal-3 can bind β-1,6-GlcNAc–branched N-linked glycans on the cell surface or in secreted glycoproteins, and several enzymes are required for the biosynthesis of these glycotopes (51). Similar results were found for the enzymes involved in O-glycans synthesis, as initiation (GALNT1, GALNT2, and GALNT7) and core modification (C1GALT1C1 and B4GALT5) enzymes were also upregulated during SARS-CoV-2 infection. The addition of α2,6-linked sialic acids to the termini of glycans by ST6GAL1 inhibits the binding of gal-1 (6). However, our results demonstrated similar expression of ST6GAL1 in SARS-CoV-2–infected and control groups. More importantly, we observed an increase of ST3GAL1 expression signatures (responsible for the addition of α2,3-linked sialic acid, which is compatible which gal-1 high-affinity binding) within the placental compartment. These results suggest that both the expression of gal-1 and of the enzymes in the placenta involved in the N- and O-glycan modifications required for gal-1 to exert its function are increased following SARS-CoV-2 infection.
We also explored the galectin fingerprint of healthy pregnant women that were fully vaccinated. Several reports indicated that COVID-19 vaccines are safe for pregnant women and can effectively protect them from getting severe COVID-19 and prevent maternal and fetal mortality (8, 66). Our results provide evidence that the status of the maternal immune system during vaccination modulates the galectin signature and could play a role for protection against COVID-19 disease. For instance, a simultaneous increase of gal-1 and gal-7 accompanied by a decrease of gal-9 in post- vaccination sera derived from pregnant individuals suggests a role of these lectins in the establishment of a protective response against the SARS-CoV-2 virus. Gal-1 is secreted by activated B cells, T cells, and macrophages, and activated lymphocytes secrete gal-7 (67, 68). We hypothesize that the increase of these two galectins in pregnant vaccinated patients could play a role in the effective immune system activation by the COVID-19 vaccine that results in the generation of neutralizing antibodies and virus-specific T-cell responses (69). Elevation of circulating levels of gal-9 in chronic and acute SARS-CoV-2 infection has been reported in non-pregnant individuals, and it was correlated with a severe disease outcome (43). On the basis of our findings, we hypothesized that a lack of increase in gal-9 levels during vaccination likely avoids a pro-inflammatory response during pregnancy vaccination that could result in detrimental effects at the maternal–fetal interface.
In conclusion, our study revealed a clear dominance of gal-1 within the maternal galectin fingerprint during SARS-CoV-2 infection and vaccination in pregnancy. One of the pregnancy-specific gal-1 ligands, PSG1, was also upregulated during the COVID-19 disease, suggesting a potential synergistic immunomodulatory role of these proteins at the maternal–fetal interface. Further work is needed to determine the functional relevance of the galectin signature in maternal circulation during the SARS-CoV-2 infection and vaccination in pregnancy. Specifically, it would be important to understand the molecular mechanism by which gal-1 may control the immune response to a virus during pregnancy.
Data availability statement
The original contributions presented in the study are included in the article/Supplementary Material. Further inquiries can be directed to the corresponding author.
Ethics statement
The studies involving human participants were reviewed and approved by Hamburg Chamber of Physicians (Ärztekammer Hamburg) approved the study protocol under the registration numbers PV3694 (PRINCE), PV 7312-4710 (PRINCE COVID) and 2021-10647-BO-ff (PRINCE VACCINE). The study was approved by the Yale Institutional Review Board (protocol #2000027690). The patients/participants provided their written informed consent to participate in this study.
Author contributions
SMB designed the study and secured grant funding. FZ, A-CT, YW, YX, AD, AL-C, PV, EK, CU, JB, PCA, SFF, GSD, and MGG performed experiments and/or analyzed data. The original draft of the manuscript was written by FZ, MGG, and SMB, and further writing, review, and editing were done by all authors. All authors contributed to the article and approved the submitted version.
Funding
This research was funded by the Deutsche Forschungsgemeinschaft (DFG) Heisenberg Program (BL1115/3-1, BL1115/7-1, and BL1115/11-1) to SMB; National Institute of Health (NIH) grants K23MH118999 and R01AI157488 to SFF; National Institute of Allergy and Infectious Diseases (NIAID)–NIH grant R21AI156058 to GSD. FZ, YX, and YW were supported by a China Scholarship Council (CSC) fellowship. The content is solely the responsibility of the authors and does not necessarily represent the official views of the NIH or the US Department of Defense. Figures 4B, D, 5 were created with BioRender.com.
Acknowledgments
We thank T. Andreas (Blois’s Team), A. Wieczorek, N. Felber, G. Hansen, E. Ehrenburg (PRINCE Study Team), and the Yale IMPACT Team for their excellent technical assistance in generating this work.
Conflict of interest
The authors declare that the research was conducted without any commercial or financial relationships that could be construed as a potential conflict of interest.
Publisher’s note
All claims expressed in this article are solely those of the authors and do not necessarily represent those of their affiliated organizations, or those of the publisher, the editors and the reviewers. Any product that may be evaluated in this article, or claim that may be made by its manufacturer, is not guaranteed or endorsed by the publisher.
Supplementary material
The Supplementary Material for this article can be found online at: https://www.frontiersin.org/articles/10.3389/fimmu.2023.1196395/full#supplementary-material
References
1. Adam S, Pheiffer C, Dias S, Hlongwane T, Vannevel V, Soma-Pillay P, et al. Coronavirus and pregnancy: the challenges of the 21(st) century: a review. Front Microbiol (2022) 13:923546. doi: 10.3389/fmicb.2022.923546
2. Silasi M, Cardenas I, Kwon JY, Racicot K, Aldo P, Mor G. Viral infections during pregnancy. Am J Reprod Immunol (2015) 73(3):199–213. doi: 10.1111/aji.12355
3. Wastnedge EAN, Reynolds RM, van Boeckel SR, Stock SJ, Denison FC, Maybin JA, et al. Pregnancy and COVID-19. Physiol Rev (2021) 101(1):303–18. doi: 10.1152/physrev.00024.2020
4. Leung C, Simões ESAC, Oliveira EA. Are in-hospital COVID-19-related mortality and morbidity in pregnancy associated with gestational age? Ultrasound Obstet Gynecol (2022) 60(2):234–42. doi: 10.1002/uog.24931
5. Metz TD, Clifton RG, Hughes BL, Sandoval GJ, Grobman WA, Saade GR, et al. Association of SARS-CoV-2 infection with serious maternal morbidity and mortality from obstetric complications. Jama (2022) 327(8):748–59. doi: 10.1001/jama.2022.1190
6. Blois SM, Dveksler G, Vasta GR, Freitag N, Blanchard V, Barrientos G. Pregnancy galectinology: insights into a complex network of glycan binding proteins. Front Immunol (2019) 10:1166. doi: 10.3389/fimmu.2019.01166
7. Li N, Han L, Peng M, Lv Y, Ouyang Y, Liu K, et al. Maternal and neonatal outcomes of pregnant women with coronavirus disease 2019 (COVID-19) pneumonia: a case-control study. Clin Infect Dis (2020) 71(16):2035–41. doi: 10.1093/cid/ciaa352
8. Male V. SARS-CoV-2 infection and COVID-19 vaccination in pregnancy. Nat Rev Immunol (2022) 22(5):277–82. doi: 10.1038/s41577-022-00703-6
9. Marchand G, Patil AS, Masoud AT, Ware K, King A, Ruther S, et al. Systematic review and meta-analysis of COVID-19 maternal and neonatal clinical features and pregnancy outcomes up to June 3, 2021. AJOG Global Rep (2022) 2(1):100049. doi: 10.1016/j.xagr.2021.100049
10. Muthuka J, Kiptoo M, Oluoch K, Nzioki JM, Nyamai EM. Association of pregnancy with coronavirus cytokine storm: systematic review and meta-analysis. JMIR Pediatr parenting. (2022) 5(4):e31579. doi: 10.2196/31579
11. Nana M, Nelson-Piercy C. COVID-19 in pregnancy. Clin Med (London England). (2021) 21(5):e446–e50. doi: 10.7861/clinmed.2021-0503
12. Wainstock T, Yoles I, Sergienko R, Sheiner E. Prenatal maternal COVID-19 vaccination and pregnancy outcomes. Vaccine (2021) 39(41):6037–40. doi: 10.1016/j.vaccine.2021.09.012
13. Nana M, Hodson K, Lucas N, Camporota L, Knight M, Nelson-Piercy C. Diagnosis and management of covid-19 in pregnancy. Bmj (2022) 377:e069739. doi: 10.1136/bmj-2021-069739
14. Beharier O, Plitman Mayo R, Raz T, Nahum Sacks K, Schreiber L, Suissa-Cohen Y, et al. Efficient maternal to neonatal transfer of antibodies against SARS-CoV-2 and BNT162b2 mRNA COVID-19 vaccine. J Clin Invest (2021) 131(13):e150319. doi: 10.1172/JCI150319
15. Carbone L, Trinchillo MG, Di Girolamo R, Raffone A, Saccone G, Iorio GG, et al. COVID-19 vaccine and pregnancy outcomes: a systematic review and meta-analysis. Int J Gynaecol Obstet (2022) 159(3):651–61. doi: 10.1002/ijgo.14336
16. Rottenstreich A, Zarbiv G, Oiknine-Djian E, Vorontsov O, Zigron R, Kleinstern G, et al. The effect of gestational age at BNT162b2 mRNA vaccination on maternal and neonatal severe acute respiratory syndrome coronavirus 2 (SARS-CoV-2) antibody levels. Clin Infect Dis (2022) 75(1):e603–e10. doi: 10.1093/cid/ciac135
17. Atyeo C, DeRiso EA, Davis C, Bordt EA, DeGuzman RM, Shook LL, et al. COVID-19 mRNA vaccines drive differential Fc-functional profiles in pregnant, lactating, and non-pregnant women. Sci Transl Med (2021) 617:eabi8631. doi: 10.1126/scitranslmed.abi8631
18. Ben-Mayor Bashi T, Amikam U, Ashwal E, Hershkovitz G, Attali E, Berkovitz-Shperling R, et al. The association of maternal SARS-CoV-2 vaccination-to-delivery interval and the levels of maternal and cord blood antibodies. Int J Gynaecol Obstet (2022) 156(3):436–43. doi: 10.1002/ijgo.14014
19. Bookstein Peretz S, Regev N, Novick L, Nachshol M, Goffer E, Ben-David A, et al. Short-term outcome of pregnant women vaccinated with BNT162b2 mRNA COVID-19 vaccine. Ultrasound Obstet Gynecol (2021) 58(3):450–6. doi: 10.1002/uog.23729
20. Citu IM, Citu C, Gorun F, Sas I, Tomescu L, Neamtu R, et al. Immunogenicity following administration of BNT162b2 and Ad26.COV2.S COVID-19 vaccines in the pregnant population during the third trimester. Viruses (2022) 14(2):307. doi: 10.3390/v14020307
21. Collier AY, McMahan K, Yu J, Tostanoski LH, Aguayo R, Ansel J, et al. Immunogenicity of COVID-19 mRNA vaccines in pregnant and lactating women. Jama (2021) 325(23):2370–80. doi: 10.1001/jama.2021.7563
22. Kugelman N, Nahshon C, Shaked-Mishan P, Cohen N, Sher ML, Gruber M, et al. Maternal and neonatal SARS-CoV-2 immunoglobulin G antibody levels at delivery after receipt of the BNT162b2 messenger RNA COVID-19 vaccine during the second trimester of pregnancy. JAMA Pediat (2022) 176(3):290–5. doi: 10.1001/jamapediatrics.2021.5683
23. Mithal LB, Otero S, Shanes ED, Goldstein JA, Miller ES. Cord blood antibodies following maternal coronavirus disease 2019 vaccination during pregnancy. Am J Obstet Gynecol (2021) 225(2):192–4. doi: 10.1016/j.ajog.2021.03.035
24. Nir O, Schwartz A, Toussia-Cohen S, Leibovitch L, Strauss T, Asraf K, et al. Maternal-neonatal transfer of SARS-CoV-2 immunoglobulin G antibodies among parturient women treated with BNT162b2 messenger RNA vaccine during pregnancy. Am J Obstet Gynecol MFM (2022) 4(1):100492. doi: 10.1016/j.ajogmf.2021.100492
25. Prabhu M, Murphy EA, Sukhu AC, Yee J, Singh S, Eng D, et al. Antibody response to coronavirus disease 2019 (COVID-19) messenger RNA vaccination in pregnant women and transplacental passage into cord blood. Obstet Gynecol (2021) 138(2):278–80. doi: 10.1097/AOG.0000000000004438
26. Freitag N, Tirado-González I, Barrientos G, Cohen M, Daher S, Goldman-Wohl D, et al. The chimera-type galectin-3 is a positive modulator of trophoblast functions with dysregulated expression in gestational diabetes mellitus. Am J Reprod Immunol (2020) 84(6):e13311. doi: 10.1111/aji.13311
27. Li YH, Zhou WH, Tao Y, Wang SC, Jiang YL, Zhang D, et al. The galectin-9/Tim-3 pathway is involved in the regulation of NK cell function at the maternal-fetal interface in early pregnancy. Cell Mol Immunol (2016) 13(1):73–81. doi: 10.1038/cmi.2014.126
28. Tirado-Gonzalez I, Freitag N, Barrientos G, Shaikly V, Nagaeva O, Strand M, et al. Galectin-1 influences trophoblast immune evasion and emerges as a predictive factor for the outcome of pregnancy. Mol Hum Reprod (2013) 19(1):43–53. doi: 10.1093/molehr/gas043
29. Mendoza M, Lu D, Ballesteros A, Blois SM, Abernathy K, Feng C, et al. Glycan characterization of pregnancy-specific glycoprotein 1 and its identification as a novel galectin-1 ligand. Glycobiology (2020) 30(11):895–909. doi: 10.1093/glycob/cwaa034
30. Blois SM, Sulkowski G, Tirado-González I, Warren J, Freitag N, Klapp BF, et al. Pregnancy-specific glycoprotein 1 (PSG1) activates TGF-β and prevents dextran sodium sulfate (DSS)-induced colitis in mice. Mucosal Immunol (2014) 7(2):348–58. doi: 10.1038/mi.2013.53
31. Moore T, Williams JM, Becerra-Rodriguez MA, Dunne M, Kammerer R, Dveksler G. Pregnancy-specific glycoproteins: evolution, expression, functions and disease associations. Reproduction (2022) 163(2):R11–23. doi: 10.1530/REP-21-0390
32. Rattila S, Dunk CEE, Im M, Grichenko O, Zhou Y, Yanez-Mo M, et al. Interaction of pregnancy-specific glycoprotein 1 with integrin A5β1 is a modulator of extravillous trophoblast functions. Cells (2019) 8(11):1369. doi: 10.3390/cells8111369
33. Rattila S, Kleefeldt F, Ballesteros A, Beltrame JS, LR M, Ergün S, et al. Pro-angiogenic effects of pregnancy-specific glycoproteins in endothelial and extravillous trophoblast cells. Reproduction (2020) 160(5):737–50. doi: 10.1530/REP-20-0169
34. Shanley DK, Kiely PA, Golla K, Allen S, Martin K, O'Riordan RT, et al. Pregnancy-specific glycoproteins bind integrin αIIbβ3 and inhibit the platelet-fibrinogen interaction. PloS One (2013) 8(2):e57491. doi: 10.1371/journal.pone.0057491
35. Ballesteros A, Mentink-Kane MM, Warren J, Kaplan GG, Dveksler GS. Induction and activation of latent transforming growth factor-β1 are carried out by two distinct domains of pregnancy-specific glycoprotein 1 (PSG1). J Biol Chem (2015) 290(7):4422–31. doi: 10.1074/jbc.M114.597518
36. Kammerer R, Ballesteros A, Bonsor D, Warren J, Williams JM, Moore T, et al. Equine pregnancy-specific glycoprotein CEACAM49 secreted by endometrial cup cells activates TGFB. Reproduction (2020) 160(5):685–94. doi: 10.1530/REP-20-0277
37. Moore JB, June CH. Cytokine release syndrome in severe COVID-19. Science (2020) 368(6490):473–4. doi: 10.1126/science.abb8925
38. Rosen DB, Murphy EA, Gejman RS, Capili A, Friedlander RL, Rand S, et al. Cytokine response over the course of COVID-19 infection in pregnant women. Cytokine (2022) 154:155894. doi: 10.1016/j.cyto.2022.155894
39. De Biasi S, Meschiari M, Gibellini L, Bellinazzi C, Borella R, Fidanza L, et al. Marked T cell activation, senescence, exhaustion and skewing towards TH17 in patients with COVID-19 pneumonia. Nat Commun (2020) 11(1):3434. doi: 10.1038/s41467-020-17292-4
40. Patel H, Ashton NJ, Dobson RJB, Andersson LM, Yilmaz A, Blennow K, et al. Proteomic blood profiling in mild, severe and critical COVID-19 patients. Sci Rep (2021) 11(1):6357. doi: 10.1038/s41598-021-85877-0
41. Kazancioglu S, Yilmaz FM, Bastug A, Ozbay BO, Aydos O, Yücel Ç, et al. Assessment of galectin-1, galectin-3, and prostaglandin E2 levels in patients with COVID-19. Japan J Infect Dis (2021) 74(6):530–6. doi: 10.7883/yoken.JJID.2021.020
42. Markovic SS, Gajovic N, Jurisevic M, Jovanovic M, Jovicic BP, Arsenijevic N, et al. Galectin-1 as the new player in staging and prognosis of COVID-19. Sci Rep (2022) 12(1):1272. doi: 10.1038/s41598-021-04602-z
43. Bozorgmehr N, Mashhouri S, Rosero EP, Xu L, Shahbaz S, Sligl W, et al. Galectin-9, a player in cytokine release syndrome and a surrogate diagnostic biomarker in SARS-CoV-2 infection. mBio (2021) 12(3):e00384–21. doi: 10.1128/mBio.00384-21
44. Baykiz D, Emet S, Ayduk-Govdeli E, Kaytaz M, Yavuz ML, Karaca-Ozer P, et al. Galectin-3 as a novel biomarker for predicting clinical outcomes in hospitalized COVID-19 patients. Clin Lab (2022) 68(12). doi: 10.7754/Clin.Lab.2022.220134
45. Portacci A, Diaferia F, Santomasi C, Dragonieri S, Boniello E, Di Serio F, et al. Galectin-3 as prognostic biomarker in patients with COVID-19 acute respiratory failure. Respir Med (2021) 187:106556. doi: 10.1016/j.rmed.2021.106556
46. Yeung ST, Premeaux TA, Du L, Niki T, Pillai SK, Khanna KM, et al. Galectin-9 protects humanized-ACE2 immunocompetent mice from SARS-CoV-2 infection. Front Immunol (2022) 13:1011185. doi: 10.3389/fimmu.2022.1011185
47. Gaughan EE, Quinn TM, Mills A, Bruce AM, Antonelli J, MacKinnon AC, et al. An inhaled galectin-3 inhibitor in COVID-19 pneumonitis: a phase Ib/IIa randomized controlled clinical trial (DEFINE). Am J Respir Crit Care Med (2023) 207(2):138–49. doi: 10.1164/rccm.202203-0477OC
48. Lu-Culligan A, Chavan AR, Vijayakumar P, Irshaid L, Courchaine EM, Milano KM, et al. Maternal respiratory SARS-CoV-2 infection in pregnancy is associated with a robust inflammatory response at the maternal-fetal interface. Med (New York NY). (2021) 2(5):591–610.e10. doi: 10.1016/j.medj.2021.04.016
49. Grigorian A, Torossian S, Demetriou M. T-Cell growth, cell surface organization, and the galectin-glycoprotein lattice. Immunol Rev (2009) 230(1):232–46. doi: 10.1111/j.1600-065X.2009.00796.x
50. Kariya Y, Oyama M, Hashimoto Y, Gu J, Kariya Y. β4-Integrin/PI3K signaling promotes tumor progression through the galectin-3-N-Glycan complex. Mol Cancer Res (2018) 16(6):1024–34. doi: 10.1158/1541-7786.MCR-17-0365
51. Croci DO, Cerliani JP, Dalotto-Moreno T, Méndez-Huergo SP, Mascanfroni ID, Dergan-Dylon S, et al. Glycosylation-dependent lectin-receptor interactions preserve angiogenesis in anti-VEGF refractory tumors. Cell (2014) 156(4):744–58. doi: 10.1016/j.cell.2014.01.043
52. Giovannone N, Liang J, Antonopoulos A, Geddes Sweeney J, King SL, Pochebit SM, et al. Galectin-9 suppresses b cell receptor signaling and is regulated by I-branching of n-glycans. Nat Commun (2018) 9(1):3287. doi: 10.1038/s41467-018-05770-9
53. Gajovic N, Markovic SS, Jurisevic M, Jovanovic M, Arsenijevic N, Mijailovic Z, et al. Galectin-3 as an important prognostic marker for COVID-19 severity. Sci Rep (2023) 13(1):1460. doi: 10.1038/s41598-023-28797-5
54. Freitag N, Tirado-Gonzalez I, Barrientos G, Powell KL, Boehm-Sturm P, Koch SP, et al. Galectin-3 deficiency in pregnancy increases the risk of fetal growth restriction (FGR) via placental insufficiency. Cell Death Dis (2020) 11(7):560. doi: 10.1038/s41419-020-02791-5
55. Meggyes M, Miko E, Polgar B, Bogar B, Farkas B, Illes Z, et al. Peripheral blood TIM-3 positive NK and CD8+ T cells throughout pregnancy: TIM-3/galectin-9 interaction and its possible role during pregnancy. PloS One (2014) 9(3):e92371. doi: 10.1371/journal.pone.0092371
56. Abu-Raya B, Michalski C, Sadarangani M, Lavoie PM. Maternal immunological adaptation during normal pregnancy. Front Immunol (2020) 11:575197. doi: 10.3389/fimmu.2020.575197
57. Mor G, Aldo P, Alvero AB. The unique immunological and microbial aspects of pregnancy. Nat Rev Immunol (2017) 17(8):469–82. doi: 10.1038/nri.2017.64
58. Robertson SA, Care AS, Moldenhauer LM. Regulatory T cells in embryo implantation and the immune response to pregnancy. J Clin Invest (2018) 128(10):4224–35. doi: 10.1172/JCI122182
59. Yockey LJ, Iwasaki A. Interferons and proinflammatory cytokines in pregnancy and fetal development. Immunity (2018) 49(3):397–412. doi: 10.1016/j.immuni.2018.07.017
60. Menkhorst E, Than NG, Jeschke U, Barrientos G, Szereday L, Dveksler G, et al. Medawar's PostEra: galectins emerged as key players during fetal-maternal glycoimmune adaptation. Front Immunol (2021) 12:784473. doi: 10.3389/fimmu.2021.784473
61. Sundblad V, Morosi LG, Geffner JR, Rabinovich GA. Galectin-1: a jack-of-All-Trades in the resolution of acute and chronic inflammation. J Immunol (2017) 199(11):3721–30. doi: 10.4049/jimmunol.1701172
62. Tay MZ, Poh CM, Rénia L, MacAry PA, Ng LFP. The trinity of COVID-19: immunity, inflammation and intervention. Nat Rev Immunol (2020) 20(6):363–74. doi: 10.1038/s41577-020-0311-8
63. Garcia-Flores V, Romero R, Xu Y, Theis KR, Arenas-Hernandez M, Miller D, et al. Maternal-fetal immune responses in pregnant women infected with SARS-CoV-2. Nat Commun (2022) 13(1):320. doi: 10.1038/s41467-021-27745-z
64. Toscano MA, Bianco GA, Ilarregui JM, Croci DO, Correale J, Hernandez JD, et al. Differential glycosylation of TH1, TH2 and TH-17 effector cells selectively regulates susceptibility to cell death. Nat Immunol (2007) 8(8):825–34. doi: 10.1038/ni1482
65. Kopcow HD, Rosetti F, Leung Y, Allan DS, Kutok JL, Strominger JL. T Cell apoptosis at the maternal-fetal interface in early human pregnancy, involvement of galectin-1. Proc Natl Acad Sci USA (2008) 105(47):18472–7. doi: 10.1073/pnas.0809233105
66. Magnus MC, Örtqvist AK, Dahlqwist E, Ljung R, Skår F, Oakley L, et al. Association of SARS-CoV-2 vaccination during pregnancy with pregnancy outcomes. Jama (2022) 327(15):1469–77. doi: 10.1001/jama.2022.3271
67. Luo Z, Ji Y, Tian D, Zhang Y, Chang S, Yang C, et al. Galectin-7 promotes proliferation and Th1/2 cells polarization toward Th1 in activated CD4+ T cells by inhibiting the TGFβ/Smad3 pathway. Mol Immunol (2018) 101:80–5. doi: 10.1016/j.molimm.2018.06.003
68. Sato S, St-Pierre C, Bhaumik P, Nieminen J. Galectins in innate immunity: dual functions of host soluble beta-galactoside-binding lectins as damage-associated molecular patterns (DAMPs) and as receptors for pathogen-associated molecular patterns (PAMPs). Immunol Rev (2009) 230(1):172–87. doi: 10.1111/j.1600-065X.2009.00790.x
Keywords: galectin-1, galectin-3, galectin-9, galectin-7, PSG1, SARS-CoV-2, COVID-19
Citation: Zhao F, Tallarek A-C, Wang Y, Xie Y, Diemert A, Lu-Culligan A, Vijayakumar P, Kittmann E, Urbschat C, Bayo J, Arck PC, Farhadian SF, Dveksler GS, Garcia MG and Blois SM (2023) A unique maternal and placental galectin signature upon SARS-CoV-2 infection suggests galectin-1 as a key alarmin at the maternal–fetal interface. Front. Immunol. 14:1196395. doi: 10.3389/fimmu.2023.1196395
Received: 29 March 2023; Accepted: 06 June 2023;
Published: 05 July 2023.
Edited by:
Ricardo Silvestre, University of Minho, PortugalReviewed by:
Patrícia Gonzalez-Dias, University of Oxford, United KingdomNora Heisterkamp, Beckman Research Institute, United States
Copyright © 2023 Zhao, Tallarek, Wang, Xie, Diemert, Lu-Culligan, Vijayakumar, Kittmann, Urbschat, Bayo, Arck, Farhadian, Dveksler, Garcia and Blois. This is an open-access article distributed under the terms of the Creative Commons Attribution License (CC BY). The use, distribution or reproduction in other forums is permitted, provided the original author(s) and the copyright owner(s) are credited and that the original publication in this journal is cited, in accordance with accepted academic practice. No use, distribution or reproduction is permitted which does not comply with these terms.
*Correspondence: Sandra M. Blois, cy5ibG9pc0B1a2UuZGU=