- Department of Microbiology-Immunology, Northwestern University Feinberg School of Medicine, Chicago, IL, United States
Theiler’s murine encephalomyelitis virus (TMEV) establishes persistent viral infections in the central nervous system and induces chronic inflammatory demyelinating disease in susceptible mice. TMEV infects dendritic cells, macrophages, B cells, and glial cells. The state of TLR activation in the host plays a critical role in initial viral replication and persistence. The further activation of TLRs enhances viral replication and persistence, leading to the pathogenicity of TMEV-induced demyelinating disease. Various cytokines are produced via TLRs, and MDA-5 signals linked with NF-κB activation following TMEV infection. In turn, these signals further amplify TMEV replication and the persistence of virus-infected cells. The signals further elevate cytokine production, promoting the development of Th17 responses and preventing cellular apoptosis, which enables viral persistence. Excessive levels of cytokines, particularly IL-6 and IL-1β, facilitate the generation of pathogenic Th17 immune responses to viral antigens and autoantigens, leading to TMEV-induced demyelinating disease. These cytokines, together with TLR2 may prematurely generate functionally deficient CD25-FoxP3+ CD4+ T cells, which are subsequently converted to Th17 cells. Furthermore, IL-6 and IL-17 synergistically inhibit the apoptosis of virus-infected cells and the cytolytic function of CD8+ T lymphocytes, prolonging the survival of virus-infected cells. The inhibition of apoptosis leads to the persistent activation of NF-κB and TLRs, which continuously provides an environment of excessive cytokines and consequently promotes autoimmune responses. Persistent or repeated infections of other viruses such as COVID-19 may result in similar continuous TLR activation and cytokine production, leading to autoimmune diseases.
1 Theiler’s virus
Theiler’s murine encephalomyelitis virus (TMEV) belongs to the family of picornavirus, possessing a single positive RNA genome (1, 2). The picornavirus family includes a wide range of human pathogens, including rhinoviruses, cardioviruses, enteroviruses, and aphthoviruses, and TMEV belongings to the cardioviral group (3, 4). Two major subgroups of TMEV have been identified. One subgroup including GDVII and FA viruses, causes rapid and fatal encephalitis. Another subgroup including the BeAn8386 and DA strains, is known as Theiler’s original (TO) viruses. The inoculation of TO viruses intracerebrally into susceptible mice results in a biphasic neurological disease (2, 5–9). The early, acute phase displays flaccid limb paralysis and the degeneration of neurons (poliomyelitis). The late phase exhibits chronic, inflammatory demyelination (2). In contrast to the DA strain, the BeAn strain of the TO virus group is known to induce a clinically undetectable early-phase disease, although it results in a severe late-phase demyelinating disease (8, 9).
Viruses such as TMEV have been associated with CNS diseases including multiple sclerosis (10–14). Multiple sclerosis (MS) is a long-lasting autoimmune-mediated disease, resulting in demyelination in the white matter of the central nervous system (15). To understand the underlying pathogenic mechanisms of MS, several virus-induced models have been investigated (5, 7, 16, 17). Among virus-induced models, TMEV-induced demyelinating disease in mice has been extensively investigated because of the similarities in its histopathologic characteristics (2, 5, 18, 19). In addition, Saffold virus is an emerging human Theiler’s virus group, infecting more than 90% of human populations, which implicates potential importance in public health (20–22). MS may therefore involve chronic viral infections associated with the development of pathogenic immune responses reactive to viral and/or self-antigens. To explain virus-induced demyelinating disease in mice, several hypotheses have been proposed. First, “bystander” damage to CNS tissues occurs because of the host immune response against viral determinants (23, 24). Second, autoimmune responses to myelin proteins from damages to the CNS by anti-viral immune responses cause the disease development (19, 25, 26). Third, CNS damage incurred by persistent antiviral and autoimmune responses are induced by chronic over-stimulation via TLRs and other pathogen pattern recognition receptors (19, 27). Defining the underlying pathogenic mechanisms associated with TLR engagement with well-defined disease system like TMEV-induced demyelination has paramount importance in understanding the role of TLRs on viral persistence, cytokine production, and development of pathogenic immune responses, including autoimmune responses.
Infection with TMEV causes demyelinating disease only in certain mouse strains. C57BL/6 (B6) mice represent a resistant strain and SJL/J (SJL) mice represent a susceptible strain (28, 29). The viral loads in the spinal cords of resistant C57BL/6 mice are significantly lower than those of susceptible SJL mice throughout the viral infection period (30). Various cell types are permissive to TMEV infection, and these include oligodendrocytes, microglia, and astrocytes in the CNS and the dendritic cells, macrophages, and B cells in the CNS and periphery (31–35). The major cell populations that support viral persistence during chronic TMEV infection are microglia and/or macrophages in the CNS (36–39). Viral replication in microglia from susceptible SJL mice is significantly higher than that in microglia from resistant C57BL/6 mice and the viral load is similarly greater in microglia from SJL mice infected with TMEV compared with microglia from C57BL/6 mice (39). Because TMEV persistence in the CNS is a critically important prerequisite in the pathogenesis of demyelination (30, 40, 41), viral persistence may lead to continuous inflammatory cytokine production and the consequent pathogenic immune responses in the development of TMEV-induced demyelinating disease (Figure 1).
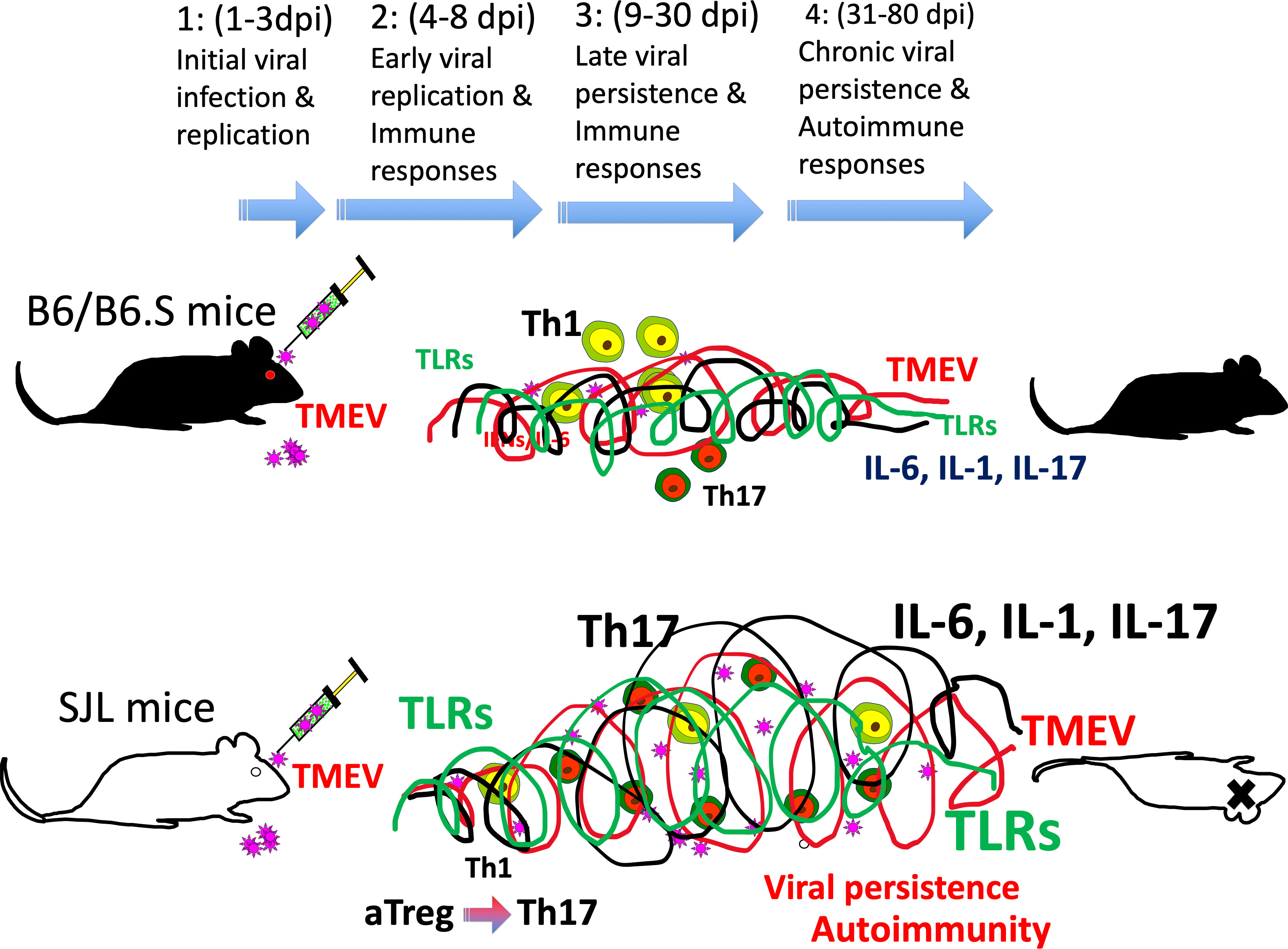
Figure 1 (1) 1-3 d post-infection: initial viral infection; (2) 4-8 d post-infection: early viral infection; (3) 9-30 d post-infection; (4) 31-80 d post-infection: chronic viral persistence. Resistant mice such as B6 and B6.S mice show rapid clearance of virus within 2-3 weeks, whereas susceptible SJL mice fail to clear viral persistence. Consequently, persistent TLR activation throughout infection leads to elevated levels of cytokines such as type I IFNs, IL-6, and IL-1beta, which favors stimulation of pathogenic Th17 response over protective Th1 response. Such pathogenic immune responses lead to the development of demyelinating disease in TMEV-infected SJL mice.
2 TLR expression and activation status may determine the susceptibility to viral infection
Toll-like receptors (TLRs) include a family of members, which recognize microbial products. This family of receptors is known to closely associate with the induction of initial innate immune responses following bacterial and viral infections (42, 43). mRNAs for TLR1–9 are expressed in microglia and stimulation of TLRs on the cells upregulates the expression of MHC class II and costimulatory molecules, which enable the microglia to present antigens to CD4+ T cells (38). Activated and/or differentiated cells are far more supportive of TMEV infection/replication (44–46). Consequently, the cytokine production levels in APCs, including microglia, in susceptible SJL mice are higher than those in resistant B6 mice (30, 39). For example, higher levels of TNFα, IL-6 and IL-1β are produced in microglia and macrophages from susceptible SJL mice after TMEV infection, compared with cells from resistant B6, B6.S, or B10.S mice (47–49). Similarly, viral loads in glia and antigen-presenting cells (APCs) from susceptible SJL mice with TMEV-induced demyelinating disease are significantly greater than that in cells from resistant B6.S, B6, and F1(B6XSJL) mice (34, 39). However, the levels of costimulatory molecule expression on APCs from susceptible mice and their ability to stimulate T cells during chronic TMEV infection are significantly poorer, which steers the pathogenic T cell responses (34, 39). Elevated TLR2, 3, 4, and 7 signaling in APCs enhances viral infection and replication (39, 50). Thus, persistent viral infection accompanied by continuous excessive cytokine production leads to viral persistence and the development of pathogenic immune responses. The association of TLR signals with pathogenesis is further supported by the fact that the administration of bacterial lipopolysaccharide (LPS), a ligand for TLR4, or poly I:C, a ligand for TLR3, increases viral loads and elevates the level of inflammatory response (50, 51). Thus, the differences in the type and intensity of TLR signals on antigen-presenting cells may affect the levels of viral infection, replication, and production of IL-1β and IL-6, which are critical factors in the development of demyelinating disease (Figure 2A).
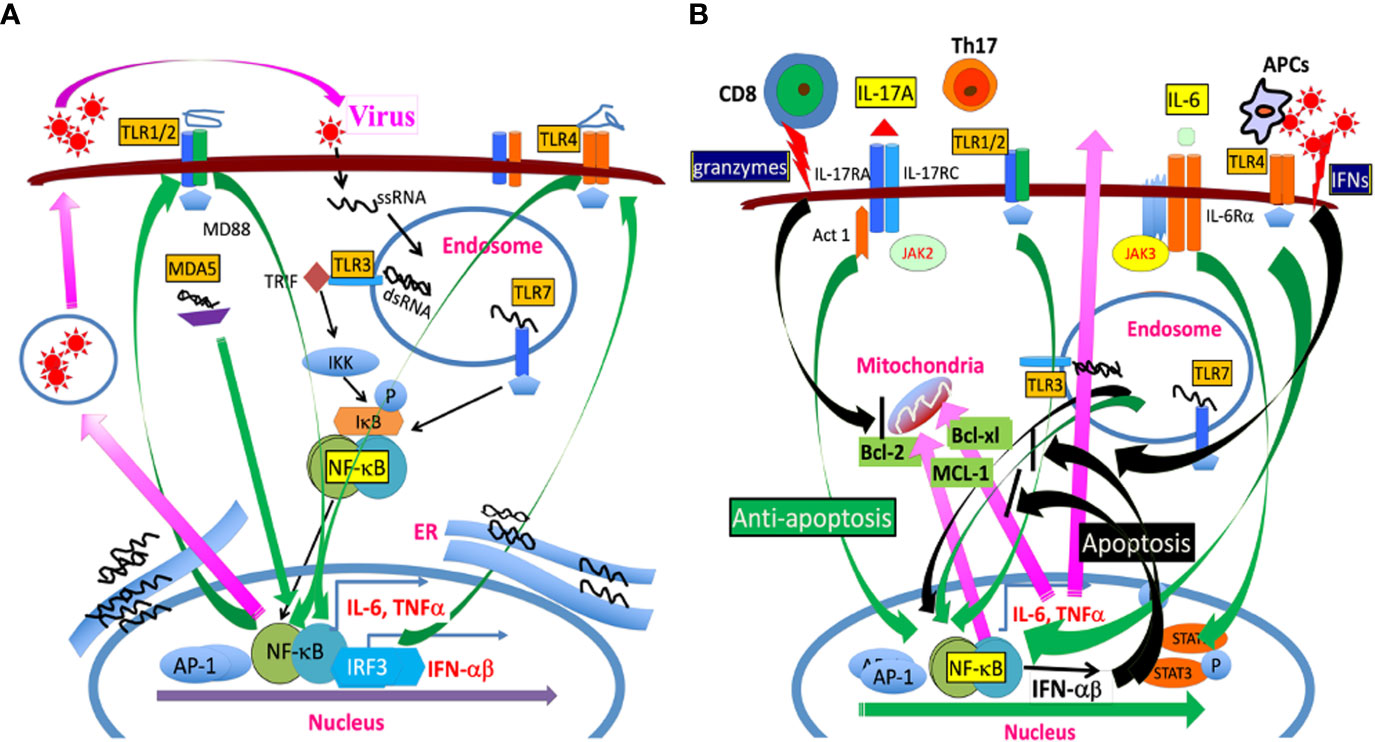
Figure 2 (A) TMEV infection releases a single-stranded RNA genome and double-stranded RNA replication intermediate in the endosome. The single-stranded RNA is recognized by TLR7 and the dsRNA intermediate is recognized by TLR3. The TLR signaling activates NF-κB, AP-1, and IRFs, which results in the production of various cytokines such as IL-6, IL-1beta, and type I IFNs. In addition, MDA5 also recognizes dsRNA replication intermediate, leading to NF-κB activation. These activations lead to the additional expression of TLR2/4 and other TLRs which further participates in activating NF-κB. The amplified NF-κB signaling further promotes TMEV replication because TMEV replication is dependent on the presence of activated NF-κB. (B) TLR signaling activates NF-κB, which results in the production of anti-apoptotic MCL-1, Bcl-2, Bcl-xL, and IL-6, inhibiting apoptosis induced by cytotoxic T cells and IFNs. IL-6 and IL-1beta further promote the generation of pathogenic Th17 cells. IL-17 produced by Th17 cells together with IL-6 synergistically inhibit the apoptosis of virus-infected cells by activating NF-κB and STAT3, leading to production of additional anti-apoptotic molecules in the mitochondria. The prolonged various TLR signals leading to NF-κB activation participate in the prolonged production of anti-apoptotic molecules and continuous TMEV replication.
3 NF-κB activation via TLRs is necessary for TMEV replication and cytokine production
TMEV has a single RNA genome which is recognized by TLR7, and a dsRNA intermediate which is recognized by TLR3 (42, 43). Consequently, TMEV infection leads to activation of NF-κB, AP-1, and IRFs via the TLR signals, resulting in the production of various cytokines, including IL-1β and IL-6 (39, 52–54). The melanoma differentiation-associated gene 5 also recognizes viral messages and participates in the activation of NF-κB (55). The infection of primary glial cells results in the activation of a wide range of chemokine genes, including CXCL1, CXCL2, CXCL10, CCL2, CCL3, CCL4, CCL5, CCL7 and CCL12 (56–58). The production of chemokines and cytokines following various viral infections is dependent on the activation of NF-κB via pattern recognition receptors (32, 52, 59–62). These chemokines further activate CXCR3, CCR7 and CCR5 genes, which further promote the cellular recruitment and infiltration to the CNS (63, 64). In addition, IL-1β, IL-6, IFNα/β, and TNFα produced following TMEV infection further stimulate cells and upregulate the production of CCL2, CCL5, CXCL10 (65–67). These cytokines further promote the development of pathogenic Th17 cells and consequent demyelination (27, 34, 39, 68). Increases in the production of fibrin deposition, adhesion molecules (ICAM and VCAM), and endothelin-1 associated with blood–brain-barrier permeability, contributing to the pathogenesis of demyelinating disease have also been observed after TMEV infection (35, 69–72).
The treatment of resistant C57BL/6 mice with LPS, a ligand of TLR4, or IL-1β increases the viral load in the CNS and leads to the pathogenesis of demyelinating disease (51, 73). Similarly, administration of poly I:C, a ligand of TLR3 leads to the rapid progression of demyelinating disease in mice infected with TMEV (50). The activation of NF-κB via TLRs is associated with TMEV replication and the production of various inflammatory cytokines and chemokine (52, 66, 67). Furthermore, TNF-α, IL-6, and IL-1β produced after TMEV infection further activate NF-κB, promoting increased TMEV replication. NF-κB activation further leads to stimulation of additional TLRs, amplifying pathogenic signals in the deployment of demyelinating disease (45, 52, 66, 74, 75). This is consistent with the fact that TMEV infection and replication are greater in the cells of susceptible mice and that excessive TLR signal and cytokine production, including IL-1β, IL-6, and TGFβ, further promotes the pathogenesis of demyelinating disease (47, 52, 66).
TMEV infection also activates NLRP3 via TLR signaling (74). Activation of NLRP3 inflammasome results in the production of IL-1β and PEG2, promoting the pathogenesis (75, 76). TMEV-infected susceptible SJL mice produce much greater levels of IL-1β and other inflammatory cytokines compared with resistant mice. The presence of high levels of IL-1β and other inflammatory cytokines such as IL-6 promotes pathogenic Th17 responses (47, 68, 73). In addition, IL-17 from Th17 responses further promotes IL-6 production (77, 78), together inhibiting cellular apoptosis (68, 79). The excessive activation of PGE2 signaling also contributes to pathogenesis (74, 80) by preventing the T cell killing of target cells (81). TMEV infection also results in the increased expression of PD-1 and PDL-1 via IL-6 signaling, inhibiting cytotoxic T cell function (81, 82). Moreover, the IL-1β signal induces IL-6 production and further promotes Th17 cell expansion (73, 83). Thus, elevated perpetual TLR signaling and consequent persistent cytokine overproduction lead to viral persistence by blocking apoptosis and consequently facilitate pathogenic immune responses in susceptible mice infected with TMEV.
4 Excessive cytokine production via TLR activation leads to pathogenic immune responses
TMEV-specific Th1 cells producing IFN-γ can lyse virus-infected cells in a Fas-dependent manner (84). The presence of capsid specific Th1 responses delays the development of demyelinating disease (85–87). In addition, mice deficient in IFN-γ or its receptor genes cannot efficiently clear TMEV infection, resulting in rapid development of demyelinating disease (88, 89). Similarly, deficiency in the IFN-γ receptor significantly accelerates the onset of disease in mice (90, 91). Thus, the level of Th1 responses may play a protective role in the development of TMEV-induced demyelinating disease.
Th17 cells, a subpopulation of Th cells which produce IL-17, are associated with the development of various autoimmune diseases (92–95). Th17 cells are developed in the presence of IL-6, and Th17 cell levels are significantly greater in susceptible mice infected with TMEV. The role of Th17 responses in the pathogenesis of TMEV-induced demyelinating disease has been verified by administrating anti-IL-17 antibodies (68) or using Th17-biased RORγt Tg mice (96). DCs infected with live TMEV producing various innate immune responses, but not epitope peptide-loaded DCs, are able to induce the development of Th17 responses (68). These results indicate that the virus-infected environment, including the presence of various cytokines, is required to induce pathogenic Th17 cells in the development of TMEV-induced demyelinating diseases.
High levels of FoxP3+CD4+ T cells, which constitute a subset of Th cells, appear first in the CNS of virus-infected mice (97, 98). The TLR2-mediated signal induced following TMEV infection may be involved in the early generation of CD25-FoxP3+CD4+ T cells (54, 99, 100). CD25lo FoxP3+CD4+ T cells are found in levels as much as two-fold higher in the CNS of virus-infected SJL mice compared with B6 mice (101). CD25loFoxP3+CD4+ T cells do not display a regulatory function (102), and the presence of a high level of CD25loFoxP3+CD4+ T cells may promote the development of TMEV-induced demyelinating disease (97). CD25loFoxP3+CD4+ T cells appear to undergo trans-differentiation into Th17 cells following the loss of FoxP3 expression (103, 104). Thus, these CD25loFoxP3+CD4+ T cells appearing early in the CNS of TMEV-infected mice may be converted into pathogenic Th17 cells in the presence of excessive cytokines (68, 101). Similarly elevated levels of CD25-FoxP3+CD4+ T cells have been detected in patients with chronic hepatitis B virus infection (105) and systemic lupus (106).
Cytotoxic CD8+ T cells producing IFN-γ and perforin play an important role in protection of mice from developing TMEV-induced demyelinating disease (107–109). Cytotoxic CD8+ T cells recognizing viral determinants appear to participate in causing damages of virus-infected, myelin-producing oligodendrocytes and other cell types in the CNS (110–114). The presence of CD8+ T cells is necessary to manifest clinical symptoms, but the protective role of these cells in the development of TMEV-induced demyelinating disease has also been demonstrated (111–114). Thus, certain CD8+ T cell populations, depending on their specificity and or cytokine profile are likely to play different roles in the pathogenesis of TMEV-induced demyelination.
TMEV productively infects approximately 50% of primary CD20+ B cells and 25% of CD19+CD20+ cells in susceptible SJL mice (27). TMEV-infected B cells express elevated levels of CD69 as well as MHC class II and costimulatory molecules and exhibit elevated levels of antibody production and enhanced antigen-presenting function to T cells. B cell activation after TMEV infection resembles B cells treated with TLR ligands for TLR2, TLR3, TLR4, TLR7, and TLR9 (27). These results strongly suggest that B cell activation following TMEV infection is associated with TLR signals, consistent with previous findings that various TLR-mediated signals activate B cells (115, 116). TMEV infection also triggers B cells of susceptible mice to produce the excessive production of IFN-α/β, IL-6, IL-1β, and PGE2 (27, 117). These innate immune responses further elevate the activation of B cells to produce antibodies to viral and self-antigens and vigorously promote pathogenic Th17 cell responses (27, 117–119).
B cells producing antibodies to TMEV capsid antigens are detected in the demyelinating lesions and spinal fluids of TMEV-infected mice (120–122). Plasma cells producing anti-TMEV antibodies are also detected in the meninges of CNS parenchyma (123). Antibodies to TMEV determinants play a protective role during the early stage of viral infection (124–126). In the absence of CD8+ T cells, antibody response is critically important in protecting mice from Theiler’s virus-induced encephalitis (125). In addition, the pathogenesis of TMEV-induced demyelinating disease is accelerated in mice treated with the monoclonal anti-CD20 antibody (127). However, the contribution of anti-TMEV antibodies to the protection of mice from demyelinating disease is relatively minor compared with the protection by CD4+ Th1 and CD8+ T cells (125, 128).
5 Role of NF-κB activated by TLRs in viral persistence
APCs from susceptible SJL mice are highly susceptible to TMEV infection and the viral infection leads to the activation of NF-κB via TLRs (27, 34, 52). The activation of NF-κB leads to the production of high levels of TNF-α, IL-1β, and IL-6, which are associated with activation of B cells and directional T cell responses (129–131). Type I IFNs produced in consequences of TLR activation contribute to the activation of NF-κB and cellular apoptosis (34, 52, 132, 133). In contrast, high levels of type I or II IFNs provide only transient protection against TMEV infection and thus high levels of these cytokines in susceptible SJL mice may not participate in controlling viral loads in TMEV-infected mice (34, 132, 134). The resistance of TMEV infection against type I IFNs is consistent with previous observations in other viruses (134–136).
Activation of NF-κB is necessary for TMEV replication (45) and consequently the state of host cell activation determines the level of viral replication (137). Following TMEV infection, TLR2, 3, 4, and 7 are associated with the production of various cytokines in different glial cells and professional antigen-presenting cells (27, 39, 52, 54, 138). TLR7 recognizes the single-stranded TMEV RNA genome and TLR3 interacts with the double-stranded RNA replication intermediate in the endosome (48, 52, 139). TMEV infection results in the engagement of various TLRs and other related signals to activate NF-κB, AP-1, and IRFs (54, 55, 140, 141). In turn, these signals result in the production of various cytokines, including TNFα, IL-1β and IL-6. NF-κB activation leads to the elevated expression and activation of TLRs including TLR2/4, which further activate NF-κB at a higher level (54). Such amplified NF-κB signaling further promotes the expression of anti-apoptotic Bcl-2 and Bcl-xL molecules, supporting vigorous TMEV replication (34, 142, 143). Such prevention of apoptosis following TMEV infection may result in further persistent viral replication and excessive cytokine production (Figure 2B).
6 Production of anti-apoptotic cytokines by virus-infected cells
IL-6 is a major cytokine produced following TMEV and other viral infections and displays an anti-apoptotic function (50, 144, 145). IL-6 inhibits the cytolytic function of CD8+ T cells and drives Th17 responses. IL-17 produced by Th17 cells also inhibits cytolytic function of virus-reactive CD8+ T cells, further promoting TMEV persistence (68). Furthermore, IL-6 and IL-17 together synergistically inhibit cytotoxic function of virus-specific CD8+ T cells (79). IL-17 is also known to enhance tumor growth in animals, supporting the inhibitory function of IL-17 on cytotoxic CD8+ T cells (146, 147). Cytotoxic CD8+ T cells and some CD4+ T cells induce the cytolysis of virus-infected cells through the granule exocytosis and or the activation of Fas–FasL pathway (86, 148–151). IL-17 upregulates the expression of Bcl-2 and Bcl-xl molecules via the NF-κB pathway and subsequently these prosurvival molecules protect the target cells from the apoptosis induced by cytotoxic CD4+ and CD8+ T cells (68). Nevertheless, the synergistic inhibition of apoptosis of virus-infected cells by IL-17 and IL-6 may serve as a powerful means for viral persistence. The inhibition of apoptosis extends the life of virus-infected cells, resulting in prolonged viral replication and persistence (Figure 2B). Thus, the interaction between virus and host cells via IL-17 and IL-6 and/or other cytokines results in persistent viral infection and prolonged harmful immune responses including various autoimmune responses, which ultimately lead to the development of TMEV-induced demyelinating disease (152). Similarly, persistent and or repeated infections of COVID-19 appear to stimulate TLRs, leading to cytokine storms and autoimmune responses in pathogenesis (153–156).
7 Viral persistence, continuous TLR signals, and cytokine storms lead to autoimmunity
Chronic infection with TMEV results in antibody responses to self-antigens including myelin basic protein (MBP) in the CNS of infected mice (25, 27, 157). Similarly, CD4+ T cell responses to viral antigens and myelin-associated autoantigens, including MBP and proteolipid protein, have been detected during persistent infection with TMEV (19). In addition, a CD8+ cytotoxic T cell population, recognizing both viral and self-antigens, has been identified in TMEV-infected SJL mice (158). Thus, various immune responses, including antibody, CD4+ T cell, and CD8+ T cell responses to viral and CNS autoantigens, are induced in mice with chronic TMEV infection. Sequestered autoantigens to the CNS may be released following TMEV-induced tissue damage, and these autoantigens subsequently induce autoimmune responses under constant elevated TLR stimulation and cytokine production (19, 27). The initial insults to the CNS by T cells reactive to viral antigens appear to be necessary to the pathogenesis of demyelinating disease (84, 113, 159). Such immune-mediated initial tissue damage in addition to virus-induced cellular apoptosis may be necessary to result in the development of TMEV-induced demyelinating disease.
Following intraperitoneal infection of systemic lupus erythematosus-prone NZBWF1 and BXSB male mice with TMEV or Coxsackie virus, the production of autoantibodies to several nuclear autoantigens has been rapidly accelerated (27). Since these mice have either duplicated or altered TLR genes (115, 160–162), the elevated expression of TLRs and their continuous stimulation is likely responsible for autoimmunity. These results strongly suggest that chronic TMEV infection continuously stimulates preexisting autoimmune cells via virus-induced TLR-mediated polyclonal activation. This notion is supported by the fact that the treatment of cells with the ligands of different TLRs activates B cells and T cells similarly to TMEV infection (27). Because virus-reactive T cell responses are required for the development of TMEV-induced demyelinating disease, immune-mediated tissue damage to the CNS may be necessary (68, 107, 111, 159). Therefore, these anti-viral and autoimmune responses appear to participate in protecting and/or damaging the related tissues (19, 68, 159, 163–165). However, the presence of high levels of proinflammatory cytokines during chronic viral infection may lead to the development of harmful autoimmune responses (166–168). Taken together, persistent TMEV infection leads to continuous stimulation of various TLRs and consequently results in TLR-mediated polyclonal activation of B and T cells. Although the roles of autoimmune responses in the pathogenesis of TMEV-induced demyelinating disease are not certain, they are likely to contribute to the overall pathogenic outcome by participating in further tissue damage and/or cytokine production.
Author contributions
BK: writing—review and editing, funding acquisition.
Funding
This work was supported by the United States Public Health Service Grants (RO1 NS28752 and RO1 NS33008) and a grant from the National Multiple Sclerosis Society (RG 4001-A6).
Acknowledgments
I acknowledge all the colleagues who have participated in original contribution to this research in the past years.
Conflict of interest
The author declares that the research was conducted in the absence of any commercial or financial relationships that could be construed as a potential conflict of interest.
Publisher’s note
All claims expressed in this article are solely those of the authors and do not necessarily represent those of their affiliated organizations, or those of the publisher, the editors and the reviewers. Any product that may be evaluated in this article, or claim that may be made by its manufacturer, is not guaranteed or endorsed by the publisher.
Abbreviations
MS, multiple sclerosis; CNS, central nervous system; TMEV, Theiler’s murine encephalomyelitis virus; TMEV-IDD, TMEV-induced demyelinating disease; Tg, transgenic mice; TCR, T cell receptor; MHC, major histocompatibility complex.
References
1. Theiler M. Spontaneous encephalomyelitis of mice-a new virus disease. J Exp Med (1934) 80:122. doi: 10.1126/science.80.2066.122-b
2. Lipton HL. Theiler's virus infection in mice: an unusual biphasic disease process leading to demyelination infect. Immun (1975) 11:1147–55. doi: 10.1128/iai.11.5.1147-1155.1975
3. Nitayaphan S, Omilianowski D, Toth MM, Parks GD, Rueckert RR, Palmenberg AC, et al. Relationship of theiler's murine encephalomyelitis viruses to the cardiovirus genus of picornaviruses. Intervirology (1986) 26:140–8. doi: 10.1159/000149693
5. Daniels JB, Pappenheimer AM, Richardson S. Observations on encephalomyelitis of mice (DA strain). J Exp Med (1952) 96:517. doi: 10.1084/jem.96.6.517
6. Lehrich JR, Arnason BG, Hochberg FH. Demyelinative myelopathy in mice induced by the DA virus. J Neurol Sci (1976) 29(2-4):149–60. doi: 10.1016/0022-510X(76)90167-2
7. Lipton HL, Friedmann A. Purification of theiler's murine encephalomyelitis virus and analysis of the structural virion polypeptides: correlation of the polypeptide profile with virulence. J Virol (1980) 33:1165–72. doi: 10.1128/jvi.33.3.1165-1172.1980
8. Dal Canto MC, Lipton HL. Primary demyelination in theiler's virus infection. an ultrastructural study. Lab Invest (1975) 33:626–37.
9. Lipton HL, Dal Canto MC. The TO strains of theiler’s viruses cause "slow virus-like" infections in mice. Ann Neurol (1979) 6:25–8. doi: 10.1002/ana.410060106
11. McFarlin DE, McFarland HF. Multiple sclerosis (first of two parts). N Engl J Med (1982) 307(19):1183– 8. doi: 10.1056/NEJM198211043071905
12. Soldan SS, Berti R, Salem N, Secchiero P, Flamand L, Calabresi PA, et al. Association of human herpes virus 6 (HHV-6) with multiple sclerosis: increased IgM response to HHV-6 early antigen and detection of serum HHV-6 DNA. Nat Med (1997) 3(12):1394–7. doi: 10.1038/nm1297-1394
13. Akhyani N, Berti R, Brennan MB, Soldan SS, Eaton JM, McFarland HF, et al. Tissue distribution and variant characterization of human herpesvirus (HHV)-6: increased prevalence of HHV-6A in patients with multiple sclerosis. J Infect Dis (2000) 182(5):1321–5. doi: 10.1086/315893
14. Martinez A, Alvarez-Lafuente R, Mas A, Bartolome M, Garcia-Montojo M, de Las Heras V, et al. Environment-gene interaction in multiple sclerosis: human herpesvirus 6 and MHC2TA. Hum Immunol (2007) 68(8):685–9. doi: 10.1016/j.humimm.2007.05.005
16. Buchmeier MJ, Lane TE. Viral-induced neurodegenerative disease. Curr Opin Microbiol (1999) 2(4):398–402. doi: 10.1016/S1369-5274(99)80070-8
17. Wege H, Siddell S, ter Meulen V. The biology and pathogenesis of coronaviruses. Curr Top Microbiol Immunol (1982) 99:165–200. doi: 10.1007/978-3-642-68528-6_5
18. Dal Canto MC, Kim BS, Miller SD, Melvold RW. Theiler's murine encephalomyelitis virus (TMEV)-induced demyelination: a model for human multiple clerosis. Methods (1996) 10(3):453–61. doi: 10.1006/meth.1996.0123
19. Miller SD, Vanderlugt CL, Begolka WS, Pao W, Yauch RL, Neville KL, et al. Persistent infection with theiler's virus leads to CNS autoimmunity via epitope spreading. Nat Med (1997) 3(10):1133–6. doi: 10.1038/nm1097-1133
20. Liang Z, Kumar AS, Jones MS, Knowles NJ, Lipton HL. Phylogenetic analysis of the species theilovirus: emerging murine and human pathogens. J Virol (2008) 82(23):11545–54. doi: 10.1128/JVI.01160-08
21. Chiu CY, Greninger AL, Kanada K, Kwok T, Fischer KF, Runckel C, et al. Identification of cardioviruses related to theiler's murine encephalomyelitis virus in human infections. Proc Natl Acad Sci U.S.A. (2008) 105(37):14124–9. doi: 10.1073/pnas.0805968105
22. Zoll J, Erkens Hulshof S, Lanke K, Verduyn Lunel F, Melchers WJ, Schoondermark-van de Ven E, et al. Saffold virus, a human theiler's-like cardiovirus, is ubiquitous and causes infection early in life. PloS Pathog (2009) 5(5):e1000416. doi: 10.1371/journal.ppat.1000416
23. Wisniewski HM, Bloom BR. Primary demyelination as a nonspecific consequence of a cell-mediated immune reaction. J Exp Med (1975) 141(2):346–59. doi: 10.1084/jem.141.2.346
24. Clatch RJ, Lipton HL, Miller SD. Characterization of theiler's murine encephalomyelitis virus (TMEV)-specific delayed-type hypersensitivity responses in TMEV-induced demyelinating disease: correlation with clinical signs. J Immunol (1986) 136:920–7. doi: 10.4049/jimmunol.136.3.920
25. Rauch HC, Montgomery IN, Hinman CL, Harb W, Benjamins JA. Chronic theiler's virus infection in mice: appearance of myelin basic protein in the cerebrospinal fluid and serum antibody directed against MBP. J Neuroimmunol (1987) 14:35–48. doi: 10.1016/0165-5728(87)90099-3
26. Yamada M, Zurbriggen A, Fujinami RS. Monoclonal antibody to theiler's murine encephalomyelitis virus defines a determinant on myelin and oligodendrocytes, and augments demyelination in experimental allergic encephalomyelitis. J Exp Med (1990) 171:1893–907. doi: 10.1084/jem.171.6.1893
27. Jin YH, Kim CX, Huang J, Kim BS. Infection and activation of b cells by theiler's murine encephalomyelitis virus (TMEV) leads to autoantibody production in an infectious model of multiple sclerosis. Cells (2020) 9(8):1787. doi: 10.3390/cells9081787
28. Lipton HL, Dal Canto MC. Susceptibility of inbred mice to chronic central nervous system infection by theiler's murine encephalomyelitis virus. Infect Immun (1979) 26:369–74. doi: 10.1128/iai.26.1.369-374.1979
29. Rodriguez M, David CS. Demyelination induced by theiler's virus: influence of the h-2 haplotype. J Immunol (1985) 135:2145–8. doi: 10.4049/jimmunol.135.3.2145
30. Jin YH, Mohindru M, Kang MH, Fuller AC, Kang B, Gallo D, et al. Differential virus replication, cytokine production, and antigen-presenting function by microglia from susceptible and resistant mice infected with theiler's virus. J Virol (2007) 81(21):11690–702. doi: 10.1128/JVI.01034-07
31. Anderson R, Harting E, Frey MS, Leibowitz JL, Miranda RC. Theiler's murine encephalomyelitis virus induces rapid necrosis and delayed apoptosis in myelinated mouse cerebellar explant cultures. Brain Res (2000) 868(2):259–67. doi: 10.1016/S0006-8993(00)02338-6
32. Palma JP, Kim BS. Induction of selected chemokines in glial cells infected with theiler's virus. J Neuroimmunol (2001) 117(1-2):166–70. doi: 10.1016/S0165-5728(01)00326-5
33. Zheng L, Calenoff MA, Dal Canto MC. Astrocytes, not microglia, are the main cells responsible for viral persistence in theiler's murine encephalomyelitis virus infection leading to demyelination. J Neuroimmunol (2001) 118(2):256–67. doi: 10.1016/S0165-5728(01)00338-1
34. Hou W, So EY, Kim BS. Role of dendritic cells in differential susceptibility to viral demyelinating disease. PloS Pathog (2007) 3(8):e124. doi: 10.1371/journal.ppat.0030124
35. Jin YH, Kang B, Kang HS, Koh CS, Kim BS. Endothelin-1 contributes to the development of virus-induced demyelinating disease. J Neuroinflamm (2020) 17(1):307. doi: 10.1186/s12974-020-01986-z
36. Clatch RJ, Miller SD, Metzner R, Dal Canto MC, Lipton HL. Monocytes/macrophages isolated from the mouse central nervous system contain infectious theiler's murine encephalomyelitis virus (TMEV). Virology (1990) 176:244–54. doi: 10.1016/0042-6822(90)90249-Q
37. Clatch RJ, Melvold RW, Miller SD, Lipton HL. Theiler's murine encephalomyelitis virus (TMEV)-induced demyelinating disease in mice is influenced by the h-2D region: correlation with TEMV-specific delayed-type hypersensitivity. J Immunol (1985) 135(2):1408–14. doi: 10.4049/jimmunol.135.2.1408
38. Olson JK, Miller SD. Microglia initiate central nervous system innate and adaptive immune responses through multiple TLRs. J Immunol (2004) 173(6):3916–24. doi: 10.4049/jimmunol.173.6.3916
39. Jin YH, Kang HS, Hou W, Meng L, Kim BS. The level of viral infection of antigen-presenting cells correlates with the level of development of theiler's murine encephalomyelitis virus-induced demyelinating disease. J Virol (2015) 89(3):1867–78. doi: 10.1128/JVI.02471-14
40. Lipton HL, Melvold R. Genetic analysis of susceptibility to theiler's virus-induced demyelinating disease in mice. J Immunol (1984) 132:1821–5. doi: 10.4049/jimmunol.132.4.1821
41. Lipton HL, Kumar AS, Trottier M. Theiler's virus persistence in the central nervous system of mice is associated with continuous viral replication and a difference in outcome of infection of infiltrating macrophages versus oligodendrocytes. Virus Res (2005) 111(2):214–23. doi: 10.1016/j.virusres.2005.04.010
42. Akira S. TLR signaling. Curr Top Microbiol Immunol (2006) 311:1–16. doi: 10.1007/3-540-32636-7_1
43. Diebold SS, Kaisho T, Hemmi H, Akira S. Innate antiviral responses by means of TLR7-mediated recognition of single-stranded RNA. Science (2004) 303:1529–31. doi: 10.1126/science.1093616
44. Son KN, Becker RP, Kallio P, Lipton HL. Theiler's virus-induced intrinsic apoptosis in M1-d macrophages is bax mediated and restricts virus infectivity: a mechanism for persistence of a cytolytic virus. J Virol (2008) 82(9):4502–10. doi: 10.1128/JVI.02349-07
45. Kang MH, So EY, Park H, Kim BS. Replication of theiler's virus requires NF-kappaB-activation: higher viral replication and spreading in astrocytes from susceptible mice. Glia (2008) 56(9):942–53. doi: 10.1002/glia.20668
46. Schneider KM, Watson NB, Minchenberg SB, Massa PT. The influence of macrophage growth factors on theiler's murine encephalomyelitis virus (TMEV) infection and activation of macrophages. Cytokine (2018) 102:83–93. doi: 10.1016/j.schres.2017.08.003
47. Chang JR, Zaczynska E, Katsetos CD, Platsoucas CD, Oleszak EL. Differential expression of TGF-beta, IL-2, and other cytokines in the CNS of theiler's murine encephalomyelitis virus-infected susceptible and resistant strains of mice. Virology (2000) 278(2):346–60. doi: 10.1006/viro.2000.0646
48. Petro TM. Disparate expression of IL-12 by SJL/J and B10.S macrophages during theiler's virus infection is associated with activity of TLR7 and mitogen-activated protein kinases. Microbes Infect (2005) 7:224–32. doi: 10.1016/j.micinf.2004.10.014
49. Moore TC, Bush KL, Cody L, Brown DM, Petro TM. Control of early theiler's murine encephalomyelitis virus replication in macrophages by interleukin-6 occurs in conjunction with STAT1 activation and nitric oxide production. J Virol (2012) 86(19):10841–51. doi: 10.1128/JVI.01402-12
50. Jin YH, Kaneyama T, Kang MH, Kang HS, Koh CS, Kim BS. TLR3 signaling is either protective or pathogenic for the development of theiler's virus-induced demyelinating disease depending on the time of viral infection. J Neuroinflamm (2011) 8:178. doi: 10.1186/1742-2094-8-178
51. Pullen LC, Park SH, Miller SD, Dal Canto MC, Kim BS. Treatment with bacterial LPS renders genetically resistant C57BL/6 mice susceptible to theiler's virus-induced demyelinating disease. J Immunol (1995) 155(9):4497–503. doi: 10.4049/jimmunol.155.9.4497
52. So EY, Kang MH, Kim BS. Induction of chemokine and cytokine genes in astrocytes following infection with theiler’s murine encephalomyelitis virus is mediated by the toll-like receptor 3. Glia (2006) 53:858–67. doi: 10.1002/glia.20346
53. Turrin NP. Central nervous system toll-like receptor expression in response to theiler’s murine encephalomyelitis virus-induced demyelinating disese in resistnt and susceptible mouse strains. Virol J (2008) 5:154. doi: 10.1186/1743-422X-5-154
54. So EY, Kim BS. Theiler's virus infection induces TLR3-dependent upregulation of TLR2 critical for proinflammatory cytokine production. Glia (2009) 57:1216–26. doi: 10.1002/glia.20843
55. Jin YH, Kim SJ, So EY, Meng L, Colonna M, Kim BS. Melanoma differentiation-associated gene 5 is critical for protection against theiler's virus-induced demyelinating disease. J Virol (2012) 86:1531–43. doi: 10.1128/JVI.06457-11
56. Palma JP, Kim BS. The scope and activation mechanisms of chemokine gene expression in primary astrocytes following infection with theiler's virus. J Neuroimmunol (2004) 149:121–9. doi: 10.1016/j.jneuroim.2003.12.025
57. Rubio N, Sanz-Rodriguez F, Lipton HL. Theiler's virus induces the MIP-2 chemokine (CXCL2) in astrocytes from genetically susceptible but not from resistant mouse strains. Cell Immunol (2006) 239:31–40. doi: 10.1016/j.cellimm.2006.03.003
58. Rubio N, Sanz-Rodriguez F. Induction of the CXCL1 (KC) chemokine in mouse astrocytes by infection with the murine encephalomyelitis virus of theiler. Virology (2007) 358:98–108. doi: 10.1016/j.virol.2006.08.003
59. Asensio VC, Campbell IL. Chemokine gene expression in the brains of mice with lymphocytic choriomeningitis. J Virol (1997) 71:7832–40. doi: 10.1128/jvi.71.10.7832-7840.1997
60. Lane TE, Asensio VC, Yu N, Paoletti AD, Campbell IL, Buchmeier MJ. Dynamic regulation of alpha- and beta-chemokine expression in the central nervous system during mouse hepatitis virus-induced demyelinating disease. J Immunol (1998) 180:970–8. doi: 10.4049/jimmunol.160.2.970
61. Hoffman LM, Eife BT, Begolka WS, Miller SD, Karpus WJ. Central nervous system chemokine expression during theiler's virus-induced demyelinating disease. J Neurovirol (1999) 5:635–42. doi: 10.3109/13550289909021292
62. Noe KH, Cenciarelli C, Moyer SA, Rota PA, Shin ML. Requirements for measles virus induction of RANTES chemokine in human astrocytoma-derived U373 cells. J Virol (1999) 73:3117–24. doi: 10.1128/JVI.73.4.3117-3124.1999
63. Guidotti LG, Chisari FV. Noncytolytic control of viral infections by the innate and adaptive immune response. Annu Rev Immunol (2001) 19:65–91. doi: 10.1146/annurev.immunol.19.1.65
64. Ludewig B, Junt T, Hengartner B, Zinkernagel RM. Dendritic cells in autoimmune diseases. Curr Opin Immunol (2001) 13:657–62. doi: 10.1016/S0952-7915(01)00275-8
65. Veckman V, Österlund P, Fagerlund R, Melén K, Matikainen S, Julkunen I. TNF-α and IFN-α enhance influenza-a-virus-induced chemokine gene expression in human A549 lung epithelial cells. Virology (2006) 345:96–104. doi: 10.1016/j.virol.2005.09.043
66. Palma JP, Kwon D, Clipston NA, Kim BS. Infection with theiler's murine encephalomyelitis virus directly induces proinflammatory cytokines in primary astrocytes via NF-kappaB activation: potential role for the initiation of demyelinating disease. J Virol (2003) 77:6322–31. doi: 10.1128/JVI.77.11.6322-6331.2003
67. Olson JK. Effect of the innate immune response on development of theiler's murine encephalomyelitis virus-induced demyelinating disease. J Neurovirol (2014) 20:427–36. doi: 10.1007/s13365-014-0262-6
68. Hou W, Kang HS, Kim BS. Th17 cells enhance viral persistence and inhibit T cell cytotoxicity in a model of chronic virus infection. J Exp Med (2009) 206(2):313–28. doi: 10.1084/jem.20082030
69. Inoue A, Koh CS, Yamazaki M, Ichikawa M, Isobe M, Ishihara Y, et al. Anti-adhesion molecule therapy in theiler's murine encephalomyelitis virus-induced demyelinating disease. Int Immunol (1997) 9(12):1837–47. doi: 10.1093/intimm/9.12.1837
70. Olson JK, Girvin AM, Miller SD. Direct activation of innate and antigen-presenting functions of microglia following infection with theiler's virus. J Virol (2001) 75(20):9780–9. doi: 10.1128/JVI.75.20.9780-9789.2001
71. Pozner RG, Berria MI, Negrotto S, Schattner M, Gomez RM. Differential astrocyte response to theiler's murine encephalomyelitis virus infection. Intervirology (2005) 48(5):279–84. doi: 10.1159/000085095
72. Rubio N, Sanz-Rodriguez F, Arevalo MA. Up-regulation of the vascular cell adhesion molecule-1 (VCAM-1) induced by theiler's murine encephalomyelitis virus infection of murine brain astrocytes. Cell Commun Adhes (2010) 17(3):57–68. doi: 10.3109/15419061.2010.507827
73. Kim BS, Jin YH, Meng L, Hou W, Kang HS, Park HS, et al. IL-1 signal affects both protection and pathogenesis of virus-induced chronic CNS demyelinating disease. J Neuroinflamm (2012) 9:217. doi: 10.1186/1742-2094-9-217
74. Kim SJ, Jin YH, Kim BS. Prostaglandin E2 produced following infection with theiler's virus promotes the pathogenesis of demyelinating disease. PloS One (2017) 12(4):e0176406. doi: 10.1371/journal.pone.0176406
75. Agostini L, Martinon F, Burns K, McDermott MF, Hawkins PN, Tschopp J. NALP3 forms an IL-1beta-processing inflammasome with increased activity in muckle-wells autoinflammatory disorder. Immunity (2004) 20:319–25. doi: 10.1016/S1074-7613(04)00046-9
76. Swanson KV, Deng M, Ting JP. The NLRP3 inflammasome: molecular activation and regulation to therapeutics. Nat Rev Immunol (2019) 19(8):477–89. doi: 10.1038/s41577-019-0165-0
77. Hwang SY, Kim JY, Kim KW, Park MK, Moon Y, Kim WU, et al. IL-17 induces production of IL-6 and IL-8 in rheumatoid arthritis synovial fibroblasts via NF-kappaB- and PI3-kinase/Akt-dependent pathways. Arthritis Res Ther (2004) 6:R120–8. doi: 10.1186/ar1038
78. Wang L, Yi T, Kortylewski M, Pardoll DW, Zeng D, Yu H. IL-17 can promote tumor growth through an IL-6-Stat3 signaling pathway. J Exp Med (2009) 206:1457–64. doi: 10.1084/jem.20090207
79. Hou W, Jin YH, Kang HS, Kim BS. Interleukin-6 (IL-6) and IL-17 synergistically promote viral persistence by inhibiting cellular apoptosis and cytotoxic T cell function. J Virol (2014) 88(15):8479–89. doi: 10.1128/JVI.00724-14
80. Weinlich R, Bortoluci KR, Chehab CF, Serezani CH, Ulbrich AG, Peters-Golden M, et al. TLR4/MYD88-dependent, LPS-induced synthesis of PGE2 by macrophages or dendritic cells prevents anti-CD3-mediated CD95L upregulation in T cells. Cell Death Differ (2008) 15:1901–9. doi: 10.1038/cdd.2008.128
81. Jin YH, Hou W, Kang HS, Koh CS, Kim BS. The role of interleukin-6 in the expression of PD-1 and PDL-1 on central nervous system cells following infection with theiler's murine encephalomyelitis virus. J Virol (2013) 87:11538–51. doi: 10.1128/JVI.01967-13
82. Chan LC, Li CW, Xia W, Hsu JM, Lee HH, Cha JH, et al. IL-6/JAK1 pathway drives PD-L1 Y112 phosphorylation to promote cancer immune evasion. J Clin Invest (2019) 129(8):3324–38. doi: 10.1172/JCI126022
83. Ben-Sasson SZ, Hu-Li J, Quiel J, Caucheltaux S, Ratner M, Shapira I, et al. IL-1 acts directly on CD4 T cells to enhance their antigen-driven expansion and differentiation. Proc Natl Acad Sci USA (2009) 106:7119–24. doi: 10.1073/pnas.0902745106
84. Palma JP, Yauch RL, Lang S, Kim BS. Potential role of CD4+ T cell-mediated apoptosis of activated astrocytes in theiler's virus-induced demyelination. J Immunol (1999) 162:6543–51. doi: 10.4049/jimmunol.162.11.6543
85. Crane MA, Yauch R, Dal Canto MC, Kim BS. Effect of immunization with theiler's virus on the course of demyelinating disease. J Neuroimmunol (1993) 45(1-2):67–73. doi: 10.1016/0165-5728(93)90165-U
86. Kang B, Kang HK, Kim BS. Identification of capsid epitopes of theiler's virus recognized by CNS-infiltrating CD4(+) T cells from virus-infected C57BL/6 mice. Virus Res (2005) 108(1-2):57–61. doi: 10.1016/j.virusres.2004.08.001
87. Rodriguez M, Roos RP, McGavern D, Zoecklein L, Pavelko K, Sang H, et al. The CD4-mediated immune response is critical in determining the outcome of infection using theiler's viruses with VP1 capsid protein point mutations. Virology (2000) 275(1):9–19. doi: 10.1006/viro.2000.0493
88. Fiette L, Aubert C, Muller U, Huang S, Aguet M, Brahic M, et al. Theiler's virus infection of 129Sv mice that lack the interferon alpha/beta or interferon gamma receptors. J Exp Med (1995) 181(6):2069–76. doi: 10.1084/jem.181.6.2069
89. Rodriguez M, Zoecklein LJ, Howe CL, Pavelko KD, Gamez JD, Nakane S, et al. Gamma interferon is critical for neuronal viral clearance and protection in a susceptible mouse strain following early intracranial theiler's murine encephalomyelitis virus infection. J Virol (2003) 77(22):12252–65. doi: 10.1128/JVI.77.22.12252-12265.2003
90. Pullen LC, Miller SD, DalCanto MC, van der Meide PH, Kim BS. Alteration in the level of interferon-gamma results in acceleration of theiler's virus-induced demyelinating disease. J Neuroimmunol (1994) 55:143–52. doi: 10.1016/0165-5728(94)90004-3
91. Rodriguez M, Pavelko K, Coffman RL. Gamma interferon is critical for resistance to theiler's virus-induced demyelination. J Virol (1995) 69(11):7286–90. doi: 10.1128/jvi.69.11.7286-7290.1995
92. Harrington LE, Hatton RD, Mangan PR, Turner H, Murphy TL, Murphy KM, et al. Interleukin 17-producing CD4+ effector T cells develop via a lineage distinct from the T helper type 1 and 2 lineages. Nat Immunol (2005) 6(11):1123–32. doi: 10.1038/ni1254
93. Park H, Li Z, Yang XO, Chang SH, Nurieva R, Wang YH, et al. A distinct lineage of CD4 T cells regulates tissue inflammation by producing interleukin 17. Nat Immunol (2005) 6(11):1133–41. doi: 10.1038/ni1261
94. Steinman L. A brief history of T(H)17, the first major revision in the T(H)1/T(H)2 hypothesis of T cell-mediated tissue damage. Nat Med (2007) 13(2):139–45. doi: 10.1038/nm1551
95. Veldhoen M, Hocking RJ, Atkins CJ, Locksley RM, Stockinger B. TGFbeta in the context of an inflammatory cytokine milieu supports de novo differentiation of IL-17-producing T cells. Immunity (2006) 24(2):179–89. doi: 10.1016/j.immuni.2006.01.001
96. Martinez NE, Sato F, Kawai E, Omura S, Takahashi S, Yoh K, et al. Th17-biased RORgammat transgenic mice become susceptible to a viral model for multiple sclerosis. Brain behavior immunit. (2015) 43:86–97. doi: 10.1016/j.bbi.2014.07.008
97. Richards MH, Getts MT, Podojil JR, Jin YH, Kim BS, Miller SD. Virus expanded regulatory T cells control disease severity in the theiler's virus mouse model of MS. J Autoimmun (2011) 36(2):142–54. doi: 10.1016/j.jaut.2010.12.005
98. Zhao J, Zhao J, Perlman S. Virus-specific regulatory T cells ameliorate encephalitis by repressing effector T cell functions from priming to effector stages. PloS Pathog (2014) 10(8):e1004279. doi: 10.1371/journal.ppat.1004279
99. Nawijn MC, Motta AC, Gras R, Shirinbak S, Maazi H, van Oosterhout AJ. TLR-2 activation induces regulatory T cells and long-term suppression of asthma manifestations in mice. PloS One (2013) 8(2):e55307. doi: 10.1371/journal.pone.0055307
100. Yamazaki S, Okada K, Maruyama A, Matsumoto M, Yagita H, Seya T. TLR2-dependent induction of IL-10 and Foxp3+ CD25+ CD4+ regulatory T cells prevents effective anti-tumor immunity induced by Pam2 lipopeptides in vivo. PloS One (2011) 6(4):e18833. doi: 10.1371/journal.pone.0018833
101. Kang HS, Hou W, Kim BS. Rapid expansion of virus-specific CD4+ T cell types in the CNS of susceptible mice infected with theiler’s virus. Int J Mol Sci (2020) 21:7719. doi: 10.3390/ijms21207719
102. Zelenay S, Lopes-Carvalho T, Caramalho I, Moraes-Fontes MF, Rebelo M, Demengeot J. Foxp3+ CD25- CD4 T cells constitute a reservoir of committed regulatory cells that regain CD25 expression upon homeostatic expansion. Proc Natl Acad Sci U.S.A. (2005) 102(11):4091–6. doi: 10.1073/pnas.0408679102
103. Radhakrishnan S, Cabrera R, Schenk EL, Nava-Parada P, Bell MP, Van Keulen VP, et al. Reprogrammed FoxP3+ T regulatory cells become IL-17+ antigen-specific autoimmune effectors in vitro and in vivo. J Immunol (2008) 181(5):3137–47. doi: 10.4049/jimmunol.181.5.3137
104. Komatsu N, Okamoto K, Sawa S, Nakashima T, Oh-hora M, Kodama T, et al. Pathogenic conversion of Foxp3+ T cells into TH17 cells in autoimmune arthritis. Nat Med (2014) 20(1):62–8. doi: 10.1038/nm.3432
105. Stoop JN, Claassen MA, Woltman AM, Binda RS, Kuipers EJ, Janssen HL, et al. Intrahepatic regulatory T cells are phenotypically distinct from their peripheral counterparts in chronic HBV patients. Clin Immunol (2008) 129(3):419–27. doi: 10.1016/j.clim.2008.07.029
106. Bonelli M, Savitskaya A, Steiner CW, Rath E, Smolen JS, Scheinecker C. Phenotypic and functional analysis of CD4+ CD25- Foxp3+ T cells in patients with systemic lupus erythematosus. J Immunol (2009) 182(3):1689–95. doi: 10.4049/jimmunol.182.3.1689
107. Murray PD, McGavern DB, Lin X, Njenga MK, Leibowitz J, Pease LR, et al. Perforin-dependent neurologic injury in a viral model of multiple sclerosis. J Neurosci (1998) 18(18):7306–14. doi: 10.1523/JNEUROSCI.18-18-07306.1998
108. Palma JP, Lee HG, Mohindru M, Kang BS, DalCanto M, Miller SD, et al. Enhanced susceptibility to theiler's virus-induced demyelinating disease in perforin-deficient mice. J Neuroimmunol (2001) 116:125–35. doi: 10.1016/S0165-5728(01)00293-4
109. Pena Rossi C, McAllister A, Fiette L, Brahic M. Theiler's virus infection induces a specific cytotoxic T lymphocyte response. Cell Immunol (1991) 138:341–8. doi: 10.1016/0008-8749(91)90158-8
110. Lindsley MD, Thiemann R, Rodriguez M. Cytotoxic T cells isolated from the central nervous systems of mice infected with theiler’s virus. J Virol (1991) 65:6612–20. doi: 10.1128/jvi.65.12.6612-6620.1991
111. Rivera-Quinones C, McGovern D, Schmeizer JD, Hunter SF, Low PA, Rodriguez M. Absence of neurological deficits following extensive demyelination in a class I-deficient murine model of multiple sclerosis. Nat Med (1998) 4:187–93. doi: 10.1038/nm0298-187
112. Borrow P, Tonks P, Welsh CJ, Nash AA. The role of CD8+T cells in the acute and chronic phases of theiler's murine encephalomyelitis virus-induced disease in mice. J Gen Virol (1992) 73:1861–5. doi: 10.1099/0022-1317-73-7-1861
113. Myoung J, Hou W, Kang B, Lyman MA, Kang JA, Kim BS. The immunodominant CD8+ T cell epitope region of theiler's virus in resistant C57BL/6 mice is critical for anti-viral immune responses, viral persistence, and binding to the host cells. Virology (2007) 360:159–71. doi: 10.1016/j.virol.2006.09.045
114. Begolka WS, Haynes LM, Olson JK, Padilla J, Neville KL, DalCanto M, et al. CD8-deficient SJL mice display enhanced susceptibility to theiler's virus infection and increased demyelinating pathology. J Neurovirol (2001) 7:409–20. doi: 10.1080/135502801753170264
115. Santiago-Raber ML, Baudino L, Izul S. Emerging roles of TLR7 and TLR9 in murine SLE. J Autoimmun (2009) 33:231–8. doi: 10.1016/j.jaut.2009.10.001
116. Mills KH. TLR-dependent T cell activation in autoimmunity. Nat Rev Immunol (2011) 11:807–22. doi: 10.1038/nri3095
117. Kiefer K, Oropallo MA, Cancro MP, Marshak-Rothstein A. Role of type I interferons in the activation of autoreactive b cells. Immunol Cell Biol (2012) 90:498–504. doi: 10.1038/icb.2012.10
118. Kishimoto T. Interleukin-6: from basic science to medicine–40 years in immunology. Annu Rev Immunol (2005) 23:1–21. doi: 10.1146/annurev.immunol.23.021704.115806
119. Kuno K, Matsushima K. The IL-1 receptor signaling pathway. J Leukoc Biol (1994) 56:542–7. doi: 10.1002/jlb.56.5.542
120. Cash E, Bandeira A, Chirinian S, Brahic M. Characterization of b lymphocytes present in the demyelinating lesions induced by theiler's virus. J Immunol (1989) 143(3):984–8. doi: 10.4049/jimmunol.143.3.984
121. Roos RP, Nalefski EA, Nitayaphan S, Variakojis R, Singh KK. An isoelectric focusing overlay study of the humoral immune response in theiler's virus demyelinating disease. JNeuroimmunol (1987) 13:305–14. doi: 10.1016/0165-5728(87)90066-X
122. Zurbriggen A, Fujinami RS. A neutralization-resistant theiler's virus variant produces an altered disease pattern in the mouse central nervous system. J Virol (1989) 63:1505–13. doi: 10.1128/jvi.63.4.1505-1513.1989
123. Pachner AR, Li L, Lagunoff D. Plasma cells in the central nervous system in the theiler's virus model of multiple sclerosis. J Neuroimmunol (2011) 232(1-2):35–40. doi: 10.1016/j.jneuroim.2010.09.026
124. Cameron K, Zhang X, Seal B, Rodriguez M, Njenga MK. Antigens to viral capsid and non-capsid proteins are present in brain tissues and antibodies in sera of theiler's virus-infected mice. J Virol Methods (2001) 91(1):11–9. doi: 10.1016/S0166-0934(00)00246-9
125. Kang BS, Palma JP, Lyman MA, Dal Canto M, Kim BS. Antibody response is required for protection from theiler's virus-induced encephalitis in C57BL/6 mice in the absence of CD8(+) T cells. Virology (2005) 340(1):84–94. doi: 10.1016/j.virol.2005.06.028
126. Kim BS, Choe YK, Crane MA, Jue CR. Identification and localization of a limited number of predominant conformation-independent antibody epitopes of theiler's murine encephalomyelitus virus. Immunol Lett (1992) 31(2):199–205. doi: 10.1016/0165-2478(92)90146-F
127. Gilli F, Li L, Campbell SJ, Anthony DC, Pachner AR. The effect of b-cell depletion in the theiler's model of multiple sclerosis. J Neurol Sci (2015) 359(1-2):40–7. doi: 10.1016/j.jns.2015.10.012
128. Inoue A, Choe YK, Kim BS. Analysis of antibody responses to predominant linear epitopes of theiler's murine encephalomyelitis virus. J Virol (1994) 68:3324–33. doi: 10.1128/jvi.68.5.3324-3333.1994
129. Delhaye S, Paul S, Blakqori G, Minet M, Weber F, Staeheli P, et al. Neurons produce type I interferon during viral encephalitis. Proc Natl Acad Sci U.S.A. (2006) 103(20):7835–40. doi: 10.1073/pnas.0602460103
130. Rubio N, Palomo M, Alcami A. Interferon-alpha/beta genes are up-regulated in murine brain astrocytes after infection with theiler's murine encephalomyelitis virus. J Interferon Cytokine Res (2010) 30(4):253–62. doi: 10.1089/jir.2009.0050
131. Jin YH, Hou W, Kim SJ, Fuller AC, Kang B, Goings G, et al. Type I interferon signals control theiler's virus infection site, cellular infiltration and T cell stimulation in the CNS. J Neuroimmunol (2010) 226:27–37. doi: 10.1016/j.jneuroim.2010.05.028
132. Jelachich ML, Lipyon HL. Restricted theiler's murine encephalomyelitis virus infection in murine macrophages induces apoptosis. J Gen Virol (1999) 80:1701–5. doi: 10.1099/0022-1317-80-7-1701
133. Yang CH, Murti A, Pfeffer SR, Basu L, Kim JG, Pfeffe LM. IFNα/β promotes cell survival by activating NF-κB. Proc Nat Acad Sci USA (2000) 97:13631–6. doi: 10.1073/pnas.250477397
134. Daher KA, Samuel CE. Mechanism of interferon action. differential effect of interferon on the synthesis of simian virus 40 and reovirus polypeptides in monkey kidney cells. Virology (1982) 117:379–90. doi: 10.1016/0042-6822(82)90477-9
135. Atreya PL, Kulkami S. Respiratory syncytial virus strain A2 is resistant to the antiviral effects of type I interferons and human MxA. Virology (1999) 261:227–41. doi: 10.1006/viro.1999.9835
136. Ho LJ, Hung LF, Weng CY, Wu WL, Chou P, Lin YL, et al. Dengue virus type 2 antagonizes IFN-alpha but not IFN-gamma antiviral effect via down-regulating Tyk2-STAT signaling in the human dendritic cell. J Immunol (2005) 174:8163–72. doi: 10.4049/jimmunol.174.12.8163
137. Jelachich ML, Bandyopadhyay P, Blum K, Lipton HL. Theiler's virus growth in murine macrophage cell lines depends on the state of differentiation. Virology (1995) 209:437–44. doi: 10.1006/viro.1995.1276
138. Turrin NP. Central nervous system toll-like receptor expression in response to theiler's murine encephalomyelitis virus-induced demyelination disease in resistant and susceptible mouse strains. Virol J (2008) 5:154. doi: 10.1186/1743-422X-5-154
139. Akira S, Hemmi H. Recognition of pathogen-associated molecular patterns by TLR family. Immunol Lett (2003) 85:85–95. doi: 10.1016/S0165-2478(02)00228-6
140. Diebold SS, Kaisho T, Hemmi H, Akira S, Sousa Re. Innate antiviral responses by means of TLR7-mediated recognition of single-stranded RNA. Science (2004) 303:1529–31. doi: 10.1126/science.1093616
141. Kawai T, Akira S. Toll-like receptors and their crosstalk with other innate receptors in infection and immunity. Immunity (2011) 34:637–50. doi: 10.1016/j.immuni.2011.05.006
142. Mattson MP, Meffert MK. Roles for NF-kappaB in nerve cell survival, plasticity, and disease. Cell Deth Difer (2006) 13(5):852–60. doi: 10.1038/sj.cdd.4401837
143. Sarnico I. NF-kappaB dimers in the regulation of neuronal survival. Int Rev Neurobiol (2009) 85:351–62. doi: 10.1016/S0074-7742(09)85024-1
144. Hsieh CY, Chen CA, Huang CY, Chang MC, Lee CN, Su YN, et al. IL-6-encoding tumor antigen generates potent cancer immunotherapy through antigen processing and anti-apoptotic pathways. Mol Ther (2007) 15(10):1890–7. doi: 10.1038/sj.mt.6300243
145. Jourdan M, Veyrune JL, De Vos J, Redal N, Couderc G, Klein B. A major role for mcl-1 antiapoptotic protein in the IL-6-induced survival of human myeloma cells. Oncogene (2003) 22(19):2950–9. doi: 10.1038/sj.onc.1206423
146. Asadzadeh Z, Mohammadi H, Safarzadeh E, Hemmatzadeh M, Mahdian-Shakib A, Jadidi-Niaragh F, et al. The paradox of Th17 cell functions in tumor immunity. Cell Immunol (2017) 322:15–25. doi: 10.1016/j.cellimm.2017.10.015
147. Varikuti S, Oghumu S, Elbaz M, Volpedo G, Ahirwar DK, Alarcon PC, et al. STAT1 gene deficient mice develop accelerated breast cancer growth and metastasis which is reduced by IL-17 blockade. Oncoimmunol (2017) 6:e1361088. doi: 10.1080/2162402X.2017.1361088
148. Kagi D, Vignaux F, Ledermann B, Burki K, Depraetere V, Nagata S, et al. Fas and perforin pathways as major mechanisms of T cell-mediated cytotoxicity. Science (1994) 265:528–30. doi: 10.1126/science.7518614
149. Lowin B, Hahne M, Mattmann C, Tschopp J. Cytolytic T-cell cytotoxicity is mediated through perforin and fas lytic pathways. Proc Natl Acad Sci USA (1994) 91:11571–5. doi: 10.1073/pnas.91.24.11571
150. Harty JT, Tvinnereim AR, White DW. CD8+ T cell effector mechanisms in resistance to infection. Ann Rev Immunol (2000) 18:275–308. doi: 10.1146/annurev.immunol.18.1.275
151. Jellison ER, Kim SK, Welsh RM. Cutting edge: MHC class II-restricted killing in vivo during viral infection. J Immunol (2005) 174:614–8. doi: 10.4049/jimmunol.174.2.614
152. Kim BS. Excessive innate immunity steers pathogenic adaptive immunity in the development of theiler's virus-induced demyelinating disease. (2021) 22(10):5254. doi: 10.3390/ijms22105254
153. Khanmohammadi S, Rezaei N. Role of toll-like receptors in the pathogenesis of COVID-19. J Med Virol (2021) 93:2735–9. doi: 10.1002/jmv.26826
154. Mabrey FL, Morrell ED, Wurfel MM. TLRs in COVID-19: how they drive immunopathology and the rationale for modulation. Innate Immun (2021) 27:503–13. doi: 10.1177/17534259211051364
155. Manik M, Singh RK. Role of toll-like receptors in modulation of cytokine storm signaling in SARS-CoV-2-induced COVID-19. J Med Virol (2022) 94:869–77. doi: 10.1002/jmv.27405
156. Chang SE, Feng A, Meng W, Apostolidis SA. New-onset IgG autoantibodies in hospitalized patients with COVID-19. Nat Commun (2021) 12(1):5417. doi: 10.1038/s41467-021-25509-3
157. Fujinami RS, Zurbriggen A, Powell HC. Monoclonal antibody defines determinant between theiler's virus and lipid-like structures. J Neuroimmunol (1988) 20:25–32. doi: 10.1016/0165-5728(88)90110-5
158. Tsunoda I, Kuang LQ, Kobayashi-Warren M, Fujinami RS. Central nervous system pathology caused by autoreactive CD8+ T-cell clones following virus infection. J Virol (2005) 79:14640–6. doi: 10.1128/JVI.79.23.14640-14646.2005
159. Myoung J, Kang HS, Hou W, Meng L, Dal Canto MC, Kim BS. Epitope-specific CD8+ T cells play a differential pathogenic role in the development of a viral disease model for multiple sclerosis. J Virol (2012) 86:13717–28. doi: 10.1128/JVI.01733-12
160. Patole PS, Grone HJ, Segerer S, Ciubar R, Belemezova E, Henger A, et al. Viral double-stranded RNA aggravates lupus nephritis through toll-like receptor 3 on glomerular mesangial cells and antigen-presenting cells. J Am Soc Nephrol (2005) 16:1326–38. doi: 10.1681/ASN.2004100820
161. Pisitkun P, Deane JA, Diffilippantonio MJ, Tarasenko T, Satterthwaite AB, Bolland S. Autoreactive b cell responses to RNA-related antigens due to TLR7 gene duplication. Science (2006) 312:1669–72. doi: 10.1126/science.1124978
162. Green NM, Marshak-Rothstein A. Toll-like receptor driven b cell activation in the induction of systemic autoimmunity. Semin Immunol (2011) 23:106–12. doi: 10.1016/j.smim.2011.01.016
163. Myoung J, Bahk Yl, Kang HS, Dal Canto MC, Kim BS. Anti-capsid immunity level, not viral persistence level, correlates with the progression of theiler's virus-induced demyelinating disease in viral P1-transgenic mice. J Virol (2008) 82:5606–17. doi: 10.1128/JVI.02442-07
164. Drescher KM, Pease LR, Rodriguez M. Antiviral immune responses modulate the nature of central nervous system (CNS) disease in a murine model of multiple sclerosis. Immunol Rev (1997) 159:177–93. doi: 10.1111/j.1600-065X.1997.tb01015.x
165. Asakura K, Miller DJ, Pease LR, Rodriguez M. Targeting of IgMkappa antibodies to oligodendrocytes promotes CNS remyelination. J Neurosci (1998) 18:7700–8. doi: 10.1523/JNEUROSCI.18-19-07700.1998
166. Hirota K, Hashimoto M, Yoshitomi H, Tanaka S, Nomura T, Yamaguchi T, et al. T Cell self-reactivity forms a cytokine milieu for spontaneous development of IL-17+ Th cells that cause autoimmune arthritis. J Exp Med (2007) 204:41–7. doi: 10.1084/jem.20062259
167. Furuzawa-Carballeda J, Icaza-Chavez ME, Aguilar-Leon D, Uribe-Uribe N, Nunez-Pompa MC, Trigos-Diaz A, et al. Is the sars-CoV-2 virus a possible trigger agent for the development of achalasia? Neurogastroenterol Motil (2023) 35(3):e14502. doi: 10.1101/2022.09.19.22280068
Keywords: TLRs, chronic viral infection, inflammation, apoptosis, autoimmunity
Citation: Kim BS (2023) Critical role of TLR activation in viral replication, persistence, and pathogenicity of Theiler’s virus. Front. Immunol. 14:1167972. doi: 10.3389/fimmu.2023.1167972
Received: 17 February 2023; Accepted: 11 April 2023;
Published: 20 April 2023.
Edited by:
Oliver Planz, University of Tübingen, GermanyReviewed by:
Sam Basta, Queen’s University, CanadaKaren Bohmwald, Pontificia Universidad Católica de Chile, Chile
Copyright © 2023 Kim. This is an open-access article distributed under the terms of the Creative Commons Attribution License (CC BY). The use, distribution or reproduction in other forums is permitted, provided the original author(s) and the copyright owner(s) are credited and that the original publication in this journal is cited, in accordance with accepted academic practice. No use, distribution or reproduction is permitted which does not comply with these terms.
*Correspondence: Byung S. Kim, YnNraW1Abm9ydGh3ZXN0ZXJuLmVkdQ==