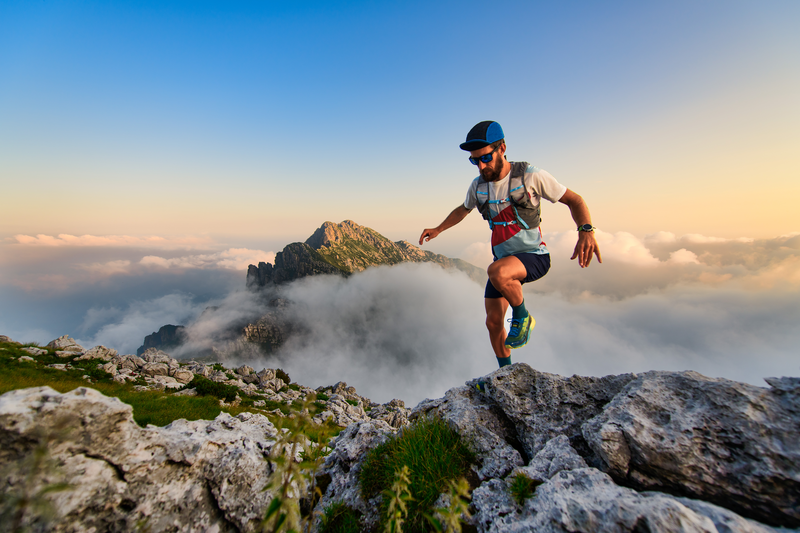
95% of researchers rate our articles as excellent or good
Learn more about the work of our research integrity team to safeguard the quality of each article we publish.
Find out more
ORIGINAL RESEARCH article
Front. Immunol. , 05 September 2023
Sec. Multiple Sclerosis and Neuroimmunology
Volume 14 - 2023 | https://doi.org/10.3389/fimmu.2023.1177721
Introduction: We aimed to identify B-cell-mediated immunomechanisms in inclusion body myositis (IBM) and polymyositis (PM) as part of the complex pathophysiology.
Materials and methods: Human primary myotube cultures were derived from orthopedic surgery. Diagnostic biopsy specimens from patients with IBM (n=9) and PM (n=9) were analyzed for markers of B cell activation (BAFF and APRIL) and for chemokines that control the recruitment of B cells (CXCL-12 and CXCL-13). Results were compared to biopsy specimens without myopathic changes (n=9) and hereditary muscular dystrophy (n=9).
Results: The mRNA expression of BAFF, APRIL, and CXCL-13 was significantly higher in IBM and PM compared to controls. Patients with IBM displayed the highest number of double positive muscle fibers for BAFF and CXCL-12 (48%) compared to PM (25%), muscular dystrophy (3%), and non-myopathic controls (0%). In vitro, exposure of human myotubes to pro-inflammatory cytokines led to a significant upregulation of BAFF and CXCL-12, but APRIL and CXCL-13 remained unchanged.
Conclusion: The results substantiate the hypothesis of an involvement of B cell-associated mechanisms in the pathophysiology of IBM and PM. Muscle fibers themselves seem to contribute to the recruitment of B cells and sustain inflammation.
The group of idiopathic inflammatory myopathies consists of dermatomyositis (DM), polymyositis (PM), inclusion body myositis (IBM), necrotizing myopathy (NM), and anti-synthetase syndrome (ASS). Over the last few years, there was growing evidence for the involvement of B cells in idiopathic inflammatory myopathies. Especially in DM and ASS, the role of B-cells in the pathophysiology is well known and several autoantibodies are well characterized (1–3). The histological picture in NM is scattered necrotic myofibers and T- or B cells may be found in focal spots, but there are no primary inflammatory lesions (3).
The pathophysiology of IBM is very complex and, in general, characterized by mechanisms of inflammation and β-amyloid associated degeneration. Recent evidence suggests that inflammation may be the primary event. IBM and PM are considered to be T cell-mediated diseases, but high frequencies of plasma cells were detected in muscle biopsy specimens (4), and infiltrating B-cells and plasma cells were found to be clonally expanded and had undergone affinity maturation locally within the muscle (5–7). Plasma cells derived from IBM muscles produce autoantibodies that are directed against antigens in muscle tissue (e.g., Desmin) (8), and the autoantibody directed against cytosolic 5’-nucleotidase 1A (cN1A) was identified in IBM (9, 10). In pure PM, usually, no myositis-associated antibodies are found. Apart from immune cells, muscle fibers are considered capable of immune cell recruitment and T-cell activation (11). The reason for analyzing IBM and PM in this study as the only two subtypes of myositis is their similar pathophysiology especially regarding CD8 cytotoxicity (3) and the limited knowledge of B-cell mechanisms in both disorders.
IBM and PM were compared to muscular dystrophy as disease control. We did not compare the expression levels with DM, NM, and ASS because B-cell mechanisms in these disorders have been characterized before and we did not aim to repeat these observations.
Another aim of this study was to examine the pathophysiological contribution of muscle cells in the context of myositis and not to analyze the impact of other immune cells.
The B-cell-activating factor of the tumor necrosis family (BAFF) and a proliferation-inducing ligand (APRIL) are crucial for B cell survival, maturation, activation, and differentiation (12, 13). Trafficking of human naive and memory B-cells is mainly orchestrated by CXC-chemokine ligands (CXCL) 12 and 13 (14).
We studied the distribution of these factors associated with B cells and the in vitro effect of an inflammatory environment to drive and sustain a humoral immune response in the skeletal muscle.
The project was approved by the institutional review board (ethics committee) and patients signed informed consent. Diagnostic biopsies were used from the skeletal muscle of patients with inclusion body myositis (n=9), polymyositis (n=9), muscular dystrophy (n=9), and non-myopathic controls (n=9). Most biopsies were obtained from the Department of Neuropathology of the University Medical Center Göttingen. In order to achieve comparable group sizes in IBM and PM, a few additional biopsies were obtained from the Departments of Neuropathology or Neurology of the University Medical Centers of München (PM; n=3), Halle (IBM; n=2), and Hannover (IBM; n=1) (all in Germany).
The anti-cN1A antibody status was documented in one out of nine IBM patients and that was negative.
Frozen sections (5µm) of muscle biopsy specimens or cultured muscle cells were fixed with 4% paraformaldehyde at room temperature (for hematoxylin and eosin staining) or acetone at -20°C (all other stainings) for 10 min. Unspecific binding was reduced by 45 min incubation with 10% bovine serum albumin (BSA) and 100% goat serum (all from Jackson ImmunoResearch, West Grove, USA). The following anti-human antibodies were used: rat anti-BAFF (1:1000, Abcam, Cambridge, USA) and mouse anti-CXCL-12 (1:1000, R&D Systems, Minneapolis, USA). The primary antibodies were diluted in BSA and incubated for 1h at room temperature. Immunoreactivity was detected using Alexa-488 or Alexa-594-conjugated goat antibodies against mice or rats (1:600, all from Molecular Probes, Leiden, Netherlands). Nuclear counterstaining was performed with DAPI (Molecular Probes/Invitrogen, Carlsbad, USA) at 1:50,000 for 1 minute, followed by mounting in Fluoromount-G (Southern Biotech, Alabama, USA). Immunofluorescence microscopy and digital photography were performed on an Axiovert 200m microscope (Zeiss, Oberkochen, Germany) using a 20x and 40x objective, appropriate filters for green (488 nm), red (594 nm), and blue (350 nm) fluorescence, and a cooled CCD digital camera (Retiga 1300; QImaging) using the QCapture software (QImaging). For every biopsy, photomicrographs that covered a cross-section of each biopsy specimen were taken by an investigator who was blinded to the source of the specimen (LM). Scion Image software was used for grey-scale analysis.
Total RNA was extracted from cell culture using a commercial kit (RNeasy from Qiagen, Venlo, Netherlands), following the supplier’s instructions. Total RNA from the muscle biopsy specimen was homogenized in 500 µl TRIzol (life technologies, Carlsbad, USA) with a plastic tissue grinder and pestle (Kimble Chase, Vineland, USA). Precipitation of mRNA was improved by Ambion 9.520 (life technologies, Carlsbad, USA). RNA was eluted in 30 µl RNA-ase free water and stored at -80°C. Complementary DNA (cDNA) synthesis was performed with SuperScript II reverse transcriptase (Invitrogen), following the supplier’s instructions. The resulting cDNA was stored at –20°C. Quantitative (real-time) polymerase chain reaction was performed as previously described (15) on an SDS 7500 Sequence Detection System (Applied Biosystems, Foster City, USA) by 6-carboxyfluorescein (FAM-) labeled specific primer/probes: Glycerinaldehyd-3-Phosphat Dehydrogenase (GAPDH) Hs99999905_m1, B-Cell-Activating Factor (BAFF) Hs00198106_m1, A Proliferation-Inducing Ligand (APRIL) Hs00182565_m1, CXCL-12 Hs00171022_m1, and CXCL-13 Hs00757930_m1 (from Applied Biosystems, Foster City, USA). The resulting mRNA expression was quantified using the Δc(t) method in relation to the expression of GAPDH mRNA.
As described previously (15, 16), satellite cells -muscle cell progenitors- from diagnostic biopsy specimens from patients without myopathic changes were grown according to the following protocol: The muscle piece was minced and washed in phosphate-buffered saline and trypsinized. The fragments were seeded to a 25 cm2 flask in Dulbecco’s modified Eagle’s medium with pyruvate, high glucose, and L-glutamine (Gibco Invitrogen, Carlsbad, USA), supplemented with 10% fetal calf serum (Cambrex Bioscience), penicillin, streptomycin (Gibco Invitrogen, Carlsbad, USA), and 0.5% chick embryo extract (Accurate). After 21 days, myotubes were labeled with neural cell adhesion molecules (anti-CD56, mouse clone Eric-1; Neomarkers/Labvision), followed by magnetic bead–labeled secondary antibodies and subsequently separated by magnets (Dynal/Invitrogen, Carlsbad, USA). For further experiments, myotubes were seeded either in 8-chamber slides (LabTek II; Nunc) or 24-well plates (Nunc), and at 80% confluence, fusion was induced by serum deprivation. Well-differentiated myotubes, as revealed by immunocytochemical staining for the muscle marker desmin, were either kept as unstimulated controls in X-Vivo 15 medium (Cambrex Bioscience) and exposed to the cytokines IFN-γ (300 units/ml), TNFα (10 ng/ml), and IL-1β (20 ng/ml) (all from Chemicon International Inc., Temecula, USA) in serum-free X-Vivo 15 medium. The duration of incubation was 12 to 48h. Scion Image software was used for grey-scale analysis.
For statistical analysis (ANOVA, t-test, Kruskal-Wallis, and Pearson correlation), *P< 0.05, **P< 0.01, and ***P< 0.001 were used as significant values, and all significant outliers (Grubb’s test) were excluded prior to analysis. Statistical analyses were performed using the software GraphPad Prism 7 (San Diego, CA, USA).
We first studied the mRNA expression of factors associated with B-cell activation and chemotaxis in muscle specimens from patients with IBM, PM, muscular dystrophies, and non-myopathic controls. In IBM compared to non-myopathic controls, there was significant overexpression of BAFF (mean 0.028 ± 0.037, P=0.0004), APRIL (mean 0.0021 ± 0.0019, P=0.001), CXCL-12 (mean 0.039 ± 0.06, P=0.003), and CXCL-13 (mean 0.00046 ± 0.00024, P=0.04) (Figure 1). In PM compared to non-myopathic controls, we noted a significant overexpression of BAFF (mean 0.013 ± 0.014, P=0.002), APRIL (mean 0.0017 ± 0.001, P=0.0002), and CXCL-13 (mean 0.0011 ± 0.0012, P=0.04) but not for CXCL-12 (mean 0.0014 ± 0.0019, P=0.067). The expression of all four targets was comparable in PM and IBM and no differences were noted. In comparison to muscular dystrophies, the expression of APRIL and CXCL-13 in IBM and PM showed a tendency for an upregulation, which did not reach statistical significance. CXCL-12 was significantly upregulated in muscular dystrophies compared to non-myopathic controls (mean 0.021 ± 0.026, P=0.03).
Figure 1 mRNA expression of BAFF, APRIL, CXCL-12, and CXCL-13 in muscle tissue specimens by quantitative real-time-PCR: Significant upregulation of BAFF, APRIL, and CXCL-13 in IBM and PM and of CXCL-12 in IBM compared to non-myopathic controls. Comparable results for all molecules in IBM and PM. Upregulation of CXCL-12 in muscular dystrophies compared to non-myopathic controls. Data shown as mean +SD. Statistics using multiple comparisons by Kruskal-Wallis testing, indicating significance by *P< 0.05; **P< 0.01; ***P< 0.001. IBM, inclusion body myositis; PM, polymyositis; Dystrophy, muscular dystrophies; BAFF, B-cell-activating factor of the tumor necrosis family; APRIL, A proliferation-inducing ligand, CXCL-12, CXC-chemokine ligand 12; CXCL-13, CXC-chemokine ligand 13.
Collectively, the B cell-associated factors BAFF and APRIL as well as the B-cell chemoattractants CXCL-12 and 13 were upregulated in muscle specimens from patients with IBM and PM compared to non-myopathic controls. Only CXCL-12 was upregulated in dystrophic muscle compared to non-myopathic controls.
At the protein level, overexpression of BAFF and CXCL-12 in muscle specimens was confirmed by immunohistochemistry (exemplary findings are shown in Figure 2A). Manual counting of the number of fibers in each specimen (range from 78 to 233) in a blinded fashion revealed a significantly higher number of muscle fibers positive for BAFF and CXCL-12 in IBM (BAFF 70%, CXCL-12 56%, and double positive 48%) compared to PM (BAFF 50%, CXCL-12 33%, and double positive 25%). Both forms of myositis displayed a significantly increased number of positive fibers in comparison to muscular dystrophies (BAFF 7%, CXCL-12 10%, and double positive 3%) and non-myopathic controls (BAFF 2.9%, CXCL-12 1.2%, and double positive 0%) (Figure 2B). Similar results were observed by automated grey scale analysis (data not shown).
Figure 2 Analysis of BAFF and CXCL-12 expression on protein levels in IBM and PM by immunohistochemistry: (A) Exemplary findings of muscle tissue specimen of IBM, PM, dystrophies, and muscle control tissue in BAFF and CXCL-12 immunostainings (microphotographs acquired using a 20x objective). (B) Significantly increased numbers of BAFF and CXCL-12 positive muscle fibers in IBM and PM compared with non-myopathic controls and muscular dystrophies. For the analysis, 78 to 233 myofibers were counted in each specimen. Similar results are found in the automated grey scale analysis (data not shown). Data shown as mean +SD. Statistics using ANOVA with Tukey’s multiple comparison test, indicating significance by **P< 0.01; ***P< 0.001. IBM, inclusion body myositis; PM, polymyositis; Dystrophy, muscular dystrophies; BAFF, B-cell-activating factor of the tumor necrosis family; CXCL-12, CXC-chemokine ligand 12.
Our data demonstrate that major sources of BAFF and CXCL-12 are muscle fibers themselves. Taken together, these data suggest that the local inflammatory milieu generated by muscle fibers could directly contribute to the recruitment and activation of B cells in IBM and PM.
Based on our biopsy findings, we assessed the effect of an inflammatory environment on human skeletal muscle in vitro. Human primary myotube cultures were incubated for 24 hours in the presence of the cytokines IFN-γ, IL-1β, and TNF-α alone or in combination, and the induction of BAFF, APRIL, CXCL-12, and CXCL-13 mRNA in muscle cells was measured by qPCR. We noted a strong and significant induction of BAFF by IFN-γ alone or in combination with any other proinflammatory cytokine (Figure 3A; IFN-γ: mean 0.02 ± 0.00067, P<0.001; IFN-γ + IL-1β: mean 0.015 ± 0.00096, P<0.001; IFN-γ + TNFα: mean 0.015 ± 0.00015, P<0.001). For CXCL-12, similar results were observed upon IL-1β alone or in combination with TNF-α (Figure 3B; IL-1β: mean 0.024 ± 0.00088, P=0.005; IL-1β + TNF-α: mean 0.014 ± 0.0062, P=0.026). The combination of IL-1β and IFN-γ did upregulate the expression of CXCL-12, but this did not reach statistical significance compared to controls (Figure 3B; IL-1β + IFN-γ: mean 0.01 ± 0.0015, P=0.17). After 12, 36, and 48 hours of stimulation, similar results were found (data not shown). The assessment for APRIL and CXCL-13 revealed no changes of mRNA levels under proinflammatory conditions (data not shown). The results are averages of two independent experiments and the myotubes were obtained from healthy donors.
Figure 3 mRNA expression of BAFF and CXCL-12 in human myotubes under pro-inflammatory conditions by quantitative (real-time)-PCR after 24 hours of stimulation: (A) Significant induction of BAFF mRNA expression upon IFN-γ (alone or in combination) and of (B) CXCL-12 upon IL-1β (alone or in combination) in human primary myotube cultures. The results are averages of two independent experiments and the myotubes were obtained from non-myopathic donors. BAFF, B-cell-activating factor of the tumor necrosis family; CXCL-12, CXC-chemokine ligand 12. IFN-γ, Interferon γ; IL-1β, Interleukin 1β; TNFα, Tumor necrosis factor α. Data shown as mean +SD. Statistics by ANOVA with Tukey's multiple comparison test, indicating significance by *P< 0.05; **P< 0.01; ***P< 0.001.
For the analysis of the protein expression, myotubes were incubated for 12, 24, or 48 hours with IFN-γ and IL-1β and afterwards stained with anti-BAFF and anti-CXCL-12 antibodies. Exemplary findings of the immunocytochemical staining for BAFF and CXCL-12 are shown in Figure 4. The staining revealed an upregulation of both factors upon exposure to IFN-γ for BAFF or to IL-1β for CXCL-12, respectively. By grey scale analysis, the upregulation of BAFF upon exposure to IFN-γ (Figure 4A; mean 52 ± 6 vs. 90 ± 13, P=0.12) and the induction of CXCL-12 upon IL-1β (Figure 4B; mean 53 ± 8 vs. 124 ± 15, P=0.057) did not reach statistical significance compared to controls. The results are averages of two independent experiments.
Figure 4 Analysis of BAFF and CXCL-12 expression on protein levels in human myotubes under pro-inflammatory conditions by immunocytochemistry: (A) Exemplary finding of BAFF expression in human myotubes after 48 hours exposure to IFN-γ compared to controls (microphotographs acquired using a 20x objective). Greyscale analysis revealed a tendency of an increased protein production, which did not reach statistical significance (t-test). (B) Exemplary finding of CXCL-12 expression in human myotubes after 48 hours exposure to IL-1β compared to controls (microphotographs acquired using a 20x objective). Greyscale analysis revealed a tendency of an increased protein production, which did not reach statistical significance (t-test). The myotubes were obtained from non-myopathic donors. The results are shown as mean +SD from two independent experiments. BAFF, B-cell-activating factor of the tumor necrosis family; CXCL-12, CXC-chemokine ligand 12. IFN-γ, Interferon γ; IL-1β, Interleukin 1β.
Collectively, these data suggest that non-diseased human myotubes can express factors involved in B cell activation and cell recruitment upon exposure to proinflammatory cytokines.
The underlying pathomechanisms of myositis are complex and only partially understood. IBM and PM are thought to be T cell-mediated disorders as infiltrates of T cells can be noted in muscle biopsies. Over the last years, B-cell mechanisms in myositis have drawn more attention and recent results support their contribution to various forms of myositis including PM and IBM (4–7). The role of B-cells is well known in DM and ASS and several autoantibodies were well characterized in these subtypes of myositis (1–3).
In this study, we demonstrate that the B-cell activating factors BAFF and APRIL as well as the chemokines CXCL-12 and CXCL-13 are upregulated on the mRNA level in muscle specimens from IBM and PM compared to controls. An elevated protein expression of BAFF and CXCL-12 in skeletal muscle biopsies was consistent with the upregulated mRNA findings in IBM and PM. Human primary myotubes derived from individuals without a neuromuscular disease produced BAFF and CXCL-12 mRNA and protein upon exposure to proinflammatory cytokines.
The immunohistochemical analysis identified muscle fibers themselves as sources of BAFF and CXCL-12 in PM and IBM. We were unable to detect these markers on or around inflammatory cells, which is likely explained by an insufficient sensitivity of the antibodies used.
Elevated serum levels for BAFF were reported for autoimmune muscular disorders like DM, PM (17–20), and Myasthenia gravis (21) but not for IBM (22). APRIL was identified in unspecified inflammatory muscle disorders only in one study (17). A significant upregulation of BAFF mRNA was reported in muscle specimens of IBM, PM, and DM (6, 23), and CXCL-13 mRNA was significantly upregulated in juvenile DM (24). A recent study observed elevated serum levels of CXCL-13 and other pro-inflammatory cytokines and chemokines in DM and ASS compared to healthy controls (25), yet CXCL-12 was not analyzed.
The expression of APRIL and CXCL-12 mRNA in muscle tissues as well as the expression of BAFF and CXCL-12 mRNA in human myotubes had not been analyzed before. We were able to confirm the finding of increased levels of BAFF mRNA in IBM and PM and could extend the findings to the expression of its ligand APRIL and the chemokines CXCL-12 and 13 in muscle tissue specimens of patients with IBM and PM.
On the protein level, few studies analyzed the expression of factors involved in B-cell activation in myositis. BAFF expression was detected by immunohistochemistry in perifascicular muscle cells in DM while no expression was found in blood vessels and normal controls (23). The expression of BAFF or APRIL in muscle cells in IBM and PM had not been reported so far, but the expression of their receptors had been analyzed by immunohistochemistry: BAFF-R, BCMA, and TACI were detected on mononuclear cells in the muscle tissue of patients with myositis (26). The highest rate of these receptors was found in IBM in five out of six analyzed patients, followed by PM (five out of 11) and DM (two out of 6). CXCL-12 was observed in macrophages (CD68+) and T cells (CD4+) invading muscle fibers (27) as well as in muscle fibers in IBM and PM (28). The protein amount of CXCL-12 evidenced by western blot was significantly increased in IBM and PM compared to healthy controls with a tendency for a higher expression in IBM (27); CXCL-13 localized to lymphoid follicle-like structures in juvenile DM and was absent in healthy muscles (24). In DM, CXCL-12 was shown to be strongly upregulated in affected blood vessels.
In DM, muscle fibers were positive for BAFF (23), and in IBM and PM, they stained positive for CXCL-12 (28). Our present data clearly demonstrate a substantial protein expression of BAFF in IBM and PM, which had not been reported before. In the present study, we demonstrated that muscle cells derived from non-myopathic subjects in vitro start to produce BAFF and CXCL-12 under proinflammatory conditions. This would be a substantial prerequisite for the induction of skeletal muscle inflammation in vivo. Muscle cells are known to be active participants rather than passive targets of an immune response (11) and, thus, exert a plethora of immune mediators (29). In vivo muscle cells have been shown to be capable of secreting proinflammatory cytokines such as IL-1α, IL-1β, TNFα, IL-6, IL-2, and IFN-γ and chemokines such as CXCL-12, and C-C motif ligand 2 (CCL2).
BAFF is known to be induced by the stimulation of IFN-α and IFN-γ (30) in salivary gland epithelial cells and in intestinal epithelial cells (31). The transcriptional up-regulation of the gene CXCL-12 can be induced in human and rat cell lines by inflammatory stimuli such as interleukin-1 and interleukin-6 (32). Our cell culture data are well in line with these findings as we were able to show a significant upregulation of BAFF and CXCL-12 mRNA in myotubes from non-myopathic individuals upon exposure to IFN-γ and IL-1β. Satellite-cell-derived myoblasts in rats express CXCL-12 protein levels with the highest amount when mature myotubes are formed (33). The staining characteristics are similar to our study with human myotubes. The finding of CXCL-12 expressed by normal muscle cells has not been reported for humans so far.
Taken together, our data demonstrate unique patterns of B cell regulatory factors in IBM and PM. Besides the known roles of T cells and macrophages in both diseases, a crucial role in the composition and regulation of muscle inflammation appears to be controlled by an interplay of muscle fibers and B cells through B cell signaling factors.
The regulation of BAFF and APRIL in autoimmune disorders of tissues other than skeletal muscle has been shown by different groups: In Lupus with the affection of kidneys and the central nervous system, serum BAFF has been shown to be elevated and serum APRIL was decreased (34, 35). The analysis of cerebrospinal fluid (CSF) from patients with inflammatory brain disorders like multiple sclerosis and Lyme neuroborreliosis revealed significantly increased levels of BAFF, CXCL-12, and CXCL-13 compared to patients with non-inflammatory neurological diseases (36), and BAFF protein levels were upregulated in patients with neuro-Behçet’s disease (37). In other B-cell-mediated disorders like rheumatoid arthritis, the levels of APRIL and BAFF were found to be elevated in serum and synovial fluid and both levels correlated with disease activity (38–40). Tabalumab, an anti-BAFF monoclonal antibody, showed efficacy and safety in rheumatoid arthritis in phase two studies but did not meet the primary or secondary outcomes in a phase three study (41), and Belimumab, a biologic BAFF inhibitor, has been the first biologic agent licensed for Lupus therapy (35).
Our findings on muscle specimens from patients with myositis are comparable with inflammatory central nervous system disorders in that increased levels of BAFF, CXCL-12, and CXCL-13 are observed. By contrast, APRIL mRNA was elevated in the skeletal muscle of IBM and PM but did not seem to be operative in inflammatory central nervous system disorders.
An explanation for increased levels of CXCL-12 in myositis as well as in muscular dystrophies could be its known role during muscle regeneration: Research has shown that injecting CXCL-12 into a damaged muscle led to an increase of muscle weight and improved muscle histology with a decreased level of fibrosis (33). The upregulation of CXCL-12 may be an attempt of the muscle fibers to improve muscular regeneration. Moreover, upregulation of CXCL-12 and its receptor CXCR4 was noted in the gastrocnemius muscle of rats after repeated periods of daily running performance compared to non-running controls (42). This can be interpreted as the involvement of CXCL-12 in adaption to muscle exercise. These findings reflect the pleiotropic role of chemokines like CXCL-12 in different pathomechanisms of the muscle and it underscores its relevance to distinct functions in the muscle. Within the inflammatory milieu of myositis, chemokines can activate unique immunomodulatory pathways including B-cell activation and support muscle regeneration with CXCL-12.
Collectively, in inflammatory myopathies, we demonstrate the muscular presence of molecular factors that are crucial for B cell survival, maturation, activation, and differentiation (BAFF) (12, 13) as well as for B-cell chemotaxis (14) (CXCL-12 and 13). These factors together provide a prerequisite for sustained B-cell involvement in the pathogenesis of IBM and PM.
B-cell mechanisms and autoantibody production seem to play an important role in specific forms of myositis like IBM and PM and are thought to be less important in unspecific inflammatory settings like in muscular dystrophies. Our data emphasize the relevance of specific muscle inflammation in IBM and PM. This is of particular relevance in view of autoantibodies like cN1A and supports the rationale for therapeutic efforts to modulate the humoral (auto-) immunity by drugs such as immunoglobulins or B cell depletion by rituximab.
The main limitation of our study is the relatively small number of patients, which is due to the rare nature of the disease subsets studied. Although the number of muscle biopsies was clearly sufficient for a meaningful statistical analysis, the data cannot be generalized without confirmatory studies.
Our data provide novel insight into the complex pathophysiology of myositis and the role of B cells. Beyond the techniques used in the present study, it will be of interest in the future to design complementary approaches including: i) further analysis of mRNA regulation by an epigenetic approach, ii) secreted protein levels of cytokines and chemokines in muscle cell culture by ELISA and Western blot, and iii) intracellular metabolism including (immune)-proteasome by a metabolomic approach.
The raw data supporting the conclusions of this article will be made available by the authors, without undue reservation.
The studies involving human participants were reviewed and approved by the University Medical Center Göttingen, Germany. The patients/participants provided their written informed consent to participate in this study.
P-OC performed parts of the experiments, analyzed the data, and wrote the article. LM performed most of the experiments. MW was responsible for the surgical preparation of skeletal muscle for expansion of progenitors (satellite cells). AW, AB, SK, SZe and SZi contributed muscle biopsy specimens and revised the manuscript for intellectual content. SZe contributed clinal and pathological data of patients and revised the manuscript. JS conceived the idea, conceptualized and supervised all experiments, and revised the manuscript. All authors contributed to the article and approved the submitted version.
The study was supported in part by the AFM (Association Française contre les Myopathies, grant no. 20987) and by intramural funds from the University Medical Center Göttingen (Forschungsförderprogramm). The Open Access Publication Funds of the Göttingen University is gratefully acknowledged. The funder was not involved in the study design, collection, analysis, interpretation of data, writing of this article, or the decision to submit it for publication.
We thank all patients for their voluntary contribution to this study. Technical assistance by Fatima Agdas and Iris Iben is gratefully acknowledged. JS, SZe and SK are members of the European Reference Network (ERN) for Rare Neuromuscular Disorders (EURO-NMD).
P-OC received reimbursement for travel from Biotest. SZi has received speaker’s honoraria from Sanofi/Genzyme and honoraria for advisory board participation from Hormosan. JS has received payments for advisory boards, speaker’s honoraria, travel expenses, and research projects from Abcuro, Alnylam, Argenx, Biotest, CSL Behring, Euroimmun, Janssen, Kezar, LFB, Novartis, Octapharma, UCB.
The remaining authors declare that the research was conducted in the absence of any commercial or financial relationships that could be construed as a potential conflict of interest.
All claims expressed in this article are solely those of the authors and do not necessarily represent those of their affiliated organizations, or those of the publisher, the editors and the reviewers. Any product that may be evaluated in this article, or claim that may be made by its manufacturer, is not guaranteed or endorsed by the publisher.
1. Piper CJM, Wilkinson MGL, Deakin CT, Otto GW, Dowle S, Duurland CL, et al. CD19+CD24hiCD38hi B cells are expanded in juvenile dermatomyositis and exhibit a pro-inflammatory phenotype after activation through toll-like receptor 7 and interferon-α. Front Immunol (2018) 9:1372. doi: 10.3389/fimmu.2018.01372
2. Young-Glazer J, Cisneros A, Wilfong EM, Smith SA, Crofford LJ, Bonami RH. Jo-1 autoantigen-specific B cells are skewed towards distinct functional b cell subsets in anti-synthetase syndrome patients. Arthritis Res Ther (2021) 23(1):33. doi: 10.1186/s13075-020-02412-8
3. Schmidt J. Current classification and management of inflammatory myopathies. J Neuromuscul Dis (2018) 5(2):109–29. doi: 10.3233/JND-180308
4. Greenberg SA, Bradshaw EM, Pinkus JL, Pinkus GS, Burleson T, Due B, et al. Plasma cells in muscle in inclusion body myositis and polymyositis. Neurology (2005) 65:1782–7. doi: 10.1212/01.wnl.0000187124.92826.20
5. Bradshaw EM, Orihuela A, McArdel SL, Salajegheh M, Amato AA, Hafler DA, et al. A local antigen-driven humoral response is present in the inflammatory myopathies. J Immunol (2007) 178:547–56. doi: 10.4049/jimmunol.178.1.547
6. Salajegheh M, Pinkus JL, Amato AA, Morehouse C, Jallal B, Yao Y, et al. Permissive environment for B-cell maturation in myositis muscle in the absence of B-cell follicles. Muscle Nerve (2010) 42:576–83. doi: 10.1002/mus.21739
7. McIntyre D, Zuckerman NS, Field M, Mehr R, Stott DI. The VH repertoire and clonal diversification of B cells in inflammatory myopathies. Eur J Immunol (2014) 44:585–96. doi: 10.1002/eji.201343315
8. Ray A, Amato AA, Bradshaw EM, Felice KJ, DiCapua DB, Goldstein JM, et al. Autoantibodies produced at the site of tissue damage provide evidence of humoral autoimmunity in inclusion body myositis. PloS One (2012) 7:e46709. doi: 10.1371/journal.pone.0046709
9. Benjamin Larman H, Salajegheh M, Nazareno R, Lam T, Sauld J, Steen H, et al. Cytosolic 5′-nucleotidase 1A autoimmunity in sporadic inclusion body myositis. Ann Neurol (2013) 73:408–18. doi: 10.1002/ana.23840
10. Pluk H, Van Hoeve BJA, Van Dooren SHJ, Stammen-Vogelzangs J, van der Heijden A, Schelhaas HJ, et al. Autoantibodies to cytosolic 5′-nucleotidase 1A in inclusion body myositis. Ann Neurol (2013) 73:397–407. doi: 10.1002/ana.23822
11. Wiendl H, Hohlfeld R, Kieseier BC. Immunobiology of muscle: advances in understanding an immunological microenvironment. Trends Immunol (2005) 26:373–80. doi: 10.1016/j.it.2005.05.003
12. Mackay F, Schneider P. Cracking the BAFF code. Nat Rev Immunol (2009) 9:491–502. doi: 10.1038/nri2572
13. Ferrer G, Bosch R, Hodgson K, Tejero R, Roué G, Colomer D, et al. B cell activation through CD40 and IL4R ligation modulates the response of chronic lymphocytic leukaemia cells to BAFF and APRIL. Br J Haematol (2014) 164:570–8. doi: 10.1111/bjh.12645
14. Roy MP, Kim CH, Butcher EC. Cytokine control of memory b cell homing machinery. J Immunol (2002) 169:1676–82. doi: 10.4049/jimmunol.169.4.1676
15. Schmidt J, Barthel K, Wrede A, Salajegheh M, Bahr M, Dalakas MC. Interrelation of inflammation and APP in sIBM: IL-1 beta induces accumulation of beta-amyloid in skeletal muscle. Brain (2008) 131:1228–40. doi: 10.1093/brain/awn053
16. Zschüntzsch J, Voss J, Creus K, Sehmisch S, Raju R, Dalakas MC, et al. Provision of an explanation for the inefficacy of immunotherapy in sporadic inclusion body myositis: quantitative assessment of inflammation and β-amyloid in the muscle. Arthritis Rheum (2012) 64:4094–103. doi: 10.1002/art.37692
17. Szodoray P, Alex P, Knowlton N, Centola M, Dozmorov I, Csipo I, et al. Idiopathic inflammatory myopathies, signified by distinctive peripheral cytokines, chemokines and the TNF family members B-cell activating factor and a proliferation inducing ligand. Rheumatol (Oxf) (2010) 49:1867–77. doi: 10.1093/rheumatology/keq151
18. Lopez De Padilla CM, McNallan KT, Crowson CS, Bilgic H, Bram RJ, Hein MS, et al. BAFF expression correlates with idiopathic inflammatory myopathy disease activity measures and autoantibodies. J Rheumatol (2013) 40:294–302. doi: 10.3899/jrheum.120555
19. Peng QL, Shu XM, Wang DX, Wang Y, Lu X, Wang GC. B-cell activating factor as a serological biomarker for polymyositis and dermatomyositis. biomark Med (2014) 8:395–403. doi: 10.2217/bmm.13.124
20. Gupta L, Chaurasia S, Srivastava P, Dwivedi S, Lawrence A, Misra R. Serum BAFF in Indian patients with IIM: a retrospective study reveals novel clinico-phenotypic associations in children and adults. Clin Rheumatol (2018) 37:1265–71. doi: 10.1007/s10067-018-4046-x
21. Kim JY, Yang Y, Moon J-S, Lee EY, So SH, Lee H-S, et al. Serum BAFF expression in patients with myasthenia gravis. J Neuroimmunol (2008) 199:151–4. doi: 10.1016/j.jneuroim.2008.05.010
22. Krystufkova O, Vallerskog T, Helmers SB, Mann H, Putova I, Belacek J, et al. Increased serum levels of b cell activating factor (BAFF) in subsets of patients with idiopathic inflammatory myopathies. Ann Rheum Dis (2009) 68:836–43. doi: 10.1136/ard.2008.091405
23. Baek A, Park HJ, Na SJ, Shim DS, Moon JS, Yang Y, et al. The expression of BAFF in the muscles of patients with dermatomyositis. J Neuroimmunol (2012) 249:96–100. doi: 10.1016/j.jneuroim.2012.04.006
24. De Paepe B, Creus KK, De Bleecker JL. Role of cytokines and chemokines in idiopathic inflammatory myopathies. Curr Opin Rheumatol (2009) 21:610–6. doi: 10.1097/BOR.0b013e3283317b31
25. Wang Y, Zheng Y, Zhao Y, Liu Y, Zhang W, Yu M, et al. Comparison of cytokine/chemokine profiles between dermatomyositis and anti-synthetase syndrome. Front Neurol (2022) 13:1042580. doi: 10.3389/fneur.2022.1042580
26. Kryštůfková O, Barbasso Helmers S, Venalis P, Malmström V, Lindroos E, Vencovský J, et al. Expression of BAFF receptors in muscle tissue of myositis patients with anti-Jo-1 or anti-Ro52/anti-Ro60 autoantibodies. Arthritis Res Ther (2014) 16:454. doi: 10.1186/s13075-014-0454-8
27. De Paepe B, De Bleecker JL. The nonnecrotic invaded muscle fibers of polymyositis and sporadic inclusion body myositis: on the interplay of chemokines and stress proteins. Neurosci Lett (2013) 535:18–23. doi: 10.1016/j.neulet.2012.11.064
28. De Paepe B, Schroder JM, Martin J-J, Racz GZ, De Bleecker JL. Localization of the alpha-chemokine SDF-1 and its receptor CXCR4 in idiopathic inflammatory myopathies. Neuromuscul Disord (2004) 14:265–73. doi: 10.1016/j.nmd.2004.01.001
29. Pedersen BK. Muscle as a secretory organ. Compr Physiol (2013) 3:1337–62. doi: 10.1002/cphy.c120033
30. Ittah M, Miceli-Richard C, Eric Gottenberg J-, Lavie F, Lazure T, Ba N, et al. B cell-activating factor of the tumor necrosis factor family (BAFF) is expressed under stimulation by interferon in salivary gland epithelial cells in primary sjögren’s syndrome. Arthritis Res Ther (2006) 8:R51. doi: 10.1186/ar1912
31. Woo S-J, Im J, Jeon JH, Kang S-S, Lee M-H, Yun C-H, et al. Induction of BAFF expression by IFN-gamma via JAK/STAT signaling pathways in human intestinal epithelial cells. J Leukoc Biol (2013) 93:363–8. doi: 10.1189/jlb.0412210
32. Calonge E, Alonso-Lobo JM, Escandon C, Gonzalez N, Bermejo M, Santiago B, et al. c/EBPbeta is a major regulatory element driving transcriptional activation of the CXCL12 promoter. J Mol Biol (2010) 396:463–72. doi: 10.1016/j.jmb.2009.11.064
33. Brzoska E, Kowalewska M, Markowska-Zagrajek A, Kowalski K, Archacka K, Zimowska M, et al. Sdf-1 (CXCL12) improves skeletal muscle regeneration via the mobilisation of Cxcr4 and CD34 expressing cells. Biol Cell (2012) 104:722–37. doi: 10.1111/boc.201200022
34. Vincent FB, Northcott M, Hoi A, Mackay F, Morand EF. Association of serum b cell activating factor from the tumour necrosis factor family (BAFF) and a proliferation-inducing ligand (APRIL) with central nervous system and renal disease in systemic lupus erythematosus. Lupus (2013) 22:873–84. doi: 10.1177/0961203313496302
35. Möckel T, Basta F, Weinmann-Menke J, Schwarting A. B cell activating factor (BAFF): structure, functions, autoimmunity and clinical implications in systemic lupus erythematosus (SLE). Autoimmun Rev (2021) 20(2):102736. doi: 10.1016/j.autrev.2020.102736
36. Kowarik MC, Cepok S, Sellner J, Grummel V, Weber MS, Korn T, et al. CXCL13 is the major determinant for b cell recruitment to the CSF during neuroinflammation. J Neuroinflamm (2012) 9:93. doi: 10.1186/1742-2094-9-93
37. Hamzaoui K, Houman H, Hentati F, Hamzaoui A. BAFF is up-regulated in central nervous system of neuro-behcet’s disease. J Neuroimmunol (2008) 200:111–4. doi: 10.1016/j.jneuroim.2008.06.006
38. Wei F, Chang Y, Wei W. The role of BAFF in the progression of rheumatoid arthritis. Cytokine (2015) 76:537–44. doi: 10.1016/j.cyto.2015.07.014
39. Weldon AJ, Moldovan I, Cabling MG, Hernandez EA, Hsu S, Gonzalez J, et al. Surface APRIL is elevated on myeloid cells and is associated with disease activity in patients with rheumatoid arthritis. J Rheumatol (2015) 42:749–59. doi: 10.3899/jrheum.140630
40. Zhang Ll, Xiao H, Zhang F, Wu Yj, Shu Jl, Li Y, et al. BAFF, involved in b cell activation through the NF-κB pathway, is related to disease activity and bone destruction in rheumatoid arthritis. Acta Pharmacol Sin (2021) 42(10):1665–75. doi: 10.1038/s41401-020-00582-4
41. Schiff M, Combe B, Dorner T, Kremer JM, Huizinga TW, Veenhuizen M, et al. Efficacy and safety of tabalumab, an anti-BAFF monoclonal antibody, in patients with moderate-to-severe rheumatoid arthritis and inadequate response to TNF inhibitors: results of a randomised, double-blind, placebo-controlled, phase 3 study. RMD Open (2015) 1:e000037. doi: 10.1136/rmdopen-2014-000037
Keywords: autoimmune diseases, neuromuscular disease, inflammatory muscle disease, myositis, B cells
Citation: Carstens P-O, Müllar LM, Wrede A, Zechel S, Wachowski MM, Brandis A, Krause S, Zierz S and Schmidt J (2023) Skeletal muscle fibers produce B-cell stimulatory factors in chronic myositis. Front. Immunol. 14:1177721. doi: 10.3389/fimmu.2023.1177721
Received: 01 March 2023; Accepted: 16 June 2023;
Published: 05 September 2023.
Edited by:
Antonios Bayas, Augsburg University Hospital, GermanyReviewed by:
Markus Christian Kowarik, University of Tübingen, GermanyCopyright © 2023 Carstens, Müllar, Wrede, Zechel, Wachowski, Brandis, Krause, Zierz and Schmidt. This is an open-access article distributed under the terms of the Creative Commons Attribution License (CC BY). The use, distribution or reproduction in other forums is permitted, provided the original author(s) and the copyright owner(s) are credited and that the original publication in this journal is cited, in accordance with accepted academic practice. No use, distribution or reproduction is permitted which does not comply with these terms.
*Correspondence: Jens Schmidt, ai5zY2htaWR0QGdteC5vcmc=
Disclaimer: All claims expressed in this article are solely those of the authors and do not necessarily represent those of their affiliated organizations, or those of the publisher, the editors and the reviewers. Any product that may be evaluated in this article or claim that may be made by its manufacturer is not guaranteed or endorsed by the publisher.
Research integrity at Frontiers
Learn more about the work of our research integrity team to safeguard the quality of each article we publish.