- 1Key Laboratory of Aquaculture Nutrition and Feed (Ministry of Agriculture and Rural Affairs) and Key Laboratory of Mariculture (Ministry of Education), Ocean University of China, Qingdao, China
- 2Guangdong Evergreen Feed Industry Co., Ltd., Key Laboratory of Aquatic, Livestock and Poultry Feed Science and Technology in South China, Ministry of Agriculture and Rural Affairs, Zhanjiang, China
- 3Laboratory for Marine Fisheries Science and Food Production Processes, Qingdao National Laboratory for Marine Science and Technology, Qingdao, China
Dietary high soybean oil (SO) levels might cause hepatic lipid deposition, induce oxidative stress and inflammatory response in aquatic animals, while octanoate (OCT) is beneficial to metabolism and health in mammals. However, the effect of OCT has been studied rarely in aquatic animals. In this study, a 10-week feeding trial was conducted to investigate the effect of supplemental OCT on hepatic lipid metabolism, serum biochemical indexes, antioxidant capacity and inflammatory response of large yellow croaker (Larimichthys crocea) fed with high SO levels diet. The negative control diet contained 7% fish oil (FO), while the positive control diet contained 7% SO. The other four experimental diets were supplemented with 0.7, 2.1, 6.3 and 18.9 g/kg sodium octanoate (OCT) based on the positive control diet. Results showed that OCT supplementation effectively reduced the hepatic crude lipid, triglyceride (TG), total cholesterol (TC) and non-esterified free fatty acids contents, and alleviated lipid accumulation caused by the SO diet. Meanwhile, OCT supplementation decreased the serum TG, TC, alanine transaminase, aspartate transaminase and low-density lipoprotein cholesterol levels, increased the serum high-density lipoprotein cholesterol level, improved the serum lipid profiles and alleviated hepatic injury. Furthermore, with the supplementation of OCT, the mRNA expression of genes related to lipogenesis (acc1, scd1, fas, srebp1, dgat1 and cebpα) and fatty acid (FA) transport (fabp3, fatp and cd36) were down-regulated, while the mRNA expression of genes related to lipolysis (atgl, hsl and lpl) and FA β-oxidation (cpt1 and mcad) were up-regulated. Besides that, dietary OCT increased the total antioxidant capacity, activities of peroxidase, catalase and superoxide dismutase and the content of reduced glutathione, decreased the content of 8-hydroxy-deoxyguanosine and malondialdehyde and relieved hepatic oxidative stress. Supplementation of 0.7 and 2.1 g/kg OCT down-regulated the mRNA expression of genes related to pro-inflammatory cytokines (tnfα, il1β and ifnγ), and suppressed hepatic inflammatory response. In conclusion, supplementation with 0.7-2.1 g/kg OCT could reduce hepatic lipid accumulation, relieve oxidative stress and regulate inflammatory response in large yellow croaker fed the diet with high SO levels, providing a new way to alleviate the hepatic fat deposition in aquatic animals.
1 Introduction
Soybean oil (SO) enriched in n-6 polyunsaturated fatty acids (n-6 PUFA) has been applied to the aquaculture industry widely, because of its high output and low price. A previous study found 8% SO in diet could alter the fatty acid composition of juvenile hybrid sturgeon (Acipenser Baeribrandt♀×A. schrenckiibrandt♂) (1). Similar results were found in grass carp (Ctenopharyngodon Idella) fed diets with 5% SO diet (2) and largemouth bass (Micropterus Salmoides) fed diets with 9% SO (3). Supplementation of 56% and 100% SO in the diet (8% dietary lipid) significantly increased hepatic lipid content in blunt snout bream (Megalobrama Amblycephala) juvenile (4). And 7.5% SO in diet increased lipid deposition in the liver of turbot (Scophthalmus Maximus L.) (5). Meanwhile, dietary 6.5% SO depressed the hepatic anti-oxidative capacity and induced the inflammatory response in large yellow croaker (Larimichthys crocea) (6). Therefore, it is necessary to find suitable additives to alleviate metabolic abnormalities caused by high levels of SO.
Octanoate (OCT) is a medium-chain fatty acid (MCFA) that is found in goat’s milk and coconut oil (7). Studies showed that dietary OCT could inhibit fat accumulation (8), ameliorate insulin resistance (9), suppress oxidative stress (10) and inflammatory response (11) in mammals. In aquatic animals, studies showed that OCT could affect the FA perception of rainbow trout (Oncorhynchus mykiss) (12), and improve oxidative stress in the gut-brain axis of zebrafish (Danio rerio) (13). However, there are few studies on effects of OCT on hepatic lipid metabolism, antioxidant capacity and inflammatory response in fish fed with high SO levels.
Large yellow croaker is an important economic fish in mariculture and a good model for studying fish lipid metabolism (14). At present, studies found that high-level SO diets could induce fat deposition, oxidative stress and inflammatory response of large yellow croaker (15–17). Considering the beneficial effect of OCT on metabolism and health in mammals, we investigated the effects of supplemental OCT on lipid metabolism, antioxidant capacity and inflammatory response of large yellow croaker fed the diet with high SO level. Our findings can be used to investigate effective nutritional strategies to alleviate fat deposition in aquatic animals.
2 Materials and methods
2.1 Experimental procedure
Large yellow croaker juveniles were provided by FuFa Aquatic Products Co., Ltd. (Ningde, China). The preparation of experimental diets referred to the previous study (18) (Supplementary Table 1). Diets containing the negative control group with 7% fish oil (FO) as the lipid source, the positive control group with 7% SO as the lipid source and treatment diets (to the SO group, 0.7, 2.1, 6.3 and 18.9 g/kg sodium octanoate (OCT) (purity: ≥ 99%, Sigma, USA) were added). Before the formal experiment, all the fish were fed commercial diets for 2 weeks to acclimate to experimental conditions. Fish were fasted for 24 h prior to the start of the feeding trial. Then 60 fish of similar size (13.00 ± 0.10 g) were randomly divided into 18 cages (1 m × 1 m × 1.5 m). Each diet was fed randomly to 3 cages twice a day (05:00 and 17:00) for 10 weeks in suitable environmental conditions (temperature: 19.5-25.5°C, salinity: 25-28‰, and dissolved oxygen content was approximately 7 mg/L).
2.2 Sample collection
At the end of the feeding trial, fish were starved for 24 h, then anesthetized using MS-222 (1: 10,000, Sigma) for sample collection. Blood samples were collected from the caudal vein to anticoagulant-free centrifuge tubes, clotted at 4°C for 4 h, then centrifuged at 2, 500 ×g (10 min) to separate serum for biochemical index analysis. Hepatic samples from five fish per cage were fixed in 4% paraformaldehyde for 24 h, then transferred to 75% ethanol for hematoxylin and eosin (H&E) staining and the Oil Red O staining. Hepatic tissues were collected from ten fish per cage, frozen in liquid nitrogen, and stored at −80°C for subsequent analysis.
2.3 Hepatic histological analysis
Paraffin-embedded sections and frozen sections of hepatic tissue were made according to the method described in a published paper (19). The paraffin sections were stained with hematoxylin and eosin and the frozen sections were stained with Oil Red O. Images were captured and analyzed using the microscope (Olympus BX53, Japan). The results of the Oil Red O area were analyzed using Image Pro Plus 6.0 software (Media Cybernetics, USA).
2.4 Hepatic biochemical analysis
The moisture and crude lipid of hepatic tissues were measured according to previous methods (19). Moisture was measured by drying samples at 105°C ventilation drying oven to constant weight. The crude lipid was measured by petroleum ether extraction using Soxhlet (Buchi, Sweden) to constant weight. Hepatic triglyceride (TG), total cholesterol (TC) and non-esterified fatty acids (NEFA) contents were detected by using kits (Nanjing Jiancheng Bioengineering Institute, China).
2.5 Serum biochemical index analysis
Serum TG, TC, high-density lipoprotein cholesterol (HDL-c), low-density lipoprotein cholesterol (LDL-c) contents and activities of alkaline phosphatase (ALP), aspartate transaminase (AST) and alanine transaminase (ALT) were measured by using kits (Nanjing Jiancheng Bioengineering Institute, China).
2.6 Hepatic fatty acids composition analysis
The FAs profiles of hepatic tissues were measured with Gas Chromatograph-Mass Spectrometer (GC-MS, QP2010 Plus, SHIMADZU, Japan) according to the method described by a previous study (20) with some modifications. The samples were subjected to a series of pre-treatments and finally quantified by GC-MS.
2.7 Hepatic antioxidant capacity analysis
Accurately weighed the weight of the hepatic tissue, added 9 times of normal saline to make the tissue homogenate, centrifuged at 2,500 rpm (10 min), then the supernatant was used for testing. Protein concentration was determined by a BCA Protein Assay Kit (Beyotime Institute of Technology, China). The total antioxidant capacity (T-AOC), activities of peroxidase (POD), catalase (CAT), superoxide dismutase (SOD) and contents of reduced glutathione (GSH), malondialdehyde (MDA), 8-hydroxydeoxyguanosine (8-OHdG) of liver were measured by using kits (Nanjing Jiancheng Bioengineering Institute, China).
2.8 RNA extraction, cDNA synthesis and real-time quantitative PCR (RT-qPCR) analysis
The RNA extraction followed the method of the previous study (20). Then, RNA was reversely transcribed to cDNA using the Prime Script-RT reagent Kit (Vazyme, China). RT-qPCR was carried out on a CFX96 Real-Time PCR Detection System (BIO-RAD, USA). The amplification was performed in a total volume of 20 μL containing 4 μL of cDNA, 0.5 μL of each primer, 10 μL of ChamQ Universal SYBR qPCR Master Mix (Vazyme, China) and 5 μL of RNase-free water. Real-time quantitative PCR procedures were 95°C for 2 min, followed by 39 cycles of 95°C for 10 s, 58°C for 10 s, and 72°C for 20 s. The primers used for RT-qPCR were presented in Table 1. Relative gene expression levels were calculated with the 2–ΔΔCT method (14).
2.9 Statistical analysis
All statistics were performed by SPSS 23.0 (IBM, America). The normality and homogeneity of variances were examined first. Then, two hypotheses were used in this study: (1) Independent-samples T-test was used in fish fed the FO diet and the SO diet. (2) A one-way analysis of variance (ANOVA) followed by Tukey’s multiple-range test and polynomial contrasts analysis was used in fish fed the diet with SO and OCT (0.7, 2.1, 6.3, 18.9 g/kg). The significance threshold was set at P < 0.05. The results were presented as means ± S.E.M. (standard error of the mean).
3 Results
3.1 Hepatic morphology
The hepatic morphology was performed by H&E staining. Compared with the FO diet, hepatocytes showed significant vacuolation and nuclear migration in fish fed with the SO diet (Figure 1). The supplementation of OCT reduced the size and number of vacuoles and alleviated nuclear atrophy and deviation, especially in fish fed the diet containing 2.1 g/kg OCT.
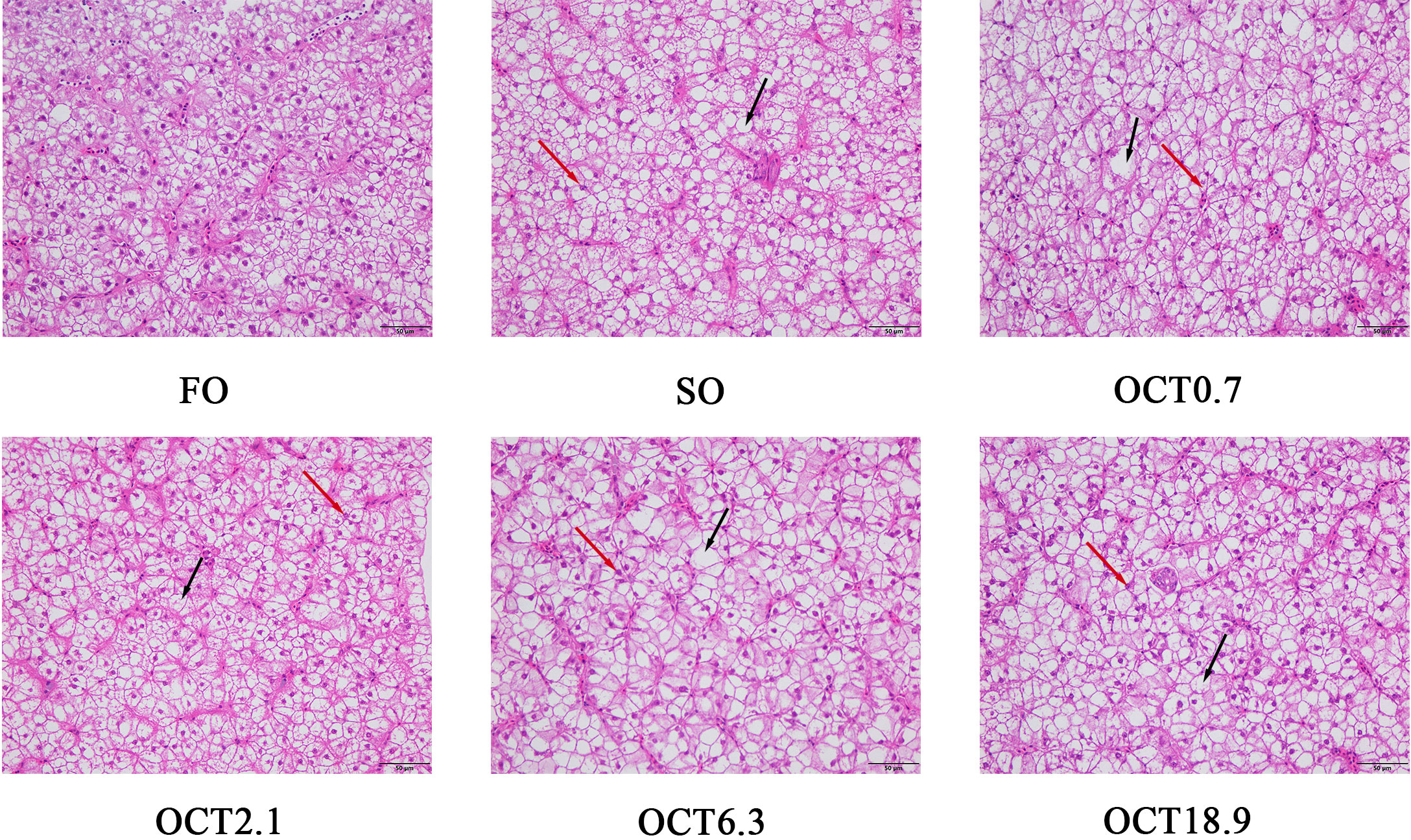
Figure 1 Effects of supplemental octanoate on hepatic morphology of large yellow croaker. The H&E staining results of different dietary treatments (200×, bars = 50 µm). The black arrows point to cellular vacuoles and the red arrows point to nuclear migration.
3.2 Hepatic biochemical index and Oil red O staining
The liver is an important metabolic organ involved in lipid metabolism. The results of Oil red O staining showed that the area of lipid droplets (LDs) was significantly increased when the FO in the diet was completely replaced by SO (P < 0.05). In contrast, OCT supplementation decreased the area of LDs in significantly linear and quadratic patterns (P < 0.05) (Figure 2A). Meanwhile, hepatic crude lipid, TG, TC and NEFA contents in fish fed the SO diet were notably higher than those in the FO diet (P < 0.01) and were effectively reduced by OCT supplementation (Figures 2B–E). As the OCT level increased, these were decreased in significantly linear and quadratic patterns (P < 0.05), and the peak values were observed in fish fed the diet containing 2.1 g/kg OCT.
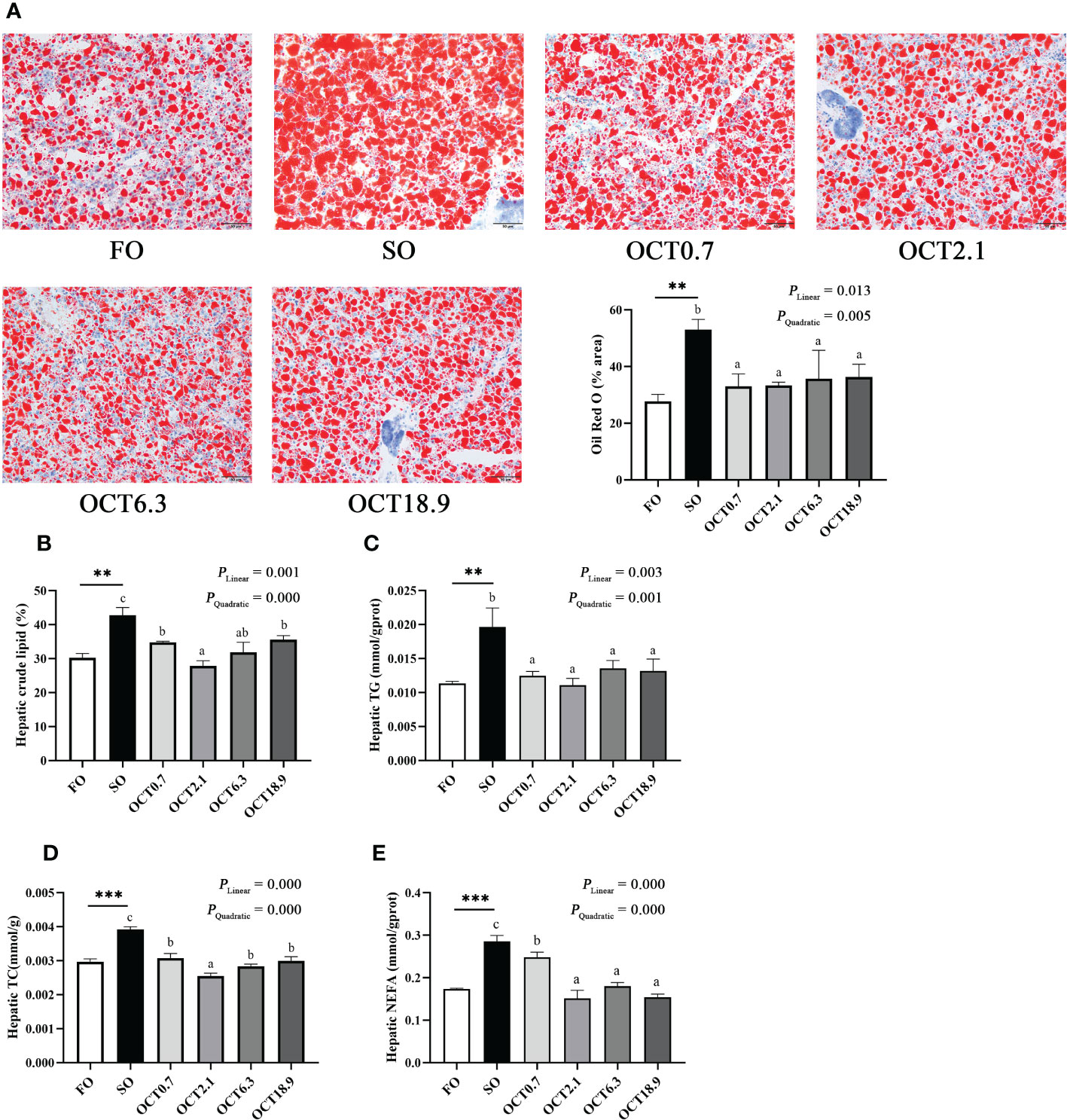
Figure 2 Effects of supplemental octanoate on hepatic lipid deposition of large yellow croaker. (A) The oil red O staining results of different dietary treatments (200×, bars = 50 µm), (B) Lipid contents in liver, (C) Total triglyceride contents in liver, (D) Total cholesterol contents in liver, (E) The non-esterified fatty acids contents in liver. The values were means (n = 3) with their standard errors represented by vertical bars. and the FO group. ∗∗ represent a significant difference of P < 0.01 between the SO group and the FO group. ∗∗∗ represent a significant difference of P < 0.001 between the SO group and the FO group. Different letters show significant difference (P < 0.05) among OCT treatment groups. Linear trend and Quadratic trend were analyzed by orthogonal polynomial contrasts.
3.3 Hepatic fatty acids composition
Hepatic FAs composition analysis (Table 2) showed that total saturated fatty acids (SFA) and total n-3 polyunsaturated fatty acids (n-3 PUFA) were significantly decreased and total n-6 PUFA were significantly increased when the FO in the diet was completely replaced by SO (P < 0.05). With the addition of OCT, the relative content of C18:2n-6 and C20:4n-6 were decreased in a significantly linear pattern (P < 0.05). The relative content of C22:6n-3 and the ratio of n-3 PUFA to n-6 PUFA showed a significant quadratic curve (P < 0.05) and maximum were observed in fish fed the diet containing 0.7 g/kg OCT.
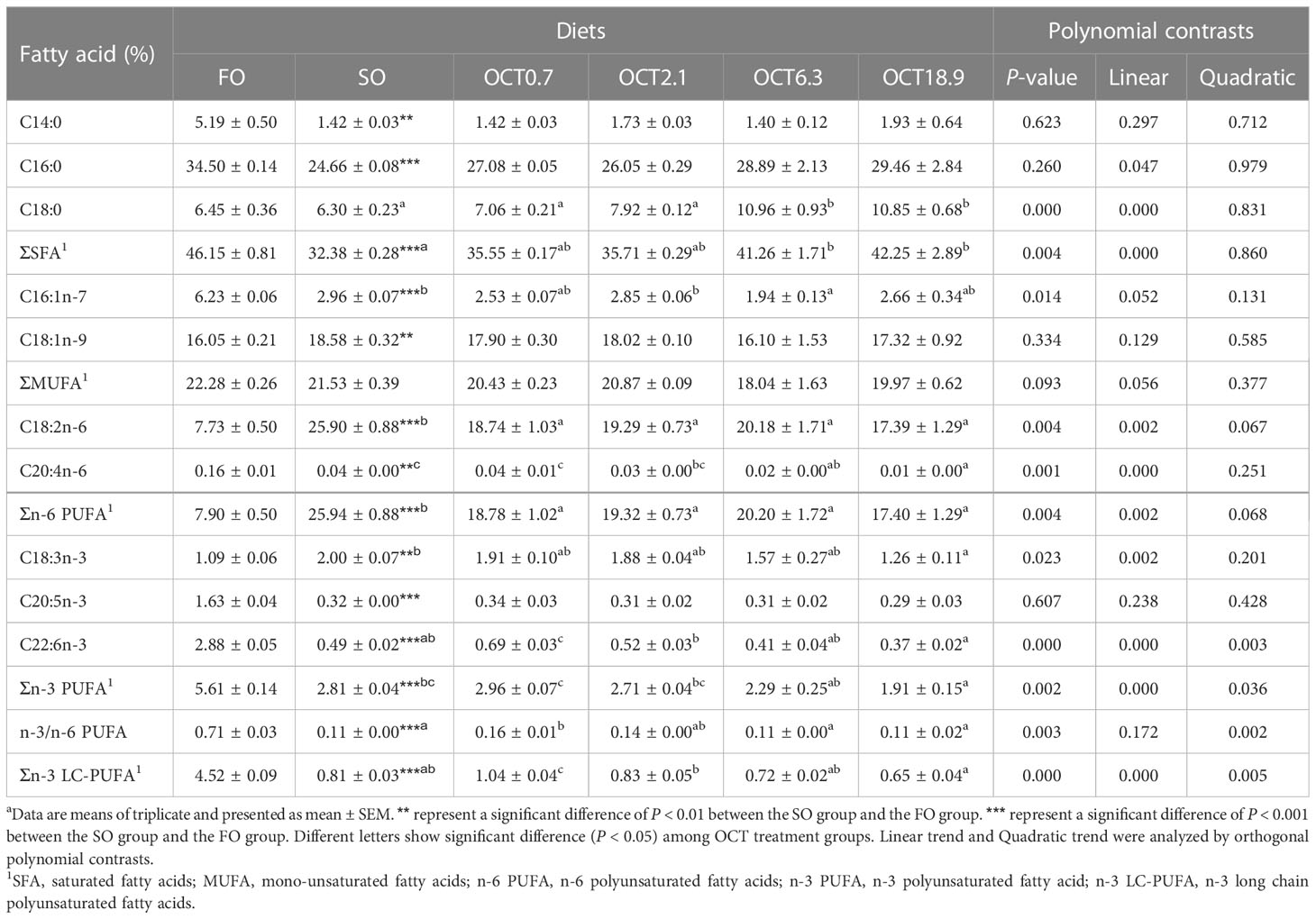
Table 2 Fatty acid profiles in liver of large yellow croaker in different groups (% total fatty acids).
3.4 Serum biochemical index
The serum TG, TC and LDL-c levels were significantly increased when the FO in the diet was completely replaced by SO (P < 0.05) (Figures 3A–C). In contrast, OCT supplementation decreased these in a significantly quadratic pattern (P < 0.001), and peak values were observed in fish fed the diet containing 2.1 g/kg OCT. The serum HDL-c level was increased in a significantly quadratic pattern with increasing dietary OCT (P < 0.01) (Figure 3D). The serum ALP levels were not significantly affected by the supplementation of OCT (P > 0.05) (Figure 3E). Both the serum ALT and AST levels were decreased in a significantly quadratic pattern with the increase of dietary OCT (P < 0.05) (Figures 3F, G), and the values in fish fed diets containing 0.7 and 2.1 g/kg OCT were lower.
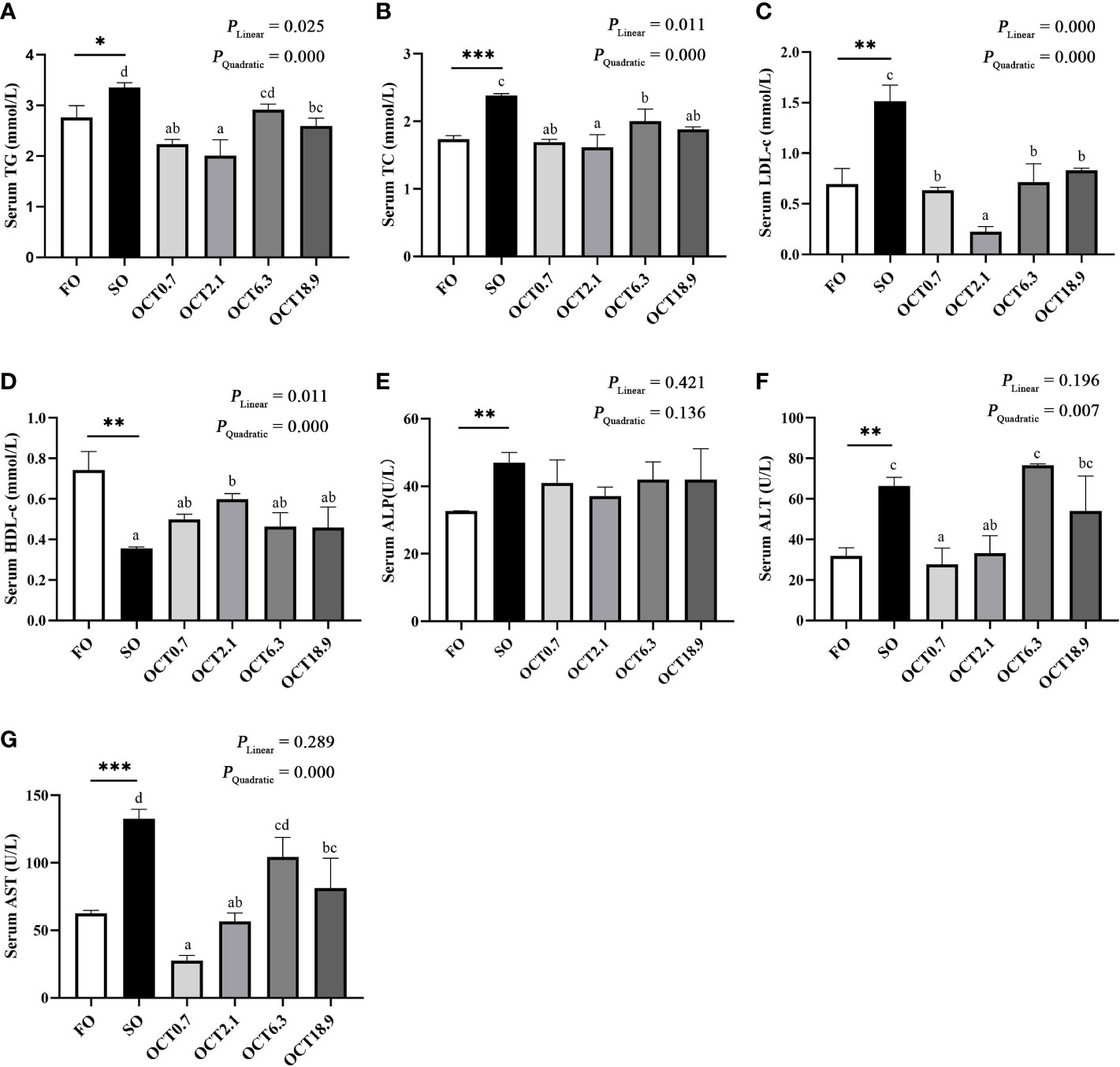
Figure 3 Effects of supplemental octanoate on serum biochemical indexes of large yellow croaker. (A) Serum TG contents, (B) Serum TC contents, (C) Serum LDL-c contents, (D) Serum HDL-c contents, (E) Serum ALP activity, (F) Serum ALT activity, (G) Serum AST activity. Values were means (n = 3) with their standard errors represented by vertical bars. ∗ represent a significant difference of P < 0.05 between the SO group and the FO group. ∗∗ represent a significant difference of P < 0.01 between the SO group and the FO group. ∗∗∗ represent a significant difference of P < 0.001 between the SO group and the FO group. Different letters show significant difference (P < 0.05) among OCT treatment groups. Linear trend and Quadratic trend were analyzed by orthogonal polynomial contrasts.
3.5 The mRNA expression of genes related to lipid metabolism in liver
Compared with the FO diet, the mRNA expression of genes related to lipogenesis including acc1, scd1, fas, srebp1, dgat1 and cebpα were significantly up-regulated in fish fed the SO diet (P < 0.05) (Figure 4A). With the dietary OCT increasing, the mRNA expression of these genes showed a significant quadratic curve (P < 0.05), and the values in fish fed diets containing 0.7 and 2.1 g/kg OCT were lower than the SO diet. The mRNA expression of FA transport-related genes, such as fabp3 and cd36, were significantly up-regulated and apob100 was significantly down-regulated when the FO in the diet was completely replaced by SO (P < 0.05) (Figure 4B). With the OCT level increasing, the mRNA expression of fabp3, fatp1 and cd36 were down-regulated in a significantly quadratic pattern, while the mRNA expression of apob100 showed the opposite trend (P < 0.05).
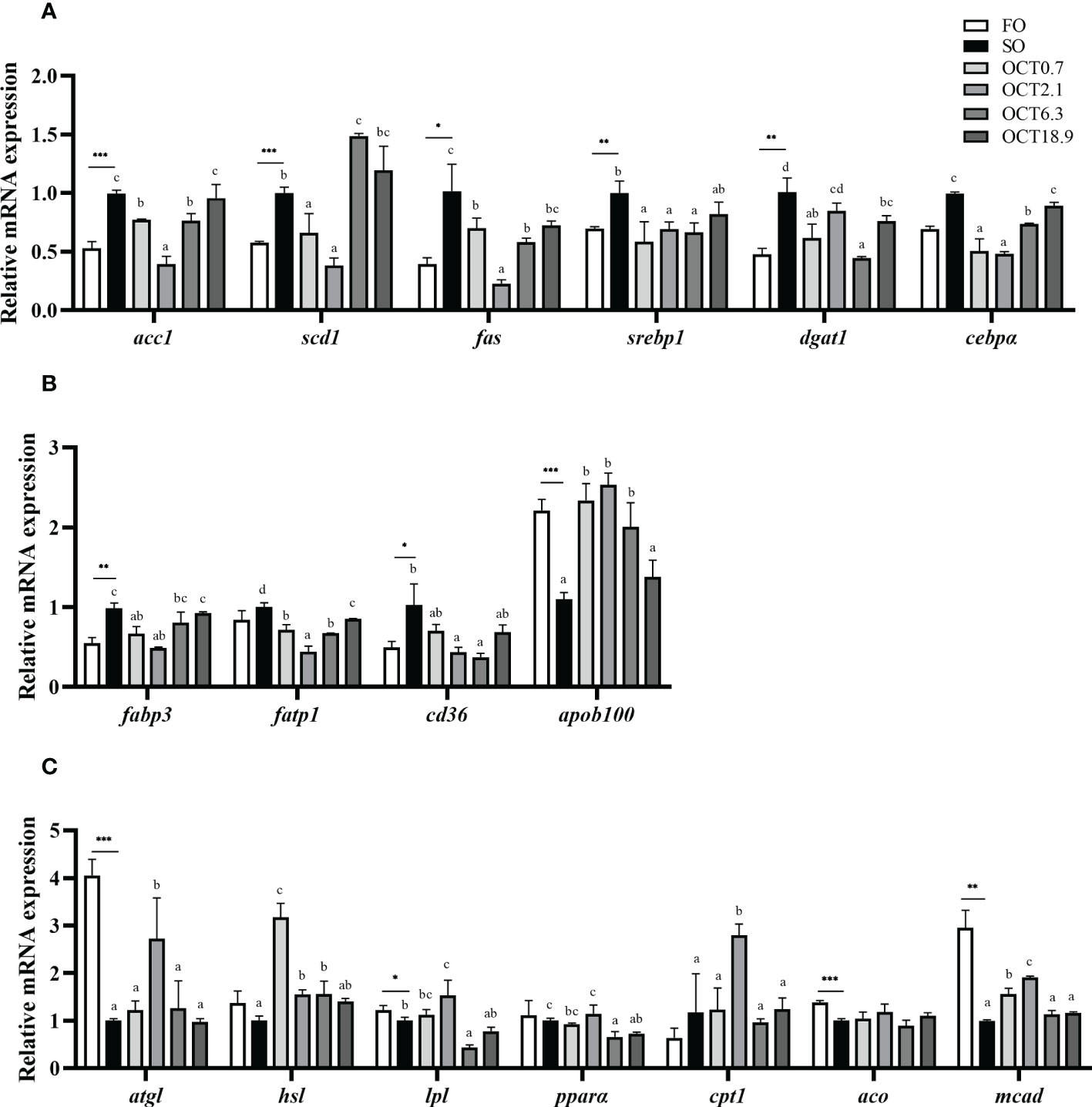
Figure 4 Effects of supplemental octanoate on the expression of lipid metabolism-related genes in the liver of large yellow croaker. (A) Lipogenesis, (B) FA transport, (C) Lipolysis and FA oxidation. Values were means (n = 3) with their standard errors represented by vertical bars. ∗ represent a significant difference of P < 0.05 between the SO group and the FO group. ∗∗ represent a significant difference of P < 0.01 between the SO group and the FO group. ∗∗∗ represent a significant difference of P < 0.001 between the SO group and the FO group. Different letters show significant difference (P < 0.05) among OCT treatment groups. acc1, acetyl CoA carboxylase 1; scd1, stearoyl-coenzyme A desaturase 1; fas, fatty acid synthase; srebp1, sterol-regulatory element binding protein-1; dgat1, diacylglycerol acyltransferase 1; cebpα, CCAAT/enhancer binding protein α; fabp3, fatty acid binding protein 3; fatp1, fatty acid transport protein 1; cd36, cluster of differentiation 36; apob100, apolipoprotein B 100; atgl, adipose triglyceride lipase; hsl, hormone-sensitive triglyceride lipase; pparα, peroxisome proliferator-activated receptor α; cpt1, carnitine palmitoyl transferase 1; aco, acyl-CoA oxidase; mcad, medium chain acyl-CoA dehydrogenase. Polynomial analysis in (A): acc1: PLinear = 0.479, PQuadratic = 0.000; scd1: PLinear = 0.000, PQuadratic = 0.000; fas: PLinear = 0.006, PQuadratic = 0.000; srebp1: PLinear = 0.194, PQuadratic = 0.002; dgat1: PLinear = 0.001, PQuadratic = 0.002; cebpα: PLinear = 0.856, PQuadratic = 0.000. Polynomial analysis in (B): fabp3: PLinear = 0.926, PQuadratic = 0.000; fatp1: PLinear = 0.003, PQuadratic = 0.000; cd36: PLinear = 0.002, PQuadratic = 0.001; apob100: PLinear = 0.539, PQuadratic = 0.000. Polynomial analysis in (C): atgl: PLinear = 0.985, PQuadratic = 0.003; hsl: PLinear = 0.044, PQuadratic = 0.000; lpl: PLinear = 0.003, PQuadratic = 0.013; pparα: PLinear = 0.001, PQuadratic = 0.088; cpt1: PLinear = 0.878, PQuadratic = 0.012; aco: PLinear = 0.829, PQuadratic = 0.735; mcad: PLinear = 0.460, PQuadratic = 0.000. Linear trend and Quadratic trend were analyzed by orthogonal polynomial contrasts.
Compared with the FO diet, the mRNA expression of genes related to lipolysis, such as atgl and lpl, were significantly down-regulated in fish fed the SO diet (P < 0.05) (Figure 4C). With OCT level increasing, the mRNA expression of atgl and hsl showed a significant quadratic curve (P < 0.05), and maximum were observed in fish fed the diet containing 2.1 g/kg OCT and 0.7 g/kg OCT. The mRNA expression of genes related to FA β-oxidation including aco and mcad were significantly lower, while the mRNA expression of cpt1 was significantly higher in fish fed the SO diet than those in the FO diet (P < 0.05). With OCT level increasing, the mRNA expression of cpt1 and mcad showed a significant quadratic curve (P < 0.05), and maximum were observed in fish fed the diet containing 2.1 g/kg OCT.
3.6 Hepatic antioxidant activities
The T-AOC and activities of POD and CAT were significantly decreased when the FO in the diet was completely replaced by SO (P < 0.05) (Figures 5A–C). With the increase of dietary OCT, the T-AOC and activities of POD, CAT and SOD showed a significant quadratic curve (P < 0.05), and maximum were observed in fish fed the diet containing 2.1 g/kg OCT (Figures 5A–D). Compared with the FO diet, the content of GSH, 8-OHdG and MDA were significantly increased in fish fed the SO diet (P < 0.05) (Figures 5E–G). As the OCT level increased, the content of GSH was increased in a significantly quadratic pattern (P < 0.05), while the contents of 8-OHdG and MDA were decreased in significantly linear and quadratic patterns (P < 0.05).
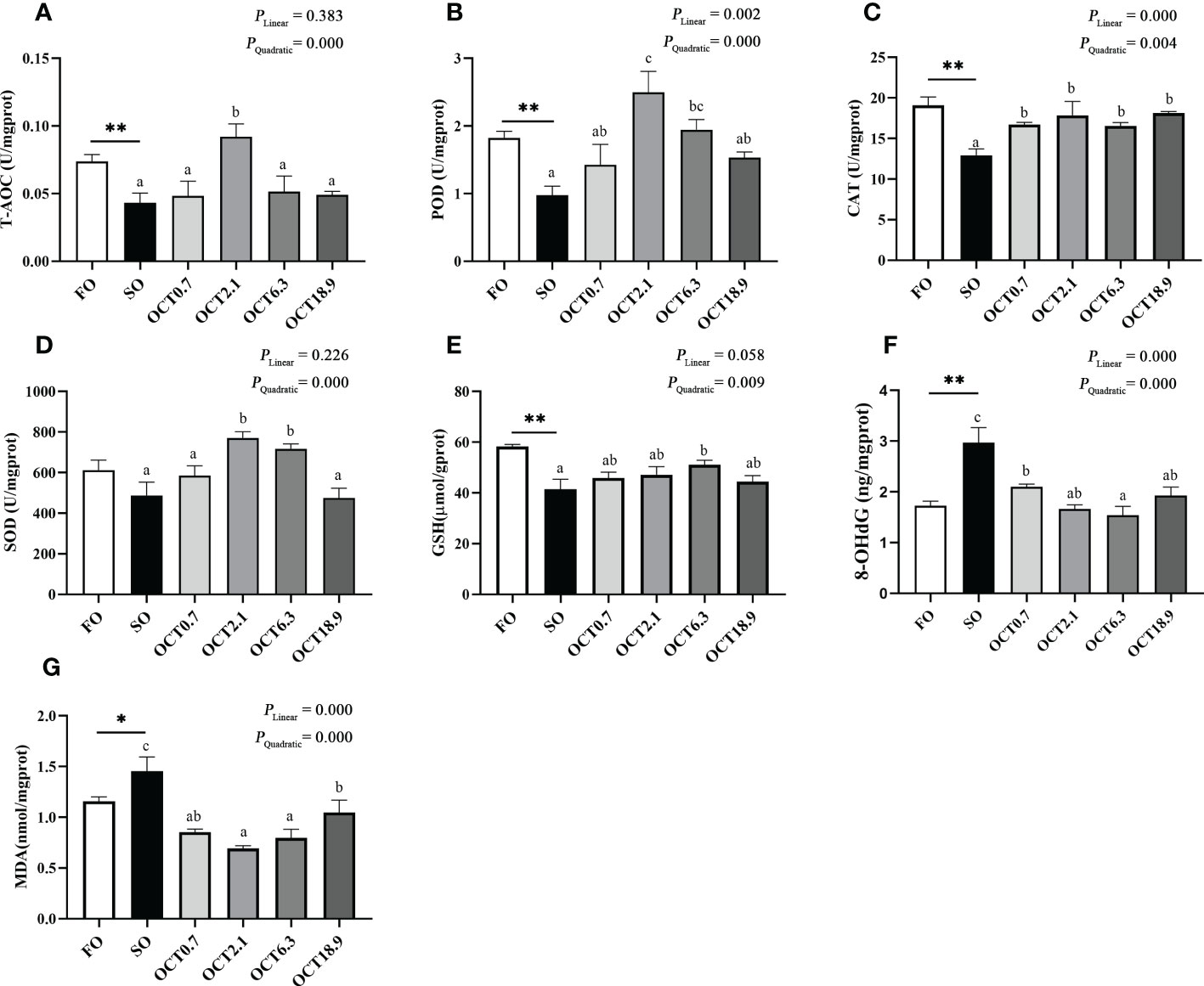
Figure 5 Effects of supplemental octanoate on hepatic antioxidant capacity of large yellow croaker. (A) Total antioxidant capacity, (B) Peroxidase activity, (C) Catalase activity, (D) Superoxide dismutase activity, (E) Reduced glutathione contents, (F) 8-OHdG contents, (G) Malondialdehyde contents. Values were means (n = 3) with their standard errors represented by vertical bars. ∗ represent a significant difference of P < 0.05 between the SO group and the FO group. ∗∗ represent a significant difference of P < 0.01 between the SO group and the FO group. Different letters show significant difference (P < 0.05) among OCT treatment groups. Linear trend and Quadratic trend were analyzed by orthogonal polynomial contrasts.
3.7 The mRNA expression of genes related to inflammation in liver
The mRNA expression of genes related to pro-inflammatory cytokines including tnfα and il1β were significantly up-regulated when the FO in the diet was completely replaced by SO (P < 0.05) (Figure 6). With the increase of dietary OCT, the mRNA expression of tnfα, ifnγ and il1β showed a significant quadratic curve (P < 0.05), and the values in fish fed diets containing 0.7 and 2.1 g/kg OCT were lower than the SO diet. The expression of anti-inflammatory cytokines il10 was up-regulated in a significantly quadratic pattern with the OCT increasing (P < 0.05).
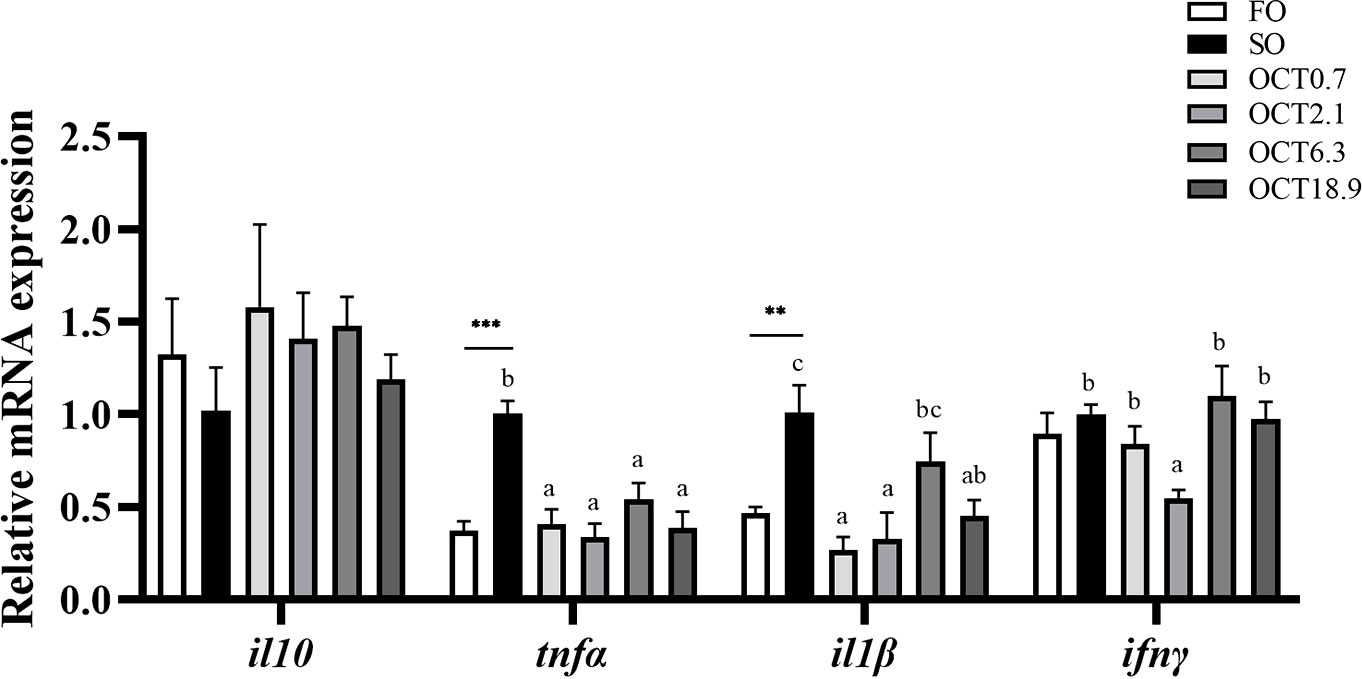
Figure 6 Effects of supplemental octanoate on the expression of inflammation-related genes in the liver of large yellow croaker. Values were means (n = 3) with their standard errors represented by vertical bars. ∗∗ represent a significant difference of P < 0.01 between the SO group and the FO group. ∗∗∗ represent a significant difference of P < 0.001 between the SO group and the FO group. Different letters show significant difference (P < 0.05) among OCT treatment groups. Linear trend and Quadratic trend were analyzed by orthogonal polynomial contrasts. il10, interleukin-10; tnfα, tumor necrosis factor-α; il1β, interleukin-1β; ifnγ, interferon-γ. Polynomial analysis: il10: PLinear = 0.638, PQuadratic = 0.031; tnfα: PLinear = 0.000, PQuadratic = 0.000; il1β: PLinear = 0.019, PQuadratic = 0.001; ifnγ: PLinear = 0.280, PQuadratic = 0.002.
4 Discussion
Dietary high SO levels might cause hepatic fat deposition in aquatic animals (4, 16), which was also confirmed in the present study. Previous study showed that OCT could effectively decrease adiposity and regulate energy balance (28–30). Whereas, the effects of supplemental OCT on hepatic lipid metabolism, antioxidant capacity and inflammatory response in fish have not been explored in detail. In this study, Oil Red O staining results showed that OCT supplementation could decrease the area of LDs induced by the SO diet. Meanwhile, the addition of OCT decreased the hepatic crude lipid, TG, TC, and serum TG and TC contents. The above results suggested that OCT can reduce the accumulation of lipids in the liver. We further explored how OCT affects hepatic lipid metabolism. Compared with the SO diet, the serum LDL-c content was reduced, and the serum HDL-c content was elevated in fish fed the diet containing 2.1 g/kg OCT. LDL-c accumulates a large amount of cholesterol in the arteriae intima and is generally considered to be one of the factors causing atherosclerosis. On the contrary, HDL-c could resist the accumulation, preservation and oxidation of LDL-c, and clear cholesterol back to hepatic from peripheral tissues (31). Similar results were observed in apoE−/− mice, where the addition of OCT reduced the transport of cholesterol to extrahepatic tissues (32). Furthermore, NEFA is the main source of hepatic TG pool, released from adipose tissue by lipolysis, and high concentrations of NEFA can induce muscle and liver insulin resistance and induce dyslipidemia (33). NEFA concentrations can be reduced when fish were fed the diet containing 2.1 g/kg OCT. Consequently, these results demonstrated that OCT could ameliorate hepatic fat deposition and improve dyslipidemia induced by high-level SO diets.
To further elucidate the mechanism whereby OCT reduces the content of hepatic lipids, the mRNA expression of genes related to lipid metabolism was examined. In this study, the mRNA expression of transcription factors (srebp1 and cebpa), genes related to de novo synthesis of fatty acids (acc1, scd1, and fas) and lipid synthesis (dagt1) were down-regulated in fish fed diets with OCT. Transcription factors (srebp1 and cebpa) are essential for the coordinated regulation of lipid synthesis, regulating normal adipocyte differentiation and targeting molecules such as stearoyl-CoA desaturase-1 (SCD-1), acetyl-CoA carboxylase (ACC) and fatty acid synthetase (FAS) (34). Similarly, a reduction in the activity of DGAT2 and ACC mediated by OCT was observed in 3T3-L1 cells and human adipocytes (28). The down-regulation of these genes and proteins might be related to OCT-mediated PPARγ inactivation (35). With the addition of OCT, the mRNA expression of these genes first decreased and then increased, showing a significant quadratic trend, indicating that suitable concentration of OCT could alleviate lipid deposition, while excessive OCT may reverse this result, similar results were found in previous study (36). Besides affecting lipid synthesis, OCT also significantly altered the mRNA expression of FA transport-related genes. Compared with the FO diet, the mRNA expression of cd36 in fish fed the SO diet was significantly up-regulated, and the addition of OCT reduced the expression of cd36. Similar results were found in H9c2 cells, OCT supplementation can restore the AVP (arginine vasopressin) induced down-regulation of cd36 expression (37). VLDL assembly requires apoB-100 to mobilize triglycerides into the microsomal lumen (38). The supplementation of OCT also significantly up-regulated the mRNA expression of apob100, promoting hepatic VLDL assembly and secretion. Compared with the SO diet, the mRNA expression of genes involved in lipolysis (atgl, hsl, and lpl) and FA β-oxidation (cpt1, aco and mcad) were up-regulated in fish fed diets containing 0.7 and 2.1 g/kg OCT. MCAD is a key enzyme in the first step of the β-oxidation process of MCFA (39). A previous study also found that OCT significantly increased lipid oxidation-related gene expression and the protein expression of MCAD in AML12 cells (40). The possible reason is that compared with long-chain fatty acids, MCFA can enter the mitochondria independently of the carnitine transport system and be oxidized preferentially, which is crucial for the oxidative decomposition of FAs (41). Collectively, these results suggested that OCT could reduce the de novo synthesis of FAs, regulate the expression of FA transport-related genes, and promote lipolysis and FA β-oxidation thereby alleviating hepatic lipid deposition.
Lipid metabolism disorders may increase the production of reactive oxygen species (42). The imbalance of oxidative and antioxidant systems induces oxidative stress. T-AOC is the total antioxidant capacity, an effective marker of oxidative stress (43). Compared with the FO diet, T-AOC was significantly reduced in fish fed the SO diet, while it was improved with OCT supplementation. POD, SOD and CAT are key antioxidant enzymes to eliminate ROS accumulation (43). In this study, OCT treatment improved the antioxidant enzymes activity, which is consistent with studies in rats and AML12 cells (44–46). Excessive oxidative products damage biological molecules such as proteins, nucleic acids and lipids, impair their biological function, and trigger hepatic injury. MDA is the product of intracellular lipid peroxidation, and 8-OHDG is a marker of DNA oxidative damage (47). Hepatic MDA and 8-OHDG contents were decreased in fish fed with OCT. In summary, our results revealed the protective effect of OCT on hepatic oxidative stress induced by high SO levels diet.
Hepatic lipid accumulation may also lead to hepatocyte injury and inflammation (48). Supplementation of 2.1 g/kg OCT improved hepatocyte swelling and nuclear migration, and decreased serum ALT and AST levels, which are important biomarkers of hepatic injury. With the addition of OCT, Serum ALT and AST contents increased in fish fed the diet containing OCT 6.3 g/kg, and decreased in fish fed the diet containing OCT 18.9 g/kg, but there was no significant difference between them (P > 0.05). This result is consistent with subsequent inflammation-related genes (tnf-α, il-1β and ifnγ) expression, suggesting that liver damage might be mitigated by reduced inflammatory response. A previous study also found OCT prevented LPS-induced acute liver injury in rats (49). IL-1β, TNF-α and IFNγ are inflammatory cytokines, which initiate the production of a series of inflammatory mediators and produce inflammatory responses (48). In this study, the mRNA expression of tnf-α and il-1β were significantly up-regulated when the FO in the diet was completely replaced by SO, causing the occurrence of hepatic inflammatory response. After OCT intervention, the expression of il-1β, ifnγ and tnf-α were down-regulated, indicating that OCT could play an anti-inflammatory role, which is consistent with the results in mammals (50, 51). These results demonstrated that OCT could ameliorate hepatic injury and inflammation induced by high SO diets.
In conclusion, a concentration range of 0.7 and 2.1 g/kg OCT can significantly reduce hepatic lipid accumulation, relieve hepatic oxidative stress and regulate inflammatory response in large yellow croaker fed with high SO level diets. Collectively, OCT supplementation might have the potential to alleviate lipid metabolism disorders and hepatic damage, and provide a new way to improve hepatic lipid deposition in fish.
Data availability statement
The original contributions presented in the study are included in the article/Supplementary Material. Further inquiries can be directed to the corresponding author.
Ethics statement
The animal study was reviewed and approved by The Animal Experiment Ethics Committee of Ocean University of China and the Management Rule of Laboratory Animals (Chinese Order No. 676 of the State Council, revised 1 March 2017).
Author contributions
MZ and QA designed the experiments, performed the main experiments and wrote the original draft. ZZ, YTL, and WZ conducted other experiments. MZ, YT, YG, and JZ analyzed the data. FC, GL, HZ, YRL, and KM revised the manuscript. All authors contributed to the article and approved the submitted version.
Funding
This research is supported by Key Program of National Natural Science Foundation of China [grant number: 31830103], the earmarked fund for CARS-47 [grant number: CARS47-11], the National Science Fund for Distinguished Young Scholars of China [grant number: 31525024], Scientific and Technological Innovation of Blue Granary [grant number: 2018YFD0900402].
Acknowledgments
We thanks FuFa Aquatic Products Co., Ltd. (Ningde, China) for providing experimental animals and facilities. We also appreciate Jiamin Li, Xianyong Bu, Wei Fang, Zengqi Zhao, Jie Sun, Qiangde Liu, Xiuneng Wang, Miao Weng, Chenxiang Zhang, Zhijie Shi, Zhihao Zhang, Qiuxin Yan and Jiahui Liu for their experimental assistance.
Conflict of interest
Author HZ is employed by Guangdong Evergreen Feed Industry Co., Ltd.
The remaining authors declare that the research was conducted in the absence of any commercial or financial relationships that could be construed as a potential conflict of interest.
Publisher’s note
All claims expressed in this article are solely those of the authors and do not necessarily represent those of their affiliated organizations, or those of the publisher, the editors and the reviewers. Any product that may be evaluated in this article, or claim that may be made by its manufacturer, is not guaranteed or endorsed by the publisher.
Supplementary material
The Supplementary Material for this article can be found online at: https://www.frontiersin.org/articles/10.3389/fimmu.2023.1162633/full#supplementary-material
References
1. Liu C, Wang J, Ma Z, Li T, Xing W, Jiang N, et al. Effects of totally replacing dietary fish oil by linseed oil or soybean oil on juvenile hybrid Sturgeon, Acipenser Baeribrandt♀×A. schrenckiibrandt♂. Aquaculture Nutr (2018) 24(1):184–94. doi: 10.1111/anu.12546
2. Liu YL, Yan Y, Han Z, Zheng YC, Wang X, Zhang MY, et al. Comparative effects of dietary soybean oil and fish oil on the growth performance, fatty acid composition and lipid metabolic signaling of grass carp, Ctenopharyngodon idella. AQUACULTURE Rep (2022) 22:101002. doi: 10.1016/j.aqrep.2021.101002
3. Chen YF, Sun ZZ, Liang ZM, Xie YD, Su JL, Luo QL, et al. Effects of dietary fish oil replacement by soybean oil and l-carnitine supplementation on growth performance, fatty acid composition, lipid metabolism and liver health of juvenile largemouth bass, Micropterus salmoides. AQUACULTURE (2020) 516:734596. doi: 10.1016/j.aquaculture.2019.734596
4. Li Y, Liang X, Zhang Y, Gao J. Effects of different dietary soybean oil levels on growth, lipid deposition, tissues fatty acid composition and hepatic lipid metabolism related gene expressions in blunt snout bream (Megalobrama amblycephala) juvenile. Aquaculture (2016) 451:16–23. doi: 10.1016/j.aquaculture.2015.08.028
5. Peng M, Xu W, Mai K, Zhou H, Zhang Y, Liufu Z, et al. Growth performance, lipid deposition and hepatic lipid metabolism related gene expression in juvenile turbot (Scophthalmus maximus l.) fed diets with various fish oil substitution levels by soybean oil. Aquaculture (2014) 433:442–9. doi: 10.1016/j.aquaculture.2014.07.005
6. Mu H, Shen H, Liu J, Xie F, Zhang W, Mai K. High level of dietary soybean oil depresses the growth and anti-oxidative capacity and induces inflammatory response in Large yellow croaker Larimichthys crocea. Fish Shellfish Immunol (2018) 77:465–73. doi: 10.1016/j.fsi.2018.04.017
7. Guo W, Choi J-K, Kirkland JL, Corkey BE, Hamilton JA. Esterification of free fatty acids in adipocytes: A comparison between octanoate and oleate. Biochem J (2000) 349(2):463–71. doi: 10.1042/bj3490463
8. Shinohara H, Ogawa A, Kasai M, Aoyama T. Effect of randomly interesterified triacylglycerols containing medium- and long-chain fatty acids on energy expenditure and hepatic fatty acid metabolism in rats. Bioscience Biotechnology Biochem (2005) 69(10):1811–8. doi: 10.1271/bbb.69.1811
9. Terada S, Sekine S, Aoyama T. Dietary intake of medium- and long-chain triacylglycerols prevents the progression of hyperglycemia in diabetic Ob/Ob mice. J Oleo Sci (2015) 64(6):683–8. doi: 10.5650/jos.ess14287
10. Papamandjaris AA, MacDougall DE, Jones PJH. Medium chain fatty acid metabolism and energy expenditure: Obesity treatment implications. Life Sci (1998) 62(14):1203–15. doi: 10.1016/S0024-3205(97)01143-0
11. Geng S, Zhu W, Xie C, Li X, Wu J, Liang Z, et al. Medium-chain triglyceride ameliorates insulin resistance and inflammation in high fat diet-induced obese mice. Eur J Nutr (2016) 55(3):931–40. doi: 10.1007/s00394-015-0907-0
12. Libran-Perez M, Lopez-Patino MA, Miguez JM, Soengas JL. In vitro response of putative fatty acid-sensing systems in rainbow trout liver to increased levels of oleate or octanoate. Comp Biochem Physiol A Mol Integr Physiol (2013) 165(2):288–94. doi: 10.1016/j.cbpa.2013.03.024
13. Cansiz D, Unal I, Ustundag UV, Alturfan AA, Altinoz MA, Elmaci I, et al. Caprylic acid ameliorates rotenone induced inflammation and oxidative stress in the gut-brain axis in zebrafish. Mol Biol Rep (2021) 48(6):5259–73. doi: 10.1007/s11033-021-06532-5
14. Cui K, Li X, Chen Q, Li Q, Gao S, Tan P, et al. Effect of replacement of dietary fish oil with four vegetable oils on prostaglandin E(2) synthetic pathway and expression of inflammatory genes in marine fish Larimichthys crocea. Fish Shellfish Immunol (2020) 107(Pt B):529–36. doi: 10.1016/j.fsi.2020.09.038
15. Tan P, Li X, Xiang X, Dong X, Li S, Mai K, et al. Adipose tissue contributes to hepatic pro-inflammatory response when dietary fish oil is replaced by vegetable oil in Large yellow croaker (Larimichthys crocea): An ex vivo study. Fish Shellfish Immunol (2019) 84:955–61. doi: 10.1016/j.fsi.2018.10.086
16. Xu N, Ding T, Liu Y, Zheng W, Liu Q, Yin Z, et al. Effects of dietary tributyrin on growth performance, body composition, serum biochemical indexes and lipid metabolism-related genes expression of juvenile Large yellow croaker (Larimichthys crocea) fed with high level soybean oil diets. Aquaculture Nutr (2020) 27(2):395–406. doi: 10.1111/anu.13192
17. Yang B, Zhou Y, Wu M, Li X, Mai K, Ai Q. Omega-6 polyunsaturated fatty acids (Linoleic acid) activate both autophagy and antioxidation in a synergistic feedback loop Via Tor-dependent and Tor-independent signaling pathways. Cell Death Dis (2020) 11(7):607. doi: 10.1038/s41419-020-02750-0
18. Zhang Z, Tang Y, Fang W, Cui K, Xu D, Liu G, et al. Octanoate alleviates dietary soybean oil-induced intestinal physical barrier damage, oxidative stress, inflammatory response and microbial dysbiosis in Large yellow croaker (Larimichthys crocea). Front Immunol (2022) 13:892901. doi: 10.3389/fimmu.2022.892901
19. Li J, Xu W, Lai W, Kong A, Zhang Z, Pang Y, et al. Effect of dietary methionine on growth performance, lipid metabolism and antioxidant capacity of Large yellow croaker (Larimichthys crocea) fed with high lipid diets. Aquaculture (2021) 536:736388. doi: 10.1016/j.aquaculture.2021.736388
20. Li X, Ji R, Cui K, Chen Q, Chen Q, Fang W, et al. High percentage of dietary palm oil suppressed growth and antioxidant capacity and induced the inflammation by activation of tlr-Nf-Kappab signaling pathway in Large yellow croaker (Larimichthys crocea). Fish Shellfish Immunol (2019) 87:600–8. doi: 10.1016/j.fsi.2019.01.055
21. Fang W, Chen Q, Cui K, Chen Q, Li X, Xu N, et al. Lipid overload impairs hepatic vldl secretion Via oxidative stress-mediated pkcdelta-Hnf4alpha-Mtp pathway in Large yellow croaker (Larimichthys crocea). Free Radic Biol Med (2021) 172:213–25. doi: 10.1016/j.freeradbiomed.2021.06.001
22. Xu D, Xiang X, Li X, Xu N, Zhang W, Mai K, et al. Effects of dietary vegetable oils replacing fish oil on fatty acid composition, lipid metabolism and inflammatory response in adipose tissue of Large yellow croaker (Larimichthys crocea). J Mar Sci Eng (2022) 10(11):1760. doi: 10.3390/jmse10111760
23. Yan J, Liao K, Wang T, Mai K, Xu W, Ai Q. Dietary lipid levels influence lipid deposition in the liver of Large yellow croaker (Larimichthys crocea) by regulating lipoprotein receptors, fatty acid uptake and triacylglycerol synthesis and catabolism at the transcriptional level. PloS One (2015) 10(6):e0129937. doi: 10.1371/journal.pone.0129937
24. Cai Z, Feng S, Xiang X, Mai K, Ai Q. Effects of dietary phospholipid on lipase activity, antioxidant capacity and lipid metabolism-related gene expression in Large yellow croaker larvae (Larimichthys crocea). Comp Biochem Physiol B Biochem Mol Biol (2016) 201:46–52. doi: 10.1016/j.cbpb.2016.06.007
25. Zuo R, Ai Q, Mai K, Xu W, Wang J, Xu H, et al. Effects of dietary docosahexaenoic to eicosapentaenoic acid ratio (Dha/Epa) on growth, nonspecific immunity, expression of some immune related genes and disease resistance of Large yellow croaker (Larmichthys crocea) following natural infestation of parasites (Cryptocaryon irritans). Aquaculture (2012) 334-337:101–9. doi: 10.1016/j.aquaculture.2011.12.045
26. Tang Y, Zhang Z, Zhang L, He Y, Xu N, Fang W, et al. Effects of supplemental glycerol monolaurate on growth, hepatic lipid metabolism, antioxidant capacity and mitochondrial function of Large yellow croaker (Larimichthys crocea) fed diets with high soybean oil level. Aquaculture (2023) 564:739024. doi: 10.1016/j.aquaculture.2022.739024
27. Wang T, Yang B, Ji R, Xu W, Mai K, Ai Q. Omega-3 polyunsaturated fatty acids alleviate hepatic steatosis-induced inflammation through Sirt1-mediated nuclear translocation of nf-kappab P65 subunit in hepatocytes of Large yellow croaker (Larmichthys crocea). Fish Shellfish Immunol (2017) 71:76–82. doi: 10.1016/j.fsi.2017.09.064
28. Guo W, Lei TG, Wang T, Corkey BE, Han JR. Octanoate inhibits triglyceride synthesis in 3T3-L1 and human adipocytes. J OF Nutr (2003) 133(8):2512–8. doi: 10.1093/jn/133.8.2512
29. Haynes VR, Michael NJ, van den Top M, Zhao FY, Brown RD, De Souza D, et al. A neural basis for octanoic acid regulation of energy balance. Mol Metab (2020) 34:54–71. doi: 10.1016/j.molmet.2020.01.002
30. St-Onge MP, Ross R, Parsons WD, Jones PJ. Medium-chain triglycerides increase energy expenditure and decrease adiposity in overweight men. Obes Res (2003) 11(3):395–402. doi: 10.1038/oby.2003.53
31. Geng Y, Liu Y, Chen Y, Zhang Z, Wang L, Li X, et al. Association of ldlc to hdlc ratio with carotid plaques in a community-based population with a high stroke risk: A cross-sectional study in China. Clin Biochem (2021) 88:43–8. doi: 10.1016/j.clinbiochem.2020.11.001
32. Zhang X, Xue C, Xu Q, Zhang Y, Li H, Li F, et al. Caprylic acid suppresses inflammation Via Tlr4/Nf-kappab signaling and improves atherosclerosis in apoe-deficient mice. Nutr Metab (Lond) (2019) 16:40. doi: 10.1186/s12986-019-0359-2
33. Koutsari C, Jensen MD. Thematic review series: Patient-oriented research. free fatty acid metabolism in human obesity. J Lipid Res (2006) 47(8):1643–50. doi: 10.1194/jlr.R600011-JLR200
34. Cho H-H, Jang S-H, Won C, Kim C-H, Kim H-D, Kim TH, et al. Derhamnosylmaysin inhibits adipogenesis Via inhibiting expression of PPARγ; and C/EbPα in 3T3-L1 cells. Molecules (2022) 27(13):4232. doi: 10.3390/molecules27134232
35. Guo W, Xie W, Han J. Modulation of adipocyte lipogenesis by octanoate: Involvement of reactive oxygen species. Nutr Metab (Lond) (2006) 3:30. doi: 10.1186/1743-7075-3-30
36. Marten B, Pfeuffer M, Schrezenmeir J. Medium-chain triglycerides. Int DAIRY J (2006) 16(11):1374–82. doi: 10.1016/j.idairyj.2006.06.015
37. Ismael S, Nair RR. Reactivation of fatty acid oxidation by medium chain fatty acid prevents myocyte hypertrophy in H9c2 cell line. Mol Cell Biochem (2021) 476(1):483–91. doi: 10.1007/s11010-020-03925-1
38. Yao Z, Zhou H, Figeys D, Wang Y, Sundaram M. Microsome-associated lumenal lipid droplets in the regulation of lipoprotein secretion. Curr Opin Lipidol (2013) 24(2):160–70. doi: 10.1097/MOL.0b013e32835aebe7
39. Puca F, Yu F, Bartolacci C, Pettazzoni P, Carugo A, Huang-Hobbs E, et al. Medium-chain acyl-coa dehydrogenase protects mitochondria from lipid peroxidation in glioblastoma. Cancer Discovery (2021) 11(11):2904–23. doi: 10.1158/2159-8290.CD-20-1437
40. Chen Q, Du J, Cui K, Fang W, Zhao Z, Chen Q, et al. Acetyl-coa derived from hepatic mitochondrial fatty acid beta-oxidation aggravates inflammation by enhancing P65 acetylation. iScience (2021) 24(11):103244. doi: 10.1016/j.isci.2021.103244
41. Zhao W, Varghese M, Vempati P, Dzhun A, Cheng A, Wang J, et al. Caprylic triglyceride as a novel therapeutic approach to effectively improve the performance and attenuate the symptoms due to the motor neuron loss in als disease. PloS One (2012) 7(11):e49191. doi: 10.1371/journal.pone.0049191
42. Chen Z, Tian R, She Z, Cai J, Li H. Role of oxidative stress in the pathogenesis of nonalcoholic fatty liver disease. Free Radic Biol Med (2020) 152:116–41. doi: 10.1016/j.freeradbiomed.2020.02.025
43. Dudzinska E, Gryzinska M, Ognik K, Gil-Kulik P, Kocki J. Oxidative stress and effect of treatment on the oxidation product decomposition processes in ibd. Oxid Med Cell Longev (2018) 2018:7918261. doi: 10.1155/2018/7918261
44. Sengupta A, Ghosh M, Bhattacharyya DK. Antioxidative effect of rice bran oil and medium chain fatty acid rich rice bran oil in arsenite induced oxidative stress in rats. J Oleo Sci (2014) 63(11):1117–24. doi: 10.5650/jos.ess14128
45. Wang D, Chen J, Sun H, Chen W, Yang X. Mcfa alleviate H2O2 -induced oxidative stress in Aml12 cells Via the Erk1/2/Nrf2 pathway. Lipids (2022) 57(3):153–62. doi: 10.1002/lipd.12339
46. Zhao J, Hu J, Ma X. Sodium caprylate improves intestinal mucosal barrier function and antioxidant capacity by altering gut microbial metabolism. Food Funct (2021) 12(20):9750–62. doi: 10.1039/d1fo01975a
47. Samarghandian S, Azimi-Nezhad M, Farkhondeh T, Samini F. Anti-oxidative effects of curcumin on immobilization-induced oxidative stress in rat brain, liver and kidney. BioMed Pharmacother (2017) 87:223–9. doi: 10.1016/j.biopha.2016.12.105
48. Koyama Y, Brenner DA. Liver inflammation and fibrosis. J Clin Invest (2017) 127(1):55–64. doi: 10.1172/JCI88881
49. He K, Cao C, Xu X, Ye Z, Ma X, Chen W, et al. Octanoic acid-rich enteral nutrition prevented lipopolysaccharide-induced acute liver injury through c-jun n-terminal kinase-dependent autophagy. JPEN J Parenter Enteral Nutr (2022) 46(6):1353–60. doi: 10.1002/jpen.2297
50. Miles EA, Calder PC. Fatty acids, lipid emulsions and the immune and inflammatory systems. World Rev Nutr Diet (2015) 112:17–30. doi: 10.1159/000365426
Keywords: octanoate, large yellow croaker, lipid metabolism, antioxidant capacity, inflammatory response
Citation: Zhao M, Zhang Z, Liu Y, Zhang W, Gong Y, Tang Y, Chen F, Zhang J, Liu G, Zhang H, Li Y, Mai K and Ai Q (2023) Effects of supplemental octanoate on hepatic lipid metabolism, serum biochemical indexes, antioxidant capacity and inflammation-related genes expression of large yellow croaker (Larimichthys crocea) fed with high soybean oil diet. Front. Immunol. 14:1162633. doi: 10.3389/fimmu.2023.1162633
Received: 09 February 2023; Accepted: 13 March 2023;
Published: 27 March 2023.
Edited by:
Tor Gjøen, University of Oslo, NorwayReviewed by:
Linghong Miao, Freshwater Fisheries Research Center (CAFS), ChinaShuang Zhang, Guangdong Ocean University, China
Copyright © 2023 Zhao, Zhang, Liu, Zhang, Gong, Tang, Chen, Zhang, Liu, Zhang, Li, Mai and Ai. This is an open-access article distributed under the terms of the Creative Commons Attribution License (CC BY). The use, distribution or reproduction in other forums is permitted, provided the original author(s) and the copyright owner(s) are credited and that the original publication in this journal is cited, in accordance with accepted academic practice. No use, distribution or reproduction is permitted which does not comply with these terms.
*Correspondence: Qinghui Ai, cWhhaUBvdWMuZWR1LmNu