- 1Department of Clinical Pharmacy and Pharmacy Administration, School of Pharmacy, Fudan University, Shanghai, China
- 2School of Pharmacy, Minhang Hospital, Fudan University, Shanghai, China
Objective: The combination of nanoparticle albumin-bound paclitaxel (nab-PTX)/paclitaxel (PTX) with immune checkpoint inhibitors (ICIs) has demonstrated significant efficacy in cancer patients. However, the safety of these combination regimens remains conflicting in former researches. Therefore, in order to address this issue, we performed a systematic review and network meta-analysis (NMA) to evaluate and compare the safety profile.
Methods: We performed a systematic review by searching randomized controlled trials (RCTs) from PubMed, EMBASE, Cochrane Library, ClinicalTrials.gov, and Web of Science up to August 15, 2022. The primary outcomes were all‐grade (grade 1‐5) and high‐grade (grade 3‐5) immune-related adverse events (irAEs). Secondary outcomes were all‐grade (grade 1‐5) and high‐grade (grade 3‐5) irAEs of subgroups of ICIs.
Results: There were 22 RCTs included in the NMA, involving a total of 15 963 patients diagnosed with any type of cancer. ICIs+nab-PTX was associated with a noticeably decreased risk of grade 3-5 pneumonitis (odds ratio [OR]=0.28, 95% credible interval [CrI]: 0.09,0.90) compared to ICI monotherapy; ICIs+PTX showed a lower risk of grade 1-5 hyperthyroidism (OR=0.46, 95% CrI: 0.22-0.96) and grade 1-5 hypothyroidism (OR=0.49, 95% CrI: 0.26-0.93) than ICIs. Compared with PD-1, PD-1+PTX was associated with a statistically significantly lower risk of grade 1-5 pneumonitis (OR=0.32, 95% CrI: 0.11-0.92). PD-L1 resulted in a noticeably lower risk of grade 1-5 hypothyroidism (OR=0.34, 95% CrI: 0.12-1.00) than PD-L1+PTX. Nearly all treatment regimens containing ICIs demonstrated significantly higher risks of irAEs compared to the standard chemotherapy groups.
Conclusion: Nab-PTX/PTX+ICIs demonstrated an approach leading to decreased risk of irAEs compared with ICI monotherapy. This finding supports that ICIs+nab-PTX/PTX may be a safer treatment strategy. Moreover, we also found that the combination regimens containing ICIs had a higher risk of irAEs than standard chemotherapy. Additionally, ICIs+nab-PTX demonstrated a decreased risk of irAEs compared to ICIs+PTX. PD-1 inhibitors were associated with a higher risk of irAEs than PD-L1 inhibitors.
1 Introduction
Immune checkpoint inhibitors (ICIs) targeting programmed cell death 1 (PD-1), programmed cell death ligand 1 (PD-L1), or cytotoxic T lymphocyte antigen 4 (CTLA4), has been become one of the most important breakthroughs in cancer therapy (1). Immune suppression plays a key role in cancer growth and progression. ICIs promote immune responses against tumor cells by blocking immune checkpoint pathways. Treatment targeting immune checkpoints, such as anti-PD-1, anti-PD-L1, and anti-CTLA4 demonstrate impressive anti-tumor activities against several tumor types (2). Over the past decades, monoclonal antibodies against the PD-1/PD-L1 pathway have been approved for melanoma, prostate cancer, lung cancer, liver cancer, cervical cancer, gastric cancer, and breast cancer (3). However, a large proportion of patients do not respond or even resistant to ICIs (4–6). In several clinical trials (7–9) using biomarkers to predict the treatment response to anti–PD-1 or anti–PD-L1 therapies, the objective response rates were still unsatisfied (<50%).
Current research had been focused heavily on the improvement of the response rate of ICIs. Taxane-based chemotherapies, including albumin-bound paclitaxel (nab-PTX) and paclitaxel (PTX) (10–13), might have a “priming effect” for the immune system and improve the response to the ICIs (14). Although the “priming effect” of chemotherapy is still unexplained, ICIs combined with nab-PTX/PTX demonstrated superior efficacy in multiple clinical trials (13, 15). ICIs combined with nab-PTX/PTX has been widely adopted in the clinical practice (16), even though the combination therapy is not strongly recommended by the National Comprehensive Cancer Network (NCCN) (17–19).
Despite the substantial clinical benefits associated with ICIs+nab-PTX/PTX (20, 21), there has been rising concerns on the safety of combination therapy. ICIs may result in the activation of the immune system and are associated with adverse events, which are known as immune-related adverse events (irAEs) (22) (23, 24). The irAEs (22) include rash, colitis, hepatitis, hypothyroidism, hyperthyroidism and pneumonitis, occurring in up to 70% of patients treated with ICIs. The irAEs (22) could be severe or even fatal (22, 25), but their mechanism is still unclear. The NCCN has released several guidelines addressing adverse events associated with ICIs. For the combination of ICIs and nab-PTX/PTX, the synergistic effect of the combination strategy may attribute to therapy-associated cytokine release and T-cell-mediated organ infiltration (10, 11, 13, 26–30), leading to the alterations in safety profiles. However, the safety profiles of ICIs and nab-PTX/PTX is still inconsistent in the literature. Previous studies suggested that ICI alone is generally better tolerated than combination regimens (26, 31), but more recent studies concluded that ICIs and nab-PTX/PTX combination regimen demonstrated better safety profiles (32, 33). To our best knowledge, there are limited studies investigating the safety of ICIs+nab-PTX/PTX. Past meta-analyses mainly focused on a specific ICI or nab-PTX/PTX, failing to cover possible combination therapies (26, 34–36). With more ICIs on the market, it is very important to compare the safety profiles between combination regimens, but the head-to-head comparison is largely lacking. Therefore, we conducted a network meta-analysis (NMA) to comprehensively evaluate the safety profile and safety ranking of nab-PTX+ICI, PTX+ICI, ICI monotherapy and chemotherapy. This approach allowed us to combine direct and indirect evidence and rank the interventions based on their relative safety profiles.
2 Methods
This study was registered in the Prospective Register of Systematic Reviews (CRD42022326742). This NMA followed the Preferred Reporting Items for Systematic Reviews and Meta-Analysis (PRISMA) and the PRISMA extension statement for network meta-analysis (37).
2.1 Data sources and searches
We conducted a comprehensive search of relevant studies using keywords in electronic databases, including PubMed, Embase, Cochrane Library, Web of Science and ClinicalTrials.gov, between January 1, 2000 and August 15, 2022. Search key terms used in the search strategy include cancer, oncology, nab-PTX/PTX, immune checkpoint inhibitors, randomized controlled trials. The search strategy is described in the Supplementary Table 1. Two reviewers (WJ and JZ) firstly screened the titles and abstracts, then reviewed the full-texts of publications. Any discrepancies were resolved through discussion and consultation with the third reviewer (YW).
2.2 Study selection criteria
The study had pre-defined inclusion and exclusion criteria. Inclusion criteria were: (1) phase II or phase III randomized clinical trials (RCTs) with head-to-head comparison; (2) trials typically involve at least two arms the following mentioned: one ICI drug (PD-1/PD-L1 inhibitors), one ICI drug in combination with nab-PTX/PTX, one ICI drug in combination with chemotherapy; (3) study subjects diagnosed with cancer, (3) reported the incidence and grade of adverse events; (4) written in English.
The publications were excluded with any of the following: (1) letters, abstracts, reviews, posters, conference reports, unfinished studies or duplicated reports; (2) trials with insufficient data; (3) single-arm studies; (4) phase I randomized trials; (5) cost-effectiveness studies.
2.3 Data extraction
Two reviewers (WJ and JZ) extracted data independently, including first author, year of publication, treatment line, type of ICIs, stage of the cancer trial phase, treatment arm, incidence of grade 1-5 and grade 3-5 irAEs, sample size, patient age, sex distribution, cancer type, PD-L1 expression, Performance Status (PS) score, median follow-up time and Common Terminology Criteria for Adverse Events (CTCAE) edition.
In terms of adverse events data, because immune-related adverse events were the outcome of interest, we first evaluated “immune-related adverse events” in the main text and Supplementary Materials of published studies. We also screened all possible information available at ClinicalTrials.gov to obtain a more comprehensive data extraction. If irAEs were not available in the study (n=2), treatment-related adverse events were used and extracted.
2.4 Quality assessment
We used the Cochrane Collaboration’s risk of bias tool (38) to assess the quality of each trial included. Two reviewers (WH and YW) assessed on the 5 aspects, including the random sequence generation, allocation concealment, blinding, outcomes assessment, and reporting. Each aspect was graded based on the risk of bias, categorized by yes, no, or unclear. Any discrepancies in data extraction and quality assessment disagreements were resolved by discussion to achieve a consensus.
2.5 Statistical analysis
We summarized characteristics of trials, including first author, year of publication, treatment line, type of ICIs, stage of the cancer, trial phase, treatment arm, incidence of grade1-5 and grade 3-5 irAEs, sample size, patient age, sex distribution, cancer type, PD-L1 expression, PS score, median follow-up time and CTCAE edition. We accessed on the total number of all irAEs and the number of each specific irAEs, respectively. Incidence rates of grade 1-5 and grade 3-5 irAEs were compared among different treatment regimens, including chemotherapy, ICI monotherapy, ICI+nab-PTX and ICI+PTX. To investigate whether the occurrence of irAEs was influenced by the type of ICIs (PD-1 and PD-L1), we further subdivided the four treatment groups into six subgroups based on different types of ICIs: chemotherapy, PD-1 monotherapy, PD-L1 monotherapy, PD-1+PTX, PD-L1+PTX and ICIs+nab-PTX. Considering the relatively small sample sizes of PD-1+nab-PTX and PD-L1+nab-PTX, they were combined into one group.
To evaluate the statistical heterogeneity of the included trials, we accessed the I2 index and the Cochran Q statistic. Heterogeneity was defined as low for I2 values as 25–49%, moderate for 50–74%, and high for >75%, respectively. For NMA, we generated network plots depicting direct and indirect comparisons using STATA V.17.0. We used ADDIS-1.16.6 for head-to-head direct meta-analyses. To evaluate the risk of irAEs, we calculated ORs and 95% CIs using the random effects model, to account for unexplained heterogeneity. The random effects model is considered to be the most conservative method (39). Two-sided P<0.05 was considered significant.
Due to the potential low irAEs rate and limited sample size, irAEs may sometimes be rare (40, 41) or even absent. To address this issue, we used frequentists-framework-based network meta-analyses for all statistical analyses, and if there were no irAEs observed in a specific arm of a trial, the classic continuity correction of 0.5 for zero cells was applied for data preparation (26). Treatment effects were reported as the surface under the cumulative ranking curves (SUCRA). The higher SUCRA scores indicates a higher risk of irAEs.
Because consistency assessment is crucial in ensuring the robustness of direct and indirect comparison results (42), we used a two-step method to evaluate consistency. First, we used the loop‐specific approach to evaluate the presence of inconsistency from direct and indirect evidence (43). We calculated the inconsistency factors (IF) values, standard error of inconsistency factors (seIF) and p-value. If the 95% CI of IF contained ‘0’ and the p-value was higher than ‘0.05’, it was considered the direct evidence to be consistent with the indirect evidence. Second, we adopted node-splitting models to identify the consistency in the entire network on particular comparisons (nodes). P>0.05 indicated no significant inconsistency.
To evaluate the transitivity of the NMA, we compared the distribution of patient characteristics, aiming to ensure the similarity of the distribution of effect modifiers across different treatment comparisons in the network of trials. Furthermore, “comparison-adjusted” funnel plots were utilized to assess the presence small-sample effect and publication bias within the network of interventions.
3 Results
3.1 Study selection and patient characteristics
We identified 3 604 citations up to August 15, 2022, including 325 records from PubMed, 464 from Embase, 43 from Web of Science, 2 543 from Cochrane and 229 from ClinicalTrials.gov (Figure 1). After removing duplicates, 3 112 records were included in the titles and abstracts screening. A total of 641 publications underwent full-text review, after excluding 2 471 publications. Twenty-two RCTs (44–65) met the study selection criteria and were included in the analysis. Figure 2 shows that among the patients included in the network meta-analysis, 3 919 patients received ICIs, 1 386 patients received ICI+nab-PTX, 3 302 patients received ICI+PTX, and 7 356 patients received chemotherapy.
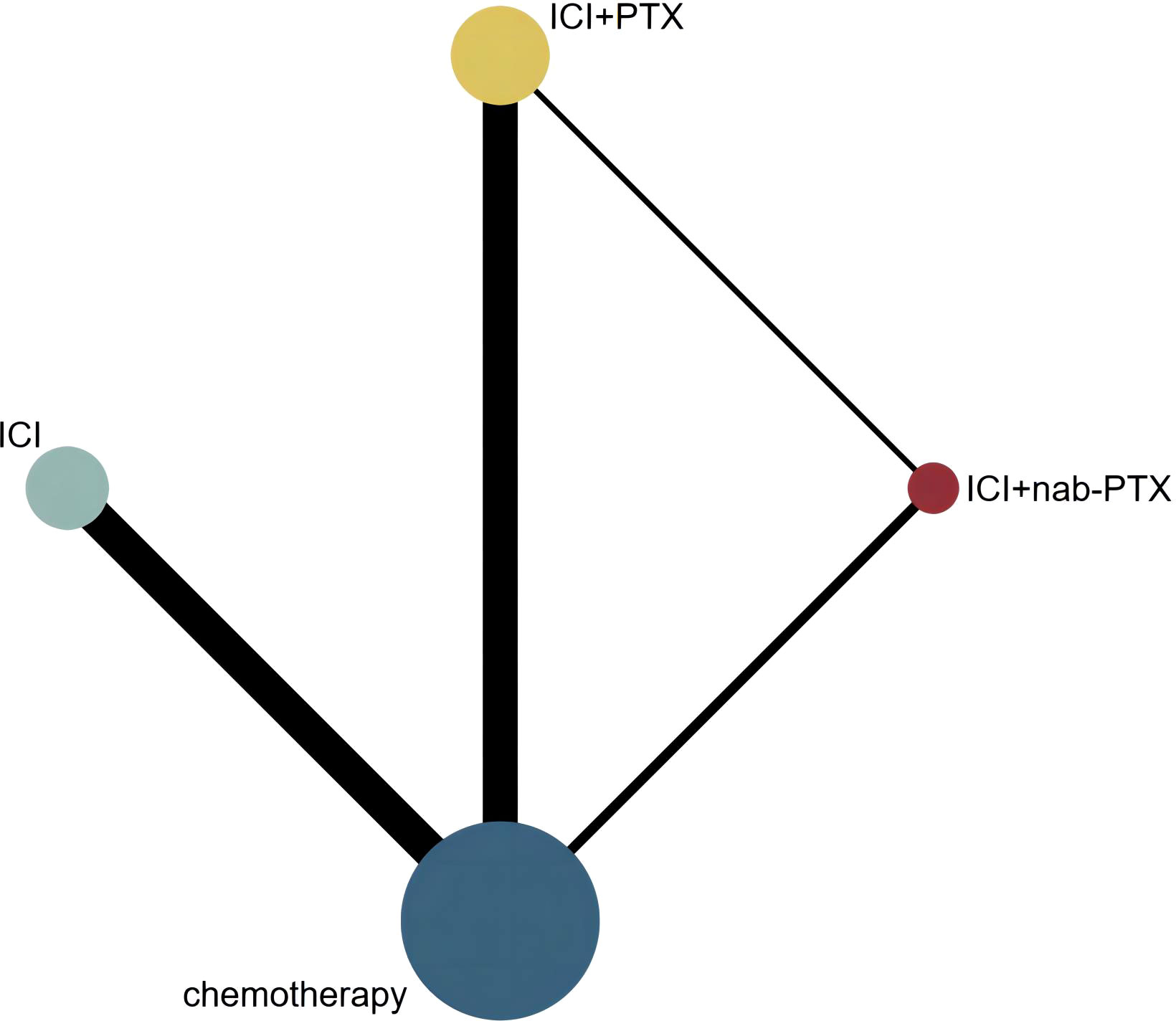
Figure 2 Network geometry of any event. The nodes in the figure represent the interventions being compared, while the edges represent the direct comparisons available between pairs of interventions (i.e. comparisons evaluated in at least one study). The node sizes are weighted based on the number of patients in each intervention arm, while the edges are weighted using inverse variance.
Table 1 show the information on the baseline characteristics of the included trials. There were 20 two arm trials, and only 2 studies have three arms. Supplementary Table 2 displays the occurrence of irAEs in different treatment groups. all studies were phase III trials. Cancer types tested in these studies included lung cancer (n=12), breast cancer (n=3), urothelial cancer (n=3), ovarian cancer (n=2), gastric cancer (n=2). More detailed information can be found in Supplementary Table 1.
Figure 3 presents the risk of bias summary for the included trials. It is worth noting that many trials were open-labeled due to the differences in infusion duration, administration schedules, and premedication requirements for immune checkpoint inhibitors, which would make masking difficult, but this does not indicate that the studies were of low quality.
3.2 Heterogeneity, inconsistency, and transitivity assessment
Pairwise comparisons with heterogeneity estimates are presented in Figure 4 and Supplementary Figure 1. Nearly all comparisons indicating low heterogeneity. Inconsistency analysis using node-splitting and loop-specific approaches showed no significant inconsistency between direct and indirect analyses. Results of the inconsistency evaluation are presented in Supplementary Tables 6, 7. All included clinical trials enrolled cancer patients; all the trials were phase III RCTs; utilizing standard doses (the dosage of PTX in the Asian population was 175 mg/m2/3 weeks, and of other races was 200mg/m2/3 weeks); median follow-up time was 23.2 months (ranging from 7.9 to 60 months); patients were at advanced stages of cancer, PS scores were mostly 0-1, and age characteristics were similar. By comparison, the baseline characteristic distribution of each treatment group was balanced, indicating acceptable transitivity. The network’s funnel plots visually indicate potential publication bias, and no significant asymmetry was observed (Supplementary Figure 6)
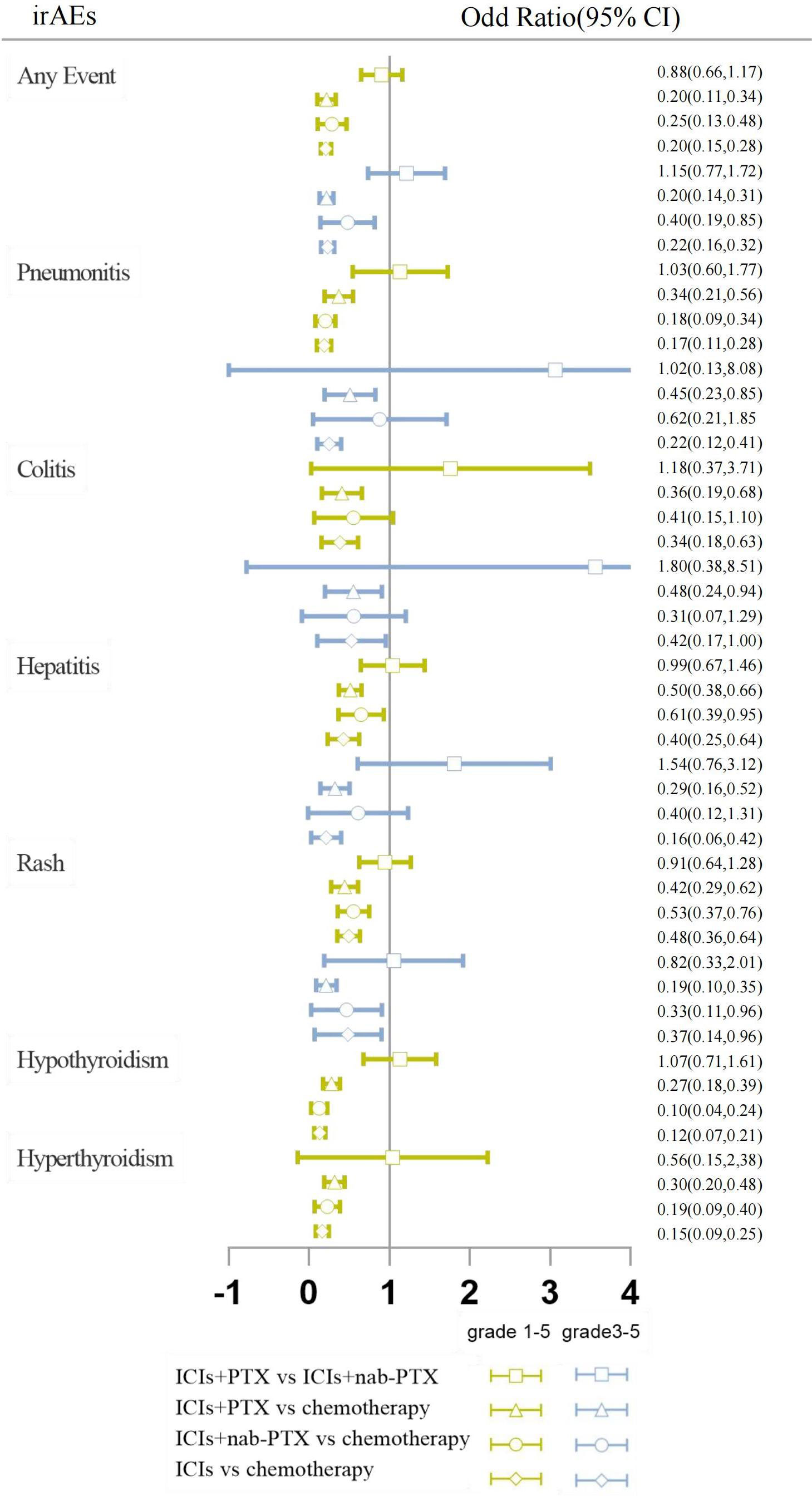
Figure 4 Forest plots results of head-to-head comparisons. The results are presented as odds ratios (ORs) with corresponding 95% confidence intervals (CIs). The vertical line represents the null effect, which is set at 1. The horizontal line depicts the CIs, and the hollow shape represents the point estimate, summarizing the ORs. When interpreting the forest plot for each pairwise comparison, it should be noted that if the hollow shape with the CI does not intersect with the vertical line of null effect, a statistically significant difference is observed. If the CI is on the left of the null effect, the event is significantly higher in the intervention arm, while if the CI is on the right, the event is statistically more frequent in the reference arm. If the CI intersects with the line of null effect, the difference between the two procedures is not statistically significant.
3.3 Comparison of irAEs
The network geometry and the contribution plots are reported in Supplement Figures 2, 3. While head-to-head direct meta-analyses are shown in Figure 4 and Supplementary Figure 1.
Based on the consistency model, the ORs for pairwise comparisons of irAEs are shown in Table 2. Almost all treatment regimens showed statistically significant differences with the chemotherapy group. The ICI+PTX had a significantly lower risk of grade 1-5 hyperthyroidism (OR=0.46, 95% CrI: 0.22-0.96) and grade 1-5 hypothyroidism (OR=0.49, 95% CrI: 0.26-0.93) than ICIs. Notably, comparing with ICI monotherapy, ICI+nab-PTX was associated with a decreased risk of grade 3-5 pneumonitis (OR=0.28, 95% CrI: 0.09,0.90).
The ranking analysis performed with SUCRA provided a ranking of each treatment group based on the incidence of irAEs, as shown in Table 3. The ICIs was associated with the worst safety ranking for grade 1-5 of any event (probability=79.3%), followed by ICI+PTX (70.8%), ICI+nab-PTX (49.9%), and finally chemotherapy (0%). The safety ranking for grade 3-5 of any adverse event was the same to irAEs: ICIs (84.6%), ICI+PTX (76.8%), ICI+nab-PTX (38.6%), and chemotherapy (0%). In addition, compared to the other three treatment groups, ICI monotherapy had the highest risk for causing pneumonitis (grade 1-5 and grade 3-5), colitis (grade 1-5), hepatitis (grade 1-5 and grade 3-5), hypothyroidism (grade 1-5) and hyperthyroidism (grade 3-5). The main irAEs caused by ICI+PTX was rare. ICI+nab-PTX mainly caused grade 3-5 colitis. Additionally, ICIs+nab-PTX has a lower risk of irAEs than ICIs+PTX. More detailed information can be found in Supplementary Table 4 and Supplementary Figures 4, 5.
3.4 Comparison of irAEs between PD-1 and PD-L1
The network geometry and the contribution plots are reported in Supplement Figures 2, 3. While head-to-head direct meta-analyses are shown in Supplementary Figure 1. Supplementary Table 3 presents the safety profiles of six treatment groups. For grade 1-5 irAEs, all treatment groups were associated with statistically significantly higher risks compared with chemotherapy. PD-1+PTX was associated with a statistically significantly lower risk of pneumonitis compared to PD-1 (OR=0.32, 95% CrI: 0.11-0.92). PD-L1+PTX showed a noticeably lower risk of rash compared to PD-1+PTX (OR=0.52, 95% CrI: 0.28-0.98). Additionally, PD-L1+PTX also presented a lower risk of hypothyroidism compared to PD-L1(OR=0.34, 95% CrI: 0.12-1.00). Of note, adding PTX to the treatment regimens can increase the risk of pneumonitis and hypothyroidism. Secondly, PD-1 showed a significantly higher risk than PD-L1.
Statistically substantial differences were observed only when comparing with chemotherapy groups for grade 3-5 irAEs. The ranking probability is presents in Table 3B, Supplementary Table 5 and Supplementary Figure 4, 5. The treatment groups containing PD-1 exhibited a higher risk of adverse reactions than those containing PD-L1.
4 Discussion
Immunotherapy has revolutionized treatment approaches for cancer, with ICIs combined with chemotherapy have shown remarkable clinical benefits, particularly the wide used nab-PTX/PTX combination strategies (13, 66). This large NMA is based on 22 RCTs including 15 963 patients. To our knowledge, this study is the first NMA that includes all cancers and provide important safety ranking of four treatment regimens involving ICI+ nab-PTX/PTX and a comparison of the safety profiles between PD-1 and PD-L1 inhibitors are provided as valuable references for clinical practice.
The nab-PTX was associated with unique advantages, such as without the use of a solvent, faster and greater tissue penetration, and slower elimination compared to PTX, which has made nab-PTX as the preferred option for combination therapy in the clinical settings (13). However, some studies have indicated that immunetherapy+chemotherapy may decrease the risk of irAEs (32, 33), while others have reached the opposite conclusion (32, 33, 67, 68). In our analysis, we found that the specific combination regimen of nab-PTX/PTX+ICIs is a safer therapeutic approach, significantly reducing the risk of irAEs occurrence. Moreover, nab-PTX demonstrates superior safety compared to PTX
The immune-related pneumonitis was associated with treatment discontinuation and mortality (69, 70). In this analysis, we specifically evaluated the immune-related pneumonitis. We found ICI monotherapy was linked to a higher risk of grade 3-5 immune‐related pneumonitis compared to nab-PTX+ICI, and comparing with PD-1, PD-1+PTX was associated with a statistically significant lower risk of grade 1-5 pneumonitis. In addition, ICI therapy was found to be associated with increased risks of grade 1-5 hypothyroidism and hyperthyroidism compared with PTX+ICI, while PD-L1+PTX presented a lower risk of hypothyroidism compared to PD-L1. In addition, according to the ranking results of irAEs, nab-PTX/PTX+ICI has a better safety profile than ICIs monotherapy for most irAEs, potentially reducing the risk of irAEs associated with ICIs. One possible reason is that in phase III clinical trials, the use of cytotoxic chemotherapy drugs is close to the maximum tolerated dose, leading to immune-suppressive (71). Consequently, the incidence of adverse events is low. Additionally, patients receiving PTX need to be pretreated with corticosteroids to prevent hypersensitivity (72), which can also suppress the immune system, reduce inflammation, and alleviate the development of irAEs (73). There may be other underlying mechanisms contributing to this phenomenon that require further investigation in basic research. In summary, our findings suggest that combining nab-PTX/PTX with ICIs offers a safer clinical treatment strategy.
For immune-related rash, PD-1+PTX was found to significantly increase the risk of grade 1-5 rash compared with PD-L1+PTX. In addition, according to the ranking of adverse reactions, our analysis found that the group containing PD-1 had s higher risk of irAEs compared to the group containing PD-L1, which is consistent with the previous research results (32, 74–76). In contrast, PD-1 antibody can simultaneously block the binding of PD-1 to both PD-L1 and PD-L2, resulting in a more comprehensive inhibition of the immune escape pathway and a higher incidence of irAEs (77). A previous study has reported that the competitive binding of PD-1 antibody to PD-L2 can disrupt the normal function of PD-L2 and other binding partners, leading to the activation of RGMb (repulsive guidance molecule) and subsequently cause pneumonitis (78). A recent study has demonstrated that exosomes derived from melanoma cells also express PD-L1. These exosomes, which contain PD-L1, travel through the bloodstream and can directly bind to the PD-1 receptor on the surface of T cells, resulting in T cell dysfunction. As a result, PD-L1 antibodies may be rendered ineffective by exosomal-PD-L1 before reaching the tumor cells. However, this issue does not arise with PD-1 antibodies, as they bind to the PD-1 receptor on T cells and exosomal-PD-L1 cannot neutralize their effects (79). These reasons may all contribute to the enhanced safety of PD-L1 inhibitors compared to PD-1 inhibitors. Numerous basic studies have reported a synergistic effect of taxane-combined immunotherapy (29, 80–82). This study further supports the potential benefits of this strategy in reducing the incidence of irAEs, providing a valuable guidance for clinical decision-making and serving as an evidence-based foundation for further basic research. However, there is still a lack of evidence of direct comparison. Therefore, further prospective RCTs and detailed basic research are needed to enrich the evidence.
Our study has several limitations. Frist, a standardized diagnostic criteria for irAEs is still lacking. In this study, irAEs data were extracted from “immune-related adverse events”. To obtain a more robust estimate of safety profile, we also extracted “treatment-related adverse events. In addition, although CTCAE 4.0 was the main version of adverse event evaluation criteria in all trials, we could not exclude the possibility that the different judgment criteria and grading strategies have been applied in the evaluation of irAEs. Second, the median follow-up time was varied among the trials included in the analysis, and it is possible that the reporting of irAEs with late-onset might be varied greatly. Third, the expression level of PD-L1 has been recognized as a potentially important and clinically valuable indicator for anti-PD-1 or anti-PD-L1 treatment (83, 84), but most trials failed to provide this important information. Fourth, the limited sample size of arms containing nab-PTX also prevents subgroup analysis, and the results involving nab-PTX should be interpreted with caution. Finally, for zero-events in any arm, STATA replaced them with the default value of 0.5, which increased the sample size per treatment by 1.
5 Conclusion
Our findings demonstrate that this combination therapies can significantly reduce the risk of immune-related adverse events, providing robust evidence to address the current controversial academic issues and offering clinical decision-making guidance for cancer patients. Furthermore, this study also confirms previous research findings that anti-PD-L1 inhibitors are safer than anti-PD-1 inhibitors and it demonstrates ICIs+nab-PTX has a lower risk of irAEs occurrence than ICIs+PTX.
Author contributions
Conception and design: WH, JY, and WC. Wrote the manuscript: WH. Acquired data: WH, JZ and YW. Analyzed the data: WH. Discussed the results and implications of findings: WH, JZ, YW, BF, and SJ. Drafting of the manuscript: WH. Review and editing: JY and WC. All authors contributed to the article and approved the submitted version.
Funding
This work was supported by grant from the National Natural Science Foundation of China [Grant No. 8217130423].
Conflict of interest
The authors declare that the research was conducted in the absence of any commercial or financial relationships that could be construed as a potential conflict of interest.
Publisher’s note
All claims expressed in this article are solely those of the authors and do not necessarily represent those of their affiliated organizations, or those of the publisher, the editors and the reviewers. Any product that may be evaluated in this article, or claim that may be made by its manufacturer, is not guaranteed or endorsed by the publisher.
Supplementary material
The Supplementary Material for this article can be found online at: https://www.frontiersin.org/articles/10.3389/fimmu.2023.1175809/full#supplementary-material
References
1. Homet Moreno B, Ribas A. Anti-programmed cell death protein-1/ligand-1 therapy in different cancers. Br J Cancer (2015) 112(9):1421–7. doi: 10.1038/bjc.2015.124
2. Hiam-Galvez KJ, Allen BM, Spitzer MH. Systemic immunity in cancer. Nat Rev Cancer (2021) 21(6):345–59. doi: 10.1038/s41568-021-00347-z
3. Huang C, Ren S, Chen Y, Liu A, Wu Q, Jiang T, et al. PD-L1 methylation restricts PD-L1/PD-1 interactions to control cancer immune surveillance. Sci Adv (2023) 9(21):eade4186. doi: 10.1126/sciadv.ade4186
4. de Miguel M, Calvo E. Clinical challenges of immune checkpoint inhibitors. Cancer Cell (2020) 38(3):326–33. doi: 10.1016/j.ccell.2020.07.004
5. Gettinger S, Horn L, Jackman D, Spigel D, Antonia S, Hellmann M, et al. Five-year follow-up of nivolumab in previously treated advanced non-Small-Cell lung cancer: results from the CA209-003 study. J Clin Oncol (2018) 36(17):1675. doi: 10.1200/JCO.2017.77.0412
6. Shergold AL, Millar R, Nibbs RJB. Understanding and overcoming the resistance of cancer to PD-1/PD-L1 blockade. Pharmacol Res (2019) 104258:145. doi: 10.1016/j.phrs.2019.104258
7. Brahmer JR, Tykodi SS, Chow LQ, Hwu WJ, Topalian SL, Hwu P, et al. Safety and activity of anti-PD-L1 antibody in patients with advanced cancer. N Engl J Med (2012) 366(26):2455–65. doi: 10.1056/NEJMoa1200694
8. Gubens MA, Davies M. NCCN guidelines updates: new immunotherapy strategies for improving outcomes in non-small cell lung cancer. J Natl Compr Canc Netw (2019) 17(5.5):574–8. doi: 10.6004/jnccn.2019.5005
9. Wolchok JD, Kluger H, Callahan MK, Postow MA, Rizvi NA, Lesokhin AM, et al. Nivolumab plus ipilimumab in advanced melanoma. N Engl J Med (2013) 369(2):122–33. doi: 10.1056/NEJMoa1302369
10. Zhang F, Huang D, Zhao L, Li T, Zhang SJ, Zhang GQ, et al. Efficacy and safety of PD-1/PD-L1 inhibitors plus nab-paclitaxel for patients with non-small cell lung cancer who have progressed after platinum-based chemotherapy. Ther Adv Med Oncol (2020) 12:1758835920936882. doi: 10.1177/1758835920936882
11. Heinhuis KM, Ros W, Kok M, Steeghs N, Beijnen JH, Schellens JHM. Enhancing antitumor response by combining immune checkpoint inhibitors with chemotherapy in solid tumors. Ann Oncol (2019) 30(2):219–35. doi: 10.1093/annonc/mdy551
12. Quartino AL, Friberg LE, Karlsson MO. A simultaneous analysis of the time-course of leukocytes and neutrophils following docetaxel administration using a semi-mechanistic myelosuppression model. Investigational New Drugs (2012) 30(2):833–45. doi: 10.1007/s10637-010-9603-3
13. Soliman HH. Nab-paclitaxel as a potential partner with checkpoint inhibitors in solid tumors. Oncotargets Ther (2017) 10:101–12. doi: 10.2147/OTT.S122974
14. Zitvogel L, Galluzzi L, Smyth MJ, Kroemer G. Mechanism of action of conventional and targeted anticancer therapies: reinstating immunosurveillance. Immunity (2013) 39(1):74–88. doi: 10.1016/j.immuni.2013.06.014
15. Guarneri V, Dieci MV, Conte P. Enhancing intracellular taxane delivery: current role and perspectives of nanoparticle albumin-bound paclitaxel in the treatment of advanced breast cancer. Expert Opin Pharmacother (2012) 13(3):395–406. doi: 10.1517/14656566.2012.651127
16. Taylor MH, Schmidt EV, Dutcus C, Pinheiro EM, Funahashi Y, Lubiniecki G, et al. The LEAP program: lenvatinib plus pembrolizumab for the treatment of advanced solid tumors. Future Oncol (2021) 17(6):637–48. doi: 10.2217/fon-2020-0937
17. Ettinger DS, Wood DE, Aisner DL, Akerley W, Bauman JR, Bharat A, et al. Non-small cell lung cancer, version 3.2022, NCCN clinical practice guidelines in oncology. J Natl Compr Canc Netw (2022) 20(5):497–530. doi: 10.6004/jnccn.2022.0025
18. Gradishar WJ, Moran MS, Abraham J, Aft R, Agnese D, Allison KH, et al. Breast cancer, version 3.2022, NCCN clinical practice guidelines in oncology. J Natl Compr Canc Netw (2022) 20(6):691–722. doi: 10.6004/jnccn.2022.0030
19. Tempero MA, Malafa MP, Al-Hawary M, Behrman SW, Benson AB, Cardin DB, et al. Pancreatic adenocarcinoma, version 2.2021, NCCN clinical practice guidelines in oncology. J Natl Compr Canc Netw (2021) 19(4):439–57. doi: 10.6004/jnccn.2021.0017
20. Scott AM, Wolchok JD, Old LJ. Antibody therapy of cancer. Nat Rev Cancer (2012) 12(4):278–87. doi: 10.1038/nrc3236
21. He X, Xu C. Immune checkpoint signaling and cancer immunotherapy. Cell Res (2020) 30(8):660–9. doi: 10.1038/s41422-020-0343-4
22. Friedman CF, Proverbs-Singh TA, Postow MA. Treatment of the immune-related adverse effects of immune checkpoint inhibitors: a review. JAMA Oncol (2016) 2(10):1346–53. doi: 10.1001/jamaoncol.2016.1051
23. Teng FF, Li M, Yu JM. Radiation recall pneumonitis induced by PD-1/PD-L1 blockades: mechanisms and therapeutic implications. BMC Med (2020) 18(1):275. doi: 10.1186/s12916-020-01718-3
24. Postow MA, Sidlow R, Hellmann MD. Immune-related adverse events associated with immune checkpoint blockade. New Engl J Med (2018) 378(2):158–68. doi: 10.1056/NEJMra1703481
25. Michot JM, Bigenwald C, Champiat S, Collins M, Carbonnel F, Postel-Vinay S, et al. Immune-related adverse events with immune checkpoint blockade: a comprehensive review. Eur J Cancer (2016) 54:139–48. doi: 10.1016/j.ejca.2015.11.016
26. Xu C, Chen YP, Du XJ, Liu JQ, Huang CL, Chen L, et al. Comparative safety of immune checkpoint inhibitors in cancer: systematic review and network meta-analysis. Bmj-Brit Med J (2018) 363:k4226. doi: 10.1136/bmj.k4226
27. Sears CR, Peikert T, Possick JD, Naidoo J, Nishino M, Patel SP, et al. Knowledge gaps and research priorities in immune checkpoint inhibitor-related pneumonitis an official American thoracic society research statement. Am J Resp Crit Care (2019) 200(6):E31–43. doi: 10.1164/rccm.201906-1202ST
28. Page DB, Postow MA, Callahan MK, Allison JP, Wolchok JD. Immune modulation in cancer with antibodies. Annu Rev Med (2014) 65:185. doi: 10.1146/annurev-med-092012-112807
29. Zhang J, Tang ZJ, Guo X, Wang YX, Zhou YH, Cai WM. Synergistic effects of nab-PTX and anti-PD-1 antibody combination against lung cancer by regulating the Pi3K/AKT pathway through the Serpinc1 gene. Front Oncol (2022) 12. doi: 10.3389/fonc.2022.933646
30. Garcia-Gonzalez J, Ruiz-Banobre J, Afonso-Afonso FJ, Amenedo-Gancedo M, Areses-Manrique MD, Campos-Balea B, et al. PD-(L)1 inhibitors in combination with chemotherapy as first-line treatment for non-Small-Cell lung cancer: a pairwise meta-analysis. J Clin Med (2020) 9(7):2093. doi: 10.3390/jcm9072093
31. Lv JW, Li JY, Luo LN, Wang ZX, Chen YP. Comparative safety and efficacy of anti-PD-1 monotherapy, chemotherapy alone, and their combination therapy in advanced nasopharyngeal carcinoma: findings from recent advances in landmark trials. J Immunother Cancer (2019) 7:159. doi: 10.1186/s40425-019-0636-7
32. Chen XR, Zhang ZH, Hou X, Zhang YX, Zhou T, Liu JQ, et al. Immune-related pneumonitis associated with immune checkpoint inhibitors in lung cancer: a network meta-analysis. J Immunother Cancer (2020) 8(2):e001170. doi: 10.1136/jitc-2020-001170
33. Wang MT, Liang HR, Wang W, Zhao S, Cai XY, Zhao Y, et al. Immune-related adverse events of a PD-(L)1 inhibitor plus chemotherapy versus a PD-(L)1 inhibitor alone in first-line treatment for advanced non-small cell lung cancer: a meta-analysis of randomized control trials. Cancer (2021) 127(5):777–86. doi: 10.1002/cncr.33270
34. Chang CY, Park H, Malone DC, Wang CY, Wilson DL, Yeh YM, et al. Immune checkpoint inhibitors and immune-related adverse events in patients with advanced melanoma: a systematic review and network meta-analysis. JAMA Netw Open (2020) 3(3):e201611. doi: 10.1001/jamanetworkopen.2020.1611
35. Sun YM, Li W, Chen ZY, Wang Y. Risk of pneumonitis associated with immune checkpoint inhibitors in melanoma: a systematic review and network meta-analysis. Front Oncol (2021) 11:651553. doi: 10.3389/fonc.2021.651553
36. Huang Y, Fan H, Li N, Du J. Risk of immune-related pneumonitis for PD1/PD-L1 inhibitors: systematic review and network meta-analysis. Cancer Med (2019) 8(5):2664–74. doi: 10.1002/cam4.2104
37. Page MJ, McKenzie JE, Bossuyt PM, Boutron I, Hoffmann TC, Mulrow CD, et al. The PRISMA 2020 statement: an updated guideline for reporting systematic reviews. Rev Esp Cardiol (2022) 75(2):192–. doi: 10.31222/osf.io/v7gm2
38. Higgins JPT, Altman DG, Gotzsche PC, Juni P, Moher D, Oxman AD, et al. The cochrane collaboration’s tool for assessing risk of bias in randomised trials. Bmj-Brit Med J (2011) f2914:343. doi: 10.1136/bmj.d5928
39. Mills EJ, Thorlund K, Ioannidis JPA. Demystifying trial networks and network meta-analysis. Bmj-Brit Med J (2013) f2914:346. doi: 10.1136/bmj.f2914
40. Baxi S, Yang A, Gennarelli RL, Khan N, Wang ZW, Boyce L, et al. Immune-related adverse events for anti-PD-1 and anti-PD-L1 drugs: systematic review and meta-analysis. Bmj-Brit Med J (2018) k973:360. doi: 10.1136/bmj.k793
41. Xu C, Zhou XQ, Zorzela L, Ju K, Furuya-Kanamori L, Lin LF, et al. Utilization of the evidence from studies with no events in meta-analyses of adverse events: an empirical investigation. BMC Med (2021) 19(1):e001170. doi: 10.1186/s12916-021-02008-2
42. Shim S, Yoon B-H, Shin I-S, Bae J-M. Network meta-analysis: application and practice using stata. Epidemiol Health (2017) 39:e2017047. doi: 10.4178/epih.e2017047
43. Song F, Altman DG, Glenny AM, Deeks JJ. Validity of indirect comparison for estimating efficacy of competing interventions: empirical evidence from published meta-analyses. Bmj-Brit Med J (2003) 326(7387):472–5. doi: 10.1136/bmj.326.7387.472
44. Socinski MA, Jotte RM, Cappuzzo F, Orlandi F, Stroyakovskiy D, Nogami N, et al. Atezolizumab for first-line treatment of metastatic nonsquamous NSCLC. New Engl J Med (2018) 378(24):2288–301. doi: 10.1056/NEJMoa1716948
45. Sugawara S, Lee JS, Kang JH, Kim HR, Inui N, Hida T, et al. Nivolumab with carboplatin, paclitaxel, and bevacizumab for first-line treatment of advanced nonsquamous non-small-cell lung cancer. Ann Oncol (2021) 32(9):1137–47. doi: 10.1016/j.annonc.2021.06.004
46. West H, McCleod M, Hussein M, Morabito A, Rittmeyer A, Conter HJ, et al. Atezolizumab in combination with carboplatin plus nab-paclitaxel chemotherapy compared with chemotherapy alone as first-line treatment for metastatic non-squamous non-small-cell lung cancer (IMpower130): a multicentre, randomised, open-label, phase 3 trial. Lancet Oncol (2019) 20(7):924–37. doi: 10.1016/S1470-2045(19)30167-6
47. Jotte R, Cappuzzo F, Vynnychenko I, Stroyakovskiy D, Rodriguez-Abreu D, Hussein M, et al. Atezolizumab in combination with carboplatin and nab-paclitaxel in advanced squamous NSCLC (IMpower131): results from a randomized phase III trial. J Thorac Oncol (2020) 15(8):1351–60. doi: 10.1016/j.jtho.2020.03.028
48. Moore KN, Bookman M, Sehouli J, Miller A, Anderson C, Scambia G, et al. Atezolizumab, bevacizumab, and chemotherapy for newly diagnosed stage III or IV ovarian cancer: placebo-controlled randomized phase III trial (IMagyn050/GOG 3015/ENGOT-OV39). J Clin Oncol (2021) 39(17):1842. doi: 10.1200/JCO.21.00306
49. Wang J, Lu S, Yu XM, Hu YP, Sun YP, Wang ZJ, et al. Tislelizumab plus chemotherapy vs chemotherapy alone as first-line treatment for advanced squamous non-Small-Cell lung cancer a phase 3 randomized clinical trial. JAMA Oncol (2021) 7(5):709–17. doi: 10.1001/jamaoncol.2021.0366
50. Monk BJ, Colombo N, Oza AM, Fujiwara K, Birrer MJ, Randall L, et al. Chemotherapy with or without avelumab followed by avelumab maintenance versus chemotherapy alone in patients with previously untreated epithelial ovarian cancer (JAVELIN ovarian 100): an open-label, randomised, phase 3 trial. Lancet Oncol (2021) 22(9):1275–89. doi: 10.1016/S1470-2045(21)00342-9
51. Powles T, van der Heijden MS, Castellano D, Galsky MD, Loriot Y, Petrylak DP, et al. Durvalumab alone and durvalumab plus tremelimumab versus chemotherapy in previously untreated patients with unresectable, locally advanced or metastatic urothelial carcinoma (DANUBE): a randomised, open-label, multicentre, phase 3 trial. Lancet Oncol (2020) 21(12):1574–88. doi: 10.1016/S1470-2045(20)30541-6
52. Sezer A, Kilickap S, Gumus M, Bondarenko I, Ozguroglu M, Gogishvili M, et al. Cemiplimab monotherapy for first-line treatment of advanced non-small-cell lung cancer with PD-L1 of at least 50%: a multicentre, open-label, global, phase 3, randomised, controlled trial. Lancet (2021) 397(10274):592–604. doi: 10.1016/S0140-6736(21)00228-2
53. Rizvi NA, Cho BC, Reinmuth N, Lee KH, Luft A, Ahn MJ, et al. Durvalumab with or without tremelimumab vs standard chemotherapy in first-line treatment of metastatic non-small cell lung cancer the MYSTIC phase 3 randomized clinical trial. JAMA Oncol (2020) 6(5):661–74. doi: 10.1001/jamaoncol.2020.0237
54. Hellmann MD, Ciuleanu TE, Pluzanski A, Lee JS, Otterson GA, Audigier-Valette C, et al. Nivolumab plus ipilimumab in lung cancer with a high tumor mutational burden. New Engl J Med (2018) 378(22):2093–104. doi: 10.1056/NEJMoa1801946
55. Herbst RS, Giaccone G, de Marinis F, Reinmuth N, Vergnenegre A, Barrios CH, et al. Atezolizumab for first-line treatment of PD-L1-Selected patients with NSCLC. New Engl J Med (2020) 383(14):1328–39. doi: 10.1056/NEJMoa1917346
56. Powles T, Csoszi T, Ozguroglu M, Matsubara N, Geczi L, Cheng SYS, et al. Pembrolizumab alone or combined with chemotherapy versus chemotherapy as first-line therapy for advanced urothelial carcinoma (KEYNOTE-361): a randomised, open-label, phase 3 trial. Lancet Oncol (2021) 22(7):931–45. doi: 10.1016/S1470-2045(21)00152-2
57. Shitara K, Van Cutsem E, Bang YJ, Fuchs C, Wyrwicz L, Lee KW, et al. Efficacy and safety of pembrolizumab or pembrolizumab plus chemotherapy vs chemotherapy alone for patients with first-line, advanced gastric cancer: the KEYNOTE-062 phase 3 randomized clinical trial. JAMA Oncol (2020) 6(10):1571–80. doi: 10.1001/jamaoncol.2020.3370
58. Reck M, Rodriguez-Abreu D, Robinson AG, Hui RN, Csoszi T, Fulop A, et al. Five-year outcomes with pembrolizumab versus chemotherapy for metastatic non-Small-Cell lung cancer with PD-L1 tumor proportion score >= 50%. J Clin Oncol (2021) 39(21):2339. doi: 10.1200/JCO.21.00174
59. Mok TSK, Wu YL, Kudaba I, Kowalski DM, Cho BC, Turna HZ, et al. Pembrolizumab versus chemotherapy for previously untreated, PD-L1-expressing, locally advanced or metastatic non-small-cell lung cancer (KEYNOTE-042): a randomised, open-label, controlled, phase 3 trial. Lancet (2019) 393(10183):1819–30. doi: 10.1016/S0140-6736(18)32409-7
60. Carbone DP, Reck M, Paz-Ares L, Creelan B, Horn L, Steins M, et al. First-line nivolumab in stage IV or recurrent non-Small-Cell lung cancer. New Engl J Med (2017) 376(25):2415–26. doi: 10.1056/NEJMoa1613493
61. Emens LA, Adams S, Barrios CH, Dieras V, Iwata H, Loi S, et al. First-line atezolizumab plus nab-paclitaxel for unresectable, locally advanced, or metastatic triple-negative breast cancer: IMpassion130 final overall survival analysis. Ann Oncol (2021) 32(8):983–93. doi: 10.1016/j.annonc.2021.05.355
62. Miles D, Gligorov J, Andre F, Cameron D, Schneeweiss A, Barrios C, et al. Primary results from IMpassion131, a double-blind, placebo-controlled, randomised phase III trial of first-line paclitaxel with or without atezolizumab for unresectable locally advanced/metastatic triple-negative breast cancer. Ann Oncol (2021) 32(8):994–1004. doi: 10.1016/j.annonc.2021.05.801
63. Schmid P, Cortes J, Pusztai L, McArthur H, Kummel S, Bergh J, et al. Pembrolizumab for early triple-negative breast cancer. New Engl J Med (2020) 382(9):810–21. doi: 10.1056/NEJMoa1910549
64. Shitara K, Ozguroglu M, Bang YJ, Di Bartolomeo M, Mandala M, Ryu MH, et al. Pembrolizumab versus paclitaxel for previously treated, advanced gastric or gastro-oesophageal junction cancer (KEYNOTE-061): a randomised, open-label, controlled, phase 3 trial. Lancet (2018) 392(10142):123–33. doi: 10.1016/S0140-6736(18)31257-1
65. Bellmunt J, de Wit R, Vaughn DJ, Fradet Y, Lee JL, Fong L, et al. Pembrolizumab as second-line therapy for advanced urothelial carcinoma. New Engl J Med (2017) 376(11):1015–26. doi: 10.1056/NEJMoa1613683
66. Schmid P, Adams S, Rugo HS, Schneeweiss A, Barrios CH, Iwata H, et al. Atezolizumab and nab-paclitaxel in advanced triple-negative breast cancer. New Engl J Med (2018) 379(22):2108–21. doi: 10.1056/NEJMoa1809615
67. Zhou XX, Yao ZR, Bai H, Duan JC, Wang ZJ, Wang X, et al. Treatment-related adverse events of PD-1 and PD-L1 inhibitor-based combination therapies in clinical trials: a systematic review and meta-analysis. Lancet Oncol (2021) 22(9):1265–74. doi: 10.1016/S1470-2045(21)00333-8
68. Carretero-Gonzalez A, Lora D, Ghanem I, Otero I, Lopez F, Castellano D, et al. Comparative safety analysis of immunotherapy combined with chemotherapy versus monotherapy in solid tumors: a meta-analysis of randomized clinical trials. Oncotarget (2019) 10(35):3294–301. doi: 10.18632/oncotarget.26908
69. Naidoo J, Page DB, Li BT, Connell LC, Schindler K, Lacouture ME, et al. Toxicities of the anti-PD-1 and anti-PD-L1 immune checkpoint antibodies. Ann Oncol (2015) 26(12):2375–91. doi: 10.1093/annonc/mdv383
70. Gangadhar TC, Vonderheide RH. Mitigating the toxic effects of anticancer immunotherapy. Nat Rev Clin Oncol (2014) 11(2):91–9. doi: 10.1038/nrclinonc.2013.245
71. Galluzzi L, Buque A, Kepp O, Zitvogel L, Kroemer G. Immunological effects of conventional chemotherapy and targeted anticancer agents. Cancer Cell (2015) 28(6):690–714. doi: 10.1016/j.ccell.2015.10.012
72. Yardley DA. Nab-paclitaxel mechanisms of action and delivery. J Control Release (2013) 170(3):365–72. doi: 10.1016/j.jconrel.2013.05.041
73. Gaucher L, Adda L, Sejourne A, Joachim C, Chaby G, Poulet C, et al. Impact of the corticosteroid indication and administration route on overall survival and the tumor response after immune checkpoint inhibitor initiation. Ther Adv Med Oncol (2021) 13:1758835921996656. doi: 10.1177/1758835921996656
74. Lin GF, Xu Y, Lin H, Yang DY, Chen L, Huang LL, et al. The association between the incidence risk of pneumonitis and PD-1/PD-L1 inhibitors in advanced NSCLC: a meta-analysis of randomized controlled trials. Int Immunopharmacol (2021) 99:108011. doi: 10.1016/j.intimp.2021.108011
75. Wang YC, Zhou SH, Yang F, Qi XY, Wang X, Guan XX, et al. Treatment-related adverse events of PD-1 and PD-L1 inhibitors in clinical trials a systematic review and meta-analysis. JAMA Oncol (2019) 5(7):1008–19. doi: 10.1001/jamaoncol.2019.0393
76. Yin JQ, Wu YJ, Yang X, Gan L, Xue JX. Checkpoint inhibitor pneumonitis induced by anti-PD-1/PD-L1 therapy in non-Small-Cell lung cancer: occurrence and mechanism. Front Immunol (2022) 13. doi: 10.3389/fimmu.2022.830631
77. Liu LH, Bai H, Wang C, Seery S, Wang ZJ, Duan JC, et al. Efficacy and safety of first-line immunotherapy combinations for advanced NSCLC: a systematic review and network meta-analysis. J Thorac Oncol (2021) 16(7):1099–117. doi: 10.1016/j.jtho.2021.03.016
78. Xiao YP, Yu SH, Zhu BG, Bedoret D, Bu X, Francisco LM, et al. RGMb is a novel binding partner for PD-L2 and its engagement with PD-L2 promotes respiratory tolerance. J Exp Med (2014) 211(5):943–59. doi: 10.1084/jem.20130790
79. Chen G, Huang AC, Zhang W, Zhang G, Wu M, Xu W, et al. Exosomal PD-L1 contributes to immunosuppression and is associated with anti-PD-1 response. Nature (2018) 560(7718):382–6. doi: 10.1038/s41586-018-0392-8
80. Peng J, Hamanishi J, Matsumura N, Abiko K, Murat K, Baba T, et al. Chemotherapy induces programmed cell death-ligand 1 overexpression via the nuclear factor-kappa b to foster an immunosuppressive tumor microenvironment in ovarian cancer. Cancer Res (2015) 75(23):5034–45. doi: 10.1158/0008-5472.CAN-14-3098
81. Grasselly C, Denis M, Bourguignon A, Talhi N, Mathe D, Tourette A, et al. The antitumor activity of combinations of cytotoxic chemotherapy and immune checkpoint inhibitors is model-dependent. Front Immunol (2018) 9. doi: 10.3389/fimmu.2018.02100
82. Markovic SN, Suman VJ, Javed A, Reid JM, Wall DJ, Erickson LA, et al. Sequencing ipilimumab immunotherapy before or after chemotherapy (Nab-paclitaxel and bevacizumab) for the treatment of BRAFwt (BRAF wild-type) metastatic malignant melanoma results of a study of academic and community cancer research united (ACCRU) RU261206I. Am J Clin Oncology-Cancer Clin Trials (2020) 43(2):115–21. doi: 10.1097/COC.0000000000000644
83. Zhou Z-J, Zhan P, Song Y. PD-L1 over-expression and survival in patients with non-small cell lung cancer: a meta-analysis. Trans Lung Cancer Res (2015) 4(2):203–8. doi: 10.3978/j.issn.2218-6751.2015.03.02
Keywords: immune-related adverse events, systematic literature review, network meta-analysis, immune checkpoint inhibitors, nab-paclitaxel, paclitaxel
Citation: Hao W, Zhang J, Wang Y, Fang B, Jin S, Yuan J and Cai W (2023) Immune-related adverse events associated with nab-paclitaxel/paclitaxel combined with immune checkpoint inhibitors: a systematic review and network meta-analysis. Front. Immunol. 14:1175809. doi: 10.3389/fimmu.2023.1175809
Received: 28 February 2023; Accepted: 23 June 2023;
Published: 14 July 2023.
Edited by:
Deborah L. Burnett, Garvan Institute of Medical Research, AustraliaReviewed by:
Aranzazu Garcia-Grande, Hospital Universitario Puerta de Hierro Majadahonda, SpainYihang Qi, Chinese Academy of Medical Sciences and Peking Union Medical College, China
Copyright © 2023 Hao, Zhang, Wang, Fang, Jin, Yuan and Cai. This is an open-access article distributed under the terms of the Creative Commons Attribution License (CC BY). The use, distribution or reproduction in other forums is permitted, provided the original author(s) and the copyright owner(s) are credited and that the original publication in this journal is cited, in accordance with accepted academic practice. No use, distribution or reproduction is permitted which does not comply with these terms.
*Correspondence: Weimin Cai, weimincai@fudan.edu.cn; Jing Yuan, jyuan@fudan.edu.cn