- 1Department of Epidemiology and Biostatistics, School of Public Health, Anhui Medical University, Hefei, Anhui, China
- 2Institute of Kidney Disease, Inflammation and Immunity Mediated Diseases, The Second Hospital of Anhui Medical University, Hefei, Anhui, China
- 3Department of Rheumatology and Immunology, The First Affiliated Hospital of Anhui Medical University, Hefei, Anhui, China
Objective: The purpose of this study was to precisely evaluate the serum Dickkopf-1 (DKK-1) level in patients with ankylosing spondylitis (AS) relative to that in normal controls and to test the causal relationship between DKK-1 and the risk of AS.
Methods: Embase, PubMed, Web of Science, WANFANG DATA, VIP, and China National Knowledge Infrastructure (CNKI) were comprehensively searched until July 2022 for pertinent studies. The pooled standardized mean difference (SMD) with a 95% confidence interval (CI) was calculated by the fixed or random-effect model. In Mendelian randomization (MR) analysis on the causal relationship between serum DKK-1 level and AS risk, the inverse variance weighting method (IVW), MR-Egger regression, weighted median method, and weighted pattern method were applied. Sensitivity analyses, including the horizontal pleiotropy test, heterogeneity test, and leave-one-out test, were also performed.
Results: The meta-analysis of 40 studies containing 2,371 AS patients and 1,633 healthy controls showed that there was no significant difference in DKK-1 serum level between AS patients and normal controls (pooled SMD=0.207, 95% CI =−0.418-0.832, P=0.516). The subgroup analysis of the CRP ≤ 10 mg/L group showed that AS patients had higher serum DKK-1 concentration than the healthy controls (SMD=2.267, 95% CI = 0.102-4.432, P=0.040). Similarly, MR analysis also demonstrated no significant association between DKK-1 serum level and AS (IVW OR=0.999, 95% CI = 0.989-1.008, P=0.800). All sensitivity analyses revealed consistent results.
Conclusions: There was no significant change in serum DKK-1 concentration between AS patients and healthy controls. In addition, no causal relationship exists between serum DKK-1 levels and AS risk.
1 Introduction
Ankylosing spondylitis (AS) is a type of spondyloarthropathy (SpA), which is immune-mediated and associated with a chronic inflammatory response. The primary pathological changes of AS are spinal and sacroiliac joint involvement, excessive ossification and inflammatory osteopenia, spinal joint fusion, bamboo-like shape, reduced mobility resulting in spinal rigidity and back pain, and other clinical manifestations (1). The worldwide prevalence of AS is 0.07%-0.32%, and men are more susceptible to the disease than women (2). It not only places a burden of disease on patients but also increases the social burden due to loss of quality of life and psychological harm to patients (3). Early diagnosis and biological therapy can enhance the life quality of patients to some extent by decreasing the incidence of spinal joint fusion (4). The etiology of AS has not been elucidated. Several relevant factors were revealed to be associated with the risk of AS including genetic, immune, microbial, and endocrine factors (1). In terms of immune factors, pro-inflammatory cytokines, such as tumor necrosis factor-α (TNF-α) and interleukin 17 (IL-17), have been known to play important roles in the etiology of AS (5). Dickkopf-1 (DKK-1) can interact with these cytokines and it may participate in inflammation and autoimmune responses (6). The Wnt signaling pathway is a crucial pathway in the new bone formation mechanism of AS, and the DKK-1 protein on the pathway may affect the occurrence of AS.
As the founding member of the DKK family, DKK-1 is a cysteine-rich secretory glycoprotein that strongly inhibits the Wnt/β-catenin pathway and is soluble (7). DKK-1 competes with Wnt ligand, occupies low-density lipoprotein receptor-associated protein 5/6 (LRP5/6), and binds frizzled proteins to promote the internalization of LRP5/6 receptor, thus inhibiting downstream Wnt signaling, further leading to the degradation of β-catenin and limiting the expression of Wnt target genes (8, 9). One previous study has shown that the serum level of DKK-1 bounding to LRP6 is down-regulated in AS patients (10). The obstruction of this typical Wnt pathway by DKK-1 may interfere with bone metabolism by affecting osteoblasts and osteoclasts (11, 12).
Many studies have been conducted to investigate the serum DKK-1 level of AS patients. A previous meta-analysis including 7 case-control studies from 2010 to 2012 indicated that the level of serum DKK-1 in patients with AS was significantly higher than that in healthy controls (13). However, another recent meta-analysis containing 23 studies from 2010-2017 revealed no significant difference in serum DKK-1 concentration between AS patients and healthy controls (14). Furthermore, more recent studies have reported controversial results since 2018 (15–30). The reason for the inconsistent results may be due to the potential bias of traditional epidemiology, such as measurement error, reverse causality, confounding bias, different races, and clinical heterogeneity, which are caused by the nature of the study design (31). Therefore, whether serum DKK-1 level is causally linked to AS remains undetermined.
In the present study, a two-stage design was applied. First, to derive a more precise estimation of the serum level of DKK-1 in AS patients, a meta-analysis was performed. Second, to determine the causal relationship between serum DKK-1 level and the risk of AS, a Mendelian randomization (MR) analysis was conducted.
2 Materials and methods
2.1 Data sources
This meta-analysis was performed according to the Preferred Reporting Items for Systematic Reviews and Meta-Analyses (PRISMA) guidelines published in 2021. We systematically conducted an electronic literature retrieval to search all available studies published up to July 2022 that were related to the correlation between DKK-1 serum levels and AS. The online searchable literature libraries included Embase, PubMed, Web of Science, WANFANG DATA, VIP, and the China National Knowledge Infrastructure (CNKI). Our search terms included ankylosing spondylitis, AS, DKK-1, Dickkopf-1, and Dickkopf-Related Protein 1, using subject terms and keywords. Inclusion criteria for the study were as follows: (1) the study was an observational study; (2) cases were patients with AS diagnosed by clear diagnostic criteria, and controls were healthy participants; (3) the study provided data on serum DKK-1 levels in AS patients and controls, such as mean and standard deviation (mean ± SD) or mean and standard error of the mean (mean ± SEM) or median and interquartile range (median (IQR)); (4) the article was written in English or Chinese. Exclusion criteria for the study were as follows: (1) the study was a conference abstract, review, meta-analysis, etc.; (2) the study had no sufficient data; (3) the study was based on animal models or only PBMCs; (4) the study used duplicate data. Moreover, references to the included literature and related reviews were searched to supplement any relevant studies.
Single-nucleotide polymorphisms (SNPs) associated with DKK-1 were screened from genome-wide association studies (GWAS) with 21,758 individuals as instrumental variables (IV), including 13, 102 756 variant loci (32). SNPs associated with the risk of AS were extracted from a European population study, which included 1,476 AS cases and 386,233 controls. The GWAS was derived from the exome sequencing data of the UK Biobank (UKB) Exome Sequencing Consortium, an organization that concentrates on each protein-coding gene in the genome by examining the exome sequences of 454,787 UKB participants (33). All data were downloaded from the publicly available GWAS Catalog database at https://www.ebi.ac.uk.
2.2 Data extraction and quality assessment
Two investigators extracted the study information, including the name of the first author, publication year, region of research, individual characteristics (age, gender), serum DKK-1 levels [mean ± SD, mean ± SEM, or median (IQR)], measurement method, duration of disease, and so on, from each of the included studies independently. For any study where there was disagreement regarding the inclusion or extraction of its data, our solution was to discuss it with the third reviewer until a consensus was reached. Two investigators evaluated the methodological quality of each study by the Newcastle-Ottawa scale (NOS) independently. The assessment scale defined articles with scores above 7 as high.
2.3 Instrument selection
Quality assessment of DKK-1 data was performed to incorporate optimal IV according to the following requirements. First of all, SNPs significantly correlated with DKK-1 were selected according to the threshold of the P value. A set of SNPs with genome-wide statistical significance (P < 5×10-8) were extracted as IVs. Second, SNPs with minor allele frequencies (MAF) less than 0.01 were excluded. Third, linkage disequilibrium (LD) among IVs was eliminated to reduce the bias in results caused by strong LD. We removed strong SNPs by the clumping procedure (R2 < 0.001, clumping length = 10 000 kb), and used 1 000 genomes of the European population as a reference. Fourth, corresponding SNPs that affect exposure and outcome should have the same effect alleles, and those with different effector genes should be corrected or deleted to guarantee that palindromic sequences are not included in IVs. Finally, after screening according to the above requirements, if there were no corresponding SNPs that affect exposure and outcome, proxy SNPs should be sought for further analysis (r2>0.8).
The included IVs should be subject to the following three assumptions: (1) IVs are closely related to exposure; (2) IVs are irrelevant to any confounding factors affecting the exposure-outcome association, F statistic was applied to evaluate the strength of correlation between the two, and those with the F statistic less than 10 were considered to be weakly correlated, which needed to be eliminated; (3) IVs do not affect outcomes except by association with exposure. Diabetes and hypertension, as common complications of ankylosing spondylitis, may affect DKK-1 production and function in the body (34–36). In order to exclude their influence as much as possible, we included them as confounding factors for further analysis.
2.4 Statistical analysis
R (version 4.1.1) was used for all statistical analyses. The correlation between serum DKK-1 and AS was assessed by calculating the standardized mean difference (SMD) with a 95% confidence interval (CI). Before calculating SMD, the data units were unified, SEM values were converted to SD using the function, SD=SEM× (37), and the median (IQR) references the methods of Zhao et al (38) and Luo et al (39) to convert into mean ± SD. The heterogeneity of the research was examined utilizing Cochran’s Q statistic and I2 test (40). The choice of effect model depended on the P value and I2. When P < 0.100 or I2>50%, the random effect model was chosen, and the fixed effect model was chosen for other cases. To further investigate the source of heterogeneity, subgroup analysis and meta-regression were implemented. Sensitivity analysis was carried out by eliminating each study to check the robustness of the results. The assessment of publication bias was adapted through Egger’s linear regression test and Begger’s rank correlation test, and funnel plots were used for its visualization. Outside the heterogeneity test (P < 0.100), other tests defined P <0.05 as significant.
The MR analysis methods used to estimate the causal effect between exposure and outcome in this study were the inverse variance weighting method (IVW), MR-Egger regression, weighted median method, and weighted method. A sensitivity analysis of the MR analysis results was also needed, which can be divided into three aspects. On one side, Cochran’s Q statistic was applied to test the heterogeneity among SNPs. Second, MR pleiotropy residual sum and outlier (MR-PRESSO) was utilized to test horizontal pleiotropy, eliminate outliers to correct the results, and compare the results before and after correction. Third, a leave-one-out analysis was conducted to examine the robustness and consistency of the result.
3 Results
3.1 Observed associations between serum DKK-1 and AS
3.1.1 Publication search and study characteristics
The detailed process of literature screening is shown in Figure 1. Of the 6,066 articles originally searched, 40 studies published from 2010 to 2022 were ultimately included in the meta-analysis. A total of 2,371 AS patients and 1,633 healthy controls were investigated. Of the 40 studies, 31 were case-control studies and 9 were cross-sectional studies. Furthermore, 6 were conducted in Euramerican and 34 in Asia. All of the studies used enzyme-linked immunosorbent assay (ELISA) to detect the serum levels of DKK-1. All the included articles were of medium to high quality, with NOS scores ranging from 5 to 8. The main information of the literature is shown in Table 1.
3.1.2 Overall effects
Meta-analysis of the overall articles showed significant heterogeneity (I2 = 97.5%, P<0.001), therefore the random-effect model was used to calculate the effect sizes. The serum DKK-1 levels of AS patients had no significant change compared with controls (pooled SMD=0.207, 95% CI = −0.418-0.832, P=0.516). The forest plot of SMDs (95% CI) of serum DKK-1 in AS patients relative to controls is shown in Figure 2.
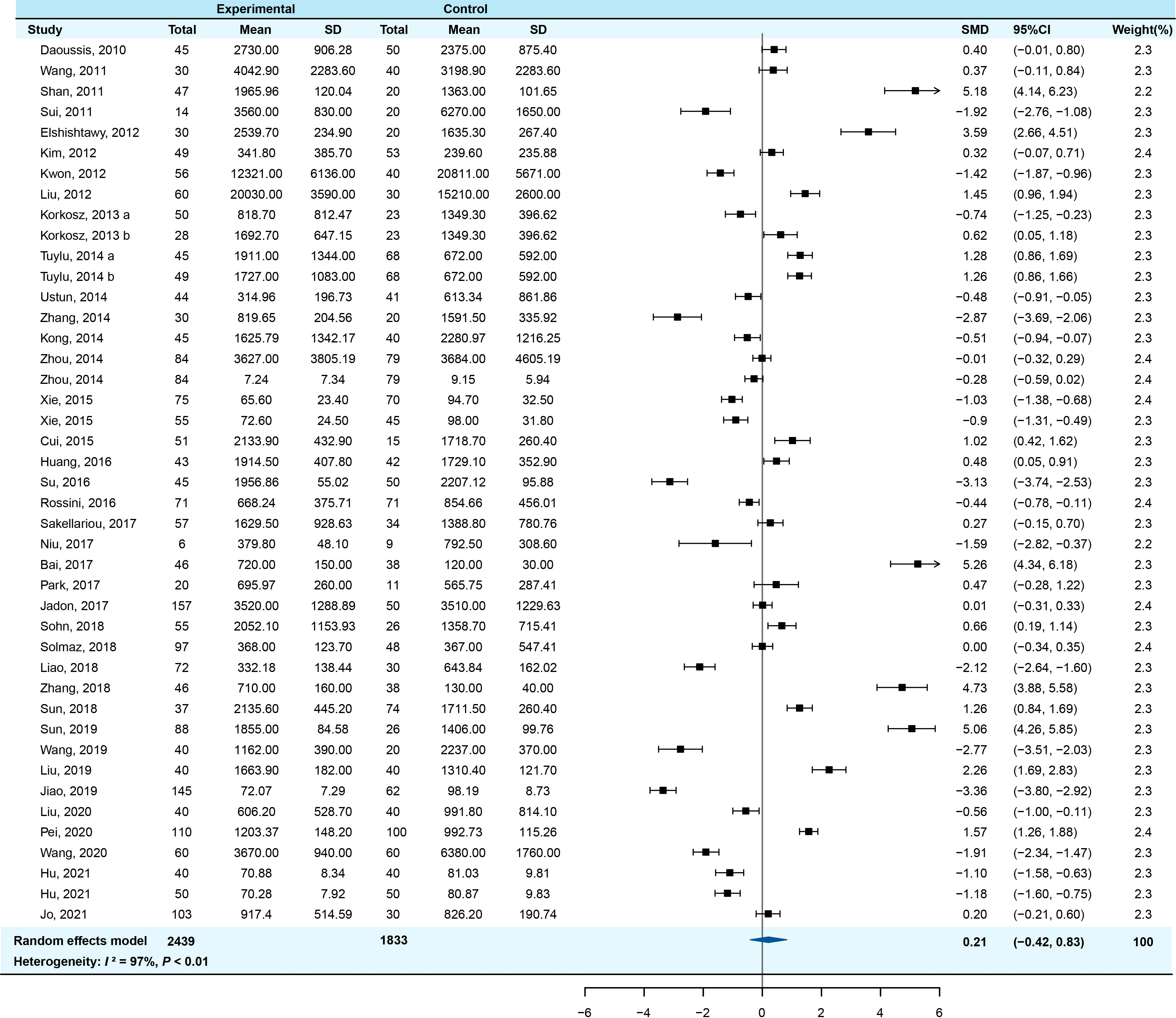
Figure 2 Forest plot of serum DKK-1 levels for AS patients vs healthy controls (a/b means the different subgroup from the same research).
3.1.3 Subgroup analysis and meta-regression analysis
Subgroup analysis was conducted by stratification according to study type, age, region, course of disease, C-reactive protein (CRP), erythrocyte sedimentation rate (ESR), the Bath AS Disease Activity Index (BASDAI), and the modified Stroke AS Spine Score (mSASSS). The results showed that among the CRP ≤10 mg/L subgroup, the serum DKK-1 concentration in AS patients was higher than that in healthy controls (SMD=2.267, 95% CI = 0.102-4.432, P=0.040) (Figure 3). The other subgroups all had no statistical significance (all P > 0.05) (Supplementary Figures 1–7).
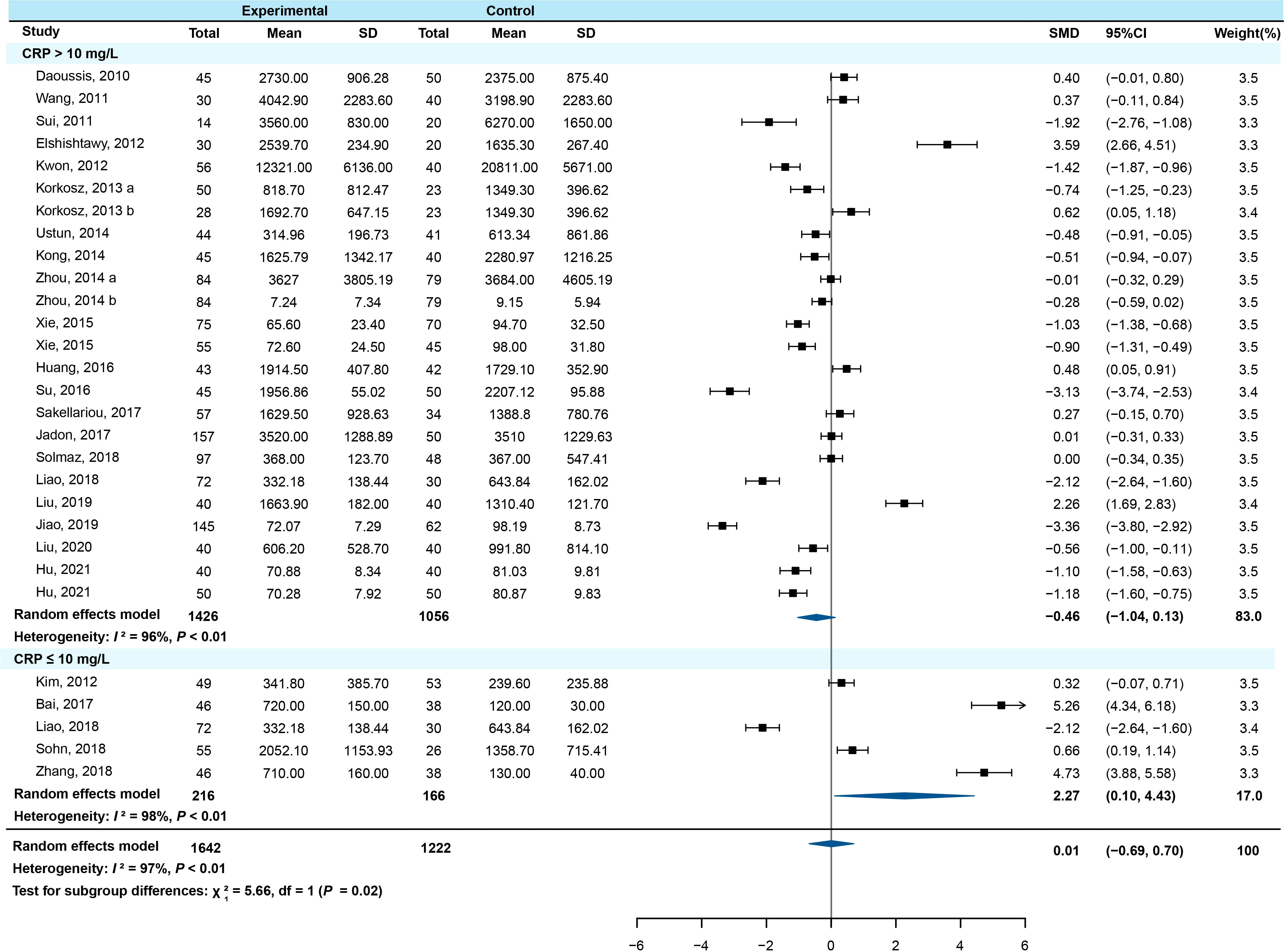
Figure 3 Forest plot with subgroup analysis of CRP (a/b means the different subgroup from the same research).
The results of meta-regression analysis on NOS, publication year, and research sample size showed that none of them were potential sources of heterogeneity (all P >0.05) (Table 2).
3.1.4 Publication bias and sensitivity analysis
Egger’s linear regression test (t = 1.14, P = 0.260) and Begger’s rank test (Z = 0.58, P = 0.565) did not detect any publication bias (Figure 4). A sensitivity analysis of all the included studies indicated that none of the 40 studies changed the overall effect size (all P >0.05), suggesting the results were robust.
3.2 Causal associations between serum DKK-1 and AS
3.2.1 IV selection
Initially, 579 were extracted as IVs from a large-scale GWAS. After eliminating SNPs that had LD effects, 9 SNPs were selected as IVs. The calculated F-statistic was 76.0, greater than 10, so there was no weak IV in these 9 SNPs.
3.2.2 Two-sample MR analysis
The result of IVW showed no evidence of an association between serum DKK-1 levels and the risk of AS (IVW OR= 0.999, 95% CI = 0.989-1.008, P = 0.800) (Figure 5). MR-Egger regression was used to assess the horizontal pleiotropy among IVs and outcome, and the results showed that there was no horizontal pleiotropy between them (P = 0.261). For the sensitivity analysis, no outliers were found in the analysis by MR-PRESSO (P = 0.692). The results of Cochran’s Q test indicated that there was no significant heterogeneity (Q= 5.527, P = 0.700) in the effect of DKK-1-related SNPs on the risk of AS, which revealed the reliability of MR results. Through the leave-one-out test of eliminating each SNP, the consistency of the results indicated the stability of the MR analysis results (Supplementary Table 2). There was no statistical significance between DKK-1 and confounding factors (All P > 0.05) (Supplementary Tables 3, 4).
4 Discussion
Ectopic formation of new bone and fusion of spinal joints in axial bones are vital factors in the disease burden of AS, and the β-catenin-dependent canonical Wnt signaling pathway has been investigated and proved to be an essential mechanism for osteogenesis and spinal sacralization (66). In a previous observational study exploring the link between Wnt signaling and AS, Diara et al. reported for the first time that the expression level of DKK-1 was lower in AS patients than in controls (10). As a recognized Wnt inhibitor, DKK-1 can be expressed through osteoblasts, antagonize the Wnt pathway, and inhibit osteoblast maturation and new bone formation. Moreover, blocking the Wnt pathway can promote the regulation of osteoclasts (67). DKK-1 can therefore impact osteoblasts and osteoclasts to participate in bone metabolism.
In subsequent studies on the serological level of DKK-1 in patients with AS, some studies (17, 20, 22, 24, 26–28, 44, 47, 49, 51–53, 55, 56, 59, 60, 62) also demonstrated lower serum DKK-1 levels in patients than in controls. In contrast, some studies (15, 16, 18, 21, 23, 25, 43, 45, 48–50, 57, 58, 63) have reported that the serum level of DKK-1 in patients was higher than that in controls. Furthermore, there were also some studies (19, 29, 41, 42, 46, 54, 61, 64, 65) showing that there was no significant diversity in DKK-1 serum levels among AS patients and healthy controls. Our current meta-analysis suggested that there was no significant association between serum DKK-1 and AS. This was similar to the overall effect result in a previous meta-analysis by Wu et al (14). In order to reduce the influence of confounding factors and make causal inference more reliable, we further conducted MR analysis, and the result likewise supported the finding of meta-analysis.
The reasons for the above results can be explained by the following points. First, DKK-1 primarily contributes to the development of new bone during AS (68). However, the expression of DKK-1 is not limited to bone, and it is also highly expressed in T cells, platelets, and a variety of cancer cells, with platelets serving as a significant source of circulating DKK-1 (11). We analyzed some complications as confounding factors, but the influence of other complications could not be excluded. Therefore, it is not accurate to ascribe DKK-1 fluctuation in the human body only to AS. Second, TNF-α and IL-1β can induce DKK-1 and sclerostin (SOST), and IL-6 stimulates B cells to differentiate into DKK-1-expressing plasma cells (6, 7). SOST is also an inhibitor of the Wnt pathway, binding low-density lipoprotein-related receptors 5 and 6 (LRP5/6) to affect Wnt signaling, and when DKK-1 is blocked, SOST may produce a compensatory response to restore Wnt signaling to a stable state (69). DKK-1 may interact with the molecules listed above to regulate Wnt signaling, which affects bone metabolism. Third, functional DKK-1 can validly play its role in blocking the Wnt pathway; however, when it is dysfunctional, it may not bind tightly to LPR5/6, resulting in the inability to inhibit Wnt signaling (34, 70). A previous study has shown that anti-TNF therapy can effectively inhibit the inflammatory response in the treatment of AS, but cannot prevent bone fusion, which may be related to DKK-1 disability (71). In addition, non-steroid anti-inflammatory drugs (NSAIDs) also affect the expression level of DKK-1 in patients, and different treatment regimens may lead to clinical heterogeneity (17).
The subgroup analysis results of the normal CRP group (CRP ≤ 10 mg/L) showed that AS patients had higher serum DKK-1 levels relative to the healthy controls. This result was partially identical to the results reported by Wu et al (14). The above phenomenon may also be related to the relative balance of proinflammatory cytokines, Wnt pathway inhibitors, and DKK-1 in the human body environment. The different results between the CRP subgroup and the ESR subgroup are probably due to the different rates at which the two indicators reflect inflammation in the body. CRP and ESR are indicators of the inflammatory response in the acute phase. CRP has higher sensitivity and can reflect the inflammatory state of the body more timely and accurately, and CRP is currently the most sensitive biomarker reflecting the disease activity of AS (72, 73). Therefore, it is not clear whether DKK-1 can reflect the inflammation of AS.
There were some limitations in our study. First, in the meta-analysis, there was significant heterogeneity in the results. Despite our subgroup and meta-regression analyses, the source of heterogeneity was still unknown. Second, the accuracy of reagents and instruments used to detect DKK-1 levels in various studies was not uniform, which was crucial to the research results. Third, the effect of participants’ medications on DKK-1 fluctuations in the body was uncertain, potentially reversing the findings. Fourth, in the MR analysis stage, the data were all from the European population, thus the extrapolation of the MR analysis results to other populations of different races should be treated with caution. Our study also had several strengths. First, the meta-analysis summarized all existing studies related to this study, increasing the sample size of the data and improving the accuracy of the results. Second, MR analysis used genetic polymorphisms of genes to select IVs from GWAS to explore the relationship between exposure and outcome (74). This can minimize confounding bias and avoid reverse causality because genetic variation occurs before disease, which makes the result more reliable (75). Third, some confounding factors that may affect the results of the MR analysis were analyzed to further reduce confounding bias. Finally, both meta-analysis and MR analysis showed that there was no statistical correlation between serum DKK-1 level and AS, so the results were more realistic and reliable. Based on these results, it can be known that no difference in the expression level of DKK-1 between AS patients and controls has been found at the serum level and gene level, and it is uncertain whether DKK-1 can be used as a clinical indicator of AS.
5 Conclusion
The results of the meta-analysis and MR analysis both revealed that there was no significant association between serum DKK-1 concentration and AS. The molecular mechanism of DKK-1 and other cytokines in the occurrence of AS still warrants further study.
Data availability statement
The original contributions presented in the study are included in the article/Supplementary Material. Further inquiries can be directed to the corresponding authors.
Author contributions
S-ST and H-FP conceived the idea and proofread the manuscript. XF drafted and revised the manuscript. CC participated in formal analysis and validation. Z-XW and YZ participated in literature search and information collection. L-QJ and YF participated in visualization. R-DZ participated in language editing. All authors contributed to the article and approved the submitted version.
Funding
This study was supported by the University Natural Science Research Project of Anhui Province (KJ2021A0230).
Conflict of interest
The authors declare that the research was conducted in the absence of any commercial or financial relationships that could be construed as a potential conflict of interest.
Publisher’s note
All claims expressed in this article are solely those of the authors and do not necessarily represent those of their affiliated organizations, or those of the publisher, the editors and the reviewers. Any product that may be evaluated in this article, or claim that may be made by its manufacturer, is not guaranteed or endorsed by the publisher.
Supplementary material
The Supplementary Material for this article can be found online at: https://www.frontiersin.org/articles/10.3389/fimmu.2023.1193357/full#supplementary-material
Glossary
References
1. Zhu W, He X, Cheng K, Zhang L, Chen D, Wang X, et al. Ankylosing spondylitis: etiology, pathogenesis, and treatments. Bone Res (2019) 7:22. doi: 10.1038/s41413-019-0057-8
2. Zhang X, Sun Z, Zhou A, Tao L, Chen Y, Shi X, et al. Association between infections and risk of ankylosing spondylitis: a systematic review and meta-analysis. Front Immunol (2021) 12:768741. doi: 10.3389/fimmu.2021.768741
3. Packham J. Optimizing outcomes for ankylosing spondylitis and axial spondyloarthritis patients: a holistic approach to care. Rheumatol (Oxford) (2018) 57(suppl_6):vi29–34. doi: 10.1093/rheumatology/key200
4. Li Z, Wu X, Leo PJ, De Guzman E, Akkoc N, Breban M, et al. Polygenic risk scores have high diagnostic capacity in ankylosing spondylitis. Ann Rheum Dis (2021) 80(9):1168–74. doi: 10.1136/annrheumdis-2020-219446
5. Braga M, Lara-Armi FF, Neves JSF, Rocha-Loures MA, Terron-Monich MS, Bahls-Pinto LD, et al. Influence of IL10 (rs1800896) polymorphism and TNF-α, IL-10, IL-17A, and IL-17F serum levels in ankylosing spondylitis. Front Immunol (2021) 12:653611. doi: 10.3389/fimmu.2021.653611
6. Yeremenko N, Zwerina K, Rigter G, Pots D, Fonseca JE, Zwerina J, et al. Tumor necrosis factor and interleukin-6 differentially regulate dkk-1 in the inflamed arthritic joint. Arthritis Rheumatol (2015) 67(8):2071–5. doi: 10.1002/art.39183
7. Cici D, Corrado A, Rotondo C, Cantatore FP. Wnt signaling and biological therapy in rheumatoid arthritis and spondyloarthritis. Int J Mol Sci (2019) 20(22):5552. doi: 10.3390/ijms20225552
8. Baetta R, Banfi C. Dkk (Dickkopf) proteins. Arterioscler Thromb Vasc Biol (2019) 39(7):1330–42. doi: 10.1161/atvbaha.119.312612
9. Xie W, Zhou L, Li S, Hui T, Chen D. Wnt/β-catenin signaling plays a key role in the development of spondyloarthritis. Ann N Y Acad Sci (2016) 1364(1):25–31. doi: 10.1111/nyas.12968
10. Diarra D, Stolina M, Polzer K, Zwerina J, Ominsky MS, Dwyer D, et al. Dickkopf-1 is a master regulator of joint remodeling. Nat Med (2007) 13(2):156–63. doi: 10.1038/nm1538
11. Jaschke N, Hofbauer LC, Göbel A, Rachner TD. Evolving functions of dickkopf-1 in cancer and immunity. Cancer Lett (2020) 482:1–7. doi: 10.1016/j.canlet.2020.03.031
12. Soós B, Szentpétery Á, Raterman HG, Lems WF, Bhattoa HP, Szekanecz Z. Effects of targeted therapies on bone in rheumatic and musculoskeletal diseases. Nat Rev Rheumatol (2022) 18(5):249–57. doi: 10.1038/s41584-022-00764-w
13. Zhang L, Ouyang H, Xie Z, Liang ZH, Wu XW. Serum DKK-1 level in the development of ankylosing spondylitis and rheumatic arthritis: a meta-analysis. Exp Mol Med (2016) 48(4):e228. doi: 10.1038/emm.2016.12
14. Wu M, Chen M, Ma Y, Yang J, Han R, Yuan Y, et al. Dickkopf-1 in ankylosing spondylitis: review and meta-analysis. Clin Chim Acta (2018) 481:177–83. doi: 10.1016/j.cca.2018.03.010
15. Sun J, Song DM, Zhu XX, Wang FM. Study on the expression and relationship of dickkopf-1 in serum of patients with central axial spinal arthritis. J Clin Med (2018) 5(60):2–3. doi: 10.16281/j.cnki.jocml.2018.60.002
16. Zhang Z, Sun QH, Bai J, Zhang YL, Xu SX, Xu MH. Clinical significance of detection of MIF, IL-23, RANKL, OPG and DKK1 in peripheral blood of patients with active ankylosing spondylitis. Hebei Med J (2018) 40(2):178–82. doi: 10.3969/j.issn.1002-7386.2018.02.004
17. Liao HT, Lin YF, Tsai CY, Chou TC. Bone morphogenetic proteins and dickkopf-1 in ankylosing spondylitis. Scand J Rheumatol (2018) 47(1):56–61. doi: 10.1080/03009742.2017.1287305
18. Sohn DH, Jeong H, Roh JS, Lee H-N, Kim E, Koh JH, et al. Serum CCL11 level is associated with radiographic spinal damage in patients with ankylosing spondylitis. Rheumatol Int (2018) 38(8):1455–64. doi: 10.1007/s00296-018-4073-6
19. Solmaz D, Uslu S, Kozaci D, Karaca N, Bulbul H, Tarhan EF, et al. Evaluation of periostin and factors associated with new bone formation in ankylosing spondylitis: periostin may be associated with the wnt pathway. Int J Rheum Dis (2018) 21(2):502–9. doi: 10.1111/1756-185X.13186
20. Wang TL, Li H, Cui JJ. The relationship between the levels of dickkopf-1 and BMP-2 in peripheral blood and the formation of osteopontine in ankylosing spondylitis. Doctor (2019) 4(6):51–2,59.
21. Liu DD. Changes in serum DKK-1, VEGF-a and IL-17 levels in patients with ankylosing spondylitis and their clinical importance. Chin J Osteoporos (2019) 25(7):980–2,1014. doi: 10.3969/j.issn.1006-7108.2019.07.019
22. Jiao AJ, He CJ, Xu AM. Relationship between serum levels of MMP-9 and DKK-1 and disease activity in patients with ankylosing spondylitis. Int J Lab Med (2019) 40(8):935–8. doi: 10.3969/j.issn.1673-4130.2019.08.011
23. Sun W, Tian L, Jiang L, Zhang S, Zhou M, Zhu J, et al. Sclerostin rather than dickkopf-1 is associated with mSASSS but not with disease activity score in patients with ankylosing spondylitis. Clin Rheumatol (2019) 38(4):989–95. doi: 10.1007/s10067-018-4356-z
24. Liu XL, Zhang HM, Tang M, Zhang J, Zou M, Hang YX. The expression of DKK-1, MMP-3,TNF−α and IL-6 in peripheral blood of ankylosing spondylitis patients with different disease stages and the changes before and after trentment. Modern Immunol (2020) 40(4):300–5. doi: 10.3969/j.issn.1001-2478.2020.04.06
25. Pei F, Jin YM. Correlation between dickkopf -1 level and disease activity, severity in patients with ankylosing spondylitis. Chin J Health Lab Tec (2020) 30(17):2116–9. doi: 10.3969/j.issn.1004-8685.2020.17.04
26. Wang C, Qiu WQ. Correlation analysis of 14-3-3 η DKK-1 and TGF-β1 levels with BASDAI BASFI in patients with ankylosing spondylitis. Anhui Med J (2020) 41(9):1066–9. doi: 10.3969/j.issn.1000-0399.2020.09.021
27. Hu JB, Hao Z, Yang YY, Wang D, Zeng K. Effect of recombinant human tumor necrosis factor-fc on serum dkk-1 and bone metabolic parameters in patients with ankylosing spondylitis. Chin J Hosp Pharm (2021) 41(8):820–3. doi: 10.13286/j.1001-5213.2021.08.10
28. Hu JB, Hao Z, Yang YY, Wang D, Zeng K. Application of serum dickkopf-1 protein detection in active ankylosing spondylitis. J Clin Intern Med (2021) 38(4):259–61. doi: 10.3969/j.issn.1001-9057.2021.04.014
29. Jo S, Nam B, Lee YL, Park H, Weon S, Choi SH, et al. The TNF-NF-κB-DKK1 axis promoted bone formation in the enthesis of ankylosing spondylitis. J Rheum Dis (2021) 28(4):216–24. doi: 10.4078/jrd.2021.28.4.216
30. Liu J, Zhao L, Yang X, Liu C, Kong N, Yu Y, et al. Bone mineral density, bone metabolism-related factors, and microRNA-218 are correlated with disease activities in Chinese ankylosing spondylitis patients. J Clin Lab Anal (2022) 36(2):e24223. doi: 10.1002/jcla.24223
31. Sun L, Zhu J, Ling Y, Mi S, Li Y, Wang T, et al. Physical activity and the risk of rheumatoid arthritis: evidence from meta-analysis and mendelian randomization. Int J Epidemiol (2021) 50(5):1593–603. doi: 10.1093/ije/dyab052
32. Folkersen L, Gustafsson S, Wang Q, Hansen DH, Hedman AK, Schork A, et al. Genomic and drug target evaluation of 90 cardiovascular proteins in 30,931 individuals. Nat Metab (2020) 2(10):1135–48. doi: 10.1038/s42255-020-00287-2
33. Backman JD, Li AH, Marcketta A, Sun D, Mbatchou J, Kessler MD, et al. Exome sequencing and analysis of 454,787 UK biobank participants. Nature (2021) 599(7886):628–34. doi: 10.1038/s41586-021-04103-z
34. Huang Y, Liu L, Liu A. Dickkopf-1: current knowledge and related diseases. Life Sci (2018) 209:249–54. doi: 10.1016/j.lfs.2018.08.019
35. Lee YT, Ng HY, Chiu TT, Li LC, Pei SN, Kuo WH, et al. Association of bone-derived biomarkers with vascular calcification in chronic hemodialysis patients. Clin Chim Acta (2016) 452:38–43. doi: 10.1016/j.cca.2015.10.031
36. Singh DK, Magrey MN. Racial differences in clinical features and comorbidities in ankylosing spondylitis in the united states. J Rheumatol (2020) 47(6):835–8. doi: 10.3899/jrheum.181019
37. Zhao R, Su Z, Wu J, Ji HL. Serious adverse events of cell therapy for respiratory diseases: a systematic review and meta-analysis. Oncotarget (2017) 8(18):30511–23. doi: 10.18632/oncotarget.15426
38. Zhao R, Su Z, Komissarov AA, Liu SL, Yi G, Idell S, et al. Associations of d-dimer on admission and clinical features of COVID-19 patients: a systematic review, meta-analysis, and meta-regression. Front Immunol (2021) 12:691249. doi: 10.3389/fimmu.2021.691249
39. Luo D, Wan X, Liu J, Tong T. Optimally estimating the sample mean from the sample size, median, mid-range, and/or mid-quartile range. Stat Methods Med Res (2018) 27(6):1785–805. doi: 10.1177/0962280216669183
40. Higgins JP, Thompson SG, Deeks JJ, Altman DG. Measuring inconsistency in meta-analyses. BMJ (2003) 327(7414):557–60. doi: 10.1136/bmj.327.7414.557
41. Daoussis D, Liossis SC, Solomou EE, Tsanaktsi A, Bounia K, Karampetsou M, et al. Evidence that dkk-1 is dysfunctional in ankylosing spondylitis. Arthritis Rheumatol (2010) 62(1):150–8. doi: 10.1002/art.27231
42. Wang SY, Liu YY, Ye H, Guo JP, Li R, Liu X, et al. Circulating dickkopf-1 is correlated with bone erosion and inflammation in rheumatoid arthritis. J Rheumato (2011) 38(5):821–7. doi: 10.3899/jrheum.100089
43. Shan ZX, Han JX, Cui YZ, Zhou XY. The expression of serum DKK - 1 and its significance in patients witll ankylosing spondyHtis. Med Lab Sci Clin (2011) 22(1):1–2,7. doi: 10.3969/j.issn.1673-5013.2011.01.001
44. Sui L, Zhang K, Wang Y. Clinical significance of serum level of dkk-1 in patients with ankylosing spondylitis. Tianjin Med J (2011) 39(10):918–20. doi: 10.3969/j.issn.0253-9896.2011.10.013
45. Elshishtawy H, Assaf N, Farouk N. Dickkopf-1 in ankylosing spondylitis: relation to spinal dysmobility and radiographic findings. Egyptian Rheumatologist (2012) 34(3):111–7. doi: 10.1016/j.ejr.2012.05.002
46. Kim TJ, Lee SJ, Cho YN, Park SC, Jin HM, Kim MJ, et al. Immune cells and bone formation in ankylosing spondylitis. Clin Exp Rheumatol (2012) 30(4):469–75.
47. Kwon SR, Lim MJ, Suh CH, Park SG, Hong YS, Yoon BY, et al. Dickkopf-1 level is lower in patients with ankylosing spondylitis than in healthy people and is not influenced by anti-tumor necrosis factor therapy. Rheumatol Int (2012) 32(8):2523–7. doi: 10.1007/s00296-011-1981-0
48. Liu H, Dong Q. Study on the content of lymphocytes,the expression of DKK1 and BMP-2 in patients of ankylosing spondylitis with HLA-B27 positive. Chongqing Med (2012) 41(36):3857–8,3861. doi: 10.3969/j.issn.1671-8348.2012.36.02
49. Korkosz M, Gąsowski J, Leszczyński P, Pawlak-Buś K, Jeka S, Kucharska E, et al. High disease activity in ankylosing spondylitis is associated with increased serum sclerostin level and decreased wingless protein-3a signaling but is not linked with greater structural damage. BMC Musculoskelet Disord (2013) 14:99. doi: 10.1186/1471-2474-14-99
50. Tuylu T, Sari I, Solmaz D, Kozaci DL, Akar S, Gunay N, et al. Fetuin-a is related to syndesmophytes in patients with ankylosing spondylitis: a case control study. Clinics (Sao Paulo) (2014) 69(10):688–93. doi: 10.6061/clinics/2014(10)07
51. Ustun N, Tok F, Kalyoncu U, Motor S, Yuksel R, Yagiz AE, et al. Sclerostin and dkk-1 in patients with ankylosing spondylitis. Acta Reumatol Port (2014) 39(2):146–51.
52. Zhang YT, Chen ZW, Deng YS, Zeng LQ. Dickkopf-1 and tartrate-resistant acid phosphatase 5b in ankylosing spondylitis. Chin J Hemo (2014) 0(3):383–6. doi: 10.3969/j.issn.1009-881X.2014.03.011
53. Kong WP, Zhou TL, Wang XT, Xu Y, Tao QW, Yan XP. Effects of bushen qiangdu formula on the level of dickkopf1 in 45 cases of ankylosing spondylitis. J Tradit Chin Med (2014) 55(20):1750–3. doi: 10.13288/j.11-2166/r.2014.20.011
54. Yucong Z, Lu L, Shengfa L, Liang YY, Ruguo S, Yikai L. Serum functional dickkopf-1 levels are inversely correlated with radiographic severity of ankylosing spondylitis. Clin Lab (2014) 60(9):1527–31. doi: 10.7754/Clin.Lab.2014.131119
55. Xie JM, Lu X. The study of association between serunl DKKl and coronsry artery calcification score in patients with ankylosing spondylitis. J Clin Intem Med (2015) 0(7):451–4. doi: 10.3969/j.issn.1001-9057.2015.07.005
56. Xie JM, Zhao C. Analyzing the correlation between serum dkk-1 levels and bone imaging changes in patients with ankylosing spondylitis. Rheum Arthritis (2015) 4(5):13–7. doi: 10.3969/j.issn.2095-4174.2015.05.003
57. Cui YF, Zhang LY, Zhang GL, Xu K, Ma D, Guo QD, et al. Serum levels of dickkopf-1 in axial spondyloarthropathy and correlation analysis. Contemp Med (2015) 5:8–11. doi: 10.3969/j.issn.1009-4393.2015.5.003
58. Huang J, Song G, Yin Z, Fu Z, Ye Z. Alteration of bone turnover markers in canonical wingless pathway in patients with ankylosing spondylitis. Arch Rheumato (2016) 31(3):221–8. doi: 10.5606/ArchRheumatol.2016.5857
59. Su XJ, Wang HD. Effect of wulao qisun formula on the level of dickkopf-a of ankylosing spondylitis. Chin J Trand Med Traum Orthop (2016) 24(3):26–8. doi: 10.3969/j.issn.1002-0202.2016.03.0026
60. Rossini M, Viapiana O, Idolazzi L, Ghellere F, Fracassi E, Troplini S, et al. Higher level of dickkopf-1 is associated with low bone mineral density and higher prevalence of vertebral fractures in patients with ankylosingspondylitis. Calcif Tissue Int (2016) 98(5):438–45. doi: 10.1007/s00223-015-0093-3
61. Sakellariou GT, Iliopoulos A, Konsta M, Kenanidis E, Potoupnis M, Tsiridis E, et al. Serum levels of dkk-1, sclerostin and VEGF in patients with ankylosing spondylitis and their association with smoking, and clinical, inflammatory and radiographic parameters. Joint Bone Spine (2017) 84(3):309–15. doi: 10.1016/j.jbspin.2016.05.008
62. Niu CC, Lin SS, Yuan LJ, Chen LH, Yang CY, Chung AN, et al. Correlation of blood bone turnover biomarkers and wnt signaling antagonists with AS, DISH, OPLL, and OYL. BMC Musculoskelet Disord (2017) 18(1):61. doi: 10.1186/s12891-017-1425-4
63. Ba J, Sun QH, Zhang Z, Zhang YL, Xu SX. Clinical significance of the levels of MIF, IL-23, RANKL, OPG, and DKK1 in patients with active ankylosing spondylitis. Hebei Med J (2017) 39(20):3071–4. doi: 10.3969/j.issn.1002-7386.2017.20.007
64. Park JH, Lee SG, Jeon YK, Park EK, Suh YS, Kim HO. Relationship between serum adipokine levels and radiographic progression in patients with ankylosing spondylitis a preliminary 2-year longitudinal study. Med (2017) 96(33):e7854. doi: 10.1097/MD.0000000000007854
65. Jadon DR, Sengupta R, Nightingale A, Lu H, Dunphy J, Green A, et al. Serum bone-turnover biomarkers are associated with the occurrence of peripheral and axial arthritis in psoriatic disease: a prospective cross-sectional comparative study. Arthritis Res Ther (2017) 19:210. doi: 10.1186/s13075-017-1417-7
66. Li X, Wang J, Zhan Z, Li S, Zheng Z, Wang T, et al. Inflammation intensity-dependent expression of osteoinductive wnt proteins is critical for ectopic new bone formation in ankylosing spondylitis. Arthritis Rheumatol (2018) 70(7):1056–70. doi: 10.1002/art.40468
67. Terpos E, Christoulas D, Gavriatopoulou M. Biology and treatment of myeloma related bone disease. Metabolism (2018) 80:80–90. doi: 10.1016/j.metabol.2017.11.012
68. Daoussis D, Kanellou A, Panagiotopoulos E, Papachristou D. DKK-1 is underexpressed in mesenchymal stem cells from patients with ankylosing spondylitis and further downregulated by IL-17. Int J Mol Sci (2022) 23(12):6660. doi: 10.3390/ijms23126660
69. Florio M, Gunasekaran K, Stolina M, Li X, Liu L, Tipton B, et al. A bispecific antibody targeting sclerostin and DKK-1 promotes bone mass accrual and fracture repair. Nat Commun (2016) 7:11505. doi: 10.1038/ncomms11505
70. Czepiel M, Stec M, Korkosz M, Guła Z, Błyszczuk P, Baran J, et al. Down-gegulation of dkk-1 in platelets of patients with axial spondyloarthritis. Arthritis Rheumatol (2021) 73(10):1831–4. doi: 10.1002/art.41739
71. Yu T, Zhang J, Zhu W, Wang X, Bai Y, Feng B, et al. Chondrogenesis mediates progression of ankylosing spondylitis through heterotopic ossification. Bone Res (2021) 9(1):19. doi: 10.1038/s41413-021-00140-6
72. Lapić I, Padoan A, Bozzato D, Plebani M. Erythrocyte sedimentation rate and c-reactive protein in acute inflammation. Am J Clin Pathol (2020) 153(1):14–29. doi: 10.1093/ajcp/aqz142
73. Maksymowych WP. Biomarkers for diagnosis of axial spondyloarthritis, disease activity, prognosis, and prediction of response to therapy. Front Immunol (2019) 10:305. doi: 10.3389/fimmu.2019.00305
74. Bowden J, Holmes MV. Meta-analysis and mendelian randomization: a review. Res Synth Methods (2019) 10(4):486–96. doi: 10.1002/jrsm.1346
Keywords: Dickkopf-1, ankylosing spondylitis, serum level, meta-analysis, Mendelian randomization analysis
Citation: Fang X, Chen C, Wang Z-X, Zhao Y, Jiang L-Q, Fang Y, Zhang R-D, Pan H-F and Tao S-S (2023) Serum DKK-1 level in ankylosing spondylitis: insights from meta-analysis and Mendelian randomization. Front. Immunol. 14:1193357. doi: 10.3389/fimmu.2023.1193357
Received: 24 March 2023; Accepted: 26 June 2023;
Published: 12 July 2023.
Edited by:
James Cheng-Chung Wei, Chung Shan Medical University Hospital, TaiwanReviewed by:
Po Cheng Shih, Changhua Christian Hospital, TaiwanShuo-Yan Gau, Chung Shan Medical University, Taiwan
Copyright © 2023 Fang, Chen, Wang, Zhao, Jiang, Fang, Zhang, Pan and Tao. This is an open-access article distributed under the terms of the Creative Commons Attribution License (CC BY). The use, distribution or reproduction in other forums is permitted, provided the original author(s) and the copyright owner(s) are credited and that the original publication in this journal is cited, in accordance with accepted academic practice. No use, distribution or reproduction is permitted which does not comply with these terms.
*Correspondence: Hai-Feng Pan, cGFuaGFpZmVuZzE5ODJAc2luYS5jb20=; cGFuaGFpZmVuZ0BhaG11LmVkdS5jbg==; Sha-Sha Tao, dGFvc2hhc2hhMTIxMkAxMjYuY29t
†These authors have contributed equally to this work and share first authorship