- 1Departamento de Enfermagem Materno-Infantil (DEMI), Faculdade de Enfermagem (FEnf), Universidade do Estado do Rio de Janeiro (UERJ), Rio de Janeiro, Brazil
- 2Programa de Oncovirologia, Instituto Nacional de Câncer (INCA), Rio de Janeiro, Brazil
- 3Programa de Pós-Graduação em Infecção HIV/aids e Hepatites Virais, Hospital Universitário Gaffrée e Guinle (HUGG/Ebserh), Universidade Federal do Estado do Rio de Janeiro (UNIRIO), Rio de Janeiro, Brazil
- 4Laboratório de Genômica Funcional e Transdução de Sinal, Departamento de Genética, Instituto de Biologia, Universidade Federal do Rio de Janeiro (UFRJ), Rio de Janeiro, Brazil
- 5Instituto de Ginecologia, Universidade Federal do Rio de Janeiro, Rio de Janeiro, Brazil
- 6Escola de Medicina e Cirurgia da Universidade Federal do Estado do Rio de Janeiro, Universidade Federal do Rio de Janeiro (UNIRIO), Rio de Janeiro, Brazil
- 7Hospital Universitário Clementino Fraga Filho, Universidade Federal do Rio de Janeiro, Rio de Janeiro, Brazil
The relationship among microbiome, immunity and cervical cancer has been targeted by several studies, yet many questions remain unanswered. We characterized herein the virome and bacteriome from cervical samples and correlated these findings with innate immunity gene expression in a Brazilian convenience sample of HPV-infected (HPV+) and uninfected (HPV-) women. For this purpose, innate immune gene expression data were correlated to metagenomic information. Correlation analysis showed that interferon (IFN) is able to differentially modulate pattern recognition receptors (PRRs) expression based on HPV status. Virome analysis indicated that HPV infection correlates to the presence of Anellovirus (AV) and seven complete HPV genomes were assembled. Bacteriome results unveiled that vaginal community state types (CST) distribution was independent of HPV or AV status, although bacterial phyla distribution differed between groups. Furthermore, TLR3 and IFNαR2 levels were higher in the Lactobacillus no iners-dominated mucosa and we detected correlations among RIG-like receptors (RLR) associated genes and abundance of specific anaerobic bacteria. Collectively, our data show an intriguing connection between HPV and AV infections that could foster cervical cancer development. Besides that, TLR3 and IFNαR2 seem to create a protective milieu in healthy cervical mucosa (L. no iners-dominated), and RLRs, known to recognize viral RNA, were correlated to anaerobic bacteria suggesting that they might be related to dysbiosis.
1 Introduction
Cervical cancer is the fourth most prevalent type of female tumor worldwide and the third most common cancer among Brazilian women (1, 2). Human papillomavirus (HPV) infection is necessary for cervical cancer development but other factors such as viral persistence, immunodeficiency and smoking are also associated with the disease (1, 3, 4). Moreover, cervical mucosa immune regulation and local microbiota also play pivotal roles in this process (5).
The female reproductive tract (FRT) has several protective components, including antimicrobial peptides (AMPs – small proteins released by different cell types with antimicrobial activity), and immune and epithelial cells (6). Besides acting as a physical barrier, epithelial cells express a wide repertory of pattern recognition receptors (PRRs), such as Toll-like receptors (TLRs) and RIG-like receptors (RLRs), responsible for recognizing conserved pathogen-associated molecular patterns (PAMPs) like nucleic acids and lipopolysaccharides from bacteria, fungi or viruses. TLRs are transmembrane receptors found in cell surface and intracellular membranes, that recognize LPS (TLR4) or nucleic acids (TLR3, TLR7, TLR8 and TLR9), while three receptors comprise the cytoplasmic RLR family – RIG-I, MDA5 and LGP2 – responsible for recognizing nucleic acids, especially non-self RNA forms (7). The pathways activated by these receptors are regulated at several levels, for instance, RIG-I activity is positively regulated by TRIM25 (8) while RNF125 mediated ubiquitination of both RIG-I and MDA5 results in their degradation (9). Once activated, both TLRs and RLRs, via different adaptor proteins, lead to engagement of transcription factors such as NFκB, IRF3, IRF7 that regulate cytokine and IFN production (6, 10). Pathogens impair these innate responses in several ways, including by handling ubiquitination machinery. In high-risk HPV (hr-HPV) infection, e.g, UCHL1 de-ubiquitinates TRAF3 and NEMO (adaptor proteins of TLRs and RLRs pathway), impairing cytokines and IFN-I production (11). These genes and cytokines are differentially expressed when comparing cervical cells from HPV-infected (HPV+) and uninfected (HPV-) women and may have important roles in HPV persistence or clearance and in susceptibility to STIs such as HIV infection (12–15). Furthermore, several studies suggest important roles of PRR expression and host microbiota in health and disease at mucosal sites (16–18), but little is known about the FRT.
Women’s vaginal bacteria are grouped based on community composition into five “community state types” (CSTs): CST I, II, III, IV and V, dominated by Lactobacillus crispatus, L. gasseri, L. iners, anaerobic bacteria (Gardnerella, Megasphera, Sneathia and Prevotella) and L. jensenii, respectively (3, 19). A healthy vaginal environment is dominated by Lactobacillus and shows low bacterial diversity (CSTs I, II or V), while a decreased concentration of Lactobacillus other than L. iners, increased diversity and anaerobic dominance typify a transitional mucosa (CST III-dominated) or dysbiosis (CST IV-dominated), the latter also known as bacterial vaginosis (BV) state (3, 15, 20). BV is commonly found in women of black (African) and Hispanic ethnicities and is also influenced by smoking and sexual activity (19, 21–24). Several studies associate CST III or IV and increasing microbiome diversity with HPV infection, cervical intraepithelial neoplasia (CIN), invasive cervical cancer (3, 25, 26) and HIV infection (27).
An initial characterization of the vaginal bacteriome of Brazilian women indicates that women under reproductive age analyzed in the study grouped into the five CSTs. Nevertheless, a predominance of CST III (36.5%), CST I (30.5%) and CST IV (27.4%) was found. In this population, factors like smoking, number of partners, demographic region of origin and vaginal douching were risk factors for dysbiosis, while ethnicity, educational level and condom use had no impact on CST dominance (28). Besides bacteria, the vaginal microbiota is also composed of a viral community able to infect either eukaryotic or prokaryotic cells. Several studies suggest that the host virome has an important role in innate and adaptative mucosal immunity and is linked to the development of BV, infertility, and adverse pregnancy outcomes such as prematurity and intrauterine growth restriction (29–31). Despite its importance, vaginal virome data are still scarce and further studies are required as they might contribute to the understanding of this complex system and how its components relate to each other in health and disease. To contribute to this understanding, herein we characterized the virome and bacteriome from cervical samples and correlated these findings with innate immunity gene expression. Although our sample size is limited, we obtained significant correlations when comparing HPV-infected or uninfected women. Virome data indicated that HPV infection is correlated to the presence of Anellovirus. Also, bacteriome results suggested that phyla distribution is different depending on HPV and Anellovirus status. When gene expression was assessed, we observed that type I IFN expression is correlated to TLR9 expression in HPV+ women, while it is correlated to TLR4 expression in HPV- samples. Moreover, when bacteriome data were assessed in conjunction with gene expression data, TLR3 and IFNαR2 levels were higher in the Lactobacillus no iners-dominated mucosa and we also detected correlations among RIG-I, MDA5, TRIM25, RNF125 and abundance of specific anaerobic bacteria.
2 Material and methods
2.1 Study patients, sample collection and ethical statements
Study participants were enrolled at Instituto de Ginecologia (IG), Universidade Federal do Rio de Janeiro (UFRJ), Brazil. This was a convenience sampling from studies of characterization of sexually transmitted infections (32) and cervical immunity analysis (12). During medical consultation in the cervical pathology and colposcopy outpatient clinic or in the gynecology ambulatory, we collected cervical epithelial cells with one cell scraper and two endocervical cytobrushes; 4 ml of whole blood was also collected from each woman. All material was used to diagnose STIs and perform other experiments as previously described (12, 32). In this study, we included cervical cells of a total of 22 women above 18 years of age who tested negative for chlamydia, trichomoniasis, syphilis, gonorrhea, HIV and HBV, and that had their innate immune genes previously quantified. They were classified as healthy women (HPV- n = 11; HPV-negative without cervical lesions) or HPV+ (n = 11; high-risk HPV-positive with high-grade squamous intraepithelial lesions (HSIL)) based on Sanger sequencing (12). Briefly, nested polymerase chain reaction (PCR) of gDNA was performed (My9/My11 primers followed by GP5+/GP6+ primers) and PCR product purified and sequenced by Sanger method. Sequences were compared to HPV sequences available in the GeneBank database (NCBI, NIH, Bethesda, USA) and the HPV type was assigned. The study was approved by the Ethical Committee of Instituto de Puericultura e Pediatria Martagão Gesteira, UFRJ (approval number: 49035215.4.0000.5264).
2.2 Circular DNA enrichment, sequencing and virome analysis
Total genomic DNA (gDNA) was extracted from cervical epithelial cells with the HiYield™ Genomic DNA Mini Kit (Blood/Bacteria/Cultured Cells) (Real Genomics, Taiwan). Circular DNA was enriched by rolling circle amplification (RCA) with the Illustra TempliPhi Amplification kit (GE Healthcare Life Sciences, Piscataway, NJ, USA). We prepared sequencing libraries using two nanograms of purified RCA product and the Nextera XT DNA Sample Preparation kit (Illumina Inc., San Diego, CA, USA). One HPV- patient was excluded from downstream analyses due to lack of sufficient gDNA. Samples were sequenced in an Illumina MiSeq platform (2 x 100 nt reads).
Reads with Phred quality score below 28 were trimmed with Sickle (33) and the remaining was mapped to the human reference genome hg19 using BWA (34) to remove human reads. Non-human reads were compared to viral sequences using BLASTX (35) and the virus protein database from RefSeq. Hits with an e-value below 0.01 were submitted to the BLASTX against the GenBank’s non-redundant database. Reads were classified as virus/non-virus and the virus family was defined according to the most similar sequence found in the database. Retroviridae virus family was excluded to avoid biased analysis since most reads from this family were removed during the disposal of human reads. Virus relative abundance was calculated by dividing the number of reads assigned to each virus family per the total of trimmed reads submitted to BLASTX. Reads were also assembled to HPV reference genomes obtained from PAVE database (http://pave.niaid.nih.gov) using Geneious R11 (Biomatters, Auckland, New Zealand) and the consensus sequences representing the HPV complete genomes were extracted.
2.3 Bacterial community state type characterization
Total gDNA from cervical cells of 21 patients (10 HPV- and 11 HPV+) were used to PCR-amplify the 16S rRNA gene variable V3-V4 regions, generating an amplicon of ~460 bp, with the 16S Metagenomic Sequencing Library Preparation kit (Illumina, Inc.). PCR reactions were carried out using 1X Taq PCR buffer (minus Mg), 1.5 mM MgCl2, 0.2 mM dNTP mix, 0.2 µM each primer, 1.5 U Taq Platinum polymerase (Invitrogen, Carlsbad, CA, USA), ~12.5ng of template and final volume was adjusted with RNase/DNase free water (Life Technologies, Carlsbad, CA). The cycling conditions carried out were recommended by the manufacturer of the 16S library. PCR products were visualized by electrophoresis in 1.5% agarose gels and bands of the expected size were purified with the Illustra GFX PCR DNA and Gel Band Purification Kit (Merck KGaA, Darmstadt, Germany). Five microliters of purified 16S rRNA PCR products were then used along with Nextera XT DNA Sample Preparation kit (Illumina, Inc.) to prepare sequencing libraries with distinct barcodes each one. Libraries were diluted to a final concentration of 10 pM and samples were sequenced in an Illumina MiSeq platform (2 x 100 nt reads).
The BCL2FastQ2 Conversion Software (version 2.18, Illumina, Inc.) was used to demultiplex data and convert BCL files to FASTQ file formats. Reads were subjected to FastQC (Babraham Bioinformatics, Cambridge, CBE) for quality analysis, were denoised, filtered and joined using the Divisive Amplicon Denoising Algorithm DADA2 (36) using QIIME2 (37). Taxonomic classification was performed using the q2-feature-classifier QIIME2 plugin and the Greengenes Database (38), and bacterial taxonomic were defined by amplicon sequence variants (ASV) analysis in QIIME2.
Unsupervised hierarchical clustering based on Bray-Curtis dissimilarity and average linkage was applied to define clusters according to abundance and taxa diversity of each sample. The clusters found were associated with vaginal microbiome CSTs classified in previous studies (19). Clusterization, diversity analyses and plots were carried out using the R environment.
2.4 Gene expression
Gene expression analyses were carried out as previously described (12). Briefly, total mRNA was extracted from cervical cells and used as template for cDNA synthesis. We prepared customized Taqman array plates, except for IFNγ which we designed primers and used SYBR Green to measure gene expression levels. Reactions were performed in 7500 Fast Real-Time PCR System (Applied Biosystems). Here we selected the following genes to study: RNF125, UCHL1 (seven HPV+ and seven HPV- patients were assessed); TLR3, TLR4, TLR7, TLR9, DDX58 (RIG-I), IFIH1 (MDA5), IFNA2, IFNB1, IFNAR2, TRIM25, (11 HPV+ and 11 HPV-); IFNG (10 HPV+ and eight HPV- patients). TLR3, TLR4, TL7 and TLR9 are receptors of TLR family; DDX58 (RIG-I), IFIH1 (MDA5) belong to RLR family and are regulated by TRIM25 and RNF125; UCHL1 negatively regulate TLR and RLR pathways; IFNα2, IFNβ1 are IFN-I that interact with IFNαR2 amplifying innate immune response; and IFNγ is the type II IFN (IFN-II) mainly produced in adaptative immune response. GAPDH was used as housekeeping gene and relative expression calculated using the 2-ΔCt formula.
2.5 Statistical analysis
Sociodemographic data have been summarized with absolute and relative frequency for categorical variables and with mean and standard deviation (SD) or median and interquartile range for numerical variables. Differences between groups were calculated in IBM SPSS Statistics for Windows, version 25.0 (IBM Corporation, Armonk, NY). For categorical variables we used chi-square or Fisher exact test; for numerical variables we used Mann-Whitney U test to compare two groups and Kruskal-Wallis test, with Dunn’s multiple comparisons as a post-hoc test to compare three groups. Odds ratio (OR) was calculated using the free online statistical software MedCalc [https://www.medcalc.org/calc/odds_ratio.php]. Correlation analyses were carried out by Spearman’s correlation in SPSS (IBM Corporation), where r corresponds to correlation coefficient. Degrees of correlation were adapted from Schober and colleagues (39) as follow: 0.7 ≤ r ≤1 – strong correlation; 0.4≤ r < 0.7 – moderate correlation; and r < 0.4 – weak correlation.
To compare gene expression levels according to anellovirus status we used Mann-Whitney U test in SPSS (IBM Corporation), and according to CSTs we used Kruskall-Wallis test in GraphPad Prim 9 (GraphPad, California, USA).
3 Results
3.1 Patient sociodemographic characteristics
Patients were grouped based on their HPV status into HPV- and HPV+ women. HPV+ women were older, but in both groups the number of married, high school educated and smokers or past smokers were similar (Table 1). Approximately a quarter of the women used condom and/or hormonal contraceptive methods, and although data did not reach statistical significance, HPV+ women had their first sexual intercourse at a younger age, met more partners in life and had a history of previous STIs (Table 1). In order to evaluate if demographic characteristics could affect HPV outcome, we performed Odds Ratio (OR) analyses. Previous STIs were considered a risk factor (OR = 7.875, 95% Confidence interval (CI) = 1.105 – 56.123) and age ≥ 41 yr a protective factor (OR = 0.127, 95% CI = 0.018 – 0.905) for HPV infection.
3.2 HPV impacts IFN-I inducible gene expression
We evaluated the association between expression of studied genes and HPV status, classifying expression levels in high or low based on the median expression of each gene (low ≤ median; high > median). We observed associations between HPV+ status with IFNα2, TLR7, TLR3 and MDA5 expression using Fisher Exact Test and decided to perform OR analyses. These assessments indicate that HPV infection is linked to high IFN-I expression (IFNα2: OR = 38.3, 95% CI = 1.8 – 820.2; IFNβ1: OR = 12.0, 95% CI = 1.1 – 128.8) and to low levels of PRRs that recognize RNA (MDA5: OR = 0.0368, 95% CI = 0.002 – 0.777; TLR3: OR = 0.022, 95% CI = 0.002 – 0.289; TLR7: OR = 0.026, 95% CI = 0.0012 – 0.558) (Supplementary Table 1).
Besides OR evaluation, the correlations among gene expression levels were also calculated using Spearman’s correlation and only the significant results are presented here (Table 2). We observed that independently of HPV, IFN-α2, IFN-β1 (type I IFN) and IFN-γ (type II IFN) genes were strongly and positively correlated with each other, as expected (40) (Table 2). We then assessed genes that correlated with IFNs, since these cytokines activate several transcription factors that then activate IFN responsive genes, changing expression profiles. We found that genes that correlated with IFNs are different when comparing HPV- and HPV+ samples, suggesting that these cytokines differentially regulate gene expression in the two groups. In HPV+, type I IFNs (IFNα2 and IFNβ1) were positively correlated to TLR9 and negatively correlated to TLR3. In HPV- cervices however, mRNA expression of these cytokines was positively correlated to TLR4 expression, and IFNβ1 mRNA levels were also correlated to MDA5 expression. Interestingly, IFNγ expression was positively correlated to those of MDA5 and RNF125 only in HPV- samples (Table 2). The opposite correlation between TLR9 and TLR4 in the two groups corroborates the idea that several genes involved in innate immunity are differentially modulated during HPV infection. We also looked for correlations restricted to each group and found that the expression of MDA5 and RNF125 are correlated only in HPV- women, while expression of RIG-I and TLR3 are correlated solely in the HPV+ group.
3.3 Virome analyses
We enriched circular DNA using RCA to characterize circular DNA viruses in cervical samples. The total number of reads obtained per sample after trimming out low-quality reads was 21,535,051 (average: 1,025,479 ± 418,145.8). Overall, we detected two viral families, Papillomaviridae (98.8% of viral reads) and Anelloviridae (1.2% of viral reads) (Table 3). By next-generation sequencing (NGS), we detected HPV reads in a total of nine women, confirming HPV classification based on Sanger sequencing, except for two patients who had no HPV reads (IG13 and IG33), but whose HPV status was confirmed by PCR. Interestingly, patient IG31 was infected by HPV51 with a deletion of 30 nucleotides in the C-terminal DNA binding domain of E2. Reads from five samples assembled seven complete HPV genomes that were classified into HPV types, lineages and sublineages (Table 3). Three women were infected by more than one HPV type (IG36, IG62 and IG63), and one was infected by eight different HPVs, most of them hr-HPV types (Table 3).
Anellovirus (AV) reads were detected in seven women (six out of seven are HPV+) and we decided to group study participants by status of this viral family (AV- = 14 women; AV+ = 7 women) and performed OR analyses. First, we observed that being infected by HPV favors Anellovirus infection (p = 0.043; OR = 10.8, 95% CI = 1.0 – 117.0). In addition, AV+ women had more partners in life (AV- (median/IQR) = 2.9/2; AV+ = 10/5; p = 0.028) and presented more HPV reads in NGS analysis (AV- (median/IQR) = 12/0; AV+ = 2638/152; p = 0.002). Nevertheless, no differences in gene expression were detected between AV- and AV+ women (Supplementary Figure 1). We also correlated Papillomaviridae and Anelloviridae read abundance with gene expression levels and observed a negative correlation between HPV abundance and UCHL1 (r = 0.829, p = 0.042).
3.4 Bacteriome analyses
Total DNA of cervical cells was used as a template to PCR-amplify variable regions V3 and V4 of the bacterial 16S rRNA gene. The proportion of phyla were different taking into account HPV status (p< 0.0001). In HPV- cervices, the predominant phyla were Firmicutes (74.7%), followed by Actinobacteria (12.7%), Bacterioidetes (5.1%) and Fusobacteria (5.1%). On the other hand, in HPV+ samples the same phyla were present, but at different percentages: Firmicutes (68.3%), Actinobacteria (27.3%), Fusobacteria (2.5%) and Bacterioidetes (0.7%) (Figure 1A). The proportion of phyla was also different (p< 0.0001) when comparing AV- (Firmicutes (65.8%), Actinobateria (27.2%) and Fusobacterium (3%)) and AV+ samples (Firmicutes (85%), Fusobacterium (6%) and Bacterioidetes (5.7%)) (Figure 1B).
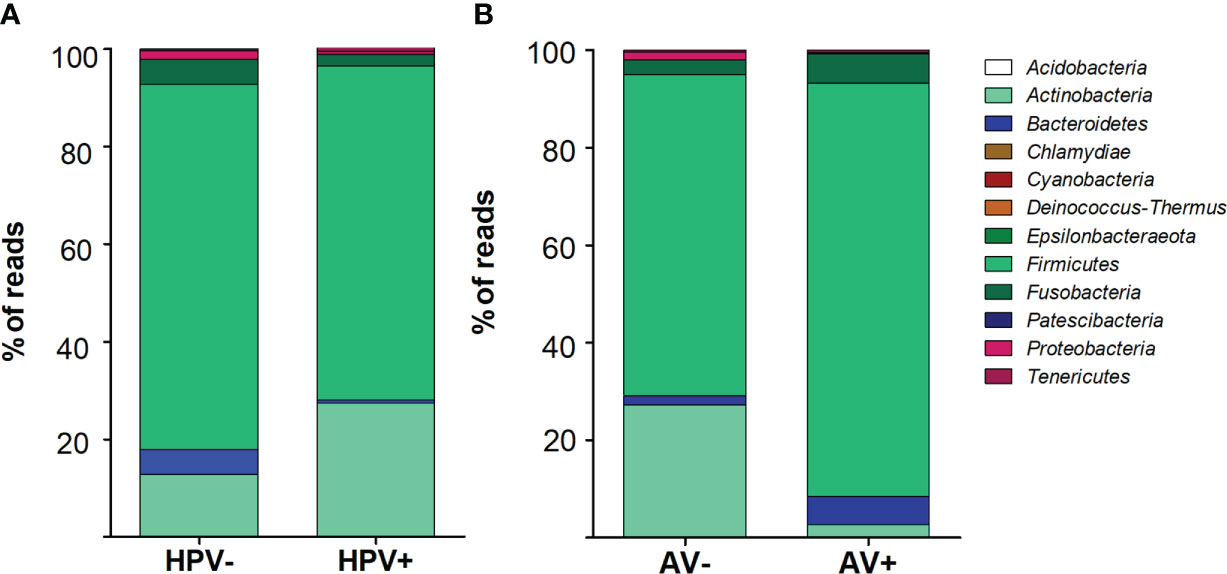
Figure 1 Bacterial phyla distribution depending on HPV (A) or Anellovirus (B) status. Stacked vertical bar graphs showing the percentage of total reads found in (A) HPV- and HPV+ women and (B) Anellovirus negative (AV-) and Anellovirus+ (AV+) women. The frequencies of phyla were assessed by chi-square test and were different between groups (p < 0.001).
We documented the presence of four distinct CSTs based on bacteriome hierarchical cluster analysis: CST I (L. crispatus dominant, n = 3; 14.2%), CST III (L. iners dominant, n = 11; 52.4%), CST IV (anaerobic dominant: Garnerella, n = 5; 23.8% and Sneathia, n = 1; 4.8%) and CST V (L. jensenii dominant, n = 1; 4.8%) (Figure 2). Since our sample size is small, we clustered the women in three groups: L. no-iners (grouping CST I and V), L. iners (CST III) and anaerobic (CST IV). Women within the L. iners group reported the use of condoms more frequently and had their first sexual intercourse earlier than women in the anaerobic group (p = 0.049). On the other hand, women within the anaerobic group smoke/smoked more than L. iners group (p = 0.026). In this sense, smoking increased the chance of anaerobic cervical dominance compared with the L. iners group (OR = 22.5, 95% CI = 1.6 – 314.6, p = 0.0207) (Supplementary Table 1). Moreover, anaerobic-colonized cervices expressed higher levels of TLR3 compared with the L. iners group (OR = 13.3, 95% CI = 1.1 – 166.4; p = 0.044) (Supplementary Table 1). We did not find association between CSTs and HPV or AV infection (Figures 3A, B). However, when we assessed gene expression in CSTs independent of HPV or AV status, cervical cells in a L. no-iners dominated mucosa expressed higher levels of IFNαR2 and TLR3 compared to the L. iners dominated group (Figures 3C, D).
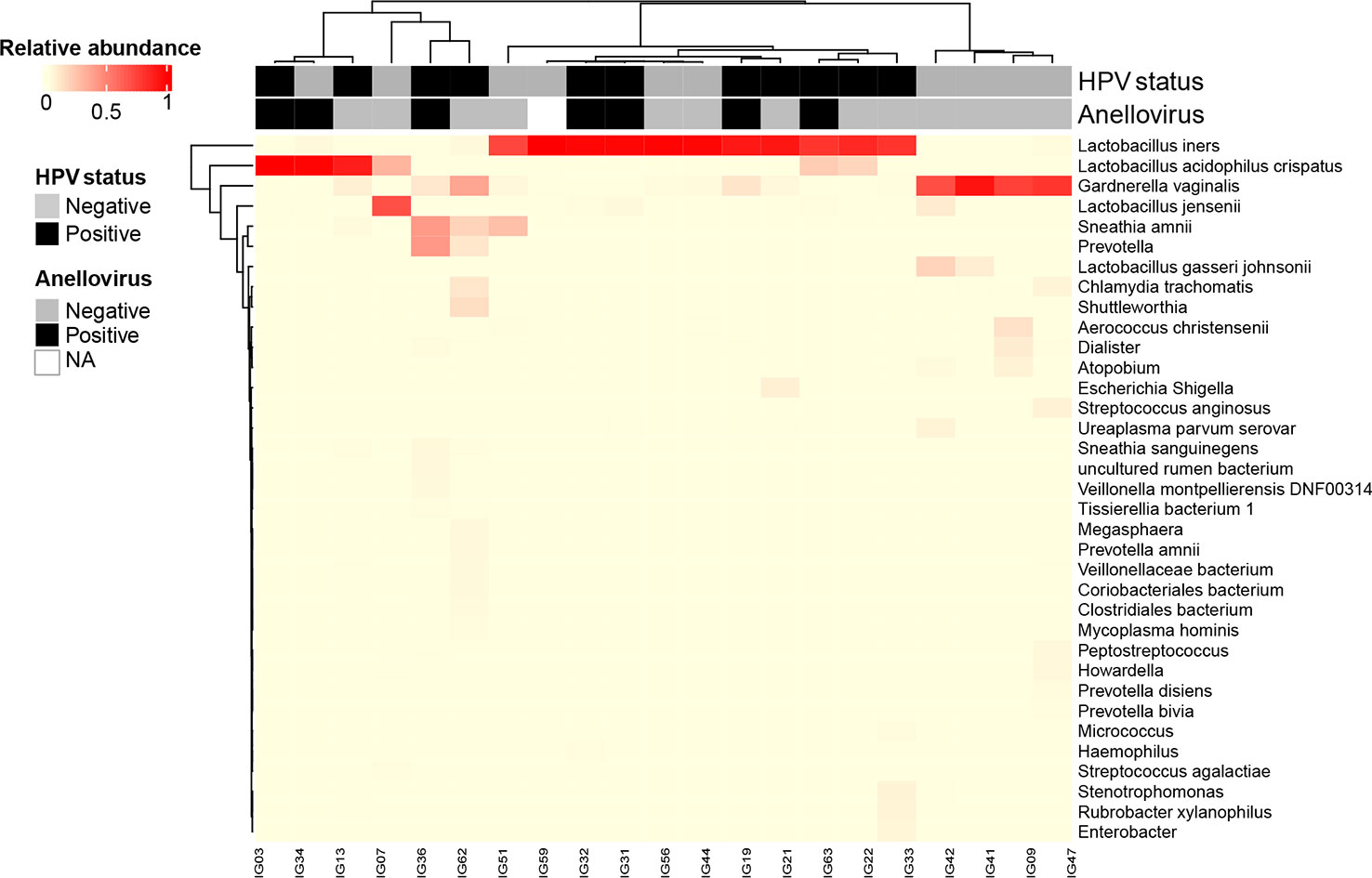
Figure 2 Heatmap generated by unsupervised hierarchical clustering analysis of cervical bacteriomes including HPV and Anellovirus status. CSTs were defined using clustering based on Bray-Curtis dissimilarity and average linkage and then grouped based on vaginal health state in: healthy state – L. no-iners dominated (grouping CSTs I and V); transitional state – L. iners dominated (CST III); and dysbiosis state – No-Lacto dominated (CST IV). Relative bacterial abundance, HPV and Anellovirus statuses are color-coded according to the legend at the left of the Figure. All taxa shown in the graph presented relative abundance >1%. NA: not available. .
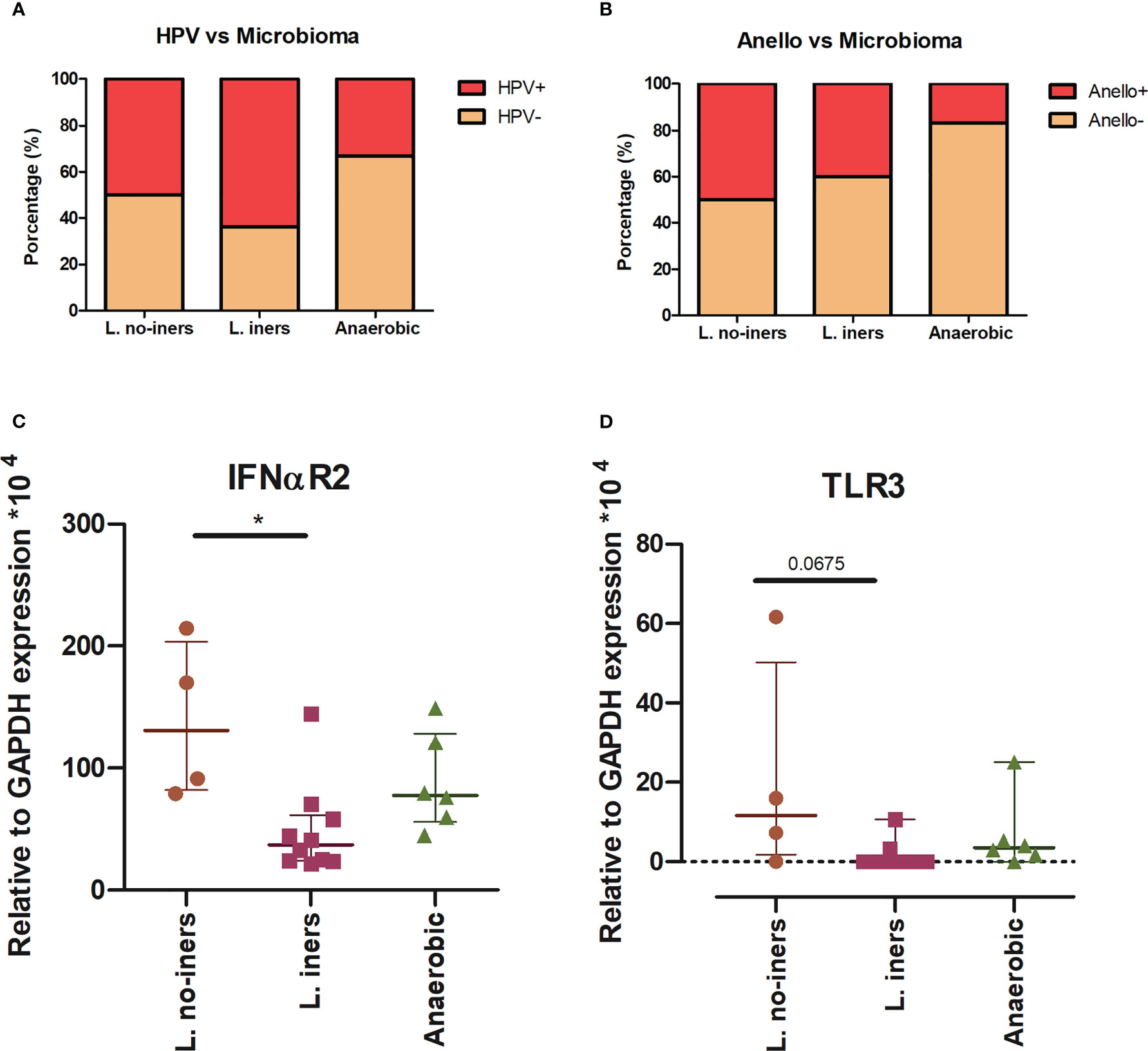
Figure 3 CST distribution related to virus status (A, B) and gene expression (C, D). Stacked bar graph of grouped CSTs showing the percentage of HPV (A) and Annelovirus (B) infected and non-infected patients. Statistical Test: qui-square. Scatter plot of IFNαR2 (C) and TLR3 (D) expression in L. no-iners, L. iners and No-Lacto groups. Central horizontal bars represent the median and upper and lower horizontal bars the interquartile range. We show only genes for which we rejected null hypothesis (Kruskal-Wallis test, (C) p = 0.0019 and (D) p = 0.0291). We performed Dunn’s multiple comparisons as a post-hoc test (* - p < 0.05).
We carried out correlation analysis comparing viral and bacterial abundance and observed strong negative correlation between HPV abundance and the Sphingomonas genus (r = -0.733, p = 0.039) as well as between AV abundance and Fusobacterium genus (r = -0.794, p = 0.033), while AV abundance showed a strong positive correlation with the presence of Shuttleworthia genus (r = 0.771, p = 0.042). We also performed correlations between the 61 bacterial genera found in the samples and the expression of assessed genes. We found 37 moderate correlations involving 22 genera and 9 innate immunity genes (Table 4). RLRs (RIG-I and MDA5) and their regulators (TRIM25 and RNF125) were involved in 30 of these correlations, where RIG-I and TRIM25 correlated to 11 genera each, 9 of these were common to both genes and 2 were common to RIG-I, TRIM25 and MDA5 (Table 4). Finally, we investigated further correlations among the 22 bacterial genera identified above and detected 54 correlations (Table 5). Interestingly, genera that correlated with TLRs, UCHL1 and type I IFN receptor (IFNαR2) had no more than three correlations with other genera. On the other hand, genera that correlated to IFNγ, RLRs and their regulators, correlated with three or more genera and mainly were correlated with each other (Table 5). Collectively, these data suggest an association between the mRNA expression of RIG-I, MDA5 and their regulators (TRIM25 and RNF125) with anaerobic bacterial abundance and diversity.
4 Discussion
Cervical cancer development is associated with HPV infection, which progression depends on the cervical milieu. Herein, we tried to elucidate part of the interplay between host innate immunity and the microbiome (virome and bacteriome) using a Brazilian convenience sample. First, we assessed whether social and immune factors could impact HPV infection and found that IFN-I expression correlated to that of different innate immunity genes depending on HPV status. Virome data from cervical samples revealed two viral families: Papillomaviridae and Anelloviridae and allowed the assembly of seven complete HPV genome sequences. The most prevalent HPV was HPV16 (6/11 women). Some samples were also infected by multiple HPV types. Bacteriome analysis identified 12 phyla that were differently distributed depending on HPV or AV presence. Overall, behavioral factors and innate immune gene expression were associated to L. iners and anaerobic dominated mucosa. Expression of RLRs and their regulators correlated to several genera abundance which in turn, correlated to each other, suggesting that this receptor family triggers innate immune signaling that may impact vaginosis.
Known risk factors for cervical cancer development (41, 42) were confirmed in our samples where the occurrence of previous STI increased eight times the risk for HPV infection. Higher expression of IFNα and IFNβ and lower levels of MDA5 and TLR3 were linked to HPV infection. HPV infection changes expression of several genes linked to innate immune response (43–46) and our correlation analysis suggest that in the presence of HPV, the relationship between these genes is altered. Changes in TLR’s and RLR’s mRNA and protein levels have been assessed in other HPV-associated cancer models. In HPV-associated oropharyngeal squamous cell carcinoma (OPSCC), TLR4 protein expression was lower while higher levels of TLR9 mRNA were observed (47). In our study, higher expression of TLR9 mRNA was found in the HPV+ cervical samples, but no changes in TLR4 expression were seen (12). Overall, in the absence of HPV a negative correlation between TRL4 and TLR9 expression was seen, while a positive one occurred in HPV+ samples. Taken together, the correlation analyses between HPV+ and HPV- women reinforces the idea that regulation of IFNs and PRRs expression during HPV infection could play a pivotal role in inflammatory process, creating a favorable environment for HPV persistence, cancer development and HIV susceptibility, which has been described in the literature by us and others (12, 48–51). The abundance of HPV was negatively correlated to UCHL1’s expression contradicting Karim et al. (2013) (11), who used a primary keratinocyte HPV16 infection model and concluded that HPV dampens host innate immunity via UCHL1 induction.
In this study, the number of sexual partners and number of HPV reads were correlated to AV reads, reinforcing idea that these viruses are sexually transmitted (52–54). There was a higher prevalence of AVs in HPV+ women and literature associate these coinfections not only in cervix, but also at other sites (53–55). It has been suggested that coinfection contributes to laryngeal carcinoma progression (55, 56) and magnifies immune responses (57–59), creating a microenvironment that favours their establishment and facilitates tumor development.
Upon bacteriome determination, the studied cervical samples were classified into three groups: L. no-iners (healthy); L. iners (transitional) and anaerobic (dysbiosis). In our samples, smoking was the only behavioral factor linked to CST IV (anaerobic), but a larger sample size is needed to perform a more robust analysis. The association between cervical microbial diversity (CST III and IV) and HPV infection/cervical dysplasia has been described (3, 25, 26); however, we did not find this association. Independently of HPV, the cervices assessed here were dominated by the same bacterial phyla, although at different proportions in HPV-infected and uninfected women. Similarly, a Chinese study that analyzed 276 cervical smear samples found that age and HPV infection were associated with microbiota structure and diversity (60). When we compared AV+ and AV- samples, bacterial proportion and phyla were different between the two groups. The great dominance of Firmicutes and small proportion of other phyla indicates the dominance of Lactobacillus spp. and low diversity in AV+ group, opposite to what was observed in the literature, where AV abundance was associated to non-Lactobacillus (59). Our data also indicates that a L. no-iners dominated mucosa expressed higher TLR3 and IFNαR2 than L. iners mucosa. It is known that different mucosa are colonized by microorganisms that are in close contact with epithelial cells. In human colon for instance, TLR3 is concentrated in mature columnar cells that create a luminal barrier protection surface (61). LPS and Gram-positive bacteria promote upregulation of TLR3 (61, 62) and IFNβ expressions when intestinal cell lines are exposed simultaneously to B. bifidum and poly(I:C) (61). Interestingly, TLR3 seems to be able to discriminate between commensal and pathogenic bacteria in mice gastrointestinal mucosa, where it is associated with an anti-inflammatory response after recognition of commensal bacterial DNA (61, 63). Little is known about the role of TLR3 in cervix, however it is possible that TLR3 acts in the maintenance of an anti-inflammatory environment in L. no-iners dominated mucosa. Concerning the expression of IFNαR2 and its association with L. no-iners mucosa, no information is available in the literature. It is possible that this receptor acts synergistically with TLR3 in maintaining a healthy mucosa, but this needs to be further evaluated.
Still in the bacteriome context, we observed that abundance of certain bacteria genera found in CST IV correlated with others in the same CST and some genera positively correlated to innate immunity genes, mainly genes involved in RLR-mediated pathway. We were surprised with the observations that RLRs and not TLRs expressions were altered in our samples (64, 65). RLRs are cytoplasmic PAMP receptors initially characterized as viral RNA sensors (e.g., short and long dsRNA, 5′-triphosphorylated RNA, ssRNA and poly(I:C)) and their role in DNA and bacterial recognition is emerging (64, 65). For instance, Salmonella typhimurium and Listeria monocytogenes nucleic acids can activate a RIG-I-mediated response in non-phagocytic and phagocytic cells (66–69). Human microglial cells also have RIG-I upregulated at protein levels when infected with either N. meningitidis, S. aureus, or S. pneumoniae. The same effect was obtained when DNA from these bacteria was used as a PAMP, and the end result was induction of type I IFN (70). More recently, the role of RIG-I in LPS-induced innate immune activation has been shown in endothelial cells. Surprisingly, this activation was independent of TLR4 LPS detection and was also independent of RIG-I RNA detection (71). The same group showed that different endothelial cells can respond to LPS in either a TLR4- or a RIG-I-dependent manner which resulted in different adhesion molecules expression (72). In mice, RIG-I depletion caused an alteration in the gut bacteriome and decreased gut IgA secretion. The latter is crucial for maintaining immunological homeostasis between intestinal bacteria and mucosa (18). We find intriguing that expression of either RIG-I and MDA5 or its regulators TRIM25 and RNF125 were correlated to some bacterial genera abundance, linking the importance of these PRRs in mucosal homeostasis and wonder if these proteins exert their function in a similar way in the gut and in the cervix. In this context, it will be interesting to analyse the mucosa in RIG-I-deficient female mice.
Taken together, despite the lack of adjustments for multiple comparisons and lack of control for covariates due to limited sample size, our data suggest differences in bacterial composition depending on HPV or AV status. Moreover, correlations between bacterial genera and immune gene expression were found, linking RLRs function to dysbiosis. Longitudinal studies and a larger sample size would further confirm the results presented here and would allow to determine the causal relationship between bacteria abundance and innate immunity.
Data availability statement
The datasets presented in this study can be found in online repositories. The names of the repository/repositories and accession number(s) can be found below: PRJNA933917 (SRA).
Ethics statement
The studies involving human participants were reviewed and approved by Ethical Committee of Instituto de Puericultura e Pediatria Martagão Gesteira, UFRJ (approval number: 49035215.4.0000.5264). The patients/participants provided their written informed consent to participate in this study.
Author contributions
AB, AG, EM and MS: conceptualization and writing original draft. AB, CP, AM, YF, and GA: sample collection and processing. AB, CP, AG, and EM: gene expression experiment and analysis. AB, LG, JS, GC: virome and bacteriome experiment and analysis. MS, AG, and EM: funding acquisition. AB, AG, EM, LG, JS, GC and MS: writing, reviewing, and editing. All authors contributed to the article and approved the submitted version.
Funding
This study was supported by grants from the Brazilian National Science Council (CNPq; # 309850/2020-7), from the Swiss Bridge Foundation (# L0V 3024060) and from intramural resources of the Brazilian National Cancer Institute to MAS.
Conflict of interest
The authors declare that the research was conducted in the absence of any commercial or financial relationships that could be construed as a potential conflict of interest.
Publisher’s note
All claims expressed in this article are solely those of the authors and do not necessarily represent those of their affiliated organizations, or those of the publisher, the editors and the reviewers. Any product that may be evaluated in this article, or claim that may be made by its manufacturer, is not guaranteed or endorsed by the publisher.
Supplementary material
The Supplementary Material for this article can be found online at: https://www.frontiersin.org/articles/10.3389/fimmu.2023.1147950/full#supplementary-material
References
1. INCA. Estimativa/2020 - incidência de câncer no Brasil. 1st ed. Rio de Janeiro: Ministério da Saúde (2019) p. 1–120 p.
2. Bruni L, Albero G, Serrano B, Mena M, Collado J, Gómez D, et al. Human papillomavirus and related diseases report world. Barcelona: ICO/IARC Inf Centre HPV Cancer (HPV Inf Centre) (2021), 1–303. Available at: www.hpvcentre.net.
3. Mitra A, MacIntyre DA, Marchesi JR, Lee YS, Bennett PR, Kyrgiou M. The vaginal microbiota, human papillomavirus infection and cervical intraepithelial neoplasia: what do we know and where are we going next? Microbiome (2016) 4(1):58. doi: 10.1186/S40168-016-0203-0
4. Walboomers JM, Jacobs M v, Manos MM, Bosch FX, Kummer JA, Shah K v, et al. Human papillomavirus is a necessary cause of invasive cervical cancer worldwide. J Pathol (1999) 189(1):12–9. doi: 10.1002/(SICI)1096-9896(199909)189:1<12::AID-PATH431>3.0.CO;2-F
5. Lin D, Kouzy R, Jaoude JA, Noticewala SS, Delgado Medrano AY, Klopp AH, et al. Microbiome factors in HPV-driven carcinogenesis and cancers. PloS Pathog (2020) 16(6):e1008524. doi: 10.1371/JOURNAL.PPAT.1008524
6. Nguyen P v, Kafka JK, Ferreira VH, Roth K, Kaushic C. Innate and adaptive immune responses in male and female reproductive tracts in homeostasis and following HIV infection. Cell Mol Immunol (2014) 11(5):410–27. doi: 10.1038/cmi.2014.41
7. Wicherska-pawłowska K, Wróbel T, Rybka J. Toll-like receptors (TLRs), NOD-like receptors (NLRs), and RIG-I-Like receptors (RLRs) in innate immunity. TLRs, NLRs, and RLRs ligands as immunotherapeutic agents for hematopoietic diseases. Int J Mol Sci (2021) 22(24):13397. doi: 10.3390/IJMS222413397
8. Gack MU, Shin YC, Joo Ch, Urano T, Liang C, Sun L, et al. TRIM25 RING-finger E3 ubiquitin ligase is essential for RIG-i-mediated antiviral activity. Nature (2007) 446(7138):916–20. doi: 10.1038/nature05732
9. Arimoto Ki, Takahashi H, Hishiki T, Konishi H, Fujita T, Shimotohno K. Negative regulation of the RIG-I signaling by the ubiquitin ligase RNF125. PNAS (2007) 104(18):7500–5. doi: 10.1073/pnas.0611551104
10. Liao Z, Su J. Progresses on three pattern recognition receptor families (TLRs, RLRs and NLRs) in teleost. Dev Comp Immunol (2021) :122:104131. doi: 10.1016/J.DCI.2021.104131
11. Karim R, Tummers B, Meyers C, Biryukov JL, Alam S, Backendorf C, et al. Human papillomavirus (HPV) upregulates the cellular deubiquitinase UCHL1 to suppress the keratinocyte’s innate immune response. PloS Pathog (2013) 9(5):e1003384. doi: 10.1371/journal.ppat.1003384
12. Britto AMA, Goes LR, Sivro A, Policarpo C, Meirelles ÂR, Furtado Y, et al. HPV induces changes in innate immune and adhesion molecule markers in cervical mucosa with potential impact on HIV infection. Front Immunol (2020) 11:2078/full. doi: 10.3389/fimmu.2020.02078/full
13. Silva J, Cerqueira F, Medeiros R. Chlamydia trachomatis infection: implications for HPV status and cervical cancer. Arch Gynecol Obstet (2014) 289(4):715–23. doi: 10.1007/s00404-013-3122-3
14. Uysal IB, Boué V, Murall CL, Graf C, Selinger C, Hirtz C, et al. Concomitant and productive genital infections by HSV-2 and HPV in two young women: a case report. IDCases (2022) 30:e01604. doi: 10.1016/j.idcr.2022.e01604
15. France M, Alizadeh M, Brown S, Ma B, Ravel J. Towards a deeper understanding of the vaginal microbiota. Nat Microbiol (2022) 7(3):367. doi: 10.1038/s41564-022-01083-2
16. Rezasoltani S, Ghanbari R, Looha MA, Mojarad EN, Yadegar A, Stewart D, et al. Expression of main toll-like receptors in patients with different types of colorectal polyps and their relationship with gut microbiota. Int J Mol Sci (2020) 21(23):8968. doi: 10.3390/ijms21238968
17. Fischer JC, Bscheider M, Eisenkolb G, Lin CC, Wintges A, Otten V, et al. RIG-I/MAVS and STING signaling promote gut integrity during irradiation- and immune-mediated tissue injury. Sci Transl Med (2017) 9(386):eaag2513. doi: 10.1126/scitranslmed.aag2513
18. Zhu H, Xu WY, Hu Z, Zhang H, Shen Y, Lu S, et al. RNA Virus receptor rig-I monitors gut microbiota and inhibits colitis-associated colorectal cancer. J Exp Clin Cancer Res (2017) 36(1):1–11. doi: 10.1186/s13046-016-0471-3
19. Ravel J, Gajer P, Abdo Z, Schneider GM, Koenig SSK, McCulle SL, et al. Vaginal microbiome of reproductive-age women. Proc Natl Acad Sci U.S.A. (2011) 108(SUPPL. 1):4680–7. doi: 10.1073/pnas.1002611107
20. Petrova MI, Lievens E, Malik S, Imholz N, Lebeer S. Lactobacillus species as biomarkers and agents that can promote various aspects of vaginal health. Front Physiol Front Media S.A (2015) 6:81. doi: 10.3389/fphys.2015.00081
21. Hellberg D, Nilsson S, Mårdh PA. Bacterial vaginosis and smoking. Int J STD AIDS. (2000) 11(9):603–6. doi: 10.1258/0956462001916461
22. Sivro A, Mwatelah R, Kambaran C, Gebrebrhan H, Becker MG, Ma H, et al. Sex work is associated with increased vaginal microbiome diversity in young women from Mombasa, Kenya. J Acquir Immune Defic Syndr (2020) 85(1):79–87. doi: 10.1097/QAI.0000000000002406
23. Fethers KA, Fairley CK, Hocking JS, Gurrin LC, Bradshaw CS. Sexual risk factors and bacterial vaginosis: a systematic review and meta-analysis. Clin Infect Dis (2008) 47(11):1426–35. doi: 10.1086/592974
24. Borgdorff H, van der Veer C, Van Houdt R, Alberts CJ, De Vries HJ, Bruisten SM, et al. The association between ethnicity and vaginal microbiota composition in Amsterdam, the Netherlands. PloS One (2017) 12(7):e0181135. doi: 10.1371/journal.pone.0181135
25. Curty G, de Carvalho PS, Soares MA. The role of the cervicovaginal microbiome on the genesis and as a biomarker of premalignant cervical intraepithelial neoplasia and invasive cervical cancer. Int J Mol Sci (2019) 21(1):222. doi: 10.3390/ijms21010222
26. Mitra A, MacIntyre DA, Lee YS, Smith A, Marchesi JR, Lehne B, et al. Cervical intraepithelial neoplasia disease progression is associated with increased vaginal microbiome diversity. Sci Rep (2015) 5(1):16865. doi: 10.1038/srep16865
27. Petrova MI, van den Broek M, Balzarini J, Vanderleyden J, Lebeer S. Vaginal microbiota and its role in HIV transmission and infection. FEMS Microbiol Rev (2013) 37(5):762–92. Available at: https://academic.oup.com/femsre/article/37/5/762/542986.
28. Marconi C, El-Zein M, Ravel J, Ma B, Lima MD, Carvalho NS, et al. Characterization of the vaginal microbiome in women of reproductive age from 5 regions in Brazil. Sex Transm Dis (2020) 47(8):562–9. doi: 10.1097/OLQ.0000000000001204
29. Jakobsen RR, Haahr T, Humaidan P, Jensen JS, Kot WP, Castro-Mejia JL, et al. Characterization of the vaginal DNA virome in health and dysbiosis. Viruses (2020) 12:1143. doi: 10.3390/v12101143
30. Happel AU, Varsani A, Balle C, Passmore JA, Jaspan H. The vaginal virome-balancing female genital tract bacteriome, mucosal immunity, and sexual and reproductive health outcomes? Viruses (2020) 12(8):832. doi: 10.3390/v12080832
31. Siqueira J, Curty G, Xutao D, Hofer C, Machado E, Seuánez H, et al. Composite analysis of the virome and bacteriome of HIV/HPV Co-infected women reveals proxies for immunodeficiency. Viruses (2019) 11(5):422. doi: 10.3390/v11050422
32. Britto AMA, Policarpo C, Pezzuto P, Meirelles ARI, Furtado YL, Almeida G, et al. Detection of sexually transmitted infections at a Brazilian gynecology center: high prevalence of co-infections. J Bras Patol Med Lab (2018) 54(6):393–400. doi: 10.5935/1676-2444.20180060
33. Joshi N, Fass J. Sickle: a sliding-window, adaptive, quality-based trimming tool for FastQ files (Version 1.33) [Software] (2011). Available at: https://github.com/najoshi/sickle.
34. Li H, Durbin R. Fast and accurate short read alignment with burrows–wheeler transform. Bioinformatics (2009) 25(14):1754. doi: 10.1093/bioinformatics/btp324
35. Camacho C, Coulouris G, Avagyan V, Ma N, Papadopoulos J, Bealer K, et al. BLAST+: architecture and applications. BMC Bioinf (2009) 10:421. doi: 10.1186/1471-2105-10-421
36. Callahan BJ, McMurdie PJ, Rosen MJ, Han AW, Johnson AJA, Holmes SP. DADA2: high resolution sample inference from illumina amplicon data. Nat Methods (2016) 13(7):581. doi: 10.1038/nmeth.3869
37. Bolyen E, Rideout JR, Dillon MR, Bokulich NA, Abnet CC, Al-Ghalith GA, et al. Reproducible, interactive, scalable and extensible microbiome data science using QIIME 2. Nat Biotechnol (2019) 37(8):852. doi: 10.1038/s41587-019-0209-9
38. McDonald D, Price MN, Goodrich J, Nawrocki EP, Desantis TZ, Probst A, et al. An improved greengenes taxonomy with explicit ranks for ecological and evolutionary analyses of bacteria and archaea. ISME J (2012) 6(3):610–8:3. doi: 10.1038/ismej.2011.139
39. Schober P, Boer C, Schwarte LA. Correlation coefficients: appropriate use and interpretation. Anesth Analg (2018) 126(5):1763–8. doi: 10.1213/ANE.0000000000002864
40. Castro F, Cardoso AP, Gonçalves RM, Serre K, Oliveira MJ. Interferon-gamma at the crossroads of tumor immune surveillance or evasion. Front Immunol (2018) 1. doi: 10.3389/fimmu.2018.00847
41. Zhang S, Xu H, Zhang L, Qiao Y. Cervical cancer: epidemiology, risk factors and screening. Chin J Cancer Res (2020) 32(6):720. doi: 10.21147/j.issn.1000-9604.2020.06.05
42. Mekuria M, Edosa K, Endashaw M, Bala ET, Chaka EE, Deriba BS, et al. Prevalence of cervical cancer and associated factors among women attended cervical cancer screening center at gahandi memorial hospital, Ethiopia. Cancer Inform (2021) 20:1–6. doi: 10.1177/11769351211068431
43. de Matos LGG, Cândido EB, Vidigal PVT, Bordoni PHC, Lamaita RM, Carneiro MM, et al. Association between toll-like receptor and tumor necrosis factor immunological pathways in uterine cervical neoplasms. Tumori J (2017) 103(1):81–6. doi: 10.5301/tj.5000576
44. Hasimu A, Ge L, Li QZ, Zhang RP, Guo X. Expressions of toll-like receptors 3, 4, 7, and 9 in cervical lesions and their correlation with HPV16 infection in uighur women. Chin J Cancer (2011) 30(5):344–50. doi: 10.5732/cjc.010.10456
45. Chiang C, Pauli EK, Biryukov J, Feister KF, Meng M, White EA, et al. The human papillomavirus E6 oncoprotein targets USP15 and TRIM25 to suppress RIG-I-Mediated innate immune signaling. J Virol (2018) 92(6):e01737–17. doi: 10.1128/JVI.01737-17
46. DeCarlo CA, Rosa B, Jackson R, Niccoli S, Escott NG, Zehbe I. Toll-like receptor transcriptome in the HPV-positive cervical cancer microenvironment. Clin Dev Immunol (2012) 2012:785825. doi: 10.1155/2012/785825
47. Tobouti PL, Bolt R, Radhakrishnan R, Sousa SCOM, Hunter KD, Tobouti PL, et al. Altered toll-like receptor expression and function in HPV-associated oropharyngeal carcinoma. Oncotarget (2018) 9(1):236–48. doi: 10.18632/oncotarget.18959
48. Cannella F, Pierangeli A, Scagnolari C, Cacciotti G, Tranquilli G, Stentella P, et al. TLR9 is expressed in human papillomavirus-positive cervical cells and is overexpressed in persistent infections. Immunobiology (2014) S0171-2985(14):00203–4. doi: 10.1016/j.imbio.2014.10.012
49. Ferreira AR, Ramalho AC, Marques M, Ribeiro D. The interplay between antiviral signalling and carcinogenesis in human papillomavirus infections. Cancers (Basel) (2020) 12(3):646. doi: 10.3390/cancers12030646
50. Li J, Rao H, Jin C, Liu J. Involvement of the toll-like Receptor/Nitric oxide signaling pathway in the pathogenesis of cervical cancer caused by high-risk human papillomavirus infection. BioMed Res Int (2017) 2017:1–8. doi: 10.1155/2017/7830262
51. Liebenberg LJP, McKinnon LR, Yende-Zuma N, Garrett N, Baxter C, Kharsany ABM, et al. HPV infection and the genital cytokine milieu in women at high risk of HIV acquisition. Nat Commun (2019) 10(1):5227. doi: 10.1038/s41467-019-13089-2
52. Fornai C, Maggi F, Vatteroni ML, Pistello M, Bendinelli M. High prevalence of TT virus (TTV) and TTV-like minivirus in cervical swabs. J Clin Microbiol (2001) 39(5):2022. doi: 10.1128/JCM.39.5.2022-2024.2001
53. Changani L, Bouzari M, Talebi A. Torque teno mini virus infection in chronic cervicitis and cervical tumors in isfahan, Iran. Intervirology (2013) 56(4):265–70. doi: 10.1159/000348514
54. Siahpoush M, Noorbazargan H, Kalantari S, Shayestehpour M, Yazdani S. Coinfection of torque teno virus (TTV) and human papillomavirus (HPV) in cervical samples of women living in Tehran, Iran. Iran J Microbiol (2022) 14(2):181. doi: 10.18502/IJM.V14I2.9185
55. Szládek G, Juhász A, Kardos K, Szöke K, Major T, Sziklai I, et al. High co-prevalence of genogroup 1 TT virus and human papillomavirus is associated with poor clinical outcome of laryngeal carcinoma. J Clin Pathol (2005) 58(4):402. doi: 10.1136/JCP.2004.022103
56. Fehér E, Gáll T, Murvai M, Kis A, Boda R, Sápy T, et al. Investigation of the occurrence of torque tenovirus in malignant and potentially malignant disorders associated with human papillomavirus. J Med Virol (2009) 81(11):1975–81. doi: 10.1002/jmv.21627
57. Rocchi J, Ricci V, Albani M, Lanini L, Andreoli E, Macera L, et al. Torquetenovirus DNA drives proinflammatory cytokines production and secretion by immune cells Via toll-like receptor 9. Virology (2009) 394(2):235–42. doi: 10.1016/j.virol.2009.08.036
58. Singh P, Ramamoorthy S. Lack of strong anti-viral immune gene stimulation in torque teno sus Virus1 infected macrophage cells. Virology (2016) 495:63–70. doi: 10.1016/j.virol.2016.04.028
59. Kaelin EA, Skidmore PT, Łaniewski P, Holland LA, Chase DM, Herbst-Kralovetz MM, et al. Cervicovaginal DNA virome alterations are associated with genital inflammation and microbiota composition. mSystems (2022) 7(2):e0006422. doi: 10.1128/msystems.00064-22
60. Hu J, Wu Y, Quan L, Yang W, Lang J, Tian G, et al. Research of cervical microbiota alterations with human papillomavirus infection status and women age in sanmenxia area of China. Front Microbiol (2022) 13. doi: 10.3389/fmicb.2022.1004664
61. Furrie E, Macfarlane S, Thomson G, Macfarlane GT. Toll-like receptors-2, -3 and -4 expression patterns on human colon and their regulation by mucosal-associated bacteria. Immunology (2005) 115(4):565. doi: 10.1111/j.1365-2567.2005.02200.x
62. Nejsum LN, Piec A, Fijak M, Ernstsen C v., Fischer D, Maier TJ, et al. Systemic LPS induces toll-like receptor 3 (TLR3) expression and apoptosis in testicular mouse tissue. Cell Tissue Res (2019) 378(1):143–54. doi: 10.1007/s00441-019-03022-w
63. Kawashima T, Kosaka A, Yan H, Guo Z, Uchiyama R, Fukui R, et al. Double-stranded RNA of intestinal commensal but not pathogenic bacteria triggers production of protective interferon-β. Immunity (2013) 38(6):1187–97. doi: 10.1016/j.immuni.2013.02.024
64. Liwinski T, Zheng D, Elinav E. The microbiome and cytosolic innate immune receptors. Immunol Rev (2020) 297(1):207–24. doi: 10.1111/imr.12901
65. Pandey S, Kawai T, Akira S. Microbial sensing by toll-like receptors and intracellular nucleic acid sensors. Cold Spring Harb Perspect Biol (2015) 7(1):a016246. doi: 10.1101/cshperspect.a016246
66. Schmolke M, Patel JR, de Castro E, Sánchez MTA, Uccellini MB, Miller JC, et al. RIG-I detects mRNA of intracellular salmonella enterica serovar typhimurium during bacterial infection. mBio (2014) 5(2):e01006–14. doi: 10.1128/MBIO.01006-14
67. Abdullah Z, Schlee M, Roth S, Mraheil MA, Barchet W, Böttcher J, et al. RIG-I detects infection with live listeria by sensing secreted bacterial nucleic acids. EMBO J (2012) 31(21):4153–64. doi: 10.1038/emboj.2012.274
68. Hagmann CA, Herzner AM, Abdullah Z, Zillinger T, Jakobs C, Schuberth C, et al. RIG-I detects triphosphorylated RNA of listeria monocytogenes during infection in non-immune cells. PloS One (2013) 8(4):e62872. doi: 10.1371/journal.pone.0062872
69. Imaizumi T, Sashinami H, Mori F, Matsumiya T, Yoshida H, Nakane A, et al. Listeria monocytogenes induces the expression of retinoic acid-inducible gene-I. Microbiol Immunol (2006) 50(10):811–5. doi: 10.1111/j.1348-0421.2006.tb03857.x
70. Johnson MB, Halman JR, Burmeister AR, Currin S, Khisamutdinov EF, Afonin KA, et al. Retinoic acid inducible gene-I mediated detection of bacterial nucleic acids in human microglial cells. J Neuroinflamm (2020) 17(1):1–14. doi: 10.1186/s12974-020-01817-1
71. Moser J, Heeringa P, Jongman RM, Zwiers PJ, Niemarkt AE, Yan R, et al. Intracellular RIG-I signaling regulates TLR4-independent endothelial inflammatory responses to endotoxin. J Immunol (2016) 196(11):4681–91. doi: 10.4049/jimmunol.1501819
Keywords: microbiome and dysbiosis, innate immunity recognition, virome analysis, human papilloma virus (HPV), squamous intraepithelial lesion of the cervix, RIG-I-like receptor family, toll-like receptors (TLR), anellovirus
Citation: Britto AMA, Siqueira JD, Curty G, Goes LR, Policarpo C, Meyrelles AR, Furtado Y, Almeida G, Giannini ALM, Machado ES and Soares MA (2023) Microbiome analysis of Brazilian women cervix reveals specific bacterial abundance correlation to RIG-like receptor gene expression. Front. Immunol. 14:1147950. doi: 10.3389/fimmu.2023.1147950
Received: 19 January 2023; Accepted: 12 April 2023;
Published: 25 April 2023.
Edited by:
Vijayakumar Velu, Emory University, United StatesReviewed by:
Sivasankaran M. Ponnan, Fred Hutchinson Cancer Research Center, United StatesSakthivel Govindaraj, Emory University, United States
Copyright © 2023 Britto, Siqueira, Curty, Goes, Policarpo, Meyrelles, Furtado, Almeida, Giannini, Machado and Soares. This is an open-access article distributed under the terms of the Creative Commons Attribution License (CC BY). The use, distribution or reproduction in other forums is permitted, provided the original author(s) and the copyright owner(s) are credited and that the original publication in this journal is cited, in accordance with accepted academic practice. No use, distribution or reproduction is permitted which does not comply with these terms.
*Correspondence: Alan Messala A. Britto, alanmessala@yahoo.com.br
†Present address: Gislaine Curty, Princess Margaret Cancer Center, University Health Network, Toronto, ON, Canada