- 1Immuno-Genetics and Human Pathology Laboratory, Faculty of Medicine and Pharmacy, Hassan II University, Casablanca, Morocco
- 2Laboratory of Pathological Anatomy, University Hospital Center (CHU) Ibn Rochd, Hassan II University, Casablanca, Morocco
- 3Department of Neurosurgery, Faculty of Medicine and Pharmacy, University of Hassan II, Casablanca, Morocco
- 4Mohammed VI Center for Research and Innovation, Rabat, Morocco and Mohammed VI University of Sciences and Health, Casablanca, Morocco
Introduction: Among all types of central nervous system cancers, glioma remains the most frequent primary brain tumor in adults. Despite significant advances in immunomodulatory therapies, notably immune checkpoint inhibitors, their effectiveness remains constrained due to glioma resistance. The discovery of TMIGD2 (Transmembrane and Immunoglobulin Domain Containing 2) as an immuno-stimulatory receptor, constitutively expressed on naive T cells and most natural killer (NK) cells, has emerged as an attractive immunotherapy target in a variety of cancers. The expression profile of TMIGD2 and its significance in the overall survival of glioma patients remains unknown.
Methods: In the present study, we first assessed TMIGD2 mRNA expression using the Cancer Genome Atlas (TCGA) glioma transcriptome dataset (667 patients), followed by validation with the Chinese Glioma Genome Atlas (CGGA) cohort (693 patients). Secondly, we examined TMIGD2 protein staining in a series of 25 paraffin-embedded blocks from Moroccan glioma patients. The statistical analysis was performed using GraphPad Prism 8 software.
Results: TMIGD2 expression was found to be significantly higher in astrocytoma, IDH-1 mutations, low-grade, and young glioma patients. TMIGD2 was expressed on immune cells and, surprisingly, on tumor cells of glioma patients. Interestingly, our study demonstrated that TMIGD2 expression was negatively correlated with angiogenesis, hypoxia, G2/M checkpoint, and epithelial to mesenchymal transition signaling pathways. We also demonstrated that dendritic cells, monocytes, NK cells, gd T cells, and naive CD8 T cell infiltration correlates positively with TMIGD2 expression. On the other hand, Mantel-Cox analysis demonstrated that increased expression of TMIGD2 in human gliomas is associated with good overall survival. Cox multivariable analysis revealed that TMIGD2 is an independent predictor of a good prognosis in glioma patients.
Discussion: Taken together, our results highlight the tight implication of TMIGD2 in glioma progression and show its promising therapeutic potential as a stimulatory target for immunotherapy.
Introduction
Since 2015, new insights into the immunology of the central nervous system (CNS) have significantly redefined the concept of its immunological privilege (1). It is now clear that the CNS has a direct lymphatic drainage pathway with the presence of various antigen-presenting cells (APCs), and is therefore accessible to different arms of the immune system (2, 3). Immune checkpoints represent a delicate network of molecules involved in co-inhibitory and co-stimulatory pathways. They regulate the immune response, enabling both effective and protective immunity (4). The clinical use of immune checkpoint inhibitors to restore a potent anti-tumor immune response has demonstrated impressive therapeutic effects, and has thus revolutionized the paradigms of cancer immunotherapy (5). In the field of CNS, specifically brain cancer, the most widespread and malignant primary tumors are gliomas. Regardless of the rigorous mainstay of therapy, the prognosis of these tumors is still largely dismal with a median survival of 14.6 months and a 5-year survival rate of only 6.8%, especially for the deadliest and most resistant form, Glioblastoma (6–8). Moreover, current immunotherapy approaches have not improved outcomes in patients with glioma. The expected increase in median overall survival (OS) was not observed in the Phase II and Phase III clinical trials of the PD-1/PD-L1 inhibitors, nivolumab or durvalumab (9, 10). On the basis of these clinical observations, there is an urgent need to develop more effective immunostimulatory strategies and to search for new biomarkers to improve response rates in gliomas (11). Along with blocking inhibitory checkpoints, fostering co-stimulatory pathways strengthens the immune system and appears to have encouraging results in cancer (12). These co-stimulatory molecules can be expressed on activated T cells, where molecules like ICOS, OX40, CD137, and GITR are present, or on naïve T cells, where molecules like CD27 and CD28 are expressed (13–16).The third class of B7-CD28 immune checkpoint family is composed of B7-H3, B7x, HHLA2, and the recently identified receptor transmembrane and immunoglobulin domain containing 2 (TMIGD2) (17, 18). Initially known as immunoglobulin-containing and proline-rich receptor-1 (IGPR-1) and CD28 homolog (CD28H),TMIGD2 is a membranous glycoprotein belonging to the immunoglobulin superfamily (IgSF) (19). Furthermore, TMIGD2 was characterized as an HHLA2 receptor that induces significant co-stimulation in human T cell responses via increasing cell growth and cytokine production through an AKT-dependent signaling cascade, both in vitro and in vivo. Regarding expression on immune cells, the work of Zhu and colleagues emphasized the presence of TMIGD2 proteins constitutively on naive T cells, and most of NK cells (20). On the other hand, endothelial and epithelial cells have been reported to express TMIGD2, which plays a role in inhibiting cell migration and enhancing capillary tube development during angiogenesis (21). A recent study using B7H7+ tumor cells showed that TMIGD2 is an activator of NK cells and demonstrated its promising potential as a target to enhance anti-tumor activity (22). In gastric cancer, elevated TMIGD2 expression predicts a bad prognosis, mainly when its ligand HHLA2 is strongly co-expressed (23). Conversely, in gliomas and pancreatic ductal adenocarcinomas, tumors with high levels of HHLA2 expression had a better prognosis, suggesting that it may act as a co-stimulator through the TMIGD2 pathway (24, 25). However, the expression profile of the TMIGD2 immune checkpoint and its prognostic value in gliomas remain unknown. In the present study, we assessed TMIGD2 expression levels in glioma patients, demonstrating its potential use as a target for immunotherapy.
Materials and methods
Patients and samples
All patients underwent initial surgery between June 2016 and August 2021 at the department of neurosurgery, Ibn Rochd University Hospital, Casablanca, Morocco. All patients have accepted surgical treatment in the hospital and have been diagnosed with glioma. A total of six patients were diagnosed with a grade 1 glioma, five with a grade 2 glioma, seven with a grade 3 glioma, and seven with a grade 4 glioma. No patient has received chemotherapy or radiotherapy before surgery. At recruitment, a consent form was signed by each subject. Clinical and pathological data were obtained from a review of the patient’s medical records.
Immunohistochemistry
The evaluation of TMIGD2 expression by immunohistochemistry was carried out in accordance with the steps of the immunohistochemistry protocol described above (26).
The primary antibodies used in this study were rabbit polyclonal TMIGD2 (Rockland Immunochemicals, USA), Rabbit IgG Isotype control (Bioss Antibodies, USA). All paraffin-embedded tumor tissue specimens (n = 25) were collected from patients with glioma who underwent surgery at the Ibn Rochd University Hospital, neurosurgery department (Casablanca, Morocco). For positive control, paraffin blocks of the normal small intestine were collected from the Department of Pathological Anatomy at the CHU Ibn Rochd. First, the sections (5 µm thick) were incubated at 65°C for 1 hour and then overnight at 37°C. For the antigen retrieval step, sections were placed in PT Link (Dako, Denmark) bath in a high-pH retrieval solution (Envision Flex target retrieval solution, low PH (27) in 30 ml, Dako, Denmark) at 98°C for 20 min. Thereafter, the tissues were imbibed in 3% hydrogen peroxide (EnVision flex peroxidase-blocking reagent, Dako, Denmark) for 10 minutes and, then, incubated in a washing buffer (EnVision flex wash buffer, Dako, Denmark) to inhibit endogenous peroxidase activity. Next, the slides were incubated for 1 hour with the primary antibody rabbit anti human TMIGD2 antibody at a concentration of 2.5 μg/ml. For all cases, a rabbit IgG isotype control was used as a negative control at a 1:200 dilution. Subsequently, sections were incubated for 20 min at room temperature with secondary horseradish peroxidase-conjugated goat anti-rabbit anti-mouse IgG (EnVision Flex/HRP, Dako, USA). The slides were then stained with chromagen 3, 3’-diaminobenzidine (DAB) (EnVision DAB + CHROMOGEN, Dako, USA). Finally, all sections were soaked in hematoxylin for 3 minutes and dehydrated in alcohol solutions (75%, 95%, 100%), and mounted.
Evaluation of immunostaining
Cells stained positive for TMIGD2 in glioma specimens were counted on the 40x (objective) images of immune cells and glioma cells. The staining intensity (SI) was determined as follows: 0 (negative), 1 (weak), 2 (intermediate) or 3 (strong). The percentage of positive cells (PP) was scored as follows: 0%–100%. The value of SI×PP in each section was regarded as the IHC score of the TMIGD2 expression on immune cells and glioma cells 1.
Pathologists were blinded to sample identity. As a positive control tissue, a small intestine was used.
TCGA and CGGA datasets analysis
Clinical data and mRNA-seq for glioma patients were downloaded from public databases. The Cancer Genome Atlas (TCGA) dataset from https://www.cbioportal.org/contains 513 LGG samples and 154 GBM samples, ranging from WHO grades 2 to 4. As a validation cohort, we used data from the Chinese Glioma Genome Atlas (CGGA) http://www.cgga.org.cn/download.jsp) database, which includes 188 grade 2, 255 grade 3, and 249 grade 4. The clinical information included diagnosis, age, sex, cancer type, grade, IDH1 mutation, radiation therapy, vital status, and OS rates.
The immune cell abundance identifier
The ImmuCellAI (http://bioinfo.life.hust.edu.cn/web/ImmuCellAI/) was used to assess the relative abundance of 24 immune cells subpopulations in glioma patient’s microenvironment expressing a low or high level of TMIGD2.
Gene set enrichment analysis
A Gene Set Enrichment Analysis (GSEA) (https://software.broadinstitute.org/gsea/index.jsp) was performed using the R package “clusterProfiler” based on TCGA and CGGA datasets to determine the enriched biological processes in TMIGD2 groups. Enriched terms with a false discovery rate (FDR) < 0.05 were significant.
Statistical analysis
All data were analyzed using GraphPad Prism 8.0 software (GraphPad Software, Inc., La Jolla, CA, USA). The overall survival (OS) according to TMIGD2 expression profile was estimated by Log-rank (Mantel-Cox) test, and t-test was used to compare means between groups. The association between TMIGD2 expression and genes of interest was assessed using Spearman’s correlation analysis. A multivariable Cox analysis was performed to estimate the association between TMIGD2 expression and patient’s prognosis. The median expression level of TMIGD2 was used to categorized all patients. For all statistical methods, p<0.05 was considered as significant difference.
Ethics approval
A study of this project was approved by the ethical committee at the Ibn Rochd University Hospital of Casablanca. Regulations and guidlines were followed to implement the methods.
Results
TMIGD2 gene expression is up-regulated in low grade glioma tissues
To assess the association between TMIGD2 gene expression and clinical features of glioma patients, we analyzed transcriptomic data from the TCGA (248 grade 2, 264 grade 3, and 153 grade 4 patients) and the CGGA cohorts (188 grade 2, 255 grade 3, and 249 grade 4 patients) (Figure 1). The features of all patients in both datasets were described in (Table 1).
TMIGD2 expression was significantly higher in young patients than older (p< 0.0001) (Figures 2A, B). As compared to grade 2 and grade 3 gliomas, glioblastomas expressed a lower level of TMIGD2 transcript (p= 0.0006; p= 0.0038) (Figures 2C, D). Besides, TMIGD2 expression was upregulated in the astrocytoma subtype compared to glioblastoma (p< 0.0001) (Figures 2E, F). In the TCGA group, oligoastrocytomas and oligodendrogliomas displayed a high level of TMIGD2 than the glioblastomas subtype (p= 0.0010; p= 0.0010). Further, mutant IDH-1 patients exhibit significantly higher levels of TMIGD2 than IDH-1 wild-type patients (p< 0.0001) (Figures 2G, H). These results were well validated in the CGGA cohort.
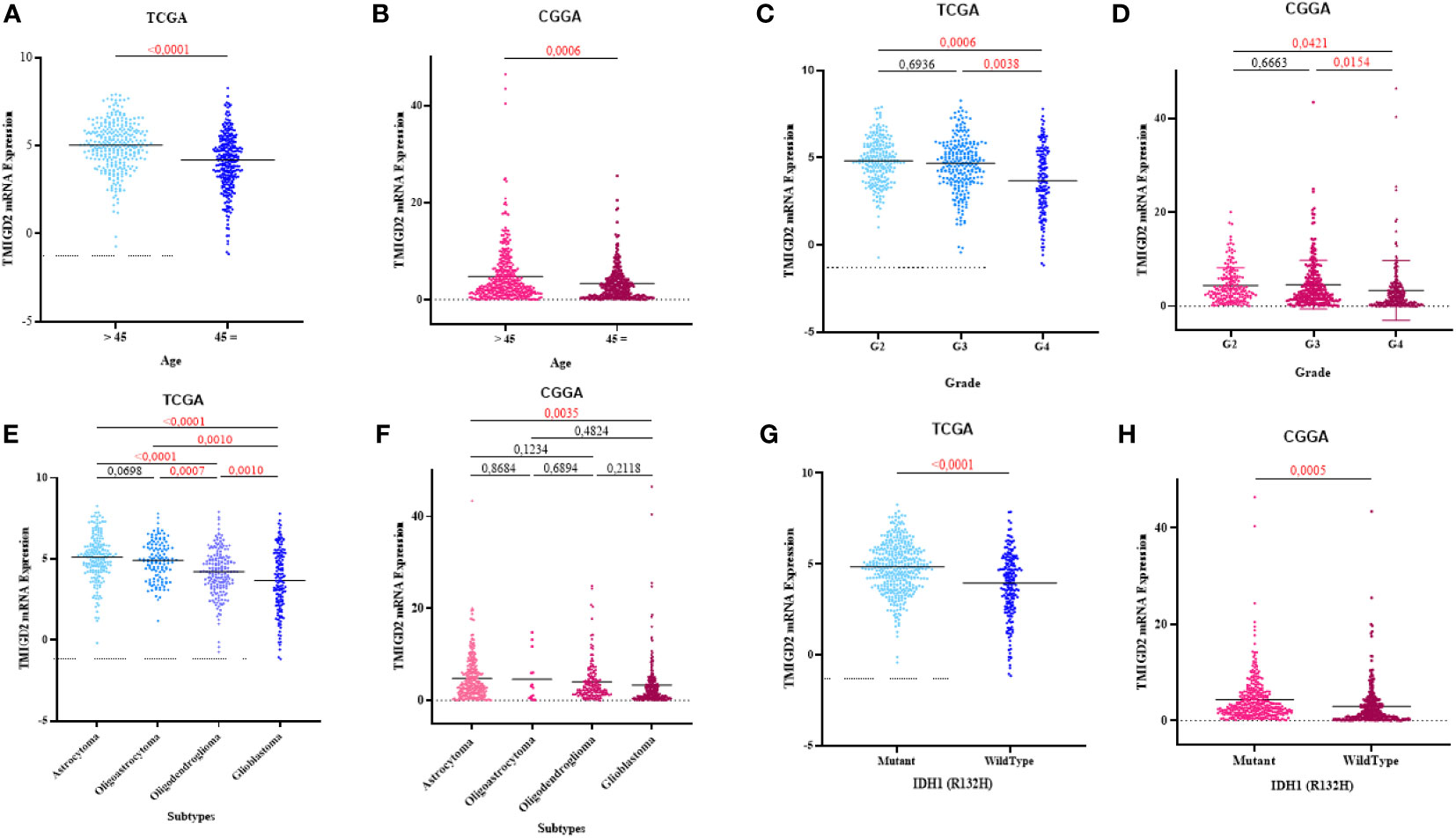
Figure 2 Association between TMIGD2 expression and clinical parameters of glioma in the TCGA and CGGA cohorts. TMIGD2 expression was significantly increased in young glioma patients (A, B). TMIGD2 was highly expressed in grade 2 gliomas at the transcription level compared with grade 3 gliomas and glioblastoma (GBM, WHO grade 4) (C, D). The astrocytoma group had a higher expression of TMIGD2 in TCGA and CGGA datasets (E, F). TMIGD2 was enriched in IDH1 mutant glioma (G, H).
To corroborate the results of TMIGD2 expression obtained in the transcript level (Figure 1), TMIGD2 protein analysis was performed on 25 glioma samples (6 Grade I, 5 Grade II, 7 Grade III, and 7 Grade 4) by immunohistochemistry (Table 2).
The normal small intestine has been reported to constitutively express the TMIGD2 protein (21). When the IgG was used as a negative control, neither the small intestine (Figure 3A) nor the glioma samples stained (Figures 3B–E). TMIGD2 staining was observed in the normal small intestine tissue once an anti-TMIGD2 mAb was applied under identical circumstances (positive control) (Figure 3F). TMIGD2 protein staining was higher in glioma grades 1 and 2 (Figures 3G, H) as opposed to grades 3 and 4 (Figures 3I, J). Interestingly, TMIGD2 protein expression was upregulated on infiltrating immune cells and tumor cells in LGG patients (p= 0.0012; p= 0.0029) (Figures 3K, N). Additionally, neither immune nor tumor cells with wild-type or muted IDH-1 displayed statistical differences in TMIGD2 expression (p= 0.0685; 0.1592) (Figures 3L, O). Moreover, our results showed that astrocytomas are enriched with immune cells expressing high levels of TMIGD2, unlike oligodendrogliomas and glioblastomas subtypes (p= 0.0091; p= 0.0006) (Figure 3M). On tumor cells, however, TMIGD2 is more highly expressed on ependymoma and astrocytoma subtypes than on glioblastoma (p= 0.0417; p= 0.0035) (Figure 3P). Collectively, these findings indicate that the TMIGD2 molecule is associated with less aggressive clinicopathological characteristics in glioma patients.
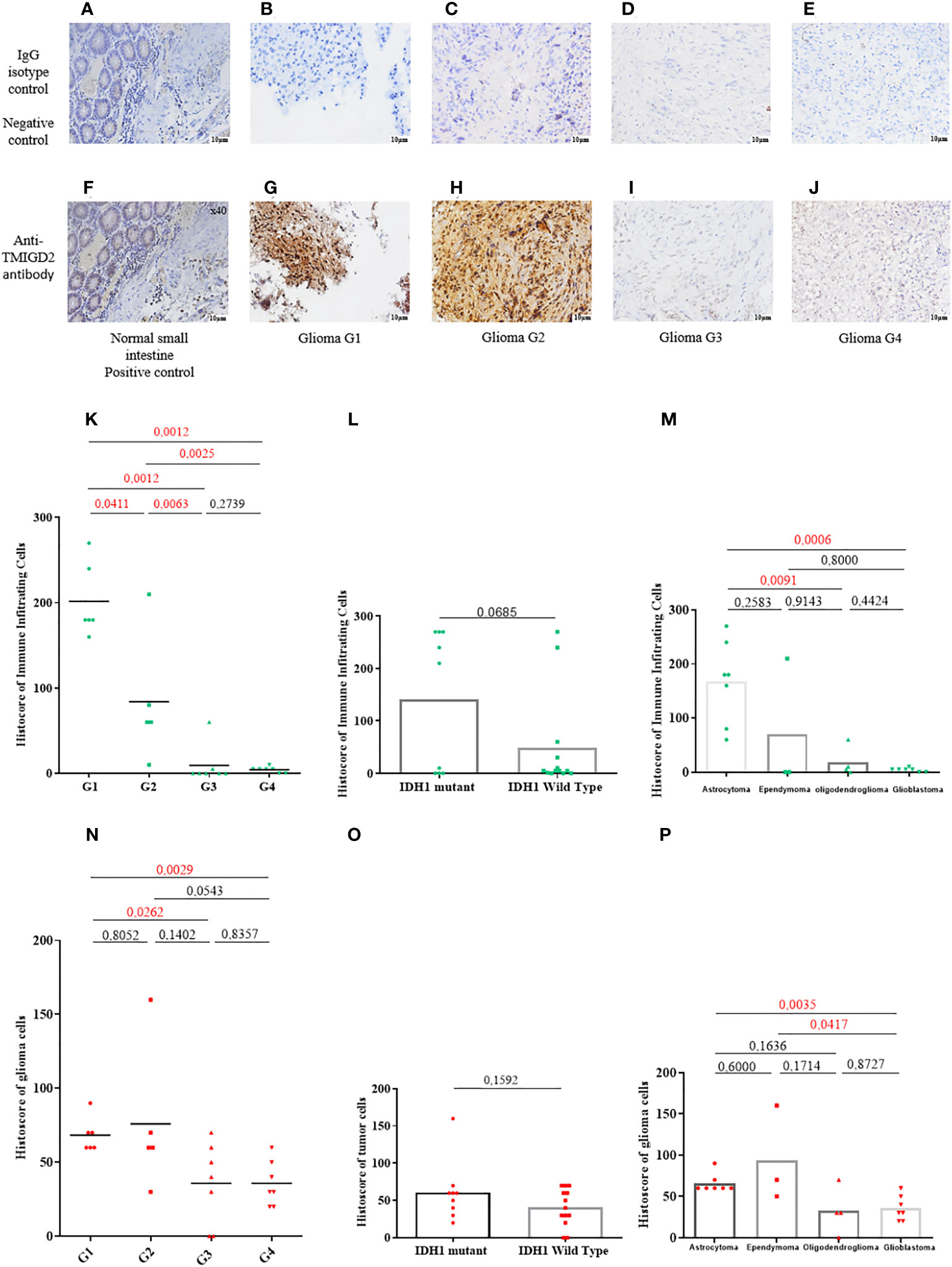
Figure 3 TMIGD2 expression in primary human glioma samples (magnification 40×). Negative control staining of normal small intestine tissue using a rabbit IgG isotype control (magnification 40×) (A). Negative control staining of glioma patients (WHO 1 grade glioma to WHO 4 grade glioma) with a rabbit IgG isotype control (B–E) (magnification 40×). Positive TMIGD2 staining in the normal small intestine (magnification 40×) (F). Glioma tissues of different grades (WHO 1 grade glioma to WHO 4 grade glioma) were immunostained for TMIGD2 using anti-TMIGD2 antibody and 3,30-diaminobenzidine (DAB; brown) (G–J) (magnification40×). Histoscores of immune infiltrating cells for TMIGD2 staining in different grades of glioma(K), IDH status (L), and histological subtypes (M). Histoscores of TMIGD2 staining on glioma cells according to WHO grades of glioma (N), IDH status (O), and histological subtypes (P).
TMIGD2 is negatively correlated with angiogenic markers
After characterizing the expression pattern of TMIGD2 according to clinical parameters in gliomas, we next investigated the association between TMIGD2 and angiogenesis markers. As illustrated in (Figures 4A, B) Fibroblast Growth Factor Receptor (FGFR), Delta Like Ligand 4 (DLL4), and Vascular Endothelial Growth Factor (VEGF) were significantly higher in a group of patients with low TMIGD2 (p<0.0001; p= 0.0002; p< 0.0001). According to the TCGA cohort, we found a weak negative correlation between TMIGD2 expression and the pro-angiogenic factors including platelet-derived growth factor (PDGFA) (r= -0.2501; p< 0.0001), hepatocyte growth factor (HGF) (r= -0.4398; p< 0.0001), and DLL4 (r= -0.2745; p< 0.0001), (Figures 4C, D, G, H, K, L). However, TMIGD2 performed a negative correlation with vascular endothelial growth factor (VEGF) (r= -0.3878; p< 0.0001), and fibroblast growth factor receptor 1 (FGFR1) (r= -0.3019; p< 0.0001) (Figures 4E, F, I, J). On the other hand, different studies have reported that epithelial to mesenchymal transition, hypoxia, and G2/M checkpoint signaling pathways promote angiogenesis (28–30). Therefore, gene set enrichment analysis (GSEA) was performed to explore the impact of TMIGD2 in these pathways. We have found that genes associated with angiogenesis (FDR= 0.00329; p<0.0001), epithelial to mesenchymal transition (FDR<0.0001; p<0.0001), hypoxia (FDR= 0.1728; p= 0.009), and G2/M checkpoint (FDR<0.0001; p<0.0001) signaling pathways were significantly enriched in patients with low TMIGD2 expression (Figures 5A–D). Similar results were reported in the CGGA cohort (Figures 5F–I). According to the above data, TMIGD2 expression decreases with glioma progression pathways, suggesting that TMIGD2 may prevent glioma development.
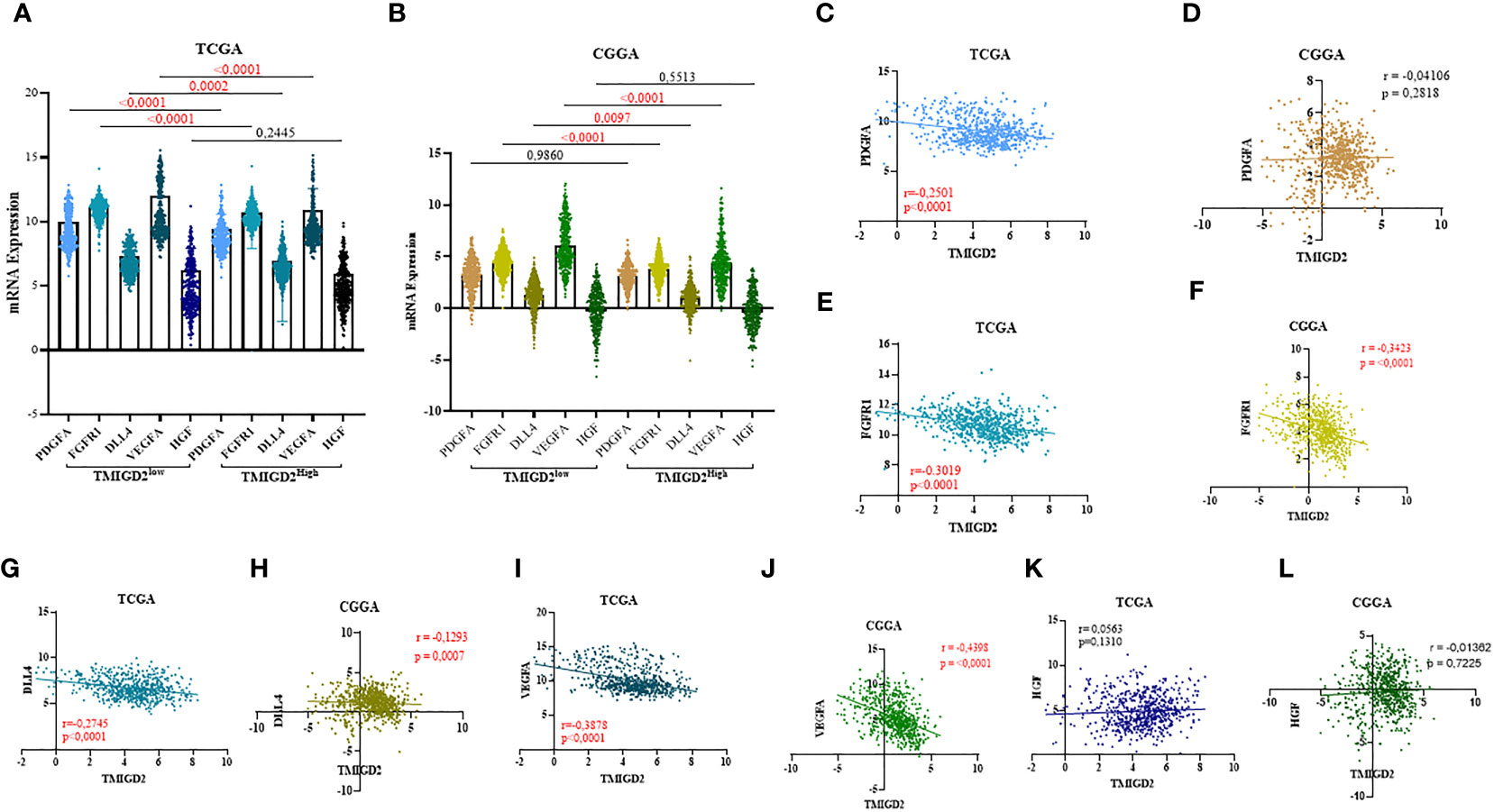
Figure 4 Correlation between TMIGD2 and angiogenic markers in TCGA and CGGA datasets. PDGFA, FGFR1, DLL4 and VEGFA, representative markers of angiogenesis, were significantly higher in the low-TMIGD2 expression group (A, B). TMIGD2 was negatively correlated with angiogenesis molecules, including PDGF (C, D), FGFR1 (E, F), DLL4 (G, H) and VEGFA (I, J), and no significant association was found between the expression of TMIGD2 and HGF in glioma TME (K, L).
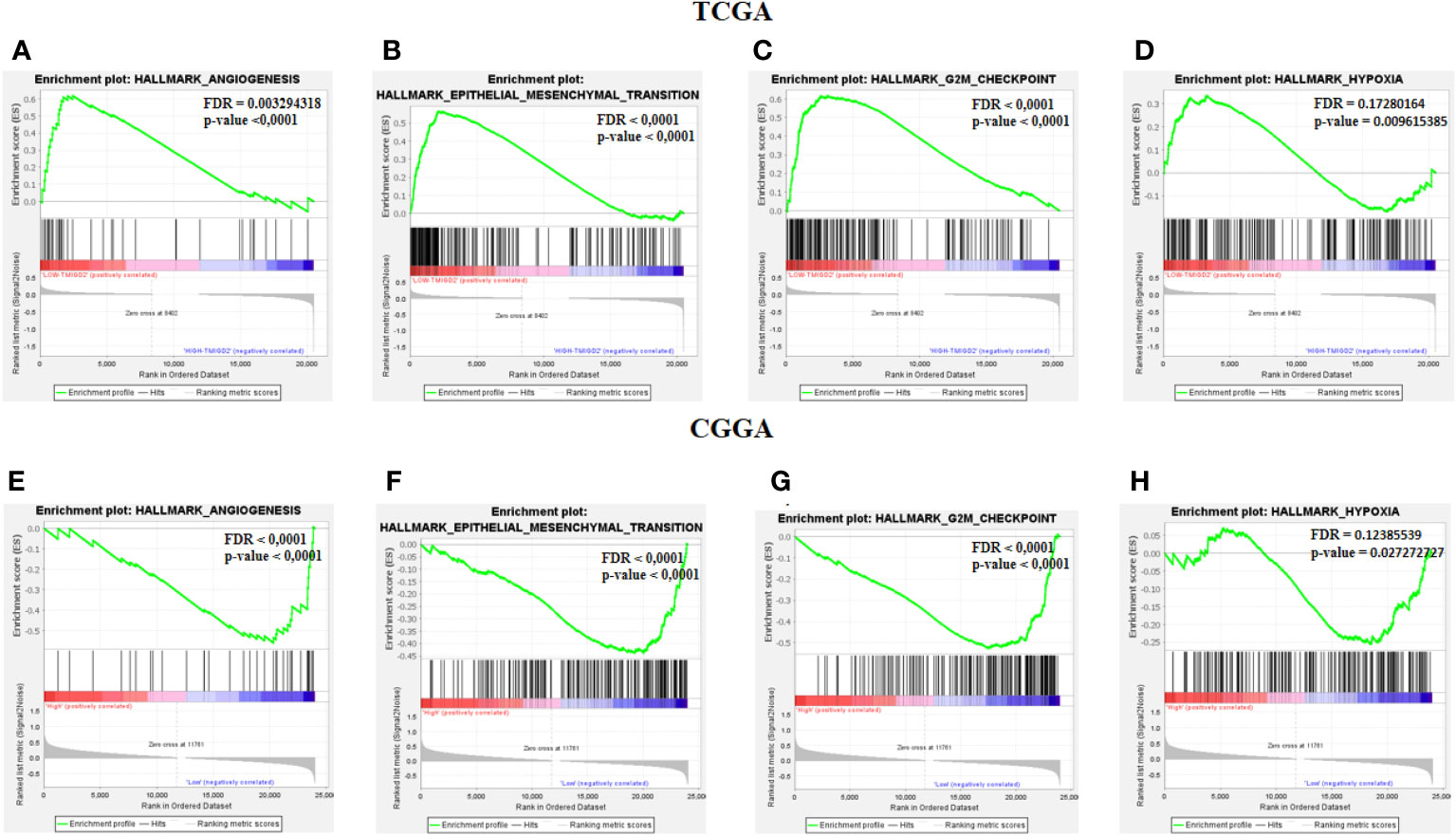
Figure 5 Gene Set Enrichement analysis. Enrichment analysis between low and high TMIGD2 groups shows the angiogenesis (A, F), epithelial mesenchymal transition (B, G), G2M checkpoint (C, H), and hypoxia (D, I), genes signaling pathway.
TMIGD2 transcripts negatively correlated with inhibitory immune checkpoints
Using the same cohorts of patients (TCGA and CGGA), we assessed the association of TMIGD2 expression with inhibitory immune checkpoints that belong to the b7-family members. These immune check points Programmed death-ligand 1 (PDL-1), B7-H3 (CD276), and B7-H6 (NCR3LG1) have been associated with aggressiveness and poor prognosis in glioma patients (31–33). Interestingly, the expression of inhibitory immune checkpoints was significantly upregulated in patients with a low TMIGD2 expression profile (Figures 6A, B). Nevertheless, the Pearson correlation showed a weak negative correlation of TMIGD2 with PDL-1(r=-0.07906; p= 0.0423), (r=-0.07529; p= 0.0492) (Figures 6C, D) and B7-H6 (r= -0.1070; p= 0.0058), (r= -0.1688; p < 0.0001) (Figures 6G, H). In addition, a weak negative correlation between TMIGD2 and B7H3 was found in the TCGA cohort (Figure 6E), while no significant correlation between TMIGD2 and B7H3 was observed in patients in the CGGA database (Figure 6F). Our results showed that TMIGD2 was not correlated with the expression of B-7 family immune checkpoints.
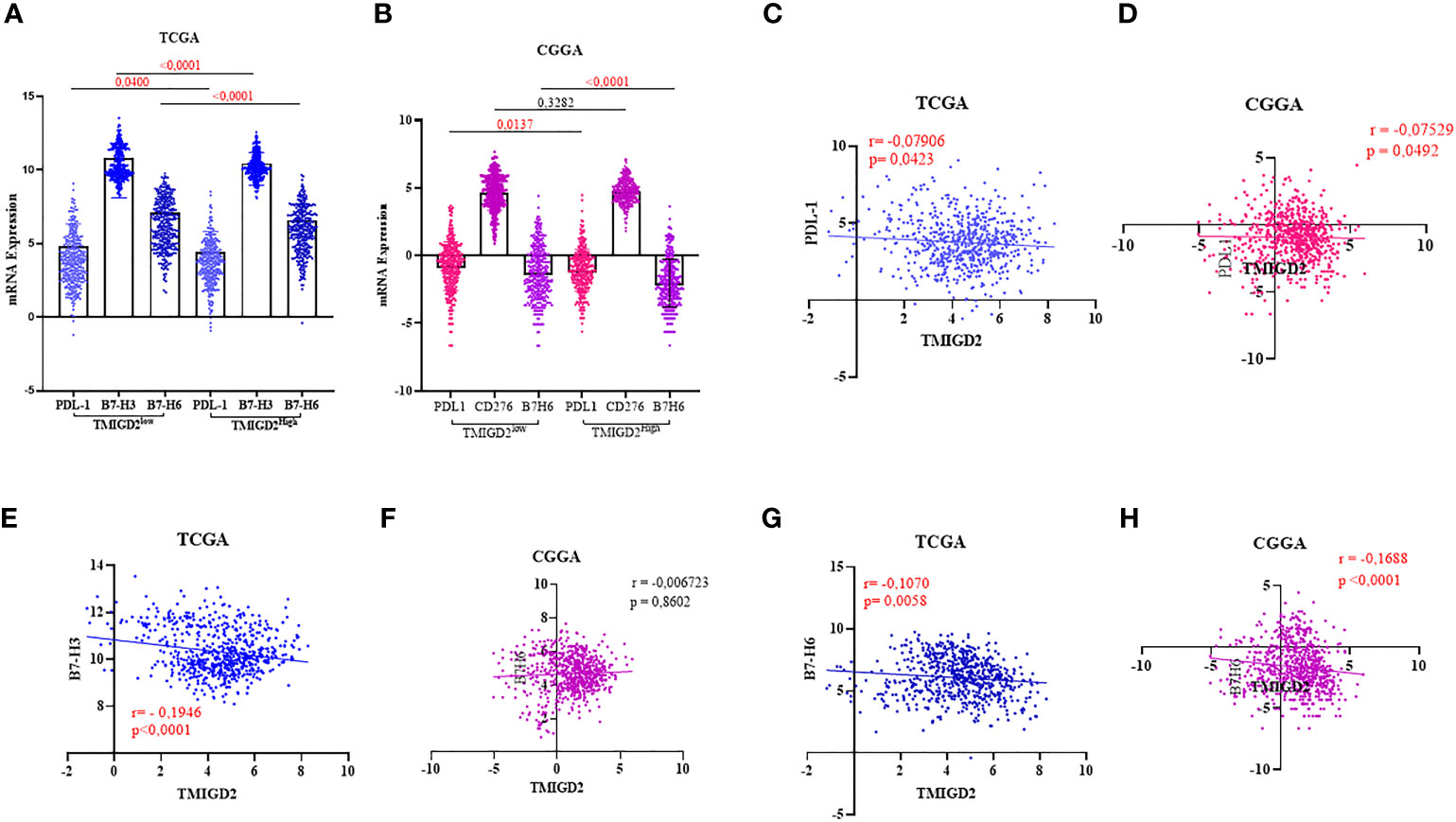
Figure 6 Association between TMIGD2 and inhibitory immune checkpoints in human gliomas from the TCGA and CGGA cohorts. PDL1, B7H3 and B7H6 were significantly higher in the low-TMIGD2 expression groups (A, B). TMIGD2 expression negativelycorrelated with PDL1 (C, D), and B7H6 (G, H). Weak negative correlation between TMIGD2 and B7H3 in TCGA cohort (E). There is no significant correlation between TMIGD2 and B7H3 in patients in the CGGA database (F).
TMIGD2 expression levels affect immune cell infiltration in gliomas
We collected data from two databases (TCGA and CGGA) to examine the abundance of 24 immune cell subsets based on the TMIGD2 profiles. In the TCGA dataset, Monocytes appeared more abundant in patients with high TMIGD2 (p=0.032), whereas, the infiltration level of Mucosal-Associated Invariant T cells (MAIT) (p= 0.043) and central memory T cells (Tcm) (p= 0.029) were less abundant (Figure 7A). In the CGGA cohort, patients with high TMIGD2 profile showed high infiltration levels of dendritic cells (DC) (p= 0.00013), monocytes (p= 0.0076), NK (p<0.0001), γδ T cells (Tgd) (p= 0.0098), MAIT (p= 0.0026), and naive CD8 T cells (p= 0.00047). In contrast, B cells (p= 0.00029), CD4 T (p<0.0001), natural killer T (NKT) cells (p= 0.041), type 1 regulatory T cell (Tr1) (p<0.0001), Natural regulatory T cells (nTregs) (p<0.0001), induced T regulatory cells (iTreg) (p<0.0001), T helper type 1 (Th1) (p= 0.0048), T helper type 17 (Th17) (p<0.0001), T follicular helper cells (Tfh) (p<0.0001), and Tcm (p<0.0001) were less infiltrated (Figure 7B). Overall, our study suggests that TMIGD2 expression was positively associated with anti-tumor immune cell infiltration, while negatively associated with pro-tumor immune cell subsets.
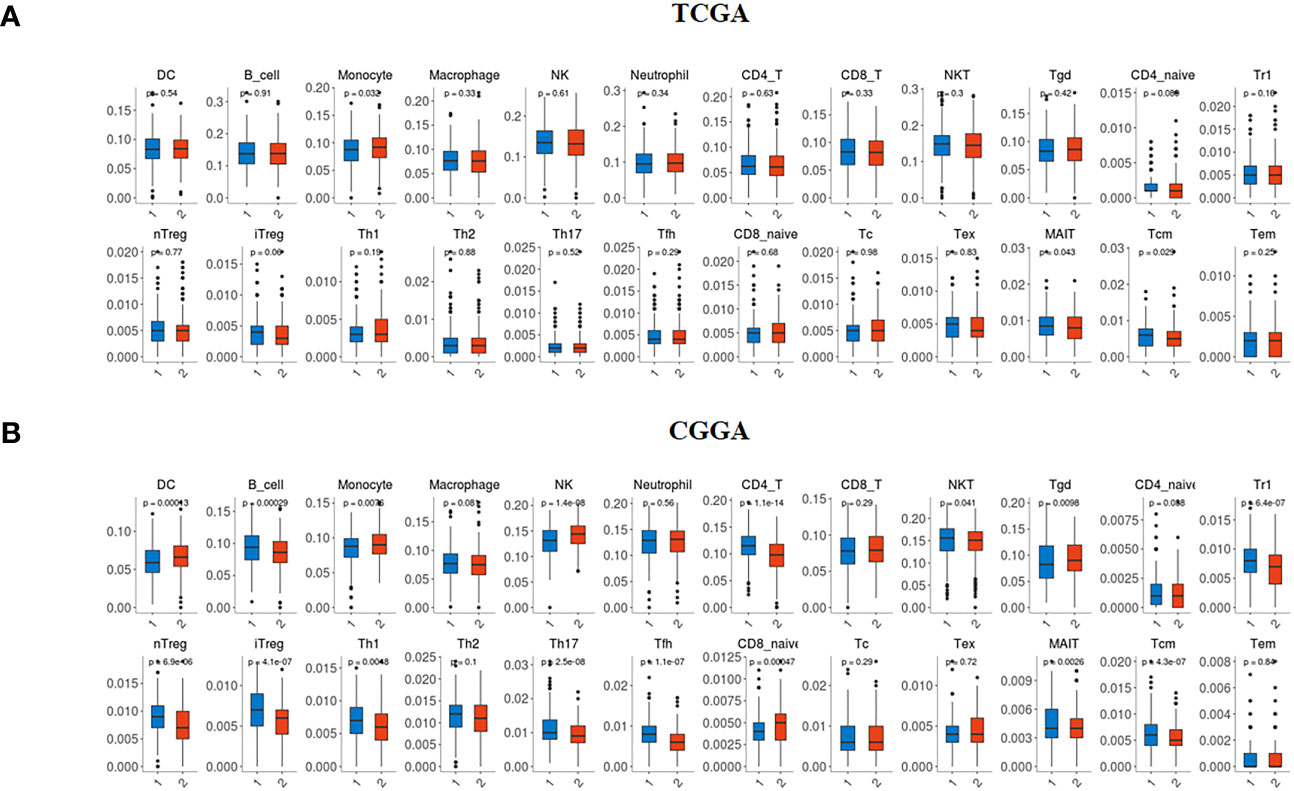
Figure 7 Immune infiltration profile according to TMIGD2 expression in glioma patients. In the TCGA (A) and CGGA (B) cohorts, blue boxes represent the abundance of immune cell subsets in low TMIGD2 group, and red boxes correspond to the infiltration rate in high TMIGD2 group.
Elevated expression of TMIGD2 in the microenvironment of glioma patients is associated with good OS
To establish whether the difference in TMIGD2 expression would reliably affect the outcome of patients, we performed Mantel-Cox analysis between low and high TMIGD2 groups. As a matter of fact, patients who presented high expression levels of TMIGD2 were strikingly associated with a good outcome (at 50% of OS, the survival median was at roughly 70 months) (p=0.0155) compared to those with low expression levels, which fits our results in (Figures 8A, B). In addition, multivariable analysis indicated that TMIGD2 and age were independent good prognostic biomarkers in the TCGA dataset (HR= 0.710; p=0.002; HR= 0.767; p=0.016) respectively (Figure 9A). However, WHO grade (HR= 2.062; p<0.0001), histological subtype (HR= 2.449; p<0.0001), and IDH Status (HR= 1.526; p= 0.001) were independent of worse prognosis biomarkers in both glioma cohorts (Figures 9A, B). These results indicate that TMIGD2 is associated with a better prognosis in human gliomas.
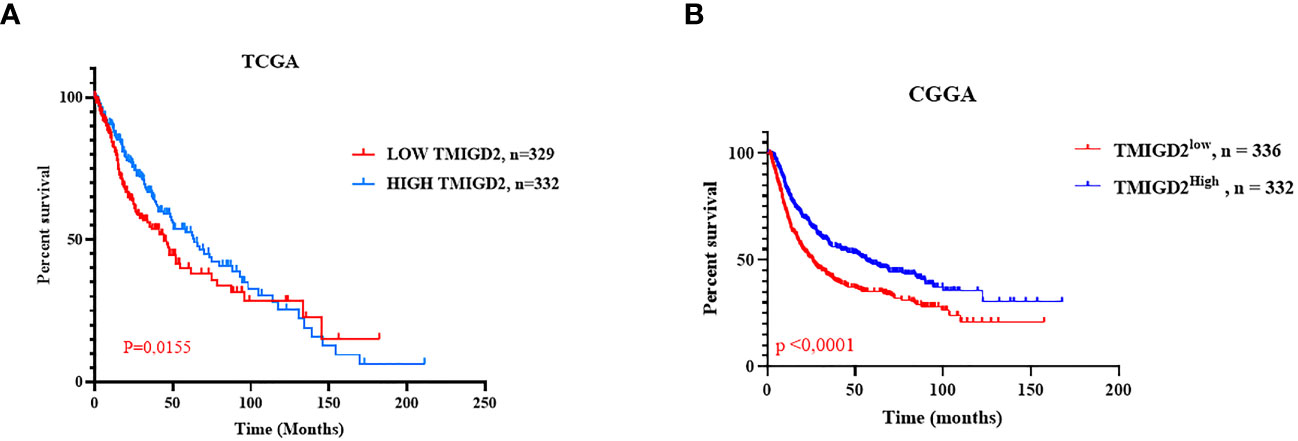
Figure 8 Overall survival in the TCGA and CGGA cohorts according to TMIGD2 expression in the glioma tumor microenvironment. Blue curves represent the OS of low TMIGD2 patients. Red curves reflect the OS of high TMIGD2 patients (A, B).
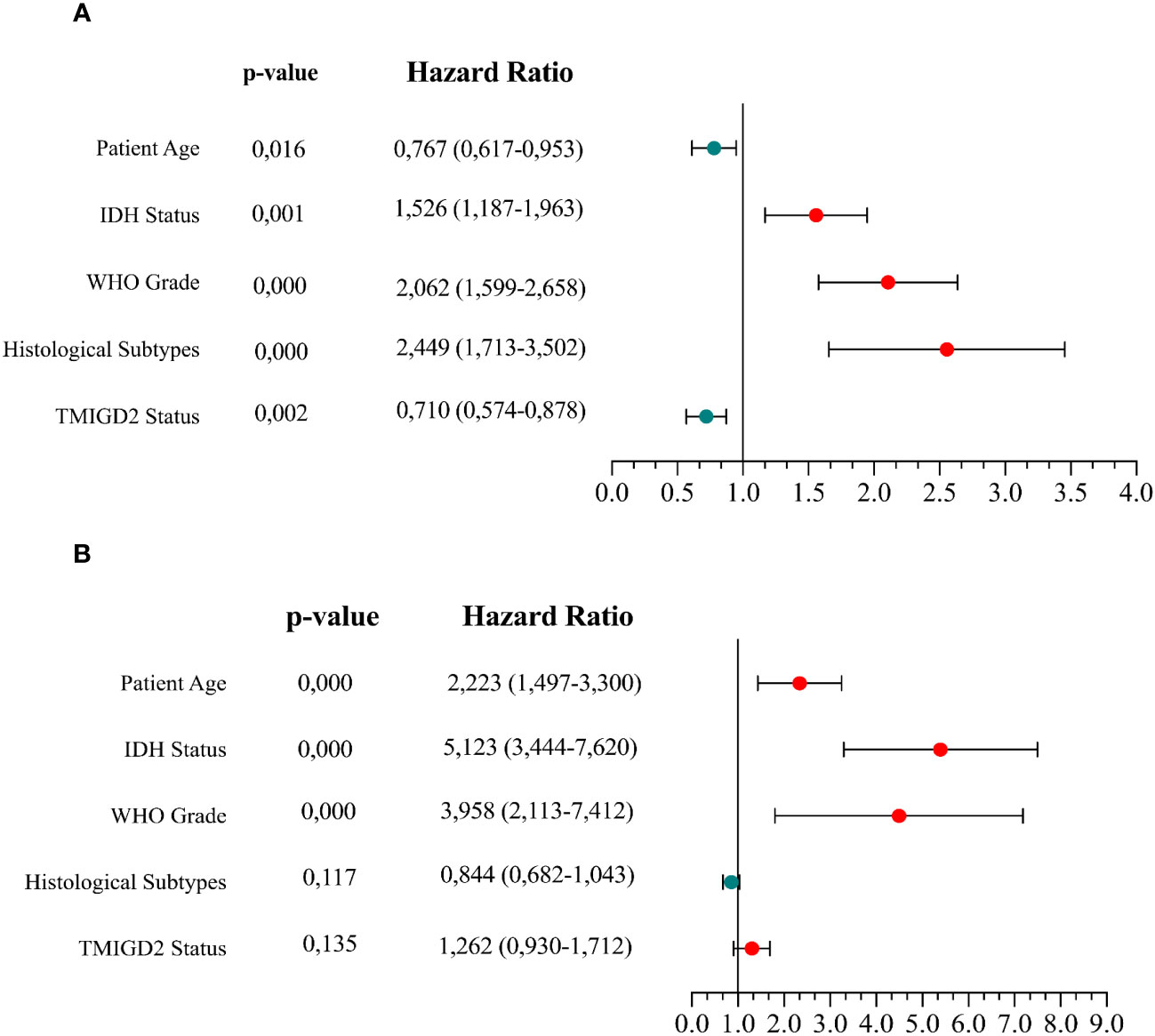
Figure 9 Cox multivariable analysis of clinical prognostic characteristics in the TCGA and CGGA datasets. Cox analyses investigating the variables for survival of glioma patients in the TCGA cohort (A) and CGGA cohort (B).
Discussion
Glioblastoma is the most aggressive type of glioma, and its proximity to sensitive areas of the brain makes surgical resection clinically arduous (34). Furthermore, conventional approaches to dealing with recidivism are inefficient (35), hence the need to explore new treatment strategies. Immunotherapy is a well-known field of cancer treatment method that involves using the immune system to combat cancer cells (36). Numerous studies have evaluatZSed the expression of inhibitory receptors on exhausted CD8+T cells, yet costimulatory molecules are still poorly explored (37). To the best of our knowledge, the present study is the first work to evaluate the expression of TMIGD2, and its association with clinical characteristics, angiogenesis markers and OS in glioma patients. Using the TCGA, the CGGA, and the Moroccan cohorts, we characterized the expression profile of TMIGD2 at the transcriptional and proteomic levels in diffuse gliomas. TMIGD2 protein staining results showed an upregulation on both tumor and immune cells in LGG versus HGG patients. This study is the first to characterize TMIGD2 expression on human glioma cells. Yet, its role on these cells is still unknown. In gastric cancer, immunohistochemistry analysis reported that TMIGD2 protein was expressed in GC and noncancerous samples, and on immune cells in both tissues. Furthermore, TMIGD2 expression on immune cells was not significantly associated with pathological grade in GC. In high grade glioma, a previous study showed a lack of naive T cells in the tumor microenvironment (TME) (38). Besides, some studies targeting NK cells have shown that they are more abundant in low-grade gliomas than in high-grade gliomas (39).Given that TMIGD2 is constitutively expressed on naive T cells and most NK cells (20), this may explain the low level of TMIGD2 in HGG tissue. In terms of patient age, our analysis showed that TMIGD2 expression is highly abundant in TME of young patients (≤ 45). In fact, the number of NK cells and their proliferative capacity decline with aging (40, 41). Furthermore, the production of naïve T cells, the repertoire of T cell receptors, and T cell activation are all negatively impacted by a patient’s age, which provides an explanation for this observation (42, 43). TCGA cohort analysis revealed a significant expression of TMIGD2 in the IDH1 mutation group. Prior findings have indicated that patients with IDH mutant status have prolonged OS compared to those with wild-type status (44–47). In addition, high TMIGD2 protein staining on immune cells was significantly associated with the astrocytoma subtype. In contrast to tumor cells, TMIGD2 protein staining was strongly associated with ependymoma subtype. It has been reported that glioblastoma multiform (GBM) was associated with a worse prognosis than astrocytoma and ependymoma subtypes (48, 49). These results demonstrate that overexpression of TMIGD2 was associated with the lower-grade IDH1-mutant tumors and may be correlated with a better outcome in patients with gliomas. This disease represents the most highly vascularized malignancy, and severe angiogenesis is one of its distinctive pathological features (27). Nader and colleagues’ study shed light on the implication of TMIGD2 in cell-cell interaction, cell migration, and angiogenesis. The angiogenic phenotype of endothelial cells in culture was considerably influenced by the ectopic expression or silencing of TMIGD2 expression, and the introduction of TMIGD2 to tumor cells promoted tumor angiogenesis via the SPIN90/WISH signaling axis (21). Moreover, several studies have shown the strong involvement of hypoxia, the epithelial to mesenchymal transition, and G2/M checkpoint signaling pathways in mediating angiogenesis (28–30). As part of our study, we assessed the association between TMIGD2 expression and various genes associated with angiogenesis and tumor progression. Interestingly, we observed a significantly lower expression of genes such as PDGFA, FGFR1, DLL4, and VEGFA in glioma patients with a higher TMIGD2 profile. Furthermore, Gene Set Enrichment Analysis revealed that angiogenesis-associated genes were mainly enriched in patients with low TMIGD2 expression. Qi Y and colleagues’ study reported that overexpression of TMIGD2 ligand may inhibit angiogenesis in gliomas through anti-VEGF and anti-PDGF processes (25). Accordingly, our results suggest that high TMIGD2 expression may be associated with low angiogenesis potential and thus with a lower risk of tumor development and metastasis. Otherwise, therapies targeting TMIGD2 may not only improve antitumor immune responses, but also affect tumor angiogenesis.In recent years, exciting progress has been made in the use of ICI in several solid tumors (50, 51). Over the past few years, there have been a growing number of studies on molecules in the B7 family, specially B7-H3, B7-H4, B7-H5, B7-H6, and B7-H7 (31). In glioma tissues, it has been reported that high expression of inhibitory molecules, namely PDL1, B7H3 and B7H6 promotes tumor progression (31–33). Previous studies have demonstrated that PDL1, B7H3, B7H6 blockade enhance naïve T cell priming in draining lymph nodes, reactivating dysfunctional T cells in tumor tissues, or by increasing antitumor response (52–55). Further, PDL1 inhibition induces the expansion of CD4/CD8 T cells expressing ICOS molecules in the tumor microenvironment of colorectal cancer patients (56). Considering that TMIGD2 belongs to the B7 family, our study is the first to examine the association of TMIGD2 expression with PD-L1, B7H3, and B7H6 in gliomas. We report here that high TMIGD2 expression was negatively associated with PDL1, B7H3 and B7H6 expression compared to low TMIGD2 profile. Our results suggest that high TMIGD2 expression may be involved in the anti-tumor immune response and in the inhibition of glioma development. On the other hand, tumor infiltrating immune cells have been reported to predict the treatment response and prognosis of glioma patients (39). Therefore, the association between TMIGD2 and immune cell infiltration was investigated. Our results showed that dendritic cells, monocytes, NK cells, γδ T cells, and naive CD8 cells were abundant in the TME of patients with high TMIGD2 expression. In addition, infiltration of B cells, CD4 T cells, NKTs, Tr1s, nTregs, iTregs, Th1s, Th17s, Tfhs, and Tcms was reduced in gliomas with high TMIGD2 levels. Indeed, many studies have found an association between long-term survival in glioma patients and dendriticcells, NK cells, γδ T cells, and CD8 cells infiltration (57–59). In contrast, CD4 T cells, Tr1s, nTregs, iTregs, Th1s, and Th17s infiltrating the glioma tumor microenvironment were correlated with immunosuppression and a poor prognosis (6, 60–62). Our findings suggest that overexpression of TMIGD2 may promote infiltration of antitumor immune cell subsets in the glioma TME. Regarding the OS analysis, our findings indicated that glioma patients with high TMIGD2 expression presented an improved outcome. Additionally, TMIGD2 represents an independent predictor of a good prognosis in glioma patients. This observation was also reported in pancreatic ductal adenocarcinoma, where the HHLA2/TMIGD2 pathway is associated with a better prognosis (24). Contrary to these results, patients with negative HHLA2/TMIGD2 expression presented a significantly higher 5-year OS compared to the positive groups of gastric cancer (23). In dysplasia and oral squamous cell carcinoma, elevated levels of both HHLA and TMIGD2 also demonstrated a poor prognosis (63). Our current data shows for the first time that a high TMIGD2 expression profile displays a favorable outcome in patients with gliomas. Overall, TMIGD2 could be a potential therapeutic target associated with improved OS in glioma patients. Low TMIGD2 expression could predict worse clinical characteristics, angiogenesis, and protumoral immune cell infiltration. More importantly, TMIGD2 may be a relevant biomarker for patient selection and for response to immunotherapy evaluation in gliomas. Considering that naive T-cell deficiencies at diagnosis and after chemotherapy impair cell therapy potential (64). Further, bevacizumab (anti-VEGF) did not show an overall survival advantage in glioma patients (65). We, hence propose combining anti-VEGF treatment and selective activation on naive T cells in patients with high TMIGD2 to enhance anti-tumor response and prognosis in glioma patients.
Data availability statement
The datasets presented in this study can be found in online repositories. The names of the repository/repositories and accession number(s) can be found in the article/supplementary material.
Ethics statement
The studies involving human participants were reviewed and approved by the ethical committee at the Ibn Rochd University Hospital of Casablanca. The patients/participants provided their written informed consent to participate in this study.
Author contributions
CB collected, analyzed, and interpreted data, and wrote the manuscript. SA and HB collected and analyzed data. IR, MK and AL analyzed and reviewed the manuscript. AB analyzed the data, revised the manuscript and supervised the study. All authors contributed to the article and approved the submitted version.
Funding
This work was supported by the Moroccan Ministry of Higher Education, Research and innovation through a “PPR1” project and by the Moroccan Ministry of Higher Education, Research and innovation and the Digital Development Agency “ADD” through an “Al-khawarizmi” project.
Acknowledgments
We would like to thank all members of the Department of neurosurgery and Department of Pathological Anatomy, specially, Dr S. Stitou, Dr FZ. Bakhtaoui, O. Aazzane.
Conflict of interest
The authors declare that the research was conducted in the absence of any commercial or financial relationships that could be construed as a potential conflict of interest.
Publisher’s note
All claims expressed in this article are solely those of the authors and do not necessarily represent those of their affiliated organizations, or those of the publisher, the editors and the reviewers. Any product that may be evaluated in this article, or claim that may be made by its manufacturer, is not guaranteed or endorsed by the publisher.
References
1. Medawar PB. Immunity to homologous grafted skin; the fate of skin homografts transplanted to the brain, to subcutaneous tissue, and to the anterior chamber of the eye. Br J Exp Pathol Févr (1948) 29(1):58−69.
2. Aspelund A, Antila S, Proulx ST, Karlsen TV, Karaman S, Detmar M, et al. A dural lymphatic vascular system that drains brain interstitial fluid and macromolecules. J Exp Med (2015) 212(7):991−9. doi: 10.1084/jem.20142290
3. Louveau A, Smirnov I, Keyes TJ, Eccles JD, Rouhani SJ, Peske JD, et al. Structural and functional features of central nervous system lymphatic vessels. Nature (2015) 523(7560):337−41. doi: 10.1038/nature14432
4. Jiang X, Liu G, Li Y, Pan Y. Immune checkpoint: the novel target for antitumor therapy. Genes Dis (2019) 8(1):25−37. doi: 10.1016/j.gendis.2019.12.004
5. Healey Bird B, Nally K, Ronan K, Clarke G, Amu S, Almeida AS, et al. Cancer immunotherapy with immune checkpoint inhibitors-biomarkers of response and toxicity; current limitations and future 351 promise. Diagn Basel (2022) 12(1):124. doi: 10.3390/diagnostics12010124
6. Ait Ssi S, Chraa D, El Azhary K, Sahraoui S, Olive D, Badou A. Prognostic gene expression signature in patients with distinct glioma grades. Front Immunol (2021) 12:685213. doi: 10.3389/fimmu.2021.685213
7. Stupp R, Hegi ME, Mason WP, van den Bent MJ, Taphoorn MJ, Janzer RC, et al. Effects of radiotherapy with concomitant and adjuvant temozolomide versus radiotherapy alone on survival in glioblastoma in a randomised phase III study: 5-year analysis of the EORTC-NCIC trial. Lancet Oncol (2009) 10(5):459−66. doi: 10.1016/S1470-2045(09)70025-7
8. Ostrom QT, Cioffi G, Gittleman H, Patil N, Waite K, Kruchko C, et al. CBTRUS statistical report: primary brain and other central nervous system tumors diagnosed in the united states in 2012–2016. Neuro- Oncol (2019) 21(Supplement_5):v1−100. doi: 10.1093/neuonc/noz150
9. Reardon DA, Kaley TJ, Dietrich J, Clarke JL, Dunn GP, Lim M, et al. Phase 2 study to evaluate safety and efficacy of MEDI4736 (durvalumab [DUR]) in glioblastoma (GBM) patients: an update. J Clin Oncol (2017) 35(15_suppl):2042−2042. doi: 10.1200/JCO.2017.35.15_suppl.2042
10. Reardon DA, Omuro A, Brandes AA, Rieger J, Wick A, Sepulveda J, et al. OS10.3 randomized phase 3 study evaluating the efficacy and safety of nivolumab vs bevacizumab in patients with recurrent glioblastoma: CheckMate 143. Neuro-Oncol (2017) 19(suppl_3):iii21. doi: 10.1093/neuonc/nox036.071
11. Kamran N, Alghamri MS, Nunez FJ, Shah D, Asad AS, Candolfi M, et al. Current state and future prospects of immunotherapy for glioma. Immunotherapy (2018) 10(4):317−39. doi: 10.2217/imt-2017-0122
12. P R, H F, P F, AM A, M S, AM L. Co-Stimulatory agonists: an insight into the immunotherapy of cancer. EXCLI J (2021) 20:1055–85. doi: 10.17179/excli2021-352
13. Iwata R, Hyoung Lee J, Hayashi M, Dianzani U, Ofune K, Maruyama M, et al. ICOSLG-mediated regulatory T-cell expansion and IL-10 production promote progression of glioblastoma. Neuro-Oncol (2020) 22(3):333−44. doi: 10.1093/neuonc/noz204
14. Turk MJ, Guevara-Patiño JA, Rizzuto GA, Engelhorn ME, Houghton AN. Concomitant tumor immunity to a poorly immunogenic melanoma is prevented by regulatory T cells. J Exp Med (2004) 200(6):771−82. doi: 10.1084/jem.20041130
15. McNamara JO, Kolonias D, Pastor F, Mittler RS, Chen L, Giangrande PH, et al. Multivalent 4-1BB binding aptamers costimulate CD8+ T cells and inhibit tumor growth in mice. J Clin Invest (2008) 118(1):376−86. doi: 10.1172/JCI33365
16. Valzasina B, Guiducci C, Dislich H, Killeen N, Weinberg AD, Colombo MP. Triggering of OX40 (CD134) on CD4+CD25+ T cells blocks their inhibitory activity: a novel regulatory role for OX40 and its comparison with GITR. Blood (2005) 105(7):2845−51. doi: 10.1182/blood-2004-07-2959
17. Kone AS, Ait Ssi S, Sahraoui S, Badou A. BTN3A: a promising immune checkpoint for cancer prognosis and treatment. Int J Mol Sci (2022) 23(21):13424. doi: 10.3390/ijms232113424
18. Zhu Y, Yao S, Iliopoulou BP, Han X, Augustine MM, Xu H, et al. B7-H5 costimulates human T cells via CD28H. Nat Commun (2013) 4(1):1−12. doi: 10.1038/ncomms3043
19. J M, C Jm, F S, F A, M C, M R, et al. Expression, clinical significance, and receptor identification of the newest B7 family member HHLA2 protein. Clin Cancer Res Off J Am Assoc Cancer Res (2015) 21(10):2359–66. doi: 10.1158/1078-0432.CCR-14-1495
20. Zhu Y, Yao S, Iliopoulou BP, Han X, Augustine MM, Xu H, et al. B7-H5 costimulates human T cells via CD28H. Nat Commun (2013) 4:2043. doi: 10.1038/ncomms3043
21. Rahimi N, Rezazadeh K, Mahoney JE, Hartsough E, Meyer RD. Identification of IGPR-1 as a novel adhesion molecule involved in angiogenesis. Mol Biol Cell (2012) 23(9):1646−56. doi: 10.1091/mbc.e11-11-0934
22. Zhuang X, Long EO. CD28 homolog is a strong activator of natural killer cells for lysis of B7H7+ tumor cells. Cancer Immunol Res (2019) 7(6):939−51. doi: 10.1158/2326-6066.CIR-18-0733
23. Hu C, Xu Z, Chen S, Lv H, Wang Y, Wang X, et al. Overexpression of B7H5/CD28H is associated with worse survival in human gastric cancer. J Cell Mol Med (2020) 24(2):1360−9. doi: 10.1111/jcmm.14812
24. Chen Q, Wang J, Chen W, Zhang Q, Wei T, Zhou Y, et al. B7−H5/CD28H is a co−stimulatory pathway and correlates with improved prognosis in pancreatic ductal adenocarcinoma. Cancer Sci (2019) 110(2):530−9. doi: 10.1111/cas.13914
25. Qi Y, Deng G, Xu P, Zhang H, Yuan F, Geng R, et al. HHLA2 is a novel prognostic predictor and potential therapeutic target in malignant glioma. Oncol Rep (2019) 42(6):2309−22. doi: 10.3892/or.2019.7343
26. Ghouzlani A, Lakhdar A, Rafii S, Karkouri M, Badou A. The immune checkpoint VISTA exhibits high expression levels in human gliomas and associates with a poor prognosis. Sci Rep (2021) 11:21504. doi: 10.1038/s41598-021-00835-0
27. Louis DN, Ohgaki H, Wiestler OD, Cavenee WK, Burger PC, Jouvet A, et al. The 2007 WHO classification of tumours of the central nervous system. Acta Neuropathol (Berl) (2007) 114(2):97−109. doi: 10.1007/s00401-007-0243-4
28. da Ponte KF, Berro DH, Collet S, Constans JM, Emery E, Valable S, et al. In vivo relationship between hypoxia and angiogenesis in human glioblastoma: a multimodal imaging study. J Nucl Med Off Publ Soc Nucl Med (2017) 58(10):1574−9. doi: 10.2967/jnumed.116.188557
29. Kurmyshkina O, Kovchur P, Schegoleva L, Volkova T. Markers of angiogenesis, lymphangiogenesis, and epithelial–mesenchymal transition (Plasticity) in CIN and early invasive carcinoma of the cervix: exploring putative molecular mechanisms involved in early tumor invasion. Int J Mol Sci (2020) 21(18):6515. doi: 10.3390/ijms21186515
30. Hu A, Huang JJ, Zhang JF, Dai WJ, Li RL, Lu ZY, et al. Curcumin induces G2/M cell cycle arrest and apoptosis of head and neck squamous cell carcinoma in vitro and in vivo through ATM/Chk2/p53-dependent pathway. Oncotarget (2017) 8(31):50747−60. doi: 10.18632/oncotarget.17096
31. Wang Z, Wang Z, Zhang C, Liu X, Li G, Liu S, et al. Genetic and clinical characterization of B7−H3 (CD276) expression and epigenetic regulation in diffuse brain glioma. Cancer Sci (2018) 109(9):2697−705. doi: 10.1111/cas.13744
32. Zhu Z, Zhang H, Chen B, Liu X, Zhang S, Zong Z, et al. PD-L1-Mediated immunosuppression in glioblastoma is associated with the infiltration and M2-polarization of tumor-associated macrophages. Front Immunol (2020) 11:588552. doi: 10.3389/fimmu.2020.588552
33. Jiang T, Wu W, Zhang H, Zhang X, Zhang D, Wang Q, et al. High expression of B7-H6 in human glioma tissues promotes tumor progression. Oncotarget (2017) 8(23):37435−47. doi: 10.18632/oncotarget.16391
34. Xia L, Fang C, Chen G, Sun C. Relationship between the extent of resection and the survival of patients with low-grade gliomas: a systematic review and meta-analysis. BMC Cancer (2018) 18(1):48. doi: 10.1186/s12885-017-3909-x
35. Weller M, Cloughesy T, Perry JR, Wick W. Standards of care for treatment of recurrent glioblastoma–are we there yet? Neuro-Oncol (2013) 15(1):4−27. doi: 10.1093/neuonc/nos273
36. Gattinoni L, Klebanoff CA, Palmer DC, Wrzesinski C, Kerstann K, Yu Z, et al. Acquisition of full effector function in vitro paradoxically impairs the in vivo antitumor efficacy of adoptively transferred CD8+ T cells. J Clin Invest (2005) 115(6):1616−26. doi: 10.1172/JCI24480
37. Kamphorst AO, Wieland A, Nasti T, Yang S, Zhang R, Barber DL, et al. Rescue of exhausted CD8 T cells by PD-1-targeted therapies is CD28-dependent. Science (2017) 355(6332):1423−7. doi: 10.1126/science.aaf0683
38. Schneider JR, Kwan K, Boockvar JA. Missing naïve T cells in the setting of glioblastoma are found to be sequestered in the bone marrow. Neurosurgery (2019) 85(2):E194. doi: 10.1093/neuros/nyz167
39. Lu J, Li H, Chen Z, Fan L, Feng S, Cai X, et al. Identification of 3 subpopulations of tumor-infiltrating immune cells for malignant transformation of low-grade glioma. Cancer Cell Int (2019) 19:265. doi: 10.1186/s12935-019-0972-1
40. Gounder SS, Abdullah BJJ, Radzuanb NEIBM, Zain FDBM, Sait NBM, Chua C, et al. Effect of aging on NK cell population and their proliferation at ex vivo culture condition. Anal Cell Pathol Amst (2018) 2018:7871814. doi: 10.1155/2018/7871814
41. Chidrawar SM, Khan N, Chan YLT, Nayak L, Moss PA. Ageing is associated with a decline in peripheral blood CD56bright NK cells. Immun Ageing (2006) 3:10. doi: 10.1186/1742-4933-3-10
42. Salam N, Rane S, Das R, Faulkner M, Gund R, Kandpal U, et al. T Cell ageing: effects of age on development, survival & function. Indian J Med Res (2013) 138(5):595−608.
43. Hong H, Wang Q, Li J, Liu H, Meng X, Zhang H. Aging, cancer and immunity. J Cancer (2019) 10(13):3021−7. doi: 10.7150/jca.30723
44. Pollack IF, Hamilton RL, Sobol RW, Nikiforova MN, Lyons-Weiler MA, LaFramboise WA, et al. IDH1 mutations are common in malignant gliomas arising in adolescents: a report from the children’s oncology group. Childs Nerv Syst ChNS Off J Int Soc Pediatr Neurosurg (2011) 27(1):87−94. doi: 10.1007/s00381-010-1264-1
45. Cohen A, Holmen S, Colman H. IDH1 and IDH2 mutations in gliomas. Curr Neurol Neurosci Rep (2013) 13(5):345. doi: 10.1007/s11910-013-0345-4
46. Sanson M, Marie Y, Paris S, Idbaih A, Laffaire J, Ducray F, et al. Isocitrate dehydrogenase 1 codon 132 mutation is an important prognostic biomarker in gliomas. J Clin Oncol Off J Am Soc Clin Oncol (2009) 27(25):4150−4. doi: 10.1200/JCO.2009.21.9832
47. Paugh BS, Qu C, Jones C, Liu Z, Adamowicz-Brice M, Zhang J, et al. Integrated molecular genetic profiling of pediatric high-grade gliomas reveals key differences with the adult disease. J Clin Oncol Off J Am Soc Clin Oncol (2010) 28(18):3061−8. doi: 10.1200/JCO.2009.26.7252
48. Deshpande RP, Y. B. V. K. C, Panigrahi M, Babu PP. Prognostic significance of anatomic origin and evaluation of survival statistics of astrocytoma patients–a tertiary experience. Indian J Surg Oncol (2019) 10(1):55−60. doi: 10.1007/s13193-018-0820-0
49. Raco A, Esposito V, Lenzi J, Piccirilli M, Delfini R, Cantore G. Long-term follow-up of intramedullary spinal cord tumors: a series of 202 cases. Neurosurgery (2005) 56(5):972−81.
50. Lipson EJ, Forde PM, Hammers HJ, Emens LA, Taube JM, Topalian SL. Antagonists of PD-1 and PD-L1 in cancer treatment. Semin Oncol (2015) 42(4):587−600. doi: 10.1053/j.seminoncol.2015.05.013
51. Emens LA, Ascierto PA, Darcy PK, Demaria S, Eggermont AMM, Redmond WL, et al. Cancer immunotherapy: opportunities and challenges in the rapidly evolving clinical landscape. Eur J Cancer Oxf Engl (2017) 81:116−29. doi: 10.1016/j.ejca.2017.01.035
52. Peng Q, Qiu X, Zhang Z, Zhang S, Zhang Y, Liang Y, et al. PD-L1 on dendritic cells attenuates T cell activation and regulates response to immune checkpoint blockade. Nat Commun (2020) 11(1):1−8. doi: 10.1038/s41467-020-18570-x
53. Ma J, Ma P, Zhao C, Xue X, Han H, Liu C, et al. B7-H3 as a promising target for cytotoxicity T cell in human cancer therapy. Oncotarget (2016) 7(20):29480−91. doi: 10.18632/oncotarget.8784
54. Liu J, Yang S, Cao B, Zhou G, Zhang F, Wang Y, et al. Targeting B7-H3 via chimeric antigen receptor T cells and bispecific killer cell engagers augments antitumor response of cytotoxic lymphocytes. J Hematol OncolJ Hematol Oncol (2021) 14(1):21. doi: 10.1186/s13045-020-01024-8
55. Wu MR, Zhang T, Gacerez AT, Coupet TA, DeMars LR, Sentman CL. B7H6-specific bispecific T cell engagers lead to tumor elimination and host antitumor immunity. J Immunol (2015) 194(11):5305−11. doi: 10.4049/jimmunol.1402517
56. Beyrend G, van der Gracht E, Yilmaz A, van Duikeren S, Camps M, Höllt T, et al. PD-L1 blockade engages tumor-infiltrating lymphocytes to co-express targetable activating and inhibitory receptors. J Immunother Cancer (2019) 7(1):217. doi: 10.1186/s40425-019-0700-3
57. Liau LM, Black KL, Prins RM, Sykes SN, DiPatre PL, Cloughesy TF, et al. Treatment of intracranial gliomas with bone marrow-derived dendritic cells pulsed with tumor antigens. J Neurosurg (1999) 90(6):1115−24. doi: 10.3171/jns.1999.90.6.1115
58. Breznik B, Ko MW, Tse C, Chen PC, Senjor E, Majc B, et al. Infiltrating natural killer cells bind, lyse and increase chemotherapy efficacy in glioblastoma stem-like tumorospheres. Commun Biol (2022) 5(1):1−15. doi: 10.1038/s42003-022-03402-z
59. Bryant NL, Suarez-Cuervo C, Gillespie GY, Markert JM, Nabors LB, Meleth S, et al. Characterization and immunotherapeutic potential of γδ T-cells in patients with glioblastoma. Neuro-Oncol (2009) 11(4):357−67. doi: 10.1215/15228517-2008-111
60. Han S, Zhang C, Li Q, Dong J, Liu Y, Huang Y, et al. Tumour-infiltrating CD4+ and CD8+ lymphocytes as predictors of clinical outcome in glioma. Br J Cancer (2014) 110(10):2560−8. doi: 10.1038/bjc.2014.162
61. Wainwright DA, Dey M, Chang A, Lesniak MS. Targeting tregs in malignant brain cancer: overcoming IDO. Front Immunol (2013) 4:116. doi: 10.3389/fimmu.2013.00116
62. Takashima Y, Kawaguchi A, Kanayama T, Hayano A, Yamanaka R. Correlation between lower balance of Th2 helper T-cells and expression of PD-L1/PD-1 axis genes enables prognostic prediction in patients with glioblastoma. Oncotarget (2018) 9(27):19065−78. doi: 10.18632/oncotarget.24897
63. Xiao Y, Li H, Yang LL, Mao L, Wu CC, Zhang WF, et al. The expression patterns and associated clinical parameters of human endogenous retrovirus-h long terminal repeat-associating protein 2 and transmembrane and immunoglobulin domain containing 2 in oral squamous cell carcinoma. Dis Markers (2019) 2019:5421985. doi: 10.1155/2019/5421985
64. Das RK, Vernau L, Grupp SA, Barrett DM. Naïve T-cell deficits at diagnosis and after chemotherapy impair cell therapy potential in pediatric cancers. Cancer Discovery (2019) 9(4):492−9. doi: 10.1158/2159-8290.CD-18-1314
Keywords: TMIGD2, glioma, costimulatory, immunotherapy, prognosis
Citation: Boulhen C, AIT SSI S, Benthami H, Razzouki I, Lakhdar A, Karkouri M and Badou A (2023) TMIGD2 as a potential therapeutic target in glioma patients. Front. Immunol. 14:1173518. doi: 10.3389/fimmu.2023.1173518
Received: 24 February 2023; Accepted: 21 April 2023;
Published: 16 May 2023.
Edited by:
Lai Wei, The Ohio State University, United StatesReviewed by:
Jessy John, University of Pittsburgh, United StatesQing Tang, University of Washington, United States
Copyright © 2023 Boulhen, AIT SSI, Benthami, Razzouki, Lakhdar, Karkouri and Badou. This is an open-access article distributed under the terms of the Creative Commons Attribution License (CC BY). The use, distribution or reproduction in other forums is permitted, provided the original author(s) and the copyright owner(s) are credited and that the original publication in this journal is cited, in accordance with accepted academic practice. No use, distribution or reproduction is permitted which does not comply with these terms.
*Correspondence: Abdallah Badou, abdallahbadou@yahoo.com