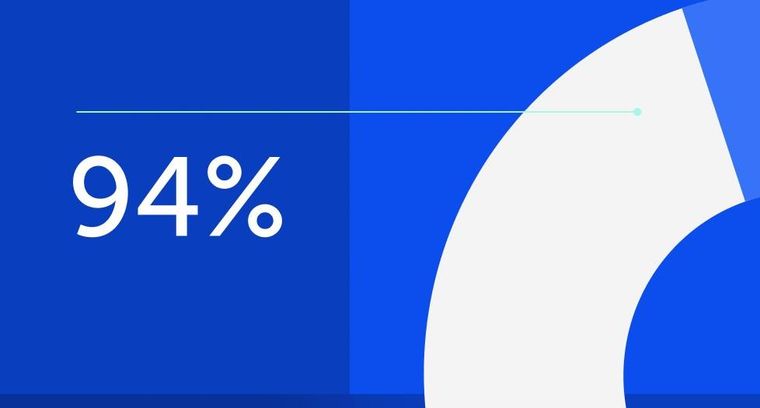
94% of researchers rate our articles as excellent or good
Learn more about the work of our research integrity team to safeguard the quality of each article we publish.
Find out more
ORIGINAL RESEARCH article
Front. Immunol., 30 March 2023
Sec. Autoimmune and Autoinflammatory Disorders : Autoimmune Disorders
Volume 14 - 2023 | https://doi.org/10.3389/fimmu.2023.1149869
Introduction: Systemic sclerosis (SSc) is a rare chronic autoimmune disease characterized by diffuse fibrosis of the skin and internal organs and vascular abnormalities. The etiology and physiopathology are complex due to the heterogeneity of its overall clinical presentation. Arsenic trioxide (ATO) has been proven to be effective against SSc, sclerodermatous Graft-versus-Host Disease, multiple sclerosis, Crohn’s disease or systemic lupus erythematosus animal models and has demonstrated promising effects in human clinical trials. Its efficacy was shown to be related at least in part to the generation of Reactive Oxygen Species (ROS) and the selective deletion of activated immune cells and fibroblasts. However, ATO can induce some adverse effects that must be considered, especially when used for the treatment of a chronic disease.
Methods: We evaluate here, in vitro and in a mouse model of SSc, the improved efficacy of ATO when associated with a Fenton-like divalent cation, namely copper chloride (CuCl2), also known to trigger the production of ROS.
Results: In preliminary experiments in vitro, ATO 1 µM + CuCl2 0.5 µM increased ROS production and increased apoptosis of NIH 3T3 murine fibroblasts compared to 1 µM ATO alone. In vivo, in the HOCl-induced mouse model of SSc, co-treatment with ATO 2.5 μg/g + CuCl2 0.5 μg/g significantly alleviated clinical signs such as the thickening of the skin (p<0.01) and cutaneous fibrosis, in a manner equivalent to treatment with ATO 5 µg/g. Our results provide evidence that co-treatment with ATO 2.5 μg/g + CuCl2 0.5 μg/g decreases the number of B cells and the activation of CD4+ T lymphocytes. The co-treatment substantially blocks the NRF2 signaling pathway, increases H2O2 production and results in the improvement of the health status of mice with experimental SSc.
Conclusion: In conclusion, copper combined with ATO treatment halved the concentration of ATO needed to obtain the same effect as a high dose of ATO alone for the treatment of SSc mice. The strategy of using lower doses of drugs with different mechanisms of action in combination has many potential advantages, the first being to lessen the potential side effects induced by ATO, a drug with side effects quickly increased with dosage.
Systemic sclerosis is a complex autoimmune disease characterized by autoimmunity, vasculopathy and progressive connective tissue fibrosis, particularly of the skin and visceral organs (1, 2). SSc causes extensive systemic tissue damage and death occurs as the result of end‐stage organ failure. Among connective autoimmune and inflammatory diseases, SSc is the one with the highest mortality rate per number of cases. Numerous studies suggest that reactive oxygen species (ROS) play an important role in the pathogenesis and development of this disease in both mouse models and humans (3, 4). In SSc, ROS are continuously produced by diseased fibroblasts, leading to their proliferation and the production of collagen and extensive fibrosis (5). Arsenic trioxide (ATO) has features of a pro-oxidant chemotherapeutic agent that increases ROS production, consumption of glutathione (GSH), intracellular oxidoreductase interference and reduction of thioredoxin activity (6). In a previous study, we showed that ATO decreases HOCl-induced skin and lung fibrosis in a HOCl-induced SSc mouse model (7). These beneficial effects were shown to be accompanied by ROS generation that adds its effects to the constitutive production of ROS by SSc fibroblasts, resulting in the selective killing of diseased fibroblasts containing low levels of GSH. ATO is currently used to treat APL, but repeated daily high doses of ATO over several weeks have been reported to induce adverse effects on liver, heart and peripheral nerves. Although these side effects are reversible to some extent, their occurrence often leads to the interruption or even the cessation of ATO therapy (8). Moreover, SSc is a chronic connective tissue disease and its treatment needs to take into account the effect of, and potentially avoid, excessively high, toxic cumulative doses. As increasing ROS in diseased fibroblasts has been shown to be effective for the treatment of SSc with ATO, we sought to find a way of associating with ATO a drug that could further increase ROS production in diseased fibroblasts but without the documented side effects of ATO. The well-known Fenton chemistry is the oxidation process activated by Fe(II) salts in the presence of H2O2 that generates radical species in solution and oxidizes a wide range of organic substrates with high activity (9). A Fenton-like reaction is based on the same principle with other metals (10). The production of ROS by these reactions is known to induce a strong oxidative stress in activated cells leading to their apoptotic death (11–13). We have previously tested several divalent cations (copper, iron, manganese, gold, zinc) for their ability to potentiate in vitro the pro-oxidative effect of ATO on human and murine cancer cells (14). Among them Cu2+ (in its CuCl2 molecular form) showed the most significant effects on the induction of oxidative stress and apoptosis of cancer cells when used along with ATO. In the present study, we associated CuCl2 and ATO in in vitro experiments on activated mouse fibroblasts, which are key players in the pathophysiological processes of SSc, and we also tested this combination in vivo in a mouse model of HOCl-induced SSc.
CuCl2 (GMP grade batches) was obtained from ChemCon (Freiburg, Germany) and ATO (Arscimed, clinical grade batch, stock solution at 1 mg/mL) was obtained from MEDSENIC (Strasbourg, France).
NIH 3T3, a mouse embryonic fibroblast cell line, was purchased from ATCC (ATCC CRL-1648). Cells were maintained throughout the experiments in a 75-cm2 flask (Falcon 250 mL 75 cm2, #353135) in Dulbecco’s Modified Eagle Medium (Sigma-Aldrich, Saint-Quentin- Fallavier, France) containing 10% fetal bovine serum (FBS) (Gibco, USA), 1% penicillin-streptomycin (Gibco, USA), 1% ciprofloxacin (Fresenius Kabi, France) and 1% fungizone (Gibco, USA).
Cells were seeded in 96-well flat-bottom plates (Falcon, Corning, #353077) and incubated for 48 hours under different experimental conditions at 37°C and 5% CO2. Three plates were prepared in parallel to measure H2O2 production and cell viability.
Fibroblasts were coated in triplicate in 96-well microplates (2.104 cells/well). After 48 hours of treatment with ATO ± CuCl2, the supernatant was removed and 50 µL per well of 50 µg/mL 2’, 7’-dichlorodihydrofluorescein di-acetate (H2DCF-DA; Sigma-Aldrich, Saint-Quentin-Fallavier, France) in PBS was added.
The H2O2 production was assessed by spectrofluorimetry using a fusion spectrofluorometer (Packard). Fluorescence intensity was recorded immediately (T0 hours) and after 6 hours of incubation (T6 hours). Fluorescence excitation/emission maxima were, for H2DCF-DA, 485/530 nm.
The medium was removed and cells were stained with 0.5% crystal violet and 30% ethanol in PBS for 30 minutes at room temperature. After two PBS washes, ethanol was added to the pellet and absorbance was measured at 550 nm using a fusion spectrofluorometer (15).
Six-week-old female BALB/c mice (H2d) were purchased from Janvier laboratory (Le Genest-Saint-Isle, France) and maintained with food and water ad libitum. The animals were given humane care, in accordance with the guidelines of our institution (Université Paris Cité, Paris). The protocols and all experimental procedures of this study were approved by the Ethics Committee of Paris Cité University (Animal facilities C75-14-05, DAP #26065).
HOCl was produced extemporaneously by adding 140 μL of NaClO (9.6% as active chorine) to 18 mL of KH2PO4 solution (100 mM; pH 7.2). HOCl was controlled by spectrophotometry at 292 nm and then adjusted to obtain an optical density between 0.7 and 0.9 as previously described (5).
Mice were randomly distributed into experimental (n=10 per group) and control groups (n=5). HOCl (200 μL) was injected with a 27-gauge needle intradermally into the shaved backs of the mice, 5 days a week for 6 weeks. Only 5 mice were used in the control group to reduce the number of animals and follow the 3R rule, as we know from previous experiments that this number is sufficient for statistically significant results in this SSc model (SSc mice). PBS-control mice received the same pattern of injections with 200 μL of PBS.
We used this well-established mouse model of SSc, triggered by intradermal injections of HOCl (7). SSc mice were randomized and treated with intraperitoneal injections of ATO ± CuCl2 or vehicle (PBS) for 36 days. Mice were divided into five groups.
In vivo the treatment by ATO 5 µg/g conditions was selected according to literature data and to our previous results in cGvHD and SSc mice models. In order to reduce the cumulative dose of injected ATO, CuCl2 was used as co-treatment with two times decreased dose of ATO (2.5 µg/g). Preliminary tests showed that a high dose of CuCl2 (4 µg/g) alone is toxic for mice (data not shown). Finally, a low dose of CuCl2, equivalent to doses used in food supplements, potentiated the effect of a low dose of ATO (2.5 µg/g) to the same level as that of a high dose of ATO (5 µg/g), as studied in a mouse model of cGvHD (14). Therefore, we used the CuCl2 0.5 µg/g dosage.
The HOCl-SSc-control mice received PBS and the four remaining groups received a daily injection of ATO alone 2.5 µg/g or 5 µg/g or ATO 2.5 µg/g with CuCl2 0.5 µg/g or CuCl2 alone 0.5 µg/g, 5 days a week during 6 weeks.
Skin thickness of the shaved backs of the mice was measured every week with a calliper (expressed in millimeters). These histogram results shown in Figure 2B correspond to day 35 measurements.
Total mRNA was extracted from crushed samples using trizol reagent (ThermoFisher Scientific, USA, #15596026) according to the manufacturer’s protocol. The expression level of α-SMA, Collagen I, NRF2, NQO1, GCLC and CCL22 were evaluated by RT-qPCR in the skin. The QuantiTect SYBR® Green RT-qPCR Kit on a LightCycler 480 II instrument (Roche Applied Science, France) was used to perform one-step RT-qPCR. It was carried out for 45 cycles, with a denaturing phase of 15 seconds at 94°C, an annealing phase of 30 seconds at 60°C and an extension of 30 seconds at 72°C. Samples were normalized to mRNA expression of housekeeping genes (β-actin) and results were expressed as fold increase using the formula 2−ΔΔCt. Primers used for PCR are listed in Supplementary Table 1.
For each mouse, spleen cell suspensions were prepared after hypotonic lysis of erythrocytes in potassium acetate solution (ACK: NH4Cl 0.15 M + KHCO3 1 mM + Na2EDTA 0.1 mM) followed by three washes in complete RPMI medium with 10% heat-inactivated FBS, 1% streptomycin-penicillin, 1% ciprofloxacin, 1% fungizone and 1% sodium pyruvate.
Splenocytes were prepared as described above. Cells were incubated with the appropriate labelled antibody at 4°C for 30 minutes in PBS with 2% FBS. Flow cytometry was performed on a FACS Fortessa II flow cytometer (BD Biosciences) according to standard techniques. For the characterization of splenocytes, the monoclonal antibodies used were: CD3-AF700 (17A2, #100216), CD4-BV510 (GK1.5, #100449), CD25-PE (PC61.5, #12-0251-81), B220-Pacific Blue (RA3-6B2, #103230), CD69-PE DAZZLE (H1.2F3, #104536), CD11b-PerCP Cy5.5 (M1/70, #101228), F4/80-BV711 (BM8, #123147), CD86-BV510 (GL-1, #105039), from eBiosciences (ThermoFisher Scientific, France). Data were analyzed with FlowJo software (Tree Star, Ashland, OR, USA).
Spleen cell suspensions (2.106 cells/well) were seeded in 12-well plates and incubated for 3 hours for the adhesion of macrophages; then the supernatant containing the lymphocytes was collected and placed in another 12-well plate. Macrophages and lymphocytes were cultured with complete RPMI medium with 10% heat-inactivated FBS, 1% streptomycin-penicillin, 1% sodium pyruvate, 1% ciprofloxacin and 1% fungizone. Macrophages were stimulated for 48 hours with a final concentration of 100 ng/mL LPS (from E. coli serotype 0127: B8 from Sigma Aldrich #L319) and lymphocytes for 72 hours with a final concentration of 5 µg/mL of concanavalin A (Sigma-Aldrich, Saint-Quentin Fallavier, France). The supernatant was collected and stored at -80°C.
Assessment of IL-17A, IL-6 and TGF-β was performed using specific mouse ELISA kits from eBiosciences (Invitrogen - ThermoFisher Scientific, France). Concentrations were calculated from a standard curve according to the manufacturer’s protocol.
The results were analyzed with GraphPad Prism8. A one-way test ANOVA with Bonferroni’s correction was used to determine the differences between two experimental groups. A difference with p<0.05 was considered as significant. All of the quantitative data are expressed as mean ± standard error of the mean (SEM).
We measured the effects of ATO (1 µM) with or without CuCl2 (0.5 µM) on the production of H2O2 by NIH 3T3 cells and on cell viability (Figure 1A). We observed an increase in H2O2 production of 59% (p<0.0001) when the cells were treated with ATO (1 µM) and CuCl2 compared to untreated cells and also to cells treated with ATO alone or CuCl2 alone at the same dosage (p<0.01; Figure 1B). Concerning cell viability, the co-treatment of cells with CuCl2 and ATO decreased cellular viability by 20% compared to untreated cells (p<0.01). CuCl2 in combination with ATO treatment potentiated the effect of ATO alone or CuCl2 alone at the same dosage (p<0.05 and p<0.01, respectively; Figure 1C).
Figure 1 Pro-oxidant effect of ATO 1 μM + CuCl2 on NIH 3T3 cells. (A) Schematic representation of the in vitro experiment on NIH 3T3 cells. The cells were treated for 48 hours with ATO at 1 μM +/- CuCl2. The H2O2 and survival of NIH 3T3 cells were measured by absorbance. (B) Standardized H2O2 level produced by NIH 3T3 cells in culture after treatment during 48 hours with one concentration of ATO (1 µM) and with CuCl2 (0.5 µM). H2DCF-DA was measured by spectrofluorometry and the results (AU) were divided by cell viability established by colorimetry (crystal violet). (C) Cell viability of NIH 3T3 cells in culture measured after treatment during 48 hours with one concentration of ATO (1 µM) and with CuCl2 (0.5 µM). NS: not significant; *p<0.05; **p<0.01; ****p<0.0001. The results are the mean of 3 replicates per sample.
As expected, repeated injections of HOCl in BALB/c mice (HOCl-SSc mice) (Figure 2A) induced a 73% increase in skin thickness at day 35 compared to the PBS-control group (Figure 2B; p<0.0001). The co-treatment with ATO 2.5 μg/g + CuCl2 significantly decreased skin thickness by 23% compared to the SSc-control group (p<0.05). This effect was equivalent to that resulting from the treatment of animals with a high dose of ATO (5 μg/g), which decreased skin thickness by 24% compared to the SSc-control group (p<0.05). However, the addition of CuCl2 to a low dose of ATO (2.5 µg/g) was significantly more effective to decrease dermal thickness than the same low dose of ATO alone (p<0.05 Figure 2B). Expression of the two pro-fibrotic markersα-SMA and Collagen I was increased by 69% and 87%, respectively, in the HOCl-SSc- mice compared to the PBS-control group (p<0.01 and p<0.05, respectively). Treatment with ATO 2.5 μg/g + CuCl2 decreased by 70% the expression of α-SMA (p<0.0001) and by 85% the expression of collagen I (p<0.0001), compared to the HOCl-SSc-mice. A high dose of ATO alone decreased by 43% the expression of α-SMA (p<0.01) and by 86% the expression of collagen I (p<0.0001) compared to the HOCl-SSc-mice. No significant differences were observed between HOCl-SSc mice treated with PBS, with CuCl2 alone and with low dose ATO alone (Figures 2C, D).
Figure 2 Effects of ATO 2.5 μg/g + CuCl2 on skin fibrosis. (A) Schematic representation of the experimental induction of SSc in mice. The mice received 5 injections of HOCl per week for 6 weeks intradermally as well as the various treatments intraperitoneally. Mice were monitored weekly for weight and clinical signs of SSc for 36 days. (B) Evolution of skin-fold thickness in millimeters at day 35. (C, D) α-SMA and Collagen I mRNA expression in the skin. Data are presented as 2(−ΔΔCT) relative to the levels of β-actin. *p<0.05; **p<0.01; ****p<0.0001. The results for each group are the mean of the measurements obtained per mouse: PBS-control (n = 5); SSc-control (n = 10); SSc-CuCl2 0.5 µg/g (n = 9, because 1 mouse died); SSc-ATO 2.5 µg/g (n = 9); SSc-ATO 2.5 µg/g + CuCl2 0.5 µg/g (n = 10); SSc-ATO 5 µg/g (n = 10). Ex vivo measurements were realized in duplicate for each mouse.
NRF2 is highly expressed in the diseased HOCl-SSc-mice compared to the PBS-control group (relative expression in PBS-control group = 1 and relative expression in HOCl-SSc-control group = 6.0699; p<0.05). The co-treatment and treatment with high dose ATO decreased the expression of NRF2 by 81% and 99.6%, respectively (p<0.01) (Figure 3A). We also assessed the effect of these treatments on NQO1 and GCLC, two target genes of the NRF2 pathway. The expression of the two target genes was increased in the HOCl-SSc-control group compared to the PBS-control group: p<0.01 for NQO1 (relative expression in PBS-control group = 1 and relative expression in HOCl-SSc-control group = 20.5954) and p<0.05 for GCLC (relative expression in PBS-control group = 1 and relative expression in HOCl-SSc-control group = 6.6559). Co-treatment with ATO+Cu2+ and treatment with high dose of ATO significantly reduced both NQO1 and GCLC gene expression compared to the HOCl-SSc-mice, by 98% and 99%, respectively, for NQO1 and by 79% and 88%, respectively, for GCLC (p<0.001 and p<0.05, respectively, for NQO1; p<0.01 and p<0.01, respectively, for GCLC). CuCl2 potentiated the effect of low dose ATO, which reached an equivalent level to that observed with a high dose of ATO (Figures 3B, C).
Figure 3 Mechanism of action of ATO 2.5 μg/g + CuCl2. (A–C) NRF2, NQO1 and GCLC mRNA expression in the skin. Data are presented as 2(−ΔΔCT) relative to the levels of β-actin. *p<0.05; **p<0.01; ***p<0.001. The results for each group are the mean of the measurements obtained per mouse: PBS-control (n = 5); SSc-control (n = 10); SSc-CuCl2 0.5 µg/g (n = 9); SSc-ATO 2.5 µg/g (n = 9); SSc-ATO 2.5 µg/g + CuCl2 0.5 µg/g (n = 10); SSc-ATO 5 µg/g (n = 10). Ex vivo measurements were realized in duplicate for each mouse.
On the day of sacrifice, we evaluated the effect of the co-treatment on the number of immune cells and on their activation status using flow cytometry. We observed an increase of 44% in the percentage of total B lymphocytes in the HOCl-SSc-mice compared to the PBS-control group (p<0.05). The co-treatment with ATO 2.5 μg/g + CuCl2 significantly decreased the B lymphocytes count by 28% (p<0.001) compared to the HOCl-SSc-control group. High dose ATO (5 μg/g) decreased the B lymphocyte count by 42% (p<0.0001) compared to the HOCl-SSc-control group (Figure 4A). However, despite the decrease in the B lymphocyte count induced by the co-treatment with ATO+CuCl2, we did not observe any difference in B cell activation status between experimental groups (Figure 4B).
Figure 4 Effects of ATO 2.5 μg/g + CuCl2 on innate and adaptive immune cells. (A–D) Flow cytometry of splenic B and CD4+ T cells. Splenic B cells were gated on CD3- B220+. Splenic CD4+ T cells were gated on CD3+ CD4+ positive cells. (E, F) Flow cytometry of splenic macrophages. Macrophages were gated on F4/80+ CD11b+. *p <0.05; **p<0.01; ***p<0.001; ****p<0.0001. The results for each group are the mean of the measurements obtained per mouse: PBS-control (n = 5); SSc-control (n = 10); SSc-CuCl2 0.5 µg/g (n = 9); SSc-ATO 2.5 µg/g (n = 9); SSc-ATO 2.5 µg/g + CuCl2 0.5 µg/g (n = 10); SSc-ATO 5 µg/g (n = 10).
Furthermore, we did not observe any modification in the percentage of CD4+ T lymphocytes in the different experimental groups (Figure 4C). Interestingly, the induction of SSc by HOCl was followed by an increase of 36% in CD4+ T lymphocyte activation (CD25+) compared to the PBS-control group (p<0.05). ATO 2.5 μg/g + CuCl2 significantly decreased CD25 levels on CD4+ T lymphocytes by 23% (p<0.01) compared to the HOCl-SSc-mice and reached the level observed in the PBS-control group and in the HOCl-SSc group treated with high dose ATO 5 μg/g (p<0.05) (Figure 4D). High dose ATO decreased the CD25 levels on CD4+ T lymphocytes by 21% (p<0.05) compared to the HOCl-SSc-control group.
Finally, the percentage of macrophages in the spleen was higher in the HOCl-SSc-control group than in the control group (p<0.0001) (Figure 4E). We found that the co-treatment had no influence on the number of macrophages. However, a 43% decrease in the number of macrophages was observed in the group treated with high-dose ATO (5 μg/g) compared to the HOCl-SSc-control group (p<0.0001) (Figure 4E). We did not observe any differences in macrophage activation status regardless of the experimental group considered (Figure 4F).
Co-treatment with ATO 2.5 μg/g + CuCl2 significantly reduced by 17% the expression of IL-17A by lymphocytes compared to the HOCl-SSc-control group (p<0.05) (Figure 5A). The co-treatment acted similarly to a high dose of ATO on the expression of IL-17A by lymphocytes (-18%, p<0.01). In our study, we showed an increased expression of CCL-22 in the skin of HOCl-SSc mice compared to the PBS-control group (p<0.05). This expression was reduced by the co-treatment with ATO 2.5 μg/g + CuCl2 and by a high concentration of ATO compared to HOCl-SSc mice (-95% p<0.01 and -99.3% p<0.05, respectively) (Figure 5B).
Figure 5 Effects of ATO 2.5 μg/g + CuCl2 on cytokines production. (A) ELISA assessment of IL-17 production by lymphocytes from the spleen after stimulation with concanavalin (A, B) CCL22 mRNA expression in the skin. Data are presented as 2(−ΔΔCT) relative to the levels of β-actin. (C, D) ELISA assessment of IL-6 and TGF-β production by macrophages from the spleen after stimulation with LPS. The results for each group are the mean of the measurements obtained per mouse: PBS-control (n = 5); SSc-control (n = 10); SSc-CuCl2 0.5 µg/g (n = 9); SSc-ATO 2.5 µg/g (n = 9); SSc-ATO 2.5 µg/g + CuCl2 0.5 µg/g (n = 10); SSc-ATO 5 µg/g (n = 10). *p <0.05; **p<0.01; ***p<0.001. Ex vivo measurements were realized in duplicate for each mouse.
In macrophages, IL-6 production was significantly increased and TGF-β also tended to be higher in HOCl-SSc mice versus PBS-control mice but any of the treatments applied to animals in the experimental groups resulted in a modification of cytokine production compared to untreated HOCl-SSc mice (Figures 5C, D).
ATO is an inorganic salt used as a treatment for APL and is under investigation for some autoimmune diseases (16–18); however, toxic side effects are a concern (19). ATO has been shown to be the most effective single agent for the treatment of APL and, following co-treatment with ATO and all-trans retinoic acid, long-term survival of patients with APL has increase to 80–90% (20). An increasing number of preclinical studies have consistently shown that ATO is effective against non-cancerous disorders, especially autoimmune diseases. In the past decade, clinical data have shown that, while most side effects of ATO are mild and can include fatigue, nausea, vomiting, diarrhea, abdominal pain and peripheral neuropathy, excessive use of ATO can also induce hepatotoxicity, nephrotoxicity and cardiotoxicity (21–23). Most of these studies showed that ATO-induced organ damage is dose dependent; therefore, limiting ATO cumulative dosage would reduce these side effects. The use of ATO has recently been highlighted for the treatment of chronic autoimmune diseases. In such disorders, the acceptability of side effects is obviously lower than in more severe diseases, such as cancer. Therefore, the use of diminished amounts of ATO in treating chronic autoimmune diseases is mandatory. Our recent studies have shown that ATO can cure scleroderma in mice (7). This beneficial effect was shown to be mediated through ROS generation by ATO, which adds its effects to the constitutive production of ROS by SSc fibroblasts to selectively kill them as they contain too low levels of GSH. In the present study, we used CuCl2 to induce an oxidative burst through a Fenton-like reaction to potentiate the beneficial effects of ATO, thereby enabling us to reduce the ATO dosage and the risk of side effects. The co-treatment was tested in a mouse model of SSc and effectively reduced cutaneous fibrosis by inducing ROS production and cell death in activated diseased fibroblasts through a drop in the NRF2 signaling pathway. In parallel, the co-treatment altered the activation of immune cells to decrease inflammation and fibrosis, both of which are hallmarks of SSc.
With regard to the results obtained in a previous work published in Frontiers in Immunology (14), we chose the lowest copper concentration that modified the GSH concentration and the survival rate in tumor cells. This choice was also determined by the fact that we wanted to use the lowest dose to avoid toxicity in animals. Indeed, preliminary tests showed that a high dose of CuCl2 (4 µg/g) alone is toxic to mice (data not shown). Furthermore, a low dose of CuCl2, equivalent to doses used in food supplements, is sufficient to potentiate the effect of a low dose of ATO (2.5 µg/g) to the same level as that of a high dose of ATO (5 µg/g), as studied in a mouse model of cGvHD. In this study, we first observed that the co-treatment induced oxidative stress in NIH 3T3 cells and increased cell death compared to the same dosage of ATO or CuCl2 alone. Accordingly, Kavian et al., showed that ATO induced strong oxidative stress with a drop in reduced GSH levels in activated diseased fibroblasts, able to selectively kill them (7). These activated fibroblasts are a key element in the development of SSc and their depletion through an induction of sufficiently strong oxidative stress explained the decreased severity of the disease. In vitro, CuCl2 potentiated the pro-oxidative effect of ATO alone on activated fibroblasts as, in the presence of H2O2, Cu2+ is able to generate through a Fenton-like reaction the highly toxic radical HO. that oxidized glutathione (GSSG) (13).
We subsequently evaluated the efficacy of ATO + CuCl2 in vivo, in our mouse model of HOCl-induced SSc. It has previously been reported that ATO is able to limit the occurrence of SSc induced by repeated injections of HOCl (7). We used the same model where BALB/c mice received daily subcutaneous injections of HOCl to induce the disease. A low dose of ATO combined with CuCl2 decreased skin thickness and the expression of pro-fibrotic markers (α-SMA and Collagen I) in the HOCl-induced SSc mice model. The addition of CuCl2 potentiated the effect of ATO alone at low doses to reach an effect as powerful as that observed for a high dose of ATO. The Fenton-like Cu2+ divalent cation associated with ATO improved ROS production to a threshold that deleted diseased fibroblasts. These results are similar to those of previous studies by Kavian et al., who showed that ATO decreased skin fibrosis in a mouse model of chronic cGvHD and in the mouse model of SSc (7, 24). Furthermore, we recently demonstrated that CuCl2 potentiates the therapeutic effect of low-dose ATO alone on skin fibrosis in a mouse model of sclerodermatous cGvHD (14). In humans, an effective dose (0.16 mg/kg) can induce side effects such as skin reactions, gastrointestinal disorders and a reversible increase in transaminases, leading to discontinuation of treatment (25). The use of half the dosage of ATO is of great importance for patients who will have to be treated over a long period.
The major pathway by which cells counteract high ROS levels is through the activation of the NRF2 antioxidant pathway (26, 27). Nuclear translocation of the transcription factor NRF2 leads to the transcription of antioxidant genes in cells (28). The loss of NRF2 in cancer cells increases oxidative stress, which can result in diminished tumorigenesis and increase the sensitivity to chemotherapy (29, 30). Treating SSc animals with a high dose of ATO at 5 μg/g or with co-treatment of ATO 2.5 μg/g + CuCl2 0.5 μg/g significantly reduced NRF2 expression compared to untreated HOCl-SSc animals. Here again, addition of CuCl2 improved the effect of ATO low dose. To confirm this effect of ATO 2.5 μg/g + CuCl2 0.5 μg/g on the NRF2 pathway, we quantified the expression of its two target genes, NQO1 and GCLC (31). The expression of NQO1 and GCLC was also significantly reduced by the co-treatment and by ATO high dose. As GCLC is a key enzyme for GSH synthesis and protection against oxidative stress, a drop in NRF2 expression and its target genes explains the high sensitivity of diseased fibroblasts treated with either a high dose of ATO or with the combination of ATO and CuCl2 (7). Our group has already explored the role of NRF2 in scleroderma and has shown that increasing NRF2 by dimethylfumarate (DMF) prevents HOCl-induced SSc. As in cancer cells, diseased SSc fibroblasts overexpress ROS, which increases their proliferation rates (32). A return to normal can be achieved either by decreasing ROS using anti-oxidant agents, such as with DMF, or by increasing ROS beyond the viability threshold to induce apoptosis of diseased activated cells, such as with ATO high dose or in combination with CuCl2.
SSc is a heterogeneous autoimmune disease characterized by interconnected hallmarks represented by aberrant immune activation and by fibroblast dysfunction leading to extracellular matrix deposition and fibrosis. The immune cells are largely involved in the pathophysiology of SSc. Their uncontrolled activation contributes to maintaining the fibro-inflammatory phenomenon of the disease. We therefore studied the ATO + CuCl2 co-treatment’s effect on the recruitment and activation of the innate and adaptive immune system. It has been described that CD4+ T lymphocytes are abnormally activated during the development of SSc and that this exacerbated activation contributes to the pathogenesis of the disease (33). B lymphocytes are also key elements in the development of SSc. Indeed, B lymphocytes have the ability to produce cytokines to maintain the loop of inflammation and fibrosis but also to produce autoantibodies (34). Whereas a low dose of ATO alone influenced neither the number of immune cells nor their activation status, we observed that associating CuCl2 with ATO 2.5 μg/g lowered the percentage of B lymphocytes and also reduced the activation of CD4+ T lymphocytes in a similar manner to ATO high dose. These results are in agreement with those of Zhao et al., who showed that ATO is able to induce a decrease in B lymphocytes but also in T lymphocyte activation (35).
The co-treatment did not decrease the percentage and the activation of macrophages in the spleen. Only ATO high dose was able to reduce the percentage and the activation of macrophages. As well, it has been shown that chronic exposure of cells to ATO induces immunocytotoxicity on human macrophages (36).
The mouse model we used reflects the phenotype observed in SSc patients, as they present increased levels of IL-6 and TGF-β in sera and tissue (37, 38). Co-treatment with ATO and CuCl2 significantly decreased the level of IL-17A in the spleen compared to low dose of ATO. Cytokines such as IL-17A are produced by Th17 lymphocytes. The main role of these cells is to protect the host against pathogens. However, this protection is associated with a risk of developing immune-mediated inflammatory diseases (39). An increase in Th17 cells and IL-17 cytokine was found in peripheral blood and target organs in animal models of SSc (39). In humans, this increase is associated with skin fibrosis (40). In addition, we observed that ATO 2.5 μg/g + CuCl2 decreased the expression of CCL22, a chemokine expressed by macrophages that attracts Th17 lymphocytes via the CCR4 receptor. CCL22 is increased in the serum of patients with diffuse or limited SSc compared to control patients. Higher rates of CCL22 were associated with pitting scars and younger ages at onset of the pathology (41).
Finally, our results are in agreement with data from the literature, which have shown that ATO diminishes the expression of the cytokine IL-17 produced by Th17 cells but also the chemokine CCL22 expressed by macrophages (42, 43).
Altogether, these results evidence the interest of combining ATO 2.5 μg/g with CuCl2 0.5 μg/g to treat SSc. The use of CuCl2 with ATO enabled us to treat mice with half of the amount of ATO, to obtain the same curative results. Being able to decrease the cumulative dose of any treatment is always a step forward. It helps to decrease or abrogate potential harmful effects but also avoids weakening the patients. Our data showed the important role of ROS in the effects of the combination of low-dose ATO associated with Cu2+ ions on overactivated fibroblasts (Graphical abstract). The association of CuCl2 with ATO treatment could also be effective in other autoimmune or pro-inflammatory diseases that begin with a loss of tolerance to modified self-antigens and immune system abnormalities, such as rheumatoid arthritis or systemic lupus erythematosus, where ROS are commonly used as destructive or modifying agents of cellular components that act as signaling molecules in and between immune cells.
The raw data supporting the conclusions of this article will be made available by the authors, without undue reservation.
The animal study was reviewed and approved by DAP #26065.
Conceptualization, Methodology, FR, FB. Investigation, CC, MT. Formal analysis, CC, CN, MT, DR-G, FB. Writing – Original Draft, CC, CN. Writing – Review CC, CN, FR, DR-G, FB. Funding Acquisition, FR. Supervision, FB, CN. All authors contributed to the article and approved the submitted version.
This work was supported by grants from MEDSENIC SAS.
The authors thank Véronique Pomi of MEDSENIC, Strasbourg, France for her helpful comments. We would also like to thank the Platform of Cytometry and Immunobiology Facility (CYBIO) of Cochin Institute, Paris, for flow cytometry, for the determination of biomarkers and data analysis.
FR and FB are listed as inventors for an early patent application family designation relative to the synergic use of arsenic salts and metallic ions for the treatment of autoimmune diseases. DR-G and FR are currently employees of MEDSENIC SAS.
The remaining authors declare that the research was conducted in the absence of any commercial or financial relationships that could be constructed as a potential conflict of interest.
All claims expressed in this article are solely those of the authors and do not necessarily represent those of their affiliated organizations, or those of the publisher, the editors and the reviewers. Any product that may be evaluated in this article, or claim that may be made by its manufacturer, is not guaranteed or endorsed by the publisher.
The Supplementary Material for this article can be found online at: https://www.frontiersin.org/articles/10.3389/fimmu.2023.1149869/full#supplementary-material
1. Barnes H, Holland AE, Westall GP, Goh NS, Glaspole IN. Cyclophosphamide for connective tissue disease-associated interstitial lung disease. Cochrane Database Syst Rev (2018) 1:CD010908. doi: 10.1002/14651858.CD010908.pub2
2. Perelas A, Arrossi AV, Highland KB. Pulmonary manifestations of systemic sclerosis and mixed connective tissue disease. Clin Chest Med (2019) 40(3):501−18. doi: 10.1016/j.ccm.2019.05.001
3. Sekiguchi A, Motegi SI, Fujiwara C, Yamazaki S, Inoue Y, Uchiyama A, et al. Inhibitory effect of kaempferol on skin fibrosis in systemic sclerosis by the suppression of oxidative stress. J Dermatol Sci (2019) 96(1):8−17. doi: 10.1016/j.jdermsci.2019.08.004
4. Łuczyñska M, Szkudlarek U, Dziankowska-Bartkowiak B, Waszczykowska E, Kasielski M, Sysa-Jedrzejowska A, et al. Elevated exhalation of hydrogen peroxide in patients with systemic sclerosis. Eur J Clin Invest (2003) 33(3):274−9. doi: 10.1046/j.1365-2362.2003.01138.x
5. Servettaz A, Goulvestre C, Kavian N, Nicco C, Guilpain P, Chéreau C, et al. Selective oxidation of DNA topoisomerase 1 induces systemic sclerosis in the mouse. J Immunol (2009) 182(9):5855−64. doi: 10.4049/jimmunol.0803705
6. Liu T, Sun L, Zhang Y, Wang Y, Zheng J. Imbalanced GSH/ROS and sequential cell death. J Biochem Mol Toxicol janv (2022) 36(1):e22942. doi: 10.1002/jbt.22942
7. Kavian N, Marut W, Servettaz A, Nicco C, Chéreau C, Lemaréchal H, et al. Reactive oxygen species-mediated killing of activated fibroblasts by arsenic trioxide ameliorates fibrosis in a murine model of systemic sclerosis. Arthritis Rheum (2012) 64(10):3430−40. doi: 10.1002/art.34534
8. Shen ZX, Chen GQ, Ni JH, Li XS, Xiong SM, Qiu QY, et al. Use of arsenic trioxide (As2O3) in the treatment of acute promyelocytic leukemia (APL): II. clinical efficacy and pharmacokinetics in relapsed patients. Blood (1997) 89(9):3354−60. doi: 10.1182/blood.V89.9.3354
9. Thomas C, Mackey MM, Diaz AA, Cox DP. Hydroxyl radical is produced via the fenton reaction in submitochondrial particles under oxidative stress: Implications for diseases associated with iron accumulation. Redox Rep (2009) 14(3):102−8. doi: 10.1179/135100009X392566
10. Hussain S, Aneggi E, Goi D. Catalytic activity of metals in heterogeneous fenton-like oxidation of wastewater contaminants: A review. Environ Chem Lett (2021) 19(3):2405−24. doi: 10.1007/s10311-021-01185-z
11. Xu X, Chen Y, Zhang Y, Yao Y, Ji P. Highly stable and biocompatible hyaluronic acid-rehabilitated nanoscale MOF-Fe2+ induced ferroptosis in breast cancer cells. J Mater Chem B (2020) 8(39):9129–38. doi: 10.1039/D0TB01616K
12. Tsvetkov P, Coy S, Petrova B, Dreishpoon M, Verma A, Abdusamad M, et al. Copper induces cell death by targeting lipoylated TCA cycle proteins. Science (2022) 375(6586):1254−61. doi: 10.1126/science.abf0529
13. Cao W, Jin M, Yang K, Chen B, Xiong M, Li X, et al. Fenton/Fenton-like metal-based nanomaterials combine with oxidase for synergistic tumor therapy. J Nanobiotechnol (2021) 19(1):325. doi: 10.1186/s12951-021-01074-1
14. Chêne C, Jeljeli MM, Rongvaux-Gaïda D, Thomas M, Rieger F, Batteux F, et al. A fenton-like cation can improve arsenic trioxide treatment of sclerodermatous chronic graft-versus-Host disease in mice. Front Immunol (2022) 13:917739. doi: 10.3389/fimmu.2022.917739
15. Ngô C, Chéreau C, Nicco C, Weill B, Chapron C, Batteux F. Reactive oxygen species controls endometriosis progression. Am J Pathol (2009) 175(1):225−34. doi: 10.2353/ajpath.2009.080804
16. Bobé P, Bonardelle D, Benihoud K, Opolon P, Chelbi-Alix MK. Arsenic trioxide: A promising novel therapeutic agent for lymphoproliferative and autoimmune syndromes in MRL/lpr mice. Blood (2006) 108(13):3967−75. doi: 10.1182/blood-2006-04-020610
17. An K, Xue MJ, Zhong JY, Yu SN, Lan TS, Qi ZQ, et al. Arsenic trioxide ameliorates experimental autoimmune encephalomyelitis in C57BL/6 mice by inducing CD4+ T cell apoptosis. J Neuroinflamm (2020) 17:147. doi: 10.1186/s12974-020-01829-x
18. Li C, Zhang J, Wang W, Wang H, Zhang Y, Zhang Z. Arsenic trioxide improves treg and Th17 balance by modulating STAT3 in treatment-naïve rheumatoid arthritis patients. . Int Immunopharmacol (2019) 73:539−51. doi: 10.1016/j.intimp.2019.05.001
19. Abaza Y, Kantarjian H, Garcia-Manero G, Estey E, Borthakur G, Jabbour E, et al. Long-term outcome of acute promyelocytic leukemia treated with all-trans-retinoic acid, arsenic trioxide, and gemtuzumab. Blood (2017) 129(10):1275−83. doi: 10.1182/blood-2016-09-736686
20. Lo-Coco F, Avvisati G, Vignetti M, Thiede C, Orlando SM, Iacobelli S, et al. Retinoic acid and arsenic trioxide for acute promyelocytic leukemia. N Engl J Med (2013) 369(2):111−21. doi: 10.1056/NEJMoa1300874
21. Messarah M, Klibet F, Boumendjel A, Abdennour C, Bouzerna N, Boulakoud MS, et al. Hepatoprotective role and antioxidant capacity of selenium on arsenic-induced liver injury in rats. Exp Toxicol Pathol (2012) 64(3):167−74. doi: 10.1016/j.etp.2010.08.002
22. Jin W, Xue Y, Xue Y, Han X, Song Q, Zhang J, et al. Tannic acid ameliorates arsenic trioxide-induced nephrotoxicity, contribution of NF-κB and Nrf2 pathways. Biomed Pharmacother (2020) 126:110047. doi: 10.1016/j.biopha.2020.110047
23. Vineetha VP, Raghu KG. An overview on arsenic trioxide-induced cardiotoxicity. Cardiovasc Toxicol (2019) 19(2):105−19. doi: 10.1007/s12012-018-09504-7
24. Kavian N, Marut W, Servettaz A, Laude H, Nicco C, Chéreau C, et al. Arsenic trioxide prevents murine sclerodermatous graft-versus-Host disease. JI (2012) 188(10):5142−9. doi: 10.4049/jimmunol.1103538
25. Kwong YL. Arsenic trioxide in the treatment of haematological malignancies. Expert Opin Drug Saf (2004) 3(6):589−97. doi: 10.1517/14740338.3.6.589
26. Hu X, Li L, Yan S, Li Z. Arsenic trioxide suppresses acute graft-versus-host disease by activating the Nrf2/HO-1 pathway in mice. Br J Haematol (2019) 186(5):e145−8. doi: 10.1111/bjh.15982
27. Nguyen LXT, Troadec E, Kalvala A, Kumar B, Hoang DH, Viola D, et al. The bcl-2 inhibitor venetoclax inhibits Nrf2 antioxidant pathway activation induced by hypomethylating agents in AML. J Cell Physiol (2019) 234(8):14040−9. doi: 10.1002/jcp.28091
28. Tu W, Wang H, Li S, Liu Q, Sha H. The anti-inflammatory and anti-oxidant mechanisms of the Keap1/Nrf2/ARE signaling pathway in chronic diseases. Aging Dis (2019) 10(3):637−51. doi: 10.14336/AD.2018.0513
29. DeNicola GM, Karreth FA, Humpton TJ, Gopinathan A, Wei C, Frese K, et al. Oncogene-induced Nrf2 transcription promotes ROS detoxification and tumorigenesis. Nature (2011) 475(7354):106−9. doi: 10.1038/nature10189
30. Sporn MB, Liby KT. NRF2 and cancer: the good, The bad and the importance of context. Nat Rev Cancer (2012) 12(8):10.1038/nrc3278. doi: 10.1038/nrc3278
31. Li L, Chen Y, Jiao D, Yang S, Li L, Li P. Protective effect of astaxanthin on ochratoxin a-induced kidney injury to mice by regulating oxidative stress-related NRF2/KEAP1 pathway. Molecules (2020) 25(6):1386. doi: 10.3390/molecules25061386
32. Laurent A, Nicco C, Chéreau C, Goulvestre C, Alexandre J, Alves A, et al. Controlling tumor growth by modulating endogenous production of reactive oxygen species. Cancer Res (2005) 65(3):948−56. doi: 10.1158/0008-5472.948.65.3
33. Liu M, Wu W, Sun X, Yang J, Xu J, Fu W, et al. New insights into CD4+ T cell abnormalities in systemic sclerosis. Cytokine Growth Factor Rev (2016) 28:31−6. doi: 10.1016/j.cytogfr.2015.12.002
34. Liossis SNC, Staveri C. The role of b cells in scleroderma lung disease pathogenesis. Front Med (2022) 9:936182. doi: 10.3389/fmed.2022.936182
35. Zhao B, Xia JJ, Wang LM, Gao C, Li JL, Liu JY, et al. Immunosuppressive effect of arsenic trioxide on islet xenotransplantation prolongs xenograft survival in mice. Cell Death Dis (2018) 9(3):1−11. doi: 10.1038/s41419-018-0446-8
36. Lemarie A, Morzadec C, Bourdonnay E, Fardel O, Vernhet L. Human macrophages constitute targets for immunotoxic inorganic arsenic. J Immunol (2006) 177(5):3019−27. doi: 10.4049/jimmunol.177.5.3019
37. O’Reilly S, Cant R, Ciechomska M, van Laar JM. Interleukin-6: A new therapeutic target in systemic sclerosis? clin trans immunol. avr (2013) 2(4):e4. doi: 10.1038/cti.2013.2
38. Ayers NB, Sun CM, Chen SY. Transforming growth factor-β signaling in systemic sclerosis. J Biomed Res (2018) 32(1):3. doi: 10.7555/JBR.31.20170034
39. Xu H, Agalioti T, Zhao J, Steglich B, Wahib R, Vesely MCA, et al. The induction and function of the anti-inflammatory fate of TH17 cells. Nat Commun (2020) 11(1):3334. doi: 10.1038/s41467-020-17097-5
40. Beirne P, Pantelidis P, Charles P, Wells AU, Abraham DJ, Denton CP, et al. Multiplex immune serum biomarker profiling in sarcoidosis and systemic sclerosis. Eur Respir J (2009) 34(6):1376−82. doi: 10.1183/09031936.00028209
41. Fujii H, Shimada Y, Hasegawa M, Takehara K, Sato S. Serum levels of a Th1 chemoattractant IP-10 and Th2 chemoattractants, TARC and MDC, are elevated in patients with systemic sclerosis. J Dermatol Sci (2004) 35(1):43−51. doi: 10.1016/j.jdermsci.2004.03.001
42. Morzadec C, Macoch M, Robineau M, Sparfel L, Fardel O, Vernhet L. Inorganic arsenic represses interleukin-17A expression in human activated Th17 lymphocytes. Toxicol Appl Pharmacol (2012) 262(3):217−22. doi: 10.1016/j.taap.2012.05.004
Keywords: arsenic trioxide, ATO, copper, fenton-like reaction, fibrosis, systemic sclerosis, Nrf2, ROS
Citation: Chêne C, Rongvaux-Gaïda D, Thomas M, Rieger F, Nicco C and Batteux F (2023) Optimal combination of arsenic trioxide and copper ions to prevent autoimmunity in a murine HOCl-induced model of systemic sclerosis. Front. Immunol. 14:1149869. doi: 10.3389/fimmu.2023.1149869
Received: 24 January 2023; Accepted: 06 March 2023;
Published: 30 March 2023.
Edited by:
Steven O’Reilly, STipe Therapeutics, DenmarkReviewed by:
Apostolos Zarros, Pharmacological Research Observatory, United KingdomCopyright © 2023 Chêne, Rongvaux-Gaïda, Thomas, Rieger, Nicco and Batteux. This is an open-access article distributed under the terms of the Creative Commons Attribution License (CC BY). The use, distribution or reproduction in other forums is permitted, provided the original author(s) and the copyright owner(s) are credited and that the original publication in this journal is cited, in accordance with accepted academic practice. No use, distribution or reproduction is permitted which does not comply with these terms.
*Correspondence: Frédéric Batteux, ZnJlZGVyaWMuYmF0dGV1eEBhcGhwLmZy; Carole Nicco, Y2Fyb2xlLm5pY2NvQGJpb3NlbmljLmNvbQ==
†These authors have contributed equally to this work and share last authorship
Disclaimer: All claims expressed in this article are solely those of the authors and do not necessarily represent those of their affiliated organizations, or those of the publisher, the editors and the reviewers. Any product that may be evaluated in this article or claim that may be made by its manufacturer is not guaranteed or endorsed by the publisher.
Research integrity at Frontiers
Learn more about the work of our research integrity team to safeguard the quality of each article we publish.