- 1Penn State Cancer Institute, Penn State University College of Medicine, Hershey, PA, United States
- 2Fujian Institute of Hematology, Fujian Provincial Key Laboratory of Hematology, Fujian Medical University Union Hospital, Fujian Medical Center of Hematology, Fuzhou, China
- 3Department of Microbiology and Immunology, Penn State University College of Medicine, Hershey, PA, United States
Acute myeloid leukemia (AML) is a devastating blood cancer with poor prognosis. Novel effective treatment is an urgent unmet need. Immunotherapy targeting T cell exhaustion by blocking inhibitory pathways, such as PD-1, is promising in cancer treatment. However, results from clinical studies applying PD-1 blockade to AML patients are largely disappointing. AML is highly heterogeneous. Identification of additional immune regulatory pathways and defining predictive biomarkers for treatment response are crucial to optimize the strategy. CD26 is a marker of T cell activation and involved in multiple immune processes. Here, we performed comprehensive phenotypic and functional analyses on the blood samples collected from AML patients and discovered that CD26lowPD-1+ CD8 T cells were associated with AML progression. Specifically, the percentage of this cell fraction was significantly higher in patients with newly diagnosed AML compared to that in patients achieved completed remission or healthy controls. Our subsequent studies on CD26lowPD-1+ CD8 T cells from AML patients at initial diagnosis demonstrated that this cell population highly expressed inhibitory receptors and displayed impaired cytokine production, indicating an exhaustion status. Importantly, CD26lowPD-1+ CD8 T cells carried features of terminal exhaustion, manifested by higher frequency of TEMRA differentiation, increased expression of transcription factors that are observed in terminally exhausted T cells, and high level of intracellular expression of granzyme B and perforin. Our findings suggest a prognostic and predictive value of CD26 in AML, providing pivotal information to optimize the immunotherapy for this devastating cancer.
Introduction
Acute myeloid leukemia (AML) is a devastating blood cancer with poor prognosis. Although treatment of AML has been significantly advanced recently with several novel targeting agents approved by FDA, five-year overall survival remains low at only 30.5% (1). Novel effective treatment is clearly an unmet need.
Multiple studies including ours have demonstrated the involvement of T cell exhaustion in AML pathogenesis (2–9). A recent study showed that T cell exhaustion may be a predominant process in AML at diagnosis and AML shaped CD8 T cell response in vitro (10). Up-regulation of PD-1 and other immune inhibitory pathways, the hallmark for T cell exhaustion, was found to be associated with AML progression (5). Importantly, PD-1 blockade enhanced T cell activity and reduced leukemia burden in mouse models of AML (6, 9). These observations suggest an important role of T cell exhaustion in AML. However, clinical studies applying PD-1 blockade to AML patients showed limited benefit (11–13). AML is highly heterogeneous. Identification of additional immune regulatory pathways and defining predictive biomarkers for treatment response are crucial to optimize treatment targeting T cell exhaustion and develop effective immunotherapy for AML.
CD26, also known as dipeptidyl peptidase 4 (DPP4), is a homodimeric type II transmembrane glycoprotein expressed on many cell types, including epithelial cells and immune components such as T cells, B cells, NK, and macrophages (14–18). CD26 is multifunctional and is involved in glucose homeostasis (19), stem cell homing (20), regulation of inflammatory diseases and multiple immune processes (21). CD26 is a marker for T cell activation. It acts as a costimulatory molecule enhancing interactions between antigen-presenting cells and T cells, subsequently initiating the signal transduction process and promoting T cell activation. Up-regulation of CD26 has been observed on both CD4 and CD8 T cells that are highly function in antiviral and anti-tumor response (22). However, the impact of T cell expression of CD26 on AML has not been studied. To fill this gap, we examined T cells of peripheral blood collected from a cohort of newly diagnosed AML patients (n=28). Subpopulations of T cells expressing different level of CD26 were further dissected for their phenotypic and functional status, as well as correlations with clinical outcome.
Materials and methods
Patient
Peripheral blood and bone marrow samples were collected from AML patients diagnosed per WHO criteria. All the patients were diagnosed at the Penn State Hershey Cancer Institute of Penn State University College of Medicine (Hershey, PA, USA). The study was approved by the Institutional Review Board of Penn State College of Medicine. Fully informed consent was obtained from all patients.
Isolation of PBMCs
Peripheral blood and bone marrow samples were collected from patients with newly diagnosed AML (n=28), AML patients in complete remission (n=15), and healthy donors (n=18). Peripheral blood mononuclear cells (PBMCs) and bone marrow mononuclear cells were isolated by density gradient centrifugation using Ficoll-Paque (Amersham Pharmacia Biotech, Stockholm, Sweden). Cells were preserved in fetal bovine serum containing 10% dimethyl sulfoxide (Gibco, Grand Island, NY, USA) and stored in liquid nitrogen.
Immunofluorescence staining and flow cytometry analysis
For surface staining, frozen PBMCs were thawed at 37°C and washed 2 times with phosphate-buffered saline containing 1% fetal bovine serum. Cells were incubated with Human BD Fc Block™ (10 minutes at room temperature) followed by staining with directly conjugated mAbs for 30 minutes at 4°C. Cells were then washed and resuspended in staining buffer before flow cytometry analysis. The monoclonal antibodies used were anti-human CD3-BV605, CD4-BV711, CD8-APC-H7, CD45RA-AF700, CD26-PE-CF594 or CD26-BV421, Ki67-AF488, Granzyme B-AF700, T-bet-PE, TCF-7/TCF-1-AF647, CD95-BV421, Annexin V-PE, hCD45-BV605 (BD Biosciences, San Diego, CA, USA), CCR7-BV421, PD-1-BV785, CD226-FITC, TIM-3-PE-Cy7, Perforin-APC (Biologend, San Diego, CA, USA), TIGIT-APC, Eomes-PE-eF610, TOX-PE, AITR/GITR-PE (invitrogen, Carlsbad, CA, USA) antibodies and corresponding isotype controls. Data were acquired using an LSR Fortessa flow cytometer (BD Biosciences) and analyzed with FlowJo software (Tree Star, Ashland, OR, USA).
In vitro stimulation and intracellular cytokine staining
PBMCs were cultured in RPMI-1640 medium (Gibco) containing 10% fetal bovine serum and stimulated with anti-CD3/CD28 (2 and 2.5 μg/mL) at the presence of Plus Golgiplug (BD Pharmingen, San Diego, CA, USA) for 5 hours. Cell viability was assessed using the Fixable viability dye eFluorTM 506 (invitrogen, Carlsbad, CA, USA). Cells were then surface stained with CD4-FITC, CD8-APC-H7, PD-1-BV785, and CD26-PE-CF594. After fixation and permeabilization, intracellular staining was performed with IL-2-PE-Cy7, TNF-α-BV421, IFN-γ-APC (BD Biosciences) antibodies.
Statistical analysis
All summary statistics (average values, s.d., s.e.m., significant differences between groups) were calculated using GraphPad Prism 9 (GraphPad Software Inc., San Diego, CA) or SPSS Statistics 26 as appropriate. For data distributed normally, the comparison of variables was performed using unpaired or paired Student t test. For data not distributed normally, the comparison of variables was performed with a Mann–Whitney U test or a Wilcoxon signedrank test for unpaired and paired data, respectively. Comparisons of categorical patient characteristics were analyzed using Fisher exact test. The ROC curve was used to predict the reasonable grouping cutoff of low CD26lowPD-1+ and high CD26lowPD-1+ in newly diagnosed AML patients and healthy controls. The overall survival was analyzed by the log-rank (Mantel–Cox) test. For all analyses, a P value of < 0.05 was considered statistically significant.
Results
The proportion of CD26lowPD-1+ CD8 T cells is significantly higher in blood of patients with untreated AML
Given its costimulatory function in T cell activation, we initially hypothesized that down-regulation of CD26 on T cells correlates with T cell hypofunction and subsequently AML progression. We performed flow cytometry analyses on PBMCs collected from AML patients at newly diagnosis (n=28) vs. that of healthy controls (n=18). The clinical characteristics of the AML patients are summarized in Table 1. Consistent with the heterogeneity of AML, there was wide variability in white blood cell (WBC) counts and blast percentages in the peripheral blood. The majority of patients carried intermediate or adverse cytogenetic features. Surprisingly, no significant differences in T cell expression of CD26 were observed (Supplemental Figure 1). However, when PD-1 was added to the analyses, in which PD-1+ T cells were divided into 3 subsets based on the expression of CD26 (Figure 1A), we made striking observation that the frequency of PD-1+ CD8 T cells expressing low level of CD26 (CD26lowPD-1+) were significantly higher in newly diagnosed AML patients compared to that in healthy controls (31.45 ± 2.129% vs. 21.83 ± 2.541%, P=0.0062; Figures 1B, C). We further examined PBMCs from AML patients who have achieved complete remission (CR) after chemotherapy (n=15), and found that similar to healthy controls, CD26lowPD-1+ CD8 T cells in these patients are significantly lower than that in newly diagnosed AML. In contrast, the frequency of CD8 T cells expressing intermediate level of CD26 (CD26intPD-1+) were lower in newly diagnosed AML compared to AML in CR or healthy controls (Figures 1B, C). Same analyses were performed on CD4 T cells and no significant differences were observed (Supplemental Figures 1, 2). These data suggest that CD26lowPD-1+ CD8 T cells correlate with AML progression.
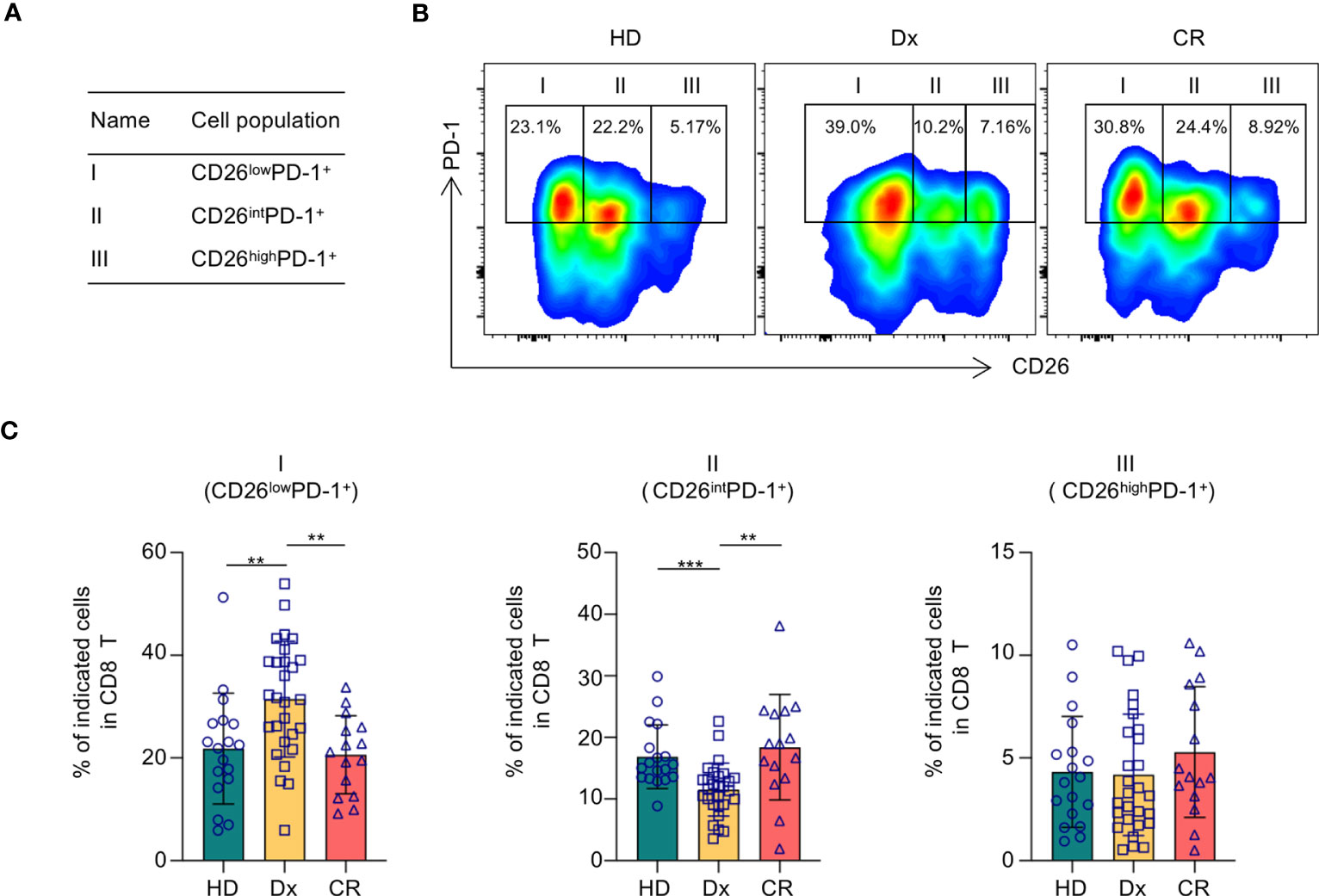
Figure 1 CD26lowPD-1+ CD8 T cells are significantly increased in AML patients at newly diagnosis. PBMCs collected from healthy donors, AML patients at newly diagnosis and in complete remission were assessed for CD26 and PD-1 expression on CD8 T cells by flow cytometry. (A) Based on the levels of CD26 and PD-1 expression, CD8 T cells are divided into three fractions. Shown is the schema of each fraction. (B) Representative flow data from healthy donors (HD), AML patient at newly diagnosis (Dx) and in complete remission (CR) displaying the percentage of CD26lowPD-1+(fraction I), CD26intPD-1+ (fraction II), CD26highPD-1+ (fraction III) among CD8 T cells. (C) The frequencies of each fraction among CD8 cells in HD(n=18), AML patients at initial diagnosis (Dx, n=28) and AML patients in complete remission (CR, n=15). Each spot represents data of an individual patient or healthy donor. P values were obtained by unpaired Student t-test or Mann-Whitney test. **P<0.01, ***P<0.001.
Terminally differentiated effector cells are significantly increased in CD26lowPD-1+ CD8 T cells
We then focused our study on characteristic analyses of CD26lowPD-1+ CD8 T cells. PBMCs from patients with newly diagnosed AML were examined. We first assessed the differentiation status of this cell population. Based on the expression of CD45RA and CCR7, T cells can be divided into four differentiation subsets (Figure 2A): naïve T cells (TN, CCR7+CD45RA+), central memory T cells (TCM, CCR7+CD45RA−), effector Memory T cells (TEM, CCR7−CD45RA−) and terminally differentiated effector cells (TEMRA, CCR7−CD45RA+). We performed multichannel flow cytometry analyses to dissect the distribution of all four differentiated subsets in CD26lowPD-1+ CD8 T cells as well as the other two PD-1+ CD8 T cell populations based on CD26 expression (CD26intPD-1+ and CD26highPD-1+). Consistent with the previous report that most CD26int T cells are naïve, we found a high frequency of naïve cells in CD26intPD-1+ CD8 T cells from our AML patients. In contrast, both CD26lowPD-1+ and CD26highPD-1+ CD8 T cells are antigen experienced. Importantly, we observed a significantly higher frequency of TEMRA cells in CD26lowPD-1+ than CD26highPD-1+ CD8 T cells (48.74% vs. 22.28%, P<0.0001; Figures 2B, C). TEMRA is considered to be a terminal effector cells with limited function. This data suggests an association of CD26lowPD-1+ CD8 T cells with T cell dysfunction and AML pathogenesis.
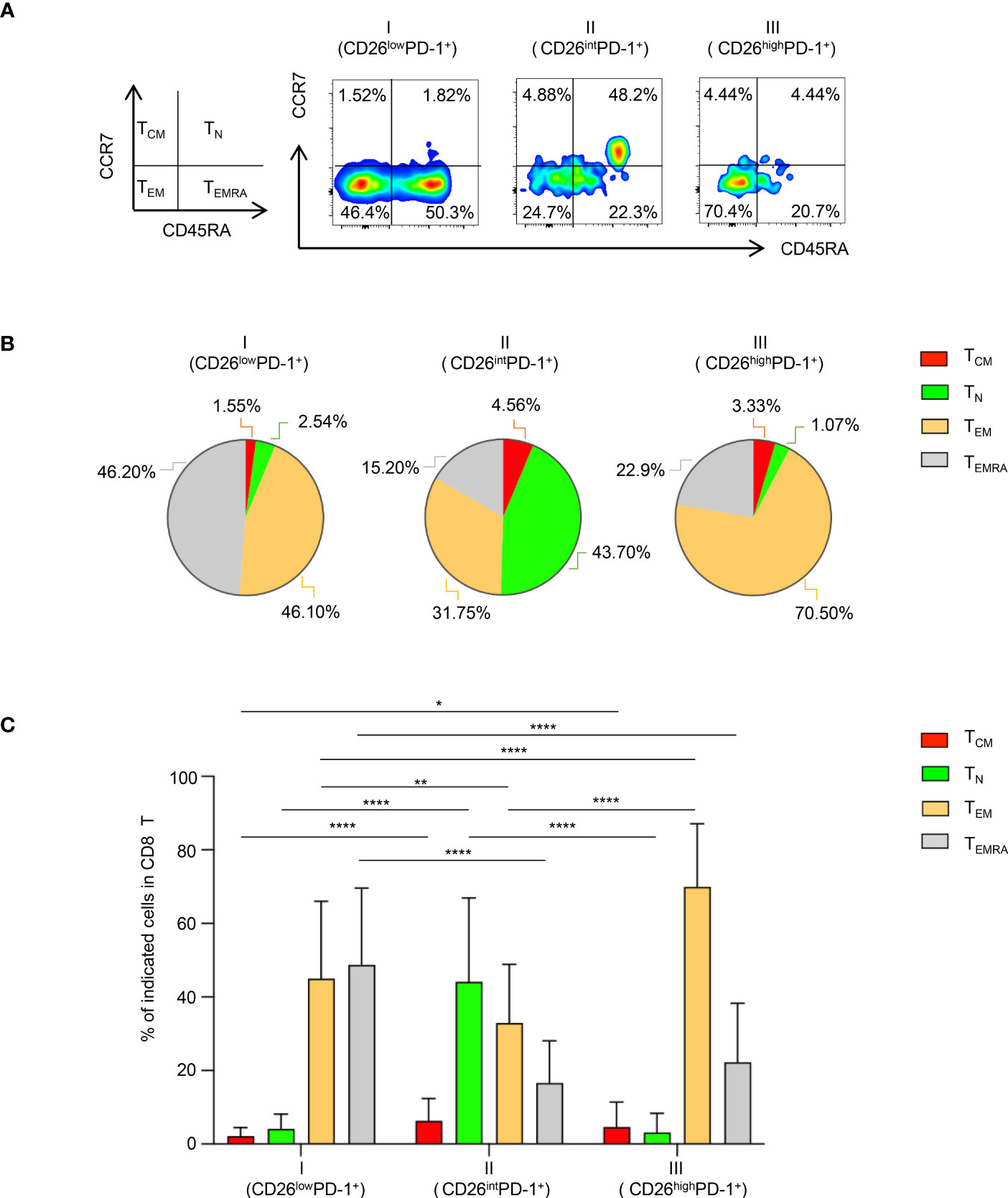
Figure 2 Terminally differentiated effector cells are significantly increased in CD26lowPD-1+ CD8 T cells. Flow cytometry analysis of surface expression of PD-1, CD26, CD45RA, CCR7 was performed on PBMCs collected from AML patients at initial diagnosis. (A) The gating strategy of Naïve (TN), central memory (TCM), effector memory (TEM) and terminal differentiated cells (TEMRA) in CD8 T cells was shown on the left. The representative flow images on the right show the distribution of the above subsets in CD26lowPD-1+ (fraction I), CD26intPD-1+ (fraction II) and CD26highPD-1+ (fraction III) in CD8 T (B) The pie chart depicts the distribution of TN, TCM, TEM and TEMRA in CD26lowPD-1+ (fraction I), CD26intPD-1+ (fraction II) and CD26highPD-1+ (fraction III) CD8 T cells. (C) Summary data for the distribution of naïve vs. memory in the three fractions of CD8 T cells. P values were obtained by paired Student t-test or Wilcoxon signed rank test. *P<0.05, **P<0.01, ****P<0.0001.
Expression of inhibitory receptors is increased on CD26lowPD-1+ CD8 T cells
We next examined the impact of CD26 expression on the inhibitory and stimulatory pathways in PD-1+ CD8 T cells. To rule out potential confounding effect of Naïve T cells, we excluded Naïve-dominant CD26intPD-1+ CD8 T cells and focused our subsequent analyses on antigen experienced cells including CD26lowPD-1+ and CD26highPD-1+ CD8 T cells. When surface expression of a number of inhibitory receptors on these two cell populations was compared, we observed significantly higher expression of TIGIT and TIM-3 on CD26lowPD-1+ CD8 T cells, compared with that of CD26highPD-1+ CD8 T cells (TIGIT: 46.09% vs. 5.71%, P<0.0001; TIM-3: 2.28% vs. 0.34%, P=0.0001; Figures 3A, B). In contrast, expression of the stimulatory receptor CD226 (counterpart of TIGIT) was significantly lower on CD26lowPD-1+ CD8 T cells (34.4% vs. 65.3%, P<0.0001, Figure 3C). Up-regulation of inhibitory receptors is a hallmark of T cell exhaustion. Our finding indicates that CD26lowPD-1+ CD8 T cells are likely in a more advanced exhaustive status, compared with CD26highPD-1+ CD8 T cells.
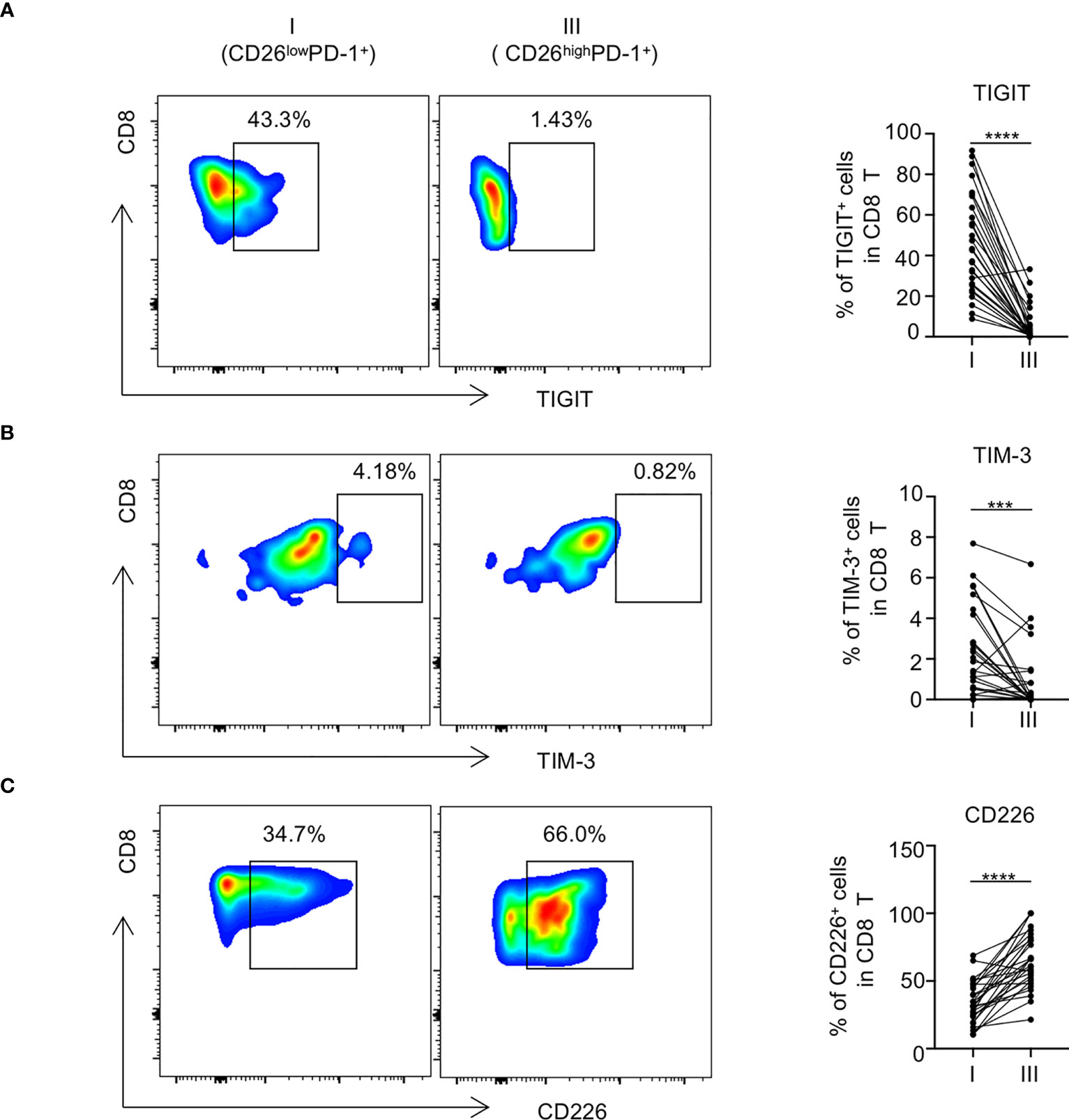
Figure 3 The expression of inhibitory receptors in CD26lowPD-1+ CD8 T cells in newly diagnosed AML patients was increased. PBMCs from AML patients at initial diagnosis were examined by flow cytometry(n=28). The expression of TIGIT [shown in (A)], TIM-3 [shown in (B)] and CD226 [shown in (C)] on CD8 T cell subpopulations [CD26lowPD-1+(fraction I) and CD26highPD-1+ (fraction III)] was assessed. (A–C) Shown are the representative flow data (left) and summary plots (right). P values were obtained by paired Student t-test or Wilcoxon signed rank test. ***P<0.001, ****P<0.0001.
CD26lowPD-1+ CD8 T cells express higher level of exhaustion related transcription factors
We further assessed the expression pattern of transcription factors in CD26lowPD-1+ CD8 T cells. Studies in models of chronic viral infection have demonstrated that several transcription factors including Eomes, T-bet, TOX, and TCF1 are important in regulating T cell exhaustion. We compared the intracellular expression of these transcription factors in CD26lowPD-1+ CD8 T cells to that in CD26highPD-1+ CD8 T cells. As shown in Figure 4A, we observed a higher percentage of TOX expression in CD26lowPD-1+ CD8 T cells (79.77% vs. 64.55%, P<0.0001). In contrast, expression of TCF1 in CD26lowPD-1+ CD8 T cells was significantly lower (16.73% vs. 24.06%, P=0.0023, Figure 4B). When expression of Eomes and T-bet was assessed, we focused our analyses on the Eomes+T-betlow subset as our previous work showed that this subset was associated with poor clinical outcome in AML patients (4). As shown in Figure 4C, we observed a higher percentage of Eomes+T-betlow cells in CD26lowPD-1+ CD8 T cells, compared to that in CD26highPD-1+ CD8 T cells (23.64% vs. 18.18%, P=0.0233). Collectively, we found that CD26lowPD-1+ CD8 T cells from untreated AML patients express higher level of TOX and Eomes, whereas lower level of TCF1. This transcription pattern is more consistent with terminal exhaustion.
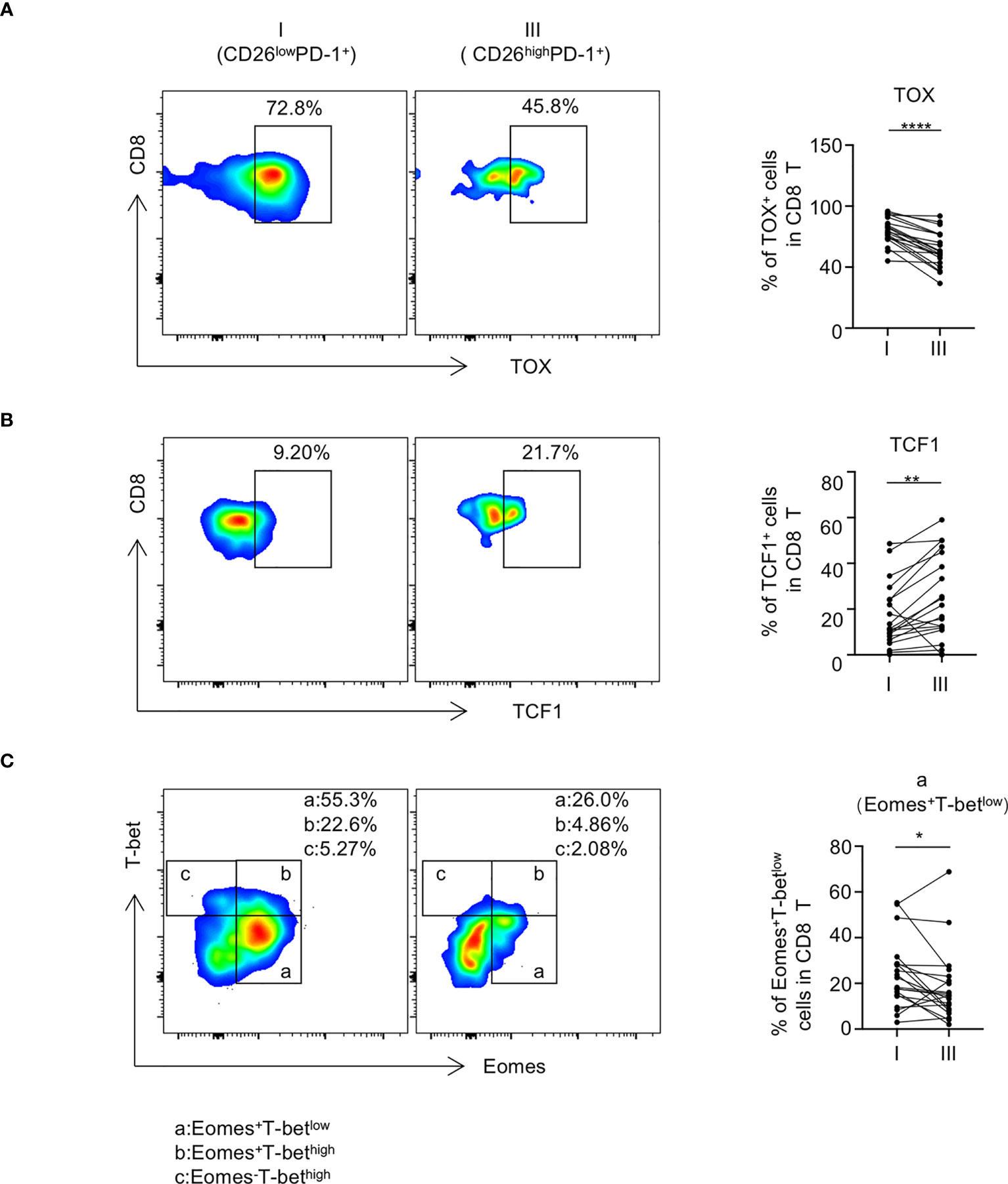
Figure 4 CD26lowPD-1+ CD8 T cells express higher level of exhaustion-related transcription factors. PBMCs collected from AML patients at initial diagnosis were assessed by flow cytometry (n=20). The expression of TOX [shown in (A)], TCF1 [shown in (B)] Eomes and T-bet [shown in (C)] on CD8 T cell subpopulations [CD26lowPD-1+ (fraction I) and CD26highPD-1+ (fraction III)] was analyzed by flow cytometry. Upon the expression of Eomes and T-bet, CD8 T cells were divided into subsets of Eomes+T-betlow(a), Eomes+T-bethigh (b) and Eomes-T-bethigh (c), (A–C) Flow cytometry representative data were shown on the left and summary plots were shown on the right. P values were obtained by paired Student t-test or Wilcoxon signed rank test. *P<0.05, **P<0.01, ****P<0.0001.
CD26lowPD-1+ CD8 T cells exhibit functional defects
To assess the functional status of CD26lowPD-1+ CD8 T cells, we performed an in vitro assay to examine intracellular cytokine productions by CD8 T cells upon anti-CD3 and anti-CD28 stimulation. PBMCs from untreated AML patients were used in this study. CD8 T cells were gated by CD26lowPD-1+ vs. CD26highPD1+ and intracellular production of IFN-γ, IL-2, and TNF-α by each cell subpopulation was assessed by flow cytometry analyses. As shown in Figures 5A–C, the CD26lowPD-1+ CD8 T cells had significantly lower production of IFN-γ, IL-2, and TNF-α compared with CD26highPD-1+ CD8 T cells (IFN-γ:13.51% vs. 21.17%, P=0.003; IL-2:2.69% vs. 18.63%, P<0.0001; TNF-α: 5.27% vs. 8.05%, P=0.0163). We also evaluated the intracellular expression of Granzyme B and perforin in each cell subpopulation as an indication of killing capacity. Interestingly, we found a significantly increased level of Granzyme B and perforin in CD26lowPD-1+ CD8 T cells compared to that in CD26highPD-1+ CD8 T cells (Figures 5D, E). The discrepancy between cytokine production and Granzyme B/perforin expression in the functional status of exhausted T cells has been observed in multiple studies (3, 24–27). It is suspected that terminally exhausted T cells may lose energy to secret Granzyme B/perforin, leading to the intracellular accumulation of these molecules (28, 29). Collectively, our data demonstrate that CD26lowPD-1+ CD8 T cells are functionally impaired demonstrated by reduced cytokine production, consistent with the status of exhaustion. In addition, they displayed higher intracellular expression of Granzyme B/perforin, suggesting a terminal exhausted status.
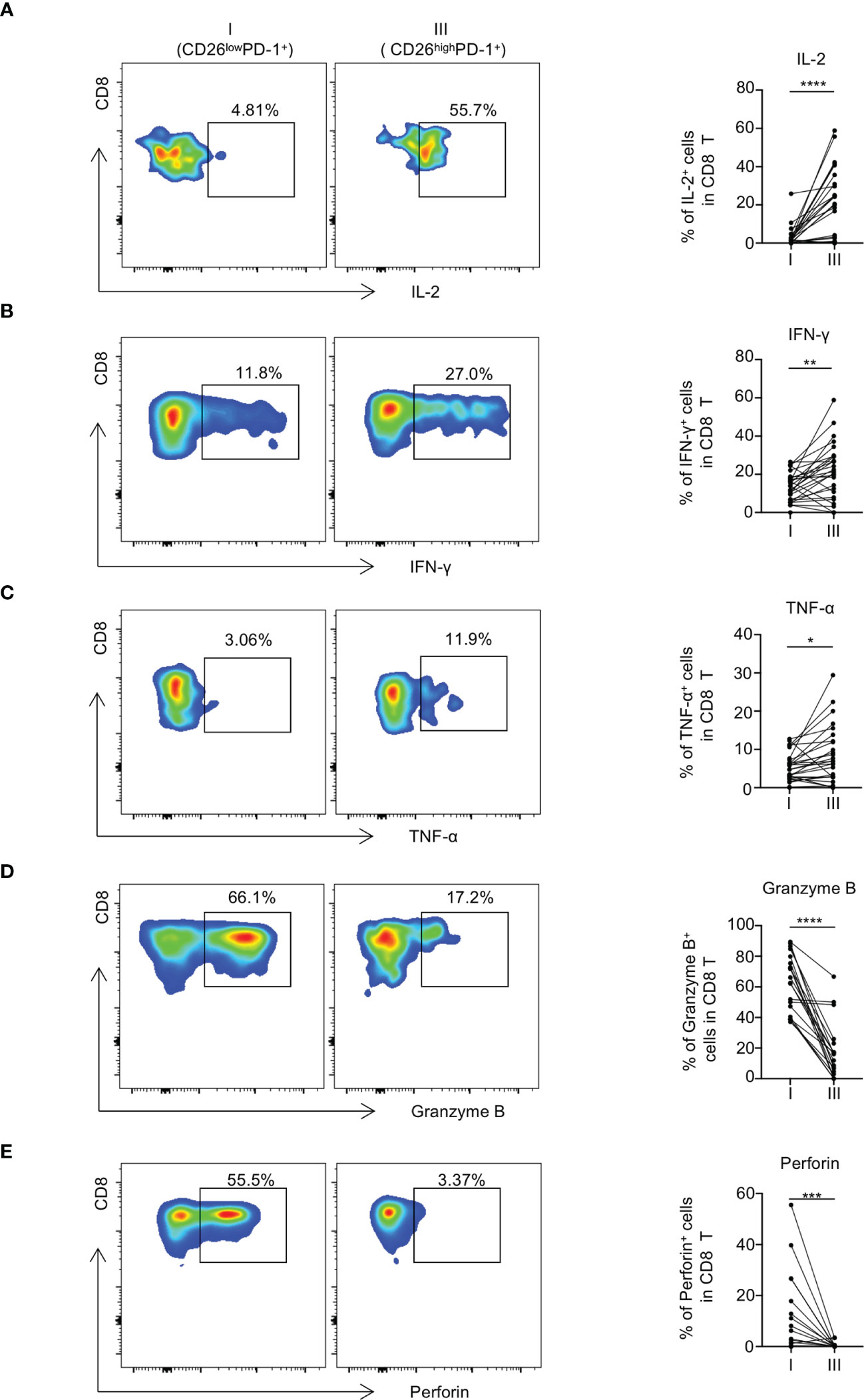
Figure 5 CD26lowPD-1+ CD8 T cells produce less cytokines and display decreased cytotoxic capacity in AML patients at initial diagnosis. (A–C) PBMCs collected from AML patients at initial diagnosis were stimulated in vitro with anti-CD3 and anti-CD28 before intracellular staining of IL-2, IFN-γ and TNF-α (n=28). Flow cytometry representative data (left) and summary plots (right) show the expressions of IL-2 (A), IFN-γ (B) and TNF-α (C) in indicated CD8 subpopulations (CD26lowPD-1+ (fraction I) and CD26highPD-1+ (fraction III)). (D, E) The expression of Granzyme B and perforin in each CD8 subpopulation. Representative data (left) and summary graphs (right) are shown. P values were obtained by paired Student t-test or Wilcoxon signed rank test. *P<0.05, **P<0.01, ***P<0.001, ****<0.0001.
Discussion
In this study, we performed comprehensive phenotypic and functional analyses on the T cells of PBMCs collected from AML patients and healthy controls. We focused our study on the impact of CD26 expression on T cells and discovered that CD26lowPD-1+ CD8 T cells were associated with AML progression. Specifically, the percentage of this cell fraction was significantly higher in patients with newly diagnosed AML (high leukemia burden) compared to that in patients who achieved CR (no leukemia) or healthy controls. Our subsequent studies on CD26lowPD-1+ CD8 T cells from newly diagnosed AML patients demonstrated that this cell population carries features of T cell exhaustion, manifested by higher frequency of TEMRA differentiation, increased expression of inhibitory receptors and exhaustion-related transcription factors, and functional defects. To our knowledge, this is the first report to uncover the important role of CD26 expression on CD8 T cells in AML.
Increased expression of PD-1 is an essential marker for T cell exhaustion. Multiple studies including ours have demonstrated an up-regulation of PD-1 on T cells from AML patients who have disease relapse (2, 5, 8, 30–33). However, T cell expression of PD-1 was not increased in AML patients at initial diagnosis (32). It may be attributed to the heterogeneity of PD-1+ T cells at this particular disease status, thus PD-1 alone is inadequate to distinguish exhausted T cells from T cells of other functional status. In fact, elegant studies of mouse models of chronic viral infection have shown a higher frequency of PD-1+ CD8 T cells at activation phase short after viral infection as well as in the exhaustion status later in the chronic phase (34–36). It is possible that PD-1+ T cells in newly diagnosed AML patients are diverse in their functional status. In our current study, we further dissected PD-1+ CD8 T cells into three subpopulations based on their expression of CD26. We made novel findings that CD26lowPD-1+ CD8 T cells were phenotypically and functionally consistent with exhaustion status. Importantly, the frequency of CD26lowPD-1+ CD8 T cells was significantly higher in newly diagnosed AML patients compared to that in healthy controls or AML patients in CR. Therefore, two-dimensional analysis testing both CD26 and PD-1 on CD8 T cells provides an optimal strategy to identify exhaustion T cells in newly diagnosed AML. With a hypothesis that higher frequency of exhausted T cells leads to poor prognosis due to compromised anti-leukemia immune response, we performed analyses on the data of newly diagnosed AML patients to determine the impact of CD26lowPD-1+ CD8 T cells on clinical outcome. Twenty-one AML patients whose overall survival (OS) were evaluable (medium follow-up time:1744 days) were divided into two groups based on their CD8 T cells expression level of CD26lowPD-1+. We observed that patients with high percentage of CD26lowPD-1+ CD8 T cells displayed a trend of lower OS compared to that of low percentage subgroup (median: 372 vs. 1369 days; P=0.231; Supplemental Figure 3). No statistical significance was achieved likely due to limited sample size. Further studies in larger cohorts of patients are warranted to make a conclusion. If validated, CD26lowPD-1+ CD8 T cells could be a prognostic biomarker for newly diagnosed AML.
TOX has been considered as an essential transcription factor governing terminally exhausted T cells, whereas TCF1 is more functional in progenitor exhausted T cells (37–41). Our finding that CD26lowPD-1+ CD8 T cells expressed higher TOX and lower TCF1 suggests that these cells are more toward terminal exhaustion. Consistently, we observed that CD26lowPD-1+ CD8 T cells contain higher frequency of Eomes+T-betlow cells, a pattern that is observed in terminal exhaustion. Furthermore, whereas being functionally impaired, evident by less cytokine production capacity, CD26lowPD-1+ CD8 T cells express more granzyme B and perforin, another feature of terminal exhausted CD8 T cells. Taking together, our data support the notion that CD26lowPD-1+ CD8 T cells in newly diagnosed AML represent a terminally exhausted T cell population. This finding has significant clinical impact. Multiple studies in preclinical models have demonstrated that reversing T cell exhaustion by PD-1 inhibition effectively reduces leukemia burden (6, 9); however, results from clinical studies applying PD-1 blockade to AML patients are largely negative (11–13). An important strategy to improve treatments targeting T cell exhaustion is to define predictive biomarkers to identify patients who are likely to respond to the treatment. It becomes clear that terminally exhausted T cells have minimal response to PD-1 blockade, a main mechanism for resistance to checkpoint inhibitors (25, 35). Our novel finding that CD26lowPD-1+ CD8 T cells are terminally exhausted is compelling: high frequency of this cell population in newly diagnosed AML may lead to poor response to PD-1-targeting agents. Therefore, optimizing clinical trial design by selecting patients with low percentage of CD26lowPD-1+ CD8 T cells has a strong potential to improve efficacy of treatment with PD-1 blockade.
In contrast to CD26lowPD-1+ CD8 T cells, we observed that CD26highPD-1+ CD8 T cells are highly functional evident by predominant differentiation stage of TEM and potent cytokine release upon in vitro stimulation with anti-CD3/CD28. This is in line with the findings of Bailey et al. that CD26high CD4 T cells showed strong anti-tumor activity when transferred into mouse models of solid tumors (22). Given the fact that CD26 has a costimulatory function in activating T cells, sitagliptin, a CD26/DPP4 inhibitor, was applied to patients undergoing allogeneic stem cell transplantation as graft vs. host disease (GVHD) prophylaxis in a phase 2 clinical trial (42). Low incidence of grade II to IV acute GVHD was observed. A major concern is a decrease of graft vs. leukemia (GVL) effect due to CD26 inhibition. Encouragingly, one-year relapse rate was comparable with historical controls, indicating a preservation of GVL. However, longer follow-up and randomized studies are needed to draw a conclusion. It has been reported that CD26 is overexpressed on tumor cells and promotes metastasis of solid tumors (43–46). Several CD26 inhibitors have been tested for their direct cytotoxic effect against tumors in preclinical models (47–49). Although promising results were observed in some studies, most data were inconclusive or negative. It is possible that systemic treatment with CD26 inhibitors significantly suppresses T cell function and compromises the anti-tumor effect. Combining approaches of regaining T cell activity while inhibiting CD26 would be helpful to circumvent this obstacle. Further investigation of the mechanisms by which CD26 mediates T cell response is essential to identify such therapeutic targets.
Of note, we also examined the differentiation status (TN, TCM, TEM and TEMRA) in subsets of CD26lowPD-1+, CD26intPD-1+ and CD26highPD-1+ CD8 T cells in healthy donor (HD) and CR groups. We observed that the differentiation status of each subset among HD and CR samples was similar to that in newly diagnosed samples (Dx) (Supplemental Figures 4A, B; Figure 2). In addition, expression patterns of TIGIT and CD226 in each subset among HD and CR samples was also similar to that in Dx samples (Supplemental Figures 4C, D). These observations indicate that each subset (CD26lowPD-1+, CD26intPD-1+ or CD26highPD-1+ CD8 T cells) carries a unique feature that is not altered in different clinical settings (HD, Dx, or CR). Instead, the change in frequencies of the subsets reflects the disease specificity, thus we observed significantly increased frequency of CD26lowPD-1+ CD8 T cells in Dx samples, compared to that in HD and CR (Figure 1).
It is unclear how CD26lowPD1+ CD8 T cells are increased in AML progression. We examined the apoptosis of CD26lowPD-1+ vs. CD26highPD-1+ CD8 T cells by evaluating the expression of CD95 and Annexin V. We observed no significant differences between the two subsets (Supplemental Figures 5A, B. In addition, when proliferation was assessed by evaluating the expression of Ki-67, no significant difference was observed either (Supplemental Figure 5C). Therefore the increased frequency of CD26lowPD-1+ CD8 T cells in AML progression is unlikely due to increased apoptosis of CD26high T cells or altered proliferation in each subset. We further assessed the frequency of CD26lowPD-1+, CD26intPD-1+ and CD26highPD-1+ CD8 T cells in the bone marrow of newly diagnosed AML patients, and observed that the percentage of each subset was comparable to peripheral blood (n=5, Supplemental Figure 6). So the increase of CD26lowPD-1+ CD8 T cells is unlikely due to migration between blood and bone marrow. As the increase of CD26lowPD-1+ T cells is associated with a decrease in CD26intPD-1+ T cells (Figure 1C), we suspect that differentiation of naïve CD26intPD-1+ T cells upon AML stimulation may be contributing. Our observation is different from the findings of Bozorgmehr et al, in which apoptosis of CD26high T cells is increased in CLL (50). The discrepancy is liked due to different disease context. This highlights the heterogeneity of T cell responses and importance of disease context-specific studies.
In summary, we made novel observations that CD26lowPD-1+ CD8 T cells are increased in newly diagnosed AML patients, and their phenotypic and functional features are consistent with terminal exhaustion status. These findings suggest a prognostic and predictive value of CD26 in AML, providing pivotal information to optimize the immunotherapy for this devastating cancer.
Data availability statement
The raw data supporting the conclusions of this article will be made available by the authors, without undue reservation.
Ethics statement
The studies involving human participants were reviewed and approved by Penn State Hershey Medical Center. The patients/participants provided their written informed consent to participate in this study.
Author contributions
HuZ and BJ designed the experiments, performed the research, analyzed the results and wrote the manuscript. CA and CZ performed the research and analyzed the results. KM, SM, WE, SM, JC, BW, NS, KR, and MN acquired samples, managed patients and discussed the data. JS discussed the data and reviewed the manuscript. HoZ conceived the concept, designed the experiments, oversaw the interpretation and presentation of the data, and wrote the manuscript. All authors contributed to the article and approved the submitted version.
Funding
This work was supported by the Penn State University Enhancing Health Initiative, the Kiesendahl Endowment funding, an Philanthropic Donation to our cancer research, and a Philanthropic Donation from Alan and Li Hao Colberg to our cancer research.
Acknowledgments
We thank all our patients for their trust, understanding, and willingness to provide their blood and bone marrow samples for our research.
Conflict of interest
The authors declare that the research was conducted in the absence of any commercial or financial relationships that could be construed as a potential conflict of interest.
Publisher’s note
All claims expressed in this article are solely those of the authors and do not necessarily represent those of their affiliated organizations, or those of the publisher, the editors and the reviewers. Any product that may be evaluated in this article, or claim that may be made by its manufacturer, is not guaranteed or endorsed by the publisher.
Supplementary material
The Supplementary Material for this article can be found online at: https://www.frontiersin.org/articles/10.3389/fimmu.2023.1169144/full#supplementary-material
References
1. National Cancer Institute Surveillance, Epidemiology, and End Results Program. Cancer stat facts: leukemia-acute myeloid leukemia (AML) (2022). Available at: https://seer.cancer.gov/statfacts/html/amyl.html (Accessed 5 January 2022).
2. Kong Y, Zhang J, Claxton DF, Ehmann WC, Rybka WB, Zhu L, et al. PD-1(hi)TIM-3(+) T cells associate with and predict leukemia relapse in AML patients post allogeneic stem cell transplantation. Blood Cancer J (2015) 5(7):e330. doi: 10.1038/bcj.2015.58
3. Kong Y, Zhu L, Schell TD, Zhang J, Claxton DF, Ehmann WC, et al. Zheng, h. T-cell immunoglobulin and ITIM domain (TIGIT) associates with CD8+ T-cell exhaustion and poor clinical outcome in AML patients. Clin Cancer Res (2016) 22(12):3057–66. doi: 10.1158/1078-0432.CCR-15-2626
4. Jia B, Zhao C, Rakszawski KL, Claxton DF, Ehmann WC, Rybka WB, et al. Eomes(+)T-bet(low) CD8(+) T cells are functionally impaired and are associated with poor clinical outcome in patients with acute myeloid leukemia. Cancer Res (2019) 79(7):1635–45. doi: 10.1158/0008-5472.Can-18-3107
5. Noviello M, Manfredi F, Ruggiero E, Perini T, Oliveira G, Cortesi F, et al. Bone marrow central memory and memory stem T-cell exhaustion in AML patients relapsing after HSCT. Nat Commun (2019) 10(1):1065. doi: 10.1038/s41467-019-08871-1
6. Zhou Q, Munger ME, Highfill SL, Tolar J, Weigel BJ, Riddle M, et al. Program death-1 signaling and regulatory T cells collaborate to resist the function of adoptively transferred cytotoxic T lymphocytes in advanced acute myeloid leukemia. Blood (2010) 116(14):2484–93. doi: 10.1182/blood-2010-03-275446
7. Mumprecht S, Schurch C, Schwaller J, Solenthaler M, Ochsenbein AF. Programmed death 1 signaling on chronic myeloid leukemia-specific T cells results in T-cell exhaustion and disease progression. Blood (2009) 114(8):1528–36. doi: 10.1182/blood-2008-09-179697
8. Norde WJ, Maas F, Hobo W, Korman A, Quigley M, Kester MG, et al. PD-1/PD-L1 interactions contribute to functional T-cell impairment in patients who relapse with cancer after allogeneic stem cell transplantation. Cancer Res (2011) 71(15):5111–22. doi: 10.1158/0008-5472.CAN-11-0108
9. Zhou Q, Munger ME, Veenstra RG, Weigel BJ, Hirashima M, Munn DH, et al. Coexpression of Tim-3 and PD-1 identifies a CD8+ T-cell exhaustion phenotype in mice with disseminated acute myelogenous leukemia. Blood (2011) 117(17):4501–10. doi: 10.1182/blood-2010-10-310425
10. Knaus HA, Berglund S, Hackl H, Blackford AL, Zeidner JF, Montiel-Esparza R, et al. Signatures of CD8+ T cell dysfunction in AML patients and their reversibility with response to chemotherapy. JCI Insight (2018) 3(21):e120974. doi: 10.1172/jci.insight.120974
11. Liu H. Emerging agents and regimens for AML. J Hematol Oncol (2021) 14(1):49. doi: 10.1186/s13045-021-01062-w
12. Zeidan AM, Boss I, Beach CL, Copeland WB, Thompson E, Fox BA, et al. A randomized phase 2 trial of azacitidine with or without durvalumab as first-line therapy for older patients with AML. Blood Adv (2022) 6(7):2219–29. doi: 10.1182/bloodadvances.2021006138
13. Zheng H, Mineishi S, Claxton D, Zhu J, Zhao C, Jia B, et al. A phase I clinical trial of avelumab in combination with decitabine as first line treatment of unfit patients with acute myeloid leukemia. Am J Hematol (2021) 96(2):E46–e50. doi: 10.1002/ajh.26043
14. Gorrell MD, Gysbers V, McCaughan GW. CD26: a multifunctional integral membrane and secreted protein of activated lymphocytes. Scandinavian J Immunol (2001) 54(3):249–64. doi: 10.1046/j.1365-3083.2001.00984.x
15. Lambeir AM, Durinx C, Scharpé S, De Meester I. Dipeptidyl-peptidase IV from bench to bedside: an update on structural properties, functions, and clinical aspects of the enzyme DPP IV. Crit Rev Clin Lab Sci (2003) 40(3):209–94. doi: 10.1080/713609354
16. Thul PJ, Åkesson L, Wiking M, Mahdessian D, Geladaki A, Ait Blal H, et al. A subcellular map of the human proteome. Science (2017) 356(6340):eaal3321. doi: 10.1126/science.aal3321
17. Uhlén M, Fagerberg L, Hallström BM, Lindskog C, Oksvold P, Mardinoglu A, et al. Proteomics. tissue-based map of the human proteome. Science (2015) 347(6220):1260419. doi: 10.1126/science.1260419
18. Waumans Y, Baerts L, Kehoe K, Lambeir AM, De Meester I. The dipeptidyl peptidase family, prolyl oligopeptidase, and prolyl carboxypeptidase in the immune system and inflammatory disease, including atherosclerosis. Front Immunol (2015) 6:387. doi: 10.3389/fimmu.2015.00387
19. Gerich J. Pathogenesis and management of postprandial hyperglycemia: role of incretin-based therapies. Int J Gen Med (2013) 6:877–95. doi: 10.2147/ijgm.S51665
20. Broxmeyer HE, Hoggatt J, O'Leary HA, Mantel C, Chitteti BR, Cooper S, et al. Dipeptidylpeptidase 4 negatively regulates colony-stimulating factor activity and stress hematopoiesis. Nat Med (2012) 18(12):1786–96. doi: 10.1038/nm.2991
21. Hatano R, Ohnuma K, Yamamoto J, Dang NH, Morimoto C. CD26-mediated co-stimulation in human CD8(+) T cells provokes effector function via pro-inflammatory cytokine production. Immunology (2013) 138(2):165–72. doi: 10.1111/imm.12028
22. Bailey SR, Nelson MH, Majchrzak K, Bowers JS, Wyatt MM, Smith AS, et al. Human CD26high T cells elicit tumor immunity against multiple malignancies via enhanced migration and persistence. Nat Commun (2017) 8(1):1961. doi: 10.1038/s41467-017-01867-9
23. Dohner H, Wei AH, Appelbaum FR, Craddock C, DiNardo CD, Dombret H, et al. Diagnosis and management of AML in adults: 2022 recommendations from an international expert panel on behalf of the ELN. Blood (2022) 140(12):1345–77. doi: 10.1182/blood.2022016867
24. Im SJ, Hashimoto M, Gerner MY, Lee J, Kissick HT, Burger MC, et al. Defining CD8+ T cells that provide the proliferative burst after PD-1 therapy. Nature (2016) 537(7620):417–21. doi: 10.1038/nature19330
25. Paley MA, Kroy DC, Odorizzi PM, Johnnidis JB, Dolfi DV, Barnett BE, et al. Progenitor and terminal subsets of CD8+ T cells cooperate to contain chronic viral infection. Science (2012) 338(6111):1220–5. doi: 10.1126/science.1229620
26. Utzschneider DT, Charmoy M, Chennupati V, Pousse L, Ferreira DP, Calderon-Copete S, et al. T Cell factor 1-expressing memory-like CD8(+) T cells sustain the immune response to chronic viral infections. Immunity (2016) 45(2):415–27. doi: 10.1016/j.immuni.2016.07.021
27. Miller BC, Sen DR, Al Abosy R, Bi K, Virkud YV, LaFleur MW, et al. Subsets of exhausted CD8(+) T cells differentially mediate tumor control and respond to checkpoint blockade. Nat Immunol (2019) 20(3):326–36. doi: 10.1038/s41590-019-0312-6
28. Wherry EJ, Ha SJ, Kaech SM, Haining WN, Sarkar S, Kalia V, et al. Molecular signature of CD8+ T cell exhaustion during chronic viral infection. Immunity (2007) 27(4):670–84. doi: 10.1016/j.immuni.2007.09.006
30. Goswami M, Oetjen K, Mulé MP, Sheela S, Wong HY, Liu Q, et al. Increased frequencies of PD-1+ CD8+ marrow-infiltrating lymphocytes associated with highly clonal T-lymphocyte expansions in relapsed and refractory AML patients but not healthy adults. Blood (2016) 128(22):1644–4. doi: 10.1182/blood.V128.22.1644.1644
31. Hutten TJA, Norde WJ, Woestenenk R, Wang RC, Maas F, Kester M, et al. Increased coexpression of PD-1, TIGIT, and KLRG-1 on tumor-reactive CD8(+) T cells during relapse after allogeneic stem cell transplantation. Biol Blood marrow Transplant (2018) 24(4):666–77. doi: 10.1016/j.bbmt.2017.11.027
32. Schnorfeil FM, Lichtenegger FS, Emmerig K, Schlueter M, Neitz JS, Draenert R, et al. Cells are functionally not impaired in AML: increased PD-1 expression is only seen at time of relapse and correlates with a shift towards the memory T cell compartment. J Hematol Oncol (2015) 8:93. doi: 10.1186/s13045-015-0189-2
33. Toffalori C, Zito L, Gambacorta V, Riba M, Oliveira G, Bucci G, et al. Immune signature drives leukemia escape and relapse after hematopoietic cell transplantation. Nat Med (2019) 25(4):603–11. doi: 10.1038/s41591-019-0400-z
34. Youngblood B, Oestreich KJ, Ha SJ, Duraiswamy J, Akondy RS, West EE, et al. Chronic virus infection enforces demethylation of the locus that encodes PD-1 in antigen-specific CD8(+) T cells. Immunity (2011) 35(3):400–12. doi: 10.1016/j.immuni.2011.06.015
35. Blackburn SD, Shin H, Freeman GJ, Wherry EJ. Selective expansion of a subset of exhausted CD8 T cells by αPD-L1 blockade. Proc Natl Acad Sci (2008) 105(39):15016–21. doi: 10.1073/pnas.0801497105
36. Barber DL, Wherry EJ, Masopust D, Zhu B, Allison JP, Sharpe AH, et al. Restoring function in exhausted CD8 T cells during chronic viral infection. Nature (2006) 439(7077):682–7. doi: 10.1038/nature04444
37. Khan O, Giles JR, McDonald S, Manne S, Ngiow SF, Patel KP, et al. TOX transcriptionally and epigenetically programs CD8+ T cell exhaustion. Nature (2019) 571(7764):211–8. doi: 10.1038/s41586-019-1325-x
38. Alfei F, Kanev K, Hofmann M, Wu M, Ghoneim HE, Roelli P, et al. TOX reinforces the phenotype and longevity of exhausted T cells in chronic viral infection. Nature (2019) 571(7764):265–9. doi: 10.1038/s41586-019-1326-9
39. Scott AC, Dündar F, Zumbo P, Chandran SS, Klebanoff CA, Shakiba M, et al. TOX is a critical regulator of tumour-specific T cell differentiation. Nature (2019) 571(7764):270–4. doi: 10.1038/s41586-019-1324-y
40. Yao C, Sun HW, Lacey NE, Ji Y, Moseman EA, Shih HY, et al. Single-cell RNA-seq reveals TOX as a key regulator of CD8(+) T cell persistence in chronic infection. Nat Immunol (2019) 20(7):890–901. doi: 10.1038/s41590-019-0403-4
41. Beltra J-C, Manne S, Abdel-Hakeem MS, Kurachi M, Giles JR, Chen Z, et al. Developmental relationships of four exhausted CD8+ T cell subsets reveals underlying transcriptional and epigenetic landscape control mechanisms. Immunity (2020) 52(5):825–41. doi: 10.1016/j.immuni.2020.04.014
42. Farag SS, Abu Zaid M, Schwartz JE, Thakrar TC, Blakley AJ, Abonour R, et al. Dipeptidyl peptidase 4 inhibition for prophylaxis of acute graft-versus-Host disease. N Engl J Med (2021) 384(1):11–9. doi: 10.1056/NEJMoa2027372
43. Okamoto T, Iwata S, Yamazaki H, Hatano R, Komiya E, Dang NH, et al. CD9 negatively regulates CD26 expression and inhibits CD26-mediated enhancement of invasive potential of malignant mesothelioma cells. PloS One (2014) 9(1):e86671. doi: 10.1371/journal.pone.0086671
44. Komiya E, Ohnuma K, Yamazaki H, Hatano R, Iwata S, Okamoto T, et al. CD26-mediated regulation of periostin expression contributes to migration and invasion of malignant pleural mesothelioma cells. Biochem Biophys Res Commun (2014) 447(4):609–15. doi: 10.1016/j.bbrc.2014.04.037
45. Donnenberg VS, Zhang JJ, Moravcikova E, Meyer EM, Lu H, Carson CT, et al. Antibody-based cell-surface proteome profiling of metastatic breast cancer primary explants and cell lines. Cytometry A (2018) 93(4):448–57. doi: 10.1002/cyto.a.23300
46. Pang R, Law WL, Chu AC, Poon JT, Lam CS, Chow AK, et al. A subpopulation of CD26+ cancer stem cells with metastatic capacity in human colorectal cancer. Cell Stem Cell (2010) 6(6):603–15. doi: 10.1016/j.stem.2010.04.001
47. Qin CJ, Zhao LH, Zhou X, Zhang HL, Wen W, Tang L, et al. Inhibition of dipeptidyl peptidase IV prevents high fat diet-induced liver cancer angiogenesis by downregulating chemokine ligand 2. Cancer Lett (2018) 420:26–37. doi: 10.1016/j.canlet.2018.01.064
48. Barreira da Silva R, Laird ME, Yatim N, Fiette L, Ingersoll MA, Albert ML. Dipeptidylpeptidase 4 inhibition enhances lymphocyte trafficking, improving both naturally occurring tumor immunity and immunotherapy. Nat Immunol (2015) 16(8):850–8. doi: 10.1038/ni.3201
49. Pech V, Abusaada K, Alemany C. Dipeptidyl peptidase-4 inhibition may stimulate progression of carcinoid tumor. Case Rep Endocrinol (2015) 2015:952019. doi: 10.1155/2015/952019
Keywords: AML, PD-1, CD26, t cell exhaustion, terminal exhaustion
Citation: Zhou H, Jia B, Annageldiyev C, Minagawa K, Zhao C, Mineishi S, Ehmann WC, Naik SG, Cioccio J, Wirk B, Songdej N, Rakszawski KL, Nickolich MS, Shen J and Zheng H (2023) CD26lowPD-1+ CD8 T cells are terminally exhausted and associated with leukemia progression in acute myeloid leukemia. Front. Immunol. 14:1169144. doi: 10.3389/fimmu.2023.1169144
Received: 18 February 2023; Accepted: 15 June 2023;
Published: 29 June 2023.
Edited by:
Eyad Elkord, University of Salford, United KingdomReviewed by:
Reshmi Parameswaran, Case Western Reserve University, United StatesSaid Dermime, National Center for Cancer Care and Research, Qatar
Copyright © 2023 Zhou, Jia, Annageldiyev, Minagawa, Zhao, Mineishi, Ehmann, Naik, Cioccio, Wirk, Songdej, Rakszawski, Nickolich, Shen and Zheng. This is an open-access article distributed under the terms of the Creative Commons Attribution License (CC BY). The use, distribution or reproduction in other forums is permitted, provided the original author(s) and the copyright owner(s) are credited and that the original publication in this journal is cited, in accordance with accepted academic practice. No use, distribution or reproduction is permitted which does not comply with these terms.
*Correspondence: Hong Zheng, aHpoZW5nQHBlbm5zdGF0ZWhlYWx0aC5wc3UuZWR1
†These authors have contributed equally to this work