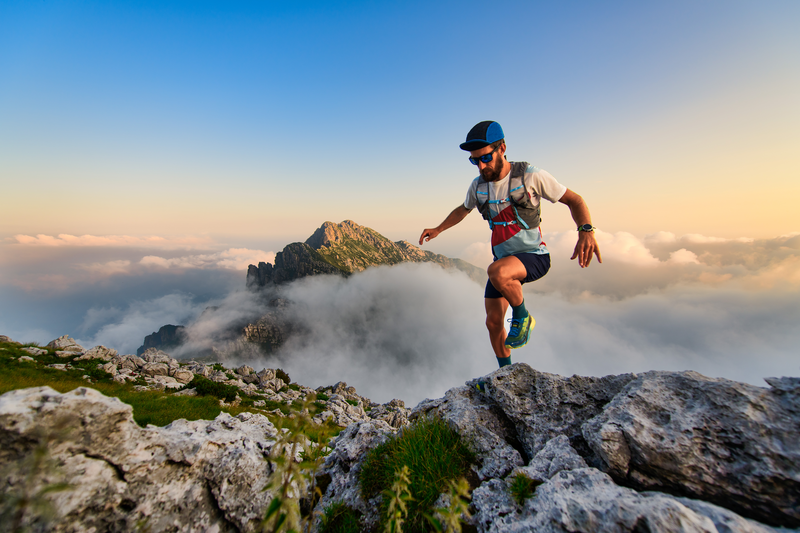
95% of researchers rate our articles as excellent or good
Learn more about the work of our research integrity team to safeguard the quality of each article we publish.
Find out more
ORIGINAL RESEARCH article
Front. Immunol. , 19 June 2023
Sec. Autoimmune and Autoinflammatory Disorders: Autoinflammatory Disorders
Volume 14 - 2023 | https://doi.org/10.3389/fimmu.2023.1184162
Objectives: Kawasaki disease (KD) is a commonly acquired pediatric systemic vasculitis disease resulting in coronary artery aneurysm (CAA). The relationship between the ITPKC polymorphism (rs7251246) and the severity and susceptibility to KD in the Han Chinese population in Southern China remains unclear.
Methods: We enrolled 262 children as controls and 221 children with KD (46 [20.8%] with intravenous immunoglobulin resistance and 82 [37.1%] with CAA). The relationship between the ITPKC rs7251246 polymorphism, KD susceptibility, and CAA formation was investigated.
Results: While the ITPKC rs7251246 T>C polymorphism was not significantly associated with KD susceptibility, it was significantly related to the CAA risk in children with KD [CC/CT vs. TT: adjusted odds ratio [OR] 2.089, 95% confidence interval [CI] 1.085–4.020]. Male children with the rs7251246 CT/TT genotype had a significantly lower risk of thrombosis [CT/TT vs. CC: adjusted OR 0.251, 95% CI 0.068–0.923]. Children with KD, especially those with CAA, had significantly downregulated ITPKC mRNA compared to healthy children. ITPKC mRNA levels were lower in children with CAA who developed thrombosis (P=0.039). In children with KD, the CC genotype showed lower mRNA levels of ITPKC (P=0.035).
Conclusion: The ITPKC rs7251246 T>C polymorphism may be a risk factor for CAA and thrombosis in children with KD in the Han Chinese population, likely due to differences in mature mRNA levels caused by interference of RNA splicing. Dual antiplatelet therapy for thrombosis is recommended for male children with the rs7251246 CC genotype.
Kawasaki disease (KD) is an acute self-limiting systemic vasculitis initially reported by Tomisaku Kawasaki in 1967 (1). KD has gradually become the most common cause of acquired heart disease in childhood (2–4). Although the etiology of KD remains unknown, it has been reported to be associated with the combined effects of infection, immune dysregulation, and genetic susceptibility. Epidemiological studies have reported that the incidence rates of KD vary among different regions, being much higher in Asian countries than in Western countries and increasing in Asian countries (especially in China) (5–7). Furthermore, male predominance and familial clustering suggest that hereditary factors may play an important role in KD occurrence and development (3, 8, 9). A genetic variation in the intracellular signaling pathway involved in immune effector function may increase the risk of KD and coronary artery disease (10, 11). Several networks may play a role in KD pathogenesis, and several susceptibility genes have been identified through genome-wide association and linkage studies. They can be classified into four groups according to their function: enhanced T cell activation, dysregulated B cell signaling, altered transforming growth factor beta signaling, and decreased apoptosis (12). In addition, a growing number of studies have demonstrated that some single-nucleotide polymorphism (SNP) gene loci have a close relationship with susceptibility to KD, the severity of KD, response to intravenous immunoglobulin (IVIG) treatment, and development of coronary artery complications (13–16).
The genetic association between KD and inositol 1,4,5-trisphosphate 3-kinase C (ITPKC) in a genome-wide scan was first reported by Onouchi et al. in 2008 (17). ITPKC, one of three isoenzymes of the ITPK family, located on chromosome 19q13.2, is responsible for the phosphorylation of inositol phosphate 3 (IP3) to 1,3,4,5-tetrakisphosphate (17); moreover, it is also involved in the Ca2+/nuclear factor of activated T cells (Ca2+/NFAT) pathway that negatively regulates T cell activation (17). A mutation in ITPKC can cause defective regulation of T cell activation (18, 19). Studies have shown that ITPKC is a crucial contributor to KD susceptibility and is associated with coronary artery aneurysm (CAA) formation (17, 20–23). Hence, ITPKC has been proposed as a susceptibility gene for KD, and its polymorphisms have become a research focus, as they may govern mRNA and protein ITPKC levels. This is consistent with ITPKC mutation and KD risk being negatively correlated (17). A functional SNP (rs28493229) located in intron 1 of ITPKC interferes with RNA splicing to affect transcriptional levels of mature mRNA, which is associated with KD susceptibility and the development of coronary artery lesions (CALs) in Japanese and American populations (17, 24). However, contrasting results were obtained in initial replication studies in the Taiwanese population (25, 26) and the Han population in mainland China (27). Furthermore, another SNP (rs2290692) was reported to be associated with susceptibility to KD in a Han Chinese population by Peng et al. in 2012 (21), which was also shown in a Taiwanese population with KD and CALs formation two years later (28). This study also suggested that a novel SNP (rs7251246) located in intron 1 of ITPKC may be used as a potential marker of KD severity in Taiwanese patients (28). Nevertheless, the mechanisms involved in the effect of rs7251246 on ITPKC expression remain unclear. Additionally, current research on the impact of the rs7251246 polymorphism on KD in the Han Chinese population in Southern China is lacking. Therefore, in the present study, we aimed to explore the association between the ITPKC (rs7251246) gene polymorphism and the susceptibility to KD in a Han Chinese population. We also explored the relationship between IVIG treatment response, CAA formation, immune/inflammation-related indicators, and genetic ITPKC polymorphisms. Furthermore, the ITPKC mRNA expression levels in the peripheral blood and potential mechanisms of action of the rs7251246 polymorphism were also investigated.
Two hundred twenty-one patients diagnosed with complete KD and 262 healthy controls were recruited from January 2021 to December 2022. KD was diagnosed according to the diagnostic criteria of the American Heart Association (AHA) (3, 29). Patients with KD attended our hospital as both outpatients with follow-ups and inpatients. The patients were of Han Chinese descent and unrelated. The healthy controls included age- and sex-matched children who visited our hospital within the same period for health examinations, presented without fever, and had no previous history of KD, infections, cardiovascular disease, autoimmune disease, or allergies. All patients with KD were treated and followed up according to the AHA guidelines. Symptoms of persistent or recurrent fever (axillary temperature ≥ 37.5 °C or rectal temperature ≥ 38.0 °C) following the initial IVIG infusion (2 g/kg) for more than 36 hours but not longer than seven days were considered IVIG resistance (3). Pediatric echocardiography was performed by experienced pediatric echocardiographers and was confirmed by two additional pediatric cardiologists; CAA was defined according to the guidelines for diagnosing and managing cardiovascular sequelae in KD (JCS/JSCS 2020) (30), and patients were followed up for at least 1 year, during which they underwent regular echocardiographic assessments. These guidelines use z-scores adjusted for body surface area, defining CAA as a coronary artery internal diameter ≥ 2.5 in either the proximal right or left main coronary artery or left anterior descending artery, persisting for more than a month after disease onset. CAA status and specific CAA were recorded based on the maximal z-scores and diameters of the coronary arteries in echocardiography reports. The z-scores were calculated using the Dallaire equations (31), and CAA was classified based on the following z-score intervals: small aneurysm, from 2.5 to 5 (non-inclusive); moderate aneurysm, from 5 to 10 (non-inclusive); and large or giant aneurysm, ≥ 10 or an internal diameter of ≥ 8 mm (30). Moreover, the time to reach the peak of CAA diameters was considered as the time at which maximum internal diameter of coronary artery was observed after illness onset, and the time of CAA persistence was defined as the time needed for CAA to return to normal appearance and size (z-score of < 2.5) in all coronary arteries as shown by echocardiography images, in addition to regaining normal cardiac function (3). Thrombotic events were diagnosed using echocardiography and confirmed using computed tomography coronary angiography. To further investigate the relationship between the severity of KD and ITPKC gene polymorphisms, the KD group was first divided into KD with IVIG resistance (n = 46) and KD with IVIG responsiveness (n = 175) subgroups. The KD group was also divided into KD with CAA (KD-CAA) (n = 82) and KD without CAA (KD-NCAA) (n = 139), and subgroup analyses were subsequently performed in patients with CAA according to the age, sex, CAA size, and prognosis (Figure 1). A total of 2 mL of peripheral venous blood sample was collected from each participant, anticoagulant EDTA-Na2 was added, and the samples were stored at −80°C.
Figure 1 Flowchart of the study. IVIG, intravenous immunoglobulin; CAA, coronary artery aneurysm; NCAA, no coronary artery aneurysm.
This study was conducted in accordance with the principles of the Declaration of Helsinki and approved by the Ethics Review Committee of the First Affiliated Hospital of Guangxi Medical University (code number: 2021(KY-E-240)). Both patients with KD and those in the control group received detailed information regarding the purpose of the study. Written informed consent was obtained from the children’s parents or legal guardians.
Genomic DNA was extracted from 400 µL of blood collected from each participant using a TIANamp Blood DNA Kit (Tiangen, Beijing, China), and 1% agarose gel electrophoresis was used to determine the quality of the DNA, which was then stained with ethidium bromide. The purity of DNA was determined by taking the optical density of samples at 260 and 280 nm using a nucleic acid quantifier (Thermo NanoDrop 2000, Shimadzu). Samples were stored at −80°C until further analysis. SNaPshot (Applied Biosystems, Foster City, CA, USA) was used for genotyping the ITPKC rs7251246 polymorphism. The ITPKC gene was amplified by PCR using primers designed using Primer Premier 5.0 (Version 5.0; Premier, Canada). Primer sequences were as follows: forward, 5′-GAA ACA GCA GTG ACC AAG AC-3′ and reverse, 5′-CCT AGG CAA CAG AGT GAA AC-3′. The PCR master mix (total volume of 10 µL, including 2× Taq PCR Master Mix + template DNA to be amplified and PCR primers) was added to a 384-well plate, and amplification detection was performed in a Veriti 384-well PCR instrument (Applied Biosystems, Foster City, CA, USA). The thermal cycling conditions were as follows: denaturation at 94°C for 5 min followed by 35 cycles of denaturation at 94°C for 20 s, annealing at 60°C for 30 s, extension at 72°C for 30 s, and a final extension step at 72°C for 3 min. DNA sequencing was performed by a biotechnology company (Tianyi-Huayu Gene Sci, Wuhan, China), and an ABI 3730xl DNA Analyzer (Applied Biosystems, Foster City, CA, USA) was used to analyze the sequencing results.
Total RNA was extracted from 500 µL of blood collected from each participant using a Blood Total RNA Kit (Simgen, Hangzhou, China). Concentration and quality of total RNA were assessed using a nucleic acid quantifier and then reverse transcribed into cDNA using the HiScript QRT SuperMix for real-time quantitative PCR (+gDNA Wiper; Vazyme, Nanjing, China). Next, real-time quantitative PCR analysis was performed using a StepOnePlus Real-Time PCR system (Applied Biosystems, Foster City, CA, USA) with SYBR® Select Master Mix (2X) under the following cycling conditions: 95°C for 30 s followed by 40 cycles of 95°C for 10 s and 60°C for 30 s. The following primers were used: ITPKC, forward 5′-TGC CAT CTG TCA AAT CGG-3′ and reverse 5′-CCA TCT GGG AGC AGT TAC CT-3′; GAPDH, forward 5′-GAG GCA TTG TGA ACC GCA-3′ and reverse 5′-GTG CTT CCT CCG TGT CTG T-3′. The relative amounts of all mRNAs were normalized to GAPDH mRNA expression levels using the 2−ΔΔCT method, and the results are shown as fold changes relative to controls.
We collected information regarding the following immune/inflammation-related indicators from children with KD: counts of white blood cell (WBC), neutrophils, and lymphocytes; platelet count; serum sodium and albumin concentrations; and C-reactive protein (CRP) concentration. Laboratory data were collected during the acute febrile period and before IVIG administration. Neutrophil-to-lymphocyte count ratio (NLR), platelet-to-lymphocyte count ratio, capillary leakage index (CLI) = CRP (mg/L)/albumin (g/L), and systemic immune-inflammation index (SII) = platelet count (×109/L)× (neutrophil count [×109/L]/lymphocyte count [×109/L]) were calculated based on the indexes mentioned above. A routine echocardiogram was performed before IVIG treatment in the acute stage (baseline, days < 10), followed by repeated exams at 1, 3, 6, and 12 months after the onset of fever, and then on a 6-month to annual basis until the CAAs had returned to their normal size.
Statistical differences in allele and genotype frequencies were evaluated using Fisher’s exact test, the chi-square test, or Pearson’s chi-square test for all groups. The chi-square test was used to assess the demographic distributions and genotype frequencies in children with KD and healthy controls. The Hardy–Weinberg equilibrium was assessed using the chi-square test with 1 degree of freedom for the control group. Differences in immune/inflammation-related indicators between different genotypes among children with KD were evaluated by one-way analysis or the Kruskal–Wallis H test. Bonferroni correction was applied for multiple comparisons. The crude and adjusted odds ratios (ORs) and 95% confidence intervals (CIs) were also calculated using unconditional univariate logistic regression analysis to evaluate the associations between genotypes and KD subgroups after adjustment and stratification by age and sex. A two-tailed P value of < 0.05 was considered significant in statistical analyses performed using IBM SPSS Statistics for Windows, version 26 (IBM Corp., Armonk, New York, USA).
A total of 221 patients with KD and 262 controls were recruited in this study; 68.3% (151/221) of the KD cases and 61.1% (160/262) of the controls were male patients. The mean age of the patients and controls was 3.31 ± 2.59 years and 3.61 ± 2.46 years, respectively. No significant difference was observed between the two groups regarding age and sex (P = 0.097 and P = 0.078, respectively). Of the children with KD, 37.1% (82/221) had CAA formation, and 20.8% (46/221) had initial IVIG resistance. Details of all included studies are shown in Supplementary Table S1.
We determined the genotype frequency distributions among the KD cases and controls to explore the association between the ITPKC rs7251246 polymorphism and KD susceptibility. As shown in Table 1, the genotype frequencies of the controls were within the Hardy–Weinberg equilibrium (P = 0.756). The genotype frequency distributions of the different ITPKC rs7251246 polymorphisms were 29.0% (TT), 51.6% (CT), and 19.5% (CC) in the KD group, and 30.5% (TT), 52.3% (CT), and 17.2% (CC) in the control group. No significant difference was observed between controls and children with KD or children with or without IVIG resistance regarding genotypes, alleles, and carrier frequencies of rs7251246 polymorphisms. Notably, a statistically significant difference was observed between children with and without CAA (genotype distribution, P = 0.046; allele frequency, P = 0.020; dominant model, P = 0.017; additive model, P = 0.021); Additional subgroup analyses, according to age, sex, and CAA size, did not identify differences between any subgroups; however, differences in allele frequency (P = 0.094), recessive model (P = 0.072), and additive model (P = 0.086) reached a borderline-significant difference between CAA with and without thrombosis. A separate sub-analysis was conducted in male children with CAA for additional prognosis analysis; a higher risk of thrombosis was observed when the CC genotype was present compared with CT/TT genotypes (recessive model, P=0.029). Unconditional univariate logistic regression analysis showed that the ITPKC SNP rs7251246 was significantly associated with CAA formation [CC/CT vs. TT: OR = 2.176, 95% CI = 1.138–4.162, P = 0.019]. After correcting for age and sex, the significance of rs7251246 remained [CC/CT vs. TT: adjusted OR = 2.089, 95% CI = 1.085–4.020, P = 0.027]. In addition, male children with the rs7251246 CT/TT genotype had a lower risk of thrombosis than male children with the CC genotype [adjusted OR = 0.251, 95% CI = 0.068–0.923, P = 0.037] (Table 2).
Table 1 Genotyping and allele frequency of ITPKC in KD cases and controls, as well as in KD subgroups.
Table 2 Stratification analysis of the association between ITPKC rs7251246 polymorphisms and the risk of Kawasaki disease among groups.
The circulating levels of immune/inflammation-related indicators measured in the plasma of affected children during the acute phase of KD are shown in Figure 2. Children with the CC genotype had the highest concentrations of these indicators (WBC, NLR, CRP, CLI, and SII) and significantly lower serum sodium concentrations. Similar results were obtained for children with or without CAA, and children with the CC genotype had a significantly higher SII than those with either CT or TT genotypes (Figure 3). Furthermore, children with CAA with the CC genotype had longer progressive coronary diameter dilatation and the duration of aneurysm and a larger maximum z-score at baseline and one month after onset (Figure 4). More details on the indicators between different genotypic groups are shown in Supplementary Table S2.
Figure 2 Relationship between rs7251246 genotypes and immune/inflammation-related indicators in patients with KD. KD, Kawasaki disease.
Figure 3 Relationship between rs7251246 genotypes and immune/inflammation-related indicators in patients with KD and CAA. KD, Kawasaki disease; CAA, coronary artery aneurysm.
Figure 4 Relationship between rs7251246 genotypes and prognostic indicators in patients with KD and CAA KD. Kawasaki disease; CAA, coronary artery aneurysm.
The elevation of immune/inflammation-related indicators is associated with T cell activation; hence, the expression of ITPKC at the mRNA level was examined using data from whole blood serial samples obtained from children with KD (n = 182) and healthy children (n = 100). Details regarding the comparison of ITPKC mRNA expression among groups with different rs7251246 genotypes are shown in Supplementary Table S3. Significant downregulation of ITPKC mRNA expression was observed in children with KD (0.50 vs. 0.77, P < 0.001, Figure 5), especially in those with KD and CAA (0.40 vs. 0.55, P = 0.005, Figure 5). Furthermore, compared to those with small-sized CAA, a more robust downregulation of ITPKC mRNA expression was observed in children with KD and mid- to large-sized CAA (0.48 vs. 0.37, P = 0.076, Figure 5). However, the small sample size limited statistical power. Notably, the mRNA levels of ITPKC were significantly lower in children with CAA who developed thrombosis than in those without thrombosis (0.34 vs. 0.45, P = 0.039, Figure 5). Interestingly, among the children with KD, those with the TT genotype expressed the highest level of ITPKC mRNA, followed in sequence by those with CT and CC genotypes. The difference between CC and TT genotypes reached statistical significance (0.43 vs. 0.58, P = 0.035, Figure 5).
Figure 5 Comparison of ITPKC gene expression among the groups (A) KD vs. control; (B) KD with vs. without CAA; (C) small-sized CAA vs. mid- to large-sized CAA; (D) CAA with vs. without thrombosis; (E) KD with different genotypes. ITPKC, Inositol 1,4,5-trisphosphate 3-kinase C; KD, Kawasaki disease; CAA, coronary artery aneurysm.
We investigated the association between the ITPKC polymorphism (rs7251246) and susceptibility to KD as well as the severity of coronary artery damage in a case-control investigation of a Han Chinese population. Our study included 221 children with KD (46 with IVIG resistance and 82 with CAA) and 262 healthy controls. We found that the rs7251246 polymorphism of ITPKC did not influence the susceptibility to KD or response to IVIG therapy in our population, only CAA development. We found that the ITPKC rs7251246 T > C polymorphism is associated with coronary aneurysm complications in children with KD. Furthermore, the rs7251246 SNPs were overrepresented in patients with elevated immune/inflammation-related marker levels. Our gene expression study also found a significant downregulation of ITPKC mRNA expression in children with KD, especially those with CAA, compared to controls.
Despite many years of research, KD’s etiology and pathogenesis remain unknown, limiting the treatment options. Genetic susceptibility is believed to be a key factor contributing to the etiopathogenesis of KD. Several genes essential to the pathogenesis of cardiovascular diseases or the systemic inflammatory immune response have been confirmed to be involved during the onset and development of KD. The ITPKC gene is known to play a role in immune activation and the T-cell receptor signaling, mediating NLRP3 inflammasome activation via control of [Ca2+]i mobilization and directing production of interleukin-1β (IL-1β) and IL-18 (18, 32, 33). Cyclosporine, an inhibitor of T cells (Ca2+/NFAT) pathway, can be combined with IVIG to reduce the incidence of CAA (34), pointing to calcium mobilization as a key mechanism of KD immunopathogenesis. Calcium-dependent inflammatory cytokines can be over-activated by decreasing the ITPKC gene expression via IP3-mediated pathways. Consequently, coronary vessel walls become damaged, causing formation of aneurysms (26). ITPKC gene polymorphisms have important functional consequences in KD, considering that treatment failure in those with the high-risk ITPKC genotype is suggested to be linked to higher intracellular calcium levels and increased IL-1β and IL-6 production, and higher circulating levels of both cytokines (18). Thus, surveys of SNPs associated with susceptibility to and severity of KD, the development of coronary artery complications, and IVIG resistance are ongoing (16) (see Supplementary File 1 for the review of SNPs of the ITPKC gene in association with KD). A novel SNP, rs7251246, was reported to be associated with KD severity in Taiwanese patients in 2014 (28); however, it has not been investigated in a different population, and ITPKC expression is affected by rs7251246 in an unknown manner. In this case-control study, we investigated the association of this SNP with KD in Han Chinese children with or without CAA.
Compared to the studies by Kuo et al. that investigated the rs7251246 SNP in Taiwanese patients (28), our study used a more sensitive body surface area-adjusted coronary artery z-score to define CAA and, as a result, reported statistically significant results. We found that the ITPKC SNP was significantly associated with CAA formation but not with KD susceptibility or IVIG resistance, which is consistent with the results of a previous study (28) supporting the hypothesis that different genes determine susceptibility to KD and CAA formation. Remarkably, our study further identified that the ITPKC rs7251246 CC/CT genotype significantly increased the risk of CAA in children with KD and that the CC genotype may increase the risk of thrombus formation even further, as a borderline difference was observed between the groups.
Male sex has been recently reported to be associated with a high incidence of coronary thrombosis (35). In the present study, we found that male patients with CAA and the rs7251246 CC genotype had a significantly higher risk of thrombosis than children with the CT/TT genotype. This result may be attributed to male patients being more genetically susceptible to CAA and thrombotic events (35, 36). As low-dose aspirin alone is not sufficient to prevent CAA thrombosis (37–39), the AHA recommends dual antiplatelet therapy as prophylaxis for patients at high risk (3); hence, dual antiplatelet therapy is recommended for male children with the CC genotype of rs7251246 to prevent thrombosis because the medical management of such patients depends on the judicious use of thromboprophylaxis. To our knowledge, this study is the first to investigate the association between the ITPKC rs7251246 polymorphism and KD susceptibility in a pediatric Han Chinese population, as well as CAA subgroups, providing evidence for the value of risk stratification among patients with CAA, which may facilitate the identification of high-risk patients in need of targeted interventions.
Genetic studies have shown that polymorphisms of ITPKC, which fail to downregulate NFAT translocation in T-cells, are an important predisposing factor for KD (17, 40). Decreased ITPKC expression results in overly active T cell activation, leading to an excessive inflammatory response (17), which is involved in KD pathogenesis (40–42). This study showed significant downregulation of ITPKC mRNA expression in children with KD, especially those with KD and CAA, compared to controls. This further supports the theory that decreased ITPKC expression promotes KD and its serious complications (41, 42). Moreover, children with CAA and the rs7251246 CC genotype had a significantly higher SII than children with the CT/TT genotype. SII is proposed as an independent risk factor for several cardiovascular complications in patients with KD (43), suggesting that a vigorous inflammatory and host immune response may occur in these children.
Additionally, a progressive increase in CAA size and a longer duration of CAA are strongly correlated with poor long-term prognosis of coronary artery disease (30, 44–48). This study explored the mechanism by which rs7251246 affected ITPKC expression for the first time and presented a promising therapeutic target for KD therapy; however, the biological impact of rs7251246 in KD pathogenesis requires further study. Furthermore, the study observed markedly prolonged dilatation of coronary diameter and aneurysm persistence time in children with CAA and the rs7251246 CC genotype. These observations reiterate that these children’s severe inflammatory reactions and tissue injury warrant additional attention. Notably, ITPKC mRNA expression was the highest in the TT genotype and lowest in the CC genotype, and statistically significant differences were observed between the two genotypes. Based on this, we hypothesize that a reduction in the splicing efficiency of ITPKC mRNA due to the rs7251246 C allele might be responsible for the decrease in ITPKC expression. However, we cannot reach a robust conclusion if rs7251246 is a functional SNP of the ITPKC gene based on the available evidence, as the result does not exclude the possibility of a contribution by other polymorphisms in ITPKC to mRNA expression, such as rs7257602 and rs890934—both also located in intron 1 of the gene—even though no association with CALs formation was observed in the Taiwanese population (28). A more extensive array of SNPs may need to be assessed to find such an association, particularly when subjects with different ethnic backgrounds are tested.
The current study expanded on the findings of Kuo et al. and included more CAA subgroup analysis and an unconditional univariate, adjusted logistic regression analysis for the prediction of CAA and thrombosis, which ultimately could be considered the more important endpoint. Additionally, the current study also examined the mean difference of ITPKC mRNA expression between different genotypic groups. Several limitations, however, apply to the present study. We only enrolled patients who fulfilled the complete criteria of KD in order to maximize the homogeneity of the clinical phenotype. Furthermore, the incidence of CAA (37.1%) was substantially higher than that in previous reports (2–24.1%) because of a significant reduction in the number of children with KD recruited due to the COVID‐19 epidemic (49, 50). Most clinical specimens were obtained from outpatients with follow-ups during this period. Further, given its invasive nature, coronary angiography was only performed in patients with giant CAA or thrombosis, which did not allow for comparisons between echocardiography and coronary angiography findings. However, the results of our study are consistent with those in the study by Kuo et al. regarding the genotyping and allele frequency of rs7251246 in KD cases and controls, as well as in children with or without CAA (see Supplementary Table S4). Additionally, while we considered the effects of age and sex in the logistic regression analysis, our limited matching criteria may have resulted in unintended biases in the results. We did not consider other factors, such as hereditary familial factors, birth history, and environmental interactions, which may have impacted the outcome of the analysis. Another limitation is that we focused on only one allele associated with ITPKC, rs7251246 T > C; other polymorphisms and ITPKC loci were omitted. Furthermore, we only analyzed a Southern Han Chinese child population, and this study had a small sample size, which might limit the generalization of our results. Finally, we did not perform ITPKC rs7251246 functional studies, which are needed to confirm the relationship with the phenotype. Prospective future studies with larger sample sizes that consider additional risk factors or other ethnicities should be conducted.
Our results suggest that the ITPKC rs7251246 T > C polymorphism may be involved in the development of CAA and the severity of coronary artery damage in children with KD. This genotype could be considered a predictive biomarker of CAA in pediatric patients with KD in the Han Chinese population, especially male children. Follow-up studies are required to investigate the affected mechanisms of ITPKC rs7251246 T > C in children with KD.
The datasets generated and/or analyzed during the current study are available from the corresponding author upon reasonable request.
Written informed consent was obtained from the individual(s), and minor(s)’ legal guardian/next of kin, for the publication of any potentially identifiable images or data included in this article.
JL drafted the manuscript, contributed to the data collection, performed the statistical analysis, provided the figures, and approved the final manuscript as submitted. PY administered primary treatment to these patients while they were admitted, contributed to the study design, and approved the final manuscript as submitted. DS contributed to the study design and performed the statistical analysis. YP and DS conceived and designed the study, contributed to the data collection, approved the final manuscript as submitted, and contributed equally to this work. All authors contributed to the article and approved the submitted version.
This work was supported by the Guangxi medical and health key discipline construction project 2019 (19) and the Guangxi Clinical Research Center for Pediatric Disease (no.: GUI KE AD22035219).
We thank Dr. Qiaoyu Yue for her helpful advice and discussions. We also thank Suyuan Qin, Bingbing Ye, Yuqin Huang, FeiFei Li, Ruoxia Chen, and Kaizhi Liang from the Pediatrics Department of First Affiliated Hospital of Guangxi Medical University, who instructed and helped us prepare the manuscript.
The authors declare that the research was conducted in the absence of any commercial or financial relationships that could be construed as a potential conflict of interest.
All claims expressed in this article are solely those of the authors and do not necessarily represent those of their affiliated organizations, or those of the publisher, the editors and the reviewers. Any product that may be evaluated in this article, or claim that may be made by its manufacturer, is not guaranteed or endorsed by the publisher.
The Supplementary Material for this article can be found online at: https://www.frontiersin.org/articles/10.3389/fimmu.2023.1184162/full#supplementary-material
1. Kawasaki T, Singh S. Kawasaki Disease - the journey over 50 years: 1967-2017. Int J Rheum Dis (2018) 21(1):7–9. doi: 10.1111/1756-185x.13215
2. Burns JC, Glodé MP. Kawasaki Syndrome. Lancet (2014) 364(9433):533–44. doi: 10.1016/s0140-6736(04)16814-1
3. McCrindle BW, Rowley AH, Newburger JW, Burns JC, Bolger AF, Gewitz M, et al. American Heart association rheumatic fever, endocarditis, and Kawasaki disease committee of the council on cardiovascular disease in the young, council on cardiovascular and stroke nursing; council on cardiovascular surgery and anesthesia, and council on epidemiology and prevention, diagnosis, treatment, and long-term management of Kawasaki disease: a scientific statement for health professionals from the American heart association. Circulation (2017) 135(17):e927–99. doi: 10.1161/cir.0000000000000484
4. Masuda H, Ae R, Koshimizu TA, Matsumura M, Kosami K, Hayashida K, et al. Epidemiology and risk factors for giant coronary artery aneurysms identified after acute Kawasaki disease. Pediatr Cardiol (2021) 42(4):969–77. doi: 10.1007/s00246-021-02571-8
5. Xie LP, Yan WL, Huang M, Huang MR, Chen S, Huang GY, et al. Epidemiologic features of Kawasaki disease in shanghai from 2013 through 2017. J Epidemiol (2020) 30(10):29–35. doi: 10.2188/jea.je20190065
6. Wu MH, Chen HC, Yeh SJ, Lin MT, Huang SC, Huang SK. Prevalence and the long-term coronary risks of patients with Kawasaki disease in a general population <40 years: a national database study. Circ Cardiovasc Qual Outcomes (2012) 5(4):566–70. doi: 10.1161/circoutcomes.112.965194
7. Du ZD, Zhao D, Du J, Zhang YL, Lin Y, Liu C, et al. Epidemiologic study on Kawasaki disease in Beijing from 2000 through 2004. Pediatr Infect Dis J (2007) 26(5):449–51. doi: 10.1097/01.inf.0000261196.79223.18
8. Singh S, Vignesh P, Burgner D. The epidemiology of Kawasaki disease: a global update. Arch Dis Child (2015) 100(11):1084–8. doi: 10.1136/archdischild-2014-307536
9. Uehara R, Yashiro M, Nakamura Y, Yanagawa H. Parents with a history of Kawasaki disease whose child also had the same disease. Pediatr Int (2011) 53(4):511–4. doi: 10.1111/j.1442-200x.2010.03267.x
10. Burns JC, Newburger JW. Genetics insights into the pathogenesis of Kawasaki disease. Circ Cardiovasc Genet (2012) 5(3):277–8. doi: 10.1161/CIRCGENETICS.112.963710
11. Onouchi Y. Genetics of Kawasaki disease: what we know and don't know. Circ J (2012) 76(7):1581–6. doi: 10.1253/circj.cj-12-0568
12. Kumrah R, Vignesh P, Rawat A, Singh S. Immunogenetics of Kawasaki disease. Clin Rev Allerg Immu (2020) 59(1):122–39. doi: 10.1007/s12016-020-08783-9
13. Arj-Ong S, Thakkinstian A, McEvoy M, Attia. A systematic review J. And meta-analysis of tumor necrosis factor α-308 polymorphism and Kawasaki disease. Pediatr Int (2010) 52(4):527–32. doi: 10.1111/j.1442-200x.2010.03105.x
14. Li Z, Han D, Jiang J, Chen J, Tian L, Yang Z. Association of PECAM-1 gene polymorphisms with Kawasaki disease in Chinese children. Dis Markers (2017) 2017:2960502. doi: 10.1155/2017/2960502
15. Weng KP, Hsieh KS, Hwang YT, Huang SH, Lai TJ, Yuh YS, et al. IL-10 polymorphisms are associated with coronary artery lesions in acute stage of Kawasaki disease. Circ J (2010) 74(5):983–9. doi: 10.1253/circj.cj-09-0801
16. Kim KY, Kim DS. Recent advances in Kawasaki disease. Yonsei Med J (2016) 57(1):15–21. doi: 10.3349/ymj.2016.57.1.15
17. Onouchi Y, Gunji T, Burns JC, Shimizu C, Newburger JW, Yashiro M, et al. ITPKC functional polymorphism associated with Kawasaki disease susceptibility and formation of coronary artery aneurysms. Nat Genet (2008) 40(1):35–42. doi: 10.1038/ng.2007.59
18. Alphonse MP, Duong TT, Shumitzu C, Hoang TL, McCrindle BW, Franco A, et al. Inositol-triphosphate 3-kinase c mediates inflammasome activation and treatment response in Kawasaki disease. J Immunol (2016) 197(9):3481–89. doi: 10.4049/jimmunol.1600388
19. Dewaste V, Moreau C, De Smedt F, Bex F, De Smedt H, Wuytack F, et al. The three isoenzymes of human inositol-1,4,5-trisphosphate 3-kinase show specific intracellular localization but comparable Ca2+ responses on transfection in COS-7 cells. Biochem J (2003) 374(Pt 1):41–9. doi: 10.1042/bj20021963
20. Onouchi Y, Suzuki Y, Suzuki H, Terai M, Yasukawa K, Hamada H, et al. ITPKC and CASP3 polymorphisms and risks for IVIG unresponsiveness and coronary artery lesion formation in Kawasaki disease. Pharmacogenomics J (2013) 13(1):52–9. doi: 10.1038/tpj.2011.45
21. Peng Q, Chen C, Zhang Y, He H, Wu Q, Liao J, et al. Single-nucleotide polymorphism rs2290692 in the 3'UTR of ITPKC associated with susceptibility to Kawasaki disease in a han Chinese population. Pediatr Cardiol (2012) 33(7):1046–53. doi: 10.1007/s00246-012-0223-x
22. Wang W, Lou J, Zhong R, Qi YQ, Shen N, Lu XZ, et al. The roles of Ca2+/NFAT signaling genes in Kawasaki disease: single- and multiple-risk genetic variants. Sci Rep (2014) 4:5208. doi: 10.1038/srep05208
23. Lou J, Xu S, Zou L, Zhong R, Zhang T, Sun Y, et al. A functional polymorphism, rs28493229, in ITPKC and risk of Kawasaki disease: an integrated meta-analysis. Mol Biol Rep (2012) 39(12):11137–44. doi: 10.1007/s11033-012-2022-0
24. Onouchi Y, Tamari M, Takahashi A, Tsunoda T, Yashiro M, Nakamura Y, et al. A genomewide linkage analysis of Kawasaki disease: evidence for linkage to chromosome 12. J Hum Genet (2007) 52(2):179–90. doi: 10.1007/s10038-006-0092-3
25. Chi H, Huang FY, Chen MR, Chiu NC, Lee HC, Lin SP, et al. ITPKC gene SNP rs28493229 and Kawasaki disease in Taiwanese children, hum. Mol Genet (2010) 19(6):1147–51. doi: 10.1093/hmg/ddp586
26. Kuo HC, Yang KD, Juo SH, Liang CD, Chen WC, Wang YS, et al. ITPKC single nucleotide polymorphism associated with the Kawasaki disease in a Taiwanese population. PloS One (2011) 6(4):e17370. doi: 10.1371/journal.pone.0017370
27. Peng Q, Chen CH, Wu Q, Li B, Liao J, Luo CD, et al. Association study of a functional SNP rs28493229 of ITPKC gene and Kawasaki disease in a Chinese population. Zhonghua Yi Xue Yi Chuan Xue Za Zhi (2011) 28(6):644–8. doi: 10.3760/cma.j.issn.1003-9406.2011.06.010
28. Kuo HC, Hsu YW, Lo MH, Huang YH, Chien SC, Chang WC. Single-nucleotide polymorphism rs7251246 in ITPKC is associated with susceptibility and coronary artery lesions in Kawasaki disease. PloS One (2014) 9(3):e91118. doi: 10.1371/journal.pone.0091118
29. Newburger JW, Takahashi M, Gerber MA, Gewitz MH, Tani LY, Burns JC, et al. Committee on rheumatic fever, endocarditis and Kawasaki disease, council on cardiovascular disease in the young, American heart association, American academy of pediatrics, diagnosis, treatment, and long-term management of Kawasaki disease: a statement for health professionals from the committee on rheumatic fever, endocarditis and Kawasaki disease, council on cardiovascular disease in the young, American heart association. Circulation (2004) 110(17):2747–71. doi: 10.1161/01.cir.0000145143.19711.78
30. Fukazawa R, Kobayashi J, Ayusawa M, Hamada H, Miura M, Mitani Y, et al. Japanese Circulation society joint working, JCS/JSCS 2020 guideline on diagnosis and management of cardiovascular sequelae in Kawasaki disease. Circ J (2020) 84(8):1348–407. doi: 10.1253/circj.cj-19-1094
31. Dallaire F, Dahdah N. New equations and a critical appraisal of coronary artery z scores in healthy children. J Am Soc Echocardiogr (2011) 24(1):60–74. doi: 10.1016/j.echo.2010.10.004
32. Lee GS, Subramanian N, Kim AI, Aksentijevich I, Goldbach-Mansky R, Sacks DB, et al. The calcium-sensing receptor regulates the NLRP3 inflammasome through Ca2+ and cAMP. Nature (2012) 492:123–7. doi: 10.1038/nature11588
33. Murakami T, Ockinger J, Yu J, Byles V, McColl A, Hofer AM, et al. Critical role for calcium mobilization in activation of the NLRP3 inflammasome. Proc Natl Acad Sci USA (2012) 109:11282–7. doi: 10.1073/pnas.1117765109
34. Hamada H, Suzuki H, Onouchi Y, Ebata R, Terai M, Fuse S, et al. Efficacy of primary treatment with immunoglobulin plus ciclosporin for prevention of coronary artery abnormalities in patients with Kawasaki disease predicted to be at increased risk of non-response to intravenous immunoglobulin (KAICA): a randomised controlled, open-label, blinded-endpoints, phase 3 trial. Lancet (London England) (2019) 393(10176):1128–37. doi: 10.1016/S0140-6736(18)32003-8
35. Peng Y, Yi. Incidence Q. And timing of coronary thrombosis in Kawasaki disease patients with giant coronary artery aneurysm. Thromb Res (2023) 221:30–4. doi: 10.1016/j.thromres.2022.11.014
36. Dietz SM, Kuipers IM, Tacke CEA, Koole JCD, Hutten BA, Kuijpers TW. Giant aneurysms: a gender-specific complication of Kawasaki disease? J Cardiol (2017) 70(4):359–65. doi: 10.1016/j.jjcc.2016.12.014
37. Israels SJ, Michelson AD. Antiplatelet therapy in children. Thromb Res (2006) 118:75–83. doi: 10.1016/j.thromres.2004.12.016
38. Patrono C, Bachmann F, Baigent C, Bode C, De Caterina R, Charbonnier B, et al. Expert consensus document on the use of antiplatelet agents. the task force on the use of antiplatelet agents in patients with atherosclerotic cardiovascular disease of the European society of cardiology. Eur Heart J (2004) 25:166–81. doi: 10.1016/j.ehj.2003.10.013
39. Patel AS, Bruce M, Harrington W, Portman MA. Coronary artery stenosis risk and time course in Kawasaki disease patients: experience at a US tertiary pediatric centre. Open Heart (2015) 2:e000206. doi: 10.1136/openhrt-2014-000206
40. Dietz SM, van Stijn D, Burgner D, Levin M, Kuipers IM, Hutten BA, et al. Dissecting Kawasaki disease: a state-of-the-art review. Eur J Pediatr (2017) 176(8):995–1009. doi: 10.1007/s00431-017-2937-5
41. Ji ML, Dong JY, Xu Y, Pan YT, Fan ZD, Yu HG. Inositol-triphosphate 3-kinase c and DNA methylation involvement in NLRP3 inflammasome activation in Kawasaki disease. Indian J Pediatr (2022) 90(1):22–8. doi: 10.1007/s12098-022-04126-y
42. Macian F. NFAT proteins: key regulators of T-cell development and function. Nat Rev Immunol (2005) 5(6):472–84. doi: 10.1038/nri1632
43. Liu X, Shao S, Wang L, Zhang N, Wu M, Liu L, et al. Predictive value of the systemic immune-inflammation index for intravenous immunoglobulin resistance and cardiovascular complications in Kawasaki disease. Front Cardiovasc Med (2021) 8:711007. doi: 10.3389/fcvm.2021.711007
44. Chih WL, Wu PY, Sun LC, Lin MT, Wang JK, Wu MH. Progressive coronary dilatation predicts worse outcome in Kawasaki disease. J Pediatr (2016) 171:78–82.e1. doi: 10.1016/j.jpeds.2015.12.076
45. Dallaire F, Fournier A, Breton J, Nguyen TD, Spigelblatt L, Dahdah N. Marked variations in serial coronary artery diameter measures in Kawasaki disease: a new indicator of coronary involvement. J Am Soc Echocardiog (2012) 25(8):859–65. doi: 10.1016/j.echo.2012.05.019
46. Mitani Y, Tsuda E, Kato H, Higaki T, Fujiwara M, Ogawa S, et al. Emergence and characterization of acute coronary syndrome in adults after confirmed or missed history of Kawasaki disease in Japan: a Japanese nationwide survey. Front Pediatr (2019) 7:275. doi: 10.3389/fped.2019.00275
47. Tsuda E, Kamiya T, Ono Y, Kimura K, Kurosaki K, Echigo S. Incidence of stenotic lesions predicted by acute phase changes in coronary arterial diameter during Kawasaki disease. Pediatr Cardiol (2005) 26(1):73–9. doi: 10.1007/s00246-004-0698-1
48. Holve TJ, Patel A, Chau Q, Marks AR, Meadows A, Zaroff JG. Long-term cardiovascular outcomes in survivors of Kawasaki disease. Pediatrics (2014) 133(2):e305–11. doi: 10.1542/peds.2013-1638
49. Ae R, Makino N, Kuwabara M, Matsubara Y, Kosami K, Sasahara T, et al. Incidence of Kawasaki disease before and after the COVID-19 pandemic in Japan: results of the 26th nationwide survey, 2019 to 2020. JAMA Pediatr (2022) 176(12):1217–24. doi: 10.1001/jamapediatrics.2022.3756
Keywords: Kawasaki disease, coronary artery aneurysm, thrombosis, ITPKC, polymorphisms
Citation: Liu J, Yuan P, Pang Y and Su D (2023) ITPKC polymorphism (rs7251246 T > C), coronary artery aneurysms, and thrombosis in patients with Kawasaki disease in a Southern Han Chinese population. Front. Immunol. 14:1184162. doi: 10.3389/fimmu.2023.1184162
Received: 11 March 2023; Accepted: 06 June 2023;
Published: 19 June 2023.
Edited by:
Li-Tung Huang, Kaohsiung Chang Gung Memorial Hospital, TaiwanReviewed by:
Rakesh Kumar Pilania, Post Graduate Institute of Medical Education and Research (PGIMER), IndiaCopyright © 2023 Liu, Yuan, Pang and Su. This is an open-access article distributed under the terms of the Creative Commons Attribution License (CC BY). The use, distribution or reproduction in other forums is permitted, provided the original author(s) and the copyright owner(s) are credited and that the original publication in this journal is cited, in accordance with accepted academic practice. No use, distribution or reproduction is permitted which does not comply with these terms.
*Correspondence: Yusheng Pang, cGFuZ3l1c2hAMTYzLmNvbQ==; Danyan Su, c3VkYW55ZWFsQDE2My5jb20=
Disclaimer: All claims expressed in this article are solely those of the authors and do not necessarily represent those of their affiliated organizations, or those of the publisher, the editors and the reviewers. Any product that may be evaluated in this article or claim that may be made by its manufacturer is not guaranteed or endorsed by the publisher.
Research integrity at Frontiers
Learn more about the work of our research integrity team to safeguard the quality of each article we publish.