- 1Department of Oncology, Hospital of Chengdu University of Traditional Chinese Medicine, Chengdu, China
- 2Department of Infectious Diseases, Hospital of Chengdu University of Traditional Chinese Medicine, Chengdu, China
- 3Traditional Chinese Medicine (TCM) Regulating Metabolic Diseases Key Laboratory of Sichuan Province, Hospital of Chengdu University of Traditional Chinese Medicine, Chengdu, China
- 4Clinical School of Medicine, Chengdu University of Traditional Chinese Medicine, Chengdu, China
The corona virus disease 2019 (COVID-19) global pandemic has had an unprecedented and persistent impact on oncological practice, especially for patients with lung cancer, who are more vulnerable to the virus than the normal population. Indeed, the onset, progression, and prognosis of the two diseases may in some cases influence each other, and inflammation is an important link between them. The original chronic inflammatory environment of lung cancer patients may increase the risk of infection with COVID-19 and exacerbate secondary damage. Meanwhile, the acute inflammation caused by COVID-19 may induce tumour progression or cause immune activation. In this article, from the perspective of the immune microenvironment, the pathophysiological changes in the lungs and whole body of these special patients will be summarised and analysed to explore the possible immunological storm, immunosuppression, and immune escape phenomenon caused by chronic inflammation complicated by acute inflammation. The effects of COVID-19 on immune cells, inflammatory factors, chemokines, and related target proteins in the immune microenvironment of tumours are also discussed, as well as the potential role of the COVID-19 vaccine and immune checkpoint inhibitors in this setting. Finally, we provide recommendations for the treatment of lung cancer combined with COVID-19 in this special group.
1 Introduction
Lung cancer is the second most diagnosed cancer and the leading cause of cancer deaths worldwide, to which 1.8 million deaths were attributed in 2020 (1, 2). As a public health concern, chronic inflammation is an important mechanism of lung cancer initiation, progression, and metastasis. Meanwhile, in patients with lung cancer, the long-term secretion of inflammatory substances also creates a special tumour immune microenvironment (TIME) and lung pathological changes (3). Cytotoxic chemotherapy has long been the main route of systemic drug therapy for lung cancer; however, it also destroys the body’s immune defence system while killing cancer cells. With the development of therapeutic technologies, targeted therapy and immunotherapy allow more patients to gradually achieve long-term tumorigenic survival through site-directed attack and immune activation.
In late 2019, there was an outbreak of corona virus disease 2019 (COVID-19) and a rapid worldwide mass spread ensued. As a single positive-strand RNA virus, severe acute respiratory syndrome coronavirus 2 (SARS-CoV-2), the causative agent of COVID-19, is constantly subject to variation, and currently, variants such as Alpha, Beta, Gamma, Delta, and Omicron are mainly found (4). The infection and replication of the virus provoke an acute inflammatory response, which can promote body self-protection and damage repair. However, an imbalanced immune response may also destroy normal tissues, causing acute lung injury, multiple organ failure, and even death. Among the various subgroups of patients, tumour patients are a special population who are often under systemic immunosuppression due to the primary lesion and anticancer treatment (5). Lung cancer patients are more susceptible to COVID-19 pneumonia than the normal population (6).
Indeed, the tumour cells themselves are in the TIME, and in this unhealed wound, a moment is accompanied by a chronic inflammatory response (7). Therefore, with the introduction of COVID-19, in addition to the original chronic inflammatory basis, patients with lung cancer were also faced with SARS-CoV-2-mediated activation of many immune cells, which led to host-pathogen interactions different from those in normal individuals (Figure 1). This article describes the changes and potential mechanisms of TIME after the consolidation of COVID-19 in lung cancer patients and discusses the therapeutic protective measures for this special population, hoping to provide a reference for future studies.
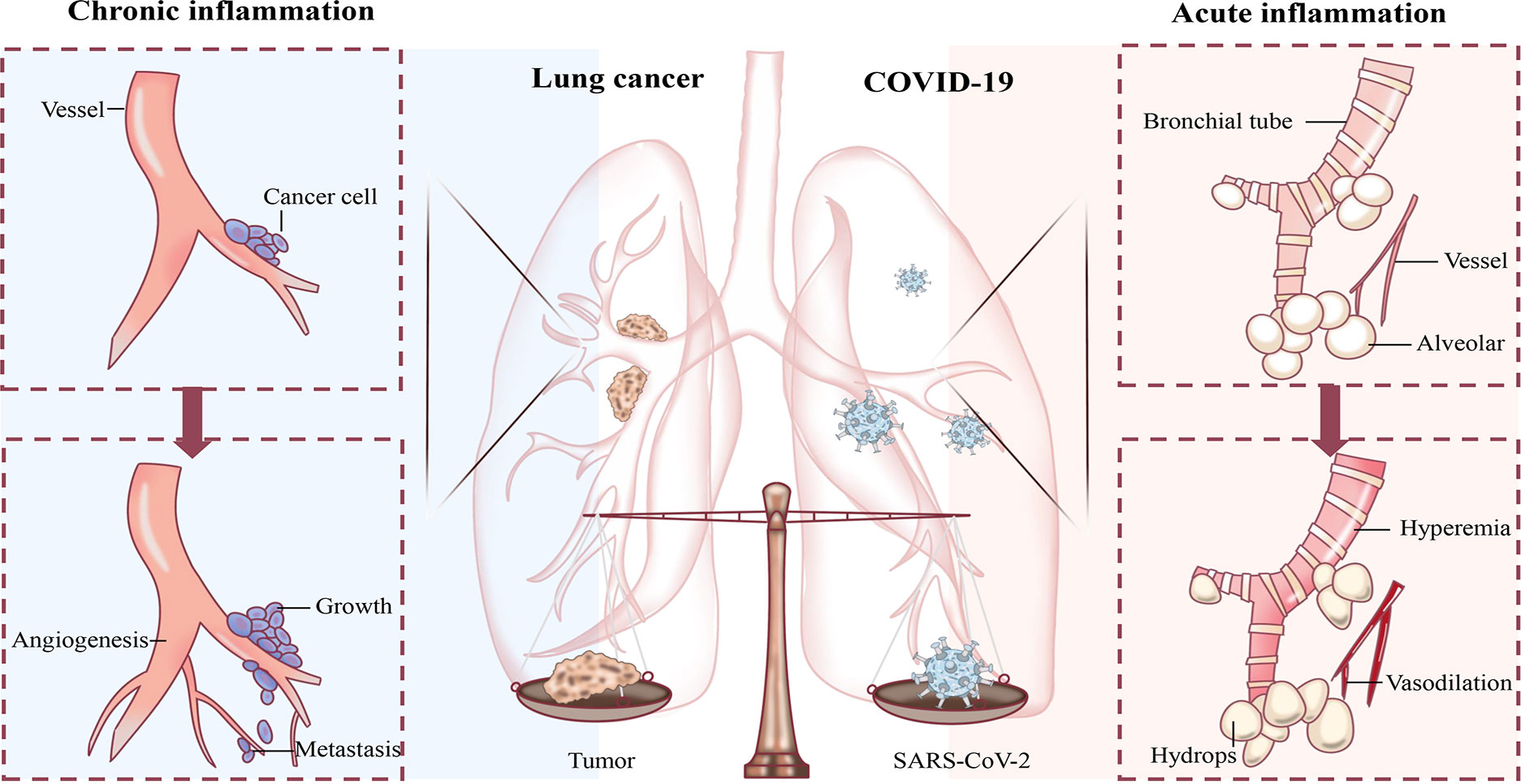
Figure 1 Schematic diagram of the influence of inflammation on lung cancer and COVID-19. Chronic inflammation creates a suitable environment for lung cancer growth, proliferation, metastasis, as well as angiogenesis. The acute inflammation inflicted by COVID-19 dilates the alveolar wall and interstitial blood vessels with increased permeability, causing the accumulation of serous fluid in the alveolar space.
2 Lung cancer and chronic inflammation
The molecular pathways involved in lung cancer remain poorly defined, but in general, smoking, occupational and environmental exposure, air pollution, ionizing radiation are mainly implicated. The above external factors can induce gene alterations and cell malignant transformation, such as activating oncogenes KRAS, EGFR, ALK1 (8, 9) and silencing tumor suppressor genes P53, Rb1, UTX (10, 11). In addition, The lungs communicate with the external environment. To this end, the respiratory system forms a sophisticated set of defence mechanisms to filter or clear various adverse factors, such as secretory fluid and cilia within the lining of the respiratory tract, cough reflex, and alveolar surface proteins (12). However, the continuous occurrence of multiple types of infection sources in nature makes damage to lung tissue difficult to avoid, such as pathogenic microorganisms, toxins, pollutants, irritants, and allergenic sources. This enables a complex immune response between pro- and anti-inflammatory factors and contributes to tissue repair and organ damage as well as secondary pathophysiological alterations (Figure 2).
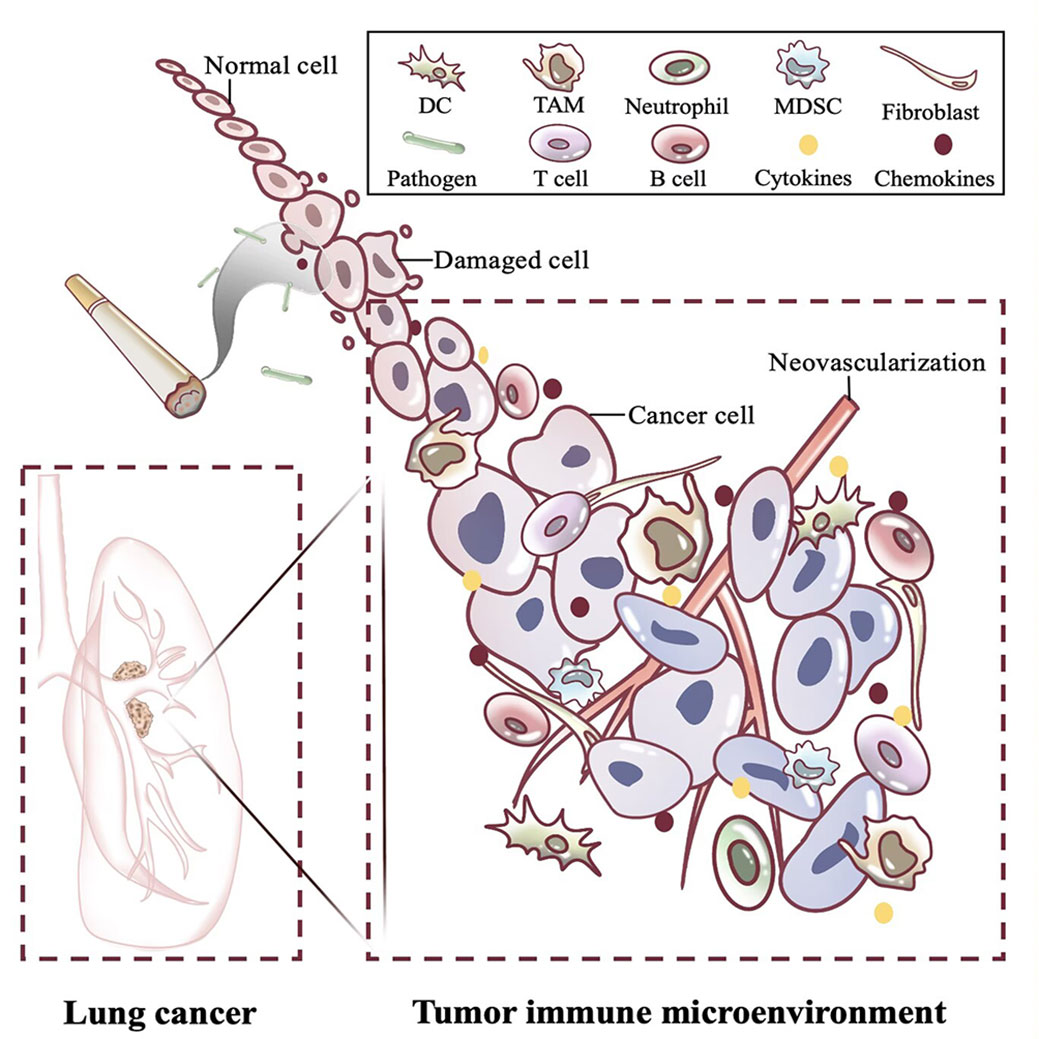
Figure 2 Changes in immune microenvironment of lung cancer caused by chronic inflammation. The long-term presence of carcinogenic factors such as smoking and pathogens causes lung cancer and shapes the tumor immune microenvironment. Around the tumor, inflammatory cells massively infiltrate and recruit cytokines and chemokines, which promote immune escape of the tumor through complex interactions. DC, Dendritic cell; TAM, Tumor macrophage; MDSC, Myeloid-derived suppressor cell; T cell, T lymphocyte cell; B cell, B lymphocyte cell.
2.1 Occurrence of lung cancer
In the 1990s, Rudolf Virchow proposed the hypothesis that chronic inflammation may induce tumours based on observations from tumour biopsies (13). Studies have confirmed that the occurrence of lung cancer is closely related to chronic inflammatory diseases of the lungs, and there is an association between the use of nonsteroidal anti-inflammatory drugs and a reduction in the incidence of lung cancer (14). Lung diseases, such as chronic obstructive pulmonary disease, tuberculosis, and interstitial lung disease, increase the risk of lung cancer. Long-term exposure to various pollutants such as external pathogens, tobacco smoke, and asbestos fibres may cause the release of various cytokines and growth factors that provide a selective growth advantage to mutant cells (15–17). In lung cancer, due to endogenous and exogenous high-risk factors, chronic inflammation is a common and important pathogenesis. Chronic inflammation in the lung can induce the accumulation of inflammatory cells, which produce many inflammatory cytokines and chemotactic molecules such as interleukin (IL)-1, IL-2, IL-6, IL-8, tumour necrosis factor-α (TNF-α), and cyclooxygenase 2 (COX2) (3). Their persistence and the cascade they cause can induce normal cell damage and immune dysregulation and create an intracellular environment that favours genotoxic damage and lung carcinogenesis (18, 19).
2.2 Progression of lung cancer
Chronic inflammation triggers lung carcinogenesis and is an important driver of lung cancer progression. Faget et al. (20) found that in lung cancer models, neutrophils, tumour-associated macrophages, and T and B cells infiltrated greatly outside the tumour tissue, which was beneficial to tumour growth. Inflammatory cells together with fibroblasts, endothelial cells, and extracellular matrix in the tumour stroma constitute TIME. TIME has a pro-tumorigenic effect. Developing tumour cells produce cytokines and chemokines to recruit inflammatory components, such as leukocytes (21). In addition, accumulated genetic changes may contribute to the malignant development of some tumours, and the chronic inflammatory response is considered an important endogenous source of mutational events (22). The inflammatory component shapes the potentially genotoxic environment by releasing chemicals, especially the sustained expression of COX-2, to maintain the abnormal status of tumours and accelerate their malignant evolution (23).
2.3 Metastasis of lung cancer
The delicate balance between inflammation and anti-inflammation is essential for the homeostatic maintenance of the lungs. In tumour patients, the TIME boundary is chronically populated with inflammatory cells, which produce extracellular matrix degrading enzymes such as matrix metalloproteinases and other pro-invasive growth factors (24, 25). For example, macrophages can suppress antitumor immunity, stimulate angiogenesis, and tumour escape, while preparing the target tissue for the arrival of tumour cells at the site of invasion (25). Lung cancer activates macrophages via Toll-like receptor (TLR) family member TLRs and produces TNF-α, and the creation of an inflammatory environment favours the occurrence of metastasis (26). In lung cancer tissue, the chemokine receptor system is also significantly altered, and in addition to regulating the inflammatory state, exerts direct effects on tumour progression, as CXCR2 ligands are closely associated with vascular growth and spontaneous migration (27). At later stages of the tumorigenic process, in the face of high levels of chemokines and cytokines, the host immune response is weakened or blunted, and failure to upregulate anti-inflammatory cytokines allows tumour cells to evade immune destruction and achieve infiltration and metastasis.
In recent years, studies on the relationship between inflammation and lung cancer have been in-depth. We consider the need to further identify the most critical inflammatory drivers affecting the TIME, taking into account the inflammatory response in different patients, in order to more precisely improve the efficacy of lung cancer treatment.
3 Three phases of COVID-19 and acute inflammation
COVID-19 is caused by SARS-CoV-2 and is mainly divided into three temporal phases: acute infection, post-acute hyperinflammatory disease, and late inflammatory and viral sequelae (28). For most young and healthy people, the adverse effects caused by COVID-19 are relatively mild and have a good prognosis; however, a few patients have rapid progression and are critically ill, mostly seen in older individuals with chronic underlying diseases (29) (Figure 3).
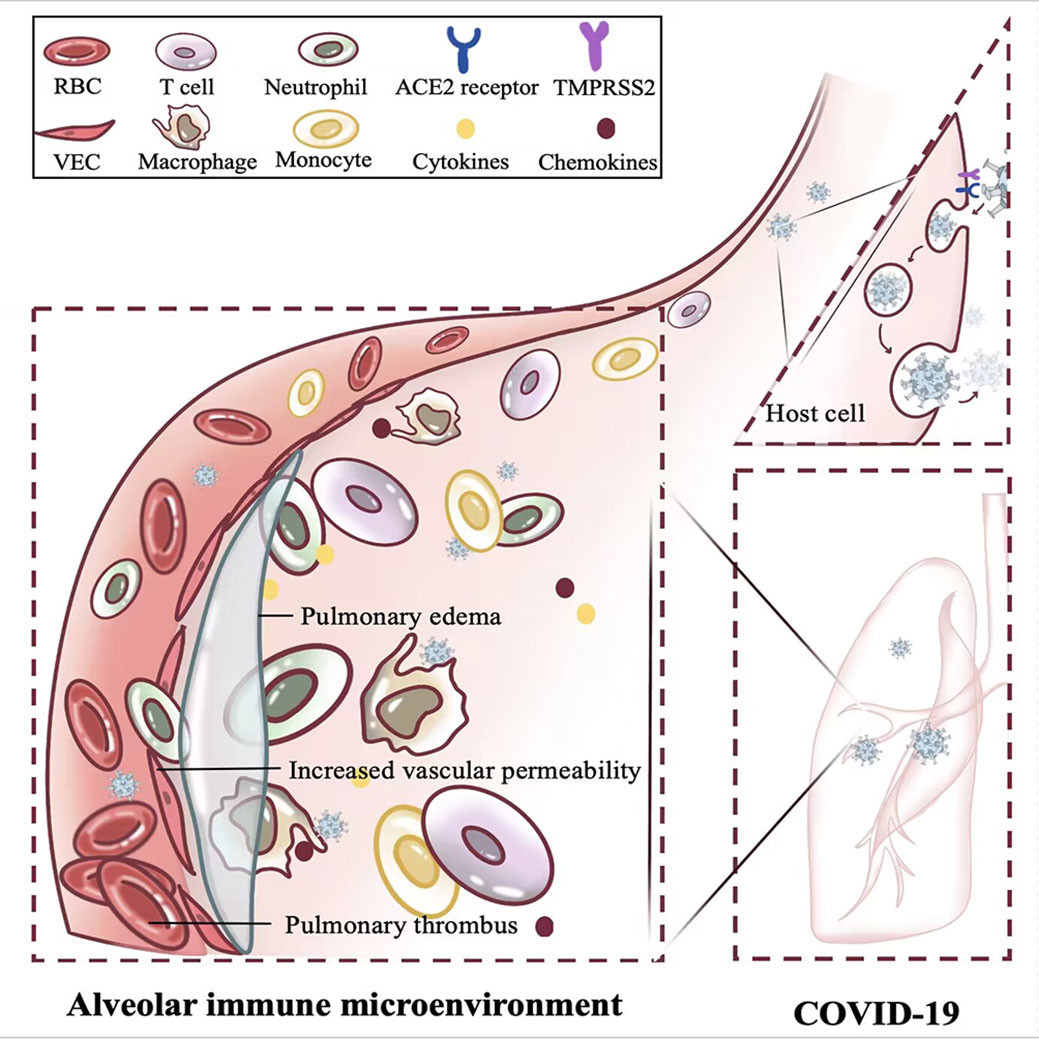
Figure 3 Effect of acute inflammation on alveolar immune microenvironment. The rapid replication of SARS-CoV-2 within the host induces acute inflammation in the lung, confers increased vascular permeability, heightened thrombotic risk, and triggers pulmonary edema. RBC, Red blood cell; T cell, T lymphocyte cell; ACE2, Angiotensin-converting enzyme 2; TMPRSS2, Transmembrane serine protease 2; VEC, Vascular endothelial cell.
3.1 Acute infection
The duration of acute infection typically ranges from days to weeks (30). At disease onset, viral replication in the body triggers an initial host immune response with positive real-time reverse transcription quantitative polymerase chain reaction (RT-qPCR) and rapid antigen testing. Patients present with typical flu-like symptoms such as fever, cough, and shortness of breath (31). In mild-to-moderate SARS-CoV-2-infected individuals, IL-6, IL-10, and TNF-α levels were mildly increased or within the normal range during the acute inflammatory phase and decreased during recovery (32). Typically, type I interferon (IFN)-α/β is the first line of defence that restricts virus replicative propagation upon entering the body and is mainly derived from uninfected cells such as resident macrophages and other phagocytic cells (33). However, human coronavirus (hCoV) can inhibit the IFN-α/β-mediated innate immune response (34, 35), making it either a delayed response or severely impaired (36). Inside the organism, an inadequate early front-line defence mechanism gives a viral multiplier, promotes COVID-19 progression, and induces an acute inflammatory response that can also lead to macrophage activation syndrome-like pathology, posing a hidden risk for subsequent acute post-hyperinflammatory diseases (37).
3.2 Post-acute hyperinflammatory disease
Following acute infection, a minority of patients develop systemic inflammation, and although RT-qPCR testing may be negative in patients at this time, the virus continues to replicate in vivo (29). Among them, in severe COVID-19 patients requiring intensive care unit (ICU) admission, the condition may be further exacerbated by massive inflammatory cell infiltration and high levels of proinflammatory cytokines and chemokines (38, 39), which in severe cases can progress to acute lung injury, acute respiratory distress syndrome (ARDS), or multiple organ failure (40). Respiratory failure due to ARDS is the leading cause of patient mortality (41). The lungs of these patients harbour a highly proinflammatory macrophage microenvironment (42), exhibiting significant elevation of IL-6, IL-8, IL-10, and TNF-α, and a decrease in CD4+ and CD8+ T cells, triggering a cytokine storm (32, 43, 44). In addition to a significant decrease in T cell counts, patients showed increased expression of PD-1 and Tim-3 on T cells, indicating that surviving T cells were functionally exhausted (45). This suggests that the cytokine storm may have suppressed the body’s adaptive immunity to viral infection.
3.3 Late inflammation and virological sequelae
Later in COVID-19 infection, the viral load decreases in the patient, and the associated symptoms generally resolve. However, increasing evidence suggests that in some patients after SARS-COV-2 infection, malaise, including tachycardia, fatigue, pain, and brain fog (46, 47). The aetiology of such symptoms is not fully defined but may be associated with the persistence of the later inflammatory state. Studies have found that peak viral levels remain detectable in plasma samples up to 12 months after diagnosis in patients with confirmed COVID-19 (48). Despite the ability of SARS-COV-2 to induce a systemic antiviral response, it also causes persistent inflammatory pathology well beyond the clearance of the primary infection (47). In addition, proinflammatory cytokines may establish a pathological proinflammatory feedback loop (TLR4/rage loop) during acute infection that remains operative even after viral clearance (49). This allows multiorgan and multisystem involvement and affects patient emotional status. Furthermore, Gold et al. (50) suggests that the prolonged presence of sequelae may not be a direct effect of SARS-COV-2 infection but a consequence of inflammation-induced Epstein–Barr virus reactivation in COVID-19.
Given the important role of inflammation in SARS-COV-2 infection, we speculate that combining antiviral and anti-inflammatory drugs may offer advantages over single agents, and natural products may provide inspiration in this regard. We believe that a comprehensive understanding of the immunological mechanism of infection and combining it with diagnosis and treatment will greatly advance the prevention and treatment of COVID-19.
4 Lung cancer patients with COVID-19
As of January 31, 2023 the World Health Organization has accumulated notifications of 753,479,439 confirmed cases and 6,812,798 deaths worldwide from COVID-19 (51). Owing to the limitations of detection capabilities across regions and the impact of death attribution, the true infection and mortality rates are likely to far exceed official statistics. In lung cancer patients, the presence of one or more additional comorbidities, such as age at presentation > 65 years, history of smoking, and presence of chronic obstructive pulmonary disease, can be confounded by the presence of several risk factors (52). This has led to increased morbidity and mortality when confronted with COVID-19.
4.1 Potential immunological impact of chronic inflammation in lung cancer on COVID-19
4.1.1 Increased risk of infection
Generally, inflammation is an organism’s resistance response to disease that is somewhat self-limiting, depending on the presence or absence of the threat. However, due to the presence of certain social, psychological, environmental, and biological factors, inflammation fails to resolve normally and instead converts into a low-grade, non-infectious chronic state (53). This distinguishes the immune components involved in the response from acute inflammation (54). The presence of chronic inflammation can lead to a breakdown of immune tolerance, impair the normal physiological function of tissue organs, and make the body more susceptible to infection, as well as a low response to vaccines (55). Patients with other underlying lung diseases, such as lung cancer, are more susceptible to infection because of the reduced clearance of the virus. Additionally, angiotensin-converting enzyme 2 (ACE2) and transmembrane serine protease 2 (TMPRSS2) are key molecules involved in the spread of SARS-CoV-2 (56). Viruses use the S protein to bind ACE2 on the surface of human cells to enter host cells, and TMPRSS2 promotes S protein activation, triggering fusion of viral and cellular membranes (56). Expression of ACE2 and TMPRSS2 is elevated in lung cancer survivors compared to non-cancer individuals (57, 58). This may be related to the susceptibility of lung cancer patients to the SARS-CoV-2 virus. Interestingly, the examination of resected tissue specimens (containing both tumor and normal part) from non-small cell lung cancer (NSCLC) patients found that ACE2 transcript detected in tumor part did not correlate with disease stage, whereas expression in normal part was higher in individuals with advanced stages (59).This suggests that normal lung tissue (but not tumour tissue) in NSCLC patients may be key to distinguishing between the expected low and high risk of severe COVID-19.
4.1.2 Exacerbated secondary injury
T cell antigen-mediated cellular immunity and humoral immunity can be provoked after SARS-CoV-2 infection in patients. An appropriate and timely immune response in most patients enables efficient clearance of SARS-CoV-2 and control of acute infection. Some groups experience prolonged exacerbation, which can be caused by excessive immune and inflammatory responses and is referred to as a cytokine storm. In lung cancer patients, chronic inflammation in the lung can cause a surge in proinflammatory immune responses, resulting in increased cytokine secretion by T cells and phagocytes (60). The TIME instead supports the SARS-CoV-2 protein by activating a cytokine storm and cell metabolism variant-related pathways, further accelerating the infection and weakening the immune system (61, 62). Compared to the general population, patients with lung cancer experience a further reduction in lung volume caused by the presence of neoplasias, which enables an earlier time to adverse effects in the case of inflammation (63). In addition, smoking, the most important high-risk factor for lung cancer (2), is associated with the severity of COVID-19 (64, 65). Smith et al. (66) found that chronic smoke exposure causes ACE2 content-dependent upregulation in the lungs of humans and animals, with protective expansion of mucus-secreting goblet cells. As a binding target of SARS-CoV-2, higher levels of ACE2 expression tend to be observed in the lung tissue of patients with severe COVID-19 (67). Downregulation of ACE2 due to binding may drive increased angiotensin II activity, contributing to pulmonary vasoconstriction and inflammatory and oxidative organ damage, thus contributing to acute lung injury and other systemic effect risks (68).
4.2 Potential immunological impact of acute inflammation of COVID-19 on lung cancer
4.2.1 Induction of tumour progression
Viral infection is thought to promote the growth of human cancers (69). Yan et al. (70) found that acute lung infection can significantly affect cancer cell homing to the lungs and lung metastasis, which may be associated with an altered lung immune microenvironment. The potential effects of the long-term prevalence of COVID-19 on lung cancer patients remain unknown, but some scholars propose (71, 72) that during acute inflammation, the immune homeostasis of tissues is disrupted, and severe COVID-19 may seriously worsen the prognosis of lung cancer patients by accelerating tumour progression (73), possibly induce the reactivation of dormant cancer cells, and increase the risk of cancer recurrence. For lung cancer patients, ACE2 and the renin-angiotensin system to which it belongs can inhibit tumour cell growth, control inflammation, and VEGF production, thus maintaining vascular homeostasis (74). Reduction of ACE2 by virus binding may then lead to NF-κB overactivation, stimulate angiogenesis and immunosuppression over time, and promote neoplastic processes (74, 75). Moreover, in patients with severe COVID-19, the cytokine storm is mainly associated with a massive production of proinflammatory cytokines (i.e. IL-1β, IL-6, and TNF-α) (44, 76). IL-1β can create an inflammatory microenvironment that favours tumour initiation and promotion, leading to an increased risk of lung cancer. Similar to IL-1β, high levels of IL-6 may contribute to the growth, metastasis, and immune escape of lung cancer cells (77).
4.2.2 Improved control condition
Severe COVID-19 is considered an acute hyperinflammatory disease characterised by massive immune cell activation, and its resulting lung infection has a certain negative impact on lung cancer patients. However, cases of an improved immune response to tumours after COVID-19 infection in sporadic cancer patients (78, 79), or even reports of improved responses to refractory cancer after mRNA vaccination, have also appeared (80). This may be related to the fact that, in the context of acute inflammation, rapid fluctuations in cytokines allow the immune system to be reactivated. For example, IFN-α can increase CD27+ and CD8+ T cell infiltration in the TIME to combat immune escape through competition for glucose metabolism (81). Additionally, it was found that the S1 protein of SARS-CoV-2 can activate the NF-κB signalling pathway in lung cancer cells, increase the expression of proinflammatory factors such as TNF-α, and thus induce lung cancer cell death (82). Second, COVID-19 acute infection prompts neutrophils to be recruited to the lungs in prophase, which may be a source of excessive neutrophil extracellular traps (NETs) (83). In mouse models inoculated with Lewis lung carcinoma, large areas of necrotic neutrophils and NET-like structures would release cytotoxic substances, impairing tumour vascular integrity (84). Additionally, during vaccination, CD4+ T cells and exhausted CD8+ T cells may also be reactivated and in turn prevent myeloid-derived suppressor cells or recruit regulatory T cells, exerting antitumor effects (80). Notably, there are many common signalling pathways between COVID-19 and lung cancer (85), and it is difficult for individual case reports to comprehensively reflect the correlation between various complex effects. So we still recommend cancer patients to be protected and avoid active or passive infection.
4.3 Potential immunological impact of COVID-19 vaccine in lung cancer
The COVID-19 vaccine is an effective way to prevent SARS-CoV-2 infection, stimulating the formation of virus-neutralising antibodies while inducing cellular immunity (86). With the marketing and popularity of different types of vaccines (87), the Society of Oncology Science recommends preferential vaccination of patients with cancer (88, 89). However, there is limited information on the safety and efficacy of the vaccine in patients with cancer because vulnerable populations, including immunocompromised patients, are not involved in research development and clinical trials of the vaccine (90). Previous evidence (91) shows that among those using immunosuppressive drugs, less than half of the vaccines developed sufficient levels of antiviral antibodies, and that the mean antibody level in positive patients was two-fold lower than that in healthy controls. As a key population in which normal immunity is compromised, early studies have argued that cancer patients have impaired neutralising IgG responses to mRNA vaccines and reduced neutralising antibody responses (92). In addition, a study found that during anti-CTLA4 and anti-PD-1 combination immunotherapy, a lung cancer patient developed cytokine release syndrome due to a new crown vaccination, elevated cytokine levels (IL-6, IL-10, and IFN-γ), and grade 2 liver and kidney dysfunction (93). However, Hernandez et al. (94) believe that the COVID-19 vaccine is safe for patients with lung cancer, and most patients can generate immunity after the first and second doses, reducing the risk of infection.
Harada et al. (95) also provided safety data on the use of the COVID-19 vaccine in patients with advanced lung cancer receiving anticancer treatments, such as chemotherapy, immunotherapy, and targeted therapy, while also noting that vaccine-related side effects tend to increase in patients with lung cancer receiving cytotoxic chemotherapy. Therefore, we call for more adequate studies focusing on the effects of vaccines on humoral and cellular immunity in lung cancer patients.
5 Treatment of lung cancer combined with COVID-19
Currently, the COVID-19 pandemic promotes the development of health care systems and severely affects the care of patients with cancer. In lung cancer patients receiving antineoplastic therapy, COVID-19 may interfere with their original treatment plan. For example, chemotherapy may weaken the anti-disease ability of the immune system for a short time, and molecular targeted drugs or immunotherapy may trigger inflammatory changes in the lungs (96). In response to this, it is recommended that the risk-benefit ratio of the various treatments of COVID-19 should be thoroughly discussed with patients and arranged based on clinical prioritisation. For example, given the risk of infection posed by surgical resection and the potentially immunosuppressive state triggered by perioperative chemotherapy, reassessment of therapy is warranted for individual patients.
Several institutions have conducted studies on the impact of various anticancer treatments received by lung cancer patients combined with COVID-19, but these are limited by sample size, geographic region, and diagnostic discrimination and data timeliness; the results obtained from preliminary reports are not yet in agreement. Calles et al. (97) found that COVID-19 morbidity and mortality were higher among lung cancer patients receiving immune checkpoint inhibitors, but no statistically significant differences were observed in either COVID-19 morbidity or mortality by treatment type. In contrast, another study concluded that cancer patients who had received antineoplastic treatments including chemotherapy, radiotherapy, targeted therapy, and immunotherapy within 14 days before COVID-19 diagnosis were at higher risk of developing serious adverse events (63). In response to this, two meta-analyses suggested that chemotherapy increases the risk of death in cancer patients with COVID-19, while immunotherapy, targeted therapy, surgery, and radiotherapy showed no significant differences in safety (98, 99).
In addition, immune checkpoint inhibitors (ICIs) have gained increasing attention because of their potential impact on the disease course of COVID-19 in cancer immunotherapy, where they either act directly on immune checkpoints and are able to enhance the immune response or relieve immunosuppression (100, 101) (Table 1). Studies have confirmed that the use of ICIs in patients with lung cancer does not affect symptom severity during COVID-19 (103). Meanwhile, Hanna et al. (107) found that prolonged doses of ICIs had similar efficacy and toxicity compared with standard doses, which provided a reference for extended dosing regimens to reduce the risk of viral exposure triggered by visits in patients with lung cancer. Indeed, accumulating evidence suggests (108, 109) that ICIs may exert antiviral effects by enhancing T cell (CD4+ and CD8+) levels and activity, improving clinical outcomes in critically ill patients. It remains to be noted that during COVID-19, ICIs may trigger an excessive release of cytokines, contributing to a systemic inflammatory disease, and we recommend close attention to therapeutic reflexes of patients.
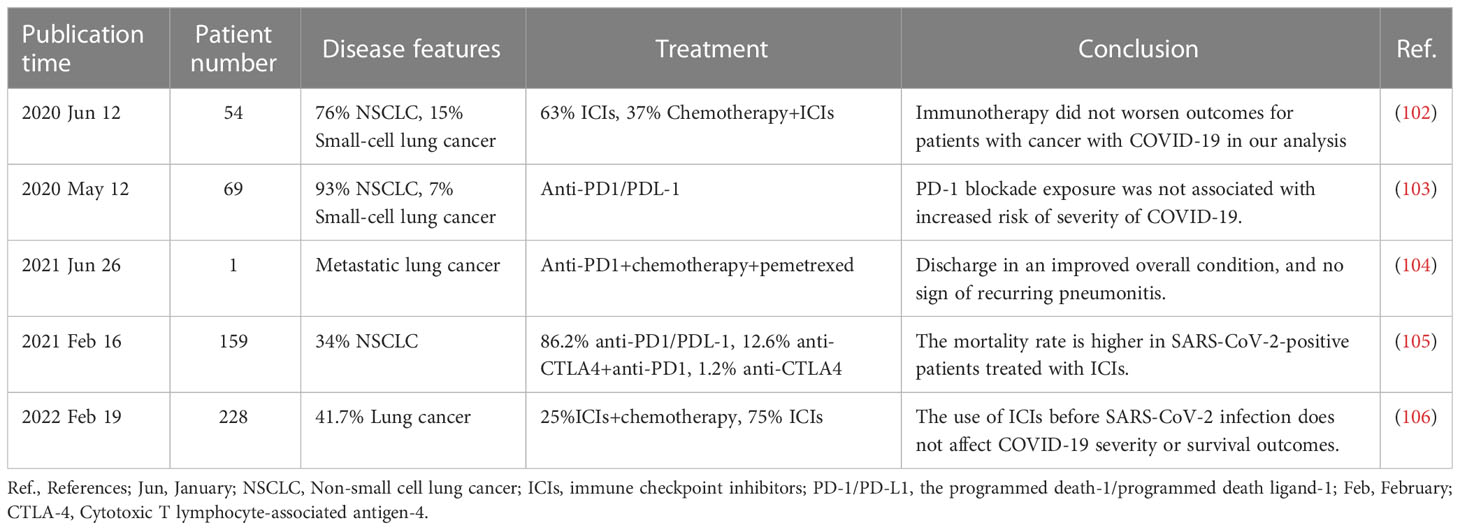
Table 1 Summary of the impact of immune checkpoint inhibitors on lung cancer combined with Corona Virus Disease 2019 (COVID-19).
6 Conclusions
Lung cancer is one of the most threatening malignancies to population health, and its relevance began to be recognised after COVID-19 caused a public health emergency that swept the globe. Analysis data showed that patients with lung cancer had a poor prognosis, higher rates of death (HR = 2.00 [95% CI 1.52, 2.63], p < 0.01) and severe infection (HR = 1.47 [95%CI 1.06, 2.03], p = 0.02) compared to patients without cancer (110). As discussed here, the onset, progression, and prognosis of the two diseases are not isolated and may, in some cases, affect each other and are causal. For example, lung cancer is a risk factor for COVID-19 infection and adverse outcomes, and the COVID-19 pandemic may worsen the condition in lung cancer patients (111–113). Among them, inflammation is an important link; it has a bifacial nature and can not only promote immune responses but also lead to immunosuppression. Some scholars have suggested (114) that although the pathological manifestations of acute inflammation and chronic inflammation are different, the driving mechanism of the two is relevant. As such, starting with acute inflammatory diseases may improve the diagnosis and treatment of chronic inflammation; conversely, therapies developed for chronic diseases may be beneficial for acute inflammation. Given the complex relationship between lung cancer and COVID-19, we think it is necessary to gain further insight into the roles and effects of acute and chronic inflammation-related cytokines, chemokines, and inflammatory mediators to provide more scientific treatment options and a basis for patients.
Author contributions
YA, Conceptualization, Supervision, Writing – original draft, Writing – review & editing. HW, Conceptualization, Supervision, Writing – original draft, Writing – review & editing. QZ, Conceptualization, Supervision, Writing – original draft, Writing – review & editing. SL, Writing, original draft, Writing – review & editing. JL Writing, original draft, Writing – review & editing. JH, Validation, Supervision, Writing – original draft, Writing – review & editing. JT, Conceptualization, Supervision, Writing – original draft, Writing – review & editing. XM, Conceptualization, Supervision, Writing – original draft, Writing – review & editing. All authors contributed to the article and approved the submitted version.
Funding
This project was supported by the National Natural Science Foundation of China (82204778). The Science and Technology Department of Sichuan Province, China [grant number: 2022YFG0145].
Conflict of interest
The authors declare that the research was conducted in the absence of any commercial or financial relationships that could be construed as a potential conflict of interest.
Publisher’s note
All claims expressed in this article are solely those of the authors and do not necessarily represent those of their affiliated organizations, or those of the publisher, the editors and the reviewers. Any product that may be evaluated in this article, or claim that may be made by its manufacturer, is not guaranteed or endorsed by the publisher.
References
1. Siegel RL, Miller KD, Fuchs HE, Jemal A. Cancer statistics, 2022. CA: Cancer J Clin (2022) 72(1):7–33. doi: 10.3322/caac.21708
2. Sung H, Ferlay J, Siegel RL, Laversanne M, Soerjomataram I, Jemal A, et al. Global cancer statistics 2020: Globocan estimates of incidence and mortality worldwide for 36 cancers in 185 countries. CA: Cancer J Clin (2021) 71(3):209–49. doi: 10.3322/caac.21660
3. Ballaz S, Mulshine JL. The potential contributions of chronic inflammation to lung carcinogenesis. Clin Lung Cancer (2003) 5(1):46–62. doi: 10.3816/CLC.2003.n.021
4. Kaklamanos A, Belogiannis K, Skendros P, Gorgoulis VG, Vlachoyiannopoulos PG, Tzioufas AG. Covid-19 immunobiology: Lessons learned, new questions arise. Front Immunol (2021) 12:719023. doi: 10.3389/fimmu.2021.719023
5. Huang X, Liang H, Zhang H, Tian L, Cong P, Wu T, et al. The potential mechanism of cancer patients appearing more vulnerable to sars-Cov-2 and poor outcomes: A pan-cancer bioinformatics analysis. Front Immunol (2021) 12:804387. doi: 10.3389/fimmu.2021.804387
6. Liang W, Guan W, Chen R, Wang W, Li J, Xu K, et al. Cancer patients in sars-Cov-2 infection: A nationwide analysis in China. Lancet Oncol (2020) 21(3):335–7. doi: 10.1016/s1470-2045(20)30096-6
7. Hanahan D, Weinberg RA. Hallmarks of cancer: The next generation. Cell (2011) 144(5):646–74. doi: 10.1016/j.cell.2011.02.013
8. Martínez-Jiménez F, Muiños F, Sentís I, Deu-Pons J, Reyes-Salazar I, Arnedo-Pac C, et al. A compendium of mutational cancer driver genes. Nat Rev Cancer (2020) 20(10):555–72. doi: 10.1038/s41568-020-0290-x
9. Wang Z, Xing Y, Li B, Li X, Liu B, Wang Y. Molecular pathways, resistance mechanisms and targeted interventions in non-Small-Cell lung cancer. Mol Biomed (2022) 3(1):42. doi: 10.1186/s43556-022-00107-x
10. Wu Q, Tian Y, Zhang J, Tong X, Huang H, Li S, et al. In vivo crispr screening unveils histone demethylase utx as an important epigenetic regulator in lung tumorigenesis. Proc Natl Acad Sci USA (2018) 115(17):E3978–e86. doi: 10.1073/pnas.1716589115
11. George J, Lim JS, Jang SJ, Cun Y, Ozretić L, Kong G, et al. Comprehensive genomic profiles of small cell lung cancer. Nature (2015) 524(7563):47–53. doi: 10.1038/nature14664
12. Kuroki Y, Takahashi M, Nishitani C. Pulmonary collectins in innate immunity of the lung. Cell Microbiol (2007) 9(8):1871–9. doi: 10.1111/j.1462-5822.2007.00953.x
13. Balkwill F, Mantovani A. Inflammation and cancer: Back to virchow? Lancet (London England) (2001) 357(9255):539–45. doi: 10.1016/s0140-6736(00)04046-0
14. Schreinemachers DM, Everson RB. Aspirin use and lung, colon, and breast cancer incidence in a prospective study. Epidemiol (Cambridge Mass) (1994) 5(2):138–46. doi: 10.1097/00001648-199403000-00003
15. Vainio H, Boffetta P. Mechanisms of the combined effect of asbestos and smoking in the etiology of lung cancer. Scandinavian J Work Environ Health (1994) 20(4):235–42. doi: 10.5271/sjweh.1402
16. Malik AA, Sheikh JA, Ehtesham NZ, Hira S, Hasnain SE. Can mycobacterium tuberculosis infection lead to cancer? call for a paradigm shift in understanding Tb and cancer. Int J Med Microbiol: IJMM (2022) 312(5):151558. doi: 10.1016/j.ijmm.2022.151558
17. Preda M, Tănase BC, Zob DL, Gheorghe AS, Lungulescu CV, Dumitrescu EA, et al. The bidirectional relationship between pulmonary tuberculosis and lung cancer. Int J Environ Res Public Health (2023) 20(2):1282. doi: 10.3390/ijerph20021282
18. Chung-man Ho J, Zheng S, Comhair SA, Farver C, Erzurum SC. Differential expression of manganese superoxide dismutase and catalase in lung cancer. Cancer Res (2001) 61(23):8578–85.
19. Yao H, Rahman I. Current concepts on the role of inflammation in copd and lung cancer. Curr Opin Pharmacol (2009) 9(4):375–83. doi: 10.1016/j.coph.2009.06.009
20. Faget J, Groeneveld S, Boivin G, Sankar M, Zangger N, Garcia M, et al. Neutrophils and snail orchestrate the establishment of a pro-tumor microenvironment in lung cancer. Cell Rep (2017) 21(11):3190–204. doi: 10.1016/j.celrep.2017.11.052
21. Coussens LM, Werb Z. Inflammation and cancer. Nature (2002) 420(6917):860–7. doi: 10.1038/nature01322
22. Schildkraut JM, Bastos E, Berchuck A. Relationship between lifetime ovulatory cycles and overexpression of mutant P53 in epithelial ovarian cancer. J Natl Cancer Inst (1997) 89(13):932–8. doi: 10.1093/jnci/89.13.932
23. Bauer AK, Dwyer-Nield LD, Malkinson AM. High cyclooxygenase 1 (Cox-1) and cyclooxygenase 2 (Cox-2) contents in mouse lung tumors. Carcinogenesis (2000) 21(4):543–50. doi: 10.1093/carcin/21.4.543
24. Kessenbrock K, Plaks V, Werb Z. Matrix metalloproteinases: Regulators of the tumor microenvironment. Cell (2010) 141(1):52–67. doi: 10.1016/j.cell.2010.03.015
25. Qian BZ, Pollard JW. Macrophage diversity enhances tumor progression and metastasis. Cell (2010) 141(1):39–51. doi: 10.1016/j.cell.2010.03.014
26. Weiss L, Ward PM. Lymphogenous and hematogenous metastasis of Lewis lung carcinoma in the mouse. Int J Cancer (1987) 40(4):570–4. doi: 10.1002/ijc.2910400423
27. Arenberg DA, Keane MP, DiGiovine B, Kunkel SL, Morris SB, Xue YY, et al. Epithelial-neutrophil activating peptide (Ena-78) is an important angiogenic factor in non-small cell lung cancer. J Clin Invest (1998) 102(3):465–72. doi: 10.1172/jci3145
28. Datta SD, Talwar A, Lee JT. A proposed framework and timeline of the spectrum of disease due to sars-Cov-2 infection: Illness beyond acute infection and public health implications. Jama (2020) 324(22):2251–2. doi: 10.1001/jama.2020.22717
29. Lino A, Cardoso MA, Gonçalves HMR, Martins-Lopes P. Sars-Cov-2 detection methods. Chemosensors (2022) 10(6):221. doi: 10.3390/chemosensors10060221
30. Tenforde MW, Billig Rose E, Lindsell CJ, Shapiro NI, Files DC, Gibbs KW, et al. Characteristics of adult outpatients and inpatients with covid-19 - 11 academic medical centers, united states, march-may 2020. MMWR Morbidity Mortality Weekly Rep (2020) 69(26):841–6. doi: 10.15585/mmwr.mm6926e3
31. Wiersinga WJ, Rhodes A, Cheng AC, Peacock SJ, Prescott HC. Pathophysiology, transmission, diagnosis, and treatment of coronavirus disease 2019 (Covid-19): A review. Jama (2020) 324(8):782–93. doi: 10.1001/jama.2020.12839
32. Liao M, Liu Y, Yuan J, Wen Y, Xu G, Zhao J, et al. The landscape of lung bronchoalveolar immune cells in covid-19 revealed by single-cell rna sequencing. MedRxiv (2020) 2020. 02. 23. 20026690. doi: 10.1101/2020.02.23.20026690
33. Grant RA, Morales-Nebreda L, Markov NS, Swaminathan S, Querrey M, Guzman ER, et al. Circuits between infected macrophages and T cells in sars-Cov-2 pneumonia. Nature (2021) 590(7847):635–41. doi: 10.1038/s41586-020-03148-w
34. Spiegel M, Pichlmair A, Martínez-Sobrido L, Cros J, García-Sastre A, Haller O, et al. Inhibition of beta interferon induction by severe acute respiratory syndrome coronavirus suggests a two-step model for activation of interferon regulatory factor 3. J Virol (2005) 79(4):2079–86. doi: 10.1128/jvi.79.4.2079-2086.2005
35. Kikkert M. Innate immune evasion by human respiratory rna viruses. J Innate Immun (2020) 12(1):4–20. doi: 10.1159/000503030
36. Hadjadj J, Yatim N, Barnabei L, Corneau A, Boussier J, Smith N, et al. Impaired type I interferon activity and inflammatory responses in severe covid-19 patients. Sci (New York NY) (2020) 369(6504):718–24. doi: 10.1126/science.abc6027
37. McGonagle D, Sharif K, O’Regan A, Bridgewood C. The role of cytokines including interleukin-6 in covid-19 induced pneumonia and macrophage activation syndrome-like disease. Autoimmun Rev (2020) 19(6):102537. doi: 10.1016/j.autrev.2020.102537
38. Zheng J, Wang Y, Li K, Meyerholz DK, Allamargot C, Perlman S. Severe acute respiratory syndrome coronavirus 2-induced immune activation and death of monocyte-derived human macrophages and dendritic cells. J Infect Dis (2021) 223(5):785–95. doi: 10.1093/infdis/jiaa753
39. Channappanavar R, Perlman S. Pathogenic human coronavirus infections: Causes and consequences of cytokine storm and immunopathology. Semin Immunopathol (2017) 39(5):529–39. doi: 10.1007/s00281-017-0629-x
40. Huang P, Zuo Q, Li Y, Oduro PK, Tan F, Wang Y, et al. A vicious cycle: In severe and critically ill covid-19 patients. Front Immunol (2022) 13:930673. doi: 10.3389/fimmu.2022.930673
41. Ruan Q, Yang K, Wang W, Jiang L, Song J. Clinical predictors of mortality due to covid-19 based on an analysis of data of 150 patients from wuhan, China. Intensive Care Med (2020) 46(5):846–8. doi: 10.1007/s00134-020-05991-x
42. Liao M, Liu Y, Yuan J, Wen Y, Xu G, Zhao J, et al. Single-cell landscape of bronchoalveolar immune cells in patients with covid-19. Nat Med (2020) 26(6):842–4. doi: 10.1038/s41591-020-0901-9
43. Zhang X, Tan Y, Ling Y, Lu G, Liu F, Yi Z, et al. Viral and host factors related to the clinical outcome of covid-19. Nature (2020) 583(7816):437–40. doi: 10.1038/s41586-020-2355-0
44. Coomes EA, Haghbayan H. Interleukin-6 in covid-19: A systematic review and meta-analysis. Rev Med Virol (2020) 30(6):1–9. doi: 10.1002/rmv.2141
45. Diao B, Wang C, Tan Y, Chen X, Liu Y, Ning L, et al. Reduction and functional exhaustion of T cells in patients with coronavirus disease 2019 (Covid-19). Front Immunol (2020) 11:827. doi: 10.3389/fimmu.2020.00827
46. Sudre CH, Murray B, Varsavsky T, Graham MS, Penfold RS, Bowyer RC, et al. Attributes and predictors of long covid. Nat Med (2021) 27(4):626–31. doi: 10.1038/s41591-021-01292-y
47. Frere JJ, Serafini RA, Pryce KD, Zazhytska M, Oishi K, Golynker I, et al. Sars-Cov-2 infection in hamsters and humans results in lasting and unique systemic perturbations after recovery. Sci Trans Med (2022) 14(664):eabq3059. doi: 10.1126/scitranslmed.abq3059
48. Swank Z, Senussi Y, Manickas-Hill Z, Yu XG, Li JZ, Alter G, et al. Persistent circulating severe acute respiratory syndrome coronavirus 2 spike is associated with post-acute coronavirus disease 2019 sequelae. Clin Infect Dis: an Off Publ Infect Dis Soc America (2023) 76(3):e487–e90. doi: 10.1093/cid/ciac722
49. Holms RD. Long covid (Pasc) is maintained by a self-sustaining pro-inflammatory Tlr4/Rage-loop of S100a8/A9 > Tlr4/Rage signalling, inducing chronic expression of il-1b, il-6 and tnfa: Anti-inflammatory ezrin peptides as potential therapy. Immuno (2022) 2(3):512–33. doi: 10.3390/immuno2030033
50. Gold JE, Okyay RA, Licht WE, Hurley DJ. Investigation of long COVID prevalence and its relationship to Epstein-Barr virus reactivation. Pathogens (2021) 10(6):763. doi: 10.3390/pathogens10060763
51. World Health Organization. Weekly epidemiological update on COVID-19 - 1 February 2023. (2023). Available at: https://www.who.int/publications/m/item/weekly-epidemiological-update-on-covid-19---1-february-2023 [Accessed March 17, 2023].
52. Bigdelou B, Sepand MR, Najafikhoshnoo S, Negrete JAT, Sharaf M, Ho JQ, et al. Covid-19 and preexisting comorbidities: Risks, synergies, and clinical outcomes. Front Immunol (2022) 13:890517. doi: 10.3389/fimmu.2022.890517
53. Straub RH. The brain and immune system prompt energy shortage in chronic inflammation and ageing. Nat Rev Rheumatol (2017) 13(12):743–51. doi: 10.1038/nrrheum.2017.172
54. Calder PC, Ahluwalia N, Albers R, Bosco N, Bourdet-Sicard R, Haller D, et al. A consideration of biomarkers to be used for evaluation of inflammation in human nutritional studies. Br J Nutr (2013) 109 Suppl 1:S1–34. doi: 10.1017/s0007114512005119
55. Furman D, Campisi J, Verdin E, Carrera-Bastos P, Targ S, Franceschi C, et al. Chronic inflammation in the etiology of disease across the life span. Nat Med (2019) 25(12):1822–32. doi: 10.1038/s41591-019-0675-0
56. Hoffmann M, Kleine-Weber H, Schroeder S, Krüger N, Herrler T, Erichsen S, et al. Sars-Cov-2 cell entry depends on Ace2 and Tmprss2 and is blocked by a clinically proven protease inhibitor. Cell (2020) 181(2):271–80.e8. doi: 10.1016/j.cell.2020.02.052
57. Wang Q, Li L, Qu T, Li J, Wu L, Li K, et al. High expression of Ace2 and Tmprss2 at the resection margin makes lung cancer survivors susceptible to sars-Cov-2 with unfavorable prognosis. Front Oncol (2021) 11:644575. doi: 10.3389/fonc.2021.644575
58. Kong Q, Xiang Z, Wu Y, Gu Y, Guo J, Geng F. Analysis of the susceptibility of lung cancer patients to sars-Cov-2 infection. Mol Cancer (2020) 19(1):80. doi: 10.1186/s12943-020-01209-2
59. Lazar V, Raynaud J, Magidi S, Bresson C, Martini JF, Galbraith S, et al. Comorbidity between lung cancer and covid-19 pneumonia: Role of immunoregulatory gene transcripts in high Ace2-expressing normal lung. Ther Adv Med Oncol (2022) 14:17588359221133893. doi: 10.1177/17588359221133893
60. Addeo A, Obeid M, Friedlaender A. Covid-19 and lung cancer: Risks, mechanisms and treatment interactions. J Immunother Cancer (2020) 8(1):e000892. doi: 10.1136/jitc-2020-000892
61. Tian S, Hu W, Niu L, Liu H, Xu H, Xiao SY. Pulmonary pathology of early-phase 2019 novel coronavirus (Covid-19) pneumonia in two patients with lung cancer. J Thorac Oncol: Off Publ Int Assoc Stud Lung Cancer (2020) 15(5):700–4. doi: 10.1016/j.jtho.2020.02.010
62. Malkani N, Rashid MU. Sars-Cov-2 infection and lung tumor microenvironment. Mol Biol Rep (2021) 48(2):1925–34. doi: 10.1007/s11033-021-06149-8
63. Zhang L, Zhu F, Xie L, Wang C, Wang J, Chen R, et al. Clinical characteristics of covid-19-Infected cancer patients: A retrospective case study in three hospitals within wuhan, China. Ann Oncol: Off J Eur Soc Med Oncol (2020) 31(7):894–901. doi: 10.1016/j.annonc.2020.03.296
64. Guan WJ, Ni ZY, Hu Y, Liang WH, Ou CQ, He JX, et al. Clinical characteristics of coronavirus disease 2019 in China. New Engl J Med (2020) 382(18):1708–20. doi: 10.1056/NEJMoa2002032
65. Cai H. Sex difference and smoking predisposition in patients with covid-19. Lancet Respir Med (2020) 8(4):e20. doi: 10.1016/s2213-2600(20)30117-x
66. Smith JC, Sheltzer JM. Cigarette smoke triggers the expansion of a subpopulation of respiratory epithelial cells that express the sars-Cov-2 receptor Ace2. Lancet Respir Med (2020) 8(4):e20.
67. Wu M, Chen Y, Xia H, Wang C, Tan CY, Cai X, et al. Transcriptional and proteomic insights into the host response in fatal covid-19 cases. Proc Natl Acad Sci USA (2020) 117(45):28336–43. doi: 10.1073/pnas.2018030117
68. South AM, Tomlinson L, Edmonston D, Hiremath S, Sparks MA. Controversies of renin-angiotensin system inhibition during the covid-19 pandemic. Nat Rev Nephrol (2020) 16(6):305–7. doi: 10.1038/s41581-020-0279-4
69. Oh JK, Weiderpass E. Infection and cancer: Global distribution and burden of diseases. Ann Global Health (2014) 80(5):384–92. doi: 10.1016/j.aogh.2014.09.013
70. Yan L, Cai Q, Xu Y. The ubiquitin-Cxcr4 axis plays an important role in acute lung infection-enhanced lung tumor metastasis. Clin Cancer Res: an Off J Am Assoc Cancer Res (2013) 19(17):4706–16. doi: 10.1158/1078-0432.Ccr-13-0011
71. Francescangeli F, De Angelis ML, Baiocchi M, Rossi R, Biffoni M, Zeuner A. Covid-19-Induced modifications in the tumor microenvironment: Do they affect cancer reawakening and metastatic relapse? Front Oncol (2020) 10:592891. doi: 10.3389/fonc.2020.592891
72. Francescangeli F, De Angelis ML, Zeuner A. Covid-19: A potential driver of immune-mediated breast cancer recurrence? Breast Cancer Res: BCR (2020) 22(1):117. doi: 10.1186/s13058-020-01360-0
73. Hattar K, Savai R, Subtil FS, Wilhelm J, Schmall A, Lang DS, et al. Endotoxin induces proliferation of nsclc in vitro and in vivo: Role of cox-2 and egfr activation. Cancer Immunol Immunother: CII (2013) 62(2):309–20. doi: 10.1007/s00262-012-1341-2
74. Feng Y, Wan H, Liu J, Zhang R, Ma Q, Han B, et al. The angiotensin-converting enzyme 2 in tumor growth and tumor-associated angiogenesis in non-small cell lung cancer. Oncol Rep (2010) 23(4):941–8. doi: 10.3892/or_00000718
75. Taniguchi K, Karin M. Nf-κb, inflammation, immunity and cancer: Coming of age. Nat Rev Immunol (2018) 18(5):309–24. doi: 10.1038/nri.2017.142
76. Liu J, Li S, Liu J, Liang B, Wang X, Wang H, et al. Longitudinal characteristics of lymphocyte responses and cytokine profiles in the peripheral blood of sars-Cov-2 infected patients. EBioMedicine (2020) 55:102763. doi: 10.1016/j.ebiom.2020.102763
77. Wang YC, Wu YS, Hung CY, Wang SA, Young MJ, Hsu TI, et al. Usp24 induces il-6 in tumor-associated microenvironment by stabilizing P300 and β-trcp and promotes cancer malignancy. Nat Commun (2018) 9(1):3996. doi: 10.1038/s41467-018-06178-1
78. Challenor S, Tucker D. Sars-Cov-2-Induced remission of Hodgkin lymphoma. Br J Haematol (2021) 192(3):415. doi: 10.1111/bjh.17116
79. Izaguirre-Pérez ME, Valencia-Salinas JJ, Mandujano-Sánchez JI, Landeros-Ruíz JP, Sánchez-García HV, Zaragoza-Valdez EP. Reducción inesperada de tumor torácico asociado a infección por sars-Cov-2. Revista Mexicana de Cirugía Torácica General (2022) 2(1):15–8.
80. Hua YJ, Liu YL, Wen K, Kurts C, Wu H, Mei Q, et al. Potentially improved response of covid-19 vaccinated nasopharyngeal cancer patients to combination therapy with anti-Pd-1 blockade and chemotherapy. Ann Oncol: Off J Eur Soc Med Oncol (2023) 34(1):121–3. doi: 10.1016/j.annonc.2022.10.002
81. Hu B, Yu M, Ma X, Sun J, Liu C, Wang C, et al. Ifnα potentiates anti-Pd-1 efficacy by remodeling glucose metabolism in the hepatocellular carcinoma microenvironment. Cancer Discovery (2022) 12(7):1718–41. doi: 10.1158/2159-8290.Cd-21-1022
82. Sheinin M, Jeong B, Paidi RK, Pahan K. Regression of lung cancer in mice by intranasal administration of sars-Cov-2 spike S1. Cancers (2022) 14(22):5648. doi: 10.3390/cancers14225648
83. Papayannopoulos V. Neutrophil extracellular traps in immunity and disease. Nat Rev Immunol (2018) 18(2):134–47. doi: 10.1038/nri.2017.105
84. Ho-Tin-Noé B, Carbo C, Demers M, Cifuni SM, Goerge T, Wagner DD. Innate immune cells induce hemorrhage in tumors during thrombocytopenia. Am J Pathol (2009) 175(4):1699–708. doi: 10.2353/ajpath.2009.090460
85. Zong Z, Wei Y, Ren J, Zhang L, Zhou F. The intersection of covid-19 and cancer: Signaling pathways and treatment implications. Mol Cancer (2021) 20(1):76. doi: 10.1186/s12943-021-01363-1
86. Sadarangani M, Marchant A, Kollmann TR. Immunological mechanisms of vaccine-induced protection against covid-19 in humans. Nat Rev Immunol (2021) 21(8):475–84. doi: 10.1038/s41577-021-00578-z
87. Callaway E. The next generation of coronavirus vaccines: A graphical guide. Nature (2023) 614(7946):22–5. doi: 10.1038/d41586-023-00220-z
88. Singh AP, Berman AT, Marmarelis ME, Haas AR, Feigenberg SJ, Braun J, et al. Management of lung cancer during the covid-19 pandemic. JCO Oncol Pract (2020) 16(9):579–86. doi: 10.1200/op.20.00286
89. Ribas A, Sengupta R, Locke T, Zaidi SK, Campbell KM, Carethers JM, et al. Priority covid-19 vaccination for patients with cancer while vaccine supply is limited. Cancer Discov (2021) 11(2):233–6. doi: 10.1158/2159-8290.Cd-20-1817
90. Polack FP, Thomas SJ, Kitchin N, Absalon J, Gurtman A, Lockhart S, et al. Safety and efficacy of the Bnt162b2 mrna covid-19 vaccine. New Engl J Med (2020) 383(27):2603–15. doi: 10.1056/NEJMoa2034577
91. Rabinowich L, Grupper A, Baruch R, Ben-Yehoyada M, Halperin T, Turner D, et al. Low immunogenicity to sars-Cov-2 vaccination among liver transplant recipients. J Hepatol (2021) 75(2):435–8. doi: 10.1016/j.jhep.2021.04.020
92. Fendler A, Shepherd STC, Au L, Wilkinson KA, Wu M, Byrne F, et al. Adaptive immunity and neutralizing antibodies against sars-Cov-2 variants of concern following vaccination in patients with cancer: The capture study. Nat Cancer (2021) 2(12):1305–20. doi: 10.1038/s43018-021-00274-w
93. Sumi T, Koshino Y, Michimata H, Nagayama D, Watanabe H, Yamada Y, et al. Cytokine release syndrome in a patient with non-small cell lung cancer on ipilimumab and nivolumab maintenance therapy after vaccination with the mrna-1273 vaccine: A case report. Trans Lung Cancer Res (2022) 11(9):1973–6. doi: 10.21037/tlcr-22-388
94. Hernandez A, Boigues M, Felip E, Cucurull M, Notario L, Pous A, et al. Immune response and effects of covid-19 vaccination in patients with lung cancer-covid lung vaccine study. Cancers (2022) 15(1):137. doi: 10.3390/cancers15010137
95. Harada D, Tamura T, Ninomiya K, Kubo T, Kuyama S, Tachibana S, et al. Safety of anti-Sars-Cov-2 messenger rna vaccine in lung cancer patients undergoing anticancer chemotherapy: A multicenter, prospective, observational, patient-reported outcome study. Thorac Cancer (2023) 14(3):231–6. doi: 10.1111/1759-7714.14737
96. Passaro A, Peters S, Mok TSK, Attili I, Mitsudomi T, de Marinis F. Testing for covid-19 in lung cancer patients. Ann Oncol: Off J Eur Soc Med Oncol (2020) 31(7):832–4. doi: 10.1016/j.annonc.2020.04.002
97. Calles A, Aparicio MI, Alva M, Bringas M, Gutierrez N, Soto J, et al. Outcomes of covid-19 in patients with lung cancer treated in a tertiary hospital in Madrid. Front Oncol (2020) 10:1777. doi: 10.3389/fonc.2020.01777
98. Yekedüz E, Utkan G, Ürün Y. A systematic review and meta-analysis: The effect of active cancer treatment on severity of covid-19. Eur J Cancer (Oxford England: 1990) (2020) 141:92–104. doi: 10.1016/j.ejca.2020.09.028
99. Liu H, Yang D, Chen X, Sun Z, Zou Y, Chen C, et al. The effect of anticancer treatment on cancer patients with covid-19: A systematic review and meta-analysis. Cancer Med (2021) 10(3):1043–56. doi: 10.1002/cam4.3692
100. Chen DS, Mellman I. Oncology meets immunology: The cancer-immunity cycle. Immunity (2013) 39(1):1–10. doi: 10.1016/j.immuni.2013.07.012
101. Blimark C, Holmberg E, Mellqvist UH, Landgren O, Björkholm M, Hultcrantz M, et al. Multiple myeloma and infections: A population-based study on 9253 multiple myeloma patients. Haematologica (2015) 100(1):107–13. doi: 10.3324/haematol.2014.107714
102. Garassino MC, Whisenant JG, Huang LC, Trama A, Torri V, Agustoni F, et al. Covid-19 in patients with thoracic malignancies (Teravolt): First results of an international, registry-based, cohort study. Lancet Oncol (2020) 21(7):914–22. doi: 10.1016/S1470-2045(20)30314-4
103. Luo J, Rizvi H, Egger JV, Preeshagul IR, Wolchok JD, Hellmann MD. Impact of pd-1 blockade on severity of covid-19 in patients with lung cancers. Cancer Discov (2020) 10(8):1121–8. doi: 10.1158/2159-8290.CD-20-0596
104. Mellinghoff SC, Vanshylla K, Dahlke C, Addo MM, Cornely OA, Klein F, et al. Case report: Clinical management of a patient with metastatic non-small cell lung cancer newly receiving immune checkpoint inhibition during symptomatic covid-19. Front Immunol (2021) 12:798276. doi: 10.3389/fimmu.2021.798276
105. Mandala M, Lorigan P, De Luca M, Bianchetti A, Merelli B, Bettini AC, et al. Sars-Cov-2 infection and adverse events in patients with cancer receiving immune checkpoint inhibitors: An observational prospective study. J Immunother Cancer (2021) 9(2):e001694. doi: 10.1136/jitc-2020-001694
106. Tan R, Yun C, Seetasith A, Sheinson D, Walls R, Ngwa I, et al. Impact of immune checkpoint inhibitors on covid-19 severity in patients with cancer. Oncologist (2022) 27(3):236–43. doi: 10.1093/oncolo/oyab083
107. Hanna L, Moffat GT, Hopman W, Gaudreau PO, Fung AS. A study of the efficacy and toxicity outcomes of extended durvalumab dosing in patients with stage iii unresectable non-small cell lung cancer (Nsclc) during the covid-19 pandemic. Cancer Treat Res Commun (2023) 34:100678. doi: 10.1016/j.ctarc.2022.100678
108. Krpina K, Mavrinac M, Samarzija M, Tolic E, Darapi D, Baticic L. Significance of the lung immune prognostic index for assessment of the reliability of the clinical treatment outcome for advanced non-Small-Cell lung cancer in patients with covid-19 infection. J Clin Med (2022) 11(22):6695. doi: 10.3390/jcm11226695
109. Xu X, Han M, Li T, Sun W, Wang D, Fu B, et al. Effective treatment of severe covid-19 patients with tocilizumab. Proc Natl Acad Sci USA (2020) 117(20):10970–5. doi: 10.1073/pnas.2005615117
110. Oldani S, Petrelli F, Dognini G, Borgonovo K, Parati MC, Ghilardi M, et al. Covid-19 and lung cancer survival: An updated systematic review and meta-analysis. Cancers (2022) 14(22):5706. doi: 10.3390/cancers14225706
111. Khoury E, Nevitt S, Madsen WR, Turtle L, Davies G, Palmieri C. Differences in outcomes and factors associated with mortality among patients with sars-Cov-2 infection and cancer compared with those without cancer: A systematic review and meta-analysis. JAMA Netw Open (2022) 5(5):e2210880. doi: 10.1001/jamanetworkopen.2022.10880
112. Rottoli M, Gori A, Pellino G, Flacco ME, Martellucci C, Spinelli A, et al. Colorectal cancer stage at diagnosis before vs during the covid-19 pandemic in Italy. JAMA Netw Open (2022) 5(11):e2243119. doi: 10.1001/jamanetworkopen.2022.43119
113. Flores R, Alpert N, McCardle K, Taioli E. Shift in lung cancer stage at diagnosis during the covid-19 pandemic in new York city. Trans Lung Cancer Res (2022) 11(7):1514–6. doi: 10.21037/tlcr-22-191
Keywords: lung cancer, COVID-19, inflammation, immunity, immune checkpoint inhibitor
Citation: Ai Y, Wang H, Zheng Q, Li S, Liu J, Huang J, Tang J and Meng X (2023) Add fuel to the fire: Inflammation and immune response in lung cancer combined with COVID-19. Front. Immunol. 14:1174184. doi: 10.3389/fimmu.2023.1174184
Received: 26 February 2023; Accepted: 13 March 2023;
Published: 23 March 2023.
Edited by:
Ping Yuan, Tongji University, ChinaCopyright © 2023 Ai, Wang, Zheng, Li, Liu, Huang, Tang and Meng. This is an open-access article distributed under the terms of the Creative Commons Attribution License (CC BY). The use, distribution or reproduction in other forums is permitted, provided the original author(s) and the copyright owner(s) are credited and that the original publication in this journal is cited, in accordance with accepted academic practice. No use, distribution or reproduction is permitted which does not comply with these terms.
*Correspondence: Jianyuan Tang, dGFuZ2p5QGNkdXRjbS5lZHUuY24=; Xiangrui Meng, am9uaWFzQDEyNi5jb20=
†These authors have contributed equally to this work and share first authorship