- 1Department of Clinical Laboratory, The Affiliated Children’s Hospital of Xi’an Jiaotong University, Xi’an, China
- 2Key Laboratory of Precision Medicine to Pediatric Diseases of Shaanxi Province, Shaanxi Institute for Pediatric Diseases, The Affiliated Children’s Hospital of Xi’an Jiaotong University, Xi’an, China
- 3Department of Infectious Diseases, The Affiliated Children’s Hospital of Xi’an Jiaotong University, Xi’an, China
- 4National Local Joint Engineering Research Center for Precision Surgery and Regenerative Medicine, The First Affiliated Hospital of Xi’an Jiaotong University, Xi’an, China
Acute liver injury (ALI) in children, which commonly leads to acute liver failure (ALF) with the need for liver transplantation, is a devastating life-threatening condition. As the orchestrated regulation of immune hemostasis in the liver is essential for resolving excess inflammation and promoting liver repair in a timely manner, in this study we focused on the immune inflammation and regulation with the functional involvement of both innate and adaptive immune cells in acute liver injury progression. In the context of the severe acute respiratory syndrome coronavirus-2 (SARS-CoV-2) pandemic, it was also important to incorporate insights from the immunological perspective for the hepatic involvement with SARS-CoV-2 infection, as well as the acute severe hepatitis of unknown origin in children since it was first reported in March 2022. Furthermore, molecular crosstalk between immune cells concerning the roles of damage-associated molecular patterns (DAMPs) in triggering immune responses through different signaling pathways plays an essential role in the process of liver injury. In addition, we also focused on DAMPs such as high mobility group box 1 (HMGB1) and cold-inducible RNA-binding protein (CIRP), as well as on macrophage mitochondrial DNA-cyclic GMP-AMP synthase (cGAS)-stimulator of interferon genes (STING) signaling pathway in liver injury. Our review also highlighted novel therapeutic approaches targeting molecular and cellular crosstalk and cell-based therapy, providing a future outlook for the treatment of acute liver injury.
1 Introduction
Acute liver injury (ALI) is characterized by a rapid decline of hepatic function with serum aminotransferases rising from mild to substantial levels, jaundice, and impaired coagulation function. It manifests in patients with baseline liver diseases and identifiable causes of liver damage, or without preexisting liver disease. Severe acute liver injury for fewer than 26 weeks duration with encephalopathy and an international normalized ratio (INR) higher than 1.5 in a patient without cirrhosis or preexisting liver disease is grouped into acute liver failure, which is a devastating life-threatening condition with a mortality of 5-10% (1). Usually, mild and moderate liver injury results in rapid and efficient regeneration through hepatocyte proliferation, whereas severe acute liver injury leads to failure of regeneration and involves a high risk of progression to acute liver failure (2). Pediatric acute liver failure (PALF) differs from adult acute liver failure due to the type and diversity of causes, and the subset of children with indeterminate etiology comprises up to 50% of the PALF population. Compared with groups of definite etiology, children with ALF of indeterminate etiology were more likely to require liver transplantation.
Upon appropriate immune activation by pathogens or tissue damage in the liver, tightly regulated inflammation is a homeostatic inflammatory process that resolves inflammation and promotes tissue regeneration, thus avoiding pathological consequences. Conversely, excessive and dysregulated hepatic immune and overactive inflammation in the liver lead to dyshomeostasis and pathological conditions. As the largest organ in the human body, the liver contains not only liver sinusoidal endothelial cells (LSECs), hepatocytes, hepatic stellate cells (HSCs), but also diverse populations of resident immune cells, such as Kupffer cells (KCs), dendritic cells (DCs), T cells, natural killer (NK) cells, natural killer T (NKT) cells, etc., which play central roles in hepatic immune balance. Therefore, the orchestrated regulation of immune hemostasis in the liver is essential for resolving excess inflammation and promoting liver repair and regeneration in a timely manner (3–5).
This review, therefore, focused on the immune activation and regulation with molecular and cellular crosstalk in the liver during acute liver injury/failure. Based on that, therapeutic strategies and targets for acute liver injury/failure have been summarized, which may provide clues to new therapeutic investigations on acute liver injury, especially in children (6).
2 Pediatric acute liver injury/failure in the SARS-CoV-2 pandemic era
Causes of acute liver injury/failure are diverse, including mainly para-acetaminophen (APAP) toxicity, cholestatic liver injury (CLI), alcoholic liver disease (ALD), non-alcoholic steatohepatitis (NASH), hepatic ischemia-reperfusion injury (I/R), virus-related liver injury, and hepatitis of unknown reasons in children (7, 8). Cause-based treatment of pediatric acute liver failure has been comprehensively reviewed by Deep et al. (1). However, virus-related liver injury became another important issue, especially in the SARS-CoV-2 pandemic era (9–11). Becchetti et al. reported alterations in liver enzymes among liver-transplanted patients with SARS-CoV-2 infection and increased in-hospital fatality in a European cohort of liver transplant recipients (12). New evidence for liver injury from SARS-CoV-2 infection has also been provided (13). As the primary receptor for SARS-CoV-2 cellular entry, the angiotensin-converting enzyme 2 (ACE2) receptors, which are expressed not only in the lung parenchyma but also in the gastrointestinal tract and liver epithelia, function in directing the contribution of the virus to the hepatic injury (11). Over-activated immune responses also contribute to liver injury in patients with SARS-CoV-2 infection, leading to cytokine storm syndrome, which may cause acute hepatitis and acute liver failure, even multiple organ failure (14–16).
Acute severe hepatitis of unknown origin in children is another new important issue that has gained international concern since it was first reported in Scotland on 31 March 2022. From 1 October 2021 to 8 July 2022, a total of 1,010 probable cases fulfilling the WHO case definition have been reported from 35 countries, of which 46 (5%) have required liver transplantation (LT), and 22 (2%) died. The etiology of this severe acute hepatitis in children is still not clear and under investigation. Based on the WHO working case definition, laboratory testing has excluded hepatitis A-E viruses that are known to cause acute viral hepatitis. Among the 1,010 probable cases, many cases had PCR assays for pathogen detection such as human adenovirus (HAdV) and SARS-CoV-2. According to the available data, HAdV was the most frequently detected pathogen with a cumulative of 209 PCR-positive cases (21%), and SARS-CoV-2 was detected in 78 cases (8%). In the European region, SARS-CoV-2 was detected by PCR in 16% of cases (54/335) and HAdV in 52% of cases (193/368). In the UK, SARS-CoV-2 was detected in 17% of cases (34/196) while HAdV was detected in 66% of cases (142/216). In the United States of America, SARS-CoV-2 was detected in 8% of cases (15/197). Reports from Japan indicated that SARS-CoV-2 was detected in 8% of cases (5/59) while HAdV was detected in 9% of cases (5/58) (17). A UK study reported that during the 2022 outbreak, over 50% of children with acute hepatitis without liver failure, and all indeterminate pediatric acute liver failure (ID-PALF) cases (10/10) had a presentation of adenoviremia, whereas the proportion of adenovirus-associated ID-PALF to all ID-PALF cases was lower from 2017 to 2021 (3/6, 1/4, 2/3, 0/3, and 0/5, respectively). The presentation of adenoviremia was probably linked to the higher rates of adenovirus infection in children during the outbreak, revealing that the 2022 outbreak was not a newly emerging disease (18). A retrospective review in the US showed an almost twofold increase of non-A-E severe acute hepatitis in children during 2021-2022 compared with the period of 2018-2021, which was associated with higher positive rates for viruses, with adenovirus (26.1%) and SARS-CoV2 (10.3%) being the most frequently detected viruses during the outbreak (19).
The results so far revealed that acute severe hepatitis in children may be related to HAdV or SARS-CoV-2 infection. It seems that HAdV infection was a facilitatory factor rather than a primary driver in the causation of the 2022 outbreak. A proposed mechanism is that superantigen-mediated aberrant immune response to HAdV in the gut following SARS-CoV-2 infection results in excessive IFN-γ release and thus in IFN- γ-mediated hepatocyte apoptosis (20, 21). SARS-CoV-2 virus in the gastrointestinal tract may release viral proteins across the intestinal epithelium and thereby accelerate the immune activation afterward. The superantigen hypothesis is so far the most popular theory to explain multisystem inflammatory syndrome in children (20, 21). Within the SARS-CoV-2 spike protein, there is a superantigen motif that resembles staphylococcal enterotoxin B. This superantigen can trigger strong and broad non-specific T cell activation, which was supported by the expansion of TRBV11-2 T cells in multisystem inflammatory syndrome in children (22–24). In addition to autoimmune hepatitis-like liver histology with CD8+ lymphocyte-predominant infiltration, Th1-type immune skewing, with remarkable peripheral CD8+ T-cell activation, was found in children with severe acute hepatitis of unknown origin (25).
3 Immune activation in acute liver injury/failure
Regulation of immune homeostasis within the liver has attracted much attention, especially under several pathological conditions. From the innate immunological aspect, the monocyte-macrophage system mediates liver inflammation and plays an essential role in acute liver injury and liver failure (26–28). Macrophages in the liver are mainly KCs, most of which are intravascular to engulf toxic solutes in blood, forming a powerful phagocyte network and providing efficient immune surveillance in the liver. Some KCs are also found in perivascular space interacting with HSCs and hepatocytes. On one hand, KCs combat foreign invaders such as bacteria and viruses; on the other hand, these cells contribute to the immune-compromised microenvironment in the liver to harness antigenic stimuli such as metabolic products by suppressing MHC expression in HSCs and hepatocytes (29, 30). Upon activation, KCs and monocyte-derived macrophages (MoMF) release pro-inflammatory cytokines to exacerbate inflammation and cause tissue damage. Neutrophils and macrophages begin to phagocytose necrotic debris and apoptotic bodies. Activated Kupffer cells, dendritic cells, stellate cells, and T cells facilitate the recruitment of neutrophils and monocytes from the systemic circulation via the secretion of chemoattractants such as CCL2 and IL-17 (31–33) (Table 1). Additionally, there are also NK cells, NKT cells, and γð T cells, which all contribute to the immune surveillance in the liver (55). The activated NK and NKT cells play roles in liver injury by impairing liver regeneration via TNF-α and IFN-γ release (53). γδ T-cells are also involved in the activation of neutrophils in liver fibrosis (54) (Figure 1A).
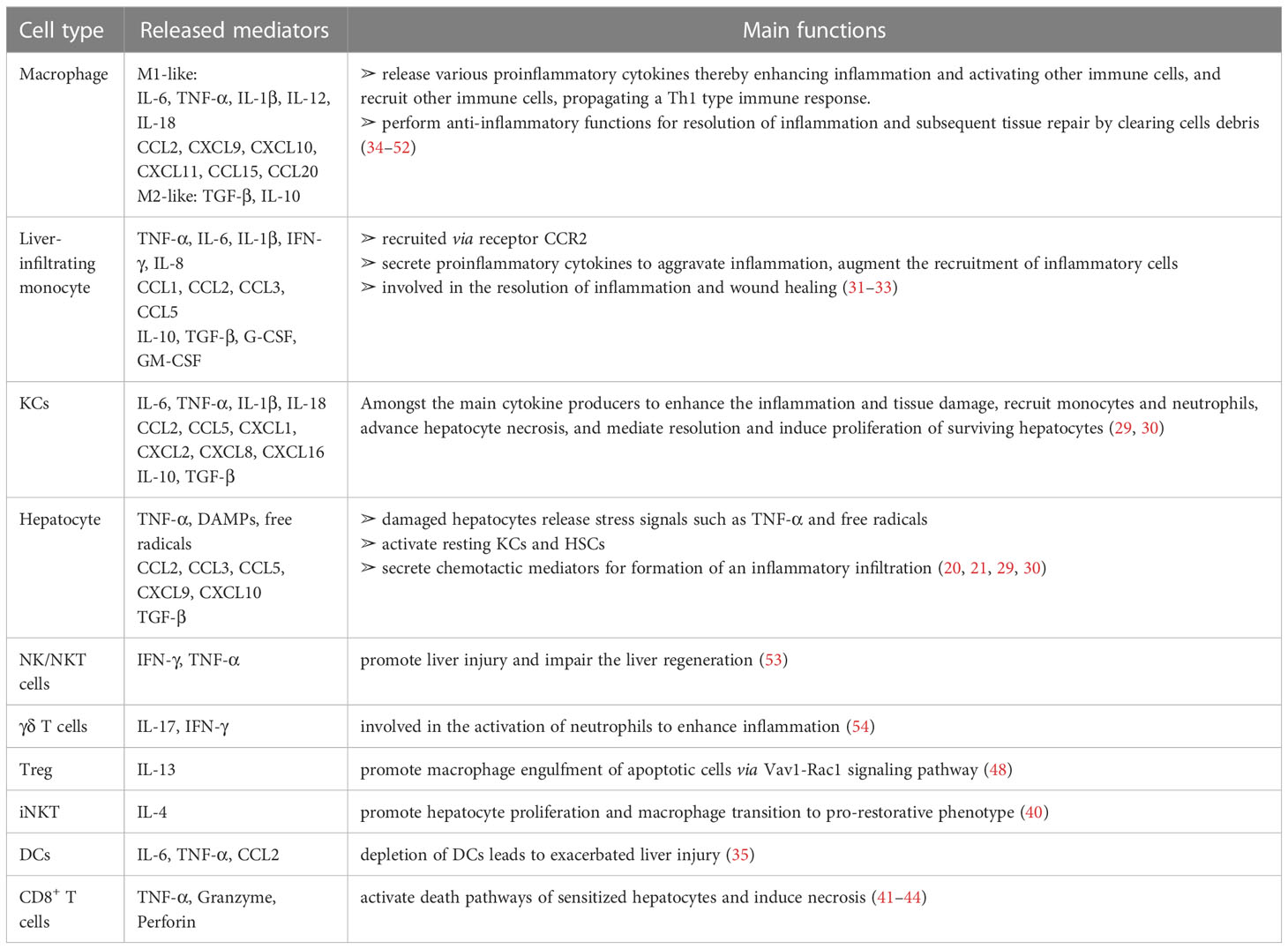
Table 1 The mediators (cytokines/chemokines/others) from various immune cells in liver inflammation during the injury/repair process.
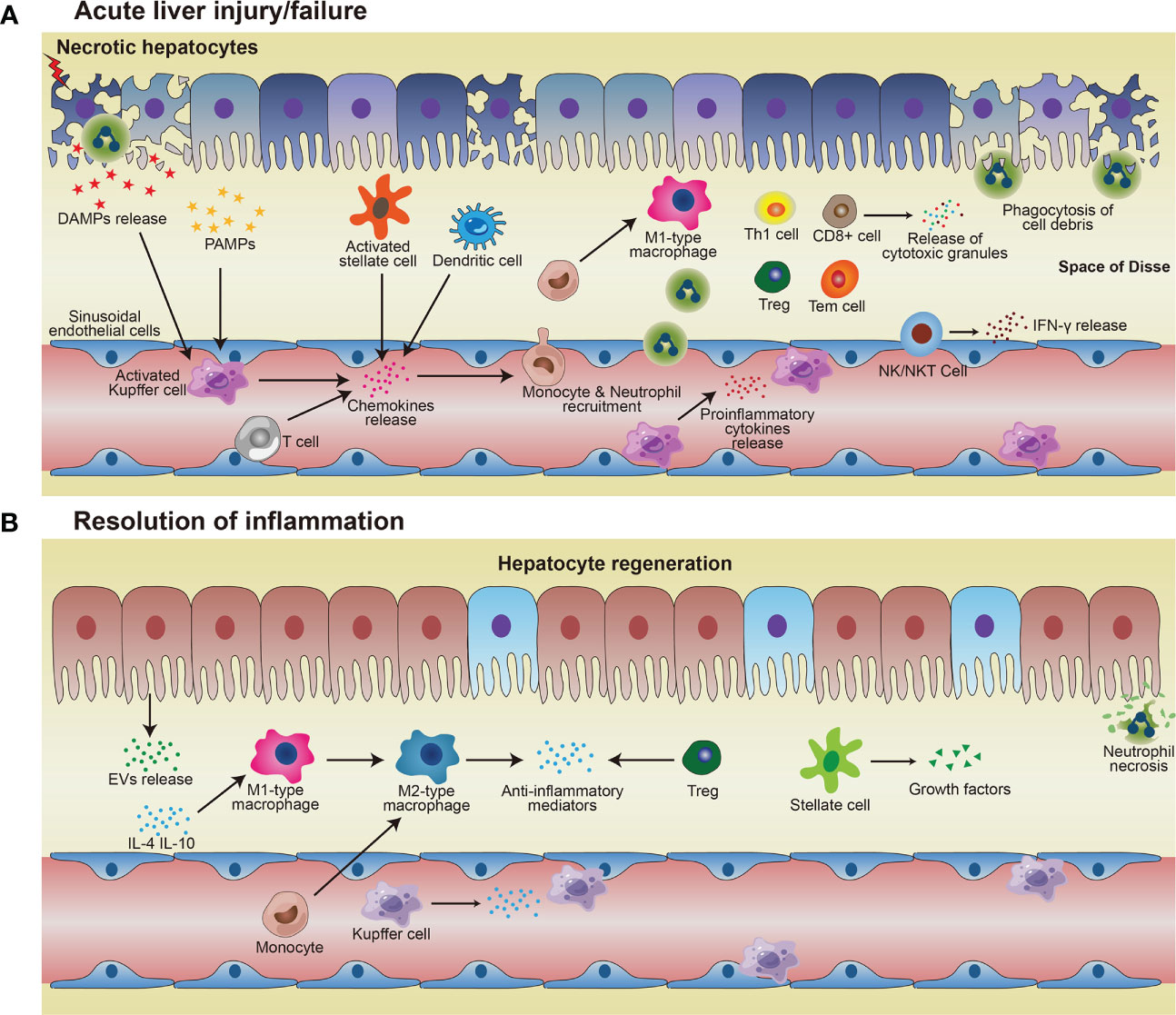
Figure 1 Immune responses in acute liver injury/failure and regeneration. (A) KCs and MoMF recognize DAMPs and PAMPs and then being activated, thus releasing pro-inflammatory cytokines to enhance inflammation and damage the local tissue. Activated KCs and other cells such as T cells, stellate cells, dendritic cells, and hepatocytes, secrete chemokines and thus attract monocytes and neutrophils to the site of injury. Monocytes migrate across the liver’s sinusoidal endothelial cell layer and differentiate into M1-type macrophages which exacerbate injury by pro-inflammatory cytokines. Neutrophils and macrophages phagocytose necrotic debris and apoptotic bodies. (B) In the process of resolution, restorative M2 macrophages phenotype release anti-inflammatory cytokines and promote neutrophil apoptosis, resulting in homeostasis restoration in the liver. Hepatocytes proliferate to replenish the lost parenchyma. Treg also contributes to anti-inflammatory conditions, to facilitate hepatocyte regeneration.
Following injury, proper resolution of the inflammation is the prerequisite for tissue repair and regeneration. Monocyte-macrophage system and cells, such as regulatory T cells (Treg) and HSCs, essentially mediate the resolution of inflammation. Induced by released anti-inflammatory mediators, such as IL-10 and TGF-β, from the inflamed liver (56), more pro-inflammatory M1-type macrophages could be polarized into restorative M2-type macrophages (34), which release anti-inflammatory cytokines and promote angiogenesis and neutrophil apoptosis, therefore aiding homeostasis restoration and tissue recovery. Depletion of DCs resulted in aggravation of liver injury, with a significantly higher expression of IL-6, CCL2, and TNF-α in serum, suggesting a protective role of DCs in liver injury (35). The mediators (cytokines/chemokines/etc.) from various immune cells in liver inflammation and repair processes are summarized in Table 1 (36, 37). Cells with immune suppressive function such as Treg, via facilitating osteoblast differentiation (38), and myeloid-derived suppressor cells (MDSCs), by promoting angiogenesis (46), are other important players within liver regeneration. Hepatocytes proliferate to replenish the lost parenchyma (Figure 1B). Self-antigen-driven invariant NKT (iNKT) cells promote hepatocyte proliferation and macrophage transition to pro-restorative phenotype via IL-4, thereby facilitating liver regeneration (40).
Besides the aforementioned, many studies revealed evidence that T cells played a role as central regulators in dysregulated and hyper-inflammatory immune activation in the liver, especially in PALF. As PALF is a complex and rapidly evolving clinical syndrome and almost 50% of PALF cases received the diagnosis of indeterminate without specific etiology, there are emerging insights into immune-mediated pathophysiology in PALF, revealing the role of T cells in inflammatory dysregulation in PALF. A model based on the percent of perforin and granzyme expression on CD8+ lymphocytes, absolute count of CD8+ T cells, and sIL2R predicted a high activation of circulating lymphocytes and linked to indeterminate etiology of PALF (41, 42). The characteristic phenotype of indeterminate PALF intrahepatic immune cells was perforin+CD103+CD8+ T-cell, which performs effector functions and expresses surface markers of tissue-resident memory T cells (43, 44). Other studies also reported that indeterminate PALF cases were characterized by predominant CD8+ T-cell infiltrates in the liver tissue (45, 46). Treg, following injections of CCl4, were remarkably expanded, and depletion of Treg enhanced the liver inflammation, demonstrating that Treg controls liver inflammation via regulating the aberrant activation and functions of immune effector cells (47). A mechanistic study expanded the role of Treg cells in inflammation resolution by secreting IL-13, which stimulates IL-10 production in macrophages, thus promoting macrophage engulfment of apoptotic cells via the Vav1-Rac1 signaling pathway (48). A transcriptional analysis identified an immune-driven PALF group, in which over 90% of patients were indeterminate PALF cases with moderate to dense CD8 staining, expressing increased levels of gene signatures for adaptive immune cells including Th1 cells, regulatory T cells, T effector memory cells, cytotoxic T cells, and innate immune cells including macrophages and activated dendritic cells. In addition, gene signatures for several immune cells, for instance, T central memory cells, Th2 cells, Th17 cells, and B cells, were not found significantly expressed in the indeterminate PALF group (49, 50). Although the exact mechanisms have not been fully clarified yet, the determined high levels of a gene signature of T cells, such as CD8+ T cells, Th1 cells, and T effector memory cells, indicate that a complex immune network regulates immune‐mediated liver injury in indeterminate PALF.
Extracellular vesicles (EV) were also reported to contribute to the M2 macrophage polarization (51, 52, 57). In a CCL4-induced ALI mouse model, the effects of EVs released from hepatocytes and other non-parenchymal cells on hepatic macrophages were explored, indicating that EVs play a pivotal role in liver regeneration by depolarizing MoMF and inducing Kuffer cells to M2-type (58, 59). Although M2-like macrophage response promotes injured liver repair and regeneration, the immune suppressive mediators released into the circulation can be harmful and lead to immune-compromised status, therefore increasing the susceptibility to infections, even immune paresis in sepsis in PALF (60–62). Therefore, immune evaluation for children with indeterminate, progressive hepatitis or indeterminate ALF should be carried out, including assessments for T cell activation, macrophage activation, NK cell function, and other serum biochemical markers (63). Such precise immune monitoring and evaluation will help to understand the phases of liver injury and the complicated coordination between immune cells and liver cells during the regeneration phase.
4 Molecular crosstalk between immune cells in the liver during acute liver injury/failure
Behind the cellular communication of immune cells in the liver, molecular crosstalk between them is essential for maintaining the “gatekeeper” function of hepatic immunity. During liver injury, release and activation of DAMPs result in a wide range of immune responses, including KCs activation, neutrophil recruitment to the site of injury, and induction of proinflammatory cytokines such as TNF-α that activate the NF-κB pathway in the hepatocyte (64).
High mobility group box 1 (HMGB1) is one of the most characterized DAMPs. HMGB1/TLR-4/NF-ĸB signaling directs the triggering of inflammation, innate and adaptive immune responses, and tissue healing after damage. Experimental evidence indicates that HMGB1 release from necrotic hepatocytes seems to be critical for neutrophils and monocyte recruitment, injury exacerbation, and lethality in paracetamol-mediated liver injury (65). Cold shock protein such as cold-inducible RNA-binding protein (CIRP) is a new DAMP molecule participating in acute live injury/failure and sepsis. Extracellular CIRP mainly stimulates monocytes/macrophages through TLR4/myeloid differentiation factor 2 (MD2) complex and the NF-κB pathway, promoting inflammatory responses (Figure 2) (66–69). On the other hand, the superfamily of heat shock proteins is also signaling molecules involved in the inflammatory response and restoration of homeostasis. Inhibition of HSP90 reduces proinflammatory cytokine production and prevents LPS-induced liver injury (70), whereas induction of endogenous HSP70 and HSP27 or treatment with exogenous HSP70 ameliorates the hepatic injury during experimental septic shock (71–73). HSPA12A, an atypical member of the heat shock protein 70 family, attenuates LPS-induced liver injury by inhibiting hepatocyte pyroptosis via PGC-1α-mediated AOAH expression (74). IL-33, also a classic hepatic DAMPs molecule, is actively involved in various inflammatory pathologies (75, 76). IL-6 trans-signaling drives the coagulopathy and hepatic endotheliopathy associated with COVID-19, which could be a possible mechanism behind liver injury as well (77).
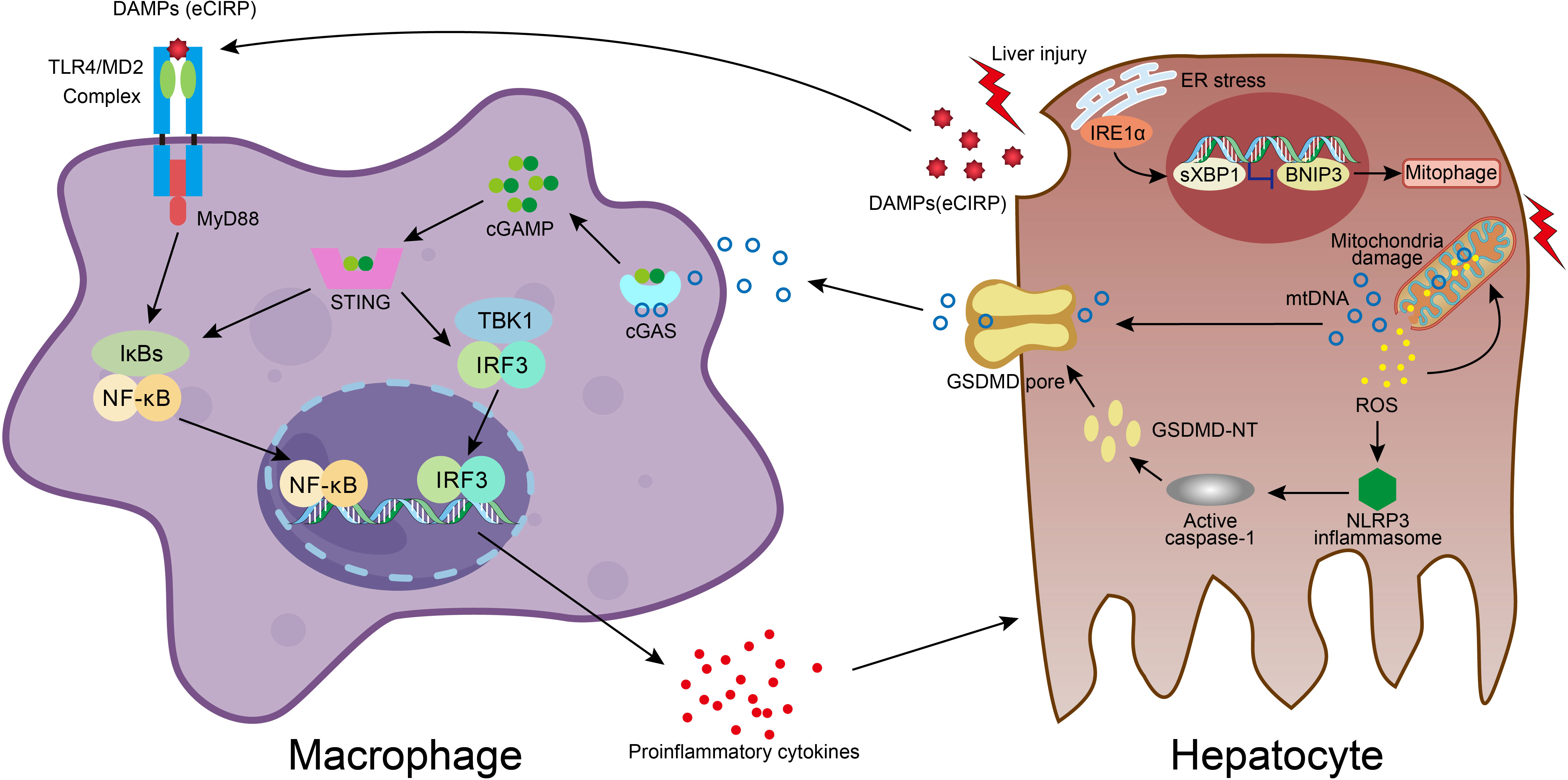
Figure 2 Molecular crosstalk between immune cells and liver cells in acute liver injury. DAMPs molecule eCIRP, released from damaged hepatocyte, stimulates macrophage through TLR4/MD2 complex and NF-κB pathway, resulting in proinflammatory mediators release, which exacerbates hepatocyte injury. In hepatocyte, accumulation of ROS in dysfunctional mitochondria upon damage leads to ROS-NLRP3-caspase-1-GSDMD activation and hepatocyte pyroptosis, which facilitates mtDNA extracellular release. Macrophage engulfs mtDNA and then the cGAS/cGAMP/STING signaling is activated with the secretion of proinflammatory cytokines. The liver injury also activates the IRE1a-XBP1 in the ER stress signaling pathway and leads to BNIP3-mediated mitophagy, which regulates mitochondrial homeostasis and promotes the clearance of cytosolic mtDNA. The XBP1 deficiency promotes mitophagy and thus effects the mtDNA release, and subsequently reduces the STING activation in macrophage.
As hepatocyte cell death is a major event and responsible for disease progression in acute liver injury, we also summarized different modes of cell death in immune dyshomeostasis. It is believed that DAMPs are released into the extracellular space largely in necrosis and necroptosis modes due to the loss of plasma membrane integrity and eventually cellular rupture, whereas integral plasma membrane and apoptotic bodies of apoptotic cells constrain DAMPs from being released into extracellular space. X-box binding protein 1 (XBP1) modulates the macrophage proinflammatory response under several pathogenic conditions. While activation of mitochondrial DNA (mtDNA)-cyclic GMP-AMP synthase (cGAS)-stimulator of interferon genes (STING) signaling in macrophage plays an important role in acute liver injury, it also plays a role in the activation of XBP1-mediated hepatocellular mitophagy and pyroptosis. Macrophage STING signaling pathways were observed in human livers with ALI and also acute lung injury in mice (Figure 2) (78–81). Pyroptosis is a profoundly inflammatory mode of regulated cell death related to the innate immune system. It has evolved to remove intracellular pathogens and has a cell-bursting morphology associated with pores in the plasma membrane formed by activated Gasdermin D (GSDMD) (82). It has been reported that inhibition and mutation of TNF-α could suppress the effects of HMGB1, thereby inhibiting the process of pyroptosis. The TNF-α/HMGB1 inflammation signaling pathway plays an important role in pyroptosis during liver failure and acute kidney injury (AKI) (83). Ferroptosis is also involved in APAP-induced cell death in primary hepatocytes, and Ferrostatin-1 as a ferroptosis inhibitor plays a protective role in APAP-treated primary hepatocytes (84).
5 Therapeutic strategies and targets for acute liver injury
Liver transplantation is the only curative treatment currently available for PALF, but there is a scarcity of livers for donation, thus clinical needs are not met. Novel therapy approaches have been explored that are less invasive than liver transplantation and could reduce posttransplant rejection risks and long-term immunosuppression management. As DAMPs play a pivotal role in the development and progression of acute liver disease, DAMPs can be harnessed as therapeutic targets. Targeting molecules such as HMGB1, HSP, CIRP, circulating free DNA, S100 proteins, and extracellular histones via reducing their release, promoting their removal, or inhibiting their signaling could be a promising strategy to ameliorate liver inflammation and minimize organ damage. For instance, treatment with a partly humanized anti-HMGB1 monoclonal antibody has hepatoprotective effects due to it blocking excessive amounts of extracellular HMGB1s, which decreases serum levels of ALT and microRNA-122 and eliminates inflammatory mediators in APAP-induced ALI (85). Accumulating evidence has shown that inhibition of different cell death modes can be utilized as a therapeutic strategy for acute liver diseases. Administration of ferric chelator Deferoxamine (Desferal) after APAP overdose significantly delayed the development of APAP-induced hepatotoxicity in mice (86). The synthetic compound Fer-1, which scavenges initiating alkoxyl radicals produced by ferrous iron from lipid hydroperoxides to produce the same anti-ferroptotic effect as GPx4, is a potent inhibitor of ferroptosis (87). Treatment with glycyrrhizin, an HMGB1 inhibitor, could increase GSH and GPX4 levels, and activate the Nrf2/HO-1/HMGB1 pathway, blocking ferroptosis in ALF models induced by the co-injection of d-galactosamine (d-GalN) and lipopolysaccharides (LPS) (88). Researchers have found that promethazine, one of the cytochrome P450 substrate compounds, functions as a lipid peroxyl radical scavenger to inhibit ferroptosis, ameliorating LPS/GalN-induced ALF and decreasing cell death (89). APAP overdose induced VDAC1 oligomerization in hepatocellular cells, and thus the application of VDAC1 oligomerization inhibitor VBIT-12 alleviated ferroptosis by protecting mitochondria and restoring ceramide and cardiolipin levels (90). In CCl4-induced acute liver injury, treatment with chloroquine downregulated HMGB1 levels and NF-kB expression, and increased the Bax/Bcl-2 ratio and caspase-3 activation in hepatic tissue, demonstrating inhibition of HMGB1-mediated inflammation and activation of pro-apoptotic pathways (91).
Cell-based therapy is a fast-developing research field in the restoration of liver function and treatment of various liver diseases and acts by remodeling and repairing the liver injury niche and promoting parenchyma regeneration. Hepatocyte transplantation has been explored for specific metabolic liver disorders, especially for pediatric patients and liver failure conditions (92), as well as for various drug-induced ALFs such as halothane, dilantin, and multiple polysubstance misuses (93, 94). Apart from human primary hepatocyte transplantation as the first form of cell therapy, stem cells are considered very promising for liver injury as well, such as embryonic stem cells (ESCs), hematopoietic stem cells (HSCs), induced pluripotent stem cells (iPSCs), and mesenchymal stem cells (MSCs), which have the ability to self-renew and differentiate, or function in immunomodulation, fibrosis degradation, and hepatocyte proliferation (95–97). Transplantation of ESC-derived hepatocytes into mice with APAP-induced acute liver failure rescued hepatic function (98). The infusion of umbilical cord-derived CD362-positive MSCs was demonstrated to alleviate hepatic inflammation by expanding the anti-inflammatory M2 phenotype of macrophages in a primary sclerosing cholangitis mouse model (99). Transplantation of human bone marrow mesenchymal stem cells (hBMSCs) into immunodeficient mice with fulminant hepatic failure induced restoration of the damaged liver by enhancing hBMSCs differentiation into cholangiocyte via delta ligand-like 4 (DLL4) activation (100). The effect of induced hepatocyte-like cells (iHEPs) generated by direct reprogramming from mouse embryonic fibroblast on acute liver injury was investigated in a CCl4-induced mouse model and significantly attenuated acute liver injury (101). Meanwhile, clinical trials on hepatocyte or stem cells transplantation have been investigated or are ongoing, among which MSCs are considered the most promising candidates (95). The overall safety and effectiveness of MSC therapy was evaluated and confirmed in 14 pediatric patients with urea cycle disorders (UCDs) or Crigler-Najjar (CN) syndrome 6 months post transplantation (102). A clinical trial on autologous MSCs transplantation for 158 patients, including teenagers, with liver failure caused by hepatitis B has been carried out to assess the short-term therapeutic effects and long-term fatality (NCT00956891) (Supplementary Table 1).
Additionally, various immune cells such as macrophages, Treg, monocytes, and DCs, are highlighted in the development of therapies for liver disorders. Given that macrophages play a crucial role in the progression and resolution of ALI, the value of bone marrow-derived macrophages (BMDMs) was identified as a cell-based therapy in an APAP-ALI mouse model. Injection of activated BMDMs reduced hepatocellular necrosis, infiltrating neutrophils, circulating proinflammatory cytokines levels, and HMGB1 translocation, meanwhile promoting hepatocyte and endothelium proliferation (103). Treg, which is pivotal in preventing autoimmunity, alloimmunity, and maintaining immunological tolerance and tissue homeostasis, has attracted great attention in adoptive cell therapy (104–106). The safety of autologous Treg infusion for four patients with autoimmune hepatitis (AIH) was evaluated, indicating that approximately 22-44% of infused Tregs homed to and resided in the liver for up to 72 hours, and acted to suppress the tissue-damaging effector T cells (107). In animal models, Treg could ameliorate APAP-induced immune-mediated liver injury and also inhibit CCl4 or triptolide-induced liver injury (51, 108–110). Treg played a crucial role in the MSC-based alleviation of acute liver inflammation, and adoptive transfer of MSC-primed Tregs completely attenuated α-GalCer-induced ALF, which indicated a novel therapeutic approach in Treg-based therapy of ALF (111).
6 Summary
In this review, we have focused on the immune activation and regulation in pediatric acute liver injury in the SARS-CoV-2 pandemic era. We summarized both the cellular and molecular crosstalk between immune cells and liver cells in this process as well as therapeutic targets and cell therapy strategies. Considerable progress in multiple therapeutic approaches has been made in the setting of acute liver injury and failure, especially in mice models. Based on former results, the combination of different therapeutic approaches might be promising for cell-based therapy of acute liver injury. However, more clinical trials are still required for validation of their safety and efficacy.
Author contributions
All authors contributed to the article and approved the submitted version.
Funding
This research was supported by grants from the National Nature Science Foundation of China (No. 82200728), the Doctoral Scientific Research Grant of Xi’an Children’s Hospital (No. 2021A03), and the Shaanxi Natural Science Research Program (No. 2022JM-480).
Conflict of interest
The authors declare that the research was conducted in the absence of any commercial or financial relationships that could be construed as a potential conflict of interest.
Publisher’s note
All claims expressed in this article are solely those of the authors and do not necessarily represent those of their affiliated organizations, or those of the publisher, the editors and the reviewers. Any product that may be evaluated in this article, or claim that may be made by its manufacturer, is not guaranteed or endorsed by the publisher.
Supplementary material
The Supplementary Material for this article can be found online at: https://www.frontiersin.org/articles/10.3389/fimmu.2023.1194588/full#supplementary-material
Abbreviations
BNIP3, BCL2/adenovirus E1B interacting protein 3; cGAS, cyclic GMP-AMP synthase; eCIRP, extracellular cold-inducible RNA-binding protein; ER, endoplasmic reticulum; GSDMD, Gasdermin D; IRE1a, inositol-requiring enzyme-1a; MD2, myeloid differentiation factor 2; mtDNA, mitochondrial DNA; STING, stimulator of interferon genes; TLR4, toll-like receptor 4; XBP1, X-box binding protein 1.
References
1. Deep A, Alexander EC, Bulut Y, Fitzpatrick E, Grazioli S, Heaton N, et al. Advances in medical management of acute liver failure in children: promoting native liver survival. Lancet Child Adolesc Health (2022) 6(10):725–37. doi: 10.1016/s2352-4642(22)00190-0
2. Stravitz RT, Lee WM. Acute liver failure. Lancet (2019) 394(10201):869–81. doi: 10.1016/s0140-6736(19)31894-x
3. Knolle PA, Wohlleber D. Immunological functions of liver sinusoidal endothelial cells. Cell Mol Immunol (2016) 13(3):347–53. doi: 10.1038/cmi.2016.5
4. Eckert C, Klein N, Kornek M, Lukacs-Kornek V. The complex myeloid network of the liver with diverse functional capacity at steady state and in inflammation. Front Immunol (2015) 6:179. doi: 10.3389/fimmu.2015.00179
5. Tacke F, Zimmermann HW. Macrophage heterogeneity in liver injury and fibrosis. J Hepatol (2014) 60(5):1090–6. doi: 10.1016/j.jhep.2013.12.025
6. Kulkarni S, Chapin CA, Alonso EM, Rudnick DA. An update on pediatric acute liver failure: emerging understanding of the impact of immune dysregulation and novel opportunities for intervention. Clin Liver Dis (2022) 26(3):461–71. doi: 10.1016/j.cld.2022.03.007
7. Berardi G, Tuckfield L, DelVecchio MT, Aronoff S. Differential diagnosis of acute liver failure in children: a systematic review. Pediatr Gastroenterol Hepatol Nutr (2020) 23(6):501–10. doi: 10.5223/pghn.2020.23.6.501
8. Jain V, Dhawan A. Prognostic modeling in pediatric acute liver failure. Liver Transpl (2016) 22(10):1418–30. doi: 10.1002/lt.24501
9. Lei F, Liu YM, Zhou F, Qin JJ, Zhang P, Zhu L, et al. Longitudinal association between markers of liver injury and mortality in COVID-19 in China. Hepatology (2020) 72(2):389–98. doi: 10.1002/hep.31301
10. Phipps MM, Barraza LH, LaSota ED, Sobieszczyk ME, Pereira MR, Zheng EX, et al. Acute liver injury in COVID-19: prevalence and association with clinical outcomes in a Large U.S. Cohor Hepatol (2020) 72(3):807–17. doi: 10.1002/hep.31404
11. Chai X, Hu L, Zhang Y, Han W, Lu Z, Ke A, et al. Specific ACE2 expression in cholangiocytes may cause liver damage after 2019-nCoV infection. bioRxiv (2020) 2020:2. doi: 10.1101/2020.02.03.931766
12. Becchetti C, Zambelli MF, Pasulo L, Donato MF, Invernizzi F, Detry O, et al. COVID-19 in an international European liver transplant recipient cohort. Gut (2020) 69(10):1832–40. doi: 10.1136/gutjnl-2020-321923
13. Wanner N, Andrieux G, Badia IMP, Edler C, Pfefferle S, Lindenmeyer MT, et al. Molecular consequences of SARS-CoV-2 liver tropism. Nat Metab (2022) 4(3):310–9. doi: 10.1038/s42255-022-00552-6
14. Liu J, Li S, Liu J, Liang B, Wang X, Wang H, et al. Longitudinal characteristics of lymphocyte responses and cytokine profiles in the peripheral blood of SARS-CoV-2 infected patients. EBioMedicine (2020) 55:102763. doi: 10.1016/j.ebiom.2020.102763
15. Bertoletti A, Le Bert N, Tan AT. SARS-CoV-2-specific T cells in the changing landscape of the COVID-19 pandemic. Immunity (2022) 55(10):1764–78. doi: 10.1016/j.immuni.2022.08.008
16. Mao R, Qiu Y, He JS, Tan JY, Li XH, Liang J, et al. Manifestations and prognosis of gastrointestinal and liver involvement in patients with COVID-19: a systematic review and meta-analysis. Lancet Gastroenterol Hepatol (2020) 5(7):667–78. doi: 10.1016/s2468-1253(20)30126-6
17. Organization WH. Severe acute hepatitis of unknown aetiology in children - multi-country. Dis Outbreak News (2022).
18. Jagadisan B, Verma A, Deheragoda M, Deep A, Grammatikopoulos T, Sudhanva M, et al. Outbreak of indeterminate acute liver failure in children with adenoviraemia - not a new disease. J Hepatol (2023). doi: 10.1016/j.jhep.2023.02.013
19. Peters AL, Kim S, Mourya R, Asai A, Taylor A, Rogers M, et al. Recent increase in incidence of severe acute hepatitis of unknown etiology in children is associated with infection with adenovirus and other non-hepatotropic viruses. J Pediatr (2023) 21:113439. doi: 10.1016/j.jpeds.2023.113439
20. Brodin P, Arditi M. Severe acute hepatitis in children: investigate SARS-CoV-2 superantigens. Lancet Gastroenterol Hepatol (2022) 7(7):594–5. doi: 10.1016/s2468-1253(22)00166-2
21. Noval Rivas M, Porritt RA, Cheng MH, Bahar I, Arditi M. COVID-19-associated multisystem inflammatory syndrome in children (MIS-c): a novel disease that mimics toxic shock syndrome-the superantigen hypothesis. J Allergy Clin Immunol (2021) 147(1):57–9. doi: 10.1016/j.jaci.2020.10.008
22. Porritt RA, Paschold L, Rivas MN, Cheng MH, Yonker LM, Chandnani H, et al. HLA class I-associated expansion of TRBV11-2 T cells in multisystem inflammatory syndrome in children. J Clin Invest (2021) 131(10):e146614. doi: 10.1172/jci146614
23. Kouo T, Chaisawangwong W. SARS-CoV-2 as a superantigen in multisystem inflammatory syndrome in children. J Clin Invest (2021) 131(10):e149327. doi: 10.1172/jci149327
24. Noval Rivas M, Porritt RA, Cheng MH, Bahar I, Arditi M. Multisystem inflammatory syndrome in children and long COVID: the SARS-CoV-2 viral superantigen hypothesis. Front Immunol (2022) 13:941009. doi: 10.3389/fimmu.2022.941009
25. Morita A, Imagawa K, Asayama K, Terakado T, Takahashi S, Yaita K, et al. Immunological characteristics of severe acute hepatitis of unknown origin in a child post SARS-CoV-2 infection. Clin Immunol (2022) 245:109138. doi: 10.1016/j.clim.2022.109138
26. Engelmann C, Habtesion A, Hassan M, Kerbert AJ, Hammerich L, Novelli S, et al. Combination of G-CSF and a TLR4 inhibitor reduce inflammation and promote regeneration in a mouse model of ACLF. J Hepatol (2022) 77(5):1325–38. doi: 10.1016/j.jhep.2022.07.006
27. Maheshwari D, Kumar D, Jagdish RK, Nautiyal N, Hidam A, Kumari R, et al. Bioenergetic failure drives functional exhaustion of monocytes in acute-on-Chronic liver failure. Front Immunol (2022) 13:856587. doi: 10.3389/fimmu.2022.856587
28. Li W, Chang N, Li L. Heterogeneity and function of kupffer cells in liver injury. Front Immunol (2022) 13:940867. doi: 10.3389/fimmu.2022.940867
29. Triantafyllou E, Woollard KJ, McPhail MJW, Antoniades CG, Possamai LA. The role of monocytes and macrophages in acute and acute-on-Chronic liver failure. Front Immunol (2018) 9:2948. doi: 10.3389/fimmu.2018.02948
30. Bonnardel J, T'Jonck W, Gaublomme D, Browaeys R, Scott CL, Martens L, et al. Stellate cells, hepatocytes, and endothelial cells imprint the kupffer cell identity on monocytes colonizing the liver macrophage niche. Immunity (2019) 51(4):638–54. doi: 10.1016/j.immuni.2019.08.017
31. Woolbright BL, Nguyen NT, McGill MR, Sharpe MR, Curry SC, Jaeschke H. Generation of pro-and anti-inflammatory mediators after acetaminophen overdose in surviving and non-surviving patients. Toxicol Lett (2022) 367:59–66. doi: 10.1016/j.toxlet.2022.07.813
32. Yin F, Wu MM, Wei XL, Ren RX, Liu MH, Chen CQ, et al. Hepatic NCoR1 deletion exacerbates alcohol-induced liver injury in mice by promoting CCL2-mediated monocyte-derived macrophage infiltration. Acta Pharmacol Sin (2022) 43(9):2351–61. doi: 10.1038/s41401-022-00863-0
33. Li J, Wang FP, She WM, Yang CQ, Li L, Tu CT, et al. Enhanced high-mobility group box 1 (HMGB1) modulates regulatory T cells (Treg)/T helper 17 (Th17) balance via toll-like receptor (TLR)-4-interleukin (IL)-6 pathway in patients with chronic hepatitis b. J Viral Hepat (2014) 21(2):129–40. doi: 10.1111/jvh.12152
34. Cho Y, Joshi R, Lowe P, Copeland C, Ribeiro M, Morel C, et al. Granulocyte colony-stimulating factor attenuates liver damage by M2 macrophage polarization and hepatocyte proliferation in alcoholic hepatitis in mice. Hepatol Commun (2022) 6(9):2322–39. doi: 10.1002/hep4.1925
35. Connolly MK, Ayo D, Malhotra A, Hackman M, Bedrosian AS, Ibrahim J, et al. Dendritic cell depletion exacerbates acetaminophen hepatotoxicity. Hepatology (2011) 54(3):959–68. doi: 10.1002/hep.24429
36. Liaskou E, Zimmermann HW, Li KK, Oo YH, Suresh S, Stamataki Z, et al. Monocyte subsets in human liver disease show distinct phenotypic and functional characteristics. Hepatology (2013) 57(1):385–98. doi: 10.1002/hep.26016
37. Campana L, Esser H, Huch M, Forbes S. Liver regeneration and inflammation: from fundamental science to clinical applications. Nat Rev Mol Cell Biol (2021) 22(9):608–24. doi: 10.1038/s41580-021-00373-7
38. Lei H, Schmidt-Bleek K, Dienelt A, Reinke P, Volk HD. Regulatory T cell-mediated anti-inflammatory effects promote successful tissue repair in both indirect and direct manners. Front Pharmacol (2015) 6:184. doi: 10.3389/fphar.2015.00184
39. Nachmany I, Bogoch Y, Sivan A, Amar O, Bondar E, Zohar N, et al. CD11b(+)Ly6G(+) myeloid-derived suppressor cells promote liver regeneration in a murine model of major hepatectomy. FASEB J (2019) 33(5):5967–78. doi: 10.1096/fj.201801733R
40. Liew PX, Lee WY, Kubes P. iNKT cells orchestrate a switch from inflammation to resolution of sterile liver injury. Immunity (2017) 47(4):752–65. doi: 10.1016/j.immuni.2017.09.016
41. Leonis MA, Miethke AG, Fei L, Maynor S, Chapin CA, Bleesing JJH, et al. Four biomarkers linked to activation of cluster of differentiation 8-positive lymphocytes predict clinical outcomes in pediatric acute liver failure. Hepatology (2021) 73(1):233–46. doi: 10.1002/hep.31271
42. Bucuvalas J, Filipovich L, Yazigi N, Narkewicz MR, Ng V, Belle SH, et al. Immunophenotype predicts outcome in pediatric acute liver failure. J Pediatr Gastroenterol Nutr (2013) 56(3):311–5. doi: 10.1097/MPG.0b013e31827a78b2
43. Chapin CA, Burn T, Meijome T, Loomes KM, Melin-Aldana H, Kreiger PA, et al. Indeterminate pediatric acute liver failure is uniquely characterized by a CD103(+) CD8(+) T-cell infiltrate. Hepatology (2018) 68(3):1087–100. doi: 10.1002/hep.29901
44. Chapin CA, Melin-Aldana H, Kreiger PA, Burn T, Neighbors K, Taylor SA, et al. Activated CD8 T-cell hepatitis in children with indeterminate acute liver failure: results from a multicenter cohort. J Pediatr Gastroenterol Nutr (2020) 71(6):713–9. doi: 10.1097/mpg.0000000000002893
45. McKenzie RB, Berquist WE, Nadeau KC, Louie CY, Chen SF, Sibley RK, et al. Novel protocol including liver biopsy to identify and treat CD8+ T-cell predominant acute hepatitis and liver failure. Pediatr Transplant (2014) 18(5):503–9. doi: 10.1111/petr.12296
46. Patel KR, Bertuch A, Sasa GS, Himes RW, Wu H. Features of hepatitis in hepatitis-associated aplastic anemia: clinical and histopathologic study. J Pediatr Gastroenterol Nutr (2017) 64(1):e7–e12. doi: 10.1097/mpg.0000000000001271
47. Ikeno Y, Ohara D, Takeuchi Y, Watanabe H, Kondoh G, Taura K, et al. Foxp3+ regulatory T cells inhibit CCl(4)-induced liver inflammation and fibrosis by regulating tissue cellular immunity. Front Immunol (2020) 11:584048. doi: 10.3389/fimmu.2020.584048
48. Proto JD, Doran AC, Gusarova G, Yurdagul A Jr., Sozen E, Subramanian M, et al. Regulatory T cells promote macrophage efferocytosis during inflammation resolution. Immunity (2018) 49(4):666–77. doi: 10.1016/j.immuni.2018.07.015
49. Chapin CA, Taylor SA, Malladi P, Neighbors K, Melin-Aldana H, Kreiger PA, et al. Transcriptional analysis of liver tissue identifies distinct phenotypes of indeterminate pediatric acute liver failure. Hepatol Commun (2021) 5(8):1373–84. doi: 10.1002/hep4.1726
50. Xu C, Lu Y, Zheng X, Feng X, Yang X, Timm J, et al. TLR2 expression in peripheral CD4+ T cells promotes Th17 response and is associated with disease aggravation of hepatitis b virus-related acute-On-Chronic liver failure. Front Immunol (2017) 8:1609. doi: 10.3389/fimmu.2017.01609
51. Isaacs-Ten A, Echeandia M, Moreno-Gonzalez M, Brion A, Goldson A, Philo M, et al. Intestinal microbiome-macrophage crosstalk contributes to cholestatic liver disease by promoting intestinal permeability in mice. Hepatology (2020) 72(6):2090–108. doi: 10.1002/hep.31228
52. Li X, Liu R, Wang Y, Zhu W, Zhao D, Wang X, et al. Cholangiocyte-derived exosomal lncRNA H19 promotes macrophage activation and hepatic inflammation under cholestatic conditions. Cells (2020) 9(1):190. doi: 10.3390/cells9010190
53. dos Santos DC, Neves PC, Azeredo EL, Pelajo-Machado M, Martinho JM, Pacheco-Moreira LF, et al. Activated lymphocytes and high liver expression of IFN-γ are associated with fulminant hepatic failure in patients. Liver Int (2012) 32(1):147–57. doi: 10.1111/j.1478-3231.2011.02654.x
54. Liu Q, Yang Q, Wu Z, Chen Y, Xu M, Zhang H, et al. IL-1β-activated mTORC2 promotes accumulation of IFN-γ(+) γδ T cells by upregulating CXCR3 to restrict hepatic fibrosis. Cell Death Dis (2022) 13(4):289. doi: 10.1038/s41419-022-04739-3
55. Chen Y, Tian Z. Innate lymphocytes: pathogenesis and therapeutic targets of liver diseases and cancer. Cell Mol Immunol (2021) 18(1):57–72. doi: 10.1038/s41423-020-00561-z
56. Possamai LA, Thursz MR, Wendon JA, Antoniades CG. Modulation of monocyte/macrophage function: a therapeutic strategy in the treatment of acute liver failure. J Hepatol (2014) 61(2):439–45. doi: 10.1016/j.jhep.2014.03.031
57. Hirsova P, Ibrahim SH, Krishnan A, Verma VK, Bronk SF, Werneburg NW, et al. Lipid-induced signaling causes release of inflammatory extracellular vesicles from hepatocytes. Gastroenterology (2016) 150(4):956–67. doi: 10.1053/j.gastro.2015.12.037
58. Lv XF, Zhang AQ, Liu WQ, Zhao M, Li J, He L, et al. Liver injury changes the biological characters of serum small extracellular vesicles and reprograms hepatic macrophages in mice. World J Gastroenterol (2021) 27(43):7509–29. doi: 10.3748/wjg.v27.i43.7509
59. Umbaugh DS, Jaeschke H. Extracellular vesicles: roles and applications in drug-induced liver injury. Adv Clin Chem (2021) 102:63–125. doi: 10.1016/bs.acc.2020.08.010
60. Lexmond WS, de Meijer VE, Scheenstra R, Bontemps STH, Duiker EW, Schölvinck EH, et al. Indeterminate pediatric acute liver failure: clinical characteristics of a temporal cluster of five children in the Netherlands in the spring of 2022. United Eur Gastroenterol J (2022) 10(8):795–804. doi: 10.1002/ueg2.12269
61. Lorencio Cárdenas C, Yébenes JC, Vela E, Clèries M, Sirvent JM, Fuster-Bertolín C, et al. Trends in mortality in septic patients according to the different organ failure during 15 years. Crit Care (2022) 26(1):302. doi: 10.1186/s13054-022-04176-w
62. Zhang Z, Yang Z, Cheng Q, Hu X, Liu M, Liu Y, et al. Establishment and validation of a prognostic model for hepatitis b virus−related acute-on-chronic liver failure patients with bacterial infection. Hepatol Int (2022) 16(1):38–47. doi: 10.1007/s12072-021-10268-6
63. DiPaola F, Grimley M, Bucuvalas J. Pediatric acute liver failure and immune dysregulation. J Pediatr (2014) 164(2):407–9. doi: 10.1016/j.jpeds.2013.10.044
64. Su L, Li N, Tang H, Lou Z, Chong X, Zhang C, et al. Kupffer cell-derived TNF-α promotes hepatocytes to produce CXCL1 and mobilize neutrophils in response to necrotic cells. Cell Death Dis (2018) 9(3):323. doi: 10.1038/s41419-018-0377-4
65. Luo L, Wang S, Chen B, Zhong M, Du R, Wei C, et al. Inhibition of inflammatory liver injury by the HMGB1-a box through HMGB1/TLR-4/NF-κB signaling in an acute liver failure mouse model. Front Pharmacol (2022) 13:990087. doi: 10.3389/fphar.2022.990087
66. Qiang X, Yang WL, Wu R, Zhou M, Jacob A, Dong W, et al. Cold-inducible RNA-binding protein (CIRP) triggers inflammatory responses in hemorrhagic shock and sepsis. Nat Med (2013) 19(11):1489–95. doi: 10.1038/nm.3368
67. Khan MM, Yang WL, Brenner M, Bolognese AC, Wang P. Cold-inducible RNA-binding protein (CIRP) causes sepsis-associated acute lung injury via induction of endoplasmic reticulum stress. Sci Rep (2017) 7:41363. doi: 10.1038/srep41363
68. Liu W, Fan Y, Ding H, Han D, Yan Y, Wu R, et al. Normothermic machine perfusion attenuates hepatic ischaemia-reperfusion injury by inhibiting CIRP-mediated oxidative stress and mitochondrial fission. J Cell Mol Med (2021) 25(24):11310–21. doi: 10.1111/jcmm.17062
69. Godwin A, Yang WL, Sharma A, Khader A, Wang Z, Zhang F, et al. Blocking cold-inducible RNA-binding protein protects liver from ischemia-reperfusion injury. Shock (2015) 43(1):24–30. doi: 10.1097/shk.0000000000000251
70. Ambade A, Catalano D, Lim A, Mandrekar P. Inhibition of heat shock protein (molecular weight 90 kDa) attenuates proinflammatory cytokines and prevents lipopolysaccharide-induced liver injury in mice. Hepatology (2012) 55(5):1585–95. doi: 10.1002/hep.24802
71. Hsu JH, Yang RC, Lin SJ, Liou SF, Dai ZK, Yeh JL, et al. Exogenous heat shock cognate protein 70 pretreatment attenuates cardiac and hepatic dysfunction with associated anti-inflammatory responses in experimental septic shock. Shock (2014) 42(6):540–7. doi: 10.1097/shk.0000000000000254
72. Zhang Y, Song C, Ni W, Pei Q, Wang C, Ying Y, et al. HSP70 ameliorates septic acute kidney injury via binding with TRAF6 to inhibit of inflammation-mediated apoptosis. J Inflammation Res (2022) 15:2213–28. doi: 10.2147/jir.S352717
73. Dai HJ, Li DW, Wang YX, Sun AJ, Lu YX, Ding X, et al. Induction of heat shock protein 27 by bicyclol attenuates d-galactosamine/lipopolysaccharide-induced liver injury. Eur J Pharmacol (2016) 791:482–90. doi: 10.1016/j.ejphar.2016.09.002
74. Liu J, Du S, Kong Q, Zhang X, Jiang S, Cao X, et al. HSPA12A attenuates lipopolysaccharide-induced liver injury through inhibiting caspase-11-mediated hepatocyte pyroptosis via PGC-1α-dependent acyloxyacyl hydrolase expression. Cell Death Differ (2020) 27(9):2651–67. doi: 10.1038/s41418-020-0536-x
75. Su Z, Lin J, Lu F, Zhang X, Zhang L, Gandhi NB, et al. Potential autocrine regulation of interleukin-33/ST2 signaling of dendritic cells in allergic inflammation. Mucosal Immunol (2013) 6(5):921–30. doi: 10.1038/mi.2012.130
76. Carriere V, Roussel L, Ortega N, Lacorre DA, Americh L, Aguilar L, et al. IL-33, the IL-1-like cytokine ligand for ST2 receptor, is a chromatin-associated nuclear factor in vivo. Proc Natl Acad Sci U.S.A. (2007) 104(1):282–7. doi: 10.1073/pnas.0606854104
77. McConnell MJ, Kawaguchi N, Kondo R, Sonzogni A, Licini L, Valle C, et al. Liver injury in COVID-19 and IL-6 trans-signaling-induced endotheliopathy. J Hepatol (2021) 75(3):647–58. doi: 10.1016/j.jhep.2021.04.050
78. Liu Z, Wang M, Wang X, Bu Q, Wang Q, Su W, et al. XBP1 deficiency promotes hepatocyte pyroptosis by impairing mitophagy to activate mtDNA-cGAS-STING signaling in macrophages during acute liver injury. Redox Biol (2022) 52:102305. doi: 10.1016/j.redox.2022.102305
79. Wang Q, Bu Q, Liu M, Zhang R, Gu J, Li L, et al. XBP1-mediated activation of the STING signalling pathway in macrophages contributes to liver fibrosis progression. JHEP Rep (2022) 4(11):100555. doi: 10.1016/j.jhepr.2022.100555
80. Zhang W, Li G, Luo R, Lei J, Song Y, Wang B, et al. Cytosolic escape of mitochondrial DNA triggers cGAS-STING-NLRP3 axis-dependent nucleus pulposus cell pyroptosis. Exp Mol Med (2022) 54(2):129–42. doi: 10.1038/s12276-022-00729-9
81. Ning L, Wei W, Wenyang J, Rui X, Qing G. Cytosolic DNA-STING-NLRP3 axis is involved in murine acute lung injury induced by lipopolysaccharide. Clin Transl Med (2020) 10(7):e228. doi: 10.1002/ctm2.228
82. Galluzzi L, Vitale I, Aaronson SA, Abrams JM, Adam D, Agostinis P, et al. Molecular mechanisms of cell death: recommendations of the nomenclature committee on cell death 2018. Cell Death Differ (2018) 25(3):486–541. doi: 10.1038/s41418-017-0012-4
83. Wang Y, Zhang H, Chen Q, Jiao F, Shi C, Pei M, et al. TNF-α/HMGB1 inflammation signalling pathway regulates pyroptosis during liver failure and acute kidney injury. Cell Prolif (2020) 53(6):e12829. doi: 10.1111/cpr.12829
84. Yamada N, Karasawa T, Kimura H, Watanabe S, Komada T, Kamata R, et al. Ferroptosis driven by radical oxidation of n-6 polyunsaturated fatty acids mediates acetaminophen-induced acute liver failure. Cell Death Dis (2020) 11(2):144. doi: 10.1038/s41419-020-2334-2
85. Lundbäck P, Lea JD, Sowinska A, Ottosson L, Fürst CM, Steen J, et al. A novel high mobility group box 1 neutralizing chimeric antibody attenuates drug-induced liver injury and postinjury inflammation in mice. Hepatology (2016) 64(5):1699–710. doi: 10.1002/hep.28736
86. Schnellmann JG, Pumford NR, Kusewitt DF, Bucci TJ, Hinson JA. Deferoxamine delays the development of the hepatotoxicity of acetaminophen in mice. Toxicol Lett (1999) 106(1):79–88. doi: 10.1016/s0378-4274(99)00021-1
87. Miotto G, Rossetto M, Di Paolo ML, Orian L, Venerando R, Roveri A, et al. Insight into the mechanism of ferroptosis inhibition by ferrostatin-1. Redox Biol (2020) 28:101328. doi: 10.1016/j.redox.2019.101328
88. Wang Y, Chen Q, Shi C, Jiao F, Gong Z. Mechanism of glycyrrhizin on ferroptosis during acute liver failure by inhibiting oxidative stress. Mol Med Rep (2019) 20(5):4081–90. doi: 10.3892/mmr.2019.10660
89. Mishima E, Sato E, Ito J, Yamada KI, Suzuki C, Oikawa Y, et al. Drugs repurposed as antiferroptosis agents suppress organ damage, including AKI, by functioning as lipid peroxyl radical scavengers. J Am Soc Nephrol (2020) 31(2):280–96. doi: 10.1681/asn.2019060570
90. Niu B, Lei X, Xu Q, Ju Y, Xu D, Mao L, et al. Protecting mitochondria via inhibiting VDAC1 oligomerization alleviates ferroptosis in acetaminophen-induced acute liver injury. Cell Biol Toxicol (2022) 38(3):505–30. doi: 10.1007/s10565-021-09624-x
91. Dai C, Xiao X, Li D, Tun S, Wang Y, Velkov T, et al. Chloroquine ameliorates carbon tetrachloride-induced acute liver injury in mice via the concomitant inhibition of inflammation and induction of apoptosis. Cell Death Dis (2018) 9(12):1164. doi: 10.1038/s41419-018-1136-2
92. Hansel MC, Gramignoli R, Skvorak KJ, Dorko K, Marongiu F, Blake W, et al. The history and use of human hepatocytes for the treatment of liver diseases: the first 100 patients. Curr Protoc Toxicol (2014) 62:14. doi: 10.1002/0471140856.tx1412s62
93. Ashmore-Harris C, Fruhwirth GO. Generation of In vivo traceable hepatocyte-like cells from human iPSCs. Methods Mol Biol (2022) 2544:15–49. doi: 10.1007/978-1-0716-2557-6_2
94. Strom SC, Fisher RA, Thompson MT, Sanyal AJ, Cole PE, Ham JM, et al. Hepatocyte transplantation as a bridge to orthotopic liver transplantation in terminal liver failure. Transplantation (1997) 63(4):559–69. doi: 10.1097/00007890-199702270-00014
95. Hofmann J, Hackl V, Esser H, Meszaros AT, Fodor M, Öfner D, et al. Cell-based regeneration and treatment of liver diseases. Int J Mol Sci (2021) 22(19):10276. doi: 10.3390/ijms221910276
96. Yang Y, Dong G, Bi Y, Zhang X, Yao X, Jin G, et al. Human liver stem cells alleviate con-a induced liver injury by regulating the balance of Treg/Th17 cells. Transpl Immunol (2022) 74:101632. doi: 10.1016/j.trim.2022.101632
97. Bi Y, Li J, Yang Y, Wang Q, Wang Q, Zhang X, et al. Human liver stem cells attenuate concanavalin a-induced acute liver injury by modulating myeloid-derived suppressor cells and CD4(+) T cells in mice. Stem Cell Res Ther (2019) 10(1):22. doi: 10.1186/s13287-018-1128-2
98. Tolosa L, Caron J, Hannoun Z, Antoni M, López S, Burks D, et al. Transplantation of hESC-derived hepatocytes protects mice from liver injury. Stem Cell Res Ther (2015) 6:246. doi: 10.1186/s13287-015-0227-6
99. Vigneswara V, Alfaifi M, Hedegaard D, Haldar D, Chen Y, Gargesha M, et al. Cd362+ human mesenchymal stromal cells reduce hepatic inflammation and induce M2 macrophage polarisation in murine models of primary sclerosing cholangitis. J Hepatol (2016) 64(2):S179. doi: 10.1016/S0168-8278(16)00109-4
100. Sun S, Yuan L, An Z, Shi D, Xin J, Jiang J, et al. DLL4 restores damaged liver by enhancing hBMSC differentiation into cholangiocytes. Stem Cell Res (2020) 47:101900. doi: 10.1016/j.scr.2020.101900
101. Park S, In Hwang S, Kim J, Hwang S, Kang S, Yang S, et al. The therapeutic potential of induced hepatocyte-like cells generated by direct reprogramming on hepatic fibrosis. Stem Cell Res Ther (2019) 10(1):21. doi: 10.1186/s13287-018-1127-3
102. Smets F, Dobbelaere D, McKiernan P, Dionisi-Vici C, Broué P, Jacquemin E, et al. Phase I/II trial of liver-derived mesenchymal stem cells in pediatric liver-based metabolic disorders: a prospective, open label, multicenter, partially randomized, safety study of one cycle of heterologous human adult liver-derived progenitor cells (HepaStem) in urea cycle disorders and crigler-najjar syndrome patients. Transplantation (2019) 103(9):1903–15. doi: 10.1097/tp.0000000000002605
103. Starkey Lewis P, Campana L, Aleksieva N, Cartwright JA, Mackinnon A, O'Duibhir E, et al. Alternatively activated macrophages promote resolution of necrosis following acute liver injury. J Hepatol (2020) 73(2):349–60. doi: 10.1016/j.jhep.2020.02.031
104. Dong S, Hiam-Galvez KJ, Mowery CT, Herold KC, Gitelman SE, Esensten JH, et al. The effect of low-dose IL-2 and treg adoptive cell therapy in patients with type 1 diabetes. JCI Insight (2021) 6(18):e147474. doi: 10.1172/jci.insight.147474
105. Roemhild A, Otto NM, Moll G, Abou-El-Enein M, Kaiser D, Bold G, et al. Regulatory T cells for minimising immune suppression in kidney transplantation: phase I/IIa clinical trial. Bmj (2020) 371:m3734. doi: 10.1136/bmj.m3734
106. Sawitzki B, Harden PN, Reinke P, Moreau A, Hutchinson JA, Game DS, et al. Regulatory cell therapy in kidney transplantation (The ONE study): a harmonised design and analysis of seven non-randomised, single-arm, phase 1/2A trials. Lancet (2020) 395(10237):1627–39. doi: 10.1016/s0140-6736(20)30167-7
107. Oo YH, Ackrill S, Cole R, Jenkins L, Anderson P, Jeffery HC, et al. Liver homing of clinical grade tregs after therapeutic infusion in patients with autoimmune hepatitis. JHEP Rep (2019) 1(4):286–96. doi: 10.1016/j.jhepr.2019.08.001
108. Wang X, Sun R, Chen Y, Lian ZX, Wei H, Tian Z. Regulatory T cells ameliorate acetaminophen-induced immune-mediated liver injury. Int Immunopharmacol (2015) 25(2):293–301. doi: 10.1016/j.intimp.2015.02.008
109. Xia F, Hu X, Zhang B, Wang X, Guan Y, Lin P, et al. Ultrasmall ruthenium nanoparticles with boosted antioxidant activity upregulate regulatory T cells for highly efficient liver injury therapy. Small (2022) 18(29):e2201558. doi: 10.1002/smll.202201558
110. Wang X, Sun L, Zhang L, Jiang Z. Effect of adoptive transfer or depletion of regulatory T cells on triptolide-induced liver injury. Front Pharmacol (2016) 7:99. doi: 10.3389/fphar.2016.00099
Keywords: acute liver injury, inflammation, damage associated molecular patterns, macrophages, cell therapy
Citation: Duan M, Liu X, Yang Y, Zhang Y, Wu R, Lv Y and Lei H (2023) Orchestrated regulation of immune inflammation with cell therapy in pediatric acute liver injury. Front. Immunol. 14:1194588. doi: 10.3389/fimmu.2023.1194588
Received: 27 March 2023; Accepted: 26 May 2023;
Published: 22 June 2023.
Edited by:
Xiaoyong Bao, University of Texas Medical Branch at Galveston, United StatesCopyright © 2023 Duan, Liu, Yang, Zhang, Wu, Lv and Lei. This is an open-access article distributed under the terms of the Creative Commons Attribution License (CC BY). The use, distribution or reproduction in other forums is permitted, provided the original author(s) and the copyright owner(s) are credited and that the original publication in this journal is cited, in accordance with accepted academic practice. No use, distribution or reproduction is permitted which does not comply with these terms.
*Correspondence: Hong Lei, bGVpaG9uZ25rQDE2My5jb20=