- 1Hematology Department, Institut Català d’Oncologia, Hospital Dr. Josep Trueta, Institut d’Investigació Biomèdica de Girona (IDIBGI), Josep Carreras Research Institute, Girona, Universitat de Girona, Girona, Spain
- 2Girona Cancer Registry, Oncology Coordination Plan, Catalan Institute of Oncology (RTH) ICO-ICS, Centre CIBER of Epidemiology and Public Health (CIBERESP), Girona, Spain
- 3Clinical Hematology Department, Institut Català d’Oncologia, L’Hospitalet, IDIBELL, Universitat de Barcelona, Hospitalet de LLobregat, Spain
- 4Hematology Department, Institut Català d’Oncologia, Hospital Germans Trias i Pujol, Josep Carreras Research Institute, Badalona, Barcelona, Spain
- 5Hematology Department, Institut Català d’Oncologia, Hospital Joan XXIII, Universitat Rovira i Virgili (URV), Tarragona, Spain
Immune dysfunction in patients with multiple myeloma (MM) affects both the innate and adaptive immune system. Molecules involved in the immune checkpoint pathways are essential to determine the ability of cancer cells to escape from the immune system surveillance. However, few data are available concerning the role of these molecules in predicting the kinetics of progression of MM. We retrospectively analysed polymorphisms of CTLA4 (rs231775 and rs733618), BTLA (rs9288953), CD28 (rs3116496), PD-1 (rs36084323 and rs11568821) and LAG-3 (rs870849) genes in 239 patients with newly diagnosed MM. Patients with a CTLA4 rs231775 AA/AG genotype showed a median progression-free survival (PFS) significantly lower than those with GG genotype (32.3 months versus 96.8 months respectively; p: 0.008). The 5-year PFS rate was 25% for patients with grouped AA and AG genotype vs 55.4% for patients with GG genotype. Multivariate analysis confirmed the CTLA4 rs231775 genotype as an independent risk factor for PFS (Hazard Ratio (HR): 2.05; 95% CI: 1.0-6.2; p: 0.047). Our results suggest that the CTLA4 genotype may identify patients with earlier progression of MM. This polymorphism could potentially be used as a prognostic biomarker.
Introduction
Multiple myeloma (MM) is a neoplastic plasma-cell disorder that is characterized by clonal proliferation of malignant plasma cells in the bone marrow, monoclonal protein in the blood or urine and associated organ dysfunction. MM develops from a premalignant condition monoclonal gammopathy of undetermined significance (MGUS), progressing to smoldering multiple myeloma and active MM. Progressive immune impairment is a feature of this MM evolution, allowing neoplastic plasma cells to escape from immune surveillance, promoting disease growth and resistance to therapy (1, 2). Survival of patients with MM has improved during the past decade with the introduction of immunomodulatory drugs, proteasome inhibitors and monoclonal antibodies. Unfortunately, it remains an incurable disease characterized by recurring relapses due to residual, drug-resistant, myeloma cells that survive to the treatment (3).
The adaptive immune system can recognize and attack malignant cells. It is generally considered that T cells specific to tumour antigens play a crucial role in cancer elimination. T cell activation is initiated through tumour antigen recognition by the T cell receptor (TCR) and is regulated by a balance between activating and inhibitory intracellular signals. These signals are initiated by engagement of co-stimulatory and co-inhibitory receptors with their cognate ligands. The balance between positive and negative co-signals determines the functionality of T cells during immunity and tolerance, preventing the appearance of autoimmune phenomena. The ability of cancer cells to evade anti-tumour T-cell activity has been recognised as an important mechanism of cancer progression. Malignant cells can enhance the expression of inhibitory immune checkpoint molecules to avoid immune recognition and elimination (4).
The pathogenesis of the immune dysregulation in MM is associated with impaired cellular immunity including profound T cell alterations with loss of effector function and increased number of immunosuppressive regulatory T cells (Treg) in the bone marrow (2). A major role in this development of the immunosuppressive state in MM patients has been attributed to an increased expression of immune checkpoint molecules that negatively regulate T-cell function, such as PDCD1, CTLA4, BTLA and T-cell immunoglobulin and ITIM domains (TIGIT) on T cells (5–7).
Genetic variants of immune checkpoint molecules have been identified as risk factors for cancer development (8). Several studies have shown a relationship between genetic polymorphisms in co-stimulatory/inhibitory molecules and susceptibility to the development of hematologic malignancies, such as non-Hodgkin lymphomas (9, 10), chronic lymphocytic leukemia (11, 12), and MM (13–17). In addition, some studies have described the correlation between CTLA4 and PDCD1 genotypes and the clinical outcome after allogeneic stem cell transplantation (18, 19) or with the risk of acute myeloid leukemia progression after achieving complete remission (20).
However, few data are available concerning the clinical impact of genetic polymorphisms in co-stimulatory/inhibitory molecules on clinical outcome in patients with MM. In our study we intend to evaluate whether the presence of genetic variations of these checkpoint molecules is associated with an increased risk of progression in patients with MM.
Patients and methods
We retrospectively analysed 239 patients with newly diagnosed MM who were eligible for first-line treatment and followed at the Catalan Institute of Oncology centers between 1995 and 2018. DNA was obtained from peripheral blood or bone marrow samples at different stages of the disease. Biological samples and clinical data were processed following standard operating procedures and approved by the Ethics and Scientific Committees. All patients signed an informed consent and the study met with the recommendations of the Helsinki declaration. Samples and data from patients included in this study were provided by the IDIBGI Biobank (Biobanc IDIBGI, B.0000872), integrated in the Spanish National Biobanks Network and they were processed following standard operating procedures with the appropriate approval of the Ethics and Scientific Committees. Clinical characteristics of patients and first-line treatments are summarized in Table 1.
In addition, a second cohort of 62 patients with newly diagnosis of smouldering MM was specifically analysed to correlate the genotype in the studied polymorphisms with the time to progression to symptomatic MM.
Genotype analysis
DNA was extracted from 200µl of whole blood using a QIAamp DNA Blood Mini Kit (Qiagen, GmbH, Hilden, Germany) according to the manufacturer’s instructions and stored at -80°C until use.
We analysed polymorphisms of CTLA4 (rs231775 and rs733618), BTLA (rs9288953), CD28 (rs3116496), PDCD1 (rs36084323 and rs11568821) and LAG3 (rs870849) genes. The genotype for these polymorphisms was determined via allelic discrimination plots on Applied Biosystems™ QuantStudio™ 7 Flex Real-Time PCR System by using TaqMan® SNP Genotyping Assays real time PCR according to the manufacturer’s instruction.
Statistical analyses
Allele frequencies and genotypes were formulated by direct counting. Hardy-Weinberg equation (p2 + 2pq + q2 = 1) was used to measure whether the observed genotype frequencies in the studied population differ from the predicted frequencies. Homogeneity between genotype groups was evaluated using the chi-square test or Fisher’s exact test for qualitative variables and Student’s test for continuous variables. Kaplan-Meier curves were derived to determine overall survival (OS) and progression free survival (PFS) and curves were compared using the log-rank test. A two-sided p value of 0.05 or lower was considered to be statistically significant. Multivariate analysis was performed using the Cox regression model. All the variables with a p value at or below 0.2 in the univariate analysis were included in the multivariate analysis.
Results
CTLA4, BTLA, CD28, PDCD1 and LAG3 genotype distribution.
Table 2 shows the genotypes distribution for each analysed polymorphism. The genotype frequencies were comparable to the previously described in Caucasian population.
Homogeneity between genotype groups
The comparison of clinical prognostic factors at diagnosis between genetic groups for each polymorphism showed a balanced distribution for age, sex, type of monoclonal protein, former history of monoclonal gammopathy of unknown significance (MGUS), International Staging System (ISS), cytogenetics, serum LDH levels or kidney failure. Moreover, the proportion of patients receiving an autologous peripheral blood stem cell transplant (PBSCT) was also comparable within genetic groups. Tables S1, S2 show the comparison of clinical characteristics of the symptomatic MM patients according to the genetic groups according to their BTLA (rs9288953), CD28 (rs3116496), PDCD1 (rs36084323 and rs11568821), LAG3 (rs870849) and CTLA4 (rs231775 and rs733618) genotypes.
Correlation between immune checkpoint molecules genotype and progression to symptomatic myeloma
When analysing the cohort of 62 patients with newly diagnosed smouldering MM, we did not find any correlation between the analysed genotypes and the time to receive a first line of therapy. In this cohort, the median the time to receive therapy was 22.8 months for patients with CTLA4 rs231775 GG genotype versus 102 months for those patients with the AA+AG genotype (p: 0.7). Similar results were obtained when analyzing the impact of the CTLA4 rs733618 (CC+CT: 174.5 months vs. TT: 65.2 months; p: 0.4), BTLA rs9288953 (CC+CT: 65.2 months vs. TT 135.1 months; p: 0.6), CD28 rs3116496 (CC+CT: 61.6 months vs. TT: 135.1 months; p: 0.7), PDCD1 rs36084323 (CC+CT: 102 months vs. TT: not reached; p: 0.6) and LAG3 rs870849 (TT+CT: 61.6 months vs. CC: 122.6 months; p: 0.3) polymorphisms.
Immune checkpoint molecules genotype and clinical outcome after treatment
We did not find any significant correlation between polymorphisms of BTLA (rs9288953), CD28 (rs3116496), PDCD1 (rs36084323 and rs11568821), LAG3 (rs870849) or CTLA4 (rs733618) and overall survival or progression-free survival (PFS) (Table 3).
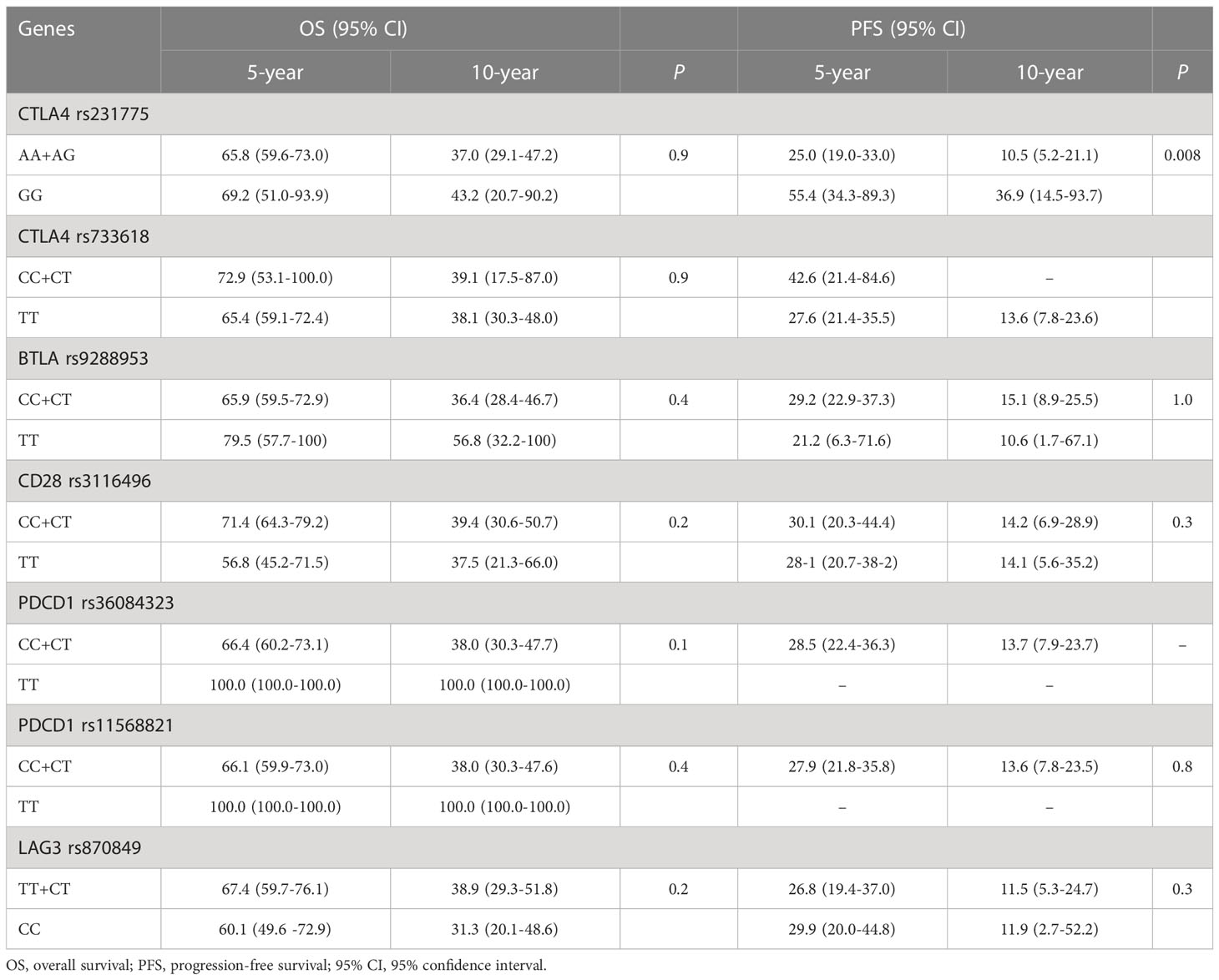
Table 3 Overall and progression-free survival of multiple myeloma patients according to immune checkpoint genotypes.
However, the analysis according to the CTLA4 rs231775 polymorphism revealed that the presence of the AA+AG genotype was associated with a median PFS significantly shorter than the GG genotype: 32.3 months (95% confidence interval (95% CI): 26.4 – 36.1) and 96.8 months (95% CI: 44.5 – not reached) respectively (p: 0.008) (Figure 1). Moreover, the median time to subsequent myeloma therapy was significantly lower for patients with CTLA4 rs231775 AA and AG genotype (36.3 months; 95% CI: 33.5-43) than in the patients with GG genotype (not reached; 95% CI 44.6-not reached) (p: 0.01).
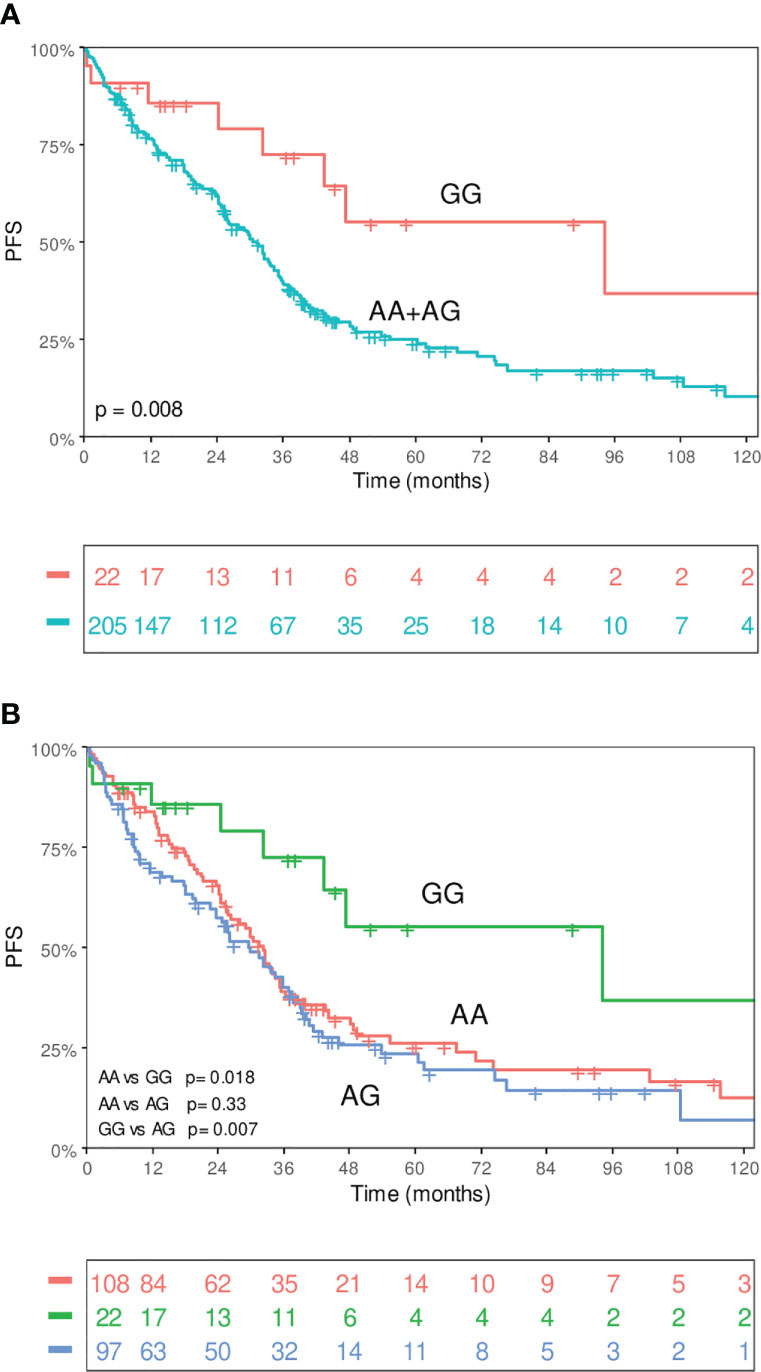
Figure 1 Kaplan-Meier curves of PFS according to CTLA4 rs2311775. (A) grouped phenotypes and (B) independent genotypes.
The 5-year PFS rate was 25% for patients with grouped AA and AG genotype vs 55.4% for patients with GG genotype. Male gender (30.8 vs 36.4 months; p: 0.03), age ≥ 69 years (30.2 vs 36.8 months; p: 0.02), high-risk cytogenetics (18.6 vs 33.5 months; p: 0.01), high ISS (24.2 vs 38.5 months; p: 0.02) and not receive a hematopoietic stem cell transplantation (39.6 vs 26.6 months; p < 0.001) were also risk factors for lower PFS.
In the multivariate analysis, the CTLA4 rs231775 polymorphism remained as an independent risk factor for PFS (Hazard Ratio (HR): 2.05; 95% CI: 1.0-6.2; p: 0.047). Table 4 shows the results of the multivariate analysis.
When performing subgroup analysis of patients with ISS I and ISS II, we detected more evident differences in PFS depending on the CTLA4 genotype. Interestingly, we observed that patients with GG genotype showed a much longer PFS (median not reached) than patients with AA+AG genotype, who had a median PFS of 34.3 months (95%CI: 31.4 – 39.8; p: 0.0062) (Figure 2).
In patients that received an autologous peripheral blood stem cell transplant (PBSCT) the AA+AG genotype was associated with a median PFS significantly lower than those with GG genotype: 35.9 months (95% CI: 32.6 – 53.7) and not reached respectively (p 0.02) (Figure 3). In contrast, no significant differences were observed in patients who did not receive auto-SCT.
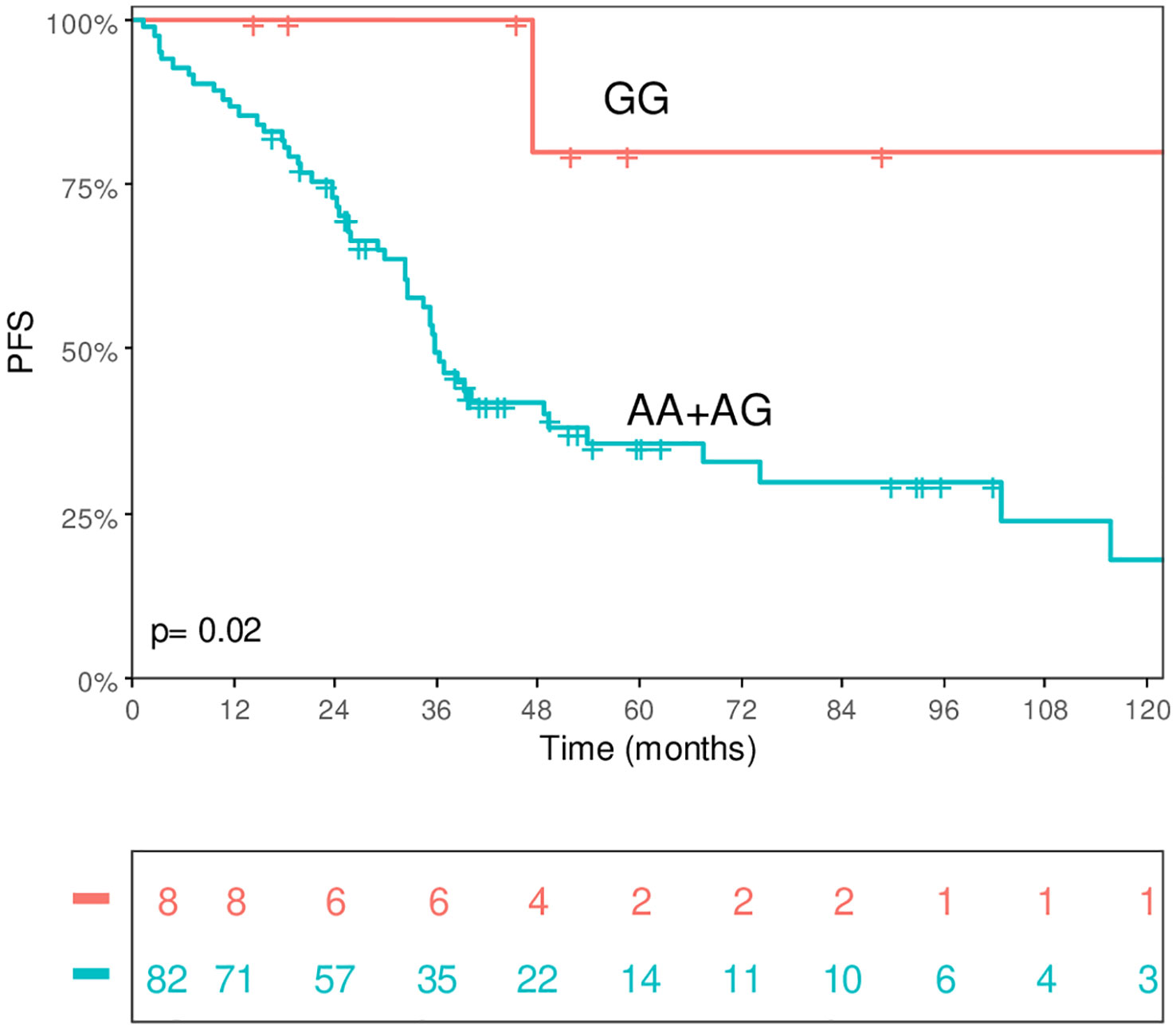
Figure 3 PFS for patients for patients receiving autologous stem cell transplant according to CTLA4 rs2311775 genotype.
Discussion
Tumor progression depends upon the acquisition of traits that allow cancer cells to evade immune surveillance and suppress an effective immune response. The immune dysfunction and neoplastic evasion in MM is facilitated by multiple cytokine and cellular signaling pathways, which decrease immune effector cell function and determine a suppressive bone marrow microenvironment (2). This impaired cellular immunity is mediated by an increased number and functional impairment of dendritic cells (21), altered circulating CD4/CD8 ratio (22), decreased Th1/Th2 ratio (23), increased number of Treg (24), lower level of naïve and transitional B cells subsets in the bone marrow (25), increased number of myeloid derived suppressor cells (MDSC) in the blood and in the bone marrow (26), and enhanced expression of inhibitory ligands such as programmed cell death ligand 1 (PDL-1) by myeloma and bone marrow microenvironment cells, together with an increased expression of PDCD1 and CTLA4 on tumor infiltrating T cells (27, 28). The immunosuppressive state in MM determines important clinical implications, there is increasing evidence that the resistance to bispecific T cell engagers (TCEs) is closely related to immunosuppressive tumor microenvironment (29). Recently, Friedrich et al. (30) has shown that a high proportion of clonal exhausted-like CD8+ T cell clones in the bone marrow of TCE-receiving patients predicts response failure in MM.
We have detected an association between the CTLA4 rs231775 genotype and PFS in patients with MM. The human CTLA4 gene is located on chromosome 2q33. Several single nucleotide polymorphisms (SNPs) have been identified within this gene, and some of them have been related with the ability of CTLA-4 to inhibit immune responses, playing an important role in the development of autoimmune diseases (31) or cancer (32). The rs231775 polymorphism is a non-synonymous SNP which affects exon 1, leading to an alanine-to-threonine amino acid substitution at codon 17 in the leader peptide (Thr17Ala). The association of genetic variants of CTLA4 have been described to be more frequent in MM patients compared with matched healthy controls. Zheng C et al. (13) showed that the CTLA4 microsatellite polymorphism might represent a susceptibility locus for MM and MGUS. Karabon et al. (14) found that CTLA-4c.49A>G[G], CT60[G], and Jo31[G] alleles were more frequently observed in MM patients than in healthy controls. However very little information is available about the role of genetic variants of CTLA-4 in determining progression kinetic of patients with MM.
Several studies have established that T cells from MM patients are able to recognize and eliminate malignant cells (33, 34) and it has recently been determined that T cell exhaustion and a suppressive bone marrow microenvironment are implicated in MM progression (7, 28). CD8+T cell exhaustion and immune checkpoint receptor expression, in both transplant and non-transplant settings, could have an active role for the disease progression (7, 35).
The immune-based therapies offer an increased potential for tumor cell control and may reverse the lack of responsiveness of T cells (36). However, the initial experience with immune checkpoint inhibitors in MM patients has shown limited efficacy, significant toxicity, and side effects. Preclinical data show a potential utility of PD-1/PD-L1 blockade in MM therapy, but early clinical trials have been discouraging (37). Pembrolizumab immunotherapy did not show any activity in MM, and its combination with IMiDs, lenalidomide, or pomalidomide in relapsed or refractory MM patients was associated with immune-related toxicities and mortality (38, 39). While PD-1 blockage has not demonstrated clinical benefits in MM patients, it is notable that some patients achieved long-term remissions after stopping pembrolizumab in clinical trials (40). As well, a phase 1 study of nivolumab in combination with ipilimumab for relapsed or refractory hematologic malignancies did not demonstrate favourable results in MM (41). Currently, TIGIT has emerged as an attractive target for MM immunotherapy. News studies have demonstrated that blocking TIGIT using monoclonal antibodies increased the effector function of MM patient CD8+ T cells and prolongs survival in preclinical MM models (6, 42). Therefore, predictive biomarkers are needed in daily practice to identify the patients who would obtain benefit from immunological checkpoint blockade therapies.
In our study CTLA4 rs733618 polymorphism was not associated with significant differences on PFS and OS, even when considering to the type of anti-myeloma therapy received, as opposed to the results of Xiao-Ying et al. (43). To explain this discrepancy, we must consider the differences in the genetic background between European and Asiatic population: the population of our study was mainly Caucasian, which rarely expresses the CTLA4 rs733618 CC genotype, whereas this allele is more prevalent in Chinese people.
In the present study, the rs231775 CTLA4 AA/AG genotype was associated with lower PFS compared with the GG genotype. Additionally, the analysis identified a subgroup of long-term survivors of myeloma for ISS stage I or II with GG genotype. This finding can be explained by the effect of the G allele and the related lower function of CTLA4 and the reduced inhibition of activated T-cells (44, 45), leading to a more intense T-lymphocyte activity and a stronger immune surveillance. These findings need to be confirmed in other cohorts, due to the low incidence of the rs231775 GG genotype.
There is increasing evidence that the clinical benefit of autologous stem cell transplantation (auto-SCT) is consequence of the cytoreduction with chemotherapy combined with immunological changes. Recent studies suggest that auto-SCT produces immunomodulatory effects including inflammatory cytokine production, immunogenic cell death, enhanced antigen presentations and microenvironment disruption (42, 46). These effects enhance the immune-mediated myeloma control and appears to restore an immune equilibrium. The expansion of T cell clones following autologous transplant, and early lymphocyte recovery has been associated with longer PFS suggesting that early immune reconstitution contributes to control of disease progression in MM (46, 47). Based upon these observations, the minimal residual disease state and lymphopenia after auto-SCT provide an excellent platform to promote the incorporation of immune-based therapies into post auto-SCT treatment regimens to induce or restore antitumor immunity. Consistent with this, our results suggest an association between the rs231775 CTLA4 genotype and PFS after auto-SCT. Despite no checkpoint inhibitor is currently approved in multiple myeloma, our results suggest that this treatment strategy may benefit a subset of multiple myeloma patients.
Several clinical trials have investigated the clinical benefits of checkpoint inhibitors a consolidation after stem cell transplantation in MM. Interim data of a phase I/II study of nivolumab with autologous-SCT in patients with suboptimal response to primary induction, described a 56% of improved response and an acceptable toxicity (48). In a phase 2 study, pembrolizumab in monotherapy was administered in patients who did not achieve a complete response to induction therapy. The study was terminated early after failing to meet its interim analysis endpoint to detect a 20% difference in rate of complete response conversion at the end of treatment (49). Combined checkpoint inhibitors with nivolumab and ipilimumab was analysed in a Phase Ib-IIA study as consolidation after autologous-SCT in patients at high risk for post-transplant recurrence, SLP and SG 18 months post-SCT were 57.1% and 87% high-risk transplant-naïve MM, and 40% and 100% for MM relapsed within 3 years of first ASCT, the treatment was considered to have been well tolerated with no significant unexpected toxicity (50). The combination of antibodies against CTLA-4 and PD-1 was studied in a phase 1 trial to assess safety and tolerability of tremelimumab and durvalumab, administered with high dose chemotherapy and autologous stem cell transplant. FDA ordered to terminate the study due to safety signals in other studies investigating combination regimens comprising similar drugs. Because only 6 subjects were enrolled, no final statistical analysis plan was issued (51). Currently, interesting clinical trial are ongoing such as a pilot study phase 2 of lenalidomide alternating with ipilimumab post allogeneic and autologous SCT in treating patients with hematologic or lymphoid malignancies (NCT01919619). Also, a phase II study of targeting CD28 in multiple myeloma with abatacept (CTLA4-Ig) determine the therapeutic efficacy to overcome resistance to chemotherapy (NCT03457142).
Our results are encouraging and support the hypothesis that this CTLA4 polymorphism could be used as a biomarker to predict the outcome of MM and we suggest that genetic variants of CTLA-4 should be used as genetic marker to identify patients with high risk of progression, who may benefit of anti-CTLA-4-based therapy. Specific studies are needed to confirm this hypothesis.
Clinical trials are ongoing to evaluate strategies that may enhance immunity response, by directly promoting T cell activity against myeloma cells, including checkpoint inhibition, bispecific T-cell engagers and chimeric antigen receptor T cells. According to our results patients with AA/AG genotype of rs231775 CTLA4 may be ideal candidates for participation in such trials to analyse the clinical impact of selected combination immune-based therapies. Further studies with exploratory immunological endpoints are needed to establish the significance of immune gene variation for future clinical interventions for patients with MM.
Data availability statement
The datasets presented in this study can be found in online repositories. The names of the repository/repositories and accession number(s) can be found in the article/Supplementary Material.
Ethics statement
The studies involving human participants were reviewed and approved by Comitè d’Ètica d’investigació amb Medicaments CEIM Girona. The patients/participants provided their written informed consent to participate in this study.
Author contributions
YG-M conceived and designed the study. RR-R, AV, GO, MG-B and FL participated in data collection; AV and GO participated in data analysis. DG participated in manuscript drafting. All authors revised the manuscript critically for important intellectual content, gave their final approval of the version to be published and agreed to be accountable for all aspects of the work in ensuring that questions related to the accuracy or integrity of any part of the work are appropriately investigated and resolved.
Funding
The study was supported by a grant from the Fundació Roses Contra el Cancer, Roses, Girona, Spain.
Conflict of interest
The authors declare that the research was conducted in the absence of any commercial or financial relationships that could be construed as a potential conflict of interest.
Publisher’s note
All claims expressed in this article are solely those of the authors and do not necessarily represent those of their affiliated organizations, or those of the publisher, the editors and the reviewers. Any product that may be evaluated in this article, or claim that may be made by its manufacturer, is not guaranteed or endorsed by the publisher.
Supplementary material
The Supplementary Material for this article can be found online at: https://www.frontiersin.org/articles/10.3389/fimmu.2023.1158105/full#supplementary-material
References
1. Van de Donk NWCJ, Pawlyn C, Yong KL. Multiple myeloma. Lancet (2021) 397(10272):410–27. doi: 10.1016/S0140-6736(21)00135-52
2. Diaz-Tejedor A, Lorenzo-Mohamed M, Puig N, García-Sanz R, Mateos M-V, Garayoa M, et al. Immune system alterations in multiple myeloma: molecular mechanisms and therapeutic strategies to reverse immunosuppression. Cancers (2021) 13(6):1353. doi: 10.3390/cancers130613533
3. Mina R, Bonello F, Oliva S. Minimal residual disease in multiple myeloma; ready for prime time? Cancer J (2021) 27(3):247–55. doi: 10.1097/PPO.0000000000000519
4. Labani-Motlagh A, Ashja-Mahdavi M, Loskog A. The tumor microenvironment: a milieu hindering and obstructing antitumor immune responses. Front Immunol (2020) 11:940. doi: 10.3389/fimmu.2020.00940
5. Kulikowska de Nalecz A, Ciszak L, Usnarska-Zubkiewics L, Frydecka I, Pawlak E, Szmyrka M, et al. Deregulated expression of immune checkpoints on circulating CD4 T cells may complicate clinical outcome and response to treatment with checkpoint inhibitors in multiple myeloma patients. Int J Mol Sci (2021) 22(17):9298. doi: 10.3390/ijms22179298
6. Guillerey C, Harjunpaa H, Carrié N, Kassem S, Teo T, Miles K, et al. TIGIT immune checkpoint blockade restores CD8+ T-cell immunity against multiple myeloma. Blood (2018) 132(16):1689–94. doi: 10.1182/blood-2018-01-825265
7. Suen H, Bronw RF, Yang S, Weatherburn C, Ho PJ, Woodland N, et al. Multiple myeloma causes clonal T-cell immunosenescence: identification of potential novel targets for promoting tumour immunity and implications for checkpoint blockade. Leukemia (2016) 30:1716–24. doi: 10.1038/leu.2016.84
8. Wagner M, Jasek M, Karabon L. Immune checkpoint molecules-inherited variations as markers for cancer risk. Front Immunol (2021) 11:606721. doi: 10.3389/fimmu.2020.606721
9. Monne M, Piras G, Palmas A, Arru L, Murineddu M, Latte G, et al. Cytotoxic T-lymphocyte antigen-4 (CTLA4) gene polymorphism and susceptibility to non-hodgkin’s lymphoma. Am J Hematol (2004) 76(1):14–8. doi: 10.1002/ajh.20045
10. Piras G, Monne M, Uras A, Palmas A, Murineddu M, Arru L, et al. Genetic analysis of the 2q33 region containing CD28-CTLA4-ICOS genes: association with non-hodgkin’s lymphoma. Br J Haematol (2005) 129(6):784–90. doi: 10.1111/j.1365-2141.2005.05525.x
11. Suwalska K, Pawlak E, Karabon L, Tomkiewicz A, Dobosz T, Urbaniak-Kujda D, et al. Association studies of CTLA4, CD28, and ICOS gene polymorphysms with b cell chronic lymphocytic leukemia in the polish population. Hum Immunol (2008) 69(3):193–201. doi: 10.1016/j.humimm.2008.01.014
12. Frydecka I, Suwalska K, Pawlak E, Karabon L, Tomkiewicz A, Dobosz T, et al. Gene polymorphisms of costimulatory molecules: CTLA4/CD28/ICOS are associated with b-cell chronic lymphocytic leukemia (B-CLL). Blood (2007) 110(11):2074. doi: 10.1182/blood.V110.11.2074.2074
13. Zheng C, Huang D, Liu L, Björkholm M, Holm G, Yi Q, et al. Cytotoxic T-lymphocyte antigen-4 microsatellite polymorphism is associated with multiple myeloma. Br J Haematol (2001) 112:216–8. doi: 10.1046/j.1365-2141.2001.02552.x
14. Karabon L, Pawlak-Adamska E, Tomkiewicz A, Jedynak A, Kielbinski M, Woszczyk D, et al. Variations in suppressor molecule CTLA4 gene are related to susceptibility to multiple myeloma in a polish population. Pathol Oncol Res (2012) 18(2):219–26. doi: 10.1007/s12253-011-9431-6
15. Brown EE, Lan Q, Zheng T, Zhang Y, Wang SS, Hoar-Zahm S, et al. Common variants in genes that mediate immunity and risk of multiple myeloma. Int J Cancer (2007) 120:2715–22. doi: 10.1002/ijc.22618
16. Kyoung-Mu L, Dalsu B, Yawei Z, Hosgood HD 3rd, Menashe I, Yeager M, et al. Common single nucleotide polymorphism in immunoregulatory genes and multiple myeloma risk among women in Connecticut. Am J Hematol (2010) 85(8):560–3. doi: 10.1002/ajh.21760
17. Kasamatsu T, Awata M, Ishihara R, Murakami Y, Gotoh N, Matsumoto M, et al. PDCD1 and PDCD1LG1 polymorphisms affect the susceptibility to multiple myeloma. Clin Exp Med (2020) 20(1):51–62. doi: 10.1007/s10238-019-00585-4
18. Pérez-García A, de la Cámara R, Román-Gómez J, Jimenez-Velasco A, Encuentra M, Nieto JB, et al. CTLA4 polymorphisms and clinical outcome after allogeneic stem cell transplantation from HLA-identical sibling donors. Blood (2007) 110:461–7. doi: 10.1182/blood-2007-01-069781
19. Santos N, Rodríguez-Romanos R, de la Cámara R, Brunet S, Nieto JB, Buño I, et al. PD-1 genotype of the donor is associated with acute graft-versus-host disease after HLA-identical sibling donor stem cell transplantation. Ann Hematol (2018) 97(11):2217–24. doi: 10.1007/s00277-018-3438-y
20. Perez-García A, Brunet S, Berlanga JJ, Tormo M, Nomdedeu J, Guardia R, et al. CTLA4 genotype and relapse incidence in patient with acute myeloid leukemia in first complete remission after induction chemotherapy. Leukemia (2009) 23(3):486–91. doi: 10.1038/leu.2008.339
21. Pessoa de Magalhães RJ, Vidriales MB, Paiva B, Fernandez-Gimenez C, García-Sanz R, Mateos M-V, et al. Analysis of the immune system of multiple myeloma patients achieving long-term disease control by multidimensional flow cytometry. Haematologica (2013) 98(1):79–86. doi: 10.3324/haematol.2012.067272
22. Koike M, Sekigawa I, Okada M, Matsumoto M, Lida N, Hashimoto H, et al. Relationship between CD4(+)/CD8(+) T cell ratio and T cell activation in multiple myeloma: reference to IL-16. Leuk Res (2002) 26(8):705–11. doi: 10.1016/S0145-2126(01)00192-8
23. Ogawara H, Handa H, Yamazaki T, Toda T, Yoshida K, Nishimoto N, et al. High Th1/Th2 ratio in patients with multiple myeloma. Leuk Res (2005) 29(2):135–40. doi: 10.1016/j.leukres.2004.06.003
24. Dosani T, Carlsten M, Maric I, Landgren O. The cellular immune system in myelomagenesis: NK cells and T cells in the development of myeloma and their uses in immunotherapies. Blood Cancer J (2015) 5:e30. doi: 10.1038/bcj.2015.32
25. Pojero F, Casuccio A, Giambanco C, Bulati M, Buffa S, Di Bassiano F, et al. Bone marrow b lymphocytes in multiple myeloma and MGUS: Focus on distribution of naive cells and memory subsets. Leukemia Res (2016) 49:51–9. doi: 10.1016/j.leukres.2016.08.008
26. Gorgon GT, Whitehill G, Anderson JL, Hideshima T, Maguire C, Laubach J, et al. Tumor-promoting immune-suppressive myeloid-derived suppressor cells in the multiple myeloma microenvironment in humans. Blood (2013) 121:2975–87. doi: 10.1182/blood-2012-08-448548
27. Rosenblatt J, Avigan D. Targeting the PD-1/PD axis in multiple myeloma: a dream or a reality? Blood (2017) 129(3):275–9. doi: 10.1182/blood-2016-08-731885
28. Zelle-Rieser C, Thangavadivel S, Biedermann R, Brunner A, Stoitzner P, Willenbacher E, et al. T Cells in multiple myeloma display features of exhaustion and senescence at the tumor site. J Hematol Oncol (2016) 9(116):1–12. doi: 10.1186/s13045-016-0345-3
29. Zhang X, Zhang H, Lan H, Wu J, Xiao Y. CAR-T cell therapy in multiple myeloma: Current limitations and potential strategies. Front Immunol (2023) 14:1101495. doi: 10.3389/fimmu.2023.1101495
30. Friedrich MJ, Neri P, Kehl N, Michel J, Steiger M, Kilian M, et al. The pre-existing T cell landscape determines the response to bispecific T cell engagers in multiple myeloma patient. Cancer Cell (2023) 41:1–15. doi: 10.1016/j.ccell.2023.02.008
31. Ueda H, Howson JM, Esposito L, Heward J, Snoo K, Chamberlain G, et al. Association of the T-cell regulatory gene CTLA4 with susceptibility to autoimmune disease. Nature (2003) 423:506–11. doi: 10.1038/nature01621
32. Sun T, Zhou Y, Yang M, Hu Z, Tan W, Han X, et al. Functional genetic variations in cytotoxic T-lymphocyte antigen 4 and susceptibility to multiple types of cancer. Cancer Res (2008) 68(17):7025–34. doi: 10.1158/0008-5472.CAN-08-0806
33. Hayashi T, Hideshima T, Akiyama M, Raje N, Richardson P, Chauhan D, et al. Ex vivo induction of multiple myeloma-specific cytotoxic T lymphocytes. Blood (2003) 102(4):1435–42. doi: 10.1182/blood-2002-09-2828
34. Wen YJ, Min R, Tricot G, Barlogie B, Yi Q. Tumor lysate-specific cytotoxic T lymphocytes in multiple myeloma: promising effector cells for immunotherapy. Blood (2002) 99(9):3280–5. doi: 10.1182/blood.V99.9.3280
35. Chung DJ, PronschinsKe KB, Shyer JA, Sharma S, Leung S, Curran SA, et al. T-Cell exhaustion in multiple myeloma relapse after autotransplant: optimal timing of immunotherapy. Cancer Immunol Res (2016) 4(1):61–71. doi: 10.1158/2326-6066.CIR-15-0055
36. Boussi LS, Avigan ZM, Rosenblatt J. Immunotherapy for the treatment of multiple myeloma. Front Immunol (2022) 13:1027385. doi: 10.3389/fimmu.2022.1027385
37. Lesokhin AM, Ansell SM, Armand P, Scott EC, Halwani A, Gutierrez M, et al. Nivolumab in patients with relapsed or refractory hematologic malignancy: preliminary results of a phase ib study. J Clin Oncol (2016) 34(23):2698–704. doi: 10.1200/JCO.2015.65.9789
38. Usmani SZ, Schjesvold F, Oriol A, Karlin L, Cavo M, Rifkin RM, et al. Pembrolizumab plus lenalidomide and dexamethasone for patients with treatment-naive multiple myeloma (KEYNOTE-185): a randomised, open-label, phase 3 trial. Lancet Haematol (2019) 6(9):e448–458. doi: 10.1016/S2352-3026(19)30109-7
39. Mateos MV, Blacklock H, Schjesvold F, Oriol A, Simpson D, George A, et al. Pembrolizumab plus pomalidomide and dexamethasone for patients with relapsed or refractory multiple myeloma (KEYNOTE-183): a randomised, open-label, phase 3 trial. Lancet Haematol (2019) 6(9):e459–69. doi: 10.1016/S2352-3026(19)30110-3
40. Badros AZ, Ma N, Rapoport AP, Lederer E, Lesokhin AM. Long-term remissions after stopping pembrolizumab for relapsed o refractory multiple myeloma. Blood Adv (2019) 3(11):1658–60. doi: 10.1182/bloodadvances.2019000191
41. Ansell S, Gutierrez ME, Shipp MA, Gladstone D, Moskowitz A, Borello I, et al. A phase 1 of nivolumab in combination with ipilimumab for relapsed or refractory hematological malignances (ChekMate039). Blood (2016) 128:183. doi: 10.1182/blood.V128.22.183.183
42. Minnie SA, Kuns RD, Gartlan KH. Myeloma escape after stem cell transplantation is a consequence of T-cell exhaustion and is prevented by TIGIT blockade. Blood (2018) 132(16):1675–88. doi: 10.1182/blood-2018-01-825240
43. Quin X-Y, Lu J, Li G-X, Wen L, Liu Y, Xu L-P, et al. CTLA4 polymorphisms are associated with treatment outcomes of patients with multiple myeloma receiving bortezomib-based regiments. Ann Hematol (2018) 97(3):485–95. doi: 10.1007/s00277-017-3203-7
44. Anjos S, Nguyen A, Ounissi-Benkalha H, Tessier M-C, Polychronakos C, et al. A common autoimmunity predisposing signal peptide variant of the cytotoxic T-lymphocyte antigen 4 results in inefficient glycosylation of the susceptibility allele. J Biol Chem (2002) 227(48):46478–86. doi: 10.1074/jbc.M206894200
45. Ligers A, Teleshova N, Masterman T, Huang W-X, Hillert J. CTLA4 gene expression is influenced by promotor and exon 1 polymorphisms. Gene Immun (2001) 2(3):145–52. doi: 10.1038/sj.gene.6363752
46. Minnie SA, Hill GR. Autologous stem cell transplantation for myeloma: cytoreduction or an immunotherapy? Front Immunol (2021) 12:1–7, 651288. doi: 10.3389/fimmu.2021.651288
47. Jimenez-Zepeda VH, Reece DE, Trudel S, Chen C, Franke N, Winter A, et al. Absolute lymphocyte count as predictor of overall survival for patients with multiple myeloma treated with single autologous stem cell transplant. Leuk Lymphoma (2015) 56(9):2668–73. doi: 10.3109/10428194.2014.1003057
48. Pirogova O, Darskaya E, Porunova V, Kudyasheva O, Babenko E, Mikhailova N, et al. Results of phase I/II study of nivolumab with autologous hematopoietic stem cell transplantation in multiple myeloma patients with suboptimal response to primary induction therapy. EHA Library (2019) 267013:PS1396. doi: 10.1097/01.HS9.0000563860.35670.9c
49. D’Souza A, Hari P, Pasquini M, Parkin B, Reddy P, Pawarode A, et al. A phase 2 study of pembrolizumab during lymphodepletion after autologous hematopoietic cell transplantation for multiple myeloma. Biol Blood Marrow Transplant (2019) 25(8):1492–7. doi: 10.1016/j.bbmt.2019.04.005
50. Skarbnik AP, Donato ML, Feinman R, Rowley SD, Vesole DH, Goy AH, et al. Safety and efficacy of consolidation therapy with ipilimumab plus nivolumab after autologous stem cell transplantation. Transplant Cell Ther (2021) 27(5):391–403. doi: 10.1016/j.jtct.2020.12.026
51. Lesokhin AM, Chung DJ, Cho HJ, Shohara L, Schwarzenberger P, Ricciardi T, et al. Phase 1 study to evaluate the safety and efficacy of immunotherapy with tremelimumab and durvalumab in multiple myeloma patients receiving high dose chemotherapy and autologous stem cell transplant (HDT/ASCT) peripheral blood lymphocyte (PBL) reinfusion. J Clin Oncol (2017) 35:TPS8051–TPS8051. doi: 10.1200/JCO.2017.35.15_suppl.TPS8051
Keywords: CTLA4 polymorphisms, multiple myeloma, immune checkpoint, bone marrow microenvironment, cytogenetics and molecular genetics
Citation: Gonzalez-Montes Y, Rodriguez-Romanos R, Villavicencio A, Osca-Gelis G, González-Bártulos M, Llopis F, Clapes V, Oriol A, Sureda A, Escoda L, Sarrà J, Garzó A, Lloveras N, Díez I, Granada I and Gallardo D (2023) Genetic variants of CTLA4 are associated with clinical outcome of patients with multiple myeloma. Front. Immunol. 14:1158105. doi: 10.3389/fimmu.2023.1158105
Received: 03 February 2023; Accepted: 29 March 2023;
Published: 12 April 2023.
Edited by:
Loredana Ruggeri, University of Perugia, ItalyReviewed by:
Jaya Lakshmi Thangaraj, University of California, San Diego, United StatesWalter Braga, Federal University of São Paulo, Brazil
Copyright © 2023 Gonzalez-Montes, Rodriguez-Romanos, Villavicencio, Osca-Gelis, González-Bártulos, Llopis, Clapes, Oriol, Sureda, Escoda, Sarrà, Garzó, Lloveras, Díez, Granada and Gallardo. This is an open-access article distributed under the terms of the Creative Commons Attribution License (CC BY). The use, distribution or reproduction in other forums is permitted, provided the original author(s) and the copyright owner(s) are credited and that the original publication in this journal is cited, in accordance with accepted academic practice. No use, distribution or reproduction is permitted which does not comply with these terms.
*Correspondence: Yolanda Gonzalez-Montes, eWdvbnphbGV6QGljb25jb2xvZ2lhLm5ldA==