- Department of Dermatology, Johns Hopkins University School of Medicine, Baltimore, MD, United States
Staphylococcus aureus is a leading cause of bacteremia, further complicated by the emergence of antibiotic-resistant strains such as methicillin-resistant S. aureus (MRSA). A better understanding of host defense mechanisms is needed for the development of host-directed therapies as an alternative approach to antibiotics. The levels of IL-1, IL-17, and TNF-α cytokines in circulation have been associated with predictive outcomes in patients with S. aureus bacteremia. However, their causative role in survival and the cell types involved in these responses during bacteremia is not entirely clear. Using a mouse model of S. aureus bacteremia, we demonstrated that IL-17A/F and TNF-α had no significant impact on survival, whereas IL-1R signaling was critical for survival during S. aureus bacteremia. Furthermore, we identified that T cells, but not neutrophils, monocytes/macrophages, or endothelial cells were the crucial cell type for IL-1R-mediated survival against S. aureus bacteremia. Finally, we determined that the expression of IL-1R on γδ T cell, but not CD4+ or CD8+ T cells was responsible for survival against the S. aureus bacteremia. Taken together, we uncovered a role for IL-1R, but not IL-17A/F and TNF-α in protection against S. aureus bacteremia. Importantly, γδ T cell-intrinsic expression of IL-1R was crucial for survival, but not on other immune cells or endothelial cells. These findings reveal potential cellular and immunological targets for host-directed therapies for improved outcomes against S. aureus bacteremia.
1 Introduction
Staphylococcus aureus is a leading cause of bacteremia (1), with a mortality rate of ~25% due to the emergence of antibiotic-resistant strains such as methicillin-resistant S. aureus (MRSA) (2). Furthermore, all vaccines to date have failed in clinical trials against S. aureus invasive infections (3, 4). Thus, a better understanding of host defense mechanisms is needed for the development of host-directed therapies as an alternative approach to antibiotics.
The IL-1, IL-17, and TNF-α cytokines have been implicated in host defense against S. aureus skin and orthopedic infections (5–8). Moreover, IL-1, IL-17, and TNF-α cytokine levels in circulation have been associated with predictive outcomes in patients with S. aureus bacteremia (4, 9–12). For instance, elevated IL-1β at the time of patient admission correlated with reduced duration of the S. aureus bacteremia (11). However, whether the IL-1, IL-17, and TNF-α cytokines have a causative role in host survival and the cell types involved in these responses during S. aureus bacteremia is not entirely clear.
In this study, we evaluated the contributions of IL-1α/β, IL-17A/F, and TNF-α to host survival during S. aureus bacteremia using a preclinical mouse model. Furthermore, we identified the specific cell types that promote host survival using mice with specific deletion of IL-1R on T cells, myeloid cells, neutrophils, and endothelial cells.
2 Materials and methods
2.1 Bacterial preparation
The community-acquired methicillin-resistant S. aureus (MRSA) USA300 SF8300 strain, a kind gift from Dr. Binh Diep (UCSF), was cultured in tryptic soy broth (TSB) as previously described (13, 14). Briefly, SF8300 was streaked onto a tryptic soy agar (TSA) plate (TSB plus 1.5% bacto agar (BD Biosciences)) and grown overnight at 37°C in a bacterial incubator. Two to three single colonies were picked and cultured in TSB at 37°C in a shaking incubator (240 rpm) overnight (18 h), followed by a 1:50 subculture at 37°C for 2 h to obtain mid-logarithmic phase bacteria. The bacteria were pelleted, washed 3 times with sterile PBS, resuspended in sterile freezing medium (10% glycerol in sterile PBS) at a concentration of 1×1010 CFU/ml and aliquots stored in cryovials at -80°C until needed. The number of CFUs was confirmed with overnight culture on TSA plates.
2.2 Mice
Age-matched 6-8-week-old female mice on C57BL/6 background were used for all experiments. The IL-1α–/–, IL-1β–/–, and IL-17A/F–/– mice were provided by Dr. Yoichiro Iwakura (University of Tokyo). The VE-CadCre×IL-1Rfl/fl (VE-Cad-IL-1R–/–) mice, which lack IL-1R signaling in endothelial cells were provided by Dr. Michael O’Connell (NIH/NIAID). WT C57BL/6, TNF-α–/– (B6.129S-tnftm1Gkl/J), IL-1R–/– (B6.129S7-l1r1tm1Imx/J), LckCre (B6.Cg-Tg(Lck-cre)548Jxm/J), LysMCre (B6.129P2-Lyz2tm1(cre)Ifo/J), CD4Cre (Tg(Cd4-cre)1Cwi/BfluJ), S100A8Cre (B6.Cg-Tg(S100A8-cre,-EGFP)1Ilw/J), TCRδCreER (B6.129S-Tcrdtm1.1(cre/ERT2)Zhu/J), and IL-1Rfl/fl mice (B6.129(Cg)-Il1r1tm1.1Rbl/J) were obtained from Jackson Laboratories (Bar Harbor, ME).
LckCre mice were crossed with IL-1Rfl/fl mice to obtain LckCre×IL-1Rfl/fl (Lck-IL-1R–/–), which lack IL-1R signaling in pan-T cells. LysMCre were crossed with IL-1Rfl/fl mice to obtain LysMCre×IL-1Rfl/fl (LysM-IL-1R–/–) mice, which lack IL-1R signaling in myeloid cells. S100A8Cre were crossed with IL-1Rfl/fl mice to obtain S100A8Cre×IL1Rfl/fl (S100A8-IL-1R–/–) mice, which lack IL-1R signaling in neutrophils. CD4Cre were crossed with IL-1Rfl/fl mice to obtain CD4Cre×IL1Rfl/fl (CD4-IL-1R–/–) mice, which lack IL-1R signaling in CD4-expressing cells, including both CD4+ and CD8+ T cells (due to dual expression of CD4 in both T cell types during thymic development). TCRδCreER mice were crossed with IL-1Rfl/fl mice to obtain TCRδCreER×IL1Rfl/fl (TCRδ-IL-1R–/–) mice, which lack IL-1R signaling in γδ T cells upon tamoxifen-inducible deletion.
2.3 Study approval
All mouse strains were bred and maintained under the same specific pathogen-free conditions, with air-isolated cages at an American Association for the Accreditation of Laboratory Animal Care (AAALAC)-accredited animal facility at Johns Hopkins University and handled according to procedures described in the Guide for the Care and Use of Laboratory Animals as well as Johns Hopkins University’s policies and procedures as outlined in the Johns Hopkins University Animal Care and Use Training Manual. This study was approved by the Johns Hopkins Animal Care and Use Committee (Protocol #: MO21M378).
2.4 Intravenous infection
The S. aureus bacteremia model was modified from previously described protocols (15, 16). Briefly, 6-to-8-week-old female C57BL/6 mice were anesthetized (inhalation of 2% isoflurane) and inoculated intravenously with 4.8-5.8 × 107 SF8300 in a 100-μL volume of PBS using a 29-gauge insulin syringe via the retro-orbital vein to achieve an LD90.
2.5 Tamoxifen-inducible deletion of IL-1R
The inducible deletion of IL-1R on γδ T cells was modified from a previously described protocol (17). The TCRδ-IL-1R–/– mice were treated daily with 100 μl of 1 mg/ml tamoxifen in sunflower oil injected intraperitoneally for 5 consecutive days. The bacteremia infections were performed 10 days after the last tamoxifen injection. Wild-type (WT) mice were subjected to the same tamoxifen regimen when paired with TCRδ-IL-1R–/– mice. Tamoxifen-inducible deletion of IL-1R was confirmed by flow cytometry, which was comparable to the ~60% deletion efficiency in γδ T cells in TCRδcreER mice based on prior reports (18).
2.6 Flow cytometry
For flow cytometric analysis, 100 μl of peripheral blood and spleen was collected from tamoxifen-treated WT and TCRδ-IL-1R–/– mice 3h after intravenous infection. Red blood cells were lysed with ACK lysis buffer (ThermoFisher Scientific) and cells were resuspended in FACS buffer (PBS containing 1% BSA and 2mM EDTA). Spleen was manually pushed through a cell separation filter (40 µm) and resuspended in FACS buffer. Single cell suspensions were stained for viability (Viobility 405/520 viability kit, Miltenyi Biotec) and TruStain fcX (Biolegend) was used to block Fc receptor binding. Next, blood single cells were surface stained with the following mAbs: PE-Vio770-CD3 (REA641, Miltenyi Biotec), PE-CD8a (REA601, Miltenyi Biote), APC-Vio770-CD4 (REA604, Miltenyi Biote) VioBlue-TCRγδ (REA633, Miltenyi Biotec), and APC-CD121α (clone JAMA-147, BioLegend). The γδ T cells were identified as CD3+CD4-CD8-TCRγδ+ cells from the live cell population. Spleen single cells were surface stained with the following mAbs: PerCP-Vio700-CD45 (REA737, Miltenyi Biotec), APC-CD11b (REA592, Miltenyi Biotec), VioBlue-Ly6C (REA796, Miltenyi Biotec), APC-Vio770-Ly6G (REA526, Miltenyi Biotec), and PE-Vio770-F4/80 (REA126, Miltenyi Biotec). Cell acquisition was performed on a MACSQuant analyzer (Miltenyi Biotec) and data analyzed using MACSQuantify software (Miltenyi Biotec). See Supplementary Figure 1 for gating strategy.
2.7 Ex vivo CFU enumeration
At 3h post infection, mice were euthanized, and the spleen, liver, and kidneys were harvested and ex vivo CFU were isolated as previously described (5, 19). The tissue specimens were homogenized (PRO200 Series homogenizer; PRO Scientific) and then serially diluted and cultured overnight on TSA plates at 37°C. Ex vivo CFU from the homogenized tissue were then enumerated from the plates.
2.8 Statistical analyses
Survival rates were compared by log rank (Mantel-Cox) test and data from single comparisons analyzed by Student’s t test (two-tailed), as indicated in the figure legends. All statistical analyses were calculated with Prism software (GraphPad 9.5 Software, La Jolla, California). CFU data are presented as geometric mean ± geometric standard deviation (SD). All other data are presented as mean ± standard error of the mean (SEM) and values of P <0.05 were considered statistically significant.
3 Results
3.1 IL-1R signaling improves survival during S. aureus bacteremia
The levels of IL-1, IL-17, and TNF-α cytokines in circulation have been associated with predictive outcomes in patients with S. aureus bacteremia (4, 9–12). Therefore, we set out to determine the mechanistic effect of IL-1α/β, TNF-α, and IL-17A/F on survival during S. aureus bacteremia using a preclinical mouse model whereby 4.8-5.8 × 107 CFUs of S. aureus USA300 (SF8300) were injected i.v. and survival measured over time (15, 16). To determine the role of IL-1R signaling, we first performed our bacteremia model on wild-type (WT) C57BL/6 and IL-1R–/– mice and found that IL-1R–/– mice had a statistically significant decrease in survival compared to WT mice (Figure 1A). Since IL-1α and IL-1β signal through the IL-1R (20), we next tested IL-1α–/– and IL-1β–/– mice and discovered that both IL-1α–/– and IL-1β–/– mice had a markedly reduced survival compared to WT mice (Figure 1A). Next, we examined IL-17A/F–/– and TNF-α –/– mice and found no statistically significant differences compared to WT mice (Figure 1B). Taken together, our data indicated that IL-1α and IL-1β signaling via IL-1R enhanced survival during S. aureus bacteremia infections.
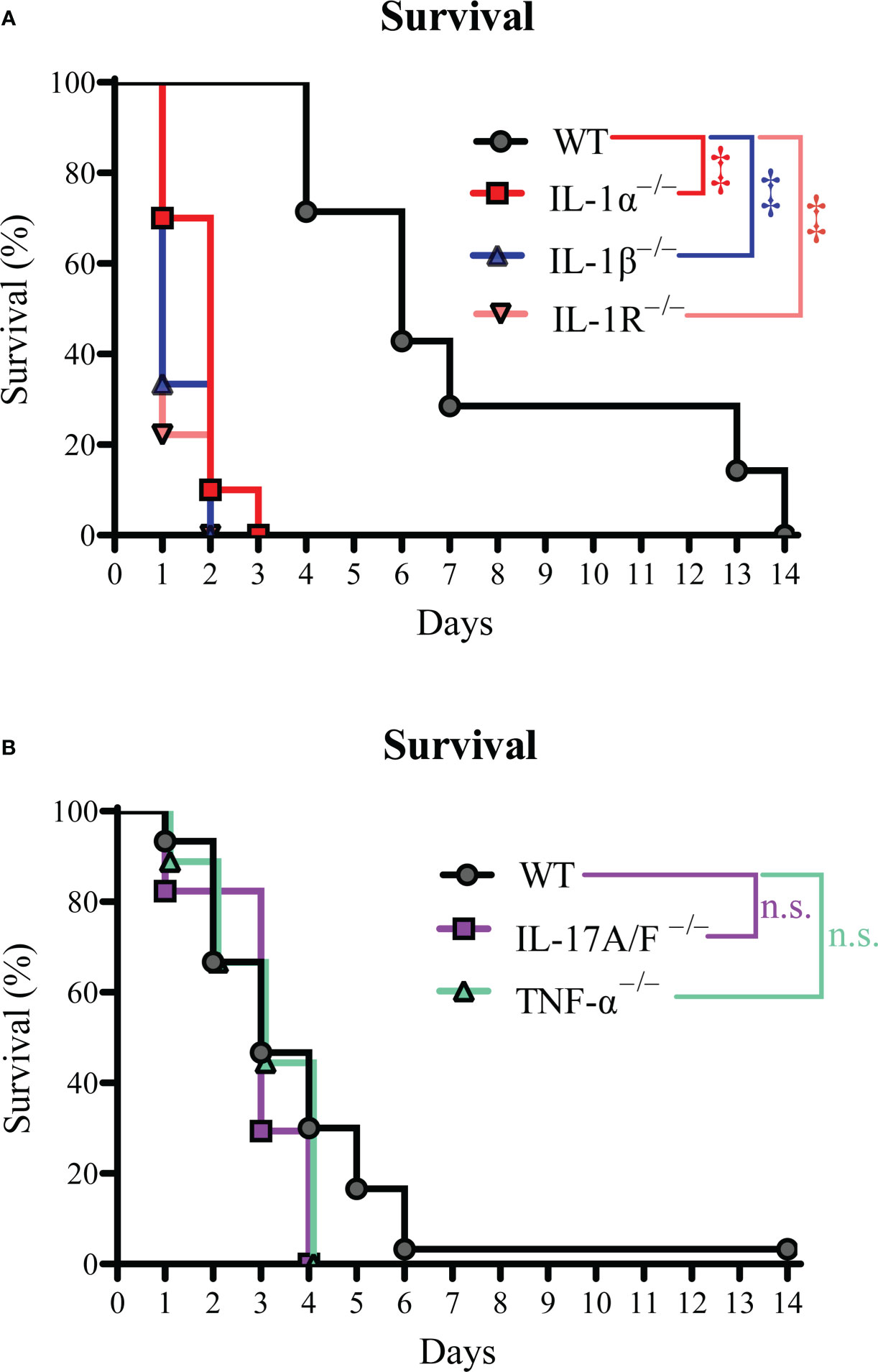
Figure 1 IL-1R signaling improves survival during S. aureus bacteremia. The S. aureus bacteremia infection was performed on WT, IL-1α–/–, IL-1β–/–, IL-1R–/–, IL-17A/F–/–, and TNF-α–/– mice. (A) Survival (%) of WT, IL-1α–/–, IL-1β–/–, and IL-1R–/– mice (n=7-10 per group, average inoculum = 4.8 × 107 CFUs). (B) Survival (%) of WT, IL-17A/F–/–, and TNF-α–/– mice (n=9-30 per group, average inoculum = 5.4 × 107 CFUs). ‡P < 0.001 and n.s. = not significant; WT versus IL-1α–/–, IL-1β–/–, IL-1R–/–, IL-17A/F–/–, and TNF-α–/– as calculated by log rank (Mantel-Cox) test. Data were combined from at least 2 independent experiments.
3.2 γδ T cell-intrinsic IL-1R signaling promotes survival during S. aureus bacteremia
Since IL-1R signaling was important for survival during S. aureus bacteremia infections, we next elucidated the specific cell types involved in the IL-1R response. Various cell types use IL-1R signaling to drive host defense and inflammation (20), including myeloid cells, T cells, and non-immune cells (21). Thus, we developed mice with specific deletion of IL-1R in T cells (Lck-IL-1R–/–), myeloid cells (LysM-IL-1R–/–), and neutrophils (S100A8-IL-1R–/–). We also used mice with specific deletion of IL-1R in endothelial cells (VE-Cad-IL-1R–/–), since S. aureus interacts with endothelial cells upon bacteremia infections (22). We discovered that only the Lck-IL-1R–/– mice had a significant defect in survival compared to WT mice (Figure 2A), suggesting that IL-1R signaling on T cells, but not myeloid cells, neutrophils, or endothelial cells was important for host survival.
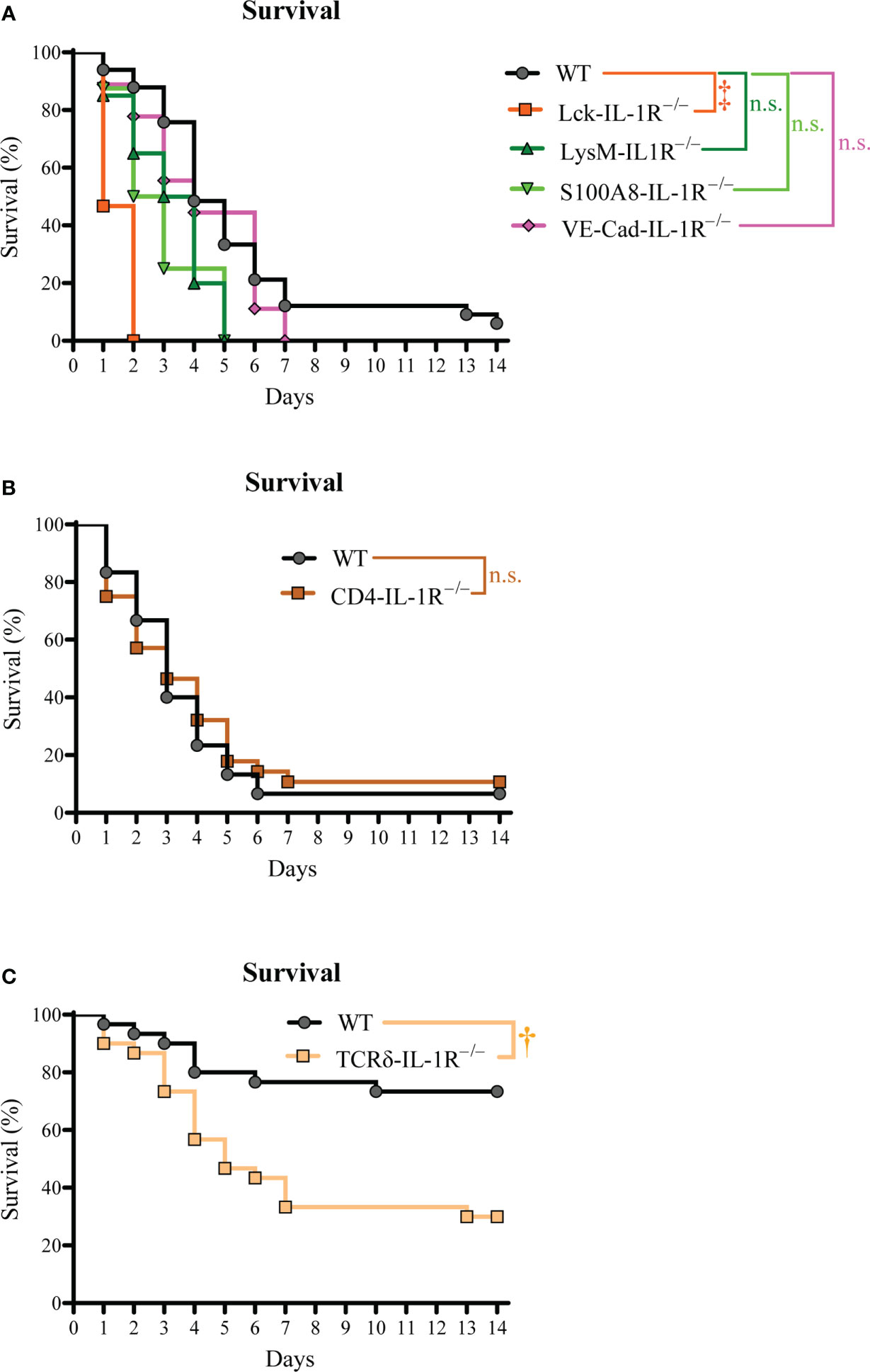
Figure 2 γδ T cell-intrinsic IL-1R signaling promotes survival during S. aureus bacteremia. The S. aureus bacteremia infection was performed on Lck-IL-1R–/–, LysM-IL-1R–/–, S100A8-IL-1R–/–, VE-Cad-IL-1R–/–, CD4-IL-1R–/–, and TCRδ-IL-1R–/– mice. (A) Survival (%) of Lck-IL-1R–/–, LysM-IL-1R–/–, S100A8-IL-1R–/–, VE-Cad-IL-1R–/– mice (n=8-35 per group, average inoculum = 5.4 × 107 CFUs). (B) Survival (%) of WT and CD4-IL-1R–/– mice (n=28-30 per group, average inoculum = 5.4 × 107 CFUs). (C) Survival (%) of WT and TCRδ-IL-1R–/– mice (n=29 per group, average inoculum = 5.4 × 107 CFUs). †P < 0.01, ‡P < 0.001, and n.s. = not significant; WT versus Lck-IL-1R–/–, LysM-IL-1R–/–, S100A8-IL-1R–/–, VE-Cad-IL-1R–/–, CD4-IL-1R–/–, TCRδ-IL-1R–/– as calculated by log rank (Mantel-Cox) test. Data were combined from at least 2 independent experiments.
We next determined the specific T cell subset required for IL-1R signaling, since CD4+ and γδ T cells are reported to be involved in host defense against S. aureus infections (7, 23–25). To this end, we developed and tested mice with specific deletion of IL-1R in CD4+ T cells (CD4-IL-1R–/–) and tamoxifen-inducible deletion of IL-1R in γδ T cells (TCRδ-IL-1R–/–). We discovered that CD4-IL-1R–/– mice had no difference in survival compared to WT mice (Figure 2B). Interestingly, there was markedly decreased survival in TCRδ-IL-1R–/– mice compared to WT mice (Figure 2C). There was a trend towards increased circulating γδ T cells counts in TCRδ-IL-1R–/– mice compared to WT mice (Figure 3A). We confirmed tamoxifen-inducible deletion of IL-1R on γδ T cells in the TCRδ-IL-1R–/– mice by flow cytometry (Figure 3B). Collectively, IL-1R signaling on γδ T cells was important for survival during S. aureus bacteremia infections.
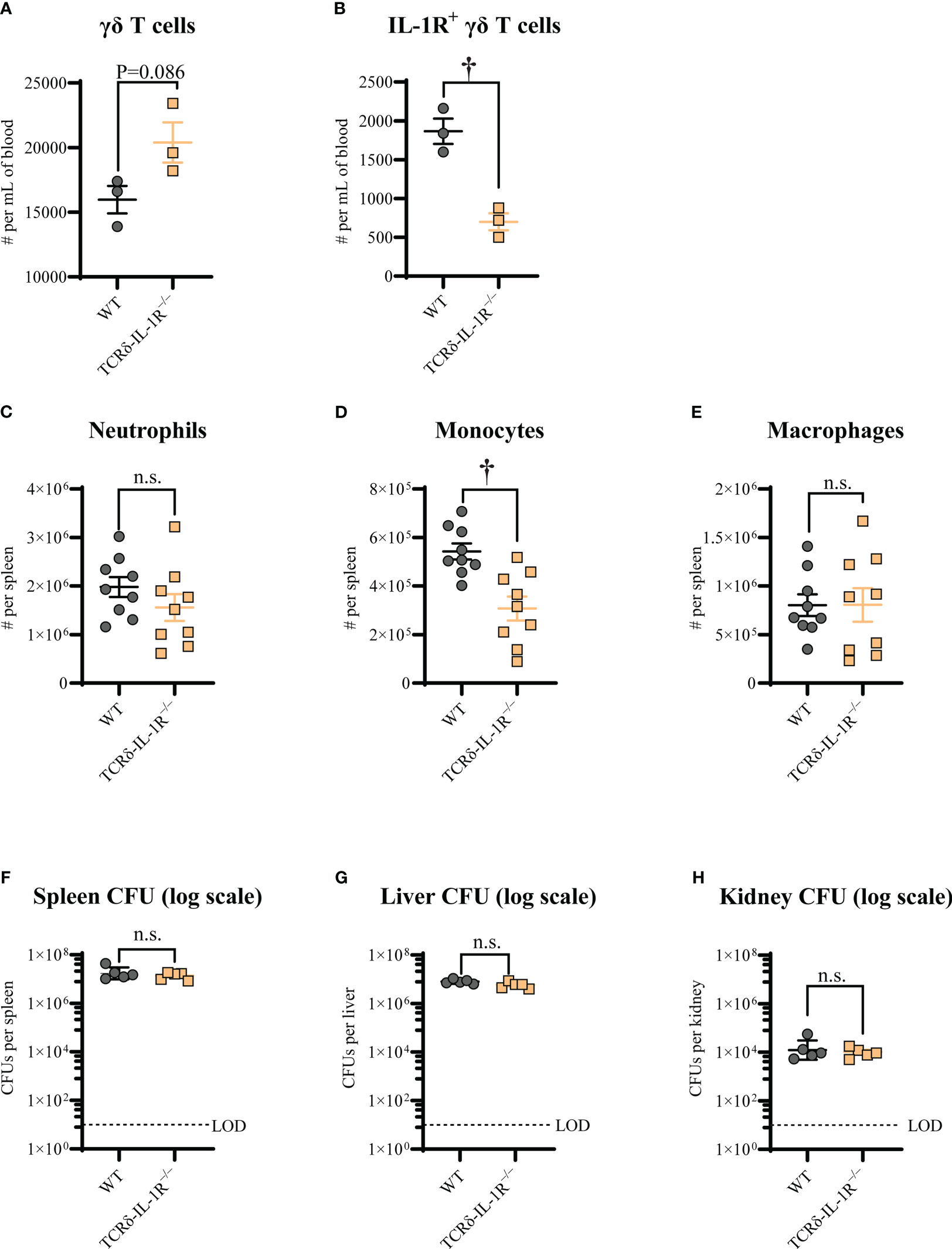
Figure 3 γδ T cell-intrinsic IL-1R signaling promotes monocyte recruitment during S. aureus bacteremia. WT and TCRδ-IL-1R–/– mice were treated daily with 100 μl of 1 mg/ml tamoxifen in sunflower oil injected intraperitoneally for 5 consecutive days. The bacteremia infections were performed 10 days after the last tamoxifen injection. After 3h post-infection, peripheral blood and spleen were collected for flow cytometry analysis, spleen, liver, and kidney were collected for ex vivo CFU enumeration. Mean cell numbers ± SEM for total γδ T cells (A), IL-1R+ γδ T cells (B), neutrophils (C), monocytes (D), and macrophages (E). Ex vivo CFU (geometric mean ± geometric SD) for spleen (F), liver (G), and kidney (H). n=3 per group for A and B, n=9 per group for C, D, and (E) n=5 per group for F, G, and (H) †P < 0.01 and n.s. = not significant; WT versus TCRδ-IL-1R–/– as calculated by Student’s t test. Data are combined or representative from 2 independent experiments.
3.3 γδ T cell-intrinsic IL-1R signaling increases monocyte recruitment to the spleen during S. aureus bacteremia
We next elucidated whether γδ T cell-intrinsic IL-1R signaling affected immune cell levels and S. aureus burden during the bacteremia. To this end, we first measured neutrophil, monocyte, and macrophage population levels in the spleens of TCRδ-IL-1R–/– and WT mice 3 hours post-infection. We found that monocytes, but not neutrophils or macrophages, were significantly decreased in TCRδ-IL-1R–/– mice compared to WT mice (Figures 3C-E). Next, we measured S. aureus CFUs in the spleen, liver, and kidney, but found no difference in bacterial burden between TCRδ-IL-1R–/– and WT mice (Figures 3F-H). These data indicated that γδ T cell-intrinsic IL-1R signaling promoted monocyte recruitment to the spleen during S. aureus bacteremia.
4 Discussion
The IL-1, IL-17, and TNF-α cytokines contribute to host defense against S. aureus skin and orthopedic infections (5–8). Although IL-1, IL-17, and TNF-α cytokine levels in circulation have been associated with predictive outcomes in patients with S. aureus bacteremia (4, 9–12), whether these cytokines mechanistically promote host survival and the cell types involved in these responses is under-investigated. Thus, we tested mice deficient in IL-1, IL-17, and TNF-α cytokines in a preclinical mouse model of S. aureus bacteremia and discovered that IL-1R signaling was important for host survival. Furthermore, we identified γδ T cells as the cell type that drives IL-1R-mediated host survival against S. aureus bacteremia. These results provide several important insights into the protective host responses during S. aureus bacteremia.
First, we found that IL-1R signaling contributed to host survival during S. aureus bacteremia, which aligns with previously published reports (26, 27). Similarly, IL-1R signaling promotes host defense against S. aureus skin, orthopedic, and pneumonia infections (5, 8, 28). Interestingly, we found that both IL-1α and IL-1β were important in our model, suggesting they have non-redundant roles in host survival. This may be explained by the differences in expression profiles between the cytokines. For instance, IL-1α is constitutively expressed in non-immune cell types (29), whereas IL-1β is induced (30). Moreover, IL-1α has a nuclear localization sequence that is absent in IL-1β (31), which has important implications in inflammation (32). Understanding the differential mechanisms of protection between IL-1α and IL-1β against S. aureus bacteremia will be the focus of future work.
We also discovered that TNF-α and IL-17A/F did not influence host survival during S. aureus bacteremia at the dose tested. This was unexpected, as both TNF-α and IL-17A/F drive host defense against S. aureus at other infection sites (e.g., skin and orthopedic implants) (5–7, 33, 34). However, in a baboon model of group A streptococcal bacteremia infection, anti-TNF-α monoclonal antibody therapy improved survival outcomes (35). Similarly, heightened TNF-α production correlated with persistent rather than resolving bacteremia in patients (12). Another possibility for the lack of phenotype in TNF-α deficient mice is that lymphotoxin-α signals through the TNF-α receptors (36, 37), which may have compensated for TNF-α deficiency in our S. aureus bacteremia model. Although IL-17A did not improve survival outcomes during bacteremia in our model, IL-17A limits the systemic dissemination of S. aureus from skin infection to kidneys (38). Thus, IL-17A may be more important in the control of S. aureus infections in the tissue rather than protection once bacteremia has occurred. Although not analyzed in this study, there may be a role for IL-10 in the infectious process during S. aureus bacteremia, as this cytokine correlates with mortality in humans (11, 12). Collectively, our findings do not support a role for TNF-α and IL-17A/F in survival outcomes during S. aureus bacteremia in our preclinical mouse model.
We uncovered that γδ T cell-intrinsic IL-1R signaling was crucial for host survival during S. aureus bacteremia. Our findings may relate to prior studies on the protective role of γδ T cells and other T cells against S. aureus skin infections and nasal colonization (7, 8, 39–41). In contrast, IL-1R signaling on non-hematopoietic cells was critical for protection against S. aureus skin infections (8). Thus, these findings indicate that the protective cell type that provides the IL-1R signal against S. aureus infections is context-dependent. Given that IL-1R deficient mice succumbed to S. aureus bacteremia within 2 days, our findings suggested that γδ T cell-mediated IL-1R signaling occurs soon after infection. In fact, γδ T cells are an innate source of pro-inflammatory responses driven by IL-1 cytokines independent of T cell receptor engagement (42, 43), perhaps explaining the importance of IL-1R signaling on this T cell subset for rapid protection against S. aureus bacteremia infections. However, since IL-17A/F cytokines were not important for host survival herein, and γδ T cells produce IL-17 cytokines in response to IL-1R signaling (42), it begs the question of how γδ T cell-intrinsic IL-1R signaling is mediating protection against S. aureus bacteremia? Our findings suggested that γδ T cell-intrinsic IL-1R signaling promotes monocyte recruitment to the spleen during S. aureus bacteremia as a mechanism of protection. This may relate to the known role of IL-1β to induce the monocyte-recruiting chemokine, CCL2 (44, 45). Other potential explanations include γδ T cell production of antimicrobial peptides, IL-22, and neutrophil recruiting chemokines to promote host survival (46, 47), which have been associated with protection against S. aureus at other infection sites (13, 48). Understanding the localization and mechanism of protection of the γδ T cell-specific IL-1R response during S. aureus bacteremia will be part of our future interrogations.
There were limitations. For instance, our study was conducted with a single S. aureus strain, limiting the broader conclusions of our findings. However, other studies have tested additional S. aureus strains in IL-1R deficient mice or mice treated with IL-1Ra with similar results (26, 27, 49), suggesting that IL-1R-mediated survival is not specific to a single S. aureus strain. Moreover, we used a high inoculum of S. aureus in the bacteremia model (i.e., LD90), which may have missed phenotypes present in a lower inoculum (e.g., LD50). Another limitation to the study is the possibility that the phenotypes in our cell-specific IL-1R deficient mice are due to changes in cytokine production in IL-1α and IL-1β rather than IL-1R-specific mechanisms. The use of tamoxifen to delete IL-1R in the TCRδ-IL-1R–/– mice may have influenced the immune responses upon the S. aureus bacteremia infection (e.g., neutrophil function) (50), which was observed in Figure 2C. To control for these effects, we similarly treated the control WT comparison group with tamoxifen. Importantly, deletion efficiency in γδ T cells in Lckcre and TCRδcreER mice is ∼20% and ∼60%, respectively (18, 51), leaving the possibility that IL-1R signaling on other T cell subsets not specifically tested in this study (e.g., NK T cells and MAIT cells) contributed to host survival during S. aureus bacteremia infections. Addressing these limitations will be performed in our future work.
Taken together, the results of this study indicate that γδ T cell-intrinsic IL-1R signaling promotes host survival during S. aureus bacteremia infections. Thus, IL-1R on γδ T cells may serve as a host-directed therapeutic target for the treatment of S. aureus bacteremia infections and potentially other antibiotic-resistant infections. Potential therapeutic strategies could include IL-1R agonism or neutralizing the IL-1R antagonist (IL-1Ra) to promote survival during S. aureus bacteremia. However, further studies are warranted to understand the protective mechanisms of γδ T cell-intrinsic IL-1R signaling against S. aureus bacteremia.
Data availability statement
The raw data supporting the conclusions of this article will be made available by the authors, without undue reservation.
Ethics statement
The animal study was reviewed and approved by the Johns Hopkins Animal Care and Use Committee (Protocol #: MO21M378).
Author contributions
YW and NA conceived and designed the study. YW conducted the experiments. YW, MA, DD, and CY collected the data. YW analyzed the data. YW and NA wrote the manuscript. All authors reviewed the final version of the manuscript.
Funding
This study was funded in part by grants R01AR073665 (NA), R01AI111205 (NA), and R01AI146177 (NA) and from the United States National Institute of Arthritis and Musculoskeletal and Skin Diseases (NIAMS) and National Institute of Allergy and Infectious Diseases (NIAID).
Acknowledgments
The IL-1α–/–, IL-1β–/–, and IL-17A/F–/– mice were kindly provided by Dr. Yoichiro Iwakura (University of Tokyo). The VE-Cad-IL-1R–/– mice were a gift from Dr. Michael O’Connell (NIH/NIAID). The S. aureus USA300 strain, SF8300, was a kind gift from Dr. Binh Diep (UCSF).
Conflict of interest
NA has received previous grant support from Pfizer and Boehringer Ingelheim and has been a paid consultant for Janssen Pharmaceuticals.
The remaining authors declare that the research was conducted in the absence of any commercial or financial relationships that could be constructed as a potential conflict of interest.
Publisher’s note
All claims expressed in this article are solely those of the authors and do not necessarily represent those of their affiliated organizations, or those of the publisher, the editors and the reviewers. Any product that may be evaluated in this article, or claim that may be made by its manufacturer, is not guaranteed or endorsed by the publisher.
Supplementary material
The Supplementary Material for this article can be found online at: https://www.frontiersin.org/articles/10.3389/fimmu.2023.1171934/full#supplementary-material
Supplementary Figure 1 | Gating strategy. (A) The gating strategy of myeloid cells in spleen (B) the gating strategy of T cells in peripheral blood.
References
1. Weiner-Lastinger LM, Abner S, Edwards JR, Kallen AJ, Karlsson M, Magill SS, et al. Antimicrobial-resistant pathogens associated with adult healthcare-associated infections: summary of data reported to the national healthcare safety network, 2015-2017. Infect Control Hosp Epidemiol (2020) 41:1–18. doi: 10.1017/ice.2019.296
2. Lam JC, Stokes W. The golden grapes of wrath - staphylococcus aureus bacteremia: a clinical review. Am J Med (2023) 136:19–26. doi: 10.1016/j.amjmed.2022.09.017
3. Proctor RA. Immunity to staphylococcus aureus: implications for vaccine development. Immun to. Microbiol Spectr (2019) 7. doi: 10.1128/microbiolspec.GPP3-0069-2019
4. Fowler VG, Allen KB, Moreira ED, Moustafa M, Isgro F, Boucher HW, et al. Effect of an investigational vaccine for preventing staphylococcus aureus infections after cardiothoracic surgery: a randomized trial. JAMA (2013) 309:1368–78. doi: 10.1001/jama.2013.3010
5. Wang Y, Ashbaugh AG, Dikeman DA, Zhang J, Ackerman NE, Kim SE, et al. IL-1β and TNF are essential in controlling an experimental orthopaedic implant associated infection. J Orthop Res (2020) 38:1800–9. doi: 10.1002/jor.24608
6. Cho JS, Pietras EM, Garcia NC, Ramos RI, Farzam DM, Monroe HR, et al. IL-17 is essential for host defense against cutaneous staphylococcus aureus infection in mice. J Clin Invest (2010) 120:1762–73. doi: 10.1172/JCI40891
7. Marchitto MC, Dillen CA, Liu H, Miller RJ, Archer NK, Ortines RV, et al. Clonal Vgamma6+Vdelta4+ T cells promote IL-17-mediated immunity against staphylococcus aureus skin infection. Proc Natl Acad Sci U.S.A. (2019) 116:10917–26. doi: 10.1073/pnas.1818256116
8. Miller LS, O’Connell RM, Gutierrez MA, Pietras EM, Shahangian A, Gross CE, et al. MyD88 mediates neutrophil recruitment initiated by IL-1R but not TLR2 activation in immunity against staphylococcus aureus. Immunity (2006) 24:79–91. doi: 10.1016/j.immuni.2005.11.011
9. Miller LS, Fowler VG, Shukla SK, Rose WE, Proctor RA. Development of a vaccine against staphylococcus aureus invasive infections: evidence based on human immunity, genetics and bacterial evasion mechanisms. FEMS Microbiol Rev (2020) 44:123–53. doi: 10.1093/femsre/fuz030
10. McNeely TB, Shah NA, Fridman A, Joshi A, Hartzel JS, Keshari RS, et al. Mortality among recipients of the Merck V710 staphylococcus aureus vaccine after postoperative s. aureus infections: an analysis of possible contributing host factors. Hum Vaccin Immunother (2014) 10:3513–6. doi: 10.4161/hv.34407
11. Rose WE, Eickhoff JC, Shukla SK, Pantrangi M, Rooijakkers S, Cosgrove SE, et al. Elevated serum interleukin-10 at time of hospital admission is predictive of mortality in patients with staphylococcus aureus bacteremia. J Infect Dis (2012) 206:1604–11. doi: 10.1093/infdis/jis552
12. Minejima E, Bensman J, She RC, Mack WJ, Tuan Tran M, Ny P, et al. A dysregulated balance of proinflammatory and anti-inflammatory host cytokine response early during therapy predicts persistence and mortality in staphylococcus aureus bacteremia. Crit Care Med (2016) 44:671–9. doi: 10.1097/CCM.0000000000001465
13. Malhotra N, Yoon J, Leyva-Castillo JM, Galand C, Archer N, Miller LS, et al. IL-22 derived from γδ T cells restricts staphylococcus aureus infection of mechanically injured skin. J Allergy Clin Immunol (2016) 138:1098–107.e3. doi: 10.1016/j.jaci.2016.07.001
14. Randad PR, Dillen CA, Ortines RV, Mohr D, Aziz M, Price LB, et al. Comparison of livestock-associated and community-associated staphylococcus aureus pathogenicity in a mouse model of skin and soft tissue infection. Sci Rep (2019) 9:6774. doi: 10.1038/s41598-019-42919-y
15. Miller RJ, Crosby HA, Schilcher K, Wang Y, Ortines RV, Mazhar M, et al. Development of a staphylococcus aureus reporter strain with click beetle red luciferase for enhanced in vivo imaging of experimental bacteremia and mixed infections. Sci Rep (2019) 9:16663. doi: 10.1038/s41598-019-52982-0
16. Gordon O, Dikeman DA, Ortines RV, Wang Y, Youn C, Mumtaz M, et al. The novel oxazolidinone TBI-223 is effective in three preclinical mouse models of methicillin-resistant staphylococcus aureus infection. Microbiol Spectr (2022) 10:e0245121. doi: 10.1128/spectrum.02451-21
17. Ravipati A, Nolan S, Alphonse M, Dikeman D, Youn C, Wang Y, et al. IL-6R/STAT3-signaling in keratinocytes rather than T cells induces psoriasis-like dermatitis in mice. J Invest Dermatol (2021) 142:1126–35. doi: 10.1016/j.jid.2021.09.012
18. Zhang B, Wu J, Jiao Y, Bock C, Dai M, Chen B, et al. Differential requirements of TCR signaling in homeostatic maintenance and function of dendritic epidermal T cells. J Immunol (2015) 195:4282–91. doi: 10.4049/jimmunol.1501220
19. Wang Y, Dikeman D, Zhang J, Ackerman N, Kim S, Alphonse MP, et al. CCR2 contributes to host defense against staphylococcus aureus orthopedic implant-associated infections in mice. J Orthop Res (2021) 19:409–19. doi: 10.1002/jor.25027
20. Mantovani A, Dinarello CA, Molgora M, Garlanda C. Interleukin-1 and related cytokines in the regulation of inflammation and immunity. Immunity (2019) 50:778–95. doi: 10.1016/j.immuni.2019.03.012
21. Dmitrieva-Posocco O, Dzutsev A, Posocco DF, Hou V, Yuan W, Thovarai V, et al. Cell-Type-Specific responses to interleukin-1 control microbial invasion and tumor-elicited inflammation in colorectal cancer. Immunity (2019) 50:166–80.e7. doi: 10.1016/j.immuni.2018.11.015
22. Kwiecinski JM, Crosby HA, Valotteau C, Hippensteel JA, Nayak MK, Chauhan AK, et al. Staphylococcus aureus adhesion in endovascular infections is controlled by the ArlRS-MgrA signaling cascade. PloS Pathog (2019) 15:e1007800. doi: 10.1371/journal.ppat.1007800
23. Dillen CA, Pinsker BL, Marusina AI, Merleev AA, Farber ON, Liu H, et al. Clonally expanded γδ T cells protect against staphylococcus aureus skin reinfection. J Clin Invest (2018) 128:1026–42. doi: 10.1172/JCI96481
24. Ishigame H, Kakuta S, Nagai T, Kadoki M, Nambu A, Komiyama Y, et al. Differential roles of interleukin-17A and -17F in host defense against mucoepithelial bacterial infection and allergic responses. Immunity (2009) 30:108–19. doi: 10.1016/j.immuni.2008.11.009
25. Brown AF, Murphy AG, Lalor SJ, Leech JM, O’Keeffe KM, Mac Aogáin M, et al. Memory Th1 cells are protective in invasive staphylococcus aureus infection. PloS Pathog (2015) 11:e1005226. doi: 10.1371/journal.ppat.1005226
26. Hultgren OH, Svensson L, Tarkowski A. Critical role of signaling through IL-1 receptor for development of arthritis and sepsis during staphylococcus aureus infection. J Immunol (2002) 168:5207–12. doi: 10.4049/jimmunol.168.10.5207
27. Verdrengh M, Thomas JA, Hultgren OH. IL-1 receptor-associated kinase 1 mediates protection against staphylococcus aureus infection. Microbes Infect (2004) 6:1268–72. doi: 10.1016/j.micinf.2004.08.009
28. Robinson KM, Choi SM, McHugh KJ, Mandalapu S, Enelow RI, Kolls JK, et al. Influenza a exacerbates staphylococcus aureus pneumonia by attenuating IL-1β production in mice. J Immunol (2013) 191:5153–9. doi: 10.4049/jimmunol.1301237
29. Rider P, Voronov E, Dinarello CA, Apte RN, Cohen I. Alarmins: feel the stress. J Immunol (2017) 198:1395–402. doi: 10.4049/jimmunol.1601342
30. Dinarello CA. Overview of the IL-1 family in innate inflammation and acquired immunity. Immunol Rev (2018) 281:8–27. doi: 10.1111/imr.12621
31. Gabay C, Lamacchia C, Palmer G. IL-1 pathways in inflammation and human diseases. Nat Rev Rheumatol (2010) 6:232–41. doi: 10.1038/nrrheum.2010.4
32. Cohen I, Idan C, Rider P, Peleg R, Vornov E, Elena V, et al. IL-1α is a DNA damage sensor linking genotoxic stress signaling to sterile inflammation and innate immunity. Sci Rep (2015) 5:14756. doi: 10.1038/srep14756
33. Archer NK, Adappa ND, Palmer JN, Cohen NA, Harro JM, Lee SK, et al. Interleukin-17A (IL-17A) and IL-17F are critical for antimicrobial peptide production and clearance of staphylococcus aureus nasal colonization. Infect Immun (2016) 84:3575–83. doi: 10.1128/IAI.00596-16
34. Kudva A, Scheller EV, Robinson KM, Crowe CR, Choi SM, Slight SR, et al. Influenza a inhibits Th17-mediated host defense against bacterial pneumonia in mice. J Immunol (2011) 186:1666–74. doi: 10.4049/jimmunol.1002194
35. Stevens DL, Bryant AE, Hackett SP, Chang A, Peer G, Kosanke S, et al. Group a streptococcal bacteremia: the role of tumor necrosis factor in shock and organ failure. J Infect Dis (1996) 173:619–26. doi: 10.1093/infdis/173.3.619
36. Wajant H, Pfizenmaier K, Scheurich P. Tumor necrosis factor signaling. Cell Death Differ (2003) 10:45–65. doi: 10.1038/sj.cdd.4401189
37. Croft M, Benedict CA, Ware CF. Clinical targeting of the TNF and TNFR superfamilies. Nat Rev Drug Discovery (2013) 12:147–68. doi: 10.1038/nrd3930
38. Chan LC, Chaili S, Filler SG, Barr K, Wang H, Kupferwasser D, et al. Nonredundant roles of interleukin-17A (IL-17A) and IL-22 in murine host defense against cutaneous and hematogenous infection due to methicillin-resistant staphylococcus aureus. Infect Immun (2015) 83:4427–37. doi: 10.1128/IAI.01061-15
39. Cho JS, Guo Y, Ramos RI, Hebroni F, Plaisier SB, Xuan C, et al. Neutrophil-derived IL-1β is sufficient for abscess formation in immunity against staphylococcus aureus in mice. PloS Pathog (2012) 8:e1003047. doi: 10.1371/journal.ppat.1003047
40. Archer NK, Harro JM, Shirtliff ME. Clearance of staphylococcus aureus nasal carriage is T cell dependent and mediated through interleukin-17A expression and neutrophil influx. Infect Immun (2013) 81:2070–5. doi: 10.1128/IAI.00084-13
41. Mulcahy ME, Leech JM, Renauld JC, Mills KH, McLoughlin RM. Interleukin-22 regulates antimicrobial peptide expression and keratinocyte differentiation to control staphylococcus aureus colonization of the nasal mucosa. Mucosal Immunol (2016) 9:1429–41. doi: 10.1038/mi.2016.24
42. Sutton CE, Lalor SJ, Sweeney CM, Brereton CF, Lavelle EC, Mills KH. Interleukin-1 and IL-23 induce innate IL-17 production from gammadelta T cells, amplifying Th17 responses and autoimmunity. Immunity (2009) 31:331–41. doi: 10.1016/j.immuni.2009.08.001
43. Duan J, Chung H, Troy E, Kasper DL. Microbial colonization drives expansion of IL-1 receptor 1-expressing and IL-17-producing gamma/delta T cells. Cell Host Microbe (2010) 7:140–50. doi: 10.1016/j.chom.2010.01.005
44. Kaplanov I, Carmi Y, Kornetsky R, Shemesh A, Shurin GV, Shurin MR, et al. Blocking IL-1β reverses the immunosuppression in mouse breast cancer and synergizes with anti-PD-1 for tumor abrogation. Proc Natl Acad Sci U.S.A. (2019) 116:1361–9. doi: 10.1073/pnas.1812266115
45. Da Ros F, Carnevale R, Cifelli G, Bizzotto D, Casaburo M, Perrotta M, et al. Targeting interleukin-1β protects from aortic aneurysms induced by disrupted transforming growth factor β signaling. Immunity (2017) 47:959–73.e9. doi: 10.1016/j.immuni.2017.10.016
46. Ismail AS, Severson KM, Vaishnava S, Behrendt CL, Yu X, Benjamin JL, et al. Gammadelta intraepithelial lymphocytes are essential mediators of host-microbial homeostasis at the intestinal mucosal surface. Proc Natl Acad Sci U.S.A. (2011) 108:8743–8. doi: 10.1073/pnas.1019574108
47. Ribot JC, Lopes N, Silva-Santos B. γδ T cells in tissue physiology and surveillance. Nat Rev Immunol (2021) 21:221–32. doi: 10.1038/s41577-020-00452-4
48. Dong X, Limjunyawong N, Sypek EI, Wang G, Ortines RV, Youn C, et al. Keratinocyte-derived defensins activate neutrophil-specific receptors Mrgpra2a/b to prevent skin dysbiosis and bacterial infection. Immunity (2022) 55:1645–62.e7. doi: 10.1016/j.immuni.2022.06.021
49. Ali A, Na M, Svensson MN, Magnusson M, Welin A, Schwarze JC, et al. IL-1 receptor antagonist treatment aggravates staphylococcal septic arthritis and sepsis in mice. PloS One (2015) 10:e0131645. doi: 10.1371/journal.pone.0131645
50. Corriden R, Hollands A, Olson J, Derieux J, Lopez J, Chang JT, et al. Tamoxifen augments the innate immune function of neutrophils through modulation of intracellular ceramide. Nat Commun (2015) 6:8369. doi: 10.1038/ncomms9369
Keywords: Staphylococcus aureus, IL-1R, bacteremia, T cells, host defense, cytokines
Citation: Wang Y, Ahmadi MZ, Dikeman DA, Youn C and Archer NK (2023) γδ T cell-intrinsic IL-1R promotes survival during Staphylococcus aureus bacteremia. Front. Immunol. 14:1171934. doi: 10.3389/fimmu.2023.1171934
Received: 22 February 2023; Accepted: 16 June 2023;
Published: 07 July 2023.
Edited by:
Pietro Ghezzi, University of Urbino Carlo Bo, ItalyReviewed by:
Xin Liu, Third Military Medical University, ChinaToby K. Eisenstein, Temple University, United States
Copyright © 2023 Wang, Ahmadi, Dikeman, Youn and Archer. This is an open-access article distributed under the terms of the Creative Commons Attribution License (CC BY). The use, distribution or reproduction in other forums is permitted, provided the original author(s) and the copyright owner(s) are credited and that the original publication in this journal is cited, in accordance with accepted academic practice. No use, distribution or reproduction is permitted which does not comply with these terms.
*Correspondence: Nathan K. Archer, bmFyY2hlcjJAamhtaS5lZHU=