- 1State Key Laboratory of Freshwater Ecology and Biotechnology, Institute of Hydrobiology, Chinese Academy of Sciences, Wuhan, China
- 2College of Advanced Agricultural Sciences, University of Chinese Academy of Sciences, Beijing, China
- 3The Innovative Academy of Seed Design, Chinese Academy of Sciences, Beijing, China
Introduction: Resveratrol (RES) is a polyphenol organic compound with antioxidant and anti-inflammatory properties. This study aimed to determine whether and how RES can alleviate liver injury in lipopolysaccharide (LPS)-induced gibel carp.
Methods: Gibel carp were fed a diet with or without RES and were cultured for 8 weeks, followed by LPS injection.
Results and discussion: The results suggested that RES attenuated the resulting oxidative stress and inflammation by activating the Nrf2/Keap1 pathway and inhibiting the NF-κB pathway, as confirmed by changes in oxidative stress, inflammation-related gene expression, and antioxidant enzyme activity. Furthermore, RES cleared damaged mitochondria and enhanced mitochondrial biogenesis to mitigate reactive oxygen species (ROS) accumulation by upregulating the SIRT1/PGC-1α and PINK1/Parkin pathways and reducing p62 expression. Overall, RES alleviated LPS-induced oxidative stress and inflammation in gibel carp through mitochondria-related mechanisms.
1 Introduction
Aquaculture makes a major contribution to human nutrition by providing a large proportion of high-quality animal protein for consumption (1). Thus, the safety of aquatic products is vital for human wellbeing. However, the deterioration of the aquaculture environment can cause oxidative stress, inflammatory reactions, and tissue damage in fish, then affecting the safety of aquatic products (2). Lipopolysaccharide (LPS), a main component of the outer membrane of Gram-negative bacteria, is composed of lipids and polysaccharides, has been found to be responsible for the pathogenicity of several bacterial diseases in fish (3). As in mammals, LPS injection in fish induces nuclear factor-kappa B (NF-κB) to disengage from inhibitor of NF-κB alpha (IκBα) and to be translocated to the nucleus, where it enhances the expression of proinflammatory cytokines including interleukin 1β (IL-1β) and tumor necrosis factor alpha (TNF-α), consequently leading to inflammation (4). Concurrently, a burst of reactive oxygen species (ROS) breaks the balance with the antioxidant defense system and impels the nuclear factor erythroid 2-related factor (Nrf2) that was originally bound to Kelch-like ECH-related protein 1 (Keap1) to dissociate and bind to the antioxidant response element (ARE) in the nucleus (5). Then, transcripts of downstream genes including heme oxygenase-1 (HO-1), NAD(P)H: quinone oxidoreductase 1 (NQO1), glutathione S-transferase (GST), and glutathione peroxidase (GPx) are activated (6, 7). Similarly, it has been reported that LPS induces oxidative stress, inflammation, and apoptosis in zebrafish (8), carp (9), and yellow catfish (10).
Mitochondria act as the main site of ROS generation, and they possess a variety of quality control mechanisms to maintain their functioning and homeostasis. Evidence has shown that after LPS injection, silent information regulator 1 (SIRT1) relies on NAD+ to mediate the deacetylation of Nrf2 and then specifically binds to the ARE located in the p62 promoter (11). P62 and oxidized proteins are transmitted to autophagosomes for degradation, and thus, the balance of ROS in the animal can be maintained (12). However, mitochondrial dysfunction may occur when excessive ROS exist via triggering the forkhead box protein O3 (FOXO3) signaling pathway and upregulating the expression of autophagy-related genes such as microtubule-associated protein 1A/1B-light chain 3 (LC3). The autophagy core protein LC3 is converted from LC3 I to LC3 II when autophagy is activated, and the latter acts as a structural protein of autophagosomes associated with the clearance of damaged mitochondria (13, 14). Normally, mitophagy is a protective mechanism against mild and moderate stress. However, over-activated mitophagy could induce cell death. For mitochondrial homeostasis, SIRT1 mediates the activation of nuclear respiratory factors 1 and 2 (NRF1 and NRF2) by peroxisome proliferator-activated receptor (PPAR) γ co-activator 1α (PGC-1α) and mitochondrial transcription factor A (TFAM) to induce mitochondrial biogenesis (15–17). Subsequently, mtDNA increases and ROS production decreases. However, the occurrence of LPS-induced mitophagy and mitochondrial biogenesis in fish has not been fully described.
At present, Chinese herbs are being increasingly used to prevent and cure diseases in aquaculture species by promoting immune function (18, 19). Resveratrol (RES), as a typical activator of SIRT1, is a non-flavonoid polyphenol organic compound derived from red grapes and other foods that can reduce the ROS and mitochondrial superoxide production caused by high levels of glucose (20). RES can decrease lipid oxidative damage in southern flounder (Paralichthys lethostigma) (21). In turbot (Scophthalmus maximus), RES alleviates oxidative stress and inflammatory reactions induced by soybean meal (22). Similarly, RES defenses liver damage and metabolic imbalances in common carp (Cyprinus carpio) (23). Thus, RES can reduce oxidative damage and enhance immunity in fish. In addition, RES can promote mitophagy and attenuate oxidative stress-induced tissue damage by regulating mitochondrial redox homeostasis and function via the SIRT1/PGC-1α signaling pathway (24). Gibel carp (Carassius gibelio) is an economically important freshwater fish in China, with the qualities of rapid growth and strong disease resistance. In the previous study, we found that RES could attenuate oxidative stress, inflammation, and mitochondrial dysfunction in gibel carp under acute ammonia exposure (25). Thus, we hypothesized that RES could attenuate LPS-induced liver injury in gibel carp by alleviating oxidative stress and the inflammatory reaction and by regulating mitophagy and mitochondrial biogenesis. To verify our hypothesis, fish were fed a diet with or without RES and were cultured for 8 weeks and then subjected to LPS injection. The biochemical indexes, histological changes, and gene and protein expression levels of the fish were then analyzed. Our study elucidates effects of RES on oxidative stress and inflammation in LPS-induced gibel carp for the first time, and provides an effective strategy to mitigate LPS-induced liver damage in fish.
2 Materials and methods
2.1 Experimental animals
Gibel carp were from the Institute of Hydrobiology, Chinese Academy of Sciences (Wuhan, Hubei, China). The experimental procedures on animals were approved by the ethics committee of the Institute of Hydrobiology, Chinese Academy of Sciences (Approval ID: IHB20140724).
2.2 Experimental protocol
Two isonitrogenous (36% crude protein) isoenergetic (7% crude lipid) diets supplemented with or without 500 mg/kg RES (≥ 98%; Solarbio, Beijing, China) were formulated for the experiment as described previously (25). The diet ingredients and chemical composition are shown in Table 1. Before the formal experiment, fish were temporarily raised in a circular aquaculture system for acclimation. Then, 180 healthy individuals initially weighing an average of 6.66 ± 0.01g were randomly assigned among six tanks (150 L water volume, 67 cm × 52 cm × 46 cm) and fed with a CON or RES diet three times per day (8:30, 13:30, and 18:30) for 8 weeks. The water temperature was maintained at 29–31°C; total ammonia nitrogen was < 0.1 mg/L; the dissolved oxygen was > 7.0 mg/L, and the photoperiod was 12L:12D.
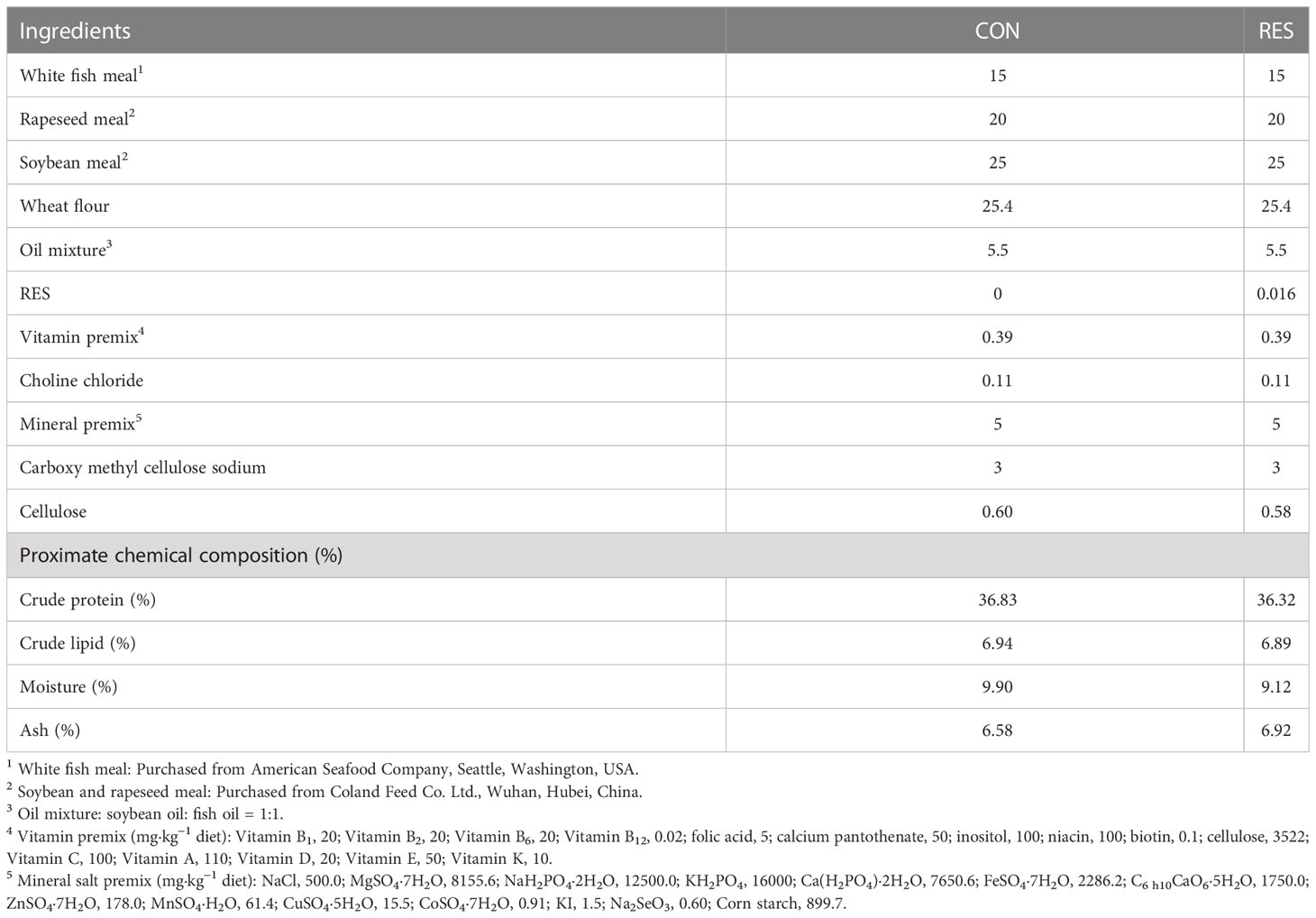
Table 1 Formulation and approximate composition (% dry matter) of practical diets supplemented with resveratrol (RES).
After the feeding experiment, gibel carp were fasted for 24 h. The fish were lightly anaesthetized with MS-222 at a concentration of 60 mg/L and then weighed. Then, they were administered an intraperitoneal injection of 5 μL/g body mass LPS (5.0 mg/mL, Sigma, St. Louis, Mo., USA) and placed into triplicate tanks per treatment (26). Two fish per tank were anaesthetized and sampled at 0, 6, and 12 h after injection (n = 6 at each sampling time).
2.3 Sample collection
Blood was drawn from a vein using syringes rinsed with heparin sodium (0.2%) at 0, 6, and 12 h after LPS injection. The blood samples of six fish per tank were centrifuged at 3000 rpm for 15 min at 4°C to obtain the plasma, and the plasma was kept at −80°C. Parts of the liver were fixed in paraformaldehyde (4%) for histopathological evaluation or in 2.5% glutaraldehyde solution for ultrastructural observation. The remaining portions of the samples were frozen in liquid nitrogen and kept at −80°C.
2.4 Biochemical analysis
The levels of alanine aminotransferase (ALT, C009-2-1), aspartate aminotransferase (AST, C010-2-1), and lactate dehydrogenase (LDH, A020-2-2) in the serum, the activity of superoxide dismutase (SOD, A001-3-2), and the content of antioxidant capacity (T-AOC, A015-2-1), malondialdehyde (MDA, A003-1-2), lipid hydroperoxide (LPO, A106-1-3), and reactive oxygen species (ROS, 69-86537) in the liver were determined using commercial kits (Nanjing Jiancheng Bioengineering Institute, China). Complement C3 (C3, H186-1-2) and Complement C4 (C4, H186-2-2) were measured with ELISA kits (Nanjing Jiancheng Bioengineering Institute, China).
2.5 Histopathology
Hematoxylin and eosin (H&E) staining was carried out to evaluate liver injury. The paraffin-embedded liver tissues were cut into sections and stained with H&E, and the stained liver sections were examined under a microscope. We also examined hepatocyte apoptosis in the liver by terminal deoxynucleotidyl transferase-mediated dUTP nick-end labeling (TUNEL) and the ultrastructure of the liver via transmission electron microscopy (TEM), as described in a previous study (27).
2.6 Quantitative real-time polymerase chain reaction
The gene transcription levels related to oxidative stress, inflammation, mitochondrial dynamics, mitochondrial biogenesis, and mitophagy in liver samples from different treatment groups were analyzed. Total RNA was extracted with TRIzol reagent (Invitrogen, Carlsbad, USA) and then reverse transcribed to cDNA with M-MLV First-Strand Transcriptase (Invitrogen, Shanghai, China). Quantitative RT-PCR was performed on a LightCycle 480 II (Roche Diagnostics, Switzerland) instrument with SYBR Green Real-time PCR Master Mix (Roche Diagnostics, Switzerland). Relevant primers are listed in Table 2. Relative quantification of gene expression was performed as described by Pfaffl (28).
2.7 Western blot analysis
The weighed liver samples were homogenized in RIPA lysis buffer (Beyotime Biotechnology, Shanghai, China) and centrifuged to obtain the supernatant, and the protein concentration was measured using a protein analysis kit (Beyotime Biotechnology, Shanghai, China). Protein preparations (20 μg) were separated by electrophoresis using 6%, 8%, and 12% SDS-polyacrylamide gels (SDS-PAGE) and then transferred onto polyvinylidene fluoride (PVDF) membranes. The membranes were blocked with 5% skim milk in 0.1% Tween-20 (TBST) buffer for 1 h and further incubated with specific primary antibodies GAPDH (#5174, Cell signaling, Danvers, MA, USA), SIRT1 (A17307, Abclonal, Wuhan, China), PGC-1α (A12348, Abclonal, Wuhan, China), P62 (A11247, Abclonal, Wuhan, China), and LC3A/B-I/II (4108S, Cell signaling, Danvers, MA, USA). The membranes were then incubated with the secondary antibody Goat Anti-Rabbit IgG H&L (HRP) (1:5000, ab205718; Abcam), and each membrane was ultimately scanned using a CCD camera (Chemidoc MP Imaging System, BioRad) to evaluate the intensity of bands.
2.8 Immunofluorescence analysis
The liver samples were made into paraffin sections and dewaxed by successively soaking in xylene (two times, 15 min each time), absolute ethanol (two times, 5 min each time), 85% alcohol (5 min), and 75% alcohol (5 min). The sections were placed in citric acid repair solution (pH = 6.0) for antigen repair and then washed three times with PBS (pH = 7.4) for 5 min each time. Endogenous peroxidase was blocked with hydrogen peroxide solution (3%), and the serum was blocked with BSA (3%). Then, the sections were incubated with the first antibody to SIRT1, PGC-1α, P62, or LC3B overnight at 4°C. P62 and LC3B were subjected to co-localization analysis. After PBST cleaning, the sample was incubated at room temperature for 50 min with HRP-labeled goat anti rabbit (SeraCare, 1:400). TSA (Tyramine-488, 1/200) was added to the liver samples, and the nuclei were stained with DAPI. Finally, the sections were observed under a fluorescence microscope.
2.9 Statistical analysis
Statistical analyses within the same group were conducted using one-way ANOVA, and comparisons at the same time point were analyzed with independent sample t-tests using SPSS version 26.0 (SPSS Inc., Chicago, USA). Comparisons between experimental groups were considered to be significantly different at P < 0.05 and extremely significant at P < 0.01. Results were presented as mean ± SEM.
3 Results
3.1 Plasma metabolites
The levels of AST, ALT, and LDH in the plasma are shown in Figure 1. The AST, ALT, and LDH levels were significantly increased at both 6 h and 12 h after LPS injection. It is worth noting that RES significantly reduced the levels of AST, ALT, and LDH in the plasma, except for the AST level at 6 h after injection.
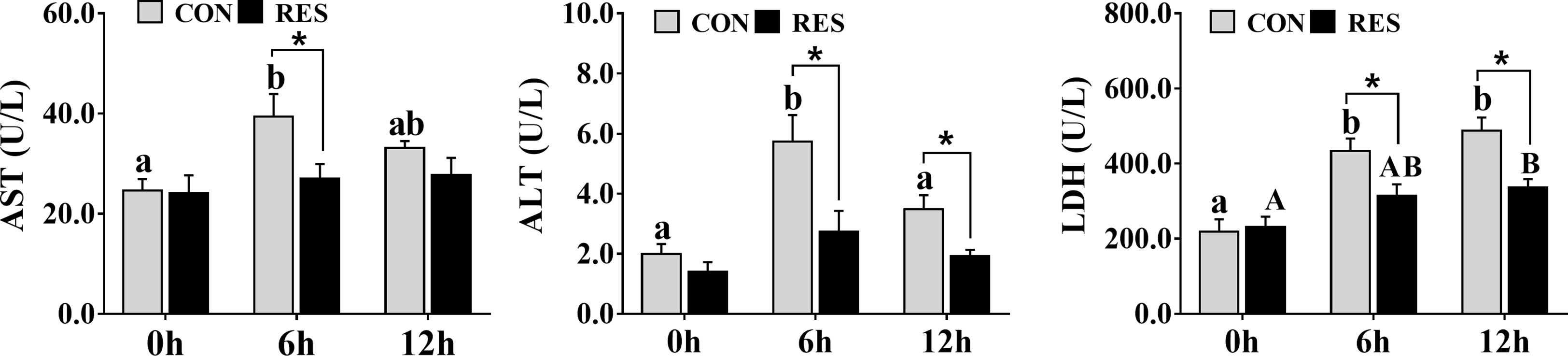
Figure 1 Effect of dietary RES on plasma AST, ALT, and LDH activities in gibel carp at 6 h and 12 h after LPS injection. Data are mean ± SEM (n = 6). The lowercase letters represent significant differences among diverse LPS durations in the CON group (P < 0.05); the uppercase letters represent significant differences among diverse LPS durations in the RES group (P < 0.05). * (P < 0.05) represent significant differences between the CON and RES groups.
3.2 Tissue injury in liver and hepatocyte apoptosis
As shown in Figure 2, the liver tissue structure of gibel carp in the CON group and RES group was basically normal. However, at 6 h and 12 h after LPS injection, the hepatocytes of the fish in the CON group showed abnormalities, with mild swelling of hepatocytes in the field of vision, vacuolation of cytoplasm, necrosis of some hepatocytes, and disappearance or fragmentation of some nuclei (Figure 2). Compared to the CON group, the liver injury of fish in the RES group was alleviated, being characterized by a complete hepatocyte structure, uniform size of hepatic sinuses, and no inflammatory cell infiltration.
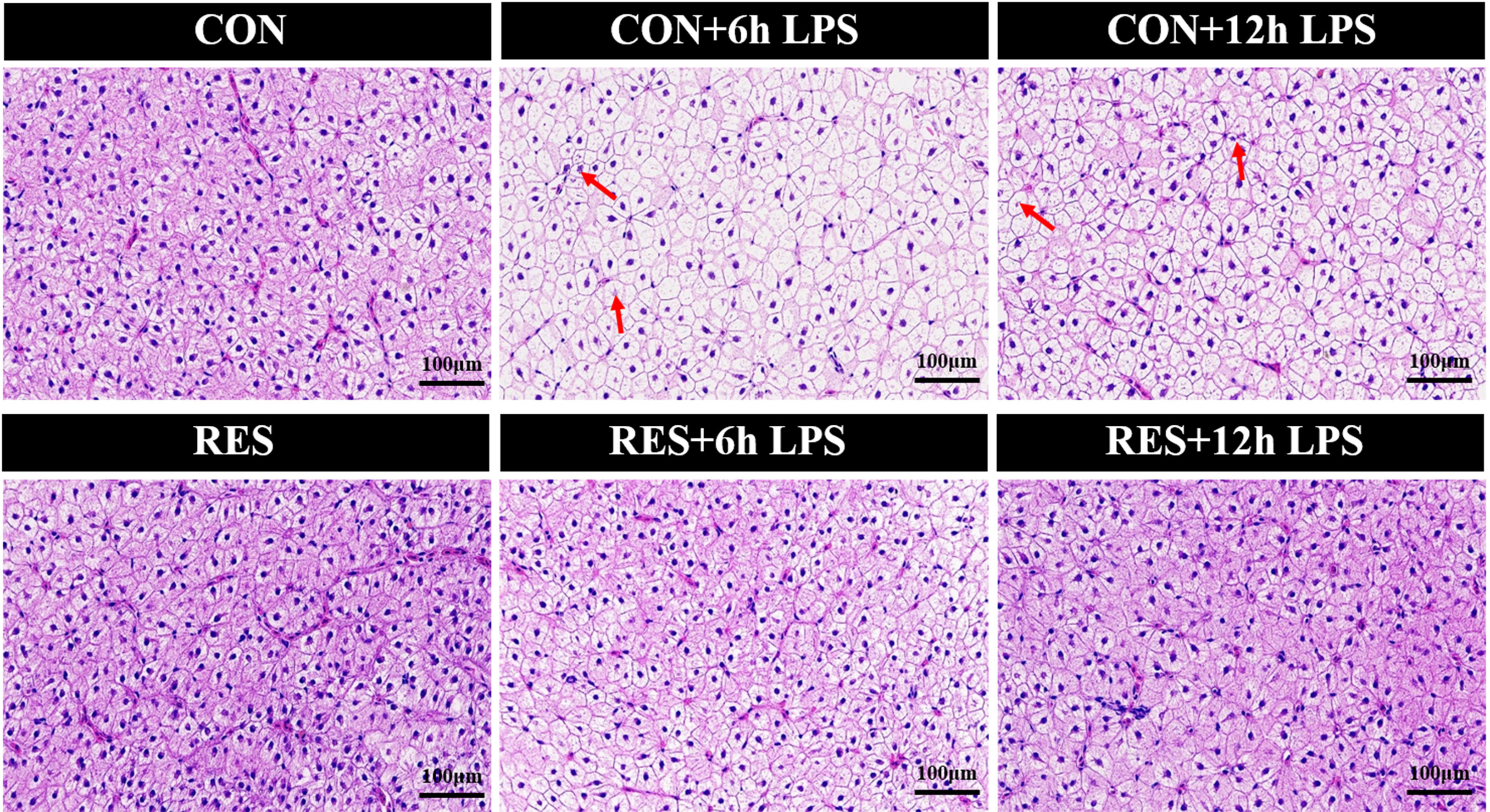
Figure 2 Effect of dietary RES on H&E staining of liver tissue in gibel carp at 6 h and 12 h after LPS injection.
In addition, the apoptosis signal of hepatocytes in gibel carp was significantly increased after LPS administration (Figure 3). Nevertheless, RES significantly reduced the rate of apoptosis in hepatocytes after LPS stimulation.
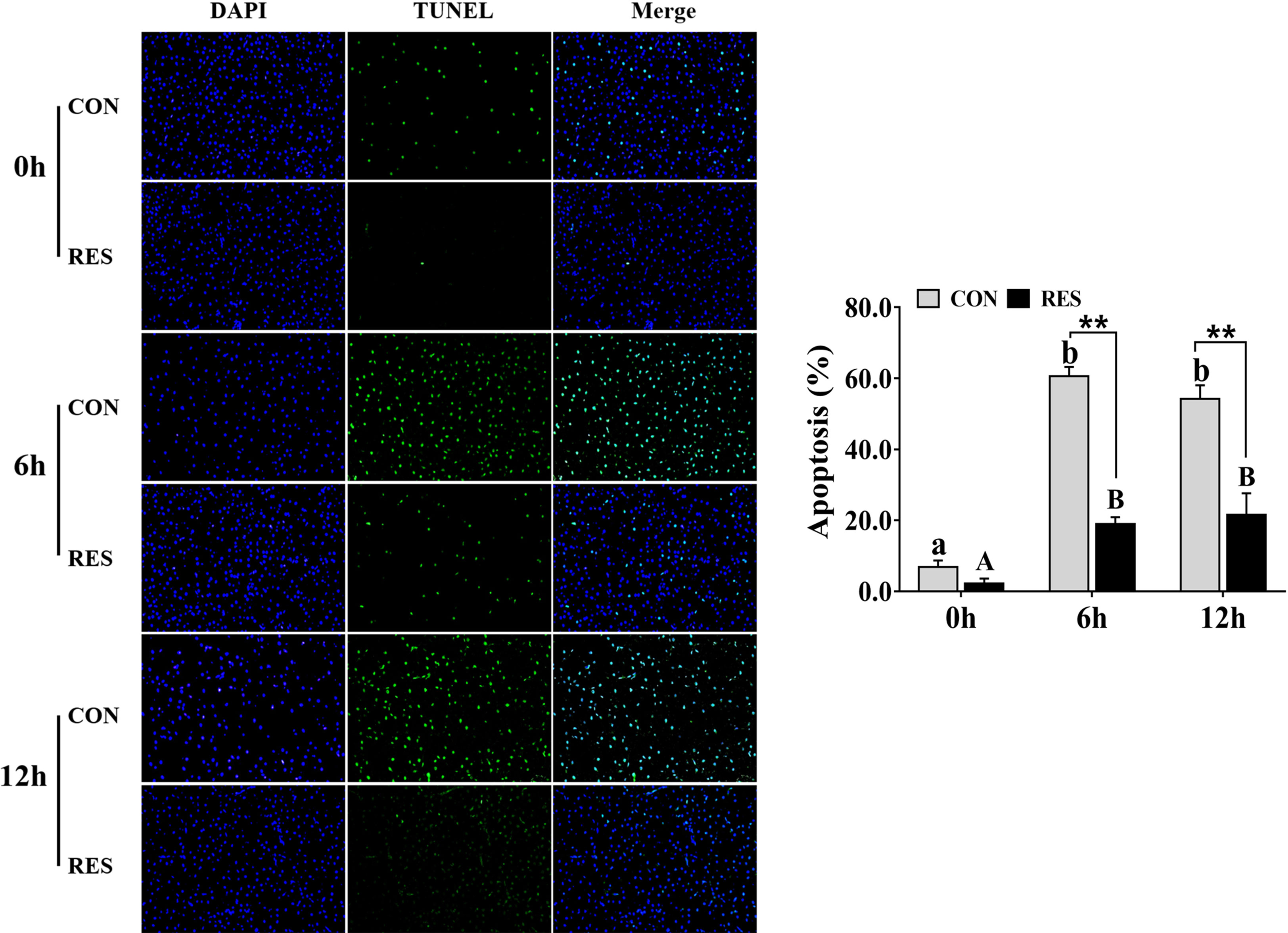
Figure 3 Effect of dietary RES on hepatocyte apoptosis in gibel carp at 6 h and 12 h after LPS injection. Data are mean ± SEM (n = 6). The lowercase letters represent significant differences among diverse LPS durations in the CON group (P < 0.05); the uppercase letters represent significant differences among diverse LPS durations in the RES group (P < 0.05). ** (P < 0.01) represents significant differences between the CON and RES groups.
3.3 Oxidative stress in the liver and expression of antioxidant-related genes in hepatocytes
As shown in Figure 4, SOD activity was increased at both 6 h and 12 h after LPS injection in the CON group, but T-AOC was decreased at 6 h. However, compared to the CON group, there was a significant increase in the RES group at 6 h after LPS injection. The MDA and LPO contents in the liver tissue of gibel carp of the CON and RES groups were increased significantly at 6 h and 12 h after LPS injection (Figure 4), and the difference was statistically significant between the CON and RES groups. At 6 h and 12 h, both MDA and LPO contents in the RES group were dramatically decreased. Similar changes were also found in ROS.
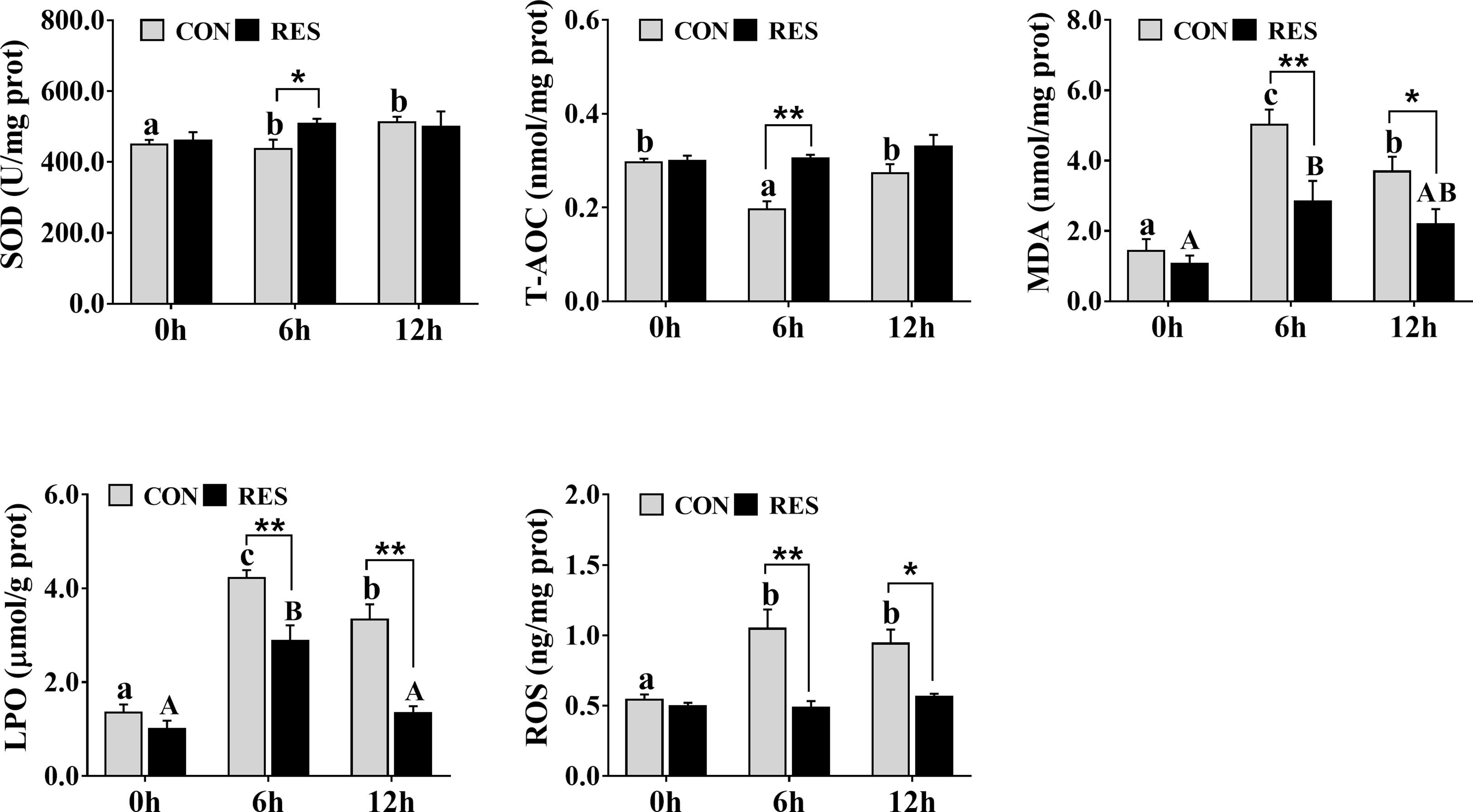
Figure 4 Effect of dietary RES on LPS-induced oxidative stress in the liver of gibel carp at 6 h and 12 h after LPS injection. Data are mean ± SEM (n = 6). The lowercase letters represent significant differences among diverse LPS durations in the CON group (P < 0.05); the uppercase letters represent significant differences among diverse LPS durations in the RES group (P < 0.05). *(P < 0.05) and **(P < 0.01) represent significant differences between the CON and RES groups.
The transcriptional levels of antioxidant-related genes in the liver of gibel carp at 0, 6, and 12 h after LPS injection are shown in Figure 5. At 6 h after LPS injection, the mRNA expression levels of nrf2, nqo-1, ho-1, and sod in the CON group were significantly decreased. At 6 h and 12 h after LPS injection, the transcript levels of nrf2 and gpx in the RES group were increased, while that of keap1 was decreased. Compared with the CON group, RES significantly upregulated the expression levels of nrf2, nqo-1, and ho-1 at 6 and 12 h after LPS injection and that of gpx and sod at 6 h after LPS injection. In contrast, keap1 was significantly downregulated in the RES group at 12 h after LPS injection. No significant differences in cat were observed.
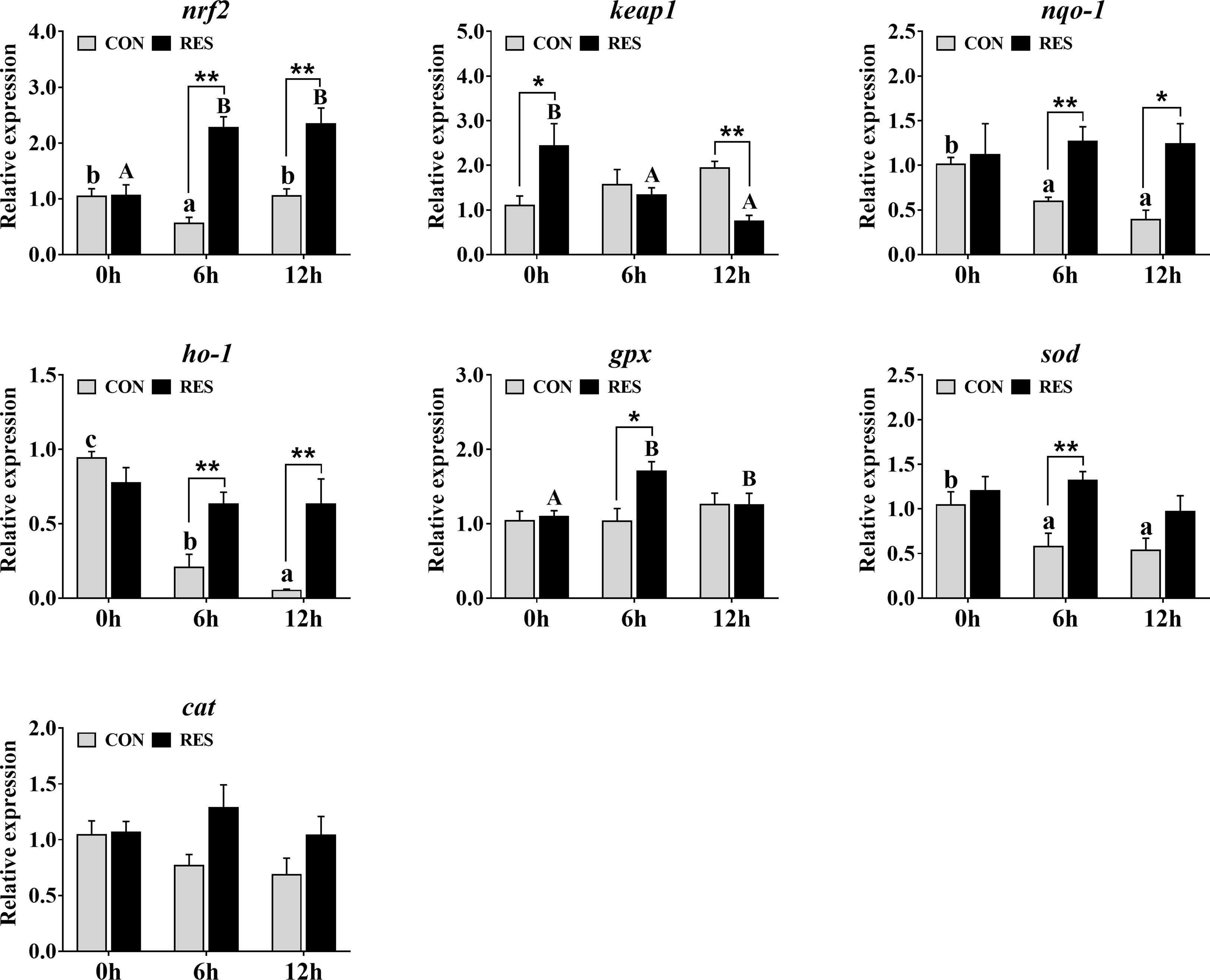
Figure 5 Effect of dietary RES on mRNA expression levels of antioxidant-related genes in the liver of gibel carp at 6 h and 12 h after LPS injection. The lowercase letters represent the significant differences among diverse LPS durations in the CON group (P < 0.05); the uppercase letters represent the significant differences among diverse LPS durations in the RES group (P < 0.05). *(P < 0.05) and **(P < 0.01) represent significant differences between the CON and RES groups. Nrf2: nuclear factor [erythroid-derived 2]-like 2; keap1: kelch-like ECH-associated protein-1; nqo-1, NADPH quinine oxidoreductase-1; ho-1, heme oxygenase-1; gpx, glutathione peroxidase; sod, superoxide dismutase; cat, catalase.
3.4 Immune response in plasma and the expression of inflammation-related genes in hepatocytes
As shown in Figure 6A, C4 was decreased in the plasma of gibel carp at 6 h and 12 h after LPS injection but was induced by RES. No significant difference was observed in C3.
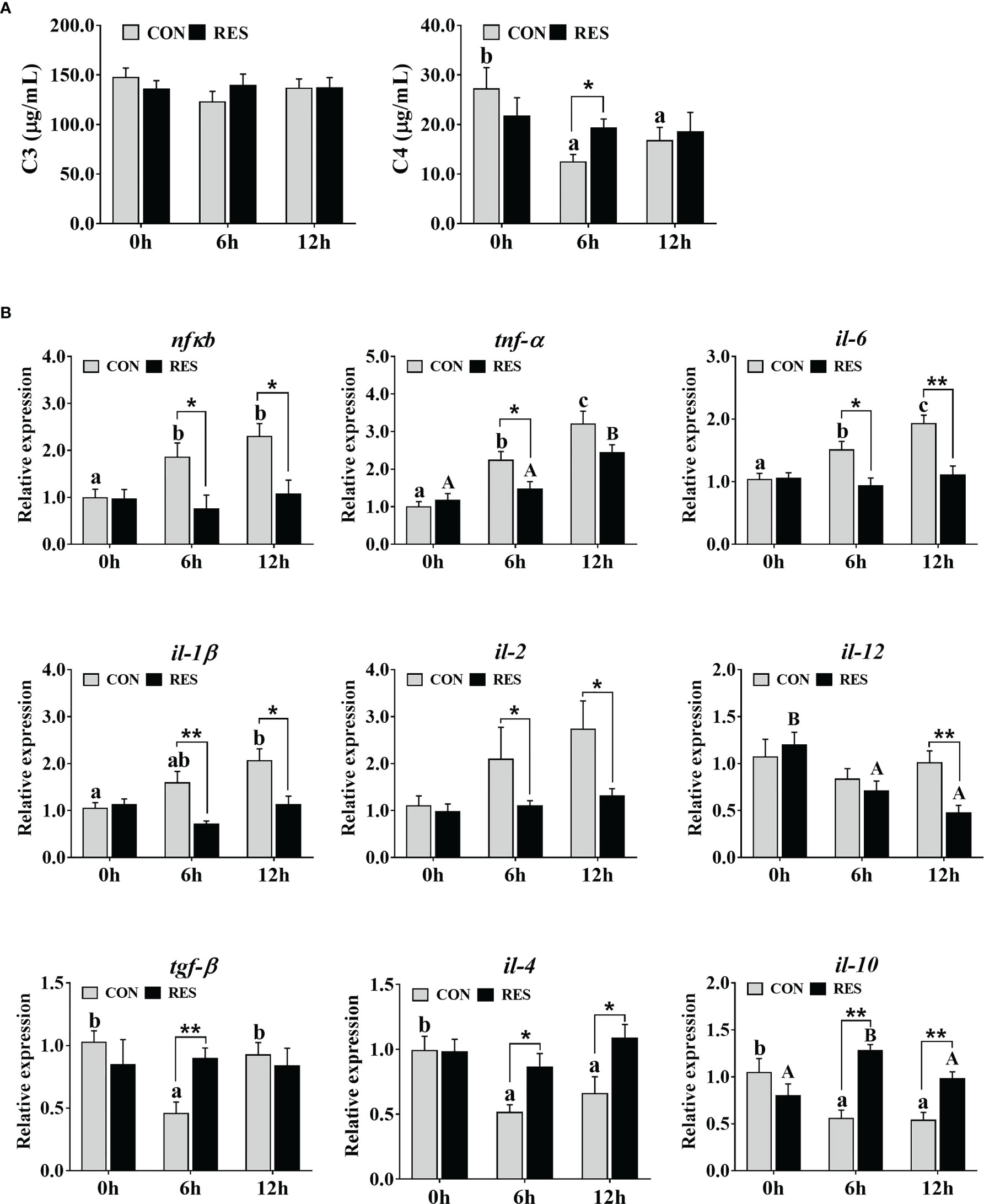
Figure 6 Effect of dietary RES on immune reaction in plasma (A) and mRNA expression levels of inflammation-related genes in the liver (B) of gibel carp at 6 h and 12 h after LPS injection. The lowercase letters represent significant differences among diverse LPS durations in the CON group (P < 0.05); the uppercase letters represent significant differences among diverse LPS durations in the RES group (P < 0.05). *(P < 0.05) and **(P < 0.01) represent significant differences between the CON and RES groups. Nfκb, nuclear factor-κB; tnf-α, tumor necrosis factor α; il-6, interleukin-6; il-1β, interleukin-1β; il-2, interleukin-2; il-12, interleukin-12; tgf-β, transforming growth factor β; il-4, interleukin-4; il-10, interleukin-10.
The transcript levels of inflammation-related genes in the liver of gibel carp at 0, 6, and 12 h after LPS injection are shown in Figure 6B. LPS enhanced the expression of the pro-inflammatory genes nfκb, tnf-α, il-6, and il-1β but inhibited the expression of the anti-inflammatory genes tgf-β, il-4, and il-10 in the CON group. Higher expression levels of tnf-α and il-10 but lower expression levels of il-12 were found in fish fed the RES diet. Compared to the CON group, the mRNA levels of nfκb, tnf-α, il-6, il-1β, il-2, and il-12 were dramatically downregulated, while that of tgf-β, il-4, and il-10 was upregulated in the RES group.
3.5 Mitochondrial fission and fusion related genes in hepatocytes
The mRNA levels of mitochondrial fission and fusion related genes in the liver are shown in Figure 7A. The drp1, fis1, and mff expression levels were upregulated, and the transcript levels of mfn1, mfn2, nrf1, and tfam were downregulated by LPS. In addition, the mRNA expression levels of mfn1, mfn2, nrf1, and tfam were significantly enhanced by RES after LPS injection. Compared with the CON group, decreased drp1, fis1, and mff expression levels were found in the RES group.
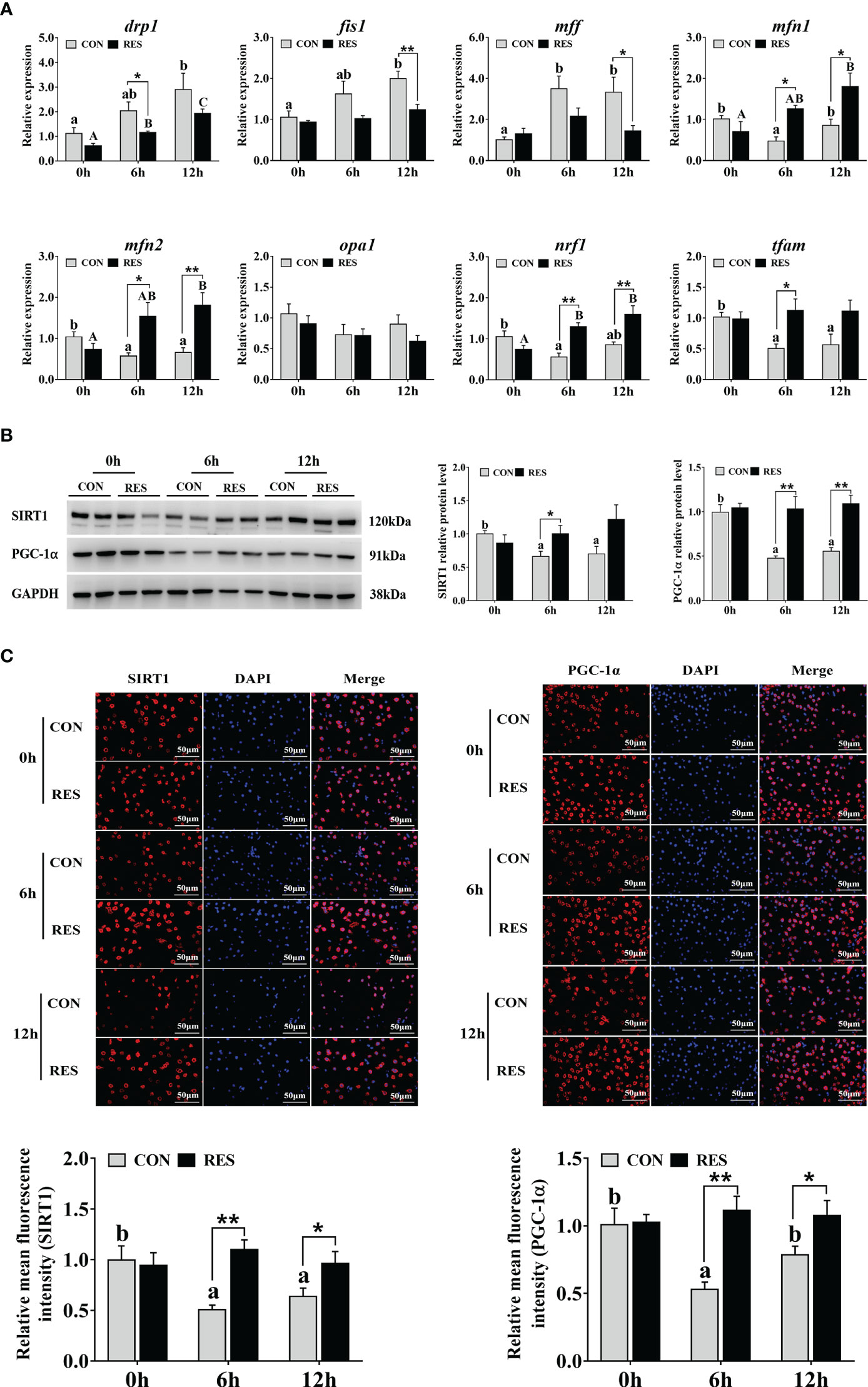
Figure 7 Effect of dietary RES on mitochondrial dynamics and mitochondrial biogenesis in the liver of gibel carp at 6 h and 12 h after LPS injection. (A) Mitochondrial dynamics related mRNA levels were determined by qRT-PCR. (B) Western blots for SIRT1 and PGC-1α and quantification results. (C) Immunofluorescent analysis of SIRT1 and PGC-1α stained with antibody (red) or DAPI (blue) and relative fluorescence intensity. Bars = 50 µm. Data are mean ± SEM (n = 6). The lowercase letters represent significant differences among diverse LPS durations in the CON group (P < 0.05); the uppercase letters represent significant differences among diverse LPS durations in the RES group (P < 0.05). *(P < 0.05) and **(P < 0.01) represent significant differences between the CON and RES groups. Drp1, dynamin-related protein 1; fis1, mitochondrial fission 1; mff, mitochondrial fission factor; mfn1, mitofusin 1; mfn2, mitofusin 2; opa1, optic atrophy 1; nrf1, nuclear respiratory factor 1; tfam, mitochondrial transcription factor A.
3.6 Mitochondrial biogenesis related SIRT1-PGC1α pathway
The expression of key proteins involved in the SIRT1-PGC1α signaling pathway in the liver of gibel carp injected with LPS is shown in Figure 7B. Both SIRT1 and PGC-1α relative protein levels were significantly inhibited in the CON group, while no change was found in the RES group. However, at 6 h after LPS injection, the levels of SIRT1 protein in the RES group were significantly enhanced compared to that in the CON group. PGC-1α levels were also increased at both 6 h and 12 h in the RES group. Similar changes were found in the results of immunofluorescence staining (Figure 7C).
3.7 Mitophagy-related SIRT1-P62/LC3B pathway
As shown in Figure 8A, LPS increased the number of autophagosomes only in the CON group. The mRNA levels of parkin, pink1, optn, and p62 were significantly upregulated by LPS (Figure 8B). Conversely, lc3b levels were inhibited after LPS administration. The expression levels of parkin, pink1, optn, and lc3b were enhanced by RES, whereas p62 expression was inhibited. No significant difference was found in bnip3 gene expression. Consistent results were found in protein expression levels of p62 and lc3b detected by Western blotting (Figure 8C) and fluorescence intensity detected by immunofluorescence staining (Figure 8D).
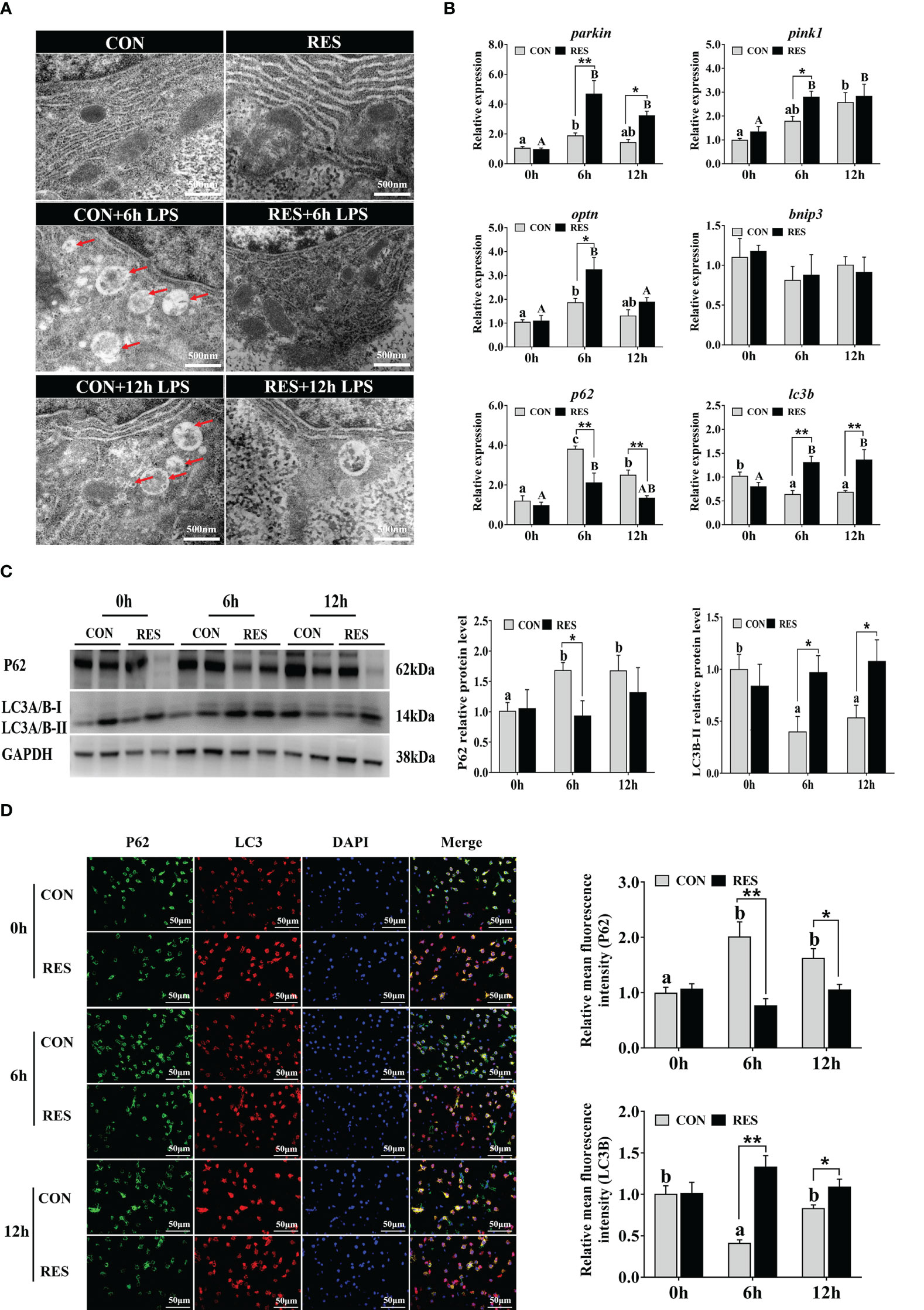
Figure 8 Effect of dietary RES on mitochondrial autophagy in the liver of gibel carp at 6 h and 12 h after LPS injection. (A) Ultrastructural changes of hepatocytes under TEM. Scale bar = 500 nm. Red arrows represent the autophagosome. (B) Mitophagy-related mRNA levels were determined with qRT-PCR. (C) Western blots for P62 and LC3B and quantification results. (D) Co-localization analysis of P62 (green) and LC3 (red) in the hepatocytes of gibel carp and the relative fluorescence intensity. DAPI staining is shown in blue. Bars = 50 µm. Data are mean ± SEM (n = 6). The lowercase letters represent significant differences among diverse LPS durations in the CON group (P < 0.05); the uppercase letters represent significant differences among diverse LPS durations in the RES group (P < 0.05). *(P < 0.05) and **(P < 0.01) represent significant differences between the CON and RES groups. Parkin, parkin RBR E3 ubiquitin-protein ligase; pink1, PTEN-induced kinase 1; optn, optineurin; bnip3, BCL2 interacting protein 3; lc3b, microtubule-associated proteins 1A/1B light chain 3B; p62, P62.
4 Discussion
LPS can activate the immune response in fish to trigger liver injury (6). Therefore, in this study, LPS was administered to gibel carp after 8 weeks of breeding, and samples were taken at 6 h and 12 h post-injection. The results showed that LPS induced significant increases in the plasma levels of AST, ALT, and LDH in gibel carp at 6 h post-injection, while by 12 h, the levels of AST and ALT were decreased compared to those at 6 h. ALT and AST are released into the plasma as markers of liver injury; elevated levels of LDH, which catalyzes the dehydrogenation of lactate to pyruvate, indicate liver dysfunction (29). Thus, the results suggested that liver injury was induced by LPS injection in the gibel carp and that the damage was time-dependent. This may have been due to the gradual metabolism of LPS by gibel carp and the cells themselves responding to external stimuli. Similarly, temporal differences were observed in mice, with plasma ALT and AST levels increasing and then decreasing during 12–72 h after LPS treatment (30). RES is a plant antitoxin polyphenol that attenuates LPS-induced hepatotoxicity in mice through changes in iron shuttle protein (31). In addition, RES has also been shown to alleviate hepatic immunotoxicity and liver injury in H2O2-treated tilapia (32). In this study, RES significantly reduced plasma AST, ALT, and LDH levels and alleviated liver injury in gibel carp after LPS injection.
After 6 h and 12 h of LPS treatment, liver HE staining showed mild swelling and even necrosis of the hepatocytes of the fish liver, accompanied by loss or fragmentation of nuclei, with significantly increased apoptosis. This suggested that LPS induced hepatocyte injury and apoptosis. Similarly, zebrafish hepatocytes were sparsely connected and showed nuclear atrophy and lysis after LPS treatment (8). In mice, TUNEL-positive staining of hepatocytes was significantly increased after LPS treatment (33). However, the liver tissues of gibel carp were basically restored to normal with intact and well-defined hepatocytes, and the number of apoptotic hepatocytes under TUNEL staining was significantly reduced in the RES group after LPS administration. Therefore, RES could alleviate the liver injury and significantly reduce the apoptosis of hepatocytes in gibel carp after LPS injection.
Oxidative stress is an imbalance between increased ROS and reduced activity of antioxidant mechanisms during physiological processes, resulting in severe damage to macromolecules (34). ROS react with lipids to induce lipid peroxidation, which directly responds to free radical damage. MDA, a metabolic end product of lipid peroxidation, is a reliable indicator of oxidative stress and inflammatory responses, and its inhibition is an effective way to combat oxidative stress (35). In this study, the MDA, LPO, and ROS levels in the liver of gibel carp were increased significantly at 6 h and 12 h after LPS injection and were decreased significantly after RES treatment, suggesting that RES can effectively alleviate LPS-induced oxidative stress. Consistent with this study, RES alleviated muscle oxidative damage in southern flounder (21). Temporally, the MDA and LPO levels in the liver were significantly lower at 12 h than at 6 h after LPS injection, indicating a reduction in lipid peroxidation. SOD is an important antioxidant enzyme in the endogenous antioxidant system, catalyzing the conversion of superoxide to oxygen and hydrogen peroxide and resisting oxidative damage (36). It was previously reported that LPS treatment reduced the antioxidant capacity of rats (31). In our study, liver SOD activity was significantly increased at 6 and 12 h after LPS injection, but T-AOC levels were remarkably reduced at 6 h. However, RES significantly increased SOD activity and T-AOC levels at 6 h compared to that in the CON group. Thus, our data indicated that LPS impaired the antioxidant system of gibel carp but reduced lipid peroxidation by enhancing antioxidant enzyme activity. Furthermore, RES could mitigate extra ROS by enhancing antioxidant capacity.
Nrf2 is critical in the endogenous antioxidant defense system, and its activation and inhibition are correlated with the degree of oxidative stress (37). In mice with acute lung injury, Galectin-1 significantly upregulated LPS-induced low expression of Nrf2 as well as the downstream antioxidant protective proteins HO-1 and NQO1 (38). In this study, the mRNA expression of nrf2 and downstream nqo-1, ho-1, and sod in gibel carp was significantly downregulated at 6 h and 12 h after LPS injection, which may be the result of Nrf2 inactivation. Previous study has shown that RES attenuated LPS-induced acute ileitis in ducks by mediating the phosphorylation of Nrf2 (39). Our results showed that RES treatment remarkably enhanced the gene expression of nrf2, nqo-1, ho-1, gpx, and sod compared with the CON group at 6 h after LPS injection. At 12 h after LPS injection, RES significantly enhanced the expression of nrf2, nqo-1, and ho-1. Thus, RES may protect against LPS-induced oxidative stress in gibel carp by upregulating the Nrf2 pathway, and its antioxidant effect was more pronounced at 6 h after LPS injection.
As key components of the immune response in fish, C3 and C4 play crucial roles in pathogen detection and clearance (40). In carp, LPS treatment significantly reduced the immunity of the fish (9). In this study, plasma C4 levels of gibel carp were significantly reduced after LPS injection. Compared with the CON group, RES significantly increased plasma C4 levels at 6 h. Numerous studies have shown that NF-κB is an important regulatory protein that is widely present in eukaryotic cells and is involved in the regulation of inflammatory responses in fish (6, 10, 41, 42). In grass carp, soybean β-globulin drove NF-κB P65 nuclear translocation and TOR phosphorylation to regulate inflammatory cytokines (41). In carp, the insecticide avermectin induced an inflammatory response by activating NF-κB pathways to release inflammatory factors that disrupted the blood-brain barrier structure (42). In addition, NF-κB has also been shown to regulate fish cytokines in the inflammatory response in gibel carp (6) and yellow catfish (10). Significant upregulation of nfkb and its downstream inflammatory factors thf-α, il-6, and il-1β, but significant downregulation of anti-inflammatory factors tgf-β, il-4, and il-10 at 6 h and 12 h after LPS injection suggested that severe inflammation was induced. However, RES reversed the expression of these genes, especially at 6 h after LPS injection. Similarly, RES exerted its antioxidant properties by regulating the Nrf2 and TLR2-Myd88-NF-κB signaling pathways that significantly attenuated the liver immunotoxicity in H2O2-treated tilapia (32). Taken together, the results suggest that RES may alleviate LPS-induced inflammation in gibel carp by activating Nrf2 and concurrently inhibiting the NF-κB pathway.
Mitochondria are important sites of ROS production and are particularly susceptible to oxidative stress (43). It has been demonstrated that LPS caused mitochondrial dysfunction through oxidative stress and excessive mitochondrial fission and that this may be a potential mechanism of inducing sepsis (44). In this study, the expression levels of the mitochondrial division genes drp1, fis1, and mff were significantly upregulated, while the mitochondrial fusion genes mfn1, mfn2, nrf1 and the mitochondrial transcription factor (tfam) were significantly downregulated at 6 h and 12 h after LPS injection, implying that LPS may stimulate a reduction in high-quality mitochondria, inhibit mitochondrial fusion, and promote mitochondrial division (45). PGC-1α is a major regulator of mitochondrial biosynthesis, whose activation is mediated by SIRT1-dependent deacetylation of NAD+, which activates and controls NRF1 and NRF2 and subsequent TFAM transcription (17). In this study, protein expression and antigen levels of SIRT1 and PGC-1α were significantly downregulated at 6 h and 12 h after LPS injection, demonstrating that LPS significantly interfered with the biological process of mitochondrial genesis in gibel carp. RES is a SIRT1 activator, and it enhanced the transcriptional regulation of SIRT1 to alleviate Cd-induced inhibition of PGC-1α and TFAM expression (46). Furthermore, RES increased the deacetylase activity of SIRT1 and reduced aberrant mitochondria in mice exposed to manganese (47). In gibel carp, RES significantly downregulated LPS-induced high expression of drp1, fis1, and mff and upregulated low expression of mfn1, mfn2, nrf1, and tfam by activating SIRT1 and PGC-1α. In addition, bisdemethoxycurcumin activated the antioxidant defense system and regulated mitochondrial biogenesis by upregulating PGC-1α and promoting the nuclear localization of Nrf2, repairing oxidative damage caused by LPS injection and maintaining mitochondrial function in broilers (48). Thus, combined with the upregulation of Nrf2 by RES, RES may induce the antioxidant system and mitochondrial biogenesis by activating the SIRT1-PGC-1α and Nrf2-Keap1 pathways to alleviate mitochondrial dysfunction after LPS injection in gibel carp.
When mitochondria were disrupted, PINK1 mediated the maturation of autophagosomes and their fusion with lysosomes by activating and recruiting parkin (49). Mitochondrial autophagy acts as a protective mechanism to remove damaged mitochondria and reduce excessive release of ROS through autophagosome formation, transport to lysosomes, and lysosomal degradation (15). The presence of autophagosomes indicates that LPS-induced mitochondrial dysfunction was ameliorated (43). In this study, autophagosomes were induced in the hepatocytes of gibel carp at 6 h and 12 h after LPS injection compared to the CON group. Similarly, the number of autophagosomes was increased in LPS-induced depressed mice (50). The expression levels of mitophagy-related genes parkin, pink1, and optn were upregulated at 6 h and 12 h after LPS injection. However, these genes were further enhanced by RES, and no autophagosomes were observed in the RES group, indicating that RES inhibited mitophagy and the formation of autophagosomes by mediating the PINK/Parkin pathway induced by LPS in gibel carp.
During mitophagy, LC3 proteins are hydrolytically cleaved to form LC3-I and subsequently processed and modified by ubiquitin-like systems to form LC3-II that is localized to the autophagosomal membrane (51). In late mitophagy, p62 binds to ubiquitinated proteins and forms a complex with LC3 to target mitochondria in the autophagosome (49, 52). It has been reported that autophagy receptor p62 recruitment in brain-injured mice promoted Parkin-dependent mitophagy, accompanied by increased LC3-II levels (53). In both in vivo and in vitro models, berberine enhanced mitophagy by increasing the LC3-II/LC3-I ratio and PINK1/Parkin protein levels and decreasing P62 protein levels, thereby protecting against acute kidney injury in mice (54). In this study, the p62 expression level was significantly enhanced at 6 h and 12 h after LPS injection, but lc3b was significantly reduced. Similar changes in the protein expression levels of P62 and LC3B-II/I were observed in the liver of gibel carp, confirming the occurrence of mitophagy to form autophagosomes induced by LPS. In contrast, opposite changes were found in the RES group, where the formation of LC3 complexes and the degradation of proteins after autophagy in the liver of gibel carp were promoted by RES.
5 Conclusions
We explored the effects of RES on oxidative stress, immunity, mitochondrial biogenesis, and mitophagy induced by LPS injection in gibel carp. The results demonstrated that a RES diet further reduced the stress in gibel carp by activating the Nrf2/Keap1 pathway and inhibiting the NF-κB pathway. RES alleviated the oxidative stress and inflammation by activating the Nrf2/Keap1 pathway and inhibiting the NF-κB pathway induced by LPS. Furthermore, RES enhanced mitochondrial autophagy and mitochondrial biogenesis to mitigate ROS accumulation by activating the SIRT1-PGC-1α and PINK/Parkin signaling pathways. Thus, RES regulated mitochondrial quality control to deal with oxidative stress and inflammation in LPS-injected gibel carp. Our results could provide a strategy for enhancing fish immunity in aquaculture.
Data availability statement
The original contributions presented in the study are included in the article/supplementary material. Further inquiries can be directed to the corresponding author.
Ethics statement
The animal study was reviewed and approved by the ethics committee of the Institute of Hydrobiology, Chinese Academy of Sciences.
Author contributions
LW, and QC performed the experiments, analyzed data, and drafted the manuscript. BD, HG, and YW researched data and contributed to the discussion. DH, XZ, and HL conducted the investigation. YY participated in the methodology. SX performed the funding acquisition and reviewed the draft. JJ performed the conceptualization, supervision, and funding acquisition, edited and revised the paper. All authors contributed to the article and approved the submitted version.
Funding
This research was financially supported by the National Natural Science Foundation of China (U19A2041; 32122089; 31972805; 32102811), the earmarked fund for CARS (CARS-45) and the National Key Research and Development Program of China (2018YFD0900605; 2019YFD0900200).
Conflict of interest
The authors declare that the research was conducted in the absence of any commercial or financial relationships that could be construed as a potential conflict of interest.
Publisher’s note
All claims expressed in this article are solely those of the authors and do not necessarily represent those of their affiliated organizations, or those of the publisher, the editors and the reviewers. Any product that may be evaluated in this article, or claim that may be made by its manufacturer, is not guaranteed or endorsed by the publisher.
References
1. Naylor RL, Hardy RW, Buschmann AH, Bush SR, Cao L, Klinger DH, et al. A 20-year retrospective review of global aquaculture. Nature (2021) 591(7851):551–63. doi: 10.1038/s41586-021-03308-6
2. Wu L, Li H, Xu W, Dong B, Geng H, Jin J, et al. Emodin alleviates acute hypoxia-induced apoptosis in gibel carp (Carassius gibelio) by upregulating autophagy through modulation of the AMPK/mTOR pathway. Aquaculture (2022) 548:737689. doi: 10.1016/j.aquaculture.2021.737689
3. Rodkhum C, Hirono I, Stork M, Di Lorenzo M, Crosa JH, Aoki T. Putative virulence-related genes in vibrio anguillarum identified by random genome sequencing. J Fish Dis (2006) 29(3):157–66. doi: 10.1111/j.1365-2761.2006.00692.x
4. Li P, Ye J, Zeng S, Yang C. Florfenicol alleviated lipopolysaccharide (LPS)-induced inflammatory responses in Ctenopharyngodon idella through inhibiting toll / NF-κB signaling pathways. Fish Shellfish Immunol (2019) 94:479–84. doi: 10.1016/j.fsi.2019.08.073
5. Ko EY, Cho SH, Kwon SH, Eom CY, Jeong MS, Lee W, et al. The roles of NF-κB and ROS in regulation of pro-inflammatory mediators of inflammation induction in LPS-stimulated zebrafish embryos. Fish Shellfish Immunol (2017) 68:525–29. doi: 10.1016/j.fsi.2017.07.041
6. Gu Y, Chen K, Xi B, Xie J, Bing X. Protective effects of paeonol against lipopolysaccharide-induced liver oxidative stress and inflammation in gibel carp (Carassius auratus gibelio). Comp Biochem Physiol Part C: Toxicol Pharmacol (2022) 257:109339. doi: 10.1016/j.cbpc.2022.109339
7. Luo S, Xiong N, Luo Z, Luo K, Liu S, Wu C, et al. Effect of lipopolysaccharide (LPS) stimulation on apoptotic process and oxidative stress in fibroblast cell of hybrid crucian carp compared with those of Carassius cuvieri and Carassius auratus red var. Comp Biochem Physiol Part C: Toxicol Pharmacol (2021) 248:109085. doi: 10.1016/j.cbpc.2021.109085
8. Zhang Y, Cen J, Jia Z, Hsiao C, Xia Q, Wang X, et al. Hepatotoxicity induced by isoniazid-lipopolysaccharide through endoplasmic reticulum stress, autophagy, and apoptosis pathways in zebrafish. Antimicrob Agents Chemother (2019) 63(5):e01639–18. doi: 10.1128/AAC.01639-18
9. Giri SS, Kim HJ, Kim SG, Kim SW, Kwon J, Lee SB, et al. Effectiveness of the guava leaf extracts against lipopolysaccharide-induced oxidative stress and immune responses in. Cyprinus carpio. Fish Shellfish Immunol (2020) 105:164–76. doi: 10.1016/j.fsi.2020.06.004
10. Liu Y, Meng F, Wang S, Xia S, Wang R. Vitamin D3 mitigates lipopolysaccharide-induced oxidative stress, tight junction damage and intestinal inflammatory response in yellow catfish, Pelteobagrus fulvidraco. Comp Biochem Physiol Part C: Toxicol Pharmacol (2021) 243:108982. doi: 10.1016/j.cbpc.2021.108982
11. Singh V, Ubaid S. Role of silent information regulator 1 (SIRT1) in regulating oxidative stress and inflammation. Inflammation (2020) 43(5):1589–98. doi: 10.1007/s10753-020-01242-9
12. Komatsu M, Kurokawa H, Waguri S, Taguchi K, Kobayashi A, Ichimura Y, et al. The selective autophagy substrate p62 activates the stress responsive transcription factor Nrf2 through inactivation of Keap1. Nat Cell Biol (2010) 12(3):213–23. doi: 10.1038/ncb2021
13. Lin Q, Li S, Jiang N, Shao X, Zhang M, Jin H, et al. PINK1-parkin pathway of mitophagy protects against contrast-induced acute kidney injury via decreasing mitochondrial ROS and NLRP3 inflammasome activation. Redox Biol (2019) 26:101254. doi: 10.1016/j.redox.2019.101254
14. Aucello M, Dobrowolny G, Musarò A. Localized accumulation of oxidative stress causes muscle atrophy through activation of an autophagic pathway. Autophagy (2009) 5(4):527–29. doi: 10.4161/auto.5.4.7962
15. Zhu J, Wang KZQ, Chu CT. After the banquet: mitochondrial biogenesis, mitophagy, and cell survival. Autophagy (2013) 9(11):1663–76. doi: 10.4161/auto.24135
16. Qiu L, Luo Y, Chen X. Quercetin attenuates mitochondrial dysfunction and biogenesis via upregulated AMPK/SIRT1 signaling pathway in OA rats. BioMed Pharmacother (2018) 103:1585–91. doi: 10.1016/j.biopha.2018.05.003
17. Rius-Pérez S, Torres-Cuevas I, Millán I, Ortega ÁL, Pérez S. PGC-1α, inflammation, and oxidative stress: an integrative view in metabolism. Oxid Med Cell Longev (2020) 2020:1452696. doi: 10.1155/2020/1452696
18. Liu B, Xie J, Ge X, Xu P, Miao L, Zhou Q, et al. Comparison study of the effects of anthraquinone extract and emodin from rheum officinale bail on the physiological response, disease resistance of megalobrama amblycephala under high temperature stress. Turkish J Fish Aqu Sci (2012) 12(4):905–16. doi: 10.4194/1303-2712-v12_4_18
19. Xie J, Liu B, Zhou Q, Su Y, He Y, Pan L, et al. Effects of anthraquinone extract from rhubarb rheum officinale bail on the crowding stress response and growth of common carp Cyprinus carpio var. Jian. Aquacult (2008) 281(1-4):5–11. doi: 10.1016/j.aquaculture.2008.03.038
20. Xu Y, Nie L, Yin Y, Tang J, Zhou J, Li D, et al. Resveratrol protects against hyperglycemia-induced oxidative damage to mitochondria by activating SIRT1 in rat mesangial cells. Toxicol Appl Pharmacol (2012) 259(3):395–401. doi: 10.1016/j.taap.2011.09.028
21. Wilson WN, Baumgarner BL, Watanabe WO, Alam MS, Kinsey ST. Effects of resveratrol on growth and skeletal muscle physiology of juvenile southern flounder. Comp Biochem Physiol Part A: Mol Integr Physiol (2015) 183:27–35. doi: 10.1016/j.cbpa.2014.12.014
22. Tan C, Zhou H, Wang X, Mai K, He G. Resveratrol attenuates oxidative stress and inflammatory response in turbot fed with soybean meal based diet. Fish Shellfish Immunol (2019) 91:130–35. doi: 10.1016/j.fsi.2019.05.030
23. Wu D, Li J, Fan Z, Wang L, Zheng X. Resveratrol ameliorates oxidative stress, inflammatory response and lipid metabolism in common carp (Cyprinus carpio) fed with high-fat diet. Front Immunol (2022) 13:965954. doi: 10.3389/fimmu.2022.965954
24. Kim MY, Lim JH, Youn HH, Hong YA, Yang KS, Park HS, et al. Resveratrol prevents renal lipotoxicity and inhibits mesangial cell glucotoxicity in a manner dependent on the AMPK-SIRT1-PGC1α axis in db/db mice. Diabetologia (2013) 56(1):204–17. doi: 10.1007/s00125-012-2747-2
25. Wu L, Chen Q, Dong B, Han D, Zhu X, Liu H, et al. Resveratrol attenuated oxidative stress and inflammatory and mitochondrial dysfunction induced by acute ammonia exposure in gibel carp (Carassius gibelio). Ecotoxicol Environ Saf (2023) 251:114544. doi: 10.1016/j.ecoenv.2023.114544
26. Chen C, Chen X, Chen C. Distribution of intraperitoneally administered Cytophaga columnaris lipopolysaccharide in gibel carp, Carassius auratus gibelio. J Huazhong Agric Univ (2003) 22(3):243–46. doi: 10.13300/j.cnki.hnlkxb.2003.03.010
27. Dong B, Wu L, Chen Q, Xu W, Li D, Han D, et al. Tolerance assessment of Atractylodes macrocephala polysaccharide in the diet of largemouth bass (Micropterus salmoides). Antioxidants (2022) 11(8):1581. doi: 10.3390/antiox11081581
28. Pfaffl MW. A new mathematical model for relative quantification in real-time RT-PCR. Nucleic Acids Res (2001) 29(9):e45. doi: 10.1093/nar/29.9.e45
29. Tahmasebi-Kohyani A, Keyvanshokooh S, Nematollahi A, Mahmoudi N, Pasha-Zanoosi H. Effects of dietary nucleotides supplementation on rainbow trout (Oncorhynchus mykiss) performance and acute stress response. Fish Physiol Biochem (2012) 38(2):431–40. doi: 10.1007/s10695-011-9524-x
30. Xiong X, Ren Y, Cui Y, Li R, Wang C, Zhang Y. Obeticholic acid protects mice against lipopolysaccharide-induced liver injury and inflammation. BioMed Pharmacother (2017) 96:1292–98. doi: 10.1016/j.biopha.2017.11.083
31. Sebai H, Sani M, Yacoubi MT, Aouani E, Ghanem-Boughanmi N, Ben-Attia M. Resveratrol, a red wine polyphenol, attenuates lipopolysaccharide-induced oxidative stress in rat liver. Ecotoxicol Environ Saf (2010) 73(5):1078–83. doi: 10.1016/j.ecoenv.2009.12.031
32. Jia R, Li Y, Cao L, Du J, Zheng T, Qian H, et al. Antioxidative, anti-inflammatory and hepatoprotective effects of resveratrol on oxidative stress-induced liver damage in tilapia (Oreochromis niloticus). Comp Biochem Physiol Part C: Toxicol Pharmacol (2019) 215:56–66. doi: 10.1016/j.cbpc.2018.10.002
33. Li Q, Tan Y, Chen S, Xiao X, Zhang M, Wu Q, et al. Irisin alleviates LPS-induced liver injury and inflammation through inhibition of NLRP3 inflammasome and NF-κB signaling. J Recept Signal Transduct (2021) 41(3):294–303. doi: 10.1080/10799893.2020.1808675
34. Yu Y, Hu L, Liu L, Yu L, Li J, Rao J, et al. Hsp22 ameliorates lipopolysaccharide-induced myocardial injury by inhibiting inflammation, oxidative stress, and apoptosis. Bioengineered (2021) 12(2):12544–54. doi: 10.1080/21655979.2021.2010315
35. Dudzińska E, Gryzinska M, Ognik K, Gil-Kulik P, Kocki J. Oxidative stress and effect of treatment on the oxidation product decomposition processes in IBD. Oxid Med Cell Longev (2018) 2018:7918261. doi: 10.1155/2018/7918261
36. Martínez-álvarez RM, Morales AE, Sanz A. Antioxidant defenses in fish: biotic and abiotic factors. Rev Fish Biol Fish (2005) 15(1-2):75–88. doi: 10.1007/s11160-005-7846-4
37. Yu X, Wu Y, Deng M, Liu Y, Wang S, He X, et al. Tetracycline antibiotics as PI3K inhibitors in the Nrf2-mediated regulation of antioxidative stress in zebrafish larvae. Chemosphere (2019) 226:696–703. doi: 10.1016/j.chemosphere.2019.04.001
38. Huang X, Liu W, Zhou Y, Sun M, Yang H, Zhang C, et al. Galectin-1 ameliorates lipopolysaccharide-induced acute lung injury via AMPK-Nrf2 pathway in mice. Free Radical Biol Med (2020) 146:222–33. doi: 10.1016/j.freeradbiomed.2019.11.011
39. Yang H, Wang Y, Jin S, Pang Q, Shan A, Feng X. Dietary resveratrol alleviated lipopolysaccharide-induced ileitis through Nrf2 and NF-κB signalling pathways in ducks (Anas platyrhynchos). J Anim Physiol Anim Nutr (2022) 106(6):1306–20. doi: 10.1111/jpn.13657
40. Magnadóttir B. Innate immunity of fish (overview). Fish Shellfish Immunol (2006) 20(2):137–51. doi: 10.1016/j.fsi.2004.09.006
41. Duan X, Jiang W, Wu P, Liu Y, Jiang J, Tan B, et al. Soybean β-conglycinin caused intestinal inflammation and oxidative damage in association with NF-κB, TOR and Nrf2 in juvenile grass carp (Ctenopharyngodon idella): varying among different intestinal segments. Fish Shellfish Immunol (2019) 95:105–16. doi: 10.1016/j.fsi.2019.10.021
42. Zhang T, Dong Z, Liu F, Pan E, He N, Ma F, et al. Avermectin induces carp neurotoxicity by mediating blood-brain barrier dysfunction, oxidative stress, inflammation, and apoptosis through PI3K/Akt and NF-κB pathways. Ecotoxicol Environ Saf (2022) 243:113961. doi: 10.1016/j.ecoenv.2022.113961
43. Chen H, Lin H, Dong B, Wang Y, Yu Y, Xie K. Hydrogen alleviates cell damage and acute lung injury in sepsis via PINK1/Parkin-mediated mitophagy. Inflammation Res (2021) 70(8):915–30. doi: 10.1007/s00011-021-01481-y
44. Jiang X, Cai S, Jin Y, Wu F, He J, Wu X, et al. Irisin attenuates oxidative stress, mitochondrial dysfunction, and apoptosis in the H9C2 cellular model of septic cardiomyopathy through augmenting fundc1-dependent mitophagy. Oxid Med Cell Longev (2021) 2021:2989974. doi: 10.1155/2021/2989974
45. Twig G, Elorza A, Molina AJA, Mohamed H, Wikstrom JD, Walzer G, et al. Fission and selective fusion govern mitochondrial segregation and elimination by autophagy. EMBO J (2008) 27(2):433–46. doi: 10.1038/sj.emboj.7601963
46. Zhang Q, Zhang C, Ge J, Lv M, Talukder M, Guo K, et al. Ameliorative effects of resveratrol against cadmium-induced nephrotoxicity via modulating nuclear xenobiotic receptor response and PINK1/Parkin-mediated mitophagy. Food Funct (2020) 11(2):1856–68. doi: 10.1039/c9fo02287b
47. Lei M, Cong L, Liu Z, Liu Z, Ma Z, Liu K, et al. Resveratrol reduces DRP1-mediated mitochondrial dysfunction via the SIRT1-PGC1α signaling pathway in manganese-induced nerve damage in mice. Environ Toxicol (2022) 37(2):282–98. doi: 10.1002/tox.23397
48. Zhang J, Yang Y, Han H, Zhang L, Wang T. Bisdemethoxycurcumin protects small intestine from lipopolysaccharide-induced mitochondrial dysfunction via activating mitochondrial antioxidant systems and mitochondrial biogenesis in broiler chickens. Oxid Med Cell Longev (2021) 2021:9927864. doi: 10.1155/2021/9927864
49. Palikaras K, Lionaki E, Tavernarakis N. Mechanisms of mitophagy in cellular homeostasis, physiology and pathology. Nat Cell Biol (2018) 20(9):1013–22. doi: 10.1038/s41556-018-0176-2
50. Li C, Zhu Y, Wu Y, Fu M, Wu Y, Wu Y, et al. Oridonin alleviates LPS-induced depression by inhibiting NLRP3 inflammasome via activation of autophagy. Front Med (2022) 8:813047. doi: 10.3389/fmed.2021.813047
51. Mizushima N, Yoshimori T. How to interpret LC3 immunoblotting. Autophagy (2007) 3(6):542–45. doi: 10.4161/auto.4600
52. Wang Y, Zhu J, Liu Z, Shu S, Fu Y, Liu Y, et al. The PINK1/PARK2/optineurin pathway of mitophagy is activated for protection in septic acute kidney injury. Redox Biol (2021) 38:101767. doi: 10.1016/j.redox.2020.101767
53. Wu M, Lu G, Lao Y, Zhang H, Zheng D, Zheng Z, et al. Garciesculenxanthone b induces PINK1-parkin-mediated mitophagy and prevents ischemia-reperfusion brain injury in mice. Acta Pharmacol Sin (2021) 42(2):199–208. doi: 10.1038/s41401-020-0480-9
Keywords: resveratrol, lipopolysaccharide, oxidative stress, inflammation, mitophagy
Citation: Wu L, Chen Q, Dong B, Geng H, Wang Y, Han D, Zhu X, Liu H, Zhang Z, Yang Y, Xie S and Jin J (2023) Resveratrol alleviates lipopolysaccharide-induced liver injury by inducing SIRT1/P62-mediated mitophagy in gibel carp (Carassius gibelio). Front. Immunol. 14:1177140. doi: 10.3389/fimmu.2023.1177140
Received: 01 March 2023; Accepted: 10 April 2023;
Published: 24 April 2023.
Edited by:
Yu Zhuang, Jiangxi Agricultural University, ChinaReviewed by:
Rui Jia, Chinese Academy of Fishery Sciences (CAFS), ChinaOla Habotta, Mansoura University, Egypt
Copyright © 2023 Wu, Chen, Dong, Geng, Wang, Han, Zhu, Liu, Zhang, Yang, Xie and Jin. This is an open-access article distributed under the terms of the Creative Commons Attribution License (CC BY). The use, distribution or reproduction in other forums is permitted, provided the original author(s) and the copyright owner(s) are credited and that the original publication in this journal is cited, in accordance with accepted academic practice. No use, distribution or reproduction is permitted which does not comply with these terms.
*Correspondence: Junyan Jin, amluanVueWFuQGloYi5hYy5jbg==
†These authors have contributed equally to this work