- Hannover Medical School, Department of Hematology, Hemostasis, Oncology and Stem Cell Transplantation, Hannover, Germany
Natural Killer (NK) cells have come a long way since their first description in the 1970’s. The most recent reports of their adaptive-like behavior changed the way the immune system dichotomy is described. Adaptive NK cells present characteristics of both the innate and adaptive immune system. This NK cell subpopulation undergoes a clonal-like expansion in response to an antigen and secondary encounters with the same antigen result in an increased cytotoxic response. These characteristics can be of extreme importance in the clinical setting, especially as adoptive immunotherapies, since NK cells present several advantages compared other cell types. This review will focus on the discovery and the path to the current knowledge of the adaptive NK cell population.
Background
The immune system is classically divided in two branches, the innate and adaptive immunity according to the cellular characteristics. The innate branch of the immune system offers a fast but unspecific response against pathogens. The adaptive immune system recognizes antigens/pathogens and cells are clonally expanded and, in the case of antigen-specific B-cells, even modified to obtain higher levels of antigen specificity (1–5).
Innate lymphoid cells (ILCs) comprise a variety of common lymphoid progenitor-derived cells that do not express somatically rearranged antigen-specific receptors and play important roles in immune homeostasis. One subset of ILCs are Natural Killer (NK) cells (6–8). In humans, they comprise between 5 to 20% of circulating lymphocytes (9). These immune cells have very diversified functions that can range from their classic innate anti-tumor and anti-viral functions, to regulatory roles involved in the modulation of other immune cells as well as tissue growth promotion (10).
NK cells were first described in the early 1970’s when a significant natural cytotoxicity to target cells was observed in lymphocytes from unimmunized mice and normal human lymphoid tissue (10–13). Until then, it was believed that non-T cell-mediated cytotoxicity was only achieved by antibody-dependent-cell-cytotoxicity (ADCC) or macrophages (14–16). R. Kiessling et al. were able to exclude a possible involvement of macrophages, T and B cells. This was achieved by the combined results observed in athymic nude mice and inability to identify the aforementioned cells. A new and unique cell population able to kill mouse tumor cells was hence recognized. Even though very little was known about this cell population, this observation opened the door to a brand-new world in immunology (11). We have come a long way in our knowledge about NK cells since these days.
In the following sections, this review aims to summarize the evolution in NK cells knowledge from their discovery to the detection of their adaptive phenotype.
Natural killer cells biology and receptors
Natural Killer cells were the first member of the ILC family to be discovered. They possess a lymphoid developmental origin and a T-bet-regulated rapid cytokine production activation profile. However, this activation does not lead, generally, to immunological memory. Furthermore, NK cells lack clonally rearranging antigen receptors (17). This was demonstrated in mice, where RAG-1 or RAG-2 disrupted genes did not affect the development of functional NK cells (18, 19). It is known that NK cells are involved in the immune response to several pathogens and are also involved in the first line of defense against tumor cells without prior sensitization (20–23). NK cells were normally characterized according to their morphology, as large granular lymphocytes and phenotypically, defined by the surface expression of the cluster of differentiation (CD)56 and lack of CD3 (24). Another definition of mature conventional NK cells relies on exclusion criteria describing NK cells as non-T, non-B lymphoid cells with the ability to rapidly produce interferon (IFN) -gamma upon stimulation with pathogens (25).
NK cells are bone marrow derived and can be found in the peripheral circulation, but are also present in other lymphoid and non-lymphoid organs (26). Two major distinct populations of circulating NK cells are recognized according to the surface expression of CD56 and CD16. It is considered that CD56dimCD16+, corresponding to approximately 90% of peripheral blood NK cells, represent a more cytotoxic population while the CD56brightCD16dim/-, corresponding to the remaining 10%, have a more regulatory role, being highly involved in the production of cytokines (27–29). There is, however, a much higher count of NK cells subpopulations when it comes to the different receptors expressed by these cells. For instance, in 2009, Milush et al. reported new subpopulations of NK cells based on the expression of CD7, a marker shared with T and pre-B cells. The surface expression of this receptor was associated with the co-expression of other NK cell-associated receptors such as Killer-Immunoglobulin Receptors (KIRs) or Natural Cytotoxicity receptors (NCRs) (30). With the help of technological advancement, and the possibility to simultaneously analyze more than 30 parameters, Horowitz et al. indicated that at least 30 000 different phenotypes of conventional peripheral blood NK cells can be present at any given point (31). The great diversity of NK cells populations among each individual or patient, comprising different functions and degrees of maturation, may result in varied responses. The challenge to current research is to understand how these different populations can be exploited in the design of specific therapies.
Different NK cell subpopulations can have different, more or less specialized, functions and responses. Nonetheless, a very strict balance between activating and inhibitory receptors regulates all NK cells. Contrary to what happens with adaptive cells, NK cell receptors are germline-encoded with no requirements for recombination and the activation status of NK cells is determined by the balance between activating or inhibitory signals. A key regulator of NK cell activation is the constitutively expressed Major Histocompatibility Complex (MHC) class I. MHC class I molecules bind to the inhibitory receptors, including members of the Killer Immunoglobulin-like Receptor (KIR) family and prevent NK cell activation (32). However, infected, tumor-transformed or stressed cells, undergo a downregulation or even loss of MHC class I expression. This will result in a lack of inhibitory, or rather a prevalence of activating signals, tipping the balance towards the activation of NK cells (33, 34). This mechanism allows for the preservation of ‘self’ while engaging in the elimination of the ‘missing-self’ (32).
The activating receptors of NK cells include receptors belonging to the C-type lectins family and NCRs mentioned above. NCRs (e.g. NKp30, NKp44, NKp46 and NKp80) arm NK cells with the ability to effectively kill tumor-transformed cells (35–38). The key role of NCRs in the elimination of tumor cells is well established and can be demonstrated, for instance, by an ineffective clearance of certain tumors in the absence of NKp46 in in vitro and in vivo models (39–41). Furthermore, NCRs are also implicated in the control and clearance of pathogens. For instance, NKp46 is essential to the elimination of virus and bacteria in vivo. This was demonstrated by the inability of NKp46-deficient mice to recognize and eliminate influenza-infected cells expressing NKp46-ligands or as observed by the reduced activation and IFN-gamma production during the early stages of Streptococcus pneumoniae infections (42, 43). In regards to C-type lectins, CD94 forms covalent bonds with members of the NKG2 family (A, C, D and E) and forms heterodimers expressed by NK cells and a subset of cytotoxic T lymphocytes (44).
An overview of some inhibitory and activating receptors expressed at the surface of NK cells is summarized in Table 1.
Besides their ability to recognize and kill tumor and virus-infected cells, NK cells are also able to interact with other cell types and orchestrate the adaptive immune response. Most notably, NK cells are able to modulate Dendritic cells (DCs), macrophages and T cell (57–59). One particular example of this modulatory capability is seen, for instance, by the ability of NK cells to edit the maturation of autologous DCs through NKp30-mediated elimination of aberrant or immature DCs while sparing fully matured DCs. This results in a correct DC-priming and subsequent antigen-specific T cell response (60, 61).
Natural killer cells; innate or adaptive?
By definition, memory or adaptive cells are a population of long-lived, self-renewing immune cells with the ability of antigen-specific recognition and memory formation. For many years, conventional NK cells were described as short-lived innate lymphocytes lacking antigen specificity. However, in recent years this idea has been challenged. Murine studies showed the ability of NK cells to acquire selective memory. This phenomenon was demonstrated by hapten-induced contact hypersensitivity and recall responses in challenged mice, lacking mature T and B cells. This feature was previously widely accepted as a T cell effect. Furthermore, this NK cell response persisted for several weeks and was able to discriminate between different haptens. This assembles three hallmarks characteristics of adaptive immunity: i) acquired activity, ii) antigen specificity and iii) long-lived memory cells (62). The hapten-specific response was further characterized by Paust et al., while the NK cell memory development in response to different viruses and possible influence of the host’s genetic background was also assessed. To elucidate this memory function, non-infectious virus-like particles containing proteins from influenza or HIV and UV-inactivated vesicular stomatitis virus were used. Immunization of naïve Rag1−/− mice and subsequent adoptive transfer of purified NK cells into naïve Rag2−/−Il2rg−/− mice resulted in a vigorous and sustained response at challenge 4 weeks later. This response was virus-specific and restricted to hepatic NK cells isolated from immunized mice, while splenic NK cells were unresponsive. Furthermore, this NK cell-specificity persisted for at least 4 months (63). Another study described the ability of NK cells to develop a memory-like behavior following cytokine stimulation. The in vitro stimulation consisted of a combination of interleukin (IL)-12, IL-15 and IL-18. NK cells were then adoptively transferred to naïve Rag1−/− mice and re-stimulation with IL-12 and IL-15 or via the engagement of activating NK cell receptors with antibodies led to a robust IFN-gamma secretion. Furthermore, the often termed ‘memory-like’ NK cells in this experimental setting, produced significantly higher levels of IFN-gamma which was detected for at least 3 weeks post-adoptive transfer of pre-activated NK cells (64).
The particular role of Cytomegalovirus
Gumá et al. described the expansion of a population of NK cells in response to cytomegalovirus (CMV)-infected fibroblasts in 2006 and this set off increasing interest in NK cell function thus accumulating knowledge over the last decade (21). The NK relationship with CMV has for long been appreciated by the observation of the high susceptibility to CMV-infections in both humans and mice lacking functional NK cells (65–69).
Murine models facilitated the intensive study of the NK cell-CMV molecular mechanisms. This was largely simplified by the identification of both receptor and ligand involved in the NK cell memory formation. The murine NK cell activating receptor Ly49H recognizes the m157 protein expressed on infected cells (70). Multiple factors have been identified as essential for the NK cell memory development during murine CMV (MCMV) infection at different stages. These include IL-12/STAT4 signaling, IL-18, miR-155, the Bim protein, the Zbtb32 transcription factor, recombination-activation genes and mitophagy (71–76). In MCMV, contrary to Paust et al. hapten-induced contact hypersensitivity study, adaptive NK cells were found in the liver but also in the spleen, lung, kidney, blood circulation and other lymphoid tissues. This population of NK cells underwent an expansion phase, followed by a contraction phase after resolution of viral infection and ultimately resulted in the generation of long-lived “memory” NK cells that were more protective during a second encounter with this pathogen (77, 78).
In humans, it is now known that this particular NK cell behavior is associated to the non-classical MHC class I molecule HLA-E and its antigenic presentation of human CMV (HCMV) viral peptide(s) to NKG2C, which is a C-type lectin that covalently bonds to CD94 (79). In HCMV, conflicting information regarding the expansion of this cell population was reported. Several studies described the expansion of NKG2C+ NK cells in other viral infections. These ranged from HIV, Hantavirus, Chikungunya virus, Hepatitis B virus and Epstein-Barr virus (80–86). Interestingly however, the expansion of NKG2C+ NK cells was almost completely limited to the HCMV seropositive population in all the studies in which CMV status was assessed. This suggests that HCMV is the common denominator in the expansion of NKG2C+ NK cells (80, 81, 85, 86). This supported the previous observation of Gumá et al. that HCMV influences the shaping of the NK cell receptor repertoire. Furthermore, the expansion of NKG2C+ NK cells was not seen in the context of other herpesviruses infections. Thus, HCMV may be unique in its ability to recall NKG2C+ NK cells responses (66, 87).
The diversity within NK cells may be the result of what has been described as an ‘arms race’ between NK cells and viruses (88) [74]. CMV infection results in the modulation and downregulation of MHC class I molecules on the surface of infected cells in an attempt to escape recognition by T cells (89). In fact, in the case of infected-cells being eliminated by class I-restricted CD8+ cytotoxic T lymphocytes (CTLs), pathogens that attenuate class I expression will become invisible, at least temporarily, to CTLs and therefore, have a selective advantage. In CMV-infection, this is achieved by different approaches. For example, the transport of peptides produced in the cytosol can be affected by the HCMV US6 gene attacking the TAP complex and preventing class I heterodimers from binding (90). Other strategies may be related to the retention or destruction of class I molecules. The US3 gene product binds to class I molecules sequestering them in the endoplasmic reticulum (91). Furthermore, US2 and US11 products bind to class I molecules and redirect the class I heavy chain to the cytosol, reversing the process by which the chain is inserted in the endoplasmic reticulum (92). This CTL evasion mechanism can, however, lead to the engagement of a NK cell response. NK cells are usually prevented from activation by the engagement of inhibitory receptors by self-MHC products. Certain viruses are capable of successfully reducing the surface expression of MHC class I, while controlling NK cell activation by the expression of class I homologues that will serve as decoys for NK cells. In the case of CMV, examples of this are UL18 in humans and m144 in mouse. In the particular case of UL18, this viral homologue binds to inhibitory receptors with higher affinity than MHC class I (93–95). Furthermore, the HCMV UL40 open reading frame, contains a segment that is homologous to the HLA-E binding leader peptide (96). However, the efforts of CMV to circumvent the immune system go one step even further. HCMV harbors a unique IL-10 homolog (cmvIL-10) which is able to compete with human IL-10 for binding sites (97). This viral cmvIL10 may facilitate HCMV replication by suppressing or tampering with inflammatory responses. Furthermore, a study by Chang et al. showed that the production of cmvIL-10 inhibits the production of IL-12 and tumor necrosis factor (TNF)-alpha by DCs in a concentration-dependent manner (98). As IL-12 promotes the cytotoxic and proliferative capacity of NK cells, cmvIL-10 may inhibit or delay the activation NK cells both directly and indirectly. All these mechanisms employed by CMV to evade NK cell-recognition point to the importance of NK cells in the control of CMV.
Interestingly however, cmvIL-10 was shown to induce NK cell activation and the increased NK cell cytotoxicity was triggered by several activating receptors. cmvIL-10 binds to the IL-10 receptor (IL-10R). Even though IL10-R is expressed at low levels on NK cells, the production of a virokine able to activate NK cells by HCMV is puzzling (99). One may hypothesize that the low level of activation promotes a pro-inflammatory environment beneficial for HCMV infection and/or dissemination all the while ensuring that a strong enough trigger does not result in an effective antiviral response.
Epigenetic profile towards adaptivity
The NK cell population is one of great heterogeneity both in phenotype and function. CMV was shown to directly influence the NK cell population and lead to lasting alterations (87, 100). Furthermore, CMV was also shown to influence the outcome after hematopoietic stem cell transplantation of acute and chronic myeloid leukemia patients since CMV reactivation controlled by CMV-CTLs led to decreased relapses in these patients (101, 102). A recent study by our group demonstrated that adaptive NK cells have the ability to recruit T cells when cultured with CMV-infected target cells (103). However, at the molecular level, the receptor modulation and NK cell differentiation has not yet been described. A study by Schlums et al. shed some light on these questions. The analysis of 196 healthy adults showed that the CD56dim NK cell population from many donors lacked expression of the adaptor protein FcεRɣ, the tyrosine kinase SYK and the intracellular adaptor protein EAT-2 (50.4% of HCMV seropositive donors vs 10.1% of HCVM seronegative donors). They demonstrated that this effect correlated to HCVM seropositivity regardless of sex and age of the donor, B cell or myeloid-cell-related signaling proteins. Furthermore, this was observed only in acute HCMV infection (de novo or reactivation). The lack of FcεRɣ, SYK and EAT-2 correlated with phenotypic characteristics of adaptive NK cells, namely, the expression of NKG2C and absence or reduced expression of NKp30. Deficiency for EAT-2 and FcεRɣ was shown to be the result of hypermethylation in their promoter region (104). Similar results were observed for SYK by Lee et al. (105). Moreover, genome-wide analysis of DNA methylation comparing canonical and adaptive NK cells to T cells were performed. This revealed that the methylation profile of adaptive NK cells was closely related to the one of effector T cells. Adaptive NK cells had 2372 differentially methylated regions (DMRs) compared to canonical NK cells while only differing in 61 DMRs when compared to CD8+ T cells. Furthermore, this methylation profile was responsible for the regulation of the gene expression on adaptive NK cells (104).
Origins of natural killer cell memory
Nagler et al. originally described CD3-CD56dim and CD3-CD56bright as subpopulations of NK cells in different differentiation stages, where the CD3-CD56bright mature overtime to the CD3-CD56dim population (28). The GATA-binding factor 2 (GATA-2) transcription factor is necessary for hematopoietic stem and progenitor cell survival and proliferation. Its haploinsufficiency results in deficiencies in Dendritic, B and NK cells and leads to clinical symptoms of immunodeficiency, lymphedema and even myelodysplastic syndrome (106, 107). A more recent analysis and characterization of symptomatic GATA-2 (+/- or mutation) patients’ showed that persisting NK cells displayed characteristics and functional properties of adaptive NK cells. Taken this into account, adaptive NK cells would be able to persist in the absence of their CD56dim precursors raising questions onto the ontogeny of adaptive NK cells. Characteristics of persisting NK cells included a decreased expression of the promyelocytic leukemia zinc finger (PLZF) and T-box transcription factors (T-bet), maintained Fc receptor expression and cytotoxic capacity in response to antibody-coated target cells, as well as, degranulation capacity and IFN-gamma and TNF secretion. Furthermore, the persistence of this cell population despite the GATA-2 insufficiency that results in the abolishment of canonical NK cells demonstrates the considerable increased longevity of adaptive NK cells (108). Another study provides further evidence into the longevity of adaptive NK cells and further supports a self-renewal hypothesis. Here, paroxysmal nocturnal hemoglobinuria patients have an X-linked acquired Phosphatidylinositol N-acetylglucosaminyltransferase subunit A (PIGA) mutation. PIGA encodes a protein required for the synthesis of glycosylphophatidylinositol (GPI) anchors. These loss-of-function mutations occur in hematopoietic stem and progenitor cells, and result in the production of hematopoietic cells deficient in GPI-anchored membrane proteins (109). Progression of the disease from a GPIpos/GPIneg mixed chimerism towards a virtually exclusive GPIneg lineage can give insight into the development of adaptive NK cells. Results from 15 patients suggested that CD56dim NK cells may persist and propagate independently of CD56bright. Furthermore, the majority of adaptive NK cells where GPIpos, while canonical NK cells were vastly GPIneg. Consistent with prior studies, GPIpos adaptive NK cells showed marked reduction in IFN-gamma production in response to co-stimulation with IL-12 and IL-18 innate cytokines while maintaining degranulation capacity upon engagement of the low affinity Fc receptor CD16. This indicates that the described long-lived GPIpos NK cells are functionally comparable to adaptive NK cells (110). These studies suggest a peripheral pathway for the maintenance of CD56dim adaptive NK cells independent of hematopoietic stem and progenitor cells production and also independent of CD56bright precursor (108, 109). A linear model representation of the developmental pathway of (adaptive) NK cells is shown in Figure 1.
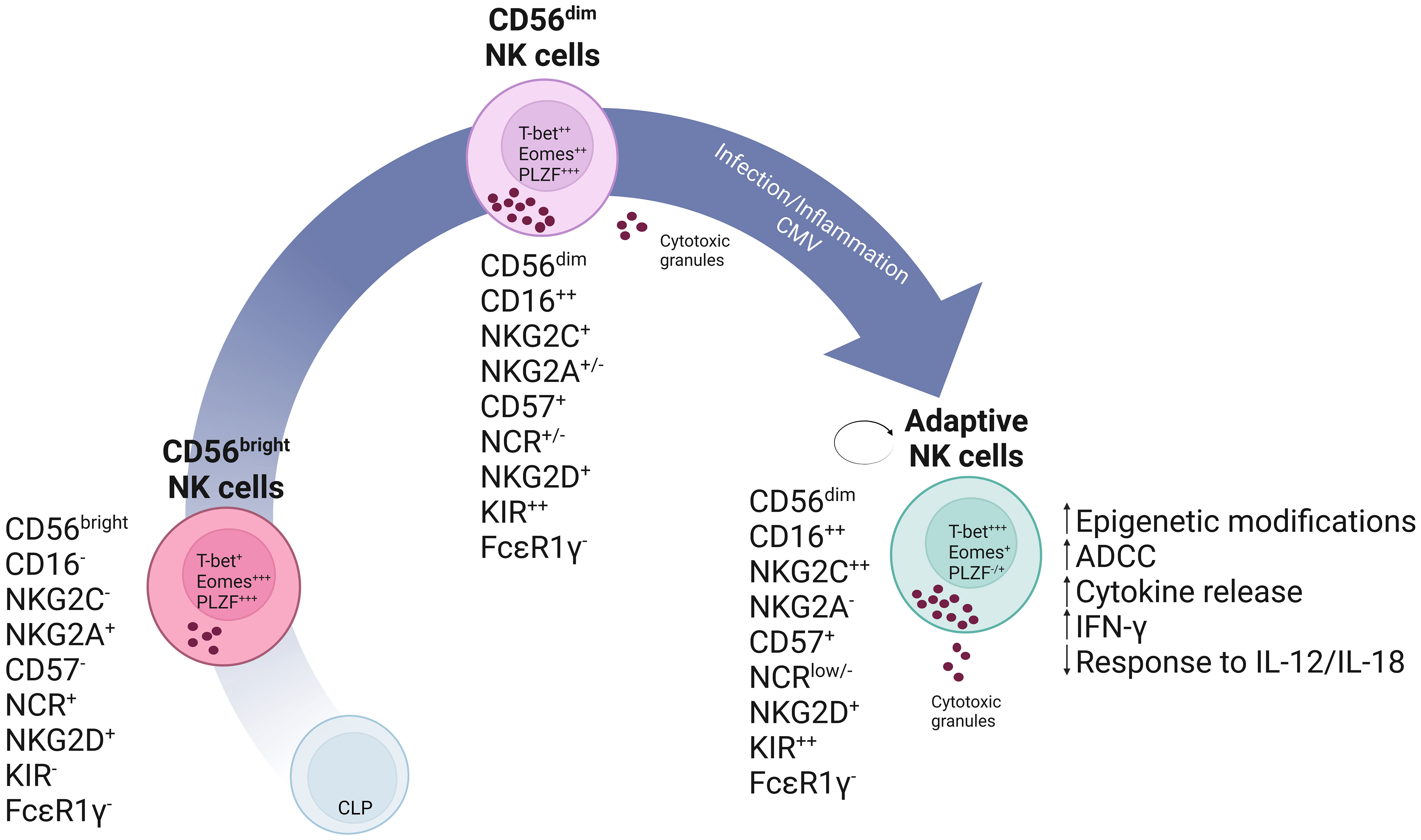
Figure 1 Schematic representation of a linear model of NK cell development. In this model it is theorized that, common lymphoid progenitor (CLP) cells commit into NK cell precursors and mature into CD56bright NK cells. CD56bright NK cells are less differentiated and express high levels of the inhibitory receptor NKG2A and natural cytotoxicity receptors (NCRs). These cells will give rise to mature CD56dim NK cells which have enhanced cytotoxic capabilities as seen, for instance, by increased production of cytotoxic granules and CD16 expression. These can subsequently further differentiate into self-renewing adaptive NK cells expressing high levels of NKG2C and Killer Immunoglobulin-like receptors (KIR) as the result of specific stimuli, which may include a combination of cytokines or CMV infection.
Importance in health and disease
Natural Killer cells play a role in the most varied biological processes and are involved in both health and disease. These range from combating infections, cancer, autoimmune disorders and even in the maintenance of normal pregnancy. NK cells contribute to the control of viral infections by both, direct cytotoxicity against virally-infected cells, but also by the production of cytokines that may control viral replication and regulate an adaptive immune response (103). They are also involved in the elimination of other types of infection that include infections caused by intracellular bacteria, fungi and some protozoa (111). One other important function of Natural Killer cells is the elimination of tumor cells. In this scenario, NK cells’ most likely role is the surveillance and elimination of malignant cells in order to prevent the formation of tumors. In regards to autoimmune disorders such as lupus erythematosus, NK cells can play a role in tolerance induction, by decreased numbers and activity, increased proportion of CD56bright cells and impaired cytotoxicity (112–114). NKp46, a NCR NK cell’s receptor, has been involved in the pathophysiology of type 1 diabetes while in rheumatoid arthritis, a subset of Natural Killer cells is greatly expanded in patients with inflamed joints (115, 116). NK cells also play an essential role in the efficacy of some vaccines and immunotherapies, such as seen as the result of, for instance, the BCG vaccine (117). NK cells further cooperate in vaccine efficacy through their interaction with vaccine-induced antibodies with NK cell-activation properties on top of the virus-neutralizing properties (118). All this demonstrates the immense possibilities of NK cells in clinical applications.
Conclusion
If NK cells are believed to be an evolutionary bridge between innate and adaptive immunity, it is not surprising that they will exhibit features of both. Implications of NK cells in such mixed processes and the recent discovery of their adaptive profile demonstrates that the exploitation of this cell population and their use in possible therapeutic approaches can have great implications in health and disease. For these reasons, further research in the full range of possible applications of adaptive NK cells is warranted.
Author contributions
DB-Q contributed to the concept and design of the article. EM-W revised the article critically for important intellectual content. All authors contributed to the article and approved the submitted version.
Conflict of interest
The authors declare that the research was conducted in the absence of any commercial or financial relationships that could be construed as a potential conflict of interest.
Publisher’s note
All claims expressed in this article are solely those of the authors and do not necessarily represent those of their affiliated organizations, or those of the publisher, the editors and the reviewers. Any product that may be evaluated in this article, or claim that may be made by its manufacturer, is not guaranteed or endorsed by the publisher.
References
1. Chaplin DD. Overview of the immune response. J Allergy Clin Immunol (2010) 125(2 Suppl 2):S3–23. doi: 10.1016/j.jaci.2009.12.980
2. Charles A, Janeway J. How the immune system works to protect the hostfrom infection: a personal view. PNAS (2001) 18(13):7461–8. doi: 10.1073/pnas.131202998
3. Dranoff G. Cytokines in cancer pathogenesis and cancer therapy. Nat Rev Cancer (2004) 4(1):11–22. doi: 10.1038/nrc1252
4. Lakkis FG, Sayegh MH. Memory T cells: a hurdle to immunologic tolerance. J Am Soc Nephrol (2003) 14(9):2402–10. doi: 10.1097/01.ASN.0000085020.78117.70
5. Nemazee D. Receptor selection in b and T lymphocytes. Annu Rev Immunol (2000) 18:19–51. doi: 10.1146/annurev.immunol.18.1.19
6. Artis D, Spits H. The biology of innate lymphoid cells. Nature (2015) 517(7534):293–301. doi: 10.1038/nature14189
7. Eberl G, Colonna M, Di Santo JP, McKenzie AN. Innate lymphoid cells. innate lymphoid cells: a new paradigm in immunology. Science (2015) 348(6237):aaa6566.
8. Spits H, Artis D, Colonna M, Diefenbach A, Di Santo JP, Eberl G, et al. Innate lymphoid cells–a proposal for uniform nomenclature. Nat Rev Immunol (2013) 13(2):145–9. doi: 10.1038/nri3365
9. Langers I, Renoux VM, Thiry M, Delvenne P, Jacobs N. Natural killer cells: role in local tumor growth and metastasis. Biologics (2012) 6:73–82.
10. Vivier E, Tomasello E, Baratin M, Walzer T, Ugolini S. Functions of natural killer cells. Nat Immunol (2008) 9(5):503–10. doi: 10.1038/ni1582
11. Kiessling R, Klein E, Pross H, Wigzell H. "Natural" killer cells in the mouse. II. cytotoxic cells with specificity for mouse moloney leukemia cells. characteristics of the killer cell. Eur J Immunol (1975) 5(2):117–21.
12. Kiessling R, Klein E, Wigzell H. "Natural" killer cells in the mouse. i. cytotoxic cells with specificity for mouse moloney leukemia cells. specificity and distribution according to genotype. Eur J Immunol (1975) 5(2):112–7.
13. Rosenberg EB, McCoy JL, Green SS, Donnelly FC, Siwarski DF, Levine PH, et al. Destruction of human lymphoid tissue-culture cell lines by human peripheral lymphocytes in 51Cr-release cellular cytotoxicity assays. J Natl Cancer Inst (1974) 52(2):345–52. doi: 10.1093/jnci/52.2.345
14. Cerottini JC, Brunner KT. Cell-mediated cytotoxicity, allograft rejection, and tumor immunity. Adv Immunol (1974) 18:67–132. doi: 10.1016/S0065-2776(08)60308-9
15. Evans R, Alexander P. Mechanism of immunologically specific killing of tumour cells by macrophages. Nature (1972) 236(5343):168–70. doi: 10.1038/236168a0
16. Norbury KC, Fidler IJ. In vitro tumor cell destruction by syngeneic mouse macrophoages: methods for assaying cytotoxicity. J Immunol Methods (1975) 7(1):109–22. doi: 10.1016/0022-1759(75)90136-2
17. Vosshenrich CA, Di Santo JP. Developmental programming of natural killer and innate lymphoid cells. Curr Opin Immunol (2013) 25(2):130–8. doi: 10.1016/j.coi.2013.02.002
18. Mombaerts P, Iacomini J, Johnson RS, Herrup K, Tonegawa S, Papaioannou VE. RAG-1-deficient mice have no mature b and T lymphocytes. Cell (1992) 68(5):869–77. doi: 10.1016/0092-8674(92)90030-G
19. Shinkai Y, Rathbun G, Lam KP, Oltz EM, Stewart V, Mendelsohn M, et al. RAG-2-deficient mice lack mature lymphocytes owing to inability to initiate V(D)J rearrangement. Cell (1992) 68(5):855–67. doi: 10.1016/0092-8674(92)90029-C
20. Barrow AD, Edeling MA, Trifonov V, Luo J, Goyal P, Bohl B, et al. Natural killer cells control tumor growth by sensing a growth factor. Cell (2018) 172(3):534–48.e19. doi: 10.1016/j.cell.2017.11.037
21. Guma M, Budt M, Saez A, Brckalo T, Hengel H, Angulo A, et al. Expansion of CD94/NKG2C+ NK cells in response to human cytomegalovirus-infected fibroblasts. Blood (2006) 107(9):3624–31. doi: 10.1182/blood-2005-09-3682
22. Schmidt S, Tramsen L, Hanisch M, Latge JP, Huenecke S, Koehl U, et al. Human natural killer cells exhibit direct activity against aspergillus fumigatus hyphae, but not against resting conidia. J Infect Dis (2011) 203(3):430–5. doi: 10.1093/infdis/jiq062
23. Vankayalapati R, Garg A, Porgador A, Griffith DE, Klucar P, Safi H, et al. Role of NK cell-activating receptors and their ligands in the lysis of mononuclear phagocytes infected with an intracellular bacterium. J Immunol (2005) 175(7):4611–7. doi: 10.4049/jimmunol.175.7.4611
24. Mandal A, Viswanathan C. Natural killer cells: in health and disease. Hematol Oncol Stem Cell Ther (2015) 8(2):47–55. doi: 10.1016/j.hemonc.2014.11.006
25. Caligiuri MA. Human natural killer cells. Blood (2008) 112(3):461–9. doi: 10.1182/blood-2007-09-077438
26. Carrega P, Ferlazzo G. Natural killer cell distribution and trafficking in human tissues. Front Immunol (2012) 3:347. doi: 10.3389/fimmu.2012.00347
27. Cooper MA, Fehniger TA, Caligiuri MA. The biology of human natural killer-cell subsets. Trends Immunol (2001) 22(11):633–40. doi: 10.1016/S1471-4906(01)02060-9
28. Nagler A, Lanier LL, Cwirla S, Phillips JH. Comparative studies of human FcRIII-positive and negative natural killer cells. J Immunol (1989) 143(10):3183–91. doi: 10.4049/jimmunol.143.10.3183
29. Cooper MA, Fehniger TA, Turner SC, Chen KS, Ghaheri BA, Ghayur T, et al. Human natural killer cells: a unique innate immunoregulatory role for the CD56(bright) subset. Blood (2001) 97(10):3146–51. doi: 10.1182/blood.V97.10.3146
30. Milush JM, Long BR, Snyder-Cappione JE, Cappione AJ 3rd, York VA, Ndhlovu LC, et al. Functionally distinct subsets of human NK cells and monocyte/DC-like cells identified by coexpression of CD56, CD7, and CD4. Blood (2009) 114(23):4823–31. doi: 10.1182/blood-2009-04-216374
31. Horowitz A, Strauss-Albee DM, Leipold M, Kubo J, Nemat-Gorgani N, Dogan OC, et al. Genetic and environmental determinants of human NK cell diversity revealed by mass cytometry. Sci Transl Med (2013) 5(208):208ra145. doi: 10.1126/scitranslmed.3006702
32. Karre K, Ljunggren HG, Piontek G, Kiessling R. Selective rejection of h-2-deficient lymphoma variants suggests alternative immune defence strategy. Nature (1986) 319(6055):675–8. doi: 10.1038/319675a0
33. Moretta A, Bottino C, Vitale M, Pende D, Biassoni R, Mingari MC, et al. Receptors for HLA class-I molecules in human natural killer cells. Annu Rev Immunol (1996) 14:619–48. doi: 10.1146/annurev.immunol.14.1.619
34. Moretta A, Vitale M, Bottino C, Orengo AM, Morelli L, Augugliaro R, et al. P58 molecules as putative receptors for major histocompatibility complex (MHC) class I molecules in human natural killer (NK) cells. anti-p58 antibodies reconstitute lysis of MHC class I-protected cells in NK clones displaying different specificities. J Exp Med (1993) 178(2):597–604.
35. Pende D, Parolini S, Pessino A, Sivori S, Augugliaro R, Morelli L, et al. Identification and molecular characterization of NKp30, a novel triggering receptor involved in natural cytotoxicity mediated by human natural killer cells. J Exp Med (1999) 190(10):1505–16. doi: 10.1084/jem.190.10.1505
36. Pessino A, Sivori S, Bottino C, Malaspina A, Morelli L, Moretta L, et al. Molecular cloning of NKp46: a novel member of the immunoglobulin superfamily involved in triggering of natural cytotoxicity. J Exp Med (1998) 188(5):953–60. doi: 10.1084/jem.188.5.953
37. Sivori S, Vitale M, Morelli L, Sanseverino L, Augugliaro R, Bottino C, et al. p46, a novel natural killer cell-specific surface molecule that mediates cell activation. J Exp Med (1997) 186(7):1129–36. doi: 10.1084/jem.186.7.1129
38. Vitale M, Bottino C, Sivori S, Sanseverino L, Castriconi R, Marcenaro E, et al. NKp44, a novel triggering surface molecule specifically expressed by activated natural killer cells, is involved in non-major histocompatibility complex-restricted tumor cell lysis. J Exp Med (1998) 187(12):2065–72. doi: 10.1084/jem.187.12.2065
39. Glasner A, Ghadially H, Gur C, Stanietsky N, Tsukerman P, Enk J, et al. Recognition and prevention of tumor metastasis by the NK receptor NKp46/NCR1. J Immunol (2012) 188(6):2509–15. doi: 10.4049/jimmunol.1102461
40. Halfteck GG, Elboim M, Gur C, Achdout H, Ghadially H, Mandelboim O. Enhanced in vivo growth of lymphoma tumors in the absence of the NK-activating receptor NKp46/NCR1. J Immunol (2009) 182(4):2221–30. doi: 10.4049/jimmunol.0801878
41. Lakshmikanth T, Burke S, Ali TH, Kimpfler S, Ursini F, Ruggeri L, et al. NCRs and DNAM-1 mediate NK cell recognition and lysis of human and mouse melanoma cell lines in vitro and in vivo. J Clin Invest (2009) 119(5):1251–63. doi: 10.1172/JCI36022
42. Elhaik-Goldman S, Kafka D, Yossef R, Hadad U, Elkabets M, Vallon-Eberhard A, et al. The natural cytotoxicity receptor 1 contribution to early clearance of streptococcus pneumoniae and to natural killer-macrophage cross talk. PLoS One (2011) 6(8):e23472. doi: 10.1371/journal.pone.0023472
43. Gazit R, Gruda R, Elboim M, Arnon TI, Katz G, Achdout H, et al. Lethal influenza infection in the absence of the natural killer cell receptor gene Ncr1. Nat Immunol (2006) 7(5):517–23. doi: 10.1038/ni1322
44. Carretero M, Palmieri G, Llano M, Tullio V, Santoni A, Geraghty DE, et al. Specific engagement of the CD94/NKG2-a killer inhibitory receptor by the HLA-e class ib molecule induces SHP-1 phosphatase recruitment to tyrosine-phosphorylated NKG2-a: evidence for receptor function in heterologous transfectants. Eur J Immunol (1998) 28(4):1280–91. doi: 10.1002/(SICI)1521-4141(199804)28:04<1280::AID-IMMU1280>3.0.CO;2-O
45. Fan QR, Mosyak L, Winter CC, Wagtmann N, Long EO, Wiley DC. Structure of the inhibitory receptor for human natural killer cells resembles haematopoietic receptors. Nature (1997) 389(6646):96–100. doi: 10.1038/38028
46. Wagtmann N, Biassoni R, Cantoni C, Verdiani S, Malnati MS, Vitale M, et al. Molecular clones of the p58 NK cell receptor reveal immunoglobulin-related molecules with diversity in both the extra- and intracellular domains. Immunity (1995) 2(5):439–49. doi: 10.1016/1074-7613(95)90025-X
47. Wagtmann N, Rajagopalan S, Winter CC, Peruzzi M, Long EO. Killer cell inhibitory receptors specific for HLA-c and HLA-b identified by direct binding and by functional transfer. Immunity (1995) 3(6):801–9. doi: 10.1016/1074-7613(95)90069-1
48. Torkar M, Norgate Z, Colonna M, Trowsdale J, Wilson MJ. Isotypic variation of novel immunoglobulin-like transcript/killer cell inhibitory receptor loci in the leukocyte receptor complex. Eur J Immunol (1998) 28(12):3959–67. doi: 10.1002/(SICI)1521-4141(199812)28:12<3959::AID-IMMU3959>3.0.CO;2-2
49. Moretta A, Sivori S, Vitale M, Pende D, Morelli L, Augugliaro R, et al. Existence of both inhibitory (p58) and activatory (p50) receptors for HLA-c molecules in human natural killer cells. J Exp Med (1995) 182(3):875–84. doi: 10.1084/jem.182.3.875
50. Colonna M, Samaridis J. Cloning of immunoglobulin-superfamily members associated with HLA-c and HLA-b recognition by human natural killer cells. Science (1995) 268(5209):405–8. doi: 10.1126/science.7716543
51. Bottino C, Sivori S, Vitale M, Cantoni C, Falco M, Pende D, et al. A novel surface molecule homologous to the p58/p50 family of receptors is selectively expressed on a subset of human natural killer cells and induces both triggering of cell functions and proliferation. Eur J Immunol (1996) 26(8):1816–24. doi: 10.1002/eji.1830260823
52. Welte S, Kuttruff S, Waldhauer I, Steinle A. Mutual activation of natural killer cells and monocytes mediated by NKp80-AICL interaction. Nat Immunol (2006) 7(12):1334–42. doi: 10.1038/ni1402
53. Le Drean E, Vely F, Olcese L, Cambiaggi A, Guia S, Krystal G, et al. Inhibition of antigen-induced T cell response and antibody-induced NK cell cytotoxicity by NKG2A: association of NKG2A with SHP-1 and SHP-2 protein-tyrosine phosphatases. Eur J Immunol (1998) 28(1):264–76. doi: 10.1002/(SICI)1521-4141(199801)28:01<264::AID-IMMU264>3.0.CO;2-O
54. Lanier LL, Corliss B, Wu J, Phillips JH. Association of DAP12 with activating CD94/NKG2C NK cell receptors. Immunity (1998) 8(6):693–701. doi: 10.1016/S1074-7613(00)80574-9
55. Bauer S, Groh V, Wu J, Steinle A, Phillips JH, Lanier LL, et al. Activation of NK cells and T cells by NKG2D, a receptor for stress-inducible MICA. Science (1999) 285(5428):727–9. doi: 10.1126/science.285.5428.727
56. Glienke J, Sobanov Y, Brostjan C, Steffens C, Nguyen C, Lehrach H, et al. The genomic organization of NKG2C, e, f, and d receptor genes in the human natural killer gene complex. Immunogenetics (1998) 48(3):163–73. doi: 10.1007/s002510050420
57. Bellora F, Castriconi R, Dondero A, Reggiardo G, Moretta L, Mantovani A, et al. The interaction of human natural killer cells with either unpolarized or polarized macrophages results in different functional outcomes. Proc Natl Acad Sci USA (2010) 107(50):21659–64. doi: 10.1073/pnas.1007654108
58. Degli-Esposti MA, Smyth MJ. Close encounters of different kinds: dendritic cells and NK cells take centre stage. Nat Rev Immunol (2005) 5(2):112–24. doi: 10.1038/nri1549
59. Shanker A, Buferne M, Schmitt-Verhulst AM. Cooperative action of CD8 T lymphocytes and natural killer cells controls tumour growth under conditions of restricted T-cell receptor diversity. Immunology (2010) 129(1):41–54. doi: 10.1111/j.1365-2567.2009.03150.x
60. Ferlazzo G, Tsang ML, Moretta L, Melioli G, Steinman RM, Munz C. Human dendritic cells activate resting natural killer (NK) cells and are recognized via the NKp30 receptor by activated NK cells. J Exp Med (2002) 195(3):343–51. doi: 10.1084/jem.20011149
61. Gerosa F, Baldani-Guerra B, Nisii C, Marchesini V, Carra G, Trinchieri G. Reciprocal activating interaction between natural killer cells and dendritic cells. J Exp Med (2002) 195(3):327–33. doi: 10.1084/jem.20010938
62. O'Leary JG, Goodarzi M, Drayton DL, von Andrian UH. T Cell- and b cell-independent adaptive immunity mediated by natural killer cells. Nat Immunol (2006) 7(5):507–16. doi: 10.1038/ni1332
63. Paust S, Gill HS, Wang BZ, Flynn MP, Moseman EA, Senman B, et al. Critical role for the chemokine receptor CXCR6 in NK cell-mediated antigen-specific memory of haptens and viruses. Nat Immunol (2010) 11(12):1127–35. doi: 10.1038/ni.1953
64. Cooper MA, Elliott JM, Keyel PA, Yang L, Carrero JA, Yokoyama WM. Cytokine-induced memory-like natural killer cells. Proc Natl Acad Sci USA (2009) 106(6):1915–9. doi: 10.1073/pnas.0813192106
65. Bancroft GJ, Shellam GR, Chalmer JE. Genetic influences on the augmentation of natural killer (NK) cells during murine cytomegalovirus infection: correlation with patterns of resistance. J Immunol (1981) 126(3):988–94. doi: 10.4049/jimmunol.126.3.988
66. Biron CA, Byron KS, Sullivan JL. Severe herpesvirus infections in an adolescent without natural killer cells. N Engl J Med (1989) 320(26):1731–5. doi: 10.1056/NEJM198906293202605
67. Bukowski JF, Woda BA, Habu S, Okumura K, Welsh RM. Natural killer cell depletion enhances virus synthesis and virus-induced hepatitis in vivo. J Immunol (1983) 131(3):1531–8. doi: 10.4049/jimmunol.131.3.1531
68. Bukowski JF, Woda BA, Welsh RM. Pathogenesis of murine cytomegalovirus infection in natural killer cell-depleted mice. J Virol (1984) 52(1):119–28. doi: 10.1128/jvi.52.1.119-128.1984
69. Shellam GR, Allan JE, Papadimitriou JM, Bancroft GJ. Increased susceptibility to cytomegalovirus infection in beige mutant mice. Proc Natl Acad Sci USA (1981) 78(8):5104–8. doi: 10.1073/pnas.78.8.5104
70. Smith HR, Heusel JW, Mehta IK, Kim S, Dorner BG, Naidenko OV, et al. Recognition of a virus-encoded ligand by a natural killer cell activation receptor. Proc Natl Acad Sci USA (2002) 99(13):8826–31. doi: 10.1073/pnas.092258599
71. Beaulieu AM, Zawislak CL, Nakayama T, Sun JC. The transcription factor Zbtb32 controls the proliferative burst of virus-specific natural killer cells responding to infection. Nat Immunol (2014) 15(6):546–53. doi: 10.1038/ni.2876
72. Karo JM, Schatz DG, Sun JC. The RAG recombinase dictates functional heterogeneity and cellular fitness in natural killer cells. Cell (2014) 159(1):94–107. doi: 10.1016/j.cell.2014.08.026
73. Madera S, Sun JC. Cutting edge: stage-specific requirement of IL-18 for antiviral NK cell expansion. J Immunol (2015) 194(4):1408–12. doi: 10.4049/jimmunol.1402001
74. Min-Oo G, Bezman NA, Madera S, Sun JC, Lanier LL. Proapoptotic bim regulates antigen-specific NK cell contraction and the generation of the memory NK cell pool after cytomegalovirus infection. J Exp Med (2014) 211(7):1289–96. doi: 10.1084/jem.20132459
75. O'Sullivan TE, Johnson LR, Kang HH, Sun JC. BNIP3- and BNIP3L-mediated mitophagy promotes the generation of natural killer cell memory. Immunity (2015) 43(2):331–42. doi: 10.1016/j.immuni.2015.07.012
76. Sun JC, Madera S, Bezman NA, Beilke JN, Kaplan MH, Lanier LL. Proinflammatory cytokine signaling required for the generation of natural killer cell memory. J Exp Med (2012) 209(5):947–54. doi: 10.1084/jem.20111760
77. Sun JC, Beilke JN, Lanier LL. Adaptive immune features of natural killer cells. Nature (2009) 457(7229):557–61. doi: 10.1038/nature07665
78. Sun JC, Beilke JN, Lanier LL. Immune memory redefined: characterizing the longevity of natural killer cells. Immunol Rev (2010) 236:83–94. doi: 10.1111/j.1600-065X.2010.00900.x
79. Hammer Q, Ruckert T, Borst EM, Dunst J, Haubner A, Durek P, et al. Peptide-specific recognition of human cytomegalovirus strains controls adaptive natural killer cells. Nat Immunol (2018) 19(5):453–63. doi: 10.1038/s41590-018-0082-6
80. Guma M, Cabrera C, Erkizia I, Bofill M, Clotet B, Ruiz L, et al. Human cytomegalovirus infection is associated with increased proportions of NK cells that express the CD94/NKG2C receptor in aviremic HIV-1-positive patients. J Infect Dis (2006) 194(1):38–41. doi: 10.1086/504719
81. Bjorkstrom NK, Lindgren T, Stoltz M, Fauriat C, Braun M, Evander M, et al. Rapid expansion and long-term persistence of elevated NK cell numbers in humans infected with hantavirus. J Exp Med (2011) 208(1):13–21. doi: 10.1084/jem.20100762
82. Brunetta E, Fogli M, Varchetta S, Bozzo L, Hudspeth KL, Marcenaro E, et al. Chronic HIV-1 viremia reverses NKG2A/NKG2C ratio on natural killer cells in patients with human cytomegalovirus co-infection. AIDS (2010) 24(1):27–34. doi: 10.1097/QAD.0b013e3283328d1f
83. Gregson JN, Kuri-Cervantes L, Mela CM, Gazzard BG, Bower M, Goodier MR. Short communication: NKG2C+ NK cells contribute to increases in CD16+CD56- cells in HIV type 1+ individuals with high plasma viral load. AIDS Res Hum Retroviruses (2013) 29(1):84–8. doi: 10.1089/aid.2011.0397
84. Oliviero B, Varchetta S, Paudice E, Michelone G, Zaramella M, Mavilio D, et al. Natural killer cell functional dichotomy in chronic hepatitis b and chronic hepatitis c virus infections. Gastroenterology (2009) 137(3):1151–60, 60.e1-7. doi: 10.1053/j.gastro.2009.05.047
85. Petitdemange C, Becquart P, Wauquier N, Beziat V, Debre P, Leroy EM, et al. Unconventional repertoire profile is imprinted during acute chikungunya infection for natural killer cells polarization toward cytotoxicity. PLoS Pathog (2011) 7(9):e1002268. doi: 10.1371/journal.ppat.1002268
86. Saghafian-Hedengren S, Sohlberg E, Theorell J, Carvalho-Queiroz C, Nagy N, Persson JO, et al. Epstein-Barr Virus coinfection in children boosts cytomegalovirus-induced differentiation of natural killer cells. J Virol (2013) 87(24):13446–55. doi: 10.1128/JVI.02382-13
87. Guma M, Angulo A, Vilches C, Gomez-Lozano N, Malats N, Lopez-Botet M. Imprint of human cytomegalovirus infection on the NK cell receptor repertoire. Blood (2004) 104(12):3664–71. doi: 10.1182/blood-2004-05-2058
88. Miletic A, Krmpotic A, Jonjic S. The evolutionary arms race between NK cells and viruses: who gets the short end of the stick? Eur J Immunol (2013) 43(4):867–77. doi: 10.1002/eji.201243101
89. Gabor F, Jahn G, Sedmak DD, Sinzger C. In vivo downregulation of MHC class I molecules by HCMV occurs during all phases of viral replication but is not always complete. Front Cell Infect Microbiol (2020) 10:283. doi: 10.3389/fcimb.2020.00283
90. Hengel H, Koopmann JO, Flohr T, Muranyi W, Goulmy E, Hammerling GJ, et al. A viral ER-resident glycoprotein inactivates the MHC-encoded peptide transporter. Immunity (1997) 6(5):623–32. doi: 10.1016/S1074-7613(00)80350-7
91. Ahn K, Angulo A, Ghazal P, Peterson PA, Yang Y, Fruh K. Human cytomegalovirus inhibits antigen presentation by a sequential multistep process. Proc Natl Acad Sci USA (1996) 93(20):10990–5. doi: 10.1073/pnas.93.20.10990
92. van der Wal FJ, Kikkert M, Wiertz E. The HCMV gene products US2 and US11 target MHC class I molecules for degradation in the cytosol. Curr Top Microbiol Immunol (2002) 269:37–55. doi: 10.1007/978-3-642-59421-2_3
93. Beck S, Barrell BG. Human cytomegalovirus encodes a glycoprotein homologous to MHC class-I antigens. Nature (1988) 331(6153):269–72. doi: 10.1038/331269a0
94. Chapman TL, Heikeman AP, Bjorkman PJ. The inhibitory receptor LIR-1 uses a common binding interaction to recognize class I MHC molecules and the viral homolog UL18. Immunity (1999) 11(5):603–13. doi: 10.1016/S1074-7613(00)80135-1
95. Farrell HE, Vally H, Lynch DM, Fleming P, Shellam GR, Scalzo AA, et al. Inhibition of natural killer cells by a cytomegalovirus MHC class I homologue in vivo. Nature (1997) 386(6624):510–4. doi: 10.1038/386510a0
96. Wang EC, McSharry B, Retiere C, Tomasec P, Williams S, Borysiewicz LK, et al. UL40-mediated NK evasion during productive infection with human cytomegalovirus. Proc Natl Acad Sci USA (2002) 99(11):7570–5. doi: 10.1073/pnas.112680099
97. Kotenko SV, Saccani S, Izotova LS, Mirochnitchenko OV, Pestka S. Human cytomegalovirus harbors its own unique IL-10 homolog (cmvIL-10). Proc Natl Acad Sci USA (2000) 97(4):1695–700. doi: 10.1073/pnas.97.4.1695
98. Chang WL, Baumgarth N, Yu D, Barry PA. Human cytomegalovirus-encoded interleukin-10 homolog inhibits maturation of dendritic cells and alters their functionality. J Virol (2004) 78(16):8720–31. doi: 10.1128/JVI.78.16.8720-8731.2004
99. Holder KA, Grant MD. Human cytomegalovirus IL-10 augments NK cell cytotoxicity. J Leukoc Biol (2019) 106(2):447–54. doi: 10.1002/JLB.2AB0418-158RR
100. Beziat V, Liu LL, Malmberg JA, Ivarsson MA, Sohlberg E, Bjorklund AT, et al. NK cell responses to cytomegalovirus infection lead to stable imprints in the human KIR repertoire and involve activating KIRs. Blood (2013) 121(14):2678–88. doi: 10.1182/blood-2012-10-459545
101. Green ML, Leisenring WM, Xie H, Walter RB, Mielcarek M, Sandmaier BM, et al. CMV reactivation after allogeneic HCT and relapse risk: evidence for early protection in acute myeloid leukemia. Blood (2013) 122(7):1316–24. doi: 10.1182/blood-2013-02-487074
102. Ito S, Pophali P, Co W, Koklanaris EK, Superata J, Fahle GA, et al. CMV reactivation is associated with a lower incidence of relapse after allo-SCT for CML. Bone Marrow Transpl (2013) 48(10):1313–6. doi: 10.1038/bmt.2013.49
103. Basilio-Queiros D, Venturini L, Luther-Wolf S, Dammann E, Ganser A, Stadler M, et al. Adaptive NK cells undergo a dynamic modulation in response to human cytomegalovirus and recruit T cells in in vitro migration assays. Bone Marrow Transpl (2022) 57(5):712–20. doi: 10.1038/s41409-022-01603-y
104. Schlums H, Cichocki F, Tesi B, Theorell J, Beziat V, Holmes TD, et al. Cytomegalovirus infection drives adaptive epigenetic diversification of NK cells with altered signaling and effector function. Immunity (2015) 42(3):443–56. doi: 10.1016/j.immuni.2015.02.008
105. Lee J, Zhang T, Hwang I, Kim A, Nitschke L, Kim M, et al. Epigenetic modification and antibody-dependent expansion of memory-like NK cells in human cytomegalovirus-infected individuals. Immunity (2015) 42(3):431–42. doi: 10.1016/j.immuni.2015.02.013
106. Dickinson RE, Milne P, Jardine L, Zandi S, Swierczek SI, McGovern N, et al. The evolution of cellular deficiency in GATA2 mutation. Blood (2014) 123(6):863–74. doi: 10.1182/blood-2013-07-517151
107. Spinner MA, Sanchez LA, Hsu AP, Shaw PA, Zerbe CS, Calvo KR, et al. GATA2 deficiency: a protean disorder of hematopoiesis, lymphatics, and immunity. Blood (2014) 123(6):809–21. doi: 10.1182/blood-2013-07-515528
108. Schlums H, Jung M, Han H, Theorell J, Bigley V, Chiang SC, et al. Adaptive NK cells can persist in patients with GATA2 mutation depleted of stem and progenitor cells. Blood (2017) 129(14):1927–39. doi: 10.1182/blood-2016-08-734236
109. Brodsky RA. Paroxysmal nocturnal hemoglobinuria. Blood (2014) 124(18):2804–11. doi: 10.1182/blood-2014-02-522128
110. Corat MA, Schlums H, Wu C, Theorell J, Espinoza DA, Sellers SE, et al. Acquired somatic mutations in PNH reveal long-term maintenance of adaptive NK cells independent of HSPCs. Blood (2017) 129(14):1940–6. doi: 10.1182/blood-2016-08-734285
111. Mody CH, Ogbomo H, Xiang RF, Kyei SK, Feehan D, Islam A, et al. Microbial killing by NK cells. J Leukoc Biol (2019) 105(6):1285–96. doi: 10.1002/JLB.MR0718-298R
112. Erkeller-Yuksel FM, Lydyard PM, Isenberg DA. Lack of NK cells in lupus patients with renal involvement. Lupus (1997) 6(9):708–12. doi: 10.1177/096120339700600905
113. Erkeller-Yusel F, Hulstaart F, Hannet I, Isenberg D, Lydyard P. Lymphocyte subsets in a large cohort of patients with systemic lupus erythematosus. Lupus (1993) 2(4):227–31. doi: 10.1177/0961203393002001081
114. Schepis D, Gunnarsson I, Eloranta ML, Lampa J, Jacobson SH, Karre K, et al. Increased proportion of CD56bright natural killer cells in active and inactive systemic lupus erythematosus. Immunology (2009) 126(1):140–6. doi: 10.1111/j.1365-2567.2008.02887.x
115. Dalbeth N, Callan MF. A subset of natural killer cells is greatly expanded within inflamed joints. Arthritis Rheumatol (2002) 46(7):1763–72. doi: 10.1002/art.10410
116. Gur C, Porgador A, Elboim M, Gazit R, Mizrahi S, Stern-Ginossar N, et al. The activating receptor NKp46 is essential for the development of type 1 diabetes. Nat Immunol (2010) 11(2):121–8. doi: 10.1038/ni.1834
117. Brandau S, Riemensberger J, Jacobsen M, Kemp D, Zhao W, Zhao X, et al. NK cells are essential for effective BCG immunotherapy. Int J Cancer (2001) 92(5):697–702. doi: 10.1002/1097-0215(20010601)92:5<697::AID-IJC1245>3.0.CO;2-Z
Keywords: natural killer cells, cytotoxic lymphocytes, adaptive natural killer cells, viral infections, immune, Cytomegalovirus
Citation: Basílio-Queirós D and Mischak-Weissinger E (2023) Natural killer cells- from innate cells to the discovery of adaptability. Front. Immunol. 14:1172437. doi: 10.3389/fimmu.2023.1172437
Received: 23 February 2023; Accepted: 04 May 2023;
Published: 18 May 2023.
Edited by:
Pietro Ghezzi, University of Urbino Carlo Bo, ItalyReviewed by:
Frank Momburg, German Cancer Research Center (DKFZ), GermanyKayla Holder, Memorial University of Newfoundland, Canada
Copyright © 2023 Basílio-Queirós and Mischak-Weissinger. This is an open-access article distributed under the terms of the Creative Commons Attribution License (CC BY). The use, distribution or reproduction in other forums is permitted, provided the original author(s) and the copyright owner(s) are credited and that the original publication in this journal is cited, in accordance with accepted academic practice. No use, distribution or reproduction is permitted which does not comply with these terms.
*Correspondence: Débora Basílio-Queirós, cXVlaXJvcy5kZWJvcmFAbWgtaGFubm92ZXIuZGU=