- 1Department of Internal Medicine and Radboud Center for Infectious Diseases, Radboud University Medical Center, Nijmegen, Netherlands
- 2Radboud Institute for Molecular Life Sciences, Radboud University Medical Center, Nijmegen, Netherlands
- 3Cantabria Labs, Innovation and Development, Madrid, Spain
- 4Servicio de Dermatología, Hospital Universitario Ramon y Cajal, IRYCIS, Madrid, Spain
The proper functioning of the immune system depends on an appropriate balance between pro-inflammation and anti-inflammation. When the balance is disrupted and the system is excessively biased towards inflammation, immune responses cannot return within the normal range, which favors the onset of diseases of autoimmune or inflammatory nature. In this scenario, it is fundamental to find new compounds that can help restore this balance and contribute to the normal functioning of the immune system in humans. Here, we show the properties of a fungal compound with a strong safety profile in humans, AM3, as an immunomodulatory molecule to decrease excessive cytokine production in human cells. Our results present that AM3 treatment of human peripheral blood mononuclear cells and monocytes decreased their pro-inflammatory cytokine secretion following the challenge with bacterial lipopolysaccharide. Additionally, AM3 skewed the differentiation profile of human monocytes to macrophages towards a non-inflammatory phenotype without inducing tolerance, meaning these cells kept their capacity to respond to different stimuli. These effects were similar in young and elderly individuals. Thus, the fungal compound, AM3 may help reduce excessive immune activation in inflammatory conditions and keep the immune responses within a normal homeostatic range, regardless of the age of the individual.
Introduction
Inflammation is a key component of immune responses. It promotes the mobilization of immune cells to the sites of infection or damage to eliminate the triggering factor, repair tissue damage, and restore homeostasis in the organism (1). Cytokines are molecules responsible for long-range intercellular communication and play a fundamental role as mediators during inflammatory processes. The actions of pro-inflammatory cytokines, such as interleukin (IL)-1, IL-6, or tumor necrosis factor-alpha (TNFα), need to be balanced with anti-inflammatory cytokines, such as IL-10 or IL-1 receptor antagonist (IL-1Ra). The latter contributes to the resolution of inflammation and the suppression of excessive immune activation, thus limiting the duration and the extent of the inflammatory responses (2). However, when there is an imbalance between these processes and the system is excessively biased towards inflammation, the immune responses cannot return within the normal range. This increases the risk of autoimmune and inflammatory conditions, such as cardiovascular diseases, inflammatory bowel disease, type II diabetes, or rheumatoid arthritis, among many others (3, 4). The globalization of the Western-type diet, a sedentary lifestyle, and the increasingly aging population led to a rise in the incidence and prevalence of diseases with an inflammatory component at an alarming rate. At present, inflammatory diseases are the leading cause of morbidity and mortality worldwide (5). This situation drives researchers from public and private institutions to find new compounds to tackle the inflammatory origin of these diseases.
Among the several available options, compounds of fungal origin are considered one of the most promising sources for molecules with immunomodulatory properties (6). In this respect, molecules derived from the cell wall of different species of fungi, such as β-glucans, zymosan, or mannans, are in the spotlight of many research projects for their possible use as (prophylactic) therapy against different types of diseases (7).
The active principle of Immunoferon, AM3, is a glycoconjugate compound renowned for its immunomodulatory effects (8, 9). This compound is produced by combining a naturally phosphorylated alpha glucomannan derived from the cell wall of a specific Candida utilis strain and soybean seed storage proteins. The polysaccharide segment is characterized by phosphoglucomannan-type α-glucan (roughly 150 kDa) with recurring polysaccharide linear chains comprised of α(1→6) and α(1→2) linkages among the mannose and glucose residues. Throughout its commercialization spanning more than four decades, the AM3 compound has been meticulously characterized at different levels, covering aspects such as molecular structure (10), bioavailability (11), metabolic processing (12), and clinical implications.
Multiple research works have shown the strong immunomodulatory properties of different molecules of fungal origin in innate immune cells, namely in monocytes/macrophages. In this regard, β-glucan derived from Candida albicans induces innate immune memory in monocytes and increases the production of inflammatory cytokines in response to a secondary stimulus (13, 14). Besides β-glucan, other fungal-derived compounds such as curdlan, yeast-b, and zymosan re-polarize macrophages towards a pro-inflammatory phenotype, with enhanced expression of CCR7, ICAM1, and CD80, and secretion of TNF-α and IL-6 (15).
We then hypothesized that AM3 may exert similar immunomodulatory activity on innate immunity cells. In this study, we describe how AM3 decreases exacerbated cytokine production by human monocytes. We show that AM3 skews the differentiation profile of macrophages towards a phenotype without pro-inflammatory features. Additionally, we show that the cell response to AM3 pre-treatment is not associated with the acquisition of immune tolerance.
Methods
Lactate dehydrogenase assay
The concentrations of AM3 used in this study were optimized in LDH assay (Promega, cat #G1780) to minimize cytotoxicity. Typically, adherent human peripheral blood mononuclear cells (PBMCs) obtained from healthy donors (Sanquin Blood Bank, Nijmegen, the Netherlands) were stimulated with different doses of AM3 (50 μg/mL, 10 μg/mL, 1 μg/mL and 0.1 μg/mL) for 24 hours. The AM3 compound (dissolved in water and sterilized by microfiltration through 0.22 µm cellulose acetate filters) was kindly provided by Cantabria Labs, Madrid, Spain. Collected supernatants were immediately used to perform the assay according to the manufacturer’s instructions.
Different doses of AM3 in vitro tolerance and innate immune memory experiments
After written informed consent, buffy coats were obtained from healthy donors (Sanquin Blood Bank, Nijmegen, the Netherlands). Samples were anonymized to safeguard donor privacy. By differential density centrifugation, PBMC isolation was performed using Ficoll‐Paque (GE Healthcare, cat # GE17-1440-02). Cells were resuspended in Dutch modified RPMI 1640 medium (Invitrogen, cat #22409031) containing 50 µg/mL Gentamicin (Centrafarm), 1 mM Sodium-Pyruvate (Thermo Fisher Scientific, cat #11360088), 2 mM Glutamax (Thermo Fisher Scientific, cat #35050087) [base medium] and counted via Sysmex XN-450.
Tolerance experiments
PBMCs (4x105 cells/well) were seeded in sterile flat-bottom 96-well tissue culture-treated plates (VWR, cat #734-2184) in the base medium and rested for an hour in a 37°C incubator supplied with 5% CO2 for monocytes to adhere. The cells were washed with warm PBS to eliminate excessive leukocytes and non-adherent cells in the wells. RPMI medium (used as a control condition), AM3 (0.1-50 μg/mL) or LPS (0.5 ng/mL, serotype O55:B5; Sigma-Aldrich, cat #L2880, further purified as described in (16)) was added on to monocytes (Day 0) and incubated for 24 hours in base medium supplemented with 10% human pooled serum. On Day 1, the cells were washed again with warm PBS and, thereafter, RPMI was replenished in the control group, while LPS was added to the AM3 group, and AM3 to the LPS group, followed by additional 24-hours incubation. At 48 hours (Day 2), cells were washed with warm PBS, resuspended in base medium with 10% human pooled serum, and incubated at 37°C, 5% CO2 for additional 4 days. On Day 6, exhausted medium was discarded and cells were restimulated with LPS (10 ng/mL) for the final 24 hours of the culture. Supernatants for cytokine measurements were collected on Days 1, 2, 6, and 7 and stored at -20°C. Secreted pro-inflammatory cytokines TNF-α, IL-6, and IL-1β and anti-inflammatory cytokines IL-10 and IL-1Ra levels were measured from supernatants after LPS restimulation using R&D Systems ELISA kits, following the manufacturer’s instructions. The results are presented in Supplementary Figure 2.
Innate immune memory experiments
PBMCs (5x105 cells/well) were seeded in wells and adherent cells were enriched as described above. RPMI medium (negative control), AM3 (0.1-50 μg/mL), β-glucan (BG, 1 μg/mL), and Bacillus Calmette-Guérin (BCG 5 μg/mL M. bovis, BCG Denmark, AJ Vaccines) were added to the cells in base medium supplemented with 10% human pooled serum. After 24 hours (Day 1), wells were washed to remove any primary stimuli and fresh base medium supplemented with 10% human pooled serum was replenished to allow cells to eventually differentiate further (17). The cells were restimulated with LPS (10 ng/mL) as a non-related secondary stimulus on Day 6. After 24 hours of restimulation, supernatants were collected and stored at -20°C. Secreted pro-inflammatory cytokines TNF-α, IL-6, and IL-1β levels were measured from supernatants after restimulation using R&D Systems ELISA kits, following the manufacturer’s instructions. The results are presented in Supplementary Figure 3.
Study approvals
Healthy donor samples were obtained from the Sanquin Blood Bank (Nijmegen, the Netherlands) after written informed consent. Sanquin Blood Bank is a commercial provider of blood products; as such, anonymized samples came without any specific ethics reference number or code. PBMCs from elderly and young donors (Table 1) were obtained from the clinical trials BCG Booster (NL58219.091.16) and BCG PRIME (NCT04537663). These samples were used to perform in vitro tolerance experiments to analyze difference in responsiveness between the PBMCs from elderly and young donors. The details of the procedure are as depicted above in the section “Different doses of AM3 in vitro tolerance and innate immune memory experiments”.
Macrophage differentiation experiments
The samples were obtained from buffy coats, as explained above. Cells were resuspended in the base medium and counted via Sysmex XN-450. The cells (30x106 for the AM3 group and 15x106 for M-CSF and GM-CSF groups) were seeded into 60 mm tissue culture dishes (Corning, cat #430166) and rested for an hour in a 37°C incubator supplied with 5% CO2 to ensure monocyte adherence. Afterwards, non-adherent cells were removed by washing with warm PBS, and then, differentiation media were added to the cells, respectively as AM3 (10 μg/mL), M-CSF (10 ng/mL), and GM-CSF (10 ng/mL). After 24 hours, the cells of all the conditions were washed to mimic the innate memory/tolerance condition, and a base medium supplemented with 10% human pooled serum was added to the cells. On Day 3, culture media was removed and replenished with base medium supplemented with 10% human pooled serum with the corresponding differentiation factor, for M-CSF and GM-CSF conditions. On Day 6, supernatants were discarded, and cells were detached with cold PBS containing 5 mM EDTA, by gentle pipetting (6-10 times) around the dish surface before harvesting cell suspensions. This procedure was repeated three times to maximize cell-recovery as needed in flow cytometric analysis. Afterwards, cells were counted with a hematocytometer.
Flow cytometry staining and analysis
Cells were resuspended in PBS for live/dead staining with 1:1000 diluted Fixable Viability Stain (FVS) 620-ECD (BD Biosciences, cat #564996) for 15 minutes at room temperature (RT). After a wash to remove the live/dead staining solution, the cells were resuspended in a 50 μL antibody panel cocktail (Table 2) prepared in PBS with 1% BSA and Brilliant Stain Buffer (BD Biosciences, cat #563794), and incubated for 45 minutes in the dark at RT. Thereafter, the antibody excess was washed and the cells were analyzed on the Cytoflex flow cytometer (Beckman Coulter).
Metabolic assay
Lactate was measured from 24-hour, 48 hours, and 6 day supernatants of the tolerance experiment through a commercial fluorimetric assay. A standard curve was established by serially diluting 40 μM Na-L-Lactate (Sigma-Aldrich, cat# L7022). The samples and standards were placed into a 96-well flat-bottom black assay plate (Corning, cat# CLS3925). Afterwards, the reaction mix containing 100μM Amplex Red (Invitrogen, cat# A12222), 0.2 U/mL Horseradish Peroxidase (Thermo Scientific, cat# 31490), 2 U/mL Lactate oxidase (Sigma-Aldrich, cat# L9795) in PBS was added onto sufficiently diluted samples and standards. After 20 minutes of incubation at RT on a shaker, the fluorescence was measured with 530±25 and 590±35 filters (Biotek, SynergyHTX).
Statistical analysis
GraphPad Prism 8 was used for all the statistical analyses. Three or more paired groups were compared using the Friedman test with Dunn’s multiple comparisons. Outcomes between two independent groups were analyzed by the Mann-Whitnney test used. A P-value of less than or equal to 0.05 was considered statistically significant. *: p ≤ 0.05, **: p ≤ 0.01, ***: p ≤ 0.001, ****: p ≤ 0.0001.
Results
AM3 decreases exacerbated pro-inflammatory cytokine secretion in human cells
We first sought to establish the ideal concentration of AM3 in our model, so we evaluated the cytotoxicity caused by four different concentrations of AM3 (50, 10, 1 and 0.1 μg/mL) to ensure we covered the full spectrum of activity. The LDH assay showed no cytotoxicity after 24 hours in any of the AM3 concentrations used (Figure S1); therefore, we decided to continue the experiments with the 10 µg/ml concentration leading to the lowest LDH production.
Next, we investigated the potential effects of AM3 on the cytokine production capacity of human PBMCs. Different from lipopolysaccharide (LPS), which induces robust cytokine production by human cells (18, 19), AM3 did not significantly increase pro-inflammatory (IL-1β, IL-6, and TNFα) or the anti-inflammatory (IL-1Ra or IL-10) cytokines, compared to control group (RPMI) (Figures 1A, B). Apart from non-significant trends in IL-6 production, there was no apparent change in cytokine production upon direct exposure to AM3, thus we tested whether AM3 treatment could modulate cytokine response in combination with (i.e., before and after) LPS stimulation. As expected, the stimulation with LPS strongly induced the production of the pro-inflammatory cytokines IL-1β, IL-6, and TNFα, and also that of anti-inflammatory IL-1Ra or IL-10, which are concomitantly produced as compensation to avoid excessive inflammation (Figure 1B). However, pre-treatment of human PBMCs with AM3, 24 hours prior to stimulation with LPS (AM3-LPS group), considerably mitigated the LPS-induced production of cytokines (Figures 1B; S2B). This suggests that AM3 exerts an immunomodulatory effect on LPS-stimulated cells. However, this decrease was significant only in the case of IL-1β and IL-1Ra. Upon this observation, we checked the ratio between IL-1β/IL-1Ra, further confirming our observations on AM3 immunomodulatory effects as all AM3 groups yielded in a ratio smaller than 1 (Figure S2A). Lower cytokine responses were observed upon AM3 treatment after LPS stimulation (LPS-AM3 group), however this was not dissimilar from the media restimulation controls (Figure S2B).
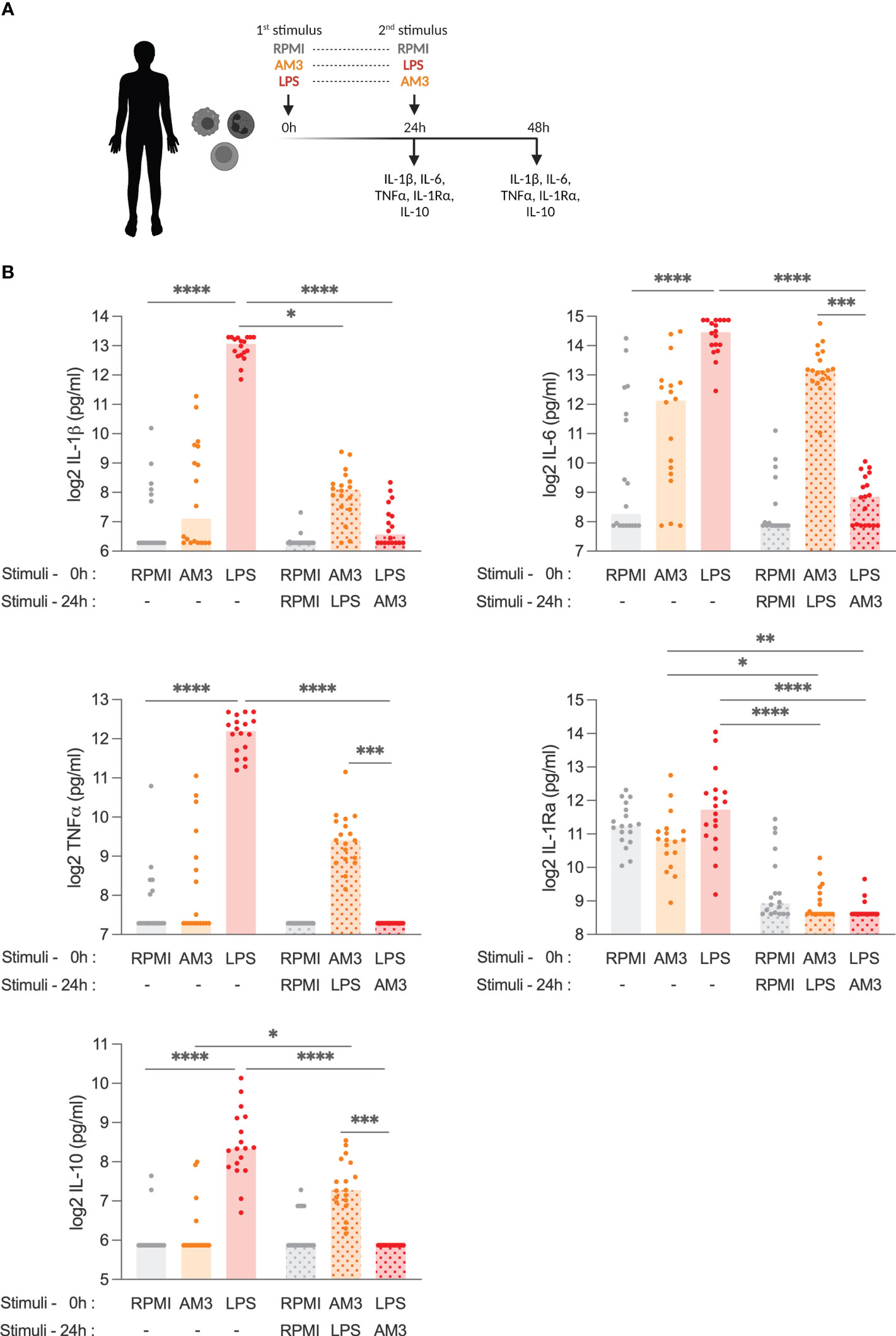
Figure 1 AM3 treatment limits LPS-driven cytokine response in human PBMCs (A) Experimental design: adherent PBMCs were stimulated with primary stimuli and supernatants were collected for cytokine detection at 24h, then cells were washed with control medium to remove any residue of the primary stimulation before secondary stimuli were added to the cultures following which the supernatants collected after an additional 24h (48h in total). (B) Cytokine production from PBMCs at 24 hours and at 48 hours after stimulation with medium control (RPMI), AM3 and LPS, eventually combined as by (A) in a number of healthy donors (n=18). Friedman test with Dunn’s multiple comparison was applied to compare experimental groups, *p<0.05, **p<0.01, ***p<0.001, ****p<0.0001. Bars without pattern: cytokine levels measured 24 hours after first stimulation, Patterned bars: cytokine levels measured 48 hours after first stimulation. Values for the groups RPMI (1st) + AM3 (2nd), RPMI (1st) + LPS (2nd), AM3 (1st) + RPMI (2nd), and LPS (1st) + RPMI (2nd) are presented in Figure S2B.
Conversely, there was no significant change in cytokine production when combined treatments (AM3-LPS and LPS-AM3) were compared to the AM3 group, except for IL-1Ra, which decreased evidently in any combination treatments. Additionally, different doses of AM3 in these settings did not yield to similar significant results (Figure S3).
AM3 is similarly effective in the cells from both young and elderly individuals
Elderly individuals tend to be vulnerable to immune-related diseases and syndromes due to imbalanced immune responses during aging (20). One of the well-known reasons is that elderly people present a dysfunctional adaptive immune response (21), making them less responsive to vaccination and more susceptible to infections. Another reason is that elderly people tend to develop chronic inflammation (so-called “inflammaging”) (22).
In this context, we hypothesized that the immunomodulatory effects of AM3 may help control dysregulated responses seen in aged individuals. Accordingly, we performed similar experiments comparing the responses to AM3 and LPS stimulation in cells obtained from young and aged (>60 years old) donors. Interestingly, we observed that when exposed to LPS, cells obtained from aged individuals produced significantly higher amounts of IL-6, TNFα, IL-1Ra, and IL-10 than those from younger donors (Figure 2). Treatment of the cells with AM3 prior to the challenge with LPS (AM3-LPS group) significantly decreased the induction of any of the cytokines studied. Furthermore, AM3 treatment maintained low cytokine responses after LPS exposure and cytokine levels equalized between the two age groups after AM3 treatment (LPS-AM3), except for IL-6 and IL-1β.
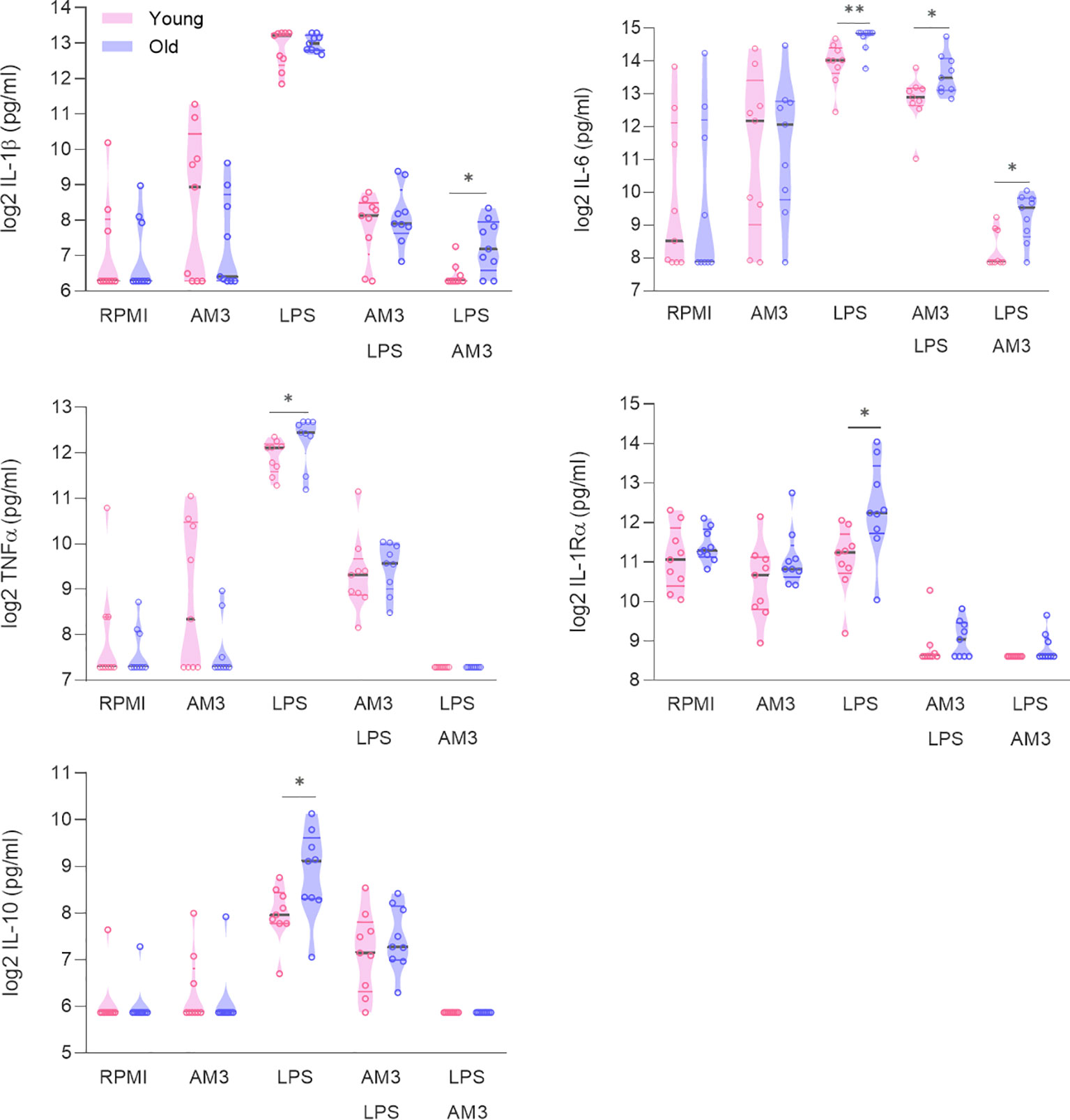
Figure 2 AM3 is similarly effective in cells from both young and elderly individuals. Cytokine production measured in supernatants from PBMCs after 24 hours and after 48 hours following primary stimulation (RPMI, AM3 and LPS) and combination treatments, in cultures derived from young (n=9, pink data points) and elderly (n=9, purple data points) healthy donors. Mann-Whitney test used to compare elderly and young individuals. *p<0.05, **p<0.01. The gray line depicts the median whereas colored lines represent the borders of interquartile range.
AM3 skews the differentiation process of monocytes towards a non-inflammatory phenotype
The effects of AM3 on human PBMCs presented so far suggest an anti-inflammatory effect that limits LPS-induced cytokine production, regardless of the donor’s age. These results led to the hypothesis that AM3 might play a role in skewing the differentiation of monocytes to macrophages towards an anti-inflammatory phenotype. To test this, we obtained monocytes from the peripheral blood of healthy donors and subjected them to well-established monocyte-to-macrophage differentiation protocols in the presence of granulocyte-macrophage colony-stimulating factor (GM-CSF) and macrophage colony-stimulating factor (M-CSF), respectively promoting pro-inflammatory and anti-inflammatory macrophages, in parallel to AM3. We then assessed phenotypic characteristics in these cultures and found that AM3-macrophages resembled the M-CSF counterparts, showing a fibroblast-like, elongated shape with lower cell density. In contrast, macrophages in the GM-CSF group were larger, showing more cytoplasmic granules and bigger nuclei (Figure 3A). This observation is in accordance with the expression of the pro-inflammatory macrophage markers CD80 and CD86 (23), measured by flow cytometry, the levels of which were considerably higher in GM-CSF-macrophages rather than in M-CSF and AM3-macrophages (Figure 3B).
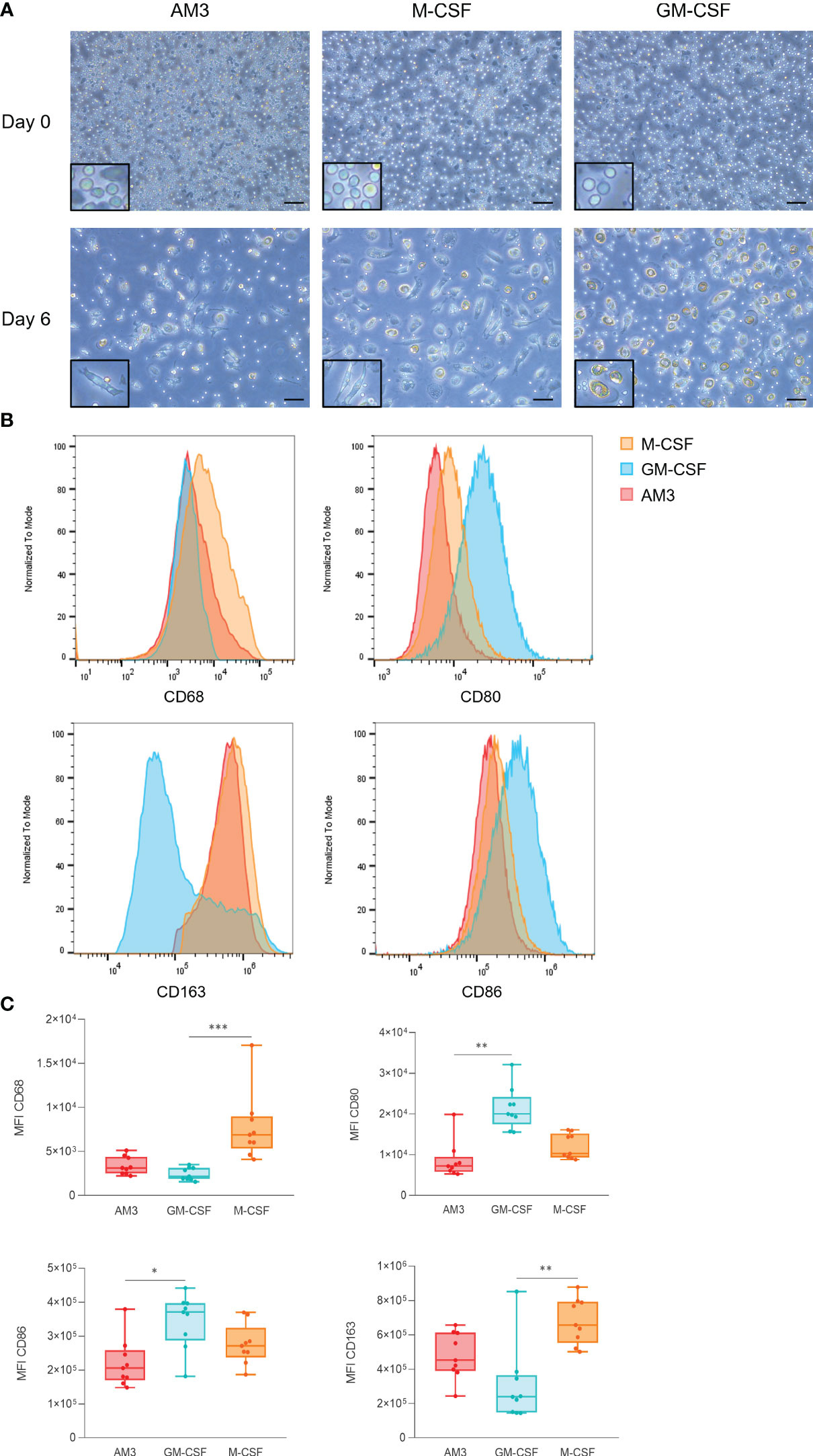
Figure 3 AM3 treatment of human monocytes skews the differentiation process towards an anti-inflammatory phenotype (A) Microscope images of cells at day 0 and day 6 of the differentiation process, magnification: 20X, scale bar: 100 µM. The areas highlighted in a black rectangle show an area of the same image with a larger magnification to better appreciate the cell morphology. (B) Flow cytometry analysis of macrophage markers at day 6 after exposure to AM3, M-CSF or GM-CSF. (C) Comparisons of MFIs by marker (CD68, CD80, CD86, and CD163) are depicted on the charts. n=9, Friedman test with Dunn’s multiple comparisons. *p<0.05, **p<0.01, ***p<0.001. For (B) and (C) all cells were gated as CD45+ CD206+ live cells.
On the other hand, the anti-inflammatory macrophage marker CD163 (23) was expressed higher in M-CSF and AM3 macrophages than GM-CSF counterparts (Figures 3B, C). CD68, a general macrophage marker, was used as an internal control to validate the assay. Overall, the phenotype of macrophages differentiated from AM3-stimulated cells resembled that of M-CSF-differentiated (anti-inflammatory) more than GM-CSF-differentiated (pro-inflammatory) macrophages.
AM3 treatment does not induce innate immune memory in human monocytes
Given that treatment with AM3 in the first 24 hours skewed the differentiation of macrophages, we sought to elucidate whether AM3 could induce innate immune memory in monocytes using an established protocol (Figure 4A, see details in the methods) (17). In these experiments, β-glucan and the live-vaccine BCG, well-established inducers of innate immune memory (13, 24), were used as positive controls.
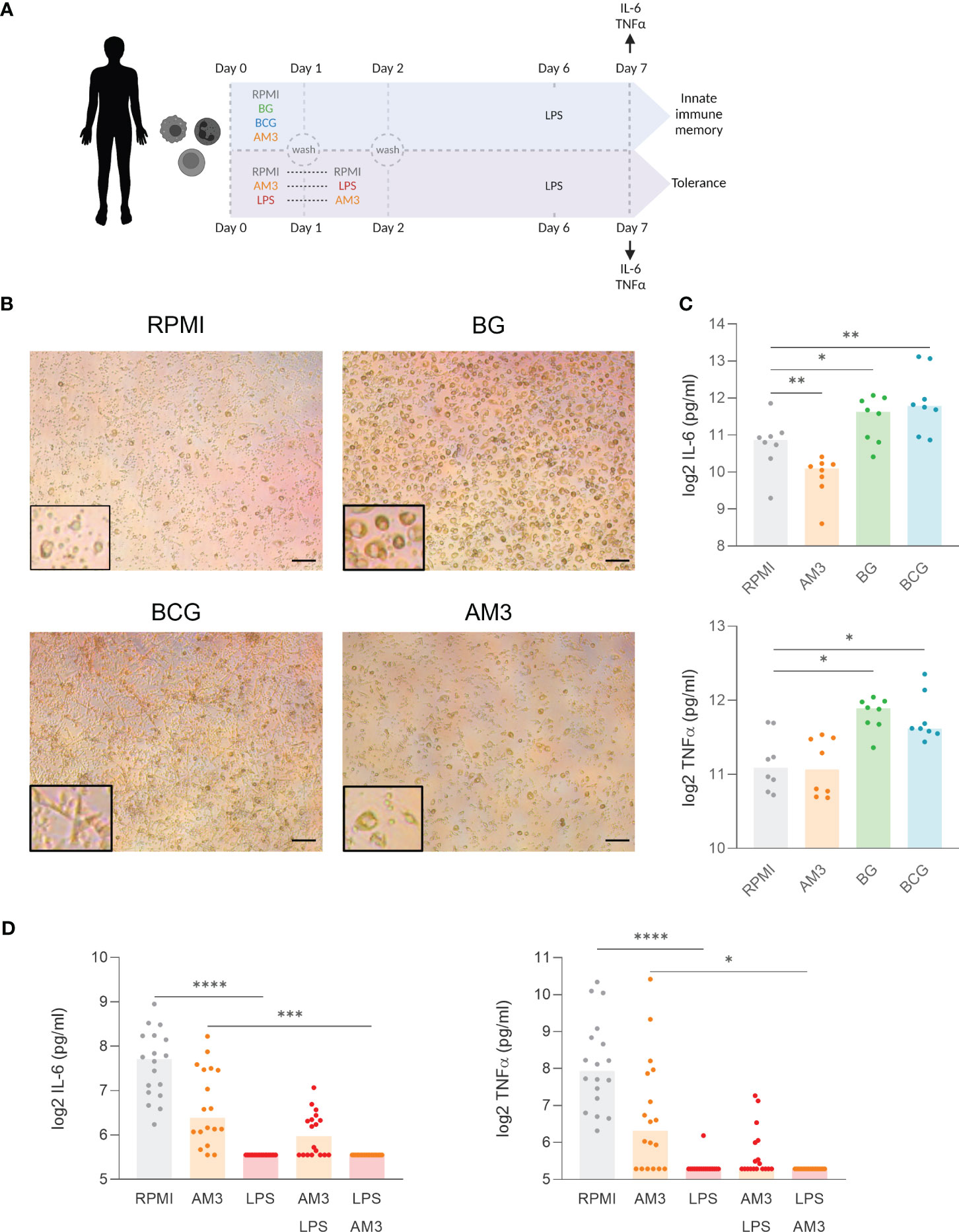
Figure 4 AM3 treatment does not induce innate immune memory in human monocyte. (A) Experimental design: First 48 hours were conducted as depicted in Figure 1A. Afterwards, adherent cells were left for rest in the base medium supplemented with 10% Human pooled serum for 5 days followed by LPS restimulation. After 24 hours of LPS stimulaion, the supernatants were collected for cytokine measurement. (B) Microscope images at day 6 after the first stimulation, magnification:10x, scale bar: 200 µM. The areas highlighted with a black rectangle show an area of the same image with a larger magnification to better appreciate the cell morphology. (C) Cytokine production after LPS restimulation at day 7 in the innate immune memory model, n=8, Wilcoxon matched-paired test comparing each condition to RPMI. (D) Cytokine production after LPS restimulation at day 7 in the tolerance model. n=18, Friedman test with Dunn’s multiple comparison. *p<0.05, **p<0.01, ***p<0.001, ****p<0.0001.
First, in terms of morphology, monocytes that differentiated in the presence of BG exhibited a prominent, granular phenotype, while cells that underwent differentiation with BCG demonstrated a distinctive elongated star-shape. In contrast, monocytes treated with AM3 remained similar to those cultured in RPMI: small cell bodies with granular appearance (Figure 4B). At the level of cytokine production, treatment with AM3 did not induce any increase in the production of cytokines compared to unstimulated cells (Figure 4C), independent from the dose used (Figure S4). The comparison of the effects in young and elderly volunteers did not show any differences in cytokine production related to age (Figure S5). Overall, our study did not reveal any measurable impact of AM3 on the induction of innate immune memory.
It is known that stimulation with LPS induces immune tolerance in the long term (19). In other words, monocytes that produce large amounts of cytokines after acute stimulation with LPS will not produce more cytokines after secondary stimulation. This resembles what happens in humans after an episode of sepsis when there is a strong induction of cytokines in the acute phase, often followed by immune paralysis that can last for weeks or even months (25). Interestingly, in our in vitro model, the pre-treatment of monocytes with AM3 prior to LPS stimulation (AM3-LPS group) was able to partially, but not significantly, revert the development of immune tolerance. Supporting this observation, we did not find significant changes in lactate concentration (Figure S6), which is an important factor in the metabolic shift in innate immune memory and tolerance (26), throughout the process. We observed that these cells maintained the ability to produce IL-6 and TNFα after secondary challenge with LPS 6 days after the original stimulation (AM3-LPS group), in contrast to cells that were challenged with LPS alone (LPS group), or with AM3 after LPS exposure (LPS-AM3 group) (Figure 4D). While the highest concentration of AM3 we used (50 μg/mL) showed a similar effect, lower concentrations had no substantial impact on cytokine production (see Figure S4).
Discussion
An equilibrium between inflammatory and anti-inflammatory factors is required during homeostasis. The immune response must be constantly kept within a homeostatic range to avoid the onset of autoimmune or inflammatory diseases derived from excessive stimulation or the development of immune tolerance with increased susceptibility to infections and cancer (27). In an aging society suffering from the increase in the burden of inflammatory diseases, new strategies for prophylaxis and intervention with safe anti-inflammatory compounds are critically needed. In this study, we have shown the potential of AM3 as a compound to limit inflammatory responses without causing immune tolerance.
When we think of infectious diseases caused by pathogens, we tend to focus on the direct effects of the pathogen on the host. However, the main cause of morbidity and mortality in many infectious diseases is not the direct interaction of the pathogen with our cells but the exacerbated immune response that the infection induces (28). There are multiple examples of this, ranging from bacterial tonsillitis causing throat swelling and fever to cases often involving the patient’s death. For example, the excessive reaction that the immune system mounts to bacteria and fungi is the underlying driver of septic shock (13, 29). Similarly, the many cases of severe COVID-19 with extensive lung damage are due to excessive inflammatory reactions. In these contexts, monoclonal antibodies against IL-6 and IL-1β provide possible therapies to dampen the levels of pro-inflammatory cytokines (30). In our experiments, we observed how pre-treating human cells with AM3 decreases the exacerbated production of cytokines induced by LPS. This property could be employed to prevent cytokine storm response and/or septic shocks in severe infections. Notably, the moderation of cytokine production is not linked with immunosuppression, since cells derived from many individuals maintained cytokine production capacity after exposure to AM3 to levels at least comparable to those of untreated cells. These anti-inflammatory effects align with the capacity of AM3 to skew the differentiation process of monocytes towards a non-proinflammatory phenotype.
In addition to the threat of infectious diseases, we are currently experiencing a silent pandemic of inflammatory diseases: diabetes, gout, rheumatoid arthritis, cardiovascular diseases, inflammatory bowel diseases, autoimmune diseases, and more (31). In this context, using molecules with anti-inflammatory activity can help greatly mitigate the signs, symptoms, and consequences of these chronic diseases (22). All these diseases have in common is the production (either constant or caused by a specific stimulus) of pro-inflammatory cytokines, which trigger a cascade of inflammatory events, increasing myelopoiesis that favors inflammation (32). Our results revealed a marked anti-inflammatory effect of AM3 in human cells suggesting a potential for this compound as a treatment for chronic inflammatory diseases. However, future studies of AM3 applications in specific disease models are needed. The onset and duration of chronic inflammatory diseases have been linked with the induction of maladaptive innate immune memory (31, 33, 34). Unlike other compounds with a fungal origin, such as β-glucan, AM3 did not show the capacity to induce innate immune memory, which supports the safety of its use in the context of inflammatory diseases. The systemic and local production of cytokines by PBMCs and macrophages can help limit disease and control infection spread, in several different conditions, such as tuberculosis, SARS, or influenza (35–38), so the study of compounds with immunomodulatory properties to use as complementary approaches to current therapies or as adjuvants is warranted.
Another intriguing effect observed in this study is the potential of AM3 to prevent the immune tolerance induced by LPS in human monocytes, which is characteristic of people who survive an inflammatory event after bacterial sepsis and causes the death of these patients due to the inability of their immune system to respond to secondary infections days, weeks, or months after the initial infection (39). To our knowledge, only the fungal-derived compound β-glucan has shown the ability to prevent the onset of tolerance (40). The differential potential of β-glucan and AM3 to revert tolerance can be attributed to chemical characteristics. β-glucan, which is known to induce innate immune memory, bears β(1→3) bonds (41). However, different glucose bonds in different glucans from various species can alter their immunomodulatory effects (42). AM3 has β(1→6) and β(1→2), which potentially makes the effects exerted by this compound distinct from those of its close relative β-glucan [β(1→3)]. Another difference is that β-glucan is known to induce long-term activation of the innate immune system, which can be useful in the case of immunocompromised patients but detrimental in those with inflammatory or autoimmune diseases. Thus, our results underline the potential of AM3 as a compound that could help prevent immune tolerance in patients without contributing to inflammation and favoring the maintenance of immune responses within the homeostatic range.
Consequently, we propose that AM3 is a potential candidate for long-term treatment to improve the health status and quality of life of patients with pro-inflammatory diseases. Future clinical trials to assess if AM3 treatment improves the condition and prognosis of patients with chronic inflammatory diseases are needed. Further research to elucidate its potential benefits in inflammatory diseases and the consequences of inflammaging are expected in the upcoming years.
Data availability statement
The raw data supporting the conclusions of this article will be made available by the authors, without undue reservation.
Ethics statement
The studies involving humans were approved by MREC Oost-Nederland p/a Radboudumc, house post 628, P.O. box 9101 6500 HB Nijmegen The Netherlands. The studies were conducted in accordance with the local legislation and institutional requirements. The participants provided their written informed consent to participate in this study.
Author contributions
Conceived and designed the study: JD-A, AR-L and PF-G. Performed the experiments: BG, (human experiments), GK, PD, KF (Documentation for clinical trial, collection, and processing of samples). Analyzed and discussed the data: BG, AR-L, PF-G, and AL. Wrote the paper: BG and JD-A. All authors contributed to the article and approved the submitted version.
Funding
The authors declare financial support was received for the research, authorship, and/or publication of this article. The Netherlands Organization for Scientific Research (VENI grant 09150161910024 and Off Road grant 04510012010022) to JD-A. The authors declare that this study received funding from Cantabria Labs. The funder was not involved in the study design, collection, analysis, interpretation of data, the writing of this article, or the decision to submit it for publication.
Conflict of interest
AR-L and AL were full-time employed by Cantabria Labs. PF-G is a scientific adviser at Cantabria Labs.
The remaining authors declare that the research was conducted in the absence of any commercial or financial relationships that could be construed as a potential conflict of interest.
Publisher’s note
All claims expressed in this article are solely those of the authors and do not necessarily represent those of their affiliated organizations, or those of the publisher, the editors and the reviewers. Any product that may be evaluated in this article, or claim that may be made by its manufacturer, is not guaranteed or endorsed by the publisher.
Supplementary material
The Supplementary Material for this article can be found online at: https://www.frontiersin.org/articles/10.3389/fimmu.2023.1165683/full#supplementary-material
References
1. Chen L, Deng H, Cui H, Fang J, Zuo Z, Deng J, et al. Inflammatory responses and inflammation-associated diseases in organs. Oncotarget (2018) 9(6):7204. doi: 10.18632/oncotarget.23208
2. Cicchese JM, Evans S, Hult C, Joslyn LR, Wessler T, Millar JA, et al. Dynamic balance of pro- and anti-inflammatory signals controls disease and limits pathology. Immunol Rev (2018) 285(1):147–67. doi: 10.1111/imr.12671
3. Guan Q, Zhang J. Recent advances: the imbalance of cytokines in the pathogenesis of inflammatory bowel disease. Mediators Inflammation (2017) 2017. doi: 10.1155/2017/4810258
4. Kany S, Vollrath JT, Relja B. Cytokines in inflammatory disease. Int J Mol Sci (2019) 20(23). doi: 10.3390/ijms20236008
5. Roth GA, Mensah GA, Johnson CO, Addolorato G, Ammirati E, Baddour LM, et al. Global burden of cardiovascular diseases and risk factors, 1990-2019: update from the GBD 2019 study. J Am Coll Cardiol (2020) 76(25):2982–3021. doi: 10.1016/j.jacc.2020.11.010
6. Liu Y, Bastiaan-Net S, Wichers HJ. Current understanding of the structure and function of fungal immunomodulatory proteins. Front Nutr (2020) 7:132. doi: 10.3389/fnut.2020.00132
7. Mercer DK, O’Neil DA. Innate inspiration: antifungal peptides and other immunotherapeutics from the host immune response. Front Immunol (2020), 11:2177. doi: 10.3389/fimmu.2020.02177
8. Brieva A, Guerrero A, Alonso-Lebrero JL, Pivel JP. Immunoferon, a glycoconjugate of natural origin, inhibits LPS-induced TNF-alpha production and inflammatory responses. Int Immunopharmacol (2001) 1(11):1979–87. doi: 10.1016/S1567-5769(01)00125-4
9. Martín-Vilchez S, Molina-Jiménez F, Alonso-Lebrero JL, Sanz-Cameno P, Rodríguez-Mũoz Y, Benedicto I, et al. AM3, a natural glycoconjugate, induces the functional maturation of human dendritic cells. Br J Pharmacol (2008) 154(3):698. doi: 10.1038/bjp.2008.87
10. Varela J, Navarro Pico ML, Guerrero A, García F, Giménez Gallego G, Pivel JP. Identification and characterization of the peptidic component of the immunomodulatory glycoconjugate Immunoferon. Methods Find Exp Clin Pharmacol (2002) 24(8):471–80. doi: 10.1358/mf.2002.24.8.705066
11. Guerrero A, Brieva A, Pivel JP. A new method for radioiodination of polysaccharides and its use in biodistribution studies of an immunomodulating glycoconjugate (Immunoferon). Methods Find Exp Clin Pharmacol (2000) 22(8):621–5. doi: 10.1358/mf.2000.22.8.802273
12. Brieva A, Guerrero A, Pivel JP. Inmunoferon, an immunomodulator of natural origin, does not affect the rat liver cytochrome P-450 and phase II conjugation enzymes. Methods Find Exp Clin Pharmacol (2003) 25(3):187–91. doi: 10.1358/mf.2003.25.3.769638
13. Dominguez-Andres J, Netea MG. Long-term reprogramming of the innate immune system. J Leukoc Biol (2019) 105(2):329–38. doi: 10.1002/JLB.MR0318-104R
14. Netea MG, Domínguez-Andrés J, Barreiro LB, Chavakis T, Divangahi M, Fuchs E, et al. Defining trained immunity and its role in health and disease. Nat Rev Immunol (2020). doi: 10.1038/s41577-020-0285-6
15. de Graaff P, Berrevoets C, Rösch C, Schols HA, Verhoef K, Wichers HJ, et al. Curdlan, zymosan and a yeast-derived β-glucan reshape tumor-associated macrophages into producers of inflammatory chemo-attractants. Cancer Immunology Immunother (2021) 70(2):547–61. doi: 10.1007/s00262-020-02707-4
16. Bekkering S, Blok BA, Joosten LAB, Riksen NP, Van Crevel R, Netea MG. In Vitro experimental model of trained innate immunity in human primary monocytes. Clin Vaccine Immunol (2016) 23(12):926–33. doi: 10.1128/CVI.00349-16
17. Domínguez-Andrés J, Arts RJW, Bekkering S, Bahrar H, Blok BA, de Bree LCJ, et al. In vitro induction of trained immunity in adherent human monocytes. STAR Protoc (2021) 2(1). doi: 10.1016/j.xpro.2021.100365
18. Ngkelo A, Meja K, Yeadon M, Adcock I, Kirkham PA. LPS induced inflammatory responses in human peripheral blood mononuclear cells is mediated through NOX4 and Giα dependent PI-3kinase signalling. J Inflammation (Lond) (2012) 9:1. doi: 10.1186/1476-9255-9-1
19. Ifrim DC, Quintin J, Joosten LAB, Jacobs C, Jansen T, Jacobs L, et al. Trained immunity or tolerance: Opposing functional programs induced in human monocytes after engagement of various pattern recognition receptors. Clin Vaccine Immunol (2014). doi: 10.1128/CVI.00688-13
20. Weyand CM, Goronzy JJ. Aging of the immune system: Mechanisms and therapeutic targets. Ann Am Thorac Soc (2016) 13(Suppl 5):S422–8. doi: 10.1513/AnnalsATS.201602-095AW
21. Fuentes E, Fuentes M, Alarcón M, Palomo I. Immune system dysfunction in the elderly. Acad Bras Cienc (2017) 89(1):285–99. doi: 10.1590/0001-3765201720160487
22. Bulut O, Kilic G, Domínguez-Andrés J. Immune memory in aging: a wide perspective covering microbiota, brain, metabolism, and epigenetics. Clin Rev Allergy Immunol (2022) 63(3):499–529. doi: 10.1007/s12016-021-08905-x
23. Jurga AM, Paleczna M, Kuter KZ. Overview of general and discriminating markers of differential microglia phenotypes. Front Cell Neurosci (2020) 14:198. doi: 10.3389/fncel.2020.00198
24. Kleinnijenhuis J, Quintin J, Preijers F, Joosten LAB, Ifrim DC, Saeed S, et al. Bacille Calmette-Guérin induces NOD2-dependent nonspecific protection from reinfection via epigenetic reprogramming of monocytes. Proc Natl Acad Sci U.S.A. (2012) 109(43):17537–42. doi: 10.1073/pnas.1202870109
25. van der Poll T, van de Veerdonk FL, Scicluna BP, Netea MG. The immunopathology of sepsis and potential therapeutic targets. Nat Rev Immunol (2017) 17(7):407–20. doi: 10.1038/nri.2017.36
26. Arts RJW, Carvalho A, La Rocca C, Palma C, Rodrigues F, Silvestre R, et al. Immunometabolic pathways in BCG-induced trained immunity. Cell Rep (2016) 17(10):2562. doi: 10.1016/j.celrep.2016.11.011
27. Mulder WJM, Ochando J, Joosten LAB, Fayad ZA, Netea MG. Therapeutic targeting of trained immunity. Nat Rev Drug Discovery (2019) 18(7):553–66. doi: 10.1038/s41573-019-0025-4
28. Fajgenbaum DC, June CH. Cytokine storm. New Engl J Med (2020) 383(23):2255–73. doi: 10.1056/NEJMra2026131
29. Alten J, Prabhakaran P. Bacterial sepsis and shock. Textbook Clin Pediatr (2012) 783:783–9. doi: 10.1007/978-3-642-02202-9_61
30. Della-Torre E, Criscuolo E, Lanzillotta M, Locatelli M, Clementi N, Mancini N, et al. IL-1 and IL-6 inhibition affects the neutralising activity of anti-SARS-CoV-2 antibodies in patients with COVID-19. Lancet Rheumatol (2021) 3(12):e829–31. doi: 10.1016/S2665-9913(21)00321-0
31. Christ A, Günther P, Lauterbach MAR, Duewell P, Biswas D, Pelka K, et al. Western diet triggers NLRP3-dependent innate immune reprogramming. Cell (2018) 172(1–2):162–175.e14. doi: 10.1016/j.cell.2017.12.013
32. Chavakis T, Mitroulis I, Hajishengallis G. Hematopoietic progenitor cells as integrative hubs for adaptation to and fine-tuning of inflammation. Nat Immunol (2019) 20(7):802–11. doi: 10.1038/s41590-019-0402-5
33. Bekkering S, Dominguez-Andres J, Joosten LAB, Riksen NP, Netea MG. Trained immunity: reprogramming innate immunity in health and disease. Annu Rev Immunol (2021) 39:667–93. doi: 10.1146/annurev-immunol-102119-073855
34. Bekkering S, van den Munckhof I, Nielen T, Lamfers E, Dinarello C, Rutten J, et al. Innate immune cell activation and epigenetic remodeling in symptomatic and asymptomatic atherosclerosis in humans in vivo. Atherosclerosis (2016) 254:228–36. doi: 10.1016/j.atherosclerosis.2016.10.019
35. Mishra A, Singh VK, Actor JK, Hunter RL, Jagannath C, Subbian S, et al. GM-CSF dependent differential control of mycobacterium tuberculosis infection in human and mouse macrophages: is macrophage source of GM-CSF critical to tuberculosis immunity? Front Immunol (2020) 11. doi: 10.3389/fimmu.2020.01599
36. Geckin B, Zoodsma M, Kilic G, Debisarun PA, Rakshit S, Adiga V, et al. Differences in immune responses in individuals of Indian and european origin: relevance for the COVID-19 pandemic. Microbiol Spectr (2023) 11(2). doi: 10.1128/spectrum.00231-23
37. Aguilera ER, Lenz LL. Inflammation as a modulator of host susceptibility to pulmonary influenza, pneumococcal, and co-infections. Front Immunol (2020) 11:509487. doi: 10.3389/fimmu.2020.00105
38. Hasanvand A. COVID-19 and the role of cytokines in this disease. Inflammopharmacology (2022) 30(3):789. doi: 10.1007/s10787-022-00992-2
39. Tang BM, Herwanto V, McLean AS. Immune paralysis in sepsis: recent insights and future development. Annual Update in Intensive Care and Emergency Medicine (2018) 13–23. doi: 10.1007/978-3-319-73670-9_2
40. Novakovic B, Habibi E, Wang SY, Arts RJW, Davar R, Megchelenbrink W, et al. β-glucan reverses the epigenetic state of LPS-induced immunological tolerance. Cell (2016) 167(5):1354–68. doi: 10.1016/j.cell.2016.09.034
41. Moorlag SJCFM, Khan N, Novakovic B, Kaufmann E, Jansen T, van Crevel R, et al. β-Glucan Induces Protective Trained Immunity against Mycobacterium tuberculosis Infection: A Key Role for IL-1. Cell Rep (2020) 31(7). doi: 10.1016/j.celrep.2020.107634
Keywords: immune response, homeostasis, cytokines, inflammation, AM3, disease, monocytes, macrophages
Citation: Geckin B, Kilic G, Debisarun PA, Föhse K, Rodríguez-Luna A, Fernández-González P, Sánchez AL and Domínguez-Andrés J (2023) The fungal-derived compound AM3 modulates pro-inflammatory cytokine production and skews the differentiation of human monocytes. Front. Immunol. 14:1165683. doi: 10.3389/fimmu.2023.1165683
Received: 14 February 2023; Accepted: 26 September 2023;
Published: 09 October 2023.
Edited by:
Stefano Caserta, Hull York Medical School, United KingdomReviewed by:
Xue-kai Wang, Chinese Academy of Medical Sciences, ChinaYahya Sohrabi, University Hospital Münster, Germany
Payal Damani-Yokota, New York University, United States
Copyright © 2023 Geckin, Kilic, Debisarun, Föhse, Rodríguez-Luna, Fernández-González, Sánchez and Domínguez-Andrés. This is an open-access article distributed under the terms of the Creative Commons Attribution License (CC BY). The use, distribution or reproduction in other forums is permitted, provided the original author(s) and the copyright owner(s) are credited and that the original publication in this journal is cited, in accordance with accepted academic practice. No use, distribution or reproduction is permitted which does not comply with these terms.
*Correspondence: Jorge Domínguez-Andrés, jorge.dominguezandres@radboudumc.nl
†Present addresses: Azahara Rodríguez-Luna, Faculty of Health Sciences, Universidad Loyola Andalucía, Seville, Spain;Department of Pharmacology, Faculty of Pharmacy, Universidad de Sevilla, Seville, Spain