- 1Institut Pasteur, Université Paris Cité, CNRS ERL9195 and Inserm U1201, Unité de Biologie des Interactions Hôte Parasites, Paris, France
- 2Sorbonne Université, Inserm U1135, CNRS ERL 8255, Paris, France
- 3Institut Pasteur, Université Paris Cité, Centre d’élevage et de production des anophèles (CEPIA), Paris, France
- 4Institut Pasteur, Université Paris Cité, Imagopole, Paris, France
- 5Institut Pasteur, Université Paris Cité, Malaria Infection and Immunity Unit, Paris, France
Introduction: Plasmodium sporozoites (SPZ) inoculated by Anopheles mosquitoes into the skin of the mammalian host migrate to the liver before infecting hepatocytes. Previous work demonstrated that early production of IL-6 in the liver is detrimental for the parasite growth, contributing to the acquisition of a long-lasting immune protection after immunization with live attenuated parasites.
Methods: Considering that IL-6 as a critical pro-inflammatory signal, we explored a novel approach whereby the parasite itself encodes for the murine IL-6 gene. We generated transgenic P. berghei parasites that express murine IL-6 during liver stage development.
Results and Discussion: Though IL-6 transgenic SPZ developed into exo-erythrocytic forms in hepatocytes in vitro and in vivo, these parasites were not capable of inducing a blood stage infection in mice. Furthermore, immunization of mice with transgenic IL-6-expressing P. berghei SPZ elicited a long-lasting CD8+ T cell-mediated protective immunity against a subsequent infectious SPZ challenge. Collectively, this study demonstrates that parasite-encoded IL-6 attenuates parasite virulence with abortive liver stage of Plasmodium infection, forming the basis of a novel suicide vaccine strategy to elicit protective antimalarial immunity.
Introduction
Major efforts have been made to design vaccines against malaria, an infectious disease that causes serious economic and health problems (1). With increasing emergence worldwide of resistant Plasmodium parasite strains to antimalarial drugs, vaccines remain a critical strategy to control and eradicate malaria. In recent years, in addition to the development of subunit vaccines and radiation-attenuated SPZ (RAS), researchers have used rodent models to test the efficacy of genetically attenuated parasites (GAPs) as vaccines against exo-erythrocytic forms (EEF) and blood-stage malaria infections. Most studies have focused on EEF of GAPs, which include mutant parasites blocked early or late during their development in the liver. As examples of these GAPs, mutations affecting the parasitophorous vacuole formation, or mutations leading to the blockade of fatty acid biosynthesis type II pathway have been reported (2). A new concept has emerged from these studies that replication competent and late stage-arresting GAPs were more efficient in generating sterile protection against malaria when compared with replication-deficient and early liver stage-arresting GAPs (1).
Few molecules of malaria parasites have been shown to counteract host innate immunity (3, 4). In early liver stages, the major SPZ surface protein called circumsporozoite protein (CSP) translocates into the hepatocyte cytosol and nucleus, where it outcompetes NF-kB nuclear import and suppresses hundreds of genes involved in the host inflammatory response (3). It has also been reported that several parasites including Plasmodium, Brugia, Trichuris, Eimeiria, Trichinella, and Onchorcerca, express a migration inhibitory factor (MIF), an orthologue of mammalian MIF with which it has a significant homology. MIF was shown to increase inflammatory cytokine production during the blood phase of Plasmodium infection in rodents and to induce antigen-specific CD4 T-cells to develop into short-lived effector cells rather than into memory cells, causing decreased CD4 T-cell recall responses to homologous parasites (4). However, parasites lacking MIF were shown to have no growth defect throughout the parasite life cycle in P. berghei (Pb) (5) or a growth defect during liver-stage development in P. yoelii (6). We have investigated the protection mediated by an erythrocytic GAP depleted of the gene encoding the immunomodulatory and secreted molecule histamine releasing factor (HRF), using the parasite strain PbNK65 that does not cause experimental cerebral malaria (ECM) and rapid death. In earlier work, we found that blood-stage infection by the mutant self-resolved at day 12 post-infection (p.i.), displaying an immune signature that comprised elevated IL-6 levels, activation of T and B cells, and antigen-specific IgG2c production (7).
During the last decade, in search of key mechanisms that determine the host inflammatory response, a set of host factors turned out to be critical for the control of malaria parasite development (7–10). Strikingly, the activation of the IL-6 signaling pathway occurred as a common theme, that apparently blocks parasite liver stage development, and more importantly allows the initiation of a robust and long-lasting anti-parasite immunity.
In this report we explored a novel approach whereby the parasite itself encodes for the murine IL-6 gene. This strategy is based on the rationale that IL-6 acts as a critical pro-inflammatory signal from the host in response to the parasite infection. We developed a novel suicide strategy to restrict Plasmodium liver stage development through parasite-encoded murine IL6. To this end, we engineered transgenic Pb parasites to express and secrete high levels of the murine IL-6 cytokine restricted to the liver stage and found that these mutants cause an attenuated infection and confer a long-lasting cross-species protective antimalarial immunity.
Materials and methods
Ethics statement
All animal care and experiments described in the present study involving mice were conducted at the Institut Pasteur, approved by the CETEA (comité d’éthique en expérimentation animale) (Permit Number N° dap180040 issued on 2018) and performed in compliance with institutional guidelines and European regulations. A statement of compliance with the French Government’s ethical and animal experiment regulations was issued by the Ministère de l’Enseignement Supérieur et de la Recherche under the number 00218.01.
Design of transgenic Plasmodium berghei parasites that express the mouse IL-6 cytokine
To generate transgenic IL-6 Tg-PbANKA/LISP2 (liver-specific protein 2) P. berghei parasites expressing the murine IL6 transgene, we used a selection-linked integration strategy (11). We assembled a plasmid construct, named GFP-SLI-IL6, containing two cassettes. The first cassette includes a 3’ terminal sequence of the GFP coding sequence, fused to a 2A skip peptide and the human dihydrofolate reductase (hDHFR) gene, followed by the 3’ UTR of P. berghei calmodulin (CAM) gene (Figure 1A; Supplementary Table 1). The second cassette corresponds to a codon-optimized version of murine IL6, under control of the promoter of P. berghei LISP2, and followed by the 3’ UTR of P. berghei DHFR. To ensure IL6 secretion, the signal peptide of mIL6 was replaced by the signal peptide of P. berghei LISP2 (Supplementary Table 1). The final construct was verified by DNA sequencing. The linearized plasmid construct was used to transfect GFP-expressing P. berghei (PbGFP, ANKA strain) parasites (12). PbGFP-infected erythrocytes were collected from an infected donor mice and cultured overnight to allow parasite maturation into erythrocytic merozoites. Merozoites were transfected by nucleofection with 10 μg of construct DNA, as described (13), and immediately injected into two mice. One day after transfection, mice were administered pyrimethamine in the drinking water to select for recombinant parasites. Once the parasitemia reached 2%, after one-week, infected erythrocytes were collected and DNA extracted for genotyping by PCR (Figure 1B) using primer combinations specific for the WT locus (GFP Fw + DHFRseq Rev), the 5’ integration event (GFP Fw + huDHFR Rev), the 3’ integration event (IL6 Fw + DHFRutrRev) or the non-integrated plasmid (IL6 Fw + eEF1aPromTestRev). The primer sequences are indicated in Supplementary Table 2. Transgenic IL-6 Tg-PbANKA/UIS4 parasites were generated in a similar way as described above, but with a different IL6-encoding cassette (Supplementary Table 1), where mIL6 was placed under control of the promoter of Up-regulated in Infective Sporozoites 4 gene (uis4), and contained the signal peptide sequence from P. berghei Perforin-Like Protein 1 (PLP1). Genotyping of IL-6 Tg-PbANKA/UIS4 parasites was performed with the same primers as for IL-6 Tg-PbANKA/LISP2 parasites (Supplementary Table 2). PCR analysis of genomic DNA from PbIL6/LISP2 and PbIL6/UIS4 parasites confirmed correct integration of the constructs and the absence of residual parental parasites or non-integrated plasmid. Thus, after a single round of pyrimethamine selection following transfection, we could obtain pure populations of transgenic PbIL6/LISP2 and PbIL6/UIS4 parasites. For PbIL6/LISP2, two independent transgenic lines (line 1 and line 2) were obtained each from one of the injected mice.
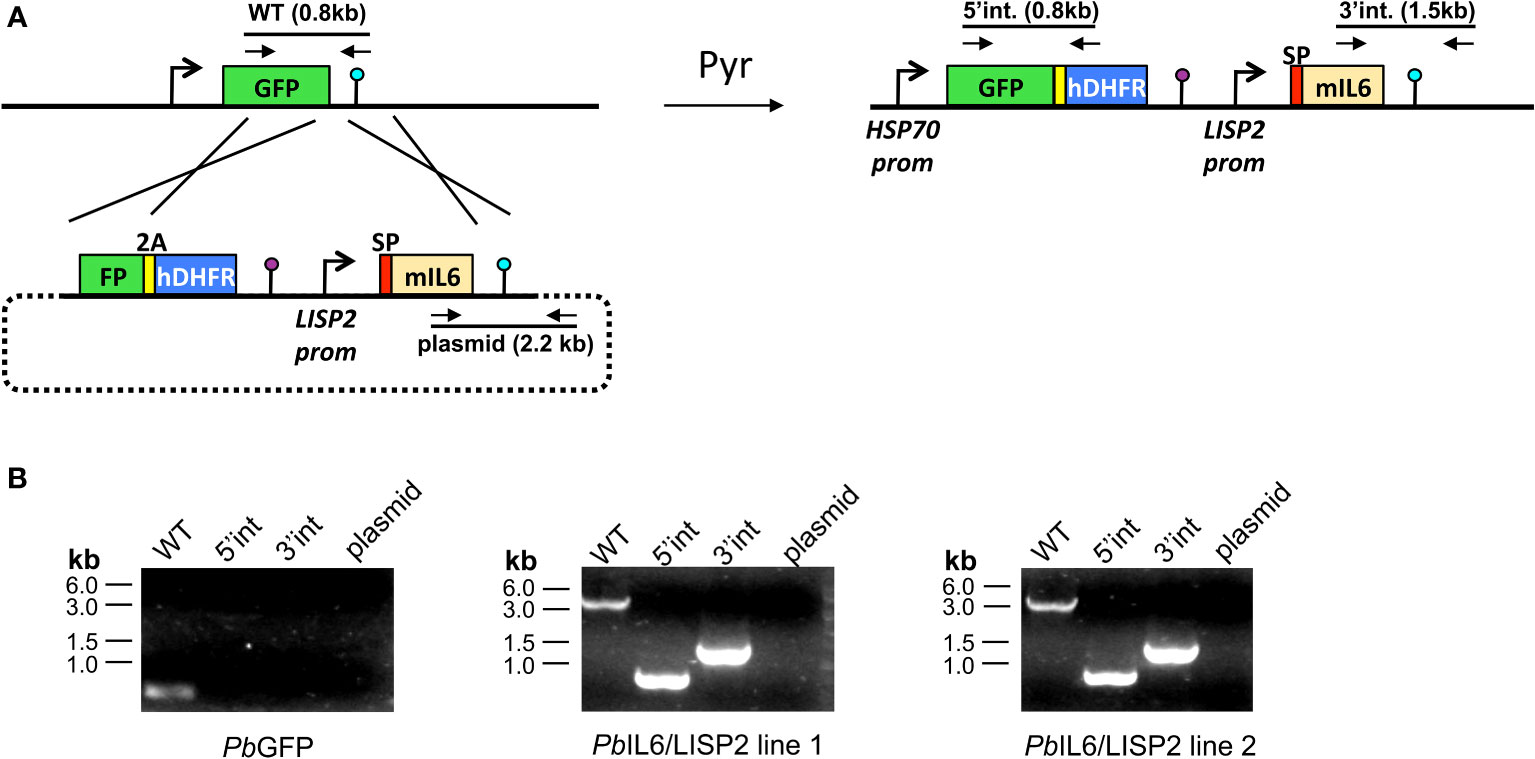
Figure 1 Design of transgenic Plasmodium berghei parasites that express the mouse IL-6 cytokine. (A) Replacement strategy to modify the PbGFP locus to express murine IL6. We used a selection-linked integration strategy to integrate a construct containing a GFP-2A-hDHFR cassette without promoter followed by the mIL6 cassette under control of the PbLISP2 promoter. The signal peptide (SP, red) of PbLISP2 was used to allow IL6 secretion. Parasites undergoing homologous recombination at the GFP and PbDHFR 3’UTR sequences were isolated after one round of pyrimethamine selection. Genotyping primers and expected PCR fragments are indicated by arrows and lines, respectively. (B) PCR analysis of genomic DNA from parental PbGFP, PbIL6/LISP2 line 1 and line 2 parasites. We used primer combinations to detect the non-recombined parental locus (WT, 830-bp), the 5’ integration event (5’int, 819-bp), the 3’ integration event (3’int, 1554-bp) or the non-integrated construct (plasmid, 2197-bp). The WT primer combination is expected to amplify a ~5.1-kb fragment from the recombined locus of PbIL6/LISP2 parasites. The results confirm the correct integration of the constructs at the GFP locus of PbGFP parasites, and show the absence of parental parasites in the transfectants after a single round of pyrimethamine selection.
Parasites and mice infection
Seven‐ to eight‐week‐old female C57BL/6J Rj mice were purchased from Janvier Laboratories (Le Genest-Saint-Isle, France), and were maintained at the animal facility of Institut Pasteur. Mice were infected with either WT PbANKA SPZ, IL-6 Tg-PbANKA/LISP2 or IL-6 Tg-PbANKA/UIS4 SPZ or with P yoelii 17XNL, collected from salivary glands of infected Anopheles stephensi. All parasites were GFP-tagged (12). Infections were performed via i.v. injection in the tail vein of an indicated amount of SPZ according to the experiments. Survival and parasitemia as determined by FACS using Cytoflex cytometer (Beckman Coulter Life Sciences, Villepinte, France) and the software FlowJo (FlowJo LLC, Ashland, OR, USA) were then monitored daily, beginning day 4 p.i. Symptoms associated with the experimental cerebral malaria (ECM) in mouse models include coat ruffling, a respiratory distress syndrome, a drop in body temperature, and neurological signs such as paralysis, and coma, followed by death. For ethical reasons, manifestation of signs such as coat ruffling and reduced motor skills which represent a limit point, constitutes a criterion for interrupting the experience.
Quantification of EEF development in vivo
C57BL/6 mice were inoculated i.v with 150,000 WT PbANKA or IL-6 Tg-PbANKA/LISP2 SPZ and livers were harvested at 24 h and 40 h post-infection. EEF density and dimensions were determined by microscopy on freshly dissected livers using a spinning-disk confocal system (UltraView ERS, Perkin Elmer) controlled by Volocity (Perkin Elmer) and composed of 4 Diode Pumped Solid State Lasers (excitation wavelengths: 405 nm, 488 nm, 561 nm and 640 nm), a Yokogawa Confocal Scanner Unit CSU22, a Z-axis piezoelectric actuator and a Hamamatsu Orca-Flash 4.0 camera mounted on a Axiovert 200 microscope (Zeiss). Z-stacks of images spaced 3 µm apart and covering 18 to 60 µm were acquired using a EC Plan-NEOFLUAR 10x/0.3 objective (Zeiss) or a Plan-APOCHROMAT 63x/1.4 oil objective (Zeiss). Image analysis was performed with the Fiji software (14). Z-stacks were converted to maximum intensity projections and the resulting images were segmented using the pre-implemented Triangle algorithm. EEFs were manually selected with the Magic Wand tool to determine their dimensions (area, Feret’s diameter).
Flow cytometric analysis of liver leukocytes
Liver resident leukocytes were obtained from naïve and infected mice twice with 10, 000 GFP-expressing PbANKA or IL-6 Tg-PbANKA/LISP2 SPZ at 3 weeks interval. Briefly, mice were lethally anesthetized one week after the last infection with a solution of Ketamine/Xylazine and mice livers were perfused with 30 mL of PBS (which contains 137 mM NaCl, 2.7 mM KCl, 8 mM Na2HPO4, and 2 mM KH2PO4) to remove red blood cells and circulating leukocytes. After the perfusion, livers were digested in vitro in a collagenase D solution (0.05%) (Roche Molecular Systems Inc., Branchburg, USA) at 37°C for 45 minutes and mixed through a 70 μm cell strainer (Falcon Thermo Fisher Scientific Inc, Brebière, France). Cells were washed in PBS (which contains 137 mM NaCl, 2.7 mM KCl, 8 mM Na2HPO4, and 2 mM KH2PO4) and purified by Percoll gradient: 5 mL of an 80% isotonic Percoll solution (in PBS 1X which contains 137 mM NaCl, 2.7 mM KCl, 8 mM Na2HPO4, and 2 mM KH2PO4) was applied at the bottom of a 14 mL Falcon tube and cells were resuspended in 5 mL of 40% isotonic Percoll solution (in PBS, which contains 137 mM NaCl, 2.7 mM KCl, 8 mM Na2HPO4, and 2 mM KH2PO4) and gently applied over the top of the first layer. The cell suspension was fractioned by centrifugation at 3000 rpm during 30 minutes without brake at 4°C. After Percoll gradient centrifugation, the leukocytes were collected at the border between the two layers. Cells were washed in cold PBS (which contains 137 mM NaCl, 2.7 mM KCl, 8 mM Na2HPO4, and 2 mM KH2PO4), resuspended in FACS buffer containing 2% Fetal calf serum (FCS) and 0.01% sodium azide and counted. Obtained liver leukocytes were stained for FACS analysis according to standard protocols in a FACS buffer with the following antibodies: AF-647-labelled anti CD45 (clone 30-F11), FITC-labelled CD4 (clone H129.19), PerCP-labelled anti-CD8 (clone (53-6.7), APC-Cy 7 labeled anti-CD3 (clone 145-2C11), PeCy7-labeled anti-CD44 (clone IM7), PE-labeled anti-CD69 (clone H1 2F3), BV605 labelled anti-CD62L (clone MEL-14), and BV785-labeled anti-CD25 (clone PC61). All antibodies were purchased from BD Biosciences, San Jose, CA, USA. Cells were washed and resuspended in FACS buffer before analysis. A total of 5 × 105 living or fixed cells were analyzed using a Cytoflex cytometer (Beckman Coulter Life Sciences, Villepinte, France) and the software FlowJo (FlowJo LLC, Ashland, OR, USA).
Preparation of total RNA and RT-qPCR analysis of mRNA
For kinetic analysis of in vivo parasite load, livers of C57BL/6J mice infected with WT PbANKA or with IL-6 Tg-PbANKA/LISP2 SPZ were surgically removed 4h, 6h, 24h, 48h, and 72h p.i., respectively. Total RNAs were extracted from the liver samples using the guanidinium-thiocyanate-phenol-chloroform method (all from Invitrogen, Waltham, MA, USA). RNA was thereafter reverse transcribed by PCR (temperature profile: 65°C for 5 min, 42°C for 50 min, and 70°C for 15 min) using 100 U of SuperScript II reverse transcriptase (Invitrogen, Waltham, MA, USA), 40 U RNase inhibitor, and 2 µM oligo (dT) 18S, HSP70, or LISP-2 rRNA primers (Eurofins MWG Operon) per sample. For detection of transcribed IL-6 mRNA by EEF, the same procedures were applied. Expression levels of diverse transcripts were analyzed by real-time RT-qPCR using Power SYBR green PCR master mix (Applied Biosystems) and various primer sets (Supplementary Table 3). All reactions were performed in a real-time PCR machine (temperature profile: 50°C for 2 min, 95°C for 10 min, 40 cycles of 15 s at 95°C, and 60°C for 1 min; ABI PRISM 7000 Sequence Detection System; Applied Biosystems). The relative abundance of parasite rRNA, or IL-6 mRNA in the liver and in SPZ was calculated using the ΔCt method and expressed as 2−ΔCt. The mouse hypoxanthine phosphoribosyltransferase (HPRT) gene was used as an internal control for the variation in input RNA amounts. A no template control was included to ensure that there was no cross-contamination during sample preparation.
Detection of the IL-6 cytokine
HepG2 cells were infected with Tg-PbANKA/LISP2 or WT PbANKA SPZ and cultured for 48 h. Supernatants were recovered and the amount of IL-6 were analyzed by cytokine-specific ELISA kits (BD Biosciences, Mountain View, CA).
Treatment of mice with anti-IL-6 receptor blocking antibodies
The rat anti-mouse IL-6R antibody (clone 15A7) (BioXcell, Lebanon, NH, USA) was administered i.v at 500 μg/mouse at four consecutive times: 1 day before SPZ inoculation, and at day 0, day 1 and day 2 after SPZ inoculation. Control mice were injected with the same doses of the control isotype IgG2b (BioXcell, Lebanon, NH, USA).
In vivo cell depletion
To determine if the protection induced by IL-6 Tg-PbANKA/LISP2 SPZ is dependent on effector CD4+ or CD8+ T cells, cell-specific depletion experiments were performed. C57BL/6 protected mice were injected i.p. with 20 μg of anti-CD8 clone 53-6.7 Armenian hamster IgG (eBioscience, San Diego, CA) or 100 μg of rat anti mouse CD4 clone GK1.5 (ATCC TIB207) 48 h before the infection with PbNK65 WT followed by 6 injections administered every other day after the infection. The cell depletion was followed and confirmed every day by taking 10 μl of blood from the tip of the mouse tail and analyzed by flow cytometry. Blood samples were labeled with anti-CD45-AF 647 (clone 30-F11), anti-CD8a-PE (clone 5H10) from Biolegend (San Diego, CA), and anti-CD4-FITC (clone H129-19) from BD Bioscience, Mountain View, CA.
Statistical analysis
All data were analyzed using Prism 5.0 software (GraphPad Software, San Diego, USA). Unpaired data between two groups at a specific time point were analyzed by a Mann-Whitney test for nonparametric analysis. Kaplan-Meier survival plots were analyzed using a Mantel-Cox test. A p-value <0.05 was considered to be statistically significant. All experiments were replicated several times as indicated in the figure legends.
Results
Transgenic P. berghei parasites expressing IL-6 during the liver stage lose infectivity to mice
To generate IL-6 expressing transgenic parasites, we integrated an IL-6 cassette by homologous recombination in the genome of P. berghei ANKA lines, which constitutively express the green fluorescent protein (GFP) (12) (Figure 1A). For this purpose, a codon-optimized IL-6 gene comprising amino acids 25-211 of murine IL-6 was fused to the signal peptide of P. berghei LISP2, to enable secretion of the protein. The resulting transgene was placed under the control of LISP2 promoter to ensure timely restricted expression to the liver stage development (15, 16). To facilitate integration of the construct, we used a selection-linked integration strategy (11) to insert the IL-6 cassette into the GFP locus of PbGFP parasites (Figure 1A). Following transfection, one single round of pyrimethamine selection was sufficient to recover pure populations of transgenic parasites. Two transfectants were selected from independent transfection experiments, called IL-6 Tg-PbANKA/LISP2 line 1 and line 2, and were confirmed by PCR using the primer combinations shown in Supplementary Table 2, to harbor the expected integration of the murine IL-6 gene in a similar way (Figure 1B).
When IL-6 Tg-PbANKA/LISP2 and WT PbANKA parasites were transmitted to female Anopheles mosquitoes, we observed similar prevalence of mosquito infection (Figure 2A) and numbers of salivary gland SPZ (Figure 2B). This indicates that the presence of the IL-6 transgene does not alter parasite development inside mosquitoes.
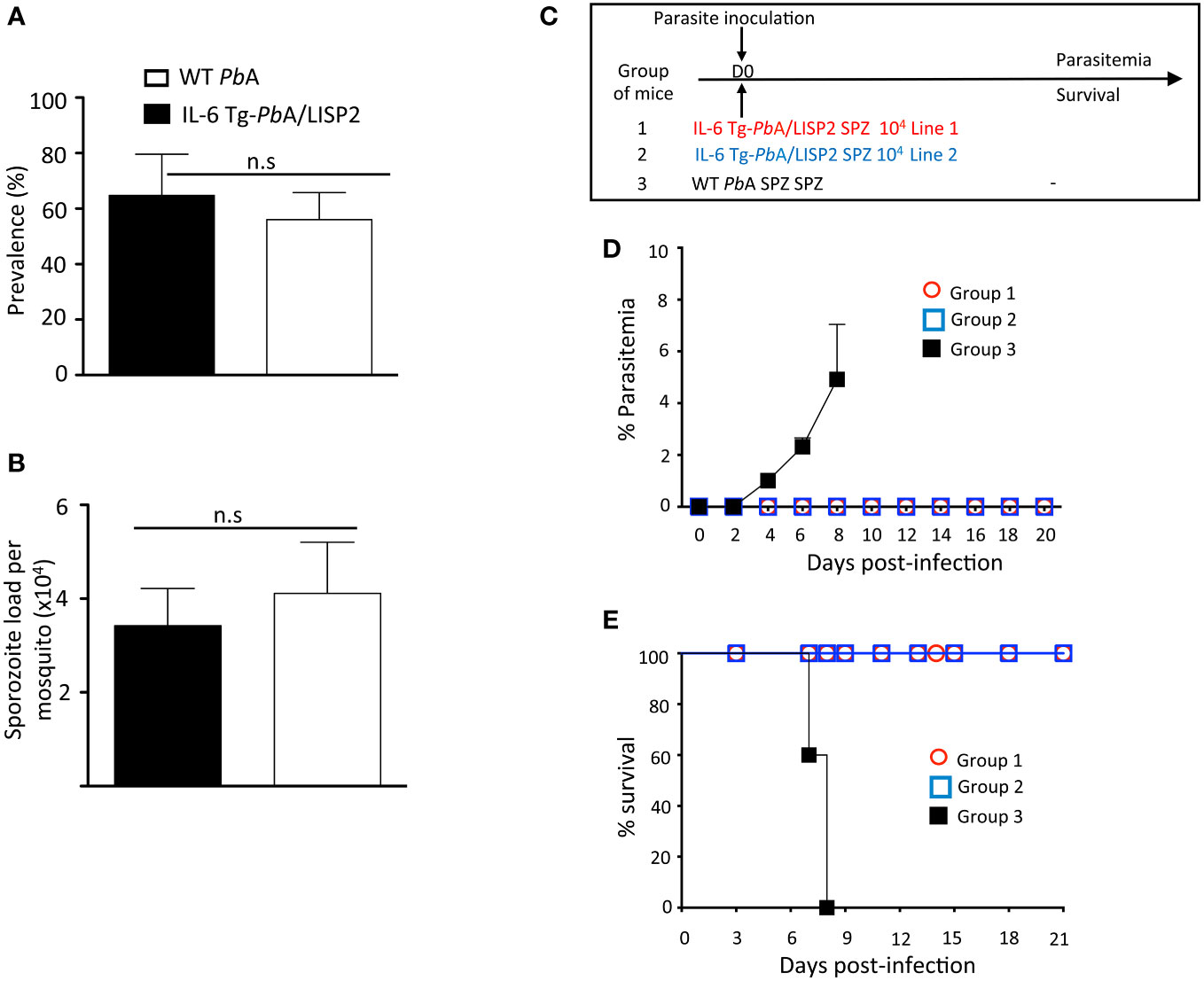
Figure 2 IL-6 Tg-PbANKA/LISP2 did not show any major development defect in the mosquito vector whereas inoculation with IL-6 Tg-PbA/LISP2 SPZ does not produce blood stage infections. (A, B) IL-6 Tg-PbA/LISP2 parasites cycle normally between the vertebrate host and Anopheles mosquitoes. Cages of 200 IL-6 Tg-PbA/LISP2‐ or WT PbANKA-infected Anopheles stephensi female mosquitoes showing GFP‐labelled SPZ in their salivary glands were counted at day 20 post‐blood feeding on infected C57BL/6 mice. Prevalence of infected mosquitoes was expressed as a ratio between positive ones and total mosquitoes (A). SPZ were extracted from salivary glands of 10 mosquitoes and counted. The number of SPZ was expressed as per pair of salivary glands (B). (C) Three groups of C57BL/mice were infected with 104 IL-6 Tg-PbA/LISP2 SPZ line 1 or with IL-6 Tg-PbA/LISP2 SPZ line 2 or with WT PbANKA SPZ. (D) Parasitemia of asexual blood forms were measured at indicated time points by flow cytometry, as all parasites were GFP tagged. (E) Survival rates were determined by Kaplan-Meier survival plots. Error bars, SEM. Data are representative of three independent experiments with 5 mice per group. n.s, stands for not significant.
To examine the capacity of IL-6 transgenic SPZ to develop into hepatic EEF in vivo and to differentiate into blood stages, C57BL/6 mice were injected i.v. with 104 SPZ of IL-6 Tg-PbANKA/LISP2 line 1, or line 2, or with parental WT (PbGFP) SPZ, as shown in Figure 2C, and parasitemia was followed over time. Parasitemia was determined by flow cytometry by counting GFP+ infected red blood cells. As shown in Figure 2D, we could observe that while mice infected with WT PbANKA parasites all developed parasitemia, mice that were inoculated with IL-6 Tg-PbANKA/LISP2 SPZ all failed to show any detectable parasitemia over a period of 20 days of follow up. Importantly, the two independent lines of IL-6 Tg-PbANKA/LISP2 displayed the same phenotype (Figure 2D). Mice infected with WT PbANKA parasites developed experimental cerebral malaria (ECM) and died around day 8 post-infection whereas mice injected with either IL-6 Tg-PbANKA/LISP2 line 1 or line 2 survived (Figure 2E). These data demonstrate that insertion of the mouse IL-6 gene in PbANKA parasites under control of a liver stage specific promoter results in the complete blockade of parasite development following SPZ injection. For the rest of the study, only the IL-6 Tg-PbANKA/LISP2 line 1 was used.
IL-6 Tg-PbANKA/LISP2 parasites arrest during liver stage development in mice
Next, mice were injected with SPZ i.v. and liver samples collected at indicated time points were subjected to RT‐qPCR analysis of parasite 18S rRNA (Figure 3A). The WT PbANKA parasite liver loads increased over time to reach a maximum at 48 h, followed by a decrease at 72 h, reflecting complete liver stage development and egress. In contrast, the liver load of IL-6 Tg-PbANKA/LISP2 parasites remained low over the course of infection, except at the 48-h time point, when the amount of IL-6 Tg-PbANKA/LISP2 parasites rose but at levels significantly lower than those obtained for WT PbANKA parasites. Therefore, RT‐qPCR analysis in the liver indicates that the loss of infectivity of IL-6 Tg-PbANKA/LISP2 SPZ is due to a defect in liver stage development in vivo.
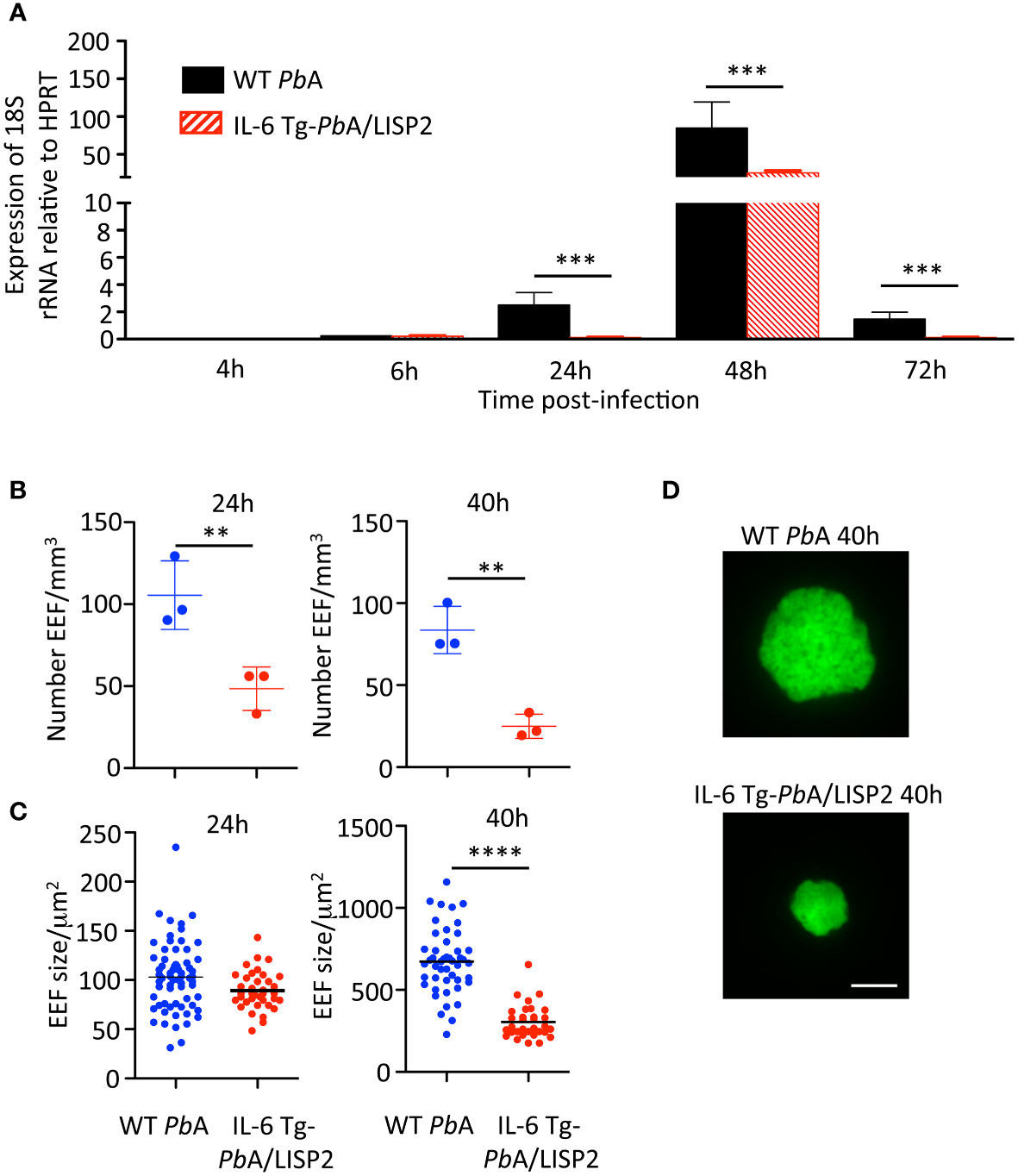
Figure 3 IL-6 Tg-PbANKA/LISP2 parasites show a development defect in the liver of infected mice. (A) Groups of C57BL/6 mice were injected i.v with 104 WT PbANKA or with IL-6 Tg-PbANKA/LISP2 SPZ and livers were collected at indicated time points. Parasite loads in the liver were assessed by measuring parasite 18S rRNA by real‐time RT‐qPCR. Gene mRNA expression was normalized to the parasite control gene HPRT Data are presented as the means ± SD from six individual values (***P < 0.001; Mann Whitney test). (B–D) Groups of 3 C57BL/6 mice were injected i.v with 150,000 WT PbANKA or IL-6 Tg-PbANKA/LISP2 SPZ and livers were harvested and imaged at the indicated time points. B) EEF density was determined for each condition and time point analyzing 8-25 Z-stacks per liver using a 10x objective. Bars denote the mean and standard deviation (Kruskall-Wallis test followed by Dunn’s multiple comparison, (**p < 0.01; Student’s t test). EEF area (C) was determined on maximal intensity projections of the acquired Z-stacks using Fiji. Graphs show pooled data from 3 livers per condition and time point (n= 32-66 per group), horizontal bars denote the mean (One-way ANOVA followed by Bonferroni post-test, **** p<0.0001, ns: non significant). (D) Representative images of WT PbANKA or IL-6 Tg-PbANKA/LISP2 EEF at 40 hours post-infection. Scale bar: 10 µm.
To confirm that IL6 expression impairs liver stage development of transgenic parasites, we examined by fluorescence microscopy EEF in the liver of mice harvested 24h or 40h after infection with 5 x 104 WT PbA or IL-6 Tg-PbANKA/LISP2 SPZ. As shown in Figure 3B, the EEF density was drastically reduced both at 24h and at 48h in mice infected with the IL-6 transgenic parasites as compared to those infected with WT PbA parasites. We also examined the size (Figure 3C) of EEF, and observed reduced size and diameter of IL-6 transgenic EEF as compared to WT PbA EEF, with a statistically significant difference only at 40h. Representative images of WT PbANKA or IL-6 Tg-PbANKA/LISP2 EEF at 40 hours post-infection are shown in Figure 3D. Altogether, these results demonstrate that IL-6 transgenic parasites display a developmental defect at the pre-erythrocytic stage in vivo.
IL-6 Tg-PbANKA/LISP2 parasites express IL-6 mRNA and secrete the murine IL-6 cytokine
We next assessed whether transgenic parasites express IL-6 in the liver of infected mice. The livers of C57BL/6 mice inoculated with 10,000 SPZ of either IL-6 Tg-PbANKA/LISP2 or WT PbANKA parasites were harvested at 48h post-infection and were subjected to RT-qPCR using primers designed to detect the codon-optimized parasite-encoded IL-6 transgene. As shown in Figure 4A, IL-6 transgene mRNA could be detected in mice infected with IL-6 Tg-PbANKA/LISP2 parasites. To verify whether the IL-6 Tg-PbANKA/LISP2 parasites secrete the IL-6 cytokine, supernatants were collected 48h after the HepG2 hepatoma cell line was cultured in the presence of IL-6 Tg-PbANKA/LISP2 or WT PbANKA SPZ. IL-6 measured by ELISA was detected only in supernatants from IL-6 Tg-PbANKA/LISP2-infected HepG2 cell cultures, but not from WT PbANKA-infected cultures (Figure 4B). These data confirm that IL-6 transgenic parasites are able to express and secrete IL-6 in vitro and in vivo, and suggest that the IL-6 transgene is fully functional, likely restricting liver stage development in vivo.
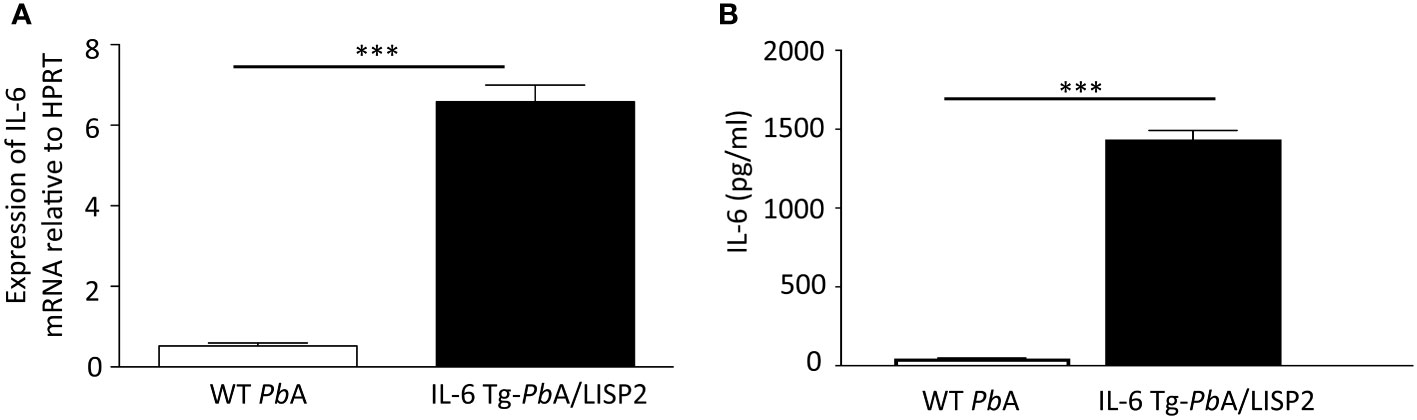
Figure 4 IL-6 Tg-PbANKA/LISP2 parasites express IL-6 mRNA and produce the murine IL-6 cytokine. (A) After infection with WT PbANKA or IL-6 Tg-PbANKA/LISP2 SPZ, livers were collected at 48h post-infection, and RT-qPCR analysis was used to measure the IL-6 mRNA expressed by EEF using a dedicated set of primers relative to the parasite control gene HSP70. (B) Determination of IL-6 by ELISA in supernatants obtained from HepG2 cultured in the presence of IL-6 Tg-PbANKA/LISP2 parasites or WT PbANKA for 48h. IL-6 was found to be secreted only in cultures with IL-6 Tg-PbANKA/LISP2 SPZ. Error bars, SEM. Data are representative of two independent experiments using triplicate samples (***p < 0.001; Mann Whitney test).
Interestingly, treatment of mice with anti-IL-6 receptor blocking antibodies before, during and after inoculation of IL-6 Tg-PbANKA/LISP2 SPZ (Supplementary Figure S1A), was not sufficient to reverse the developmental defect of transgenic parasites neither in terms of parasitemia (Supplementary Figure S1B) nor survival (Supplementary Figure S1C), suggesting that IL-6 produced by transgenic parasites very likely exerts its signaling effects intracellularly in infected hepatocytes.
To corroborate these results, an additional transgenic parasite line was generated where the mouse IL-6 transgene was placed under the control of uis4 gene promoter (IL-6 Tg-PbANKA/UIS4 parasites). UIS4 (for up-regulated in infectious sporozoites 4) is a parasitophorous vacuole membrane protein expressed in early liver stages (17). In this construct, the signal peptide of mIL6 was replaced by that of the Perforin-Like Protein 1 (PLP1) (Supplementary Figure S2A; Supplementary Table 1). As shown in Supplementary Figure S2B, similar to IL-6 Tg-PbANKA/LISP2 parasites, PCR analysis of genomic DNA from IL-6 Tg-PbANKA/UIS4 parasites confirmed correct integration of the constructs and the absence of residual parental parasites. IL-6 Tg-PbANKA/UIS4 parasites were transmitted to mosquitoes and SPZ infectivity was tested in C57BL/6 mice. In contrast with mice infected with IL-6 Tg-PbANKA/LISP2 SPZ, which failed to show any detectable parasitemia, all mice infected with IL-6 Tg-PbANKA/UIS4 parasites developed a parasitemia, similar to WT parasites (Supplementary Figure S2C). Mice infected with IL-6 Tg-PbANKA/UIS4, like WT PbANKA infected mice, developed experimental cerebral malaria and did not survive (Supplementary Figure S2D). Further in vitro experiments showed that the lack of phenotypic defect observed with IL-6 Tg-PbANKA/UIS4 parasites was associated with a defect to secrete IL6. Indeed, dosage of IL-6 cytokine in supernatants collected 48h after infection of HepG2 cell cultures with IL-6 Tg-PbANKA/LISP2, IL-6 Tg-PbANKA/UIS4 or WT PbANKA SPZ, showed that IL-6 was detectable only in supernatants from IL-6 Tg-PbANKA/LISP2-infected HepG2 cultures, but not in IL-6 Tg-PbANKA/UIS4 or from PbANKA-infected HepG2 cell cultures (Supplementary Figure S2E). These data indicate that in IL-6 Tg-PbANKA/UIS4 parasites the transgene construct does not allow proper secretion of IL6, and suggest that active secretion of IL-6 by transfected parasites is required for the blockade of the parasites at the liver stage.
Infection with IL-6 Tg-PbANKA/LISP2 parasites confers protection against challenge with WT PbANKA SPZ
We decided to investigate whether the abortive development in mice of IL-6 Tg-PbANKA/LISP2 parasites could result in protection against secondary infection with WT PbANKA SPZ. For this, different groups of mice were infected with IL-6 Tg-PbANKA/LISP2 SPZ using either 104 SPZ (group 1) or 5 x 104 SPZ (group 2) followed by a challenge with 104 WT PbANKA SPZ at day 30 post-infection with IL-6 Tg parasites (Figure 5A). Control groups consisted in mice infected with 104 or 5 x 104 WT PbANKA SPZ at day 0 (groups 3 and 4) and mice that received 104 WT PbANKA SPZ at day 30 (group 5). As expected, all control mice that received only WT PbANKA SPZ, either at day 0 (Groups 3 and 4, Figures 5B, C) or at day 30 (group 5) developed parasitemia and died. Remarkably, mice previously immunized with IL-6 Tg-PbANKA/LISP2 parasites displayed low parasitemia at day 6 post-challenge, irrespective of the dose of parasites used for immunization (Groups 1 and 2), with a delay in the pre-patent period of 2 days (day 36 versus day 34, highlighted by the log scale representation of the data) as compared to control mice infected with WT PbANKA SPZ (Group 5). This delay represents approximately 2 Log difference (100-fold) in parasite load between control and protected mice. Consistently, no mice which received IL-6 Tg-PbANKA/LISP2 SPZ died from ECM (Figure 5C). Similar observations were made when secondary infection with WT PbANKA SPZ was done at 60 days post priming (Supplementary Figure S3A). IL-6 Tg-PbANKA/LISP2 SPZ-immunized mice (Groups 1 and 2) were protected from ECM but however later succumbed from hyperparasitemia (Supplementary Figures S3B, C). These data demonstrate that one single inoculation with IL-6 transgenic PbANKA/LISP2 parasites is efficient in protecting mice against disease, but is not sufficient to induce sterile protection, even at the high dose of 5 x 104 SPZ.
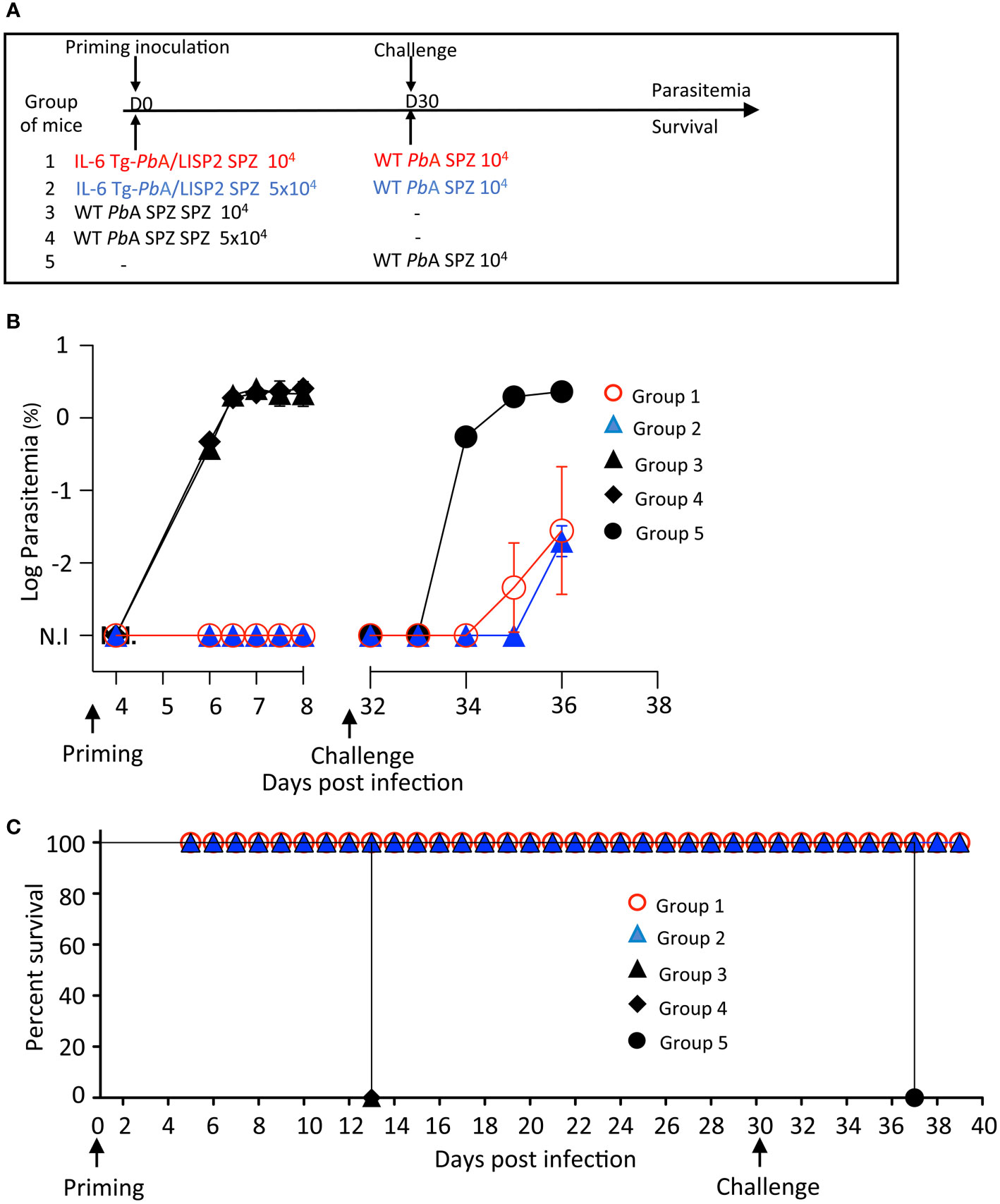
Figure 5 A single inoculation with IL-6 Tg-PbA/LISP2 SPZ is partially efficient in protecting mice against a lethal challenge. (A) Groups of C57BL/6 mice were infected with either 104 or 5 x 104 IL-6 Tg-PbA/LISP2 SPZ or with the same doses of WT PbANKA SPZ. Mice were then challenged with 104 PbANKA SPZ 30 days after priming. (B) Parasitemia of asexual blood forms were measured at indicated time points by flow cytometry, as all parasites were GFP tagged. (C) Survival rates were determined by Kaplan-Meier survival plots. Error bars, SEM. Data are representative of three independent experiments with 5 mice per group.
To optimize the protocol of the IL-6 Tg parasite-mediated protection, additional experiments were conducted in which mice were infected twice with 104 IL-6 Tg-PbANKA/LISP2 SPZ at three weeks interval, and then challenged 30 days (Figure 6A) or 60 days (Supplementary Figure S4A) later with 104 WT PbANKA SPZ. As shown in Figure 6B, mice that were infected with 104 IL-6 Tg-PbANKA/LISP2 SPZ (group 1) not only did not show any parasitemia after the first and the second infection with the recombinant parasites, as expected, but more interestingly did not develop any parasitemia upon challenge with WT parasites. Using the same infection scheme, we examined whether the protection against a lethal challenge depends on the dose of the primary IL-6 Tg-PbANKA/LISP2 infection. Groups of mice were infected twice at three weeks interval with IL-6 Tg-PbANKA/LISP2 SPZ at 103, or 102 SPZ per mouse followed 30 days later by a challenge with 104 WT PbANKA SPZ (Figure 6A). As shown in Figure 6B, the two doses of 103, or 102 of IL-6 Tg-PbANKA/LISP2 SPZ (group 2 and group 3, respectively) were inefficient at inducing sterile protection as challenged mice developed parasitemia at the same magnitude as the control mice, which received WT PbANKA SPZ only. All mice that had received the doses of 104 and 103 of IL-6 Tg-PbANKA/LISP2 SPZ, and 40% of mice injected with the dose of 102 SPZ, were protected from ECM (Figure 6C). In a long-term follow up, all mice immunized twice with 104 IL-6 Tg-PbANKA/LISP2 SPZ were sterily protected and survived, while those treated with the 103 and 102 doses of IL-6 Tg-PbANKA/LISP2 SPZ ultimately died from hyperparasitemia at day 9 and 10 after challenge, respectively. These data indicate that the prime boost immunization at the dose of 104 IL-6 Tg-PbANKA/LISP2 SPZ was efficient in conferring both clinical and sterilizing immunity.
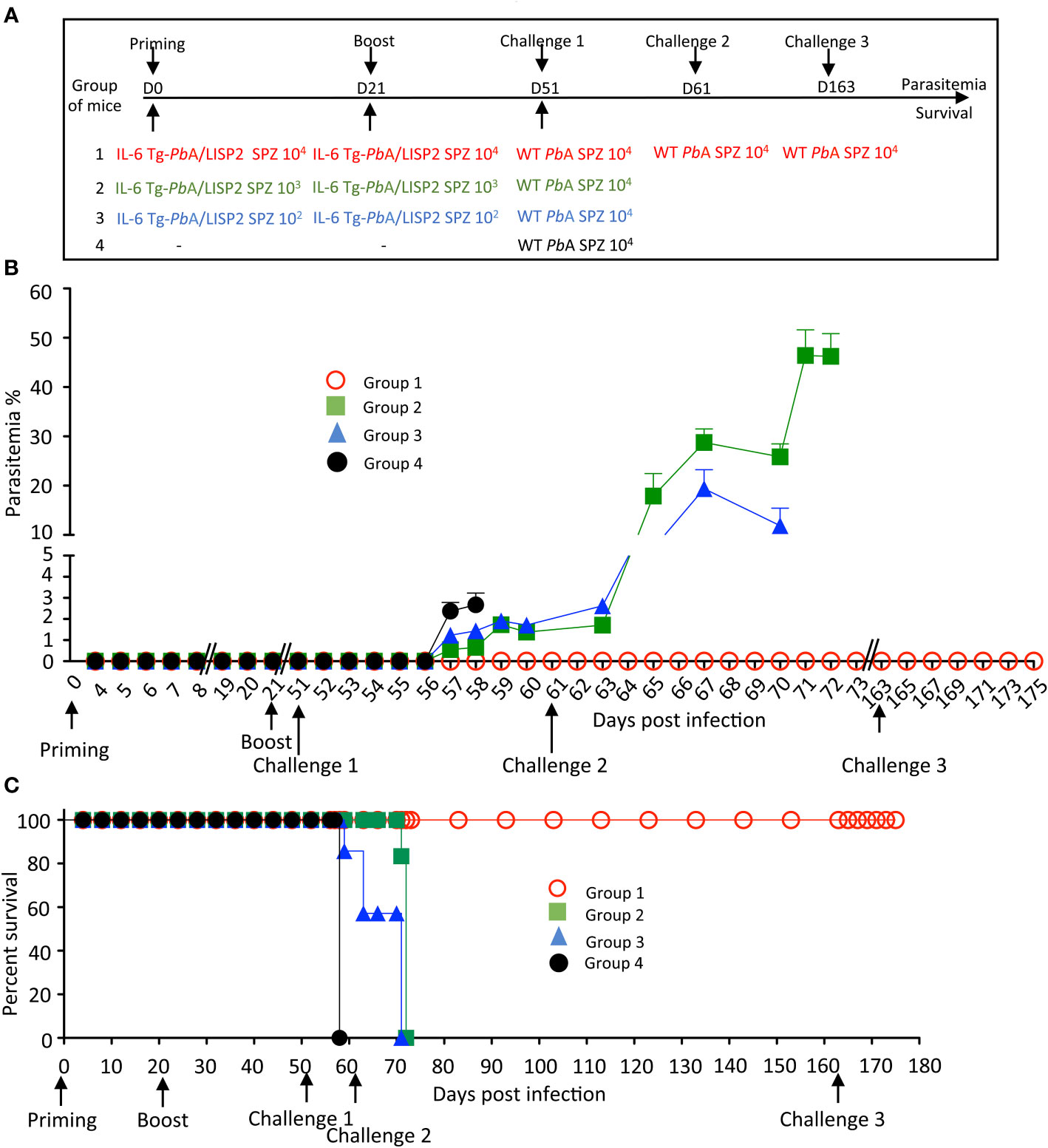
Figure 6 Homologous prime/boost immunization regimen with IL-6 Tg-PbANKA/LISP2 parasites confers a stable and efficient protection against challenge with WT PbANKA parasites. (A) Groups of C57BL/6 mice were infected with various doses of IL-6 Tg-PbA/LISP2 SPZ and boosted with the same doses at 3 weeks interval. Mice were then challenged 30 days after the boost (day 51) with 104 WT PbANKA SPZ. Mice of group 1, which all survived the lethal challenge, were then re-challenged at day 61 and day 163 with 104 PbANKA SPZ. (B) Parasite development was measured at indicated time points by flow cytometry, as all parasites were tagged with GFP. (C) Survival rates were determined by Kaplan-Meier survival plots. Error bars, SEM. Data are representative of three independent experiments with 5 mice per group.
In order to explore how long this protection could last, a slightly different protocol (Supplementary Figure S4A) was carried out where mice which received the priming and booster injections with IL-6 Tg-PbANKA/LISP2 SPZ were challenged 60 days after the boost. Similar results were obtained in terms of parasitemia (Group 1, Supplementary Figure S4B) and survival (Group 1, Supplementary Figure S4C) as in the mice challenged 30 days after the second IL-6 Tg-PbANKA/LISP2 infection, shown in Figure 6, with a sterile protection against a challenge with WT PbANKA SPZ in a homologous prime/boost immunization regimen (Group 1, Supplementary Figure S4A). This sterile protection which occurred 30 or 60 days after the second IL-6 Tg-PbANKA/LISP2 SPZ infection strongly suggests the induction of a robust memory response. To assess how efficient was the anti-malaria protection induced by IL-6 transgenic parasites, protected mice already challenged with WT PbANKA at day 51, were re-challenged twice with WT PbANKA at day 61 and later at day 163 (Group 1, Figure 6A). As shown in Figures 6B, C, none of the mice of this group developed parasitemia and all mice survived, indicating that protected mice survived and remained parasite free after multiple and temporally distant challenges with WT PbANKA parasites.
Infection with IL-6 Tg-PbANKA/LISP2 SPZ confers lasting protection in a species- transcending manner
To establish whether resolved IL-6 Tg-PbANKA/LISP2 SPZ-induced parasite infection might confer protection against challenge with heterologous parasites, C57BL/6 mice infected twice with IL-6 Tg-PbANKA/LISP2 SPZ and challenged one month later with WT PbANKA SPZ and which were completely immune to reinfection, were infected 4 months later either with homologous WT PbANKA SPZ or with heterologous nonlethal P. yoelii 17XNL SPZ (Figure 7A). In contrast to the control group of mice infected with P. yoelii 17XNL SPZ (Figure 7B, group 3) which developed parasitemia that steadily increased to reach a peak of around 20% at day 15 post-infection, mice that were previously immunized with IL-6 Tg-PbANKA/LISP2 SPZ and challenged with WT PbANKA SPZ showed only a limited parasitemia (1.8%) after a second challenge with P. yoelii 17XNL SPZ, which resolved rapidly and spontaneously until clearance by day 13 post-infection (Figure 7B, group 2). It must be pointed out that mice challenged with the homologous WT PbANKA SPZ (Figure 7B, group 1) did not develop any parasitemia, confirming our previous results. These data clearly demonstrate that priming with IL-6 Tg-PbANKA/LISP2 SPZ followed by a boost with the same mutant parasites protected mice not only against homologous but also against heterologous parasite infections. It must be pointed out that a challenge with WT PbANKA SPZ was not necessary since a challenge with P. yoelii 17XNL SPZ right after immunization with two injections of mutant SPZs was efficient in protecting mice against P. yoelii 17XNL infection (Supplementary Figure S5).
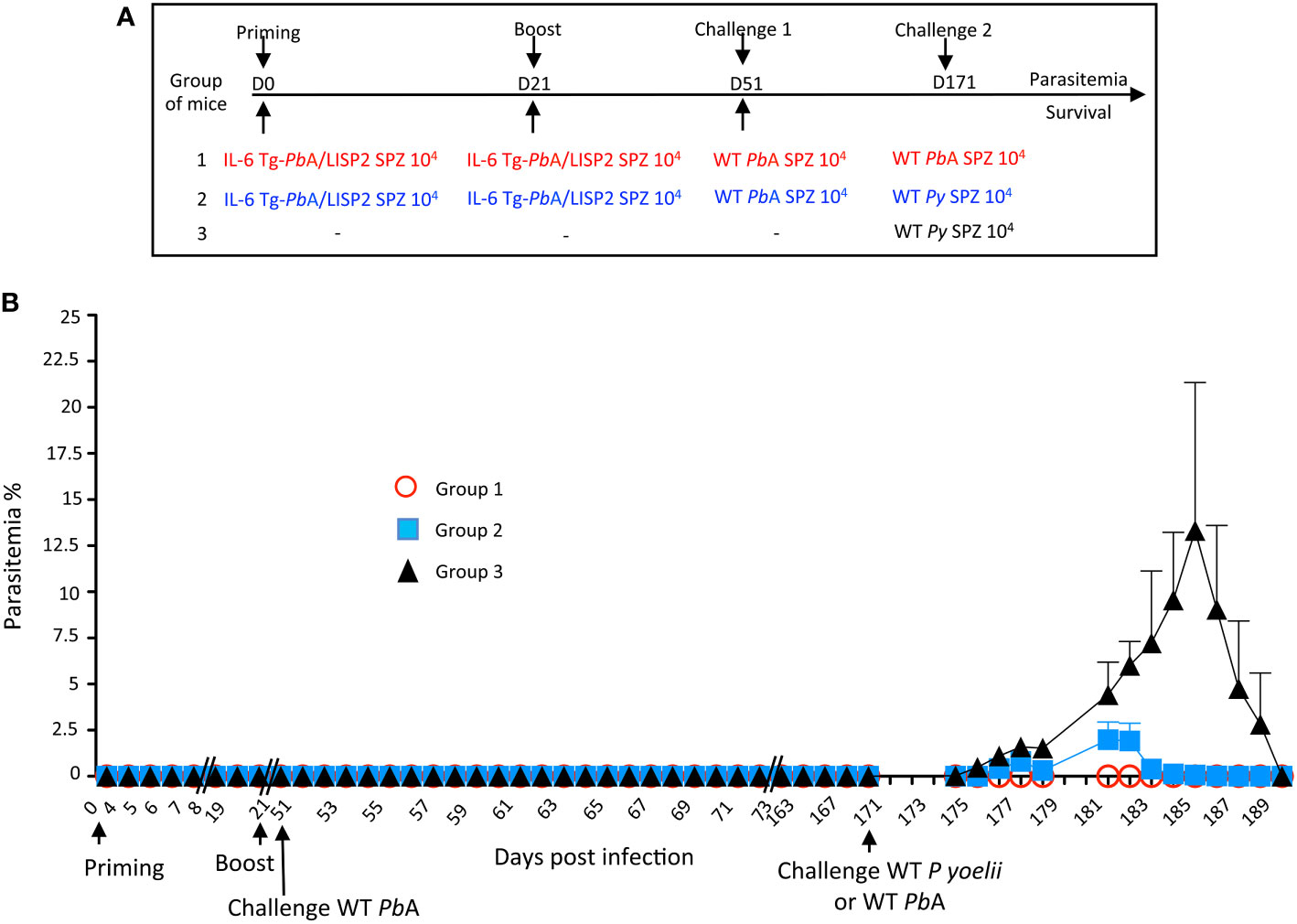
Figure 7 Prime/boost immunization regimen with IL-6 Tg-PbANKA/LISP2 parasites confers a stable and efficient protection against challenge with heterologous P. yoelii 17XNL SPZ. (A) Groups of C57BL/6 mice were infected twice with 104 IL-6 Tg-PbA/LISP2 SPZ at 3 weeks interval, and then challenged 30 days later (day 51) with 104 WT PbANKA SPZ. Mice were then challenged 4 months later (day 171) either with 104 WT PbANKA SPZ (group 1) or with 104 WT P. yoelii 17XNL SPZ (group 2). A control group of age-matched naïve mice received only 104 WT P. yoelii 17XNL SPZ. (B) Parasite development was measured at indicated time points by flow cytometry, as all parasites were tagged with GFP. Error bars, SEM. Data are representative of two independent experiments with 5 mice per group.
Protection conferred by IL-6 Tg-PbANKA/LISP2 SPZ is dependent on effector CD8+ T cells
In order to address whether the protection induced by IL-6 Tg-PbANKA/LISP2 parasites was dependent on effector CD8+ and/or CD4+ T cells, mice previously infected twice with IL-6 Tg-PbANKA/LISP2 parasites at 3-weeks interval were treated with normal mouse IgG, anti-CD8 or anti-CD4 depleting antibodies two days before and every other day during a challenge with 104 WT PbANKA SPZ, performed 30 days after the boost (Figure 8A). After challenge with WT PbANKA SPZ, parasite growth and cell depletion efficacy were monitored daily by flow cytometry in blood samples. Efficacy of CD4+ and CD8+ T cell depletion was continuously monitored during administration of T-cell depleting antibodies (Supplementary Figure S6). Interestingly, the measurement of parasitemia indicated a loss of protection upon treatment of mice with anti-CD8 (group 2) but not in mice treated with anti-CD4 (group 1) depleting antibodies, while as a control all mice treated with control IgG antibodies (group 3) showed sterile protection (Figure 8B). In terms of survival, in contrast to mice treated with control IgG and with anti-CD4 depleting antibodies, which all survived, mice depleted of CD8+ cells died from hyperparasitemia (Figure 8C). Control naive mice which received WT PbANKA parasites during challenge (Group 4, Figures 8B, C) developed parasitemia and died from cerebral malaria. These data strongly suggest that protection induced by IL-6 Tg-PbANKA/LISP2 parasites relies on CD8+ T cells but not CD4+ T cells.
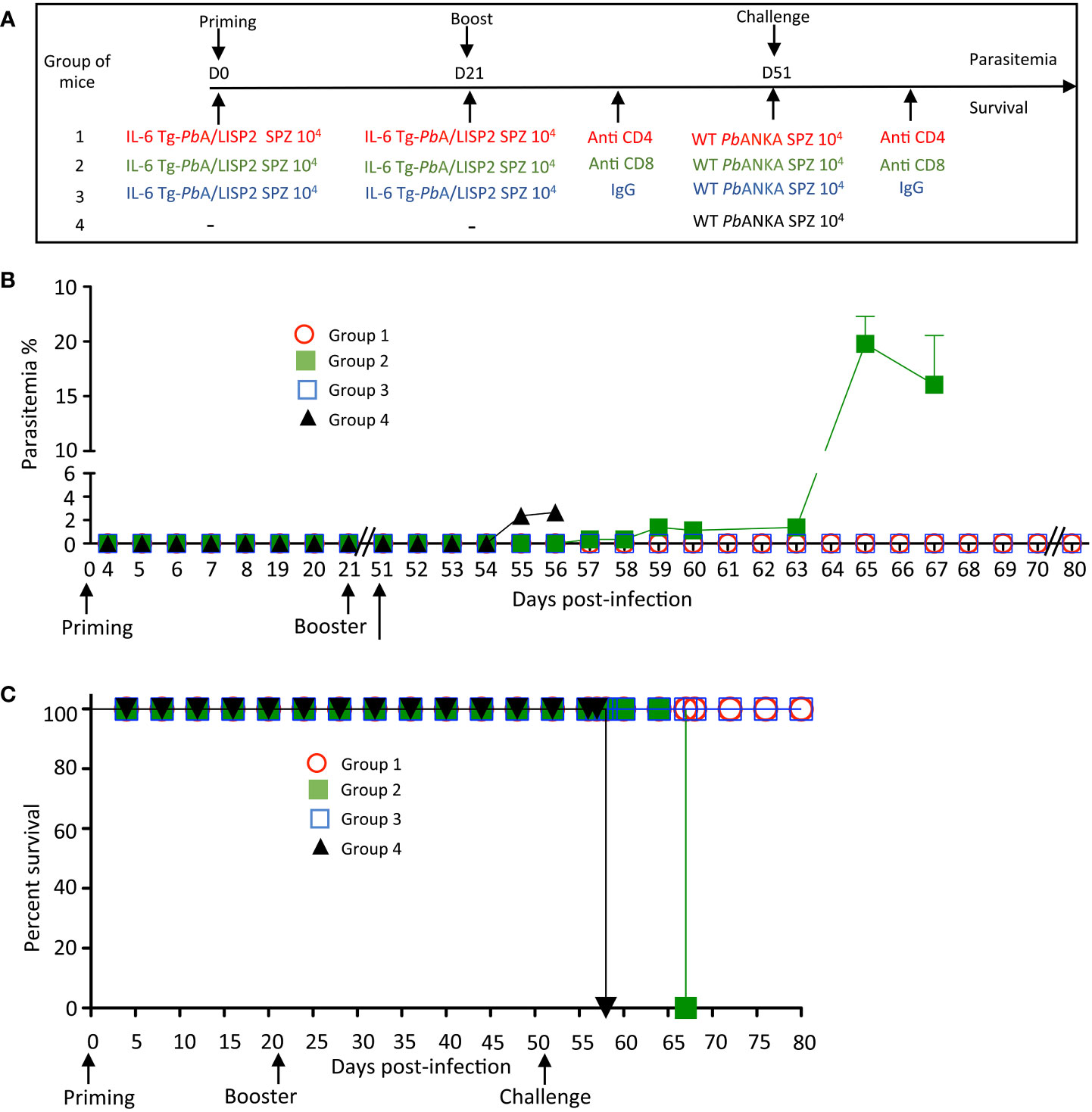
Figure 8 IL-6 Tg-PbANKA/LISP2 SPZ-mediated protection is dependent on CD8+ T cells. (A) Groups of C57BL/6 mice were infected twice with 104 IL-6 Tg-PbA/LISP2 SPZ at 3 weeks interval, and then challenged 30 days later (day 51) with 104 WT PbANKA SPZ. Two groups of mice were treated with either anti-CD4 (group 1) or anti-CD8 (group 2) depleting antibodies 2 days before and every other day after challenge. Control mice (group 3) were treated with non-specific IgG. (B) Parasitemia was monitored over time by FACS analysis, as parasites were tagged with GFP. (C) Survival rates were determined by Kaplan-Meier survival plots. Error bars, SEM. Data are representative of two independent experiments with 5 mice per group.
IL-6 Tg-PbANKA/LISP2-induced protection is associated with the accumulation of memory T cells
To examine if sterile protection against malaria infection and disease induced by IL-6 Tg-PbANKA/LISP2 parasites was associated with the development of memory T cells, a FACS analysis was performed using a gating strategy shown in Figure 9A. Cells were first gated on CD45+ CD3+ cells, then positive cells were gated on the expression of CD44 and CD25 markers. CD44+ CD25- T cells were then gated on either CD4+ or CD8+ T cells. CD4+ and CD8+ T cells were further gated based on the expression of CD69 and CD62L-positive cells to analyze various subsets of memory T cells: Effector memory T cells (TEM): CD69– CD62L– CD44+ (Figure 9B, panels e to h), Central memory T cells (TCM): CD69– CD62L+ CD44+ (Figure 9B, panels i to l), and liver resident memory T cells (TRM): CD69+ CD62L- CD44+ (Figure 9B, panels m to p). Figure 9B shows the analysis of the expansion and the phenotype of liver CD4 and CD8 T cells following a recall response one week after mice were inoculated twice with 10, 000 IL-6 Tg-PbANKA/LISP2 SPZ at three weeks interval (Group 1, G1, Figure 9B) in comparison to mice infected with WT PbA SPZ (Group 2, G2, Figure 9B) and to naive mice (Group 3, G3, Figure 9B). The absolute number of CD4 (panel b) and CD8 (panel d) were much higher in protected mice (G1) than in infected (G2) and in naïve (G3) mice. A higher percentage of CD8+ T cells (panel c) but not CD4+ T cells (panel a) was also observed in G1 protected mice. A significantly higher total number of TEM, TCM, and TRM CD4 (panels f, j, n, respectively), and CD8 (panels h, l, p, respectively) were observed in protected G1 mice as compared to WT PbA G2 infected mice and to naïve G3 mice. These results are consistent with the efficient and long-lasting CD8-dependent immune memory elicited by IL-6 Tg-PbANKA/LISP2 SPZ.
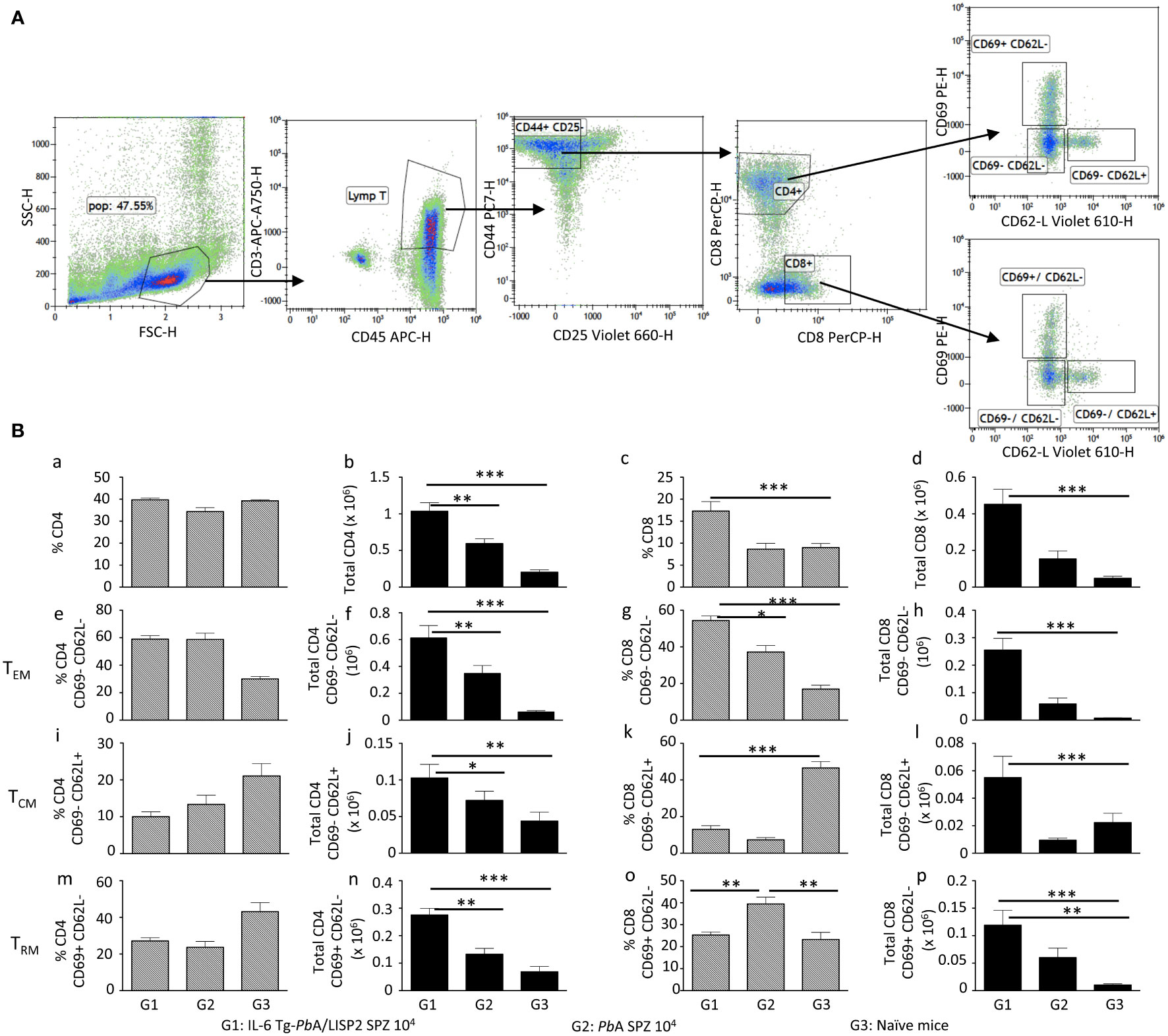
Figure 9 IL-6 Tg-PbANKA/LISP2-induced protection is associated with the accumulation of memory T cells. Livers were harvested one week after 6-week-old female C57BL/6 mice were infected twice with 104 IL-6 Tg-PbA/LISP2 SPZ at 3 weeks interval (G1) or one week after mice were infected with WT PbA SPZ (G2) or from naive mice (G3) (n=6 per group). Leukocytes present in liver tissue were analyzed using the following markers: CD45, CD3, CD4, CD8, CD25, CD44, CD69, and CD62L. The gating strategy is shown in (A). Results are expressed in percentage (a, c, e, g, i, k, m, o) and in absolute number (b, d, f, h, j, l, n, p) of TEM (e, f, g, h), TCM (I, j, k, l), and T RM (m, n, o, p) positive cells (B). Results are representative for two independent experiments (Mann Whitney test; * p<0.05; **p<0.01; ***p<0.001).
Discussion
The development of vaccines against malaria infection (prevalence and parasite density) and disease (clinical episodes) needs improvements to overcome existing shortcomings (18). In this study, we developed a new strategy based on the use of transgenic parasites expressing a host cytokine. Successfully devised transgenic PbANKA parasites, expressing murine IL-6 restricted to the liver stage, induced two important processes. First, the presence of the IL6 transgene impaired parasite maturation in the host liver, leading to abortive development and a failure to progress toward blood stage parasites. Second, a homologous prime/boost immunization of mice with IL-6 Tg-PbANKA/LISP2 SPZ was able to protect mice against multiple and temporally distant challenges with WT PbANKA parasites and against heterologous challenge with P. yoelii. Collectively, our data show that a suicide strategy based on IL-6 expression by the parasite is an efficient approach not only to attenuate Plasmodium infection at the liver stage but also to elicit protective immunity against an infectious SPZ challenge.
IL-6 is known to be a key cytokine involved in different arms of the immune response. It was shown to support the growth and enhancement of antibody production by B cells (19), as IL-6-deficient mice are impaired in their IgG production upon immunization (20). On the other hand, IL-6 is critical in regulating CD4 T cell differentiation by promoting IL-4 production during T cell activation (21) and IL-21 production, an essential effector cytokine produced by Tfh cells (22). Moreover, IL-6 in combination with IL-7 signaling promotes CD8 memory T cell generation after vaccination (23). By enhancing locally two main features of the IL-6 cytokine, namely its pro-inflammatory and immune-stimulatory properties at the very moment when Plasmodium SPZ invade hepatocytes, we provide proof-of-principle that this strategy could be considered for future human vaccine strategies.
Our laboratory has gathered during the last decade experimental evidence, using various murine models for malaria, that the IL-6 response is critical in controlling the parasite growth by generating an effective anti-parasite immune response (7–10). We decided to focus our study on the IL-6 cytokine for multiple reasons. First, during inflammation, IL-6 was found to be constitutively stored by murine neutrophils (10, 24) and its secretion upon TLR activation is the major inducer of the hepatic acute phase proteins (25). In a more recent work, we used mice deficient in the multidrug resistance-2 gene (Mdr2−/−), which encodes the canalicular phospholipid flippase leading to a complete absence of phospholipids from bile, which were found to spontaneously develop liver injury with a typical inflammatory profile. In this model, we demonstrated that the intra-hepatocyte parasite development was impaired via an IL-6-dependent mechanism (9) and the abortive infection resulted in a long-lasting immunity in Mdr2−/− mice against infectious SPZ. This IL-6-driven potent immune response was observed in the Mdr2-/- mouse model we reported recently where not only total CD8+ and CD4+ cells but also CD8+ and CD4+ tissue resident memory T cells were present at a significantly higher levels in SPZ challenged Mdr2-/- mice (9).
The use of IL-6 transgenic parasites for vaccine purposes must meet safety requirements. Accordingly, while developing this concept, the caveat that we imposed to ourselves was to avoid expression of the IL-6 cytokine systemically, i.e. during the blood stage. Second, side effects such as the genesis of tumors due to chronic IL-6 production may be avoided by the time-frame limited development of the Plasmodium hepatic stage (26). Our strategy was to express the mouse il-6 gene under the promoter of LISP2 selected for its unique profile expression which is limited to the mid‐to‐late liver stage, as analysis by RT-qPCR showed that LISP2 expression peaked at 48 hpi, and gradually decreased until reaching 60 h post infection (16). Subsequently, production of IL-6 by transgenic parasites would be limited at this window of time, not exceeding the hepatic late stage merozoites. To assess whether IL-6 gene transfected PbANKA parasites do indeed transcribe and secrete the mouse IL-6, we demonstrated that IL-6 was present in supernatants of HepG2 cell line cultured in the presence of IL-6 Tg-PbANKA/LISP2 SPZ but not in those cultured with WT PbANKA SPZ, indicating that the IL-6 production machinery was perfectly operational in IL-6 gene transfected PbANKA parasites. More importantly, in vivo analysis showed that at the peak of IL-6 transgenic parasite growth in the liver, namely at 48h post-infection, IL-6 mRNA transcripts were detected at the same time, suggesting an in vivo secretion of IL-6. This in vivo IL-6 production correlates with the infection pattern of mice infected with IL-6 Tg-PbANKA/LISP2 SPZ, namely, a complete blockade of the parasite development at the liver stage. These data are in accordance with the elicitation of an anti-parasite immune response by the IL-6 Tg-PbANKA/LISP2 SPZ due to the readily transcribed and secreted IL-6 early during parasite development into hepatocytes. Interestingly, no reversal of the infection phenotype of the IL-6 transgenic parasites was observed upon IL-6R blockade. These data strongly suggest that the IL-6-mediated signaling events most probably occur intracellularly and therefore are not affected by the receptor blockade of infused antibodies. There is indeed evidence that IL-6 acts as an intracrine growth factor in a variety of renal cell carcinoma cell lines which express no surface IL-6 receptors (27). Intriguingly, IL6 secretion was not observed in IL-6 Tg-PbANKA/UIS4 parasites, and accordingly no defect during liver stage development was observed with this parasite line. In the IL-6 Tg-PbANKA/UIS4 parasites, the transgene contains the signal peptide from PLP1, a SPZ-specific protein (28), which may not function properly in liver stages, explaining the failure to secrete the cytokine.
With regard to other Plasmodium parasites engineered to produce host cytokines, only one early study demonstrated that transgenic P. knowlesi parasites express bioactive IFN-γ. Unfortunately, the authors did not examine further the infectious behavior and whether this transgenic parasite was protective or not against an infectious parasite challenge (29). In contrast to our present study, none of these reports could investigate the mechanisms underlying these partial protective capabilities. There has been a number of reports to show that SPZs genetically attenuated that not complete their development in hepatocytes can elicit protection against subsequent infections. What is original in the present study is that the parasite was specifically targeted to express a host cytokine that is crucial in acquisition of immunity. For now, we consider these findings as proof of concept and future studies will be necessary to determine how protection induced by IL-6-expressing parasites compare with other attenuated parasite vaccines, including RAS.
To assess whether the inoculation of mice with IL-6 Tg-PbANKA/LISP2 SPZ was able to prime the immune system to cope with a subsequent challenge with WT PbANKA SPZ, we designed several protocols where we explored various parameters including single immunization at different dose levels, and homologous prime/boost immunization regimens with various time intervals between the immunization and the challenge with WT PbANKA SPZ, which was spanning between 30 and 60 days. Our results indicate that a single dose of IL-6 Tg-PbANKA/LISP2 SPZ, did not fully protect mice against blood stage infection. However, we observed a significantly prolonged pre-patent period. In contrast, a full anti-disease and anti-parasite immunity were achieved after a homologous prime/boost delivery of IL-6 Tg-PbANKA/LISP2 SPZ whether the challenge with WT parasites occurred at 30 days or even at 60 days after the booster dose of IL-6 Tg-PbANKA/LISP2 SPZ. Furthermore, this protection was persistent and was maintained even after multiple challenges with WT parasites. This is indicative of a long-lasting immune memory elicited by IL-6 transgenic parasites. Protection was also observed in mice that were challenged with P. yoelii SPZ, indicating that immunization with IL6-expressing parasites induces species-transcending immunity. We noticed that while induction of sterile immunity was dose-dependent, the observed liver stage-arrest of the transgenic parasites was dose-independent. This suggests that the protective immunity not only relies on the intrahepatic developmental arrest of the parasite but also on an optimal load of parasite antigens.
The failure to mount robust CD4+ and CD8+ T cell responses during natural infection via infectious mosquito bites is believed to be related to relatively low amounts of inoculated SPZ (about 100 SPZ), ultimately leading to a very limited number of infected hepatocytes, followed by a rapid release of merozoites in the bloodstream (2 days), at least in rodents. To overcome this insufficient parasite antigen availability during the short window of liver stage development, a more optimal liver stage antigen-specific CD4+ and CD8+ T cell responses can be triggered by a more persistent liver stage-arresting GAP. In support of this, efficient CD8+ T cell responses can be elicited by late liver stage-arresting GAPs which provide a persistent source of parasite antigens (1). In addition to CD8+ T cells which are critical effectors for the control of Plasmodium-infected hepatocytes in various species including humans, non-human primates and rodents (30), CD4+ T cells that recognize parasite-derived immunogenic peptides in the context of MHC class II molecules expressed on infected hepatocytes also contribute in effector mechanisms against Plasmodium parasite liver stages (31). CD4+ T cells were also found to participate to protection against Plasmodium infection by inducing antibody production and macrophage activation (32, 33). As an alternative strategy, we generated a liver stage-arresting IL-6 transgenic Plasmodium parasites which not only blocks blood stage infection but also conferred a long-lasting protection against virulent challenge with WT parasites. The requirement of only CD8 T cells in the protection induced by IL-6 transgenic parasites was demonstrated by the loss of control of parasite development into blood stages in mice treated with anti-CD8 but not in those treated with anti-CD4 depleting antibodies.
The defect in parasite development in IL-6 transgenic SPZ-infected mice which are completely refractory to infection even after multiple challenges with high doses of SPZ predicts that elicitation of T cells upon a successful vaccination regimen might have taken place in these mice. It is important to ask whether the abortive development of EEF resulted in the priming of immune cells such as CD8+ T cells which were shown to correlate with protection against challenge with P. berghei in mice (34, 35) and against radiation-attenuated P. falciparum SPZ immunization in humans (36). A subset of CD8+ T cells, termed tissue-resident memory T cells (Trm), has been reported to act as sentinels against invading pathogens, in particular these cells are capable of recognizing infected hepatocytes, and their depletion abrogated protection in mice (37, 38). Our results show indeed that not only total CD8+ and CD4+ cells but also CD8+ and CD4+ Trm cells were present at a significantly higher absolute number in mice infected with IL-6 transgenic SPZ than in WT PbA-infected mice or naïve mice. In search of other subsets of memory T cells, we examined the generation of CD4 and CD8 effector memory (Tem) and central memory (Tcm) T cells, defined on the basis of the surface expression of CD44, CD69, and CD62L after a prime/boost immunization protocol of mice infected with IL-6 transgenic SPZ. Similar to Trm cells, it was striking to observe that protected mice displayed elevated absolute numbers of CD4 and CD8 Tcm and Tem in their livers even after parasites were completely cleared. These two subsets of memory T cells were previously reported in an alternative immunization protocol whereby following radiation-attenuated SPZ immunization, the overall population of intrahepatic CD8 T cells significantly increases as compared to naïve mice and protection was linked to the increase of both CD8 Tem and Tcm (39).
In conclusion, we demonstrate that transgenic P. berghei parasites that express and secrete the host immune modulatory factor IL-6 during liver stage development exhibit striking new features with a dual action on malaria infection outcome. First, IL-6 expression abolishes parasite growth at the pre-erythrocytic stage and second, establishes a long-lasting immune protection against a lethal parasite challenge against different rodent malaria species. Our strategy to express murine IL-6 gene under a stage-specific promoter restricts its production locally to the liver. This work opens new avenues for the development of effective live attenuated vaccines against EEF of human Plasmodium parasites and is highly relevant to combat other pathogens that infect the liver.
Data availability statement
The original contributions presented in the study are included in the article/Supplementary Material. Further inquiries can be directed to the corresponding author.
Ethics statement
The animal study was reviewed and approved by CETEA (comité d’éthique en expérimentation animale) (Permit Number N° dap180040 issued on 2018).
Author contributions
SM: formulation of the initial concept, preparation of the original draft. SyB, SeB, RP, JP, P-HC, PF: performed the experiments. AS: funding acquisition and discussion. OS, SyB: design and creation of the IL-6 transgenic Plasmodium berghei lines. OS, RA, AS: critical review and editing of the manuscript. All authors contributed to the article and approved the submitted version.
Funding
This work has been supported by the French Parasitology consortium ParaFrap (ANR-11-LABX0024), and by a grant from Institut Pasteur to SM.
Acknowledgments
We thank the CEPIA (Centre d’élevage, de production et d’infection des anopheles, Institut Pasteur, Paris) for providing Anopheles mosquitoes, and the cytometry platform (CB-UtechS facility) at the Institut Pasteur for providing technical assistance. We thank Emma Brito-Fravallo (Genetics and genomics of insect vectors Unit, Institut Pasteur) for providing technical assistance.
Conflict of interest
The authors declare that the research was conducted in the absence of any commercial or financial relationships that could be construed as a potential conflict of interest.
Publisher’s note
All claims expressed in this article are solely those of the authors and do not necessarily represent those of their affiliated organizations, or those of the publisher, the editors and the reviewers. Any product that may be evaluated in this article, or claim that may be made by its manufacturer, is not guaranteed or endorsed by the publisher.
Supplementary material
The Supplementary Material for this article can be found online at: https://www.frontiersin.org/articles/10.3389/fimmu.2023.1143012/full#supplementary-material
Supplementary Figure 1 | Treatment with anti-IL-6R blocking antibodies does not reverse the infection phen otype of IL-6 transgenic parasites. Parasitemia (B) and survival (C) of 6-week-old female C57BL/6 mice (n=5 per group) treated i.v. with either anti-IL-6R antibody (Group 2) or with the control IgG isotype (Group 1) at 500 mg/mouse 1 day before with 104 IL-6 Tg-PbA/LISP2 SPZ. Mice received three additional doses of antibodies at day 0, day 1 and day 2 post-infection (A). Control mice were infected with 104 WT PbANKA SPZ Group 3). Parasitemia and Kaplan-Meier survival plots (Mantel-Cox test **p<0.0061, ***p=0.001) were recorded over time. Results are from two independent experiments.
Supplementary Figure 2 | Generation and characterization of IL-6 Tg-PbA/UIS4 parasites. (A) Replacement strategy to modify the PbGFP locus to express murine IL6 under control of the uis4 promoter. We used the selection-linked integration strategy to integrate the construct containing a GFP-2A-hDHFR cassette without promoter followed by the mIL6 cassette under control of the PbUIS4 promoter. The signal peptide (SP, red) of PbPLP1 was used in this construct. Parasites undergoing homologous recombination at the GFP and PbDHFR 3’UTR sequences were isolated after one round of pyrimethamine selection. Genotyping primers and expected PCR fragments are indicated by arrows and lines, respectively. (B) PCR analysis of genomic DNA from IL-6 Tg-PbA/UIS4 parasites. We used primer combinations to detect the non-recombined parental locus (WT, 830-bp), the 5’ integration event (5’int, 819-bp), the 3’ integration event (3’int, 1554-bp) or the non-integrated construct (plasmid, 2197-bp). The WT primer combination is expected to amplify a ~5.6-bp fragment from the recombined locus of IL-6 Tg-PbA/UIS4 parasites. The results confirm the correct integration of the constructs at the GFP locus of PbGFP parasites, and show the absence of parental parasites in the transfectants after a single round of pyrimethamine selection. (C) Three groups of C57BL/6 mice (5 mice per group) were infected with 104 WT PbANKA, IL-6 Tg-PbA/LISP2 or IL-6 Tg-PbA/UIS4 SPZ. Parasitemia were measured at indicated time points by flow cytometry, as all parasites were GFP tagged. (D) Survival rates were determined by Kaplan-Meier survival plots. (E) Quantification of IL-6 by ELISA in supernatants obtained from HepG2 cultured for 48h in the presence of WT PbANKA, IL-6 Tg-PbANKA/LISP2 or IL-6 Tg-PbANKA/UIS4 parasites. IL-6 was found to be secreted only in cultures infected with IL-6 Tg-PbANKA/LISP2 SPZ. Error bars, SEM.
Supplementary Figure 3 | Delayed challenge after priming with a single IL-6 Tg-PbA/LISP2 SPZ inoculation is efficient but not fully protecting mice against lethal infection. (A) Groups of C57BL/6 mice were inoculated with either 104 or 5 x 104 IL-6 Tg-PbA/LISP2 SPZ or with the same doses of WT PbANKA SPZ. Mice were then challenged with 104 PbANKA SPZ 60 days after priming. (B) Parasite development was measured at indicated time points by flow cytometry, as all parasites were tagged with GFP. (C) Survival rates were determined by Kaplan-Meier survival plots. Error bars, SEM. Data are representative of three independent experiments with 5 mice per group.
Supplementary Figure 4 | Homologous prime/boost immunization regimen with IL-6 Tg-PbANKA/LISP2 parasites confers protection against temporally distant challenge with WT PbANKA parasites. (A) Groups of C57BL/6 mice were infected twice with 104 IL-6 Tg-PbA/LISP2 SPZ at 3 weeks interval or with the same dose of WT PbANKA SPZ as control. Mice were then challenged with 104 PbANKA SPZ 60 days after the booster injection. (B) Parasite development was measured at indicated time points by flow cytometry, as all parasites were tagged with GFP. (C) Survival rates were determined by Kaplan-Meier survival plots. Error bars, SEM. Data are representative of three independent experiments with 5 mice per group.
Supplementary Figure 5 | Prime/boost immunization regimen with IL-6 Tg-PbANKA/LISP2 parasites confers a stable and efficient protection against challenge with heterologous P. yoelii 17XNL SPZ. (A) Groups of C57BL/6 mice were infected twice with 104 IL-6 Tg-PbA/LISP2 SPZ at 3 weeks interval, and then challenged 30 days later (day 51) with either 104 WT PbANKA SPZ (Group 1) or with WT P. yoelii 17XNL SPZ (group 2). Control groups of age-matched naïve mice received only 104 WT P. yoelii 17XNL SPZ (Group 3) or WT PbANKA SPZ (Group 4). (B) Parasite development was measured at indicated time points by flow cytometry, as all parasites were tagged with GFP. Error bars, SEM. Data are representative of one experiment with 5 mice per group.
Supplementary Figure 6 | Assessment of leukocyte depletion. In vivo depletion of CD4+ or CD8+ T cells in immunized mice using anti-CD4 or anti-CD8 depleting antibodies was assessed by measuring daily the percentage of residual (A) CD8+ or (B) CD4+ T cells in the blood by FACS analysis. Typical analysis performed at day 2 post treatment, corresponding to the day of challenge with WT PbANKA SPZ (refer to ), is shown in this figure. Representative data from two independent experiments with 5 mice per group are shown.
Supplementary Table 1 | Sequences of the transgenes used to generate IL-6 Tg-PbA/LISP2 and IL-6 Tg-PbA/UIS4 transgenic P. berghei parasites.
Supplementary Table 2 | Primers used to assess the integration of the murine IL-6 transgene into the PbANKA parasite genome.
Supplementary Table 3 | List of oligonucleotides used for RT-qPCR analyses to assess parasite load and to quantify IL-6 expression.
Abbreviations
ECM, experimental cerebral malaria; EEF, exo-erythrocytic form; GAP, genetically-attenuated parasite; Pb, Plasmodium berghei; SPZ, sporozoite; WT, wild-type.
References
1. Goswami D, Minkah NK, Kappe SHI. Designer parasites: Genetically engineered plasmodium as vaccines to prevent malaria infection. J Immunol (2019) 202(1):20–8. doi: 10.4049/jimmunol.1800727
2. Nganou-Makamdop K, Sauerwein RW. Liver or blood-stage arrest during malaria sporozoite immunization: the later the better? Trends Parasitol (2013) 29(6):304–10. doi: 10.1016/j.pt.2013.03.008
3. Singh AP, Buscaglia CA, Wang Q, Levay A, Nussenzweig DR, Walker JR, et al. Plasmodium circumsporozoite protein promotes the development of the liver stages of the parasite. Cell (2007) 131(3):492–504. doi: 10.1016/j.cell.2007.09.013
4. Sun T, Holowka T, Song Y, Zierow S, Leng L, Chen Y, et al. A plasmodium-encoded cytokine suppresses T-cell immunity during malaria. Proc Natl Acad Sci U.S.A. (2012) 109(31):E2117–26. doi: 10.1073/pnas.1206573109
5. Augustijn KD, Kleemann R, Thompson J, Kooistra T, Crawford CE, Reece SE, et al. Functional characterization of the plasmodium falciparum and p. berghei homologues of macrophage migration inhibitory factor. Infect Immun (2007) 75(3):1116–28. doi: 10.1128/iai.00902-06
6. Miller JL, Harupa A, Kappe SH, Mikolajczak SA. Plasmodium yoelii macrophage migration inhibitory factor is necessary for efficient liver-stage development. Infect Immun (2012) 80(4):1399–407. doi: 10.1128/iai.05861-11
7. Demarta-Gatsi C, Smith L, Thiberge S, Peronet R, Commere PH, Matondo M, et al. Protection against malaria in mice is induced by blood stage-arresting histamine-releasing factor (HRF)-deficient parasites. J Exp Med (2016) 213(8):1419–28. doi: 10.1084/jem.20151976
8. Demarta-Gatsi C, Peronet R, Smith L, Thiberge S, Ménard R, Mécheri S. Immunological memory to blood-stage malaria infection is controlled by the histamine releasing factor (HRF) of the parasite. Sci Rep (2017) 7(1):9129. doi: 10.1038/s41598-017-09684-2
9. Grand M, Waqasi M, Demarta-Gatsi C, Wei Y, Peronet R, Commere P-H, et al. Hepatic inflammation confers protective immunity against liver stages of malaria parasite. Front Immunol (2020) 11:585502(585502). doi: 10.3389/fimmu.2020.585502
10. Mathieu C, Demarta-Gatsi C, Porcherie A, Brega S, Thiberge S, Ronce K, et al. Plasmodium berghei histamine-releasing factor favours liver-stage development via inhibition of IL-6 production and associates with a severe outcome of disease. Cell Microbiol (2015) 17(4):542–58. doi: 10.1111/cmi.12382
11. Birnbaum J, Flemming S, Reichard N, Soares AB, Mesén-Ramírez P, Jonscher E, et al. A genetic system to study plasmodium falciparum protein function. Nat Methods (2017) 14(4):450–6. doi: 10.1038/nmeth.4223
12. Manzoni G, Briquet S, Risco-Castillo V, Gaultier C, Topçu S, Ivănescu ML, et al. A rapid and robust selection procedure for generating drug-selectable marker-free recombinant malaria parasites. Sci Rep 4 4760 (2014). doi: 10.1038/srep04760
13. Janse CJ, Ramesar J, Waters AP. High-efficiency transfection and drug selection of genetically transformed blood stages of the rodent malaria parasite plasmodium berghei. Nat Protoc (2006) 1(1):346–56. doi: 10.1038/nprot.2006.53
14. Schindelin J, Arganda-Carreras I, Frise E, Kaynig V, Longair M, Pietzsch T, et al. Fiji: an open-source platform for biological-image analysis. Nat Methods (2012) 9(7):676–82. doi: 10.1038/nmeth.2019
15. De Niz M, Helm S, Horstmann S, Annoura T, Del Portillo HA, Khan SM, et al. In vivo and in vitro characterization of a plasmodium liver stage-specific promoter. PloS One (2015) 10(4):e0123473. doi: 10.1371/journal.pone.0123473
16. Orito Y, Ishino T, Iwanaga S, Kaneko I, Kato T, Menard R, et al. Liver-specific protein 2: a plasmodium protein exported to the hepatocyte cytoplasm and required for merozoite formation. Mol Microbiol (2013) 87(1):66–79. doi: 10.1111/mmi.12083
17. Mueller AK, Camargo N, Kaiser K, Andorfer C, Frevert U, Matuschewski K, et al. Plasmodium liver stage developmental arrest by depletion of a protein at the parasite-host interface. Proc Natl Acad Sci U.S.A. (2005) 102(8):3022–7. doi: 10.1073/pnas.0408442102
18. Laurens MB. The promise of a malaria vaccine-are we closer? Annu Rev Microbiol (2018) 72:273–92. doi: 10.1146/annurev-micro-090817-062427
19. Hirano T, Yasukawa K, Harada H, Taga T, Watanabe Y, Matsuda T, et al. Complementary DNA for a novel human interleukin (BSF-2) that induces b lymphocytes to produce immunoglobulin. Nature (1986) 324(6092):73–6. doi: 10.1038/324073a0
20. Kopf M, Baumann H, Freer G, Freudenberg M, Lamers M, Kishimoto T, et al. Impaired immune and acute-phase responses in interleukin-6-deficient mice. Nature (1994) 368(6469):339–42. doi: 10.1038/368339a0
21. Diehl S, Chow CW, Weiss L, Palmetshofer A, Twardzik T, Rounds L, et al. Induction of NFATc2 expression by interleukin 6 promotes T helper type 2 differentiation. J Exp Med (2002) 196(1):39–49. doi: 10.1084/jem.20020026
22. Weinstein JS, Herman EI, Lainez B, Licona-Limon P, Esplugues E, Flavell R, et al. TFH cells progressively differentiate to regulate the germinal center response. Nat Immunol (2016) 17(10):1197–205. doi: 10.1038/ni.3554
23. Castellino F, Germain RN. Chemokine-guided CD4+ T cell help enhances generation of IL-6RalphahighIL-7Ralpha high prememory CD8+ T cells. J Immunol (2007) 178(2):778–87. doi: 10.4049/jimmunol.178.2.778
24. Terebuh PD, Otterness IG, Strieter RM, Lincoln PM, Danforth JM, Kunkel SL, et al. Biologic and immunohistochemical analysis of interleukin-6 expression in vivo. constitutive and induced expression in murine polymorphonuclear and mononuclear phagocytes. Am J Pathol (1992) 140(3):649–57.
25. Gabay C, Kushner I. Acute-phase proteins and other systemic responses to inflammation. N Engl J Med (1999) 340(6):448–54. doi: 10.1056/nejm199902113400607
26. Schmidt-Arras D, Rose-John S. IL-6 pathway in the liver: From physiopathology to therapy. J Hepatol (2016) 64(6):1403–15. doi: 10.1016/j.jhep.2016.02.004
27. Alberti L, Thomachot MC, Bachelot T, Menetrier-Caux C, Puisieux I, Blay JY. IL-6 as an intracrine growth factor for renal carcinoma cell lines. Int J Cancer (2004) 111(5):653–61. doi: 10.1002/ijc.20287
28. Ishino T, Chinzei Y, Yuda M. A plasmodium sporozoite protein with a membrane attack complex domain is required for breaching the liver sinusoidal cell layer prior to hepatocyte infection. Cell Microbiol (2005) 7(2):199–208. doi: 10.1111/j.1462-5822.2004.00447.x
29. Ozwara H, Langermans JA, Kocken CH, van der Wel A, van der Meide PH, Vervenne RA, et al. Transfected plasmodium knowlesi produces bioactive host gamma interferon: a new perspective for modulating immune responses to malaria parasites. Infect Immun (2003) 71(8):4375–81. doi: 10.1128/iai.71.8.4375-4381.2003
30. Kelemen RK, Rajakaruna H, Cockburn IA, Ganusov VV. Clustering of activated CD8 T cells around malaria-infected hepatocytes is rapid and is driven by antigen-specific cells. Front Immunol (2019) 10:2153. doi: 10.3389/fimmu.2019.02153
31. Renia L, Grillot D, Marussig M, Corradin G, Miltgen F, Lambert PH, et al. Effector functions of circumsporozoite peptide-primed CD4+ T cell clones against plasmodium yoelii liver stages. J Immunol (1993) 150(4):1471–8.
32. Jafarshad A, Dziegiel MH, Lundquist R, Nielsen LK, Singh S, Druilhe PL. A novel antibody-dependent cellular cytotoxicity mechanism involved in defense against malaria requires costimulation of monocytes FcgammaRII and FcgammaRIII. J Immunol (2007) 178(5):3099–106. doi: 10.4049/jimmunol.178.5.3099
33. Langhorne J, Ndungu FM, Sponaas AM, Marsh K. Immunity to malaria: more questions than answers. Nat Immunol (2008) 9(7):725–32. doi: 10.1038/ni.f.205
34. Nganou-Makamdop K, Ploemen I, Behet M, Van Gemert GJ, Hermsen C, Roestenberg M, et al. Reduced plasmodium berghei sporozoite liver load associates with low protective efficacy after intradermal immunization. Parasite Immunol (2012) 34(12):562–9. doi: 10.1111/pim.12000.x
35. Nganou-Makamdop K, van Gemert GJ, Arens T, Hermsen CC, Sauerwein RW. Long term protection after immunization with p. berghei sporozoites correlates with sustained IFNgamma responses of hepatic CD8+ memory T cells. PloS One (2012) 7(5):e36508. doi: 10.1371/journal.pone.0036508
36. Ishizuka AS, Lyke KE, DeZure A, Berry AA, Richie TL, Mendoza FH, et al. Protection against malaria at 1 year and immune correlates following PfSPZ vaccination. Nat Med (2016) 22(6):614–23. doi: 10.1038/nm.4110
37. Fernandez-Ruiz D, Ng WY, Holz LE, Ma JZ, Zaid A, Wong YC, et al. Liver-resident memory CD8(+) T cells form a front-line defense against malaria liver-stage infection. Immunity (2019) 51(4):780. doi: 10.1016/j.immuni.2019.09.019
38. Gola A, Silman D, Walters AA, Sridhar S, Uderhardt S, Salman AM, et al. Prime and target immunization protects against liver-stage malaria in mice. Sci Transl Med (2018) 10(460). doi: 10.1126/scitranslmed.aap9128
Keywords: IL-6, CD8 T cells, inflammation, liver, malaria, vaccine
Citation: Belhimeur S, Briquet S, Peronet R, Pham J, Commere P-H, Formaglio P, Amino R, Scherf A, Silvie O and Mecheri S (2023) Plasmodium-encoded murine IL-6 impairs liver stage infection and elicits long-lasting sterilizing immunity. Front. Immunol. 14:1143012. doi: 10.3389/fimmu.2023.1143012
Received: 12 January 2023; Accepted: 27 March 2023;
Published: 11 April 2023.
Edited by:
Manuel Elkin Patarroyo, Colombian Institute of Immunology Foundation, ColombiaReviewed by:
Irene Andia Biraro, Makerere University, UgandaJessica Molina-Franky, Beckman Research Institute, City of Hope, United States
Copyright © 2023 Belhimeur, Briquet, Peronet, Pham, Commere, Formaglio, Amino, Scherf, Silvie and Mecheri. This is an open-access article distributed under the terms of the Creative Commons Attribution License (CC BY). The use, distribution or reproduction in other forums is permitted, provided the original author(s) and the copyright owner(s) are credited and that the original publication in this journal is cited, in accordance with accepted academic practice. No use, distribution or reproduction is permitted which does not comply with these terms.
*Correspondence: Salaheddine Mecheri, c21lY2hlcmlAcGFzdGV1ci5mcg==
†ORCID: Salaheddine Mecheri, orcid.org/0000-0003-3597-8810