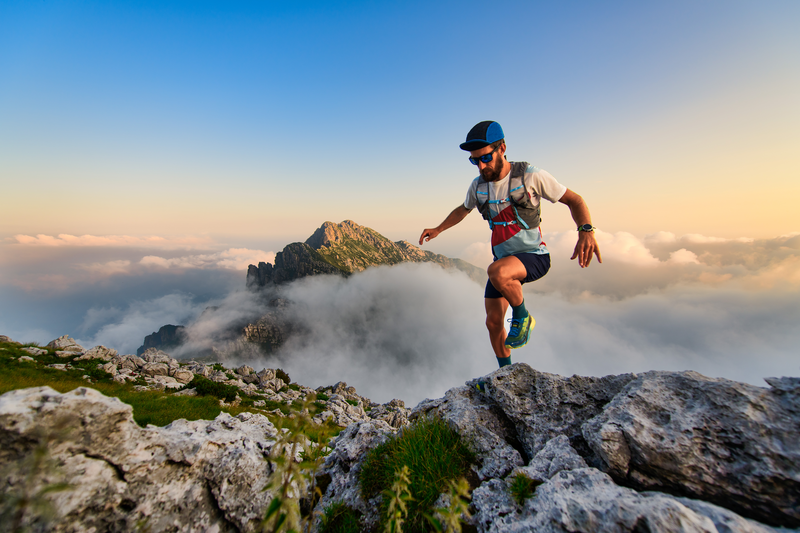
94% of researchers rate our articles as excellent or good
Learn more about the work of our research integrity team to safeguard the quality of each article we publish.
Find out more
REVIEW article
Front. Immunol. , 14 March 2023
Sec. Inflammation
Volume 14 - 2023 | https://doi.org/10.3389/fimmu.2023.1153901
Mesenchymal stem cells (MSCs) are pluripotent stem cells of mesodermal origin with the ability of self-renewal and multidirectional differentiation, which have all the common characteristics of stem cells and the ability to differentiate into adipocytes, osteoblasts, neuron-like cells and other cells. Stem cell derivatives are extracellular vesicles(EVs) released from mesenchymal stem cells that are involved in the process of body’s immune response, antigen presentation, cell differentiation, and anti-inflammatory. EVs are further divided into ectosomes and exosomes are widely used in degenerative diseases, cancer, and inflammatory diseases due to their parental cell characteristics. However, most diseases are closely related to inflammation, and exosomes can mitigate the damage caused by inflammation in terms of suppressing the inflammatory response, anti-apoptosis and promoting tissue repair. Stem cell-derived exosomes have become an emerging modality for cell-free therapy because of their high safety and ease of preservation and transportation through intercellular communication. In this review, we highlight the characteristics and functions of MSCs-derived exosomes and discuss the regulatory mechanisms of MSCs-derived exosomes in inflammatory diseases and their potential applications in clinical diagnosis and therapy.
Mesenchymal stem cells (MSCs) are a class of non-hematopoietic multifunctional stem cells that originate from the mesoderm and were first discovered in the bone marrow by Friedenstein in the late 1960’s. They grow clonally against the wall and have a morphology similar to that of fibroblasts, with the potential for self-renewal and multidirectional differentiation into adipocytes, osteoblasts and chondrocytes in vitro (1). Bone marrow and subcutaneous fat are common sources of cells (2). In addition, MSCs can also be isolated and prepared from muscle, lung, liver, pancreas, as well as from amniotic fluid and umbilical cord blood (3). In addition to having proliferative and self-renewal properties, the International Committee determined that MSCs must meet the following criteria: maintain adherent growth under standard laboratory conditions; express the typical MSC markers: CD73, CD90 and CD105, but not the haematopoietic markers: CD14, CD34 and CD45 or CD11b, CD19 and CD79α (4). MSCs play an important role in the normal development and maturation of hematopoietic stem cells (5) and are also involved in angiogenesis (6), production of various growth factors and cytokines to protect damaged or dead cells (7). In addition to their direct effects, MSCs can also release extracellular vesicles (EVs) to alter the microenvironment. EVs are a family of fluid-containing particles/vesicles which divided into three main categories: categories, microvesicles and exosomes (8).MSCs protect and regenerate damaged cells and attenuate immune responses by EVs delivery of a variety of proteins, including chemokines, growth factors and cytokines, membrane receptors, lipids, and various nucleic acids (9).
Exosomes are spherical vesicles composed of a double layer of lipid membranes, 40-160 nm in diameter, and are the most studied EVs type at present (10). Most cells in the body can secrete exosome, such as MSCs, natural killer and T lymphocyte cells, and neutrophils, which have many of the distinctive features of their parent cells, but also have their own unique characteristics, containing biologically active macromolecules such as DNA, RNA, nucleic acids, proteins and lipids, and regulates the function of receptor cells by transferring these substances to other receptor cells (11).
Inflammation, which can be classified as infectious inflammation caused by infection or non-infectious inflammation caused by factors such as tumors, trauma and autoimmune reactions. Inflammatory diseases are caused by inflammation, including infectious diseases, immune diseases, and destructive diseases. Previous studies have shown that inflammatory diseases involve all organs of the body, and inflammatory diseases not only lead to local apoptosis and necrosis but also deposit large amounts of extracellular matrix in the tissue, which is extremely destructive to the organism (12). Although inflammatory mediators are thought to be major players in triggering the inflammatory response and intercellular communication, a growing body of evidence supports the relationship between exosomes and inflammation (13). Exosomes play an important role in the pathogenesis and treatment of different inflammatory diseases by promoting proliferation, inhibiting apoptosis, reducing oxidative stress, and promoting cell repair and regeneration (14–16). However, exosomes also has challenges as a treatment in terms of tissue origin differences, age dependence, storage and transport, precise dosing and route of administration (17). There is still a long way to go before Exosomes are widely used as generic drugs for the treatment of inflammatory diseases
In this review, we provide a systematic review of the characteristics and effect of exosomes and the relationship between exosomes and inflammatory diseases; emphasized the current molecular and cellular mechanisms regarding the use of exosomes as a therapeutic approach for inflammatory diseases and its limitations and challenges.
Bonucci and Anderson first observed chondrocytes secreting small, secretory vesicles of approximately 100 nm in the late 1960s (18, 19). In 1983, scholars first discovered the existence of exosomes in the reticulocytes of sheep (20), which were initially thought to be a kind of excretion in the human body without any role and were not much concerned. Later, it was found to be functional, mainly involved in the exchange of information between substances and signal transduction, and was subsequently named exosomes in 1987 (21, 22). Exosomes are released by almost all cells except mature red blood cells (Figure 1).
Figure 1 Biogenesis and composition of exosomes. Exosomes secreted by almost all types of cells in the body, such as MSCs, dendritic and B lymphocyte cells. Fluid and extracellular components can enter cells along with cell surface proteins through endocytosis and plasma membrane invagination. This process leads to the formation of early-sorting endosomes (ESEs), endoplasmic reticulum and pre-formed Golgi apparatus, develops into late endosomes (LSEs), and interconnects with cell membrane network structures to form intraluminal vesicles (ILVs) containing vesicle structures. the ILVs are ultimately secreted as exosomes of 40 ~ 160 nm in diameter through MVB fusion to the plasma membrane and cytosolic exocytosis. Exosomes expres EV surface markers CD63, CD9 and CD81, and also contain DNA, RNA and some common proteins, including MVB biogenesis proteins (Alix, TSG101, and ESCRT Complex), membrane transporter and heat shock proteins (HSP60, 70, and 90), lipid related proteins, and phospholipases.
Exosomes originate from endosomes, the process of exosome production involves plasma membrane invagination and subsequent formation of early-sorting endosomes (ESEs), which further mature into late-sorting endosomes (LSEs) and eventually form intracellular multivesicular bodies (MVBs) containing luminal vesicles (ILVs). When MVBs fuse with the plasma membrane, exosomes with diameters of 40 ~ 160 nm are released (Figure 1) (23).
Exosomes express the MSC surface markers CD73, CD44 and CD90 in addition to the EV surface markers CD9, CD63 and CD81 (24). They contain biological macromolecules such as membrane proteins, nuclear proteins, cytoplasmic proteins, nucleic acids, metabolites, DNA, mRNA and non-coding RNA. The mRNAs and miRNAs encapsulated in Exosomes form the molecular basis of its function. Common proteins in exosomes also play an important role, including membrane transporter and fusion proteins (GTPases, RAB protein and annexins), heat shock proteins (HSP60, 70, and 90), as well as other proteins that are also used as markers for exosomes (Alix, TSG101, and ESCRT Complex), phospholipases and lipid related proteins. Delivery of exosome contents can bind to target cells through three pathways: ligand-receptor binding, endocytosis and direct binding (25). Therefore, intercellular communication of exosomes in these three ways, thereby altering the function and activity of target cells (26).
Because exosomes contain growth factors, RNA, DNA and other components from parental cells, and transferred to the recipient cell via intercellular communication, they are widely used in the treatment of inflammatory diseases Table 1 such as chronic inflammatory diseases, autoimmune diseases and destructive diseases and other inflammatory diseases (39). Exosomes act as intercellular messengers, carrying proteins and DNA, transporting RNA, and playing an important role in intercellular material and information transduction. For example, MSC-Exo significantly reduced pulmonary edema and lung protein permeability by reducing inflammatory cell invasion and inflammatory factor production in a mouse model of endotoxin-induced acute lung injury (40). Based on the functional similarity to parental cells, exosomes can cross the blood-brain barrier and are an effective cell-free reagent that may be safer in clinical treatment and may have fewer side effects compared to cell transplantation. However, its safety specification and methodological standardization have not yet been agreed upon in clinical studies. Therefore, these safety issues need to be further explored in future large-scale replications in clinical trials.
Fibrosis can occur in a variety of organs, and the main pathological changes are an increase in fibrous connective tissue and a decrease in parenchymal cells in the organ tissues, which can lead to structural destruction and functional decompensation of organs and even failure, posing a serious threat to human health and life. The liver is a central organ for metabolic and immune regulation, and most chronic liver diseases, such as fatty liver, viral hepatitis and autoimmune diseases can all lead to liver fibrosis. Liver fibrosis is an imbalance between the synthesis and degradation of the liver’s extracellular matrix (such as collagen glycoproteins and proteoglycans) that progresses further into liver cirrhosis (41). If left unprevented and untreated, liver fibrosis may eventually lead to cirrhosis, liver cancer and even liver failure.
Exosomes alleviate liver fibrosis by reducing inflammation and promoting liver tissue repair (42). Li et al. (43) showed that human umbilical cord MSCs-derived exosomes attenuated carbon tetrachloride (CCl4)-induced liver fibrosis in mice by restoring serum AST levels and reducing the deposition of cross-linked type I and type III collagen and decrease transforming growth factor (TGF)-β1 and phosphorylation Smad2in the liver. Through TGF-β1 production, Kupffer cells induce enhanced expression of pro-fibrotic genes (waveform protein, type I collagen, fibronectin and α-SMA) in hematopoietic stem cells, leading to the development of liver fibrosis. Exosomes can reduce liver fibrosis by inhibiting the production of inflammatory factors such as interleukin-6 (IL-6), interleukin-1β (IL-1β) and tumor necrosis factor-α (TNF-α) and pro-fibrotic transforming growth factor-β1 in Kupffer cells (44). Based on these previous studies, Qu and colleagues designed miRNA-181-5p overexpression of adipose tissue-derived mesenchymal stem cells (MSCsmiRNA-181-5p-Exos) to increase autophagy levels in liver fibrosis by promoting the expression of autophagy-related protein Beclin-1 and inhibiting the expression of anti-apoptotic protein Bcl-2. It also significantly downregulated the expression of pro-fibrotic genes (waveform protein, type I collagen, fibronectin and α-SMA) in hematopoietic stem cells, thereby attenuating CCl4-induced liver fibrosis in mice (45).
Exosomes also contain miRNAs, such as miRNA-181-5, MiR-690, MiR-223 and miR-122, which have antifibrotic effects in liver fibrosis. Recent studies have indicated that exosomal miR-124 attenuates liver injury after partial hepatectomy in rats and promotes hepatocyte proliferation and liver regeneration by inhibiting Foxg1 expression (27). Similarly, MiR-690 treatment has been found to reduce liver fibrosis and steatosis and restore specific Kupffer cell functions (28). Hou et al. (46) demonstrated that IL-6 treatment promotes the release of miR-223-enriched exosomes from macrophages and thus exerts an anti-fibrotic effect in Nonalcoholic fatty liver disease patients.
Inflammatory bowel disease (IBD) is a non-specific inflammatory disease of the intestine involving the ileum, rectum and colon mediated by abnormal immunity and infection. Histologically, IBD is characterized by shortening and branching of the crypt, crypt abscesses or cryptitis. IBD includes Crohn’s disease (CD) and ulcerative colitis (UC), characterized by disruption of the intestinal mucosal barrier, imbalance of immune cells and their secreted cytokines, and dysregulation of the immune response to intestinal microorganisms, causing chronic intestinal mucosal damage (47).
Macrophages are important in maintaining intestinal homeostasis, they are involved in the regulation of BD pathogenesis, and some evidence supports the ability of exosomes to activate macrophages (48). Bone marrow mesenchymal stem cells-derived exosomes for the treatment IBD leads to polarization of M2b macrophages, reduces inflammation and maintenance of the integrity of the intestinal barrier.
These exosomes are enriched with proteins involved in the regulation of biological processes in anti-colitis, in particular metallothionein-2, which is necessary for suppression of inflammatory responses thereby attenuating colitis (29). Wong et al. identified the role of circulating exosomal proteins in bone marrow-derived macrophages(BMDMs) activation by establishing a model of acute colitis in athymic mice. Proteomics and bioinformatics analysis of RAW264.7 macrophage-treated sera identified 56 proteins (include immunoglobulins and acute phase proteins) that are involved in coagulation and complement cascades and ultimately activate macrophages (30). Further, some exosomal heat shock proteins such as HSP72, HSP20 and HSP90 have also been shown to be associated with the development of IBD (49).
In addition to proteins, exosome-mediated miRNAs play an important role in IBD. Exosomal miRNAs coordinate the immune system by regulating the functions of T cells, dendritic cells (DCs), macrophages and NF-κB-related signaling pathways. Such as miR-146a and miR-155, can regulate the inflammatory response in IBD. miR-155 deficiency inhibits the function and growth of DC in intestinal lamina propria and thereby ameliorates intestinal inflammation (50). miR-1246 also activates T cells and thus pro-inflammatory nuclear factors in active IBD (51). A recent study found that platelet-derived exosomal MiR-223 regulates immune cell and intestinal barrier function in IBD by blocking the MAPK pathway (downregulating phosphorylation ofc-JNK, ERK and p38) and NF-κB p65 nuclear translocation and inhibiting intercellular adhesion molecule-1 in inflammation (52). Similarly, it was shown that after treatment with exosomes, microbiome alterations in colitis model mice return to concordance compared to healthy mice. Exosome treatment also improved overall intestinal health and healing by increasing the number of Lgr5+ intestinal stem cells, promote intestinal angiogenesis and stimulating intestinal epithelial cell proliferation (53).
Acute lung injury (ALI) is an injury to alveolar epithelial cells and capillary endothelial cells caused by various direct and indirect injury factors, resulting in diffuse interstitial lung and alveolar edema, which can eventually progress to pulmonary fibrosis and therefore has a high clinical mortality rate (54). Recently, an increasing number of reports have showed that exosomes play an important role in tissue repair, immune response and reduction of pro-inflammatory cytokines in sepsis and non-sepsis-induced ALI (55–57). Li et al. (58) found that injection of MSCs-derived exosomes into an ALI mouse model significantly reduced neutrophil and macrophage inflammatory protein-2 levels. Moreover, alveolar macrophage-derived exosomes inhibit secretion of pro-inflammatory factors IL-1β and TNF-α and increased secretion of anti-inflammatory factors TGF-β (59). Similarly, Wang et al. found that MSCs-derived exosomes could alleviate sepsis-induced lung injury by inhibiting the release of IL-27, suppressing the expression of pro-inflammatory factors IL-6, IL-1β and TNF-α, and inhibiting the aggregation of pulmonary macrophages (31). Exosomes not only play an anti-inflammatory role, but also regulate apoptosis. For example, Sun et al. reported that exosomes regulate the expression of mtDNA damage makers and apoptosis-related proteins through the nod-like receptor protein 3 (NLRP3) pathway to alleviate mitochondrial DNA damage and alveolar epithelial cell apoptosis and mitigate lung injury (60). Adipose stem cell-derived exosomes attenuate sepsis-induced lung injury by promoting autophagy, reducing alveolar epithelial cell apoptosis and pro-inflammatory factor expression (61). Exosomes promote the regeneration of alveolar epithelial and endothelial cells and attenuate lung injury. Dinh et al. found that exosomes of pulmonary spherocyte origin attenuate fibrosis by reconstructing normal alveolar structure, suggesting the therapeutic potential of exosomes by increasing cell regeneration (62). Wu et al. also reported that exosomes of endothelial progenitor cell origin improve endothelial cell function by promoting the migration and proliferation of endothelial cells to attenuate lipopolysaccharide (LPS) induced inflammation in the lung (63).
Exosomes act as carriers of miRNAs that regulate pathological processes in lung diseases. For example, MSCs-derived exosomes inhibit NF-κB and hedgehog signaling pathways by disrupting IKKβand silencing Ikbkb, thus delivering miR-182-5p and miR-23a-3p and reversing the progression of LPS-induced lung injury and fibrosis (64). Exosomal miR-1-3p promotes apoptosis and cytoskeleton contraction, inhibits cell proliferation and impairs vascular barrier function involved in ALI progress. MiR-1-3p may be a potential therapeutic target for sepsis-induced ALI (65). Liu et al. found that miR-384-5p was enriched in bone marrow mesenchymal stem cell-derived exosomes and alleviated impaired autophagy in alveolar macrophages in an LPS-induced ALI model by targeting Beclin-1 (32).Besides, MiR-377-3p released from MSCs-derived exosomes attenuated lipopolysaccharide-induced ALI by targeting the regulatory-associated protein of mTOR to induce autophagy (66). Exosomes can also regulate ALI in other ways. Exosomes from adipose-derived MSCs can mitigate ALI by transferring mitochondrial components to improve oxidative phosphorylation levels and macrophage mitochondrial integrity, thereby restoring airway macrophage immune and metabolic homeostasis (67).
Atherosclerosis is an inflammatory disease of the blood vessels, and impaired lipid metabolism is the basis of atherosclerosis. It is characterized by lesions of the affected arteries starting from the intima, generally with accumulation of lipids and complex sugars, hemorrhage and thrombosis, followed by proliferation of fibrous tissue and calcium deposits, and gradual metamorphosis and calcification of the middle layer of the arteries, resulting in thickening and hardening of the arterial walls and narrowing of the lumen and activation of inflammatory pathways. Atherosclerosis is the leading cause of cardiovascular disease and the leading cause of death worldwide.
Atherosclerosis is caused by a combination of multiple factors, and the pathogenesis is complex and has not been fully elucidated. The main risk factors are hypertension, hyperlipidemia and smoking, as well as diabetes, obesity and genetic factors (68, 69). Lipid infiltration, endothelial cell damage theory, vascular smooth muscle proliferation, inflammation, macrophage polarization and so on are involved in the process of atherosclerosis (70). Therefore, intercellular communication is important in atherosclerosis, and exosomes are also participants in intercellular communication. Extracellular vesicles as well as exosomes are involved in atherosclerotic microcalcifications (71). Exosomes can be used not only as biomarkers for the diagnosis of atherosclerosis, but also as potential therapeutic targets for atherosclerosis (10, 72).
Macrophages differentiated from monocytes play an important role in atherosclerosis, and macrophage-derived exosomes are involved in the whole process of atherosclerosis (73). It has been shown that atherosclerosis-stimulated treated mouse macrophages are enriched in miR-146a, which reduces cell migration and promotes macrophage retention in the vessel wall thereby exacerbating atherosclerosis. Suggesting a biomarker role for miR-146a in atherosclerosis progress (74). Similarly, the exosome miR-146 from atherogenic macrophages was found to promote the development of atherosclerosis by promoting neutrophil extracellular traps (75). Furthermore, the BMDMs-derived exosome miR-21-3p promoted the proliferation and migration of vascular smooth muscle cells(VSMCs) thereby promoting atherosclerosis (76). In addition to macrophages, VSMCs-derived exosomes are also key regulators of atherosclerosis. For example, exosomes from VSMCs were found to accelerate calcification and thus promote atherosclerosis by propagating precalcification signals (77). Besides, exosomes are released by VSMCs and deposit them in pre-calcified vessels, which may provide the conditions for vessel wall calcification (78).
Although exosomes can promote atherosclerosis, on the other hand, exosomes can also reduce the extent of atherosclerosis. For example, exosomal miRNAs play an important role in the suppression of atherosclerosis. Bai et al. showed that endothelial progenitor cell-derived exosomes significantly reduced the production of atherosclerotic plaque and inflammatory factors and improved atherosclerotic diabetes by improving endothelial dysfunction (33). In addition, in high-fat diet-fed apolipoprotein E-deficient (ApoE(-/-)) mice, MSCs-derived exosomes reduced the size of atherosclerotic plaques and macrophage infiltration in plaques and promoted the polarization of M2 macrophages (79). Similarly, the mouse bone marrow MSCs-derived exosome miR-512-3p prevents atherosclerosis by reducing apoptosis and inflammatory factor expression to inhibit endothelial cell dysfunction (80). Lin et al. demonstrated that exosomal miR-125b-5p from bone marrow mesenchymal stem cells can be used as a treatment for atherosclerosis by reducing plaque size, lipids, and inhibiting apoptosis in mice with atherosclerosis (34). In a high-fat diet-fed ApoE(-/-) mouse model, BMDMs-derived exosomes were able to reduce inflammation and hematopoiesis while inducing a macrophage polarization. BMDMs-derived exosomes containing miR-146b, miR-99 and miR-378a have anti-inflammatory properties, inhibit inflammation and accelerate M2 polarization in BMDMs thereby inhibiting atherosclerosis (81).
Rheumatoid arthritis (RA) is a chronic, predominantly inflammatory synovitis systemic disease of unknown etiology. It is characterized by polyarticular, symmetric, aggressive joint inflammation of the small joints of the hands and feet and also damage to extra-articular organs, including the heart, lungs, kidneys, eyes, skin and nervous system (82). MSCs-derived exosomes have been shown to be beneficial in treating RA by reducing bone destruction and erosion, joint inflammation and mitigating the formation of panniculitis through immunomodulation, anti-inflammation and differentiation (83, 84). RA is primarily due to an imbalance between effector T cell subpopulations of T helper type 1/T helper type 17 (Th1/Th17) and IL-10 producing immunomodulatory Treg, which leads to injury and inflammation in target tissues. Since immunomodulation by bone marrow MSC-derived exosomes is most effective against CD4 cells, it is not surprising that exosomes treat autoimmune diseases (85). For example, Ma et al. found that both human umbilical cord-MSCs-derived exosomes inhibited T cell proliferation, promoted T cell apoptosis, decreased rorr γ levels, increased forkhead box P3(Foxp3) levels, and regulated Treg/Th17 cell homeostasis, thereby inhibiting synovial proliferation and delaying the progression of RA through in vitro and in vivo experiments (35). Dendritic cells treated with IL-10 have been found to release exosomes and reduce the severity of RA (86). In addition, dc exosomes treated with IL-10 prevented the invasion of RA in mice and reduced the severity of existing arthritis (87).
In addition, exosomal miRNAs play an important role in mitigating the development of RA. Studies in RA model mice showed that increased expression of exosomal miR-424 targeting Foxp3 significantly inhibited T cell differentiation, decreased Treg cells and increased Th17 cells. Thus, exosomal miR-424 overexpression may mitigate RA progression (36). Exosomal miR-204-5p can translocate to synovial fibroblasts and inhibit cell proliferation. Wu et al. found that exosomal miR-204-5p expression was negatively correlated with disease parameters such as rheumatoid factor, erythrocyte sedimentation rate, and c-reactive protein in RA patients and could be a potential biomarker target for RA therapy (88). Similarly, MSCs-derived exosomal miR-192-5p significantly reduced the levels of pro-inflammatory cytokines, including PGE2, IL-1β and TNF-α, in synovial tissue and serum of collagen-induced arthritis (CIA) rats, and decreased the release of NO and inducible NO synthase (iNOS) in serum (89). A recent study showed that miR-146a-transduced MSCs-derived exosomes also increased Foxp3, TGFβ and IL-10 gene expression in CIA mice, and that exosomes appear to promote direct intracellular transfer and immunomodulatory effects of intercellular MiRNA and represent a possible therapeutic strategy for RA (90). Lim et al. also found that serum exosomal miR-1915-3p levels were negatively correlated with serum c-reactive protein levels, predicting that miR-1915-3p could serve as a potential marker of RA disease activity (91). By preparing human mesenchymal stem cell exosomes overexpressing miRNA-124a, Meng et al. found that this exosome pretreatment inhibited the migration and proliferation of fibroblast-like synoviocytes during coincubation and promoted apoptosis of the cell, which may be a suitable vehicle for therapeutic drugs (92).
Multiple sclerosis (MS) is an autoimmune disease characterized by inflammatory demyelinating lesions in the white matter of the central nervous system caused by autoimmune and viral infections. The most frequent sites of involvement are the periventricular white matter, optic nerve, spinal cord, brainstem and cerebellum (93). The characteristic pathological changes of MS are the invasion of autoreactive CD4+ T cells (especially th17 and Th1 cells) into the central nervous system (CNS) and multiple demyelinated plaques in the white matter of the CNS, mostly located around the lateral ventricles, with reactive gliosis and axonal damage (84). In addition to this, microRNAs are involved in the pathological changes of MS (94). However, current therapeutic approaches regarding MS are still limited, mostly immunomodulatory treatments (including immunomodulators and immunosuppressants, etc.) are performed. In addition to crossing the blood-brain barrier, exosomes contain a range of anti-inflammatory and neuroprotective agents that may have a protective effect on immune modulation and demyelinated tissue repair (95).
The understanding of exosomes in the diagnosis and treatment of MS has progressed significantly in recent years. Riazifar et al. found that MSCs-derived exosomes stimulated by IFN γ attenuated neuroinflammation and demyelination and upregulated the number of CD4 + CD25 + FOXP3 + regulatory T cells (Tregs) in a mouse model of experimental autoimmune encephalomyelitis (EAE) (37). It was also found that MSCs-derived exosomes treatment promoted myelin regeneration in microglia in the EAE mouse model, increased polarization of the M2 phenotype and blocked TLR2 signaling, providing the animal and cellular basis for this cell-free exosome treatment of MS (38). Not only that, exosomal miRNA can be used as a biomarker to diagnose MS. In a cohort study, five miRNA (hsa-miR-140-5p, hsa-miR-484, hsa-miR-486-5p, hsa-miR-320a and hsa-miR-320c) were significantly different between MS patients and healthy subjects (96). Similarly, another study identified 15 separate miRNAs that were differentially expressed in serum exosomes of patients with active versus quiescent MS disease after treatment (97).
In 1995, the first clinical trial of MSCs was reported in patients with malignant hematological diseases. Lazarus et al. (98) confirmed that no adverse infusion reactions were observed after patients were treated with MSCs (Figure 2). A growing number of clinical trials have investigated the effectiveness and safety of MSCs in the treatment of various diseases.
Figure 2 Timeline of advances in the research on exosomes. The first discovery and designation of exosomes and their clinical application as therapeutic tools.
Exosomes are safer and more effective than MCSs treatment as a novel cell-free treatment strategy. Inspired by the promising results observed in preclinical studies on the therapeutic efficacy of MSCs-derived exosomes, the first case report of the application of MSCs-derived exosomes was published by Kordelas et al. in 2014. Four doses of exosomes were obtained from 4 × 107 bone marrow-MSCs supernatant isolated and injected every 2 ~ 3 days for individualized treatment of patients with refractory graft-versus-host disease. After treatment, patients showed a significant reduction in pro-inflammatory cytokines and significant improvement in clinical symptoms (99).
MSCs-derived exosomes seem to be an interesting approach for clinical trials in various kind of diseases, especially those with an inflammatory component. The beneficial effects of MSCs-derived exosomes can be enhanced by genetic modifications and bioengineering, drug encapsulation or stimulation with different growth factors. As of December 2022, 295 clinical studies involving exosomes are listed at www.clinicaltrials.gov, with 98 trials (33.2%) in recruiting phase, 31 trials (10.5%) in not yet recruited phase, only 71 studies (24.1%) have been completed. Of these completed studies, most of which used exosomes as a diagnostic tool, those related to inflammatory diseases were shown in Table 2. To date, among the 295 trials using MSC-derived exosomes:46 trials in phase 1, 46 trials in phase 2, 73 trials combined phase 1/2; 6 trials in phase 3, 48 trials combined phase 2/3; 2 trials in phase 4 trials, and 78 trials unspecified phases.
Table 2 The role of Exosomes in the diagnose and treatment of inflammatory diseases, as discussed in the text.
Despite more than 1,000 trials in more than 40 countries using various types of msc or MSC-free derivatives, only nine MSC-based products are approved globally for the treatment of regenerative or immune-related diseases (100). Therefore, further research is needed to establish therapeutic doses, suitable culture conditions and routes of administration of MSCs, and standardized protocols for exosome isolation and storage, so that exosomes can be widely used in the diagnosis and treatment of different diseases.
Despite the advantages of MSCs therapy, there are still problems with cell senescence and differentiation, and clinical outcomes are not promising (17). MSCs also have a growth-promoting effect on different types of cancer. Moreover, there are some risks associated with cell treatment, such as immune rejection, viral infection, storage methods and transport issues (21). MSCs-derived exosomes represent a new, cell-free drug that effectively attenuates clear systemic inflammation. Both topical and systemic administration have the potential to effectively suppress deleterious immune responses in inflamed tissues by reducing apoptotic and pro-inflammatory cytokines, alleviating cellular dysfunction, and promoting the survival and regeneration of damaged parenchymal cells (101). MSCs exert a therapeutic effect by paracrine action, MSCs-derived exosomes can replace their parental cells and have greater advantages: they cannot self-replicate, avoiding to a large extent the risk of tumorigenicity (102); as nanoparticles, exosomes are safer than cell therapy, they are biocompatible and have low immunogenicity, allowing them to cross blood-brain barrier (103); exosomes are protected by their lipid membrane structure by internal biomolecular activity and can be stored at -80°C for a long time without easy inactivation (104); Exosomes act as natural carriers of exogenous nucleic acids and drugs and can be loaded in donor cells and then released into the extracellular environment (105). In addition, exosome can also prevent miRNAs from being degraded, allowing miRNAs to negatively regulate the expression of target proteins (39).
Although there have been many preclinical studies involving MSCs -derived exosomes, several important issues remain unresolved. First, when MSCs from different tissues are in different states of differentiation, exosomes assembly may differ in molecular content and type, with significant tissue origin and age-dependent differences in immunosuppressive and tissue regenerative capacity, thus affecting their function in recipient cells and thus therapeutic efficacy. Secondly, the miRNA of MSCs-derived exosomes is not randomized into exosomes, however, the sorting mechanism that adjusts and selects cells from the parent cells is not known (106). Finally, the beneficial effects of MSCs-derived exosomes depend mainly on mRNA, miRNAs, anti-apoptotic and immunosuppressive proteins, and further clinical trials should confirm the exact disease-specific MSCs source molecules. In addition, route of administration and the precise dose of MSCs-derived exosomes should be determined for different inflammatory disease to prevent uncontrolled immunosuppression of MSCs-derived exosomes receptors. Different laboratories use different methods to isolate and purify MSCs-derived exosomes, therefore, an efficient method for standardizing the mass production of MSCs -derived exosomes is essential (107).
Before exosomes can be used in large numbers as clinical therapeutic agents, considerable researches are also needed to identify those components of exosomes that are of diagnostic and therapeutic importance, particularly as key targets in the regulation of inflammatory disease processes.
Most of the therapeutic effects of MSCs depend on their paracrine action, and exosomes are particularly suitable for studying disease diagnosis or prognosis due to their parental cellular properties. Exosomes also act as membrane nanocarriers that modify the activity and function of target cells through intercellular communication thus playing a role in inflammation. The growing interest in exosomes and the accumulating findings suggest that their role in inflammatory diseases is extensive and complex. Therefore, research should be continued to elucidate the properties of exosomes and the whole process that mediates the inflammatory response. Understanding the role of the various exosomal contents and their respective functions will be key to understanding the diagnostic and therapeutic tools that exosomes play. In addition to this, once key questions surrounding the isolation, transport, dosing, storage, donor and tissue sources of MSCs-derived exosomes are answered, it will realize its full potential as a new therapy in treatment inflammatory diseases. Continued extensive researches are still needed in the future to gain a deeper understanding of exosomes in treatment and diagnosis of inflammatory diseases and to provide new clinical insights and solutions.
YY design of the work and write the manuscript. YP, YLi and TS make the table of the manuscript; YLu and CY substantively revised the manuscript. All authors contributed to the article and approved the submitted version.
This study was financially supported by the National Natural Science Foundation of China (Nos. 81873943), and the National Key Research and Development Program of China (Nos. 2016YFC1000100).
The authors declare that the research was conducted in the absence of any commercial or financial relationships that could be construed as a potential conflict of interest.
All claims expressed in this article are solely those of the authors and do not necessarily represent those of their affiliated organizations, or those of the publisher, the editors and the reviewers. Any product that may be evaluated in this article, or claim that may be made by its manufacturer, is not guaranteed or endorsed by the publisher.
1. Dabrowska S, Andrzejewska A, Janowski M, Lukomska B. Immunomodulatory and regenerative effects of mesenchymal stem cells and extracellular vesicles: Therapeutic outlook for inflammatory and degenerative diseases. Front Immunol (2020) 11:591065. doi: 10.3389/fimmu.2020.591065
2. Gardner OF, Alini M, Stoddart MJ. Mesenchymal stem cells derived from human bone marrow. Methods Mol Biol (2015) 1340:41–52. doi: 10.1007/978-1-4939-2938-2_3
3. Shen Z, Huang W, Liu J, Tian J, Wang S, Rui K. Effects of mesenchymal stem cell-derived exosomes on autoimmune diseases. Front Immunol (2021) 12:749192. doi: 10.3389/fimmu.2021.749192
4. Dominici M, Le Blanc K, Mueller I, Slaper-Cortenbach I, Marini F, Krause D, et al. Minimal criteria for defining multipotent mesenchymal stromal cells. the international society for cellular therapy position statement. Cytotherapy (2006) 8(4):315–7. doi: 10.1080/14653240600855905
5. Charbord P. Bone marrow mesenchymal stem cells: historical overview and concepts. Hum Gene Ther (2010) 21(9):1045–56. doi: 10.1089/hum.2010.115
6. Bronckaers A, Hilkens P, Martens W, Gervois P, Ratajczak J, Struys T, et al. Mesenchymal stem/stromal cells as a pharmacological and therapeutic approach to accelerate angiogenesis. Pharmacol Ther (2014) 143(2):181–96. doi: 10.1016/j.pharmthera.2014.02.013
7. Preda MB, Rønningen T, Burlacu A, Simionescu M, Moskaug J, Valen G. Remote transplantation of mesenchymal stem cells protects the heart against ischemia-reperfusion injury. Stem Cells (2014) 32(8):2123–34. doi: 10.1002/stem.1687
8. Koniusz S, Andrzejewska A, Muraca M, Srivastava AK, Janowski M, Lukomska B. Extracellular vesicles in physiology, pathology, and therapy of the immune and central nervous system, with focus on extracellular vesicles derived from mesenchymal stem cells as therapeutic tools. Front Cell Neurosci (2016) 10:109. doi: 10.3389/fncel.2016.00109
9. Mellman I, Emr SD. A Nobel prize for membrane traffic: vesicles find their journey's end. J Cell Biol (2013) 203(4):559–61. doi: 10.1083/jcb.201310134
10. Kalluri R, LeBleu VS. The biology, function, and biomedical applications of exosomes. Science (2020) 367(6478):eaau6977. doi: 10.1126/science.aau6977
11. Santamaria G, Brandi E, Vitola P, Grandi F, Ferrara G, Pischiutta F, et al. Intranasal delivery of mesenchymal stem cell secretome repairs the brain of alzheimer's mice. Cell Death Differ (2021) 28(1):203–18. doi: 10.1038/s41418-020-0592-2
12. Su J, Ge X, Jiang N, Zhang Z, Wu X. Efficacy of mesenchymal stem cells from human exfoliated deciduous teeth and their derivatives in inflammatory diseases therapy. Curr Stem Cell Res Ther (2022) 17(4):302–16. doi: 10.2174/1574888x17666220417153309
13. Chan BD, Wong WY, Lee MM, Cho WC, Yee BK, Kwan YW, et al. Exosomes in inflammation and inflammatory disease. Proteomics (2019) 19(8):e1800149. doi: 10.1002/pmic.201800149
14. Chen L, Xiang B, Wang X, Xiang C. Exosomes derived from human menstrual blood-derived stem cells alleviate fulminant hepatic failure. Stem Cell Res Ther (2017) 8(1):9. doi: 10.1186/s13287-016-0453-6
15. Chen L, Lu FB, Chen DZ, Wu JL, Hu ED, Xu LM, et al. BMSCs-derived miR-223-containing exosomes contribute to liver protection in experimental autoimmune hepatitis. Mol Immunol (2018) 93:38–46. doi: 10.1016/j.molimm.2017.11.008
16. Mead B, Tomarev S. Bone marrow-derived mesenchymal stem cells-derived exosomes promote survival of retinal ganglion cells through miRNA-dependent mechanisms. Stem Cells Transl Med (2017) 6(4):1273–85. doi: 10.1002/sctm.16-0428
17. Kou M, Huang L, Yang J, Chiang Z, Chen S, Liu J, et al. Mesenchymal stem cell-derived extracellular vesicles for immunomodulation and regeneration: a next generation therapeutic tool? Cell Death Dis (2022) 13(7):580. doi: 10.1038/s41419-022-05034-x
18. Bonucci E. Fine structure of early cartilage calcification. J Ultrastruct Res (1967) 20(1):33–50. doi: 10.1016/s0022-5320(67)80034-0
19. Anderson HC. Vesicles associated with calcification in the matrix of epiphyseal cartilage. J Cell Biol (1969) 41(1):59–72. doi: 10.1083/jcb.41.1.59
20. Colombo M, Raposo G, Théry C. Biogenesis, secretion, and intercellular interactions of exosomes and other extracellular vesicles. Annu Rev Cell Dev Biol (2014) 30:255–89. doi: 10.1146/annurev-cellbio-101512-122326
21. Zhou T, Yuan Z, Weng J, Pei D, Du X, He C, et al. Challenges and advances in clinical applications of mesenchymal stromal cells. J Hematol Oncol (2021) 14(1):24. doi: 10.1186/s13045-021-01037-x
22. Johnstone RM, Adam M, Hammond JR, Orr L, Turbide C. Vesicle formation during reticulocyte maturation. association of plasma membrane activities with released vesicles (exosomes). J Biol Chem (1987) 262(19):9412–20.
23. Mathieu M, Martin-Jaular L, Lavieu G, Théry C. Specificities of secretion and uptake of exosomes and other extracellular vesicles for cell-to-cell communication. Nat Cell Biol (2019) 21(1):9–17. doi: 10.1038/s41556-018-0250-9
24. Racchetti G, Meldolesi J. Extracellular vesicles of mesenchymal stem cells: Therapeutic properties discovered with extraordinary success. Biomedicines (2021) 9(6):667. doi: 10.3390/biomedicines9060667
25. Gurung S, Perocheau D, Touramanidou L, Baruteau J. The exosome journey: from biogenesis to uptake and intracellular signalling. Cell Commun Signal (2021) 19(1):47. doi: 10.1186/s12964-021-00730-1
26. Varderidou-Minasian S, Lorenowicz MJ. Mesenchymal stromal/stem cell-derived extracellular vesicles in tissue repair: challenges and opportunities. Theranostics (2020) 10(13):5979–97. doi: 10.7150/thno.40122
27. Song XJ, Zhang L, Li Q, Li Y, Ding FH, Li X. hUCB-MSC derived exosomal miR-124 promotes rat liver regeneration after partial hepatectomy via downregulating Foxg1. Life Sci (2021) 265:118821. doi: 10.1016/j.lfs.2020.118821
28. Gao H, Jin Z, Bandyopadhyay G, Cunha ERK, Liu X, Zhao H, et al. MiR-690 treatment causes decreased fibrosis and steatosis and restores specific kupffer cell functions in NASH. Cell Metab (2022) 34(7):978–990.e974. doi: 10.1016/j.cmet.2022.05.008
29. Liu H, Liang Z, Wang F, Zhou C, Zheng X, Hu T, et al. Exosomes from mesenchymal stromal cells reduce murine colonic inflammation via a macrophage-dependent mechanism. JCI Insight (2019) 4(24):e131273. doi: 10.1172/jci.insight.131273
30. Wong WY, Lee MM, Chan BD, Kam RK, Zhang G, Lu AP, et al. Proteomic profiling of dextran sulfate sodium induced acute ulcerative colitis mice serum exosomes and their immunomodulatory impact on macrophages. Proteomics (2016) 16(7):1131–45. doi: 10.1002/pmic.201500174
31. Wang X, Liu D, Zhang X, Yang L, Xia Z, Zhang Q. Exosomes from adipose-derived mesenchymal stem cells alleviate sepsis-induced lung injury in mice by inhibiting the secretion of IL-27 in macrophages. Cell Death Discovery (2022) 8(1):18. doi: 10.1038/s41420-021-00785-6
32. Liu X, Gao C, Wang Y, Niu L, Jiang S, Pan S. BMSC-derived exosomes ameliorate LPS-induced acute lung injury by miR-384-5p-Controlled alveolar macrophage autophagy. Oxid Med Cell Longev (2021) 2021:9973457. doi: 10.1155/2021/9973457
33. Bai S, Yin Q, Dong T, Dai F, Qin Y, Ye L, et al. Endothelial progenitor cell-derived exosomes ameliorate endothelial dysfunction in a mouse model of diabetes. BioMed Pharmacother (2020) 131:110756. doi: 10.1016/j.biopha.2020.110756
34. Lin F, Zhang S, Liu X, Wu M. Mouse bone marrow derived mesenchymal stem cells-secreted exosomal microRNA-125b-5p suppresses atherosclerotic plaque formation via inhibiting Map4k4. Life Sci (2021) 274:119249. doi: 10.1016/j.lfs.2021.119249
35. Ma D, Xu K, Zhang G, Liu Y, Gao J, Tian M, et al. Immunomodulatory effect of human umbilical cord mesenchymal stem cells on T lymphocytes in rheumatoid arthritis. Int Immunopharmacol (2019) 74:105687. doi: 10.1016/j.intimp.2019.105687
36. Ding Y, Wang L, Wu H, Zhao Q, Wu S. Exosomes derived from synovial fibroblasts under hypoxia aggravate rheumatoid arthritis by regulating Treg/Th17 balance. Exp Biol Med (2020) 245(14):1177–86. doi: 10.1177/1535370220934736
37. Riazifar M, Mohammadi MR, Pone EJ, Yeri A, Lässer C, Segaliny AI, et al. Stem cell-derived exosomes as nanotherapeutics for autoimmune and neurodegenerative disorders. ACS Nano (2019) 13(6):6670–88. doi: 10.1021/acsnano.9b01004
38. Zhang J, Buller BA, Zhang ZG, Zhang Y, Lu M, Rosene DL, et al. Exosomes derived from bone marrow mesenchymal stromal cells promote remyelination and reduce neuroinflammation in the demyelinating central nervous system. Exp Neurol (2022) 347:113895. doi: 10.1016/j.expneurol.2021.113895
39. Vader P, Mol EA, Pasterkamp G, Schiffelers RM. Extracellular vesicles for drug delivery. Adv Drug Delivery Rev (2016) 106(Pt A):148–56. doi: 10.1016/j.addr.2016.02.006
40. Monsel A, Zhu YG, Gennai S, Hao Q, Hu S, Rouby JJ, et al. Therapeutic effects of human mesenchymal stem cell-derived microvesicles in severe pneumonia in mice. Am J Respir Crit Care Med (2015) 192(3):324–36. doi: 10.1164/rccm.201410-1765OC
41. Roehlen N, Crouchet E, Baumert TF. Liver fibrosis: Mechanistic concepts and therapeutic perspectives. Cells (2020) 9(4):875. doi: 10.3390/cells9040875
42. Tadokoro T, Morishita A, Masaki T. Diagnosis and therapeutic management of liver fibrosis by MicroRNA. Int J Mol Sci (2021) 22(15):8139. doi: 10.3390/ijms22158139
43. Li T, Yan Y, Wang B, Qian H, Zhang X, Shen L, et al. Exosomes derived from human umbilical cord mesenchymal stem cells alleviate liver fibrosis. Stem Cells Dev (2013) 22(6):845–54. doi: 10.1089/scd.2012.0395
44. Ohara M, Ohnishi S, Hosono H, Yamamoto K, Yuyama K, Nakamura H, et al. Extracellular vesicles from amnion-derived mesenchymal stem cells ameliorate hepatic inflammation and fibrosis in rats. Stem Cells Int (2018) 2018:3212643. doi: 10.1155/2018/3212643
45. Qu Y, Zhang Q, Cai X, Li F, Ma Z, Xu M, et al. Exosomes derived from miR-181-5p-modified adipose-derived mesenchymal stem cells prevent liver fibrosis via autophagy activation. J Cell Mol Med (2017) 21(10):2491–502. doi: 10.1111/jcmm.13170
46. Hou X, Yin S, Ren R, Liu S, Yong L, Liu Y, et al. Myeloid-Cell-Specific IL-6 signaling promotes MicroRNA-223-Enriched exosome production to attenuate NAFLD-associated fibrosis. Hepatology (2021) 74(1):116–32. doi: 10.1002/hep.31658
47. Markandey M, Bajaj A, Ilott NE, Kedia S, Travis S, Powrie F, et al. Gut microbiota: sculptors of the intestinal stem cell niche in health and inflammatory bowel disease. Gut Microbes (2021) 13(1):1990827. doi: 10.1080/19490976.2021.1990827
48. Ocansey DKW, Zhang L, Wang Y, Yan Y, Qian H, Zhang X, et al. Exosome-mediated effects and applications in inflammatory bowel disease. Biol Rev Camb Philos Soc (2020) 95(5):1287–307. doi: 10.1111/brv.12608
49. Hoter A, Naim HY. The functions and therapeutic potential of heat shock proteins in inflammatory bowel disease-an update. Int J Mol Sci (2019) 20(21):5331. doi: 10.3390/ijms20215331
50. Hou Q, Huang J, Ayansola H, Masatoshi H, Zhang B. Intestinal stem cells and immune cell relationships: Potential therapeutic targets for inflammatory bowel diseases. Front Immunol (2020) 11:623691. doi: 10.3389/fimmu.2020.623691
51. Cooks T, Pateras IS, Jenkins LM, Patel KM, Robles AI, Morris J, et al. Mutant p53 cancers reprogram macrophages to tumor supporting macrophages via exosomal miR-1246. Nat Commun (2018) 9(1):771. doi: 10.1038/s41467-018-03224-w
52. Li J, Tan M, Xiang Q, Zhou Z, Yan H. Thrombin-activated platelet-derived exosomes regulate endothelial cell expression of ICAM-1 via microRNA-223 during the thrombosis-inflammation response. Thromb Res (2017) 154:96–105. doi: 10.1016/j.thromres.2017.04.016
53. Soontararak S, Chow L, Johnson V, Coy J, Wheat W, Regan D, et al. Mesenchymal stem cells (MSC) derived from induced pluripotent stem cells (iPSC) equivalent to adipose-derived MSC in promoting intestinal healing and microbiome normalization in mouse inflammatory bowel disease model. Stem Cells Transl Med (2018) 7(6):456–67. doi: 10.1002/sctm.17-0305
54. Long ME, Mallampalli RK, Horowitz JC. Pathogenesis of pneumonia and acute lung injury. Clin Sci (Lond) (2022) 136(10):747–69. doi: 10.1042/cs20210879
55. Jones Buie JN, Zhou Y, Goodwin AJ, Cook JA, Vournakis J, Demcheva M, et al. Application of deacetylated poly-N-Acetyl glucosamine nanoparticles for the delivery of miR-126 for the treatment of cecal ligation and puncture-induced sepsis. Inflammation (2019) 42(1):170–84. doi: 10.1007/s10753-018-0882-8
56. Guervilly C, Lacroix R, Forel JM, Roch A, Camoin-Jau L, Papazian L, et al. High levels of circulating leukocyte microparticles are associated with better outcome in acute respiratory distress syndrome. Crit Care (2011) 15(1):R31. doi: 10.1186/cc9978
57. Park EJ, Appiah MG, Myint PK, Gaowa A, Kawamoto E, Shimaoka M. Exosomes in sepsis and inflammatory tissue injury. Curr Pharm Des (2019) 25(42):4486–95. doi: 10.2174/1381612825666191116125525
58. Li L, Jin S, Zhang Y. Ischemic preconditioning potentiates the protective effect of mesenchymal stem cells on endotoxin-induced acute lung injury in mice through secretion of exosome. Int J Clin Exp Med (2015) 8(3):3825–32.
59. Ye C, Li H, Bao M, Zhuo R, Jiang G, Wang W. Alveolar macrophage - derived exosomes modulate severity and outcome of acute lung injury. Aging (Albany NY) (2020) 12(7):6120–8. doi: 10.18632/aging.103010
60. Sun L, Zhu M, Feng W, Lin Y, Yin J, Jin J, et al. Exosomal miRNA let-7 from menstrual blood-derived endometrial stem cells alleviates pulmonary fibrosis through regulating mitochondrial DNA damage. Oxid Med Cell Longev (2019) 2019:4506303. doi: 10.1155/2019/4506303
61. Shen W, Zhao X, Li S. Exosomes derived from ADSCs attenuate sepsis-induced lung injury by delivery of circ-fryl and regulation of the miR-490-3p/SIRT3 pathway. Inflammation (2022) 45(1):331–42. doi: 10.1007/s10753-021-01548-2
62. Dinh PC, Paudel D, Brochu H, Popowski KD, Gracieux MC, Cores J, et al. Inhalation of lung spheroid cell secretome and exosomes promotes lung repair in pulmonary fibrosis. Nat Commun (2020) 11(1):1064. doi: 10.1038/s41467-020-14344-7
63. Wu X, Liu Z, Hu L, Gu W, Zhu L. Exosomes derived from endothelial progenitor cells ameliorate acute lung injury by transferring miR-126. Exp Cell Res (2018) 370(1):13–23. doi: 10.1016/j.yexcr.2018.06.003
64. Xiao K, He W, Guan W, Hou F, Yan P, Xu J, et al. Mesenchymal stem cells reverse EMT process through blocking the activation of NF-κB and hedgehog pathways in LPS-induced acute lung injury. Cell Death Dis (2020) 11(10):863. doi: 10.1038/s41419-020-03034-3
65. Gao M, Yu T, Liu D, Shi Y, Yang P, Zhang J, et al. Sepsis plasma-derived exosomal miR-1-3p induces endothelial cell dysfunction by targeting SERP1. Clin Sci (2021) 135(2):347–65. doi: 10.1042/cs20200573
66. Wei X, Yi X, Lv H, Sui X, Lu P, Li L, et al. MicroRNA-377-3p released by mesenchymal stem cell exosomes ameliorates lipopolysaccharide-induced acute lung injury by targeting RPTOR to induce autophagy. Cell Death Dis (2020) 11(8):657. doi: 10.1038/s41419-020-02857-4
67. Xia L, Zhang C, Lv N, Liang Z, Ma T, Cheng H, et al. AdMSC-derived exosomes alleviate acute lung injury via transferring mitochondrial component to improve homeostasis of alveolar macrophages. Theranostics (2022) 12(6):2928–47. doi: 10.7150/thno.69533
68. Libby P. The changing landscape of atherosclerosis. Nature (2021) 592(7855):524–33. doi: 10.1038/s41586-021-03392-8
69. Jebari-Benslaiman S, Galicia-García U, Larrea-Sebal A, Olaetxea JR, Alloza I, Vandenbroeck K, et al. Pathophysiology of atherosclerosis. Int J Mol Sci (2022) 23(6):3346. doi: 10.3390/ijms23063346
70. Wang C, Li Z, Liu Y, Yuan L. Exosomes in atherosclerosis: performers, bystanders, biomarkers, and therapeutic targets. Theranostics (2021) 11(8):3996–4010. doi: 10.7150/thno.56035
71. Hutcheson JD, Goettsch C, Bertazzo S, Maldonado N, Ruiz JL, Goh W, et al. Genesis and growth of extracellular-vesicle-derived microcalcification in atherosclerotic plaques. Nat Mater (2016) 15(3):335–43. doi: 10.1038/nmat4519
72. Lu M, Yuan S, Li S, Li L, Liu M, Wan S. The exosome-derived biomarker in atherosclerosis and its clinical application. J Cardiovasc Transl Res (2019) 12(1):68–74. doi: 10.1007/s12265-018-9796-y
73. Tabas I, Bornfeldt KE. Macrophage phenotype and function in different stages of atherosclerosis. Circ Res (2016) 118(4):653–67. doi: 10.1161/circresaha.115.306256
74. Nguyen MA, Karunakaran D, Geoffrion M, Cheng HS, Tandoc K, Perisic Matic L, et al. Extracellular vesicles secreted by atherogenic macrophages transfer MicroRNA to inhibit cell migration. Arterioscler Thromb Vasc Biol (2018) 38(1):49–63. doi: 10.1161/atvbaha.117.309795
75. Zhang YG, Song Y, Guo XL, Miao RY, Fu YQ, Miao CF, et al. Exosomes derived from oxLDL-stimulated macrophages induce neutrophil extracellular traps to drive atherosclerosis. Cell Cycle (2019) 18(20):2674–84. doi: 10.1080/15384101.2019.1654797
76. Zhu J, Liu B, Wang Z, Wang D, Ni H, Zhang L, et al. Exosomes from nicotine-stimulated macrophages accelerate atherosclerosis through miR-21-3p/PTEN-mediated VSMC migration and proliferation. Theranostics (2019) 9(23):6901–19. doi: 10.7150/thno.37357
77. Yang W, Zou B, Hou Y, Yan W, Chen T, Qu S. Extracellular vesicles in vascular calcification. Clin Chim Acta (2019) 499:118–22. doi: 10.1016/j.cca.2019.09.002
78. Chen NX, O'Neill KD, Moe SM. Matrix vesicles induce calcification of recipient vascular smooth muscle cells through multiple signaling pathways. Kidney Int (2018) 93(2):343–54. doi: 10.1016/j.kint.2017.07.019
79. Lo Sasso G, Schlage WK, Boué S, Veljkovic E, Peitsch MC, Hoeng J. The apoe(-/-) mouse model: a suitable model to study cardiovascular and respiratory diseases in the context of cigarette smoke exposure and harm reduction. J Transl Med (2016) 14(1):146. doi: 10.1186/s12967-016-0901-1
80. Chen S, Zhou H, Zhang B, Hu Q. Exosomal miR-512-3p derived from mesenchymal stem cells inhibits oxidized low-density lipoprotein-induced vascular endothelial cells dysfunction via regulating Keap1. J Biochem Mol Toxicol (2021) 35(6):1–11. doi: 10.1002/jbt.22767
81. Bouchareychas L, Duong P, Covarrubias S, Alsop E, Phu TA, Chung A, et al. Macrophage exosomes resolve atherosclerosis by regulating hematopoiesis and inflammation via MicroRNA cargo. Cell Rep (2020) 32(2):107881. doi: 10.1016/j.celrep.2020.107881
82. Radu AF, Bungau SG. Management of rheumatoid arthritis: An overview. Cells (2021) 10(11):2857. doi: 10.3390/cells10112857
83. Liu H, Li R, Liu T, Yang L, Yin G, Xie Q. Immunomodulatory effects of mesenchymal stem cells and mesenchymal stem cell-derived extracellular vesicles in rheumatoid arthritis. Front Immunol (2020) 11:1912. doi: 10.3389/fimmu.2020.01912
84. Karami Fath M, Azami J, Jaafari N, Akbari Oryani M, Jafari N, Karim Poor A, et al. Exosome application in treatment and diagnosis of b-cell disorders: leukemias, multiple sclerosis, and arthritis rheumatoid. Cell Mol Biol Lett (2022) 27(1):74. doi: 10.1186/s11658-022-00377-x
85. Wang LT, Liu KJ, Sytwu HK, Yen ML, Yen BL. Advances in mesenchymal stem cell therapy for immune and inflammatory diseases: Use of cell-free products and human pluripotent stem cell-derived mesenchymal stem cells. Stem Cells Transl Med (2021) 10(9):1288–303. doi: 10.1002/sctm.21-0021
86. Withrow J, Murphy C, Liu Y, Hunter M, Fulzele S, Hamrick MW. Extracellular vesicles in the pathogenesis of rheumatoid arthritis and osteoarthritis. Arthritis Res Ther (2016) 18(1):286. doi: 10.1186/s13075-016-1178-8
87. Payandeh Z, Pirpour Tazehkand A, Azargoonjahromi A, Almasi F, Alagheband Bahrami A. The role of cell organelles in rheumatoid arthritis with focus on exosomes. Biol Proced Online (2021) 23(1):20. doi: 10.1186/s12575-021-00158-4
88. Wu LF, Zhang Q, Mo XB, Lin J, Wu YL, Lu X, et al. Identification of novel rheumatoid arthritis-associated MiRNA-204-5p from plasma exosomes. Exp Mol Med (2022) 54(3):334–45. doi: 10.1038/s12276-022-00751-x
89. Zheng J, Zhu L, Iok In I, Chen Y, Jia N, Zhu W. Bone marrow-derived mesenchymal stem cells-secreted exosomal microRNA-192-5p delays inflammatory response in rheumatoid arthritis. Int Immunopharmacol (2020) 78:105985. doi: 10.1016/j.intimp.2019.105985
90. Tavasolian F, Hosseini AZ, Soudi S, Naderi M. miRNA-146a improves immunomodulatory effects of MSC-derived exosomes in rheumatoid arthritis. Curr Gene Ther (2020) 20(4):297–312. doi: 10.2174/1566523220666200916120708
91. Lim MK, Yoo J, Sheen DH, Ihm C, Lee SK, Kim SA. Serum exosomal miRNA-1915-3p is correlated with disease activity of Korean rheumatoid arthritis. In Vivo (2020) 34(5):2941–5. doi: 10.21873/invivo.12124
92. Meng HY, Chen LQ, Chen LH. The inhibition by human MSCs-derived miRNA-124a overexpression exosomes in the proliferation and migration of rheumatoid arthritis-related fibroblast-like synoviocyte cell. BMC Musculoskelet Disord (2020) 21(1):150. doi: 10.1186/s12891-020-3159-y
93. Dobson R, Giovannoni G. Multiple sclerosis - a review. Eur J Neurol (2019) 26(1):27–40. doi: 10.1111/ene.13819
94. Mycko MP, Baranzini SE. microRNA and exosome profiling in multiple sclerosis. Mult Scler (2020) 26(5):599–604. doi: 10.1177/1352458519879303
95. Sidoryk-Węgrzynowicz M, Dąbrowska-Bouta B, Sulkowski G, Strużyńska L. Nanosystems and exosomes as future approaches in treating multiple sclerosis. Eur J Neurosci (2021) 54(9):7377–404. doi: 10.1111/ejn.15478
96. Regev K, Healy BC, Paul A, Diaz-Cruz C, Mazzola MA, Raheja R, et al. Identification of MS-specific serum miRNAs in an international multicenter study. Neurol Neuroimmunol Neuroinflamm (2018) 5(5):e491. doi: 10.1212/nxi.0000000000000491
97. Ebrahimkhani S, Beadnall HN, Wang C, Suter CM, Barnett MH, Buckland ME, et al. Serum exosome MicroRNAs predict multiple sclerosis disease activity after fingolimod treatment. Mol Neurobiol (2020) 57(2):1245–58. doi: 10.1007/s12035-019-01792-6
98. Lazarus HM, Haynesworth SE, Gerson SL, Rosenthal NS, Caplan AI. Ex vivo expansion and subsequent infusion of human bone marrow-derived stromal progenitor cells (mesenchymal progenitor cells): implications for therapeutic use. Bone Marrow Transplant (1995) 16(4):557–64.
99. Kordelas L, Rebmann V, Ludwig AK, Radtke S, Ruesing J, Doeppner TR, et al. MSC-derived exosomes: a novel tool to treat therapy-refractory graft-versus-host disease. Leukemia (2014) 28(4):970–3. doi: 10.1038/leu.2014.41
100. López-Beas J, Guadix JA, Clares B, Soriano-Ruiz JL, Zugaza JL, Gálvez-Martín P. An overview of international regulatory frameworks for mesenchymal stromal cell-based medicinal products: From laboratory to patient. Med Res Rev (2020) 40(4):1315–34. doi: 10.1002/med.21659
101. Harrell CR, Jovicic N, Djonov V, Arsenijevic N, Volarevic V. Mesenchymal stem cell-derived exosomes and other extracellular vesicles as new remedies in the therapy of inflammatory diseases. Cells (2019) 8(12):1605. doi: 10.3390/cells8121605
102. Xunian Z, Kalluri R. Biology and therapeutic potential of mesenchymal stem cell-derived exosomes. Cancer Sci (2020) 111(9):3100–10. doi: 10.1111/cas.14563
103. Milbank E, Dragano NRV, González-García I, Garcia MR, Rivas-Limeres V, Perdomo L, et al. Small extracellular vesicle-mediated targeting of hypothalamic AMPKα1 corrects obesity through BAT activation. Nat Metab (2021) 3(10):1415–31. doi: 10.1038/s42255-021-00467-8
104. Zhuang WZ, Lin YH, Su LJ, Wu MS, Jeng HY, Chang HC, et al. Mesenchymal stem/stromal cell-based therapy: mechanism, systemic safety and biodistribution for precision clinical applications. J BioMed Sci (2021) 28(1):28. doi: 10.1186/s12929-021-00725-7
105. Herrmann IK, Wood MJA, Fuhrmann G. Extracellular vesicles as a next-generation drug delivery platform. Nat Nanotechnol (2021) 16(7):748–59. doi: 10.1038/s41565-021-00931-2
106. Kordelas L, Schwich E, Dittrich R, Horn PA, Beelen DW, Börger V, et al. Individual immune-modulatory capabilities of MSC-derived extracellular vesicle (EV) preparations and recipient-dependent responsiveness. Int J Mol Sci (2019) 20(7):1642. doi: 10.3390/ijms20071642
Keywords: stem cells, exosome, treatment, inflammatory diseases, diagnose
Citation: Yang Y, Peng Y, Li Y, Shi T, Luan Y and Yin C (2023) Role of stem cell derivatives in inflammatory diseases. Front. Immunol. 14:1153901. doi: 10.3389/fimmu.2023.1153901
Received: 30 January 2023; Accepted: 02 March 2023;
Published: 14 March 2023.
Edited by:
Frank Alexander Schildberg, University Hospital Bonn, GermanyReviewed by:
Qi Yang, Second Affiliated Hospital of Dalian Medical University, ChinaCopyright © 2023 Yang, Peng, Li, Shi, Luan and Yin. This is an open-access article distributed under the terms of the Creative Commons Attribution License (CC BY). The use, distribution or reproduction in other forums is permitted, provided the original author(s) and the copyright owner(s) are credited and that the original publication in this journal is cited, in accordance with accepted academic practice. No use, distribution or reproduction is permitted which does not comply with these terms.
*Correspondence: Yingyi Luan, bHVhbnlpbmd5aTJAMTYzLmNvbQ==; Chenghong Yin, eWluY2hoQGNjbXUuZWR1LmNu
Disclaimer: All claims expressed in this article are solely those of the authors and do not necessarily represent those of their affiliated organizations, or those of the publisher, the editors and the reviewers. Any product that may be evaluated in this article or claim that may be made by its manufacturer is not guaranteed or endorsed by the publisher.
Research integrity at Frontiers
Learn more about the work of our research integrity team to safeguard the quality of each article we publish.