- 1Department of Zoology, College of Science, King Saud University, Riyadh, Saudi Arabia
- 2Department of Science and Technology, Al-Nairiyah University College, University of Hafr Al-Batin, Hafr Al-Batin, Saudi Arabia
- 3Department of Biology, College of Science, Princess Nourah bint Abdulrahman University, Riyadh, Saudi Arabia
- 4Department of Zoology and Entomology, Faculty of Science, Helwan University, Cairo, Egypt
- 5Applied Science Research Center, Applied Science Private University, Amman, Jordan
One of the most crucial approaches for treating human diseases, particularly parasite infections, is nanomedicine. One of the most significant protozoan diseases that impact farm and domestic animals is coccidiosis. While, amprolium is one of the traditional anticoccidial medication, the advent of drug-resistant strains of Eimeria necessitates the development of novel treatments. The goal of the current investigation was to determine whether biosynthesized selenium nanoparticles (Bio-SeNPs) using Azadirachta indica leaves extract might treat mice with Eimeria papillata infection in the jejunal tissue. Five groups of seven mice each were used, as follows: Group 1: Non-infected-non-treated (negative control). Group 2: Non-infected treated group with Bio-SeNPs (0.5 mg/kg of body weight). Groups 3-5 were orally inoculated with 1×103 sporulated oocysts of E. papillata. Group 3: Infected-non-treated (positive control). Group 4: Infected and treated group with Bio-SeNPs (0.5 mg/kg). Group 5: Infected and treated group with the Amprolium. Groups 4 and 5 daily received oral administration (for 5 days) of Bio-SeNPs and anticoccidial medication, respectively, after infection. Bio-SeNPs caused a considerable reduction in oocyst output in mice feces (97.21%). This was also accompanied by a significant reduction in the number of developmental parasitic stages in the jejunal tissues. Glutathione reduced (GSH), glutathione peroxidase (GPx), and superoxide dismutase (SOD) levels were dramatically reduced by the Eimeria parasite, whereas, nitric oxide (NO) and malonaldehyde (MDA) levels were markedly elevated. The amount of goblet cells and MUC2 gene expression were used as apoptotic indicators, and both were considerably downregulated by infection. However, infection markedly increased the expression of inflammatory cytokines (IL-6 and TNF-α) and the apoptotic genes (Caspase-3 and BCL2). Bio-SeNPs were administrated to mice to drastically lower body weight, oxidative stress, and inflammatory and apoptotic indicators in the jejunal tissue. Our research thus showed the involvement of Bio-SeNPs in protecting mice with E. papillata infections against jejunal damage.
Introduction
The apicomplexan parasite genus Eimeria contains several species that cause coccidiosis in a range of animals (1). The sporulated oocyst, which is the infectious stage for Eimeria species, hatches to release eight sporozoites when a new host is ingested by these oocysts (2). Animal intestinal mucosa cells were invaded by sporozoites, which quickly split to create merozoites contained in a meront, resulting in tissue destruction and a severe local and systemic inflammatory reaction (3, 4). Decreased feed intake, reduced body-weight gain, dehydration, and higher vulnerability to other diseases are all consequences of Eimeria species infection (5–7).
Anticoccidial medications have long been used in conjunction with water and food to treat coccidiosis, but these methods are no longer thought to be viable due to rising parasite resistance, high prices, and concerns about residues in animal products (8, 9). However, because they are promising sources for new anti-parasitic candidate compounds, researchers were instructed to replace this parasite management program with plant-based natural remedies (10). These substances not only attack parasites but may also protect organs in target hosts that are parasite-infected (11). Azadirachta indica, also referred to as neem, nimtree, or Indian lilac, is a plant in the family Meliaceae. It is said to have health-promoting properties since it is a rich source of both non-isoprenoids and isoprenoids (especially azadirachtin) (12). Earlier investigators have confirmed their role as antibacterial (13), antifungal (14, 15), antimalarial (16), anti-inflammatory (17, 18), antiparasitic activities (19).
Currently, new trends such as nanotechnological approaches have been innovative antiparasitic agents (20). Since particles of nanoscale size exhibit unique properties that are distinct from both isolated atoms and bulk material, nanoparticles are attractive agents for the treatment of numerous parasite diseases (21, 22). For instance, selenium at the nanoscale has several advantageous benefits on health. According to Rayman (23), selenium nanoparticles (SeNPs) have antioxidant and anti-inflammatory properties. SeNPs are also known to have anti-leishmanial (24), and anti-coccidial (25, 26, 27) properties. Additionally, potential roles for SeNPs against murine schistosomiasis (28, 4), Giardiasis (29), and murine trichinosis (30), have been reported previously.
This study was designed to examine the ameliorative effect of biosynthesized SeNPs utilizing Azadirachta indica leaf extracts against intestinal E. papillata infection in mice based on the prior biological activities of neem.
Materials and methods
Experimental animals
Prior to the experiment, 35 male C57BL/6 mice were procured from the animal facility in the College of Pharmacy at King Saud University, when they were 10-12 weeks old and weighed 20-25 g. Parasitological examinations had been performed on all experimental mice to be free from parasites. All mice were given a regular feed as well as water ad libitum. Animals were kept in plastic cages under controlled conditions of temperature (23±5°C), humidity, and 12/12 hrs light-dark cycle. The week before the experiment, the animals were acclimated.
Plant collection and extract preparation
Leaves of Azadirachta indica were gathered during summer 2022 from the botanical gardens in Riyadh, Saudi Arabia. The plant identification was verified by a taxonomist at the Department of Botany and Microbiology, College of Science (King Saud University) (KSU-10804). The A. indica leaves methanolic extract (AILE) was produced in accordance with Manikandan et al. (31).
Plant extract analysis
The concentrations of phenolic and flavonoid contents were evaluated using the Folin–Ciocalteu technique and the aluminum chloride colorimetric method as described by Dkhil et al. (32) and determined as mg gallic acid/g dry leaves and mg Quercetin/g dry leaves, respectively.
Synthesis of selenium nanoparticles
According to Vahdati and Moghadam (33), SeNPs were prepared by reducing sodium selenite (Nanocs Inc., Boston, MA, USA). Using a methanolic extract of A. indica in 9:1 ratio, an aqueous solution of Bio-SeNPs was prepared, warmed at 45 °C for 15 min, and then allowed to cool at 23±2 °C. Using a scanning electron microscope (SEM) (JSM-6060LV, JEOL) at an accelerating voltage of 15 kV, the size and morphology of the Bio-SeNPs were examined. Synthesis of Bio-SeNPs was confirmed by sampling the reaction mixture at regular intervals and the absorption maxima were scanned by UV-3600 Shimadzu spectrophotometer.
Infection and treatment
This study used the coccidian parasite, Eimeria papillata, as a model parasite. Eimeria parasite oocysts were passaged in laboratory mice (Mus musculus). Unsporulated oocysts were collected from feces and allowed to sporulate in a solution containing 2.5% (w/v) potassium dichromate. They were then washed in phosphate buffer solution to prepare them for the remainder of the experiment.
Five groups of seven mice each were used, as follows: Group 1: Non-infected-non-treated (negative control). Group 2: Non-infected treated group with Bio-SeNPs (0.5 mg/kg of body weight). Groups 3-5 were orally inoculated with 1×103 sporulated oocysts of E. papillata, according to Abdel-Tawab et al. (34). Group 3: Infected-non-treated (positive control). Group 4: Infected and treated group with Bio-SeNPs (0.5 mg/kg) (26). Group 5: Infected and treated group with the Amprolium (120 mg/kg body weight) (35). Groups 4 and 5 daily received oral administration (for 5 days) of Bio-SeNPs and anticoccidial medication, respectively, after 60 min of infection.
The Bio-SeNPs dose was chosen based on our preliminary experiment for determining the best dose inhibiting oocyst shed in mouse faeces (see Figure S1), as well as our previous work (26).
Weight change was assessed on day 0 and day 5 of the experiment, according to Al-Quraishy et al. (36). Fecal pellets from each mouse in the 3rd, 4th, and 5th groups were collected on the 5th day post-infection (p.i.), and the total number of shed oocysts was determined in accordance with Schito et al. (37). All the mice were sacrificed, and the jejuna were harvested and kept for use in the experiment’s subsequent stages.
Parasitic number and goblet cells
According to Adam and Caihak (38), portions of jejuna were fixed in 10% formalin for 24 hr, dehydrated and embedded in paraffin wax to be used for histological examinations. Hematoxylin-eosin (H&E) and Alcian blue staining of jejuna sections were employed to identify the parasite stages and goblet cells, respectively. Under an Olympus B×61 microscope (Tokyo, Japan), all slides were examined, photographed, and the number of parasitic stages and goblet cells were counted on at least ten well-orientated villous-crypt units (VCU).
Immunohistochemistry
Jejunal sections were deparaffinized, treated with 3% H2O2 for 10 min, blocked with normal serum (5%), and then incubated overnight at 4 °C with a primary polyclonal rabbit anti-mouse antibody specific for cysteine aspartic acid protease-3 (Caspase-3) (1:100 dilution in PBS, Santa Cruz Biotechnology, CA, USA) (39). Samples were treated with a biotin-conjugated secondary antibody (1:2,000 dilution in PBS) after three PBS washes. The sections were counterstained with hematoxylin and re-incubated with streptavidin that was labeled with horseradish peroxidase and then examined and photographed using an Olympus B×61 microscope (Tokyo, Japan).
Oxidative damage in mice jejunum
According to Tsakiris et al. (40), pieces of mice jejuna from all experimental groups were weighed and homogenized to produce 10% (w/v) homogenate in an ice-cold solution containing 300 mM sucrose and 50 mM Tris(hydroxymethyl)aminomethane-HCl (Sigma, St Louis, MO, USA). The homogenate was centrifuged at 500×g at 4 °C for 10 min. The supernatant was separated and used in a variety of biochemical analyses. According to the technique used by Beutler et al. (41), the concentrations of reduced glutathione (GSH) were calculated using the absorbance measurement at 405 nm and expressed as mg/g. The level of glutathione peroxidase (GPx) was assessed using the Pagila and Valentine (42) method, which reported values as U/g. Lipid peroxide by-product as malondialdehyde (MDA) contents (43) were estimated in the supernatant of jejunum homogenate at 534 nm and represented as nmol/g. The Montgomery and Dymock (44) procedure were used to measure the level of nitric oxide (NO), which was then represented as µmol/L. The quantity of superoxide dismutase (SOD) was quantified at 560 nm and displayed as U/g (45). All these parameters were measured using the proper kits (Biodiagnostic Co., Egypt), which were supported by the software of SoftMax® Pro v. 6.3.1. The molecular device used for the analysis was the Spectra MAX 190.
Gene expression analysis
Using Trizol (Invitrogen), total RNA was extracted from the preserved jejunal samples RNA later. Following the manufacturer’s instructions, RNA samples were digested with DNase (Applied Biosystems, Darmstadt, Germany) for at least 1 hr before being transformed into cDNA using the reverse transcription kit (Qiagen, Hilden, Germany). Using the suggested procedure, the QuantiTectTM SYBR® Green PCR kit (Qiagen, Hilden, Germany) was used to carry out the Real-time polymerase chain reaction (qRT-PCR). TaqMan 7500 system software v.1.2.3f2 (Applied Biosystems) was used to do relative quantitative evaluation of the amplification data. Using primers, the genes encoding the mRNAs for mucin (MUC2), caspase-3 (Casp3), B cell leukemia/lymphoma 2 (BCL2), interleukin-6 (IL-6), and tumor necrosis factor-alpha (TNF-α) were examined (see Supplementary Table S1). The PCR procedures followed those formerly outlined by Dkhil et al. (19). The Ct (2−ΔΔCT) method described by Livak and Schmittgen (46) was used to evaluate the differences between the mean of the gene expression and the reference gene was glyceraldehyde-3-phosphate dehydrogenase (GAPDH).
Statistical analysis
We used one-way ANOVA to compare multiple variable comparisons, and the data is presented as the mean and standard deviation. We used Duncan’s test to compare the significance of the classes. The statistical significance level was set at p ≤ 0.05.
Results
Total phenolic and flavonoid contents
The total concentration of phenolics and flavonoids in the investigated plant extract was found to be 108.76 ± 1.64 mg gallic acid/g dry leaves and 165.4 ± 2.25 mg quercetin/g dry leaves, respectively (Figure 1).
Characterization of Bio-SeNPs
The SEM image (Figure 2A) revealed that the majority of the Bio-SeNPS has a spherical shape, where the size ranged from 62.1 to 77.6 nm with an average diameter of ∼67.97 nm. UV-visible absorption spectra of Bio-SeNPs showed that after 5 min of a reduction reaction had only one absorption peak at 308 nm (Figure 2B).
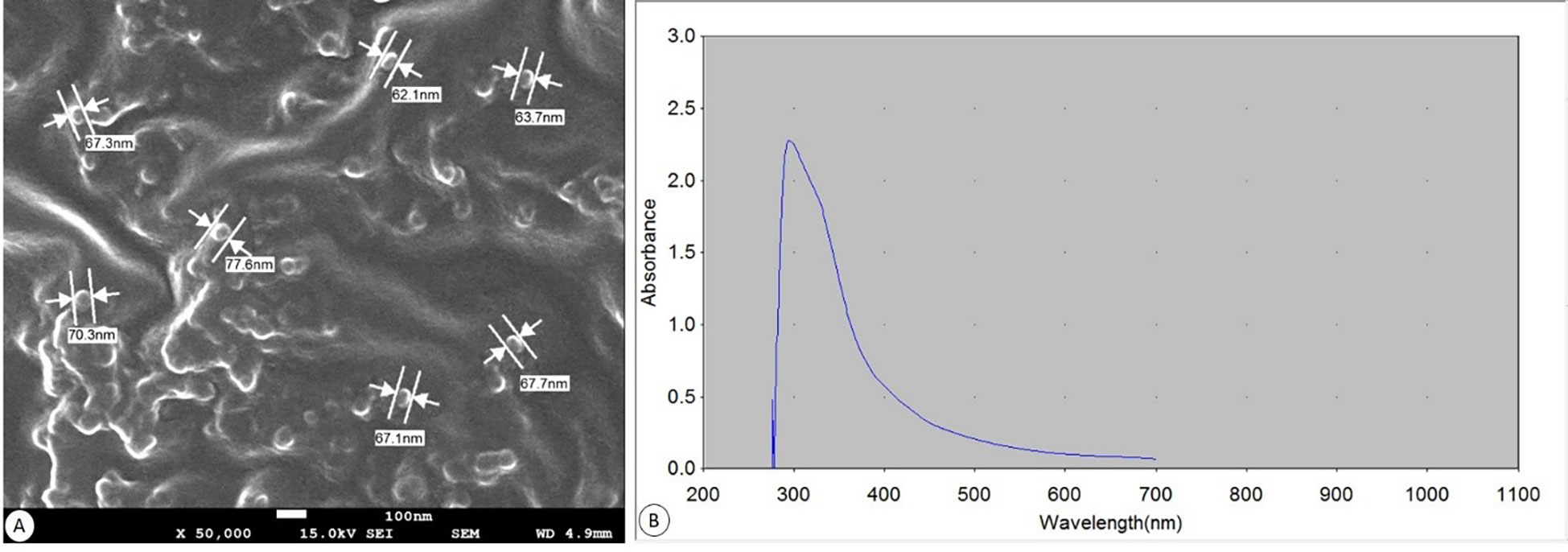
Figure 2 Biosynthesized SeNPs. (A) SEM at a magnification of ×50.000 (scale bar= 100 nm). (B) UV spectroscopic graph showing absorbance.
Body weight change
The non infected non treated control group grew normally resulting in an average weight gain of about 3.92% (Figure 3). Whereas, the experimentally infected group with E. papillata had lost their original weight by about 6.07%. After treatment with Bio-SeNPs, the loss of the original weight was reduced to -2.39% (Figure 3).
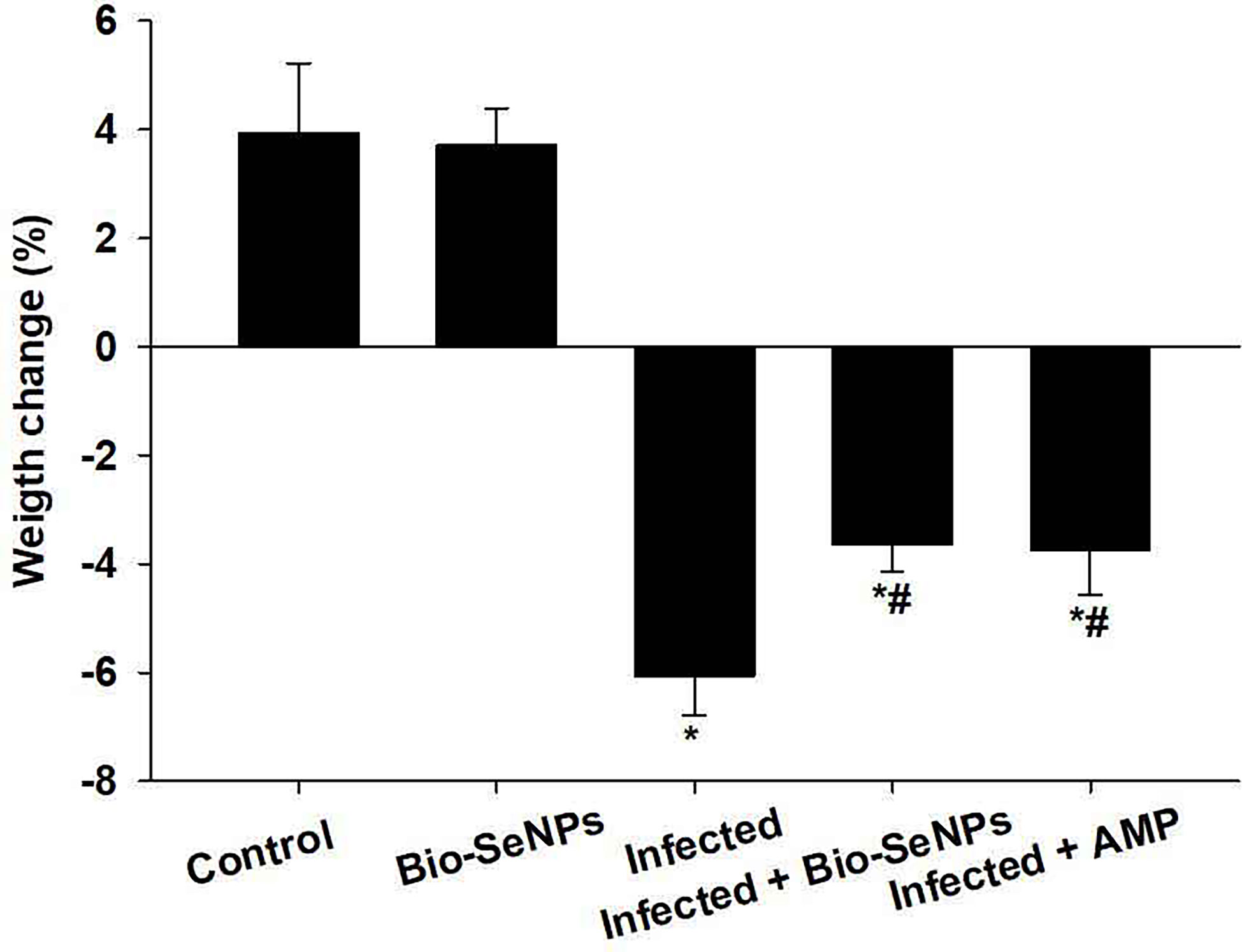
Figure 3 Bio-SeNPs improved weight loss due to infection with Eimeria papillata in mice. Values are means ± SEM. * significance (P ≤ 0.05) against non-infected control group, # significance (P ≤ 0.05) against infected group.
Oocysts output
After 3 days following infection, all mice inoculated with 1×103 sporulated E. papillata oocysts began excreting the oocysts with feces. The oocysts’ output varied between the infected-treated and infected-non treated groups on day 5 post-infection (p.i.). In the infected group, there were around 5.130 × 106 ± 0.38 × 106 oocysts shedding per g of feces. On day 5 p.i., the Bio-SeNPs treatment significantly reduced the shedding rate, which was 0.148 × 106 ± 0.87 × 105 oocysts/g feces (∼ 97.21%) compared to 0.138 × 106 ± 0.52 × 105 oocysts/g feces with the reference anticoccidial drug.
Histological and immunohistochemical studies
Meronts, microgamonts, macrogamonts, and developing oocysts were developed in the epithelial cells of the jejunum in experimentally infected of mice with E. papillata oocysts, as observed in the hematoxylin-eosin-stained sections (Figure 4). In the jejunal villi of the infected mice, these stages were counted per 10 VCUs as 96.83 ± 13.19 meronts, 23.01 ± 5.40 gamonts, and 15.52 ± 1.08 developing oocysts (Figure 5). Remarkably, the number of meronts and male and female gamonts were significantly reduced following treatment with Bio-SeNPs by 14.86 ± 2.38 and 10.83 ± 2.04, respectively, compared to the reference drug’s values of 31.63 ± 7.02 and 12.22 ± 2.54 (Figure 5).
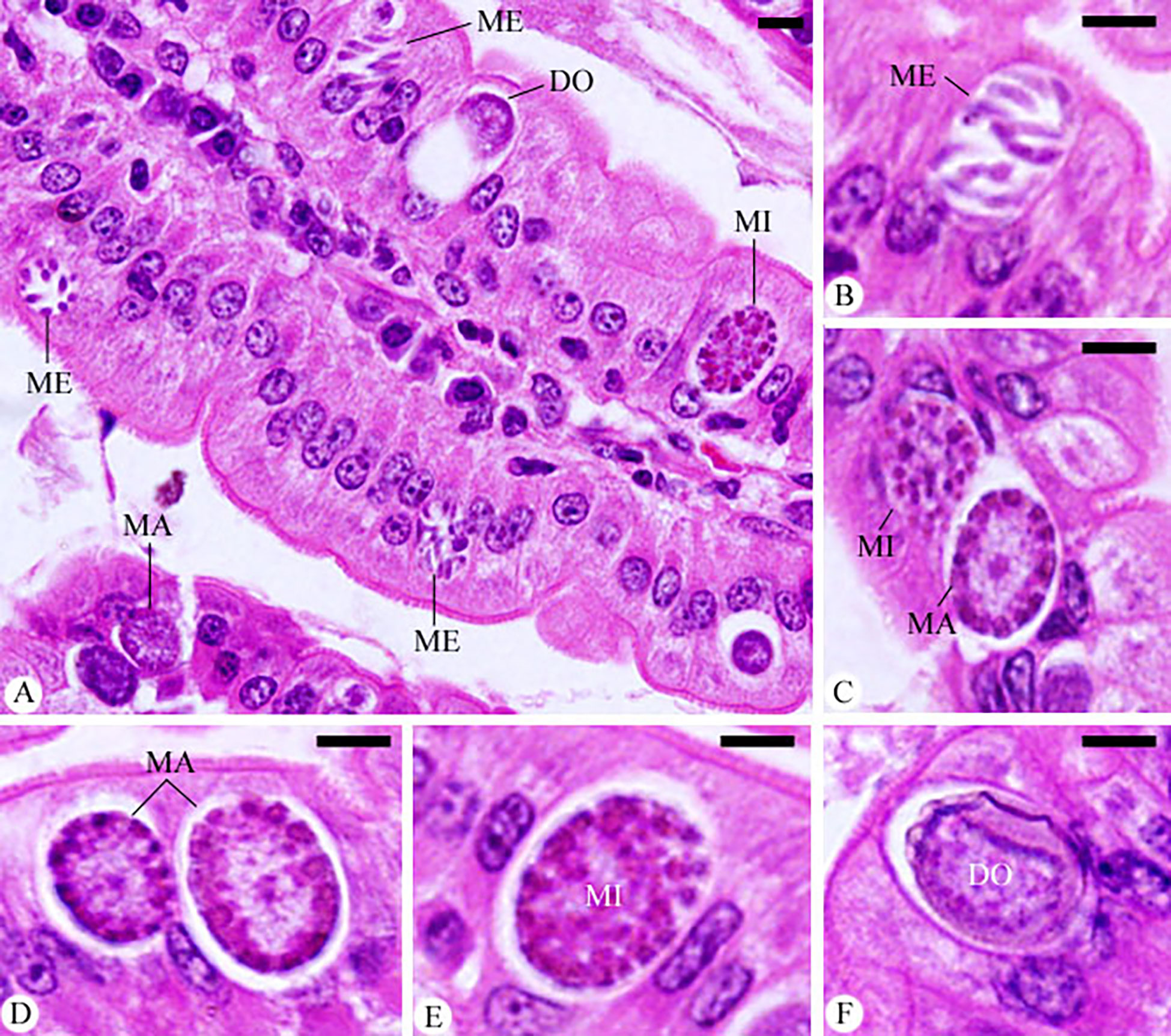
Figure 4 Infected jejunum with Eimeria papillata on day 5 p.i. (A) different developmental stages. (B) meronts (ME). (C) macrogamont (MA) and microgamont (MI). (D) macrogamont (MA). (E) microgamont (MI). (F) developing oocyst (DO). Sections were stained with hematoxylin and eosin (H&E). Scale bar= 25µm.
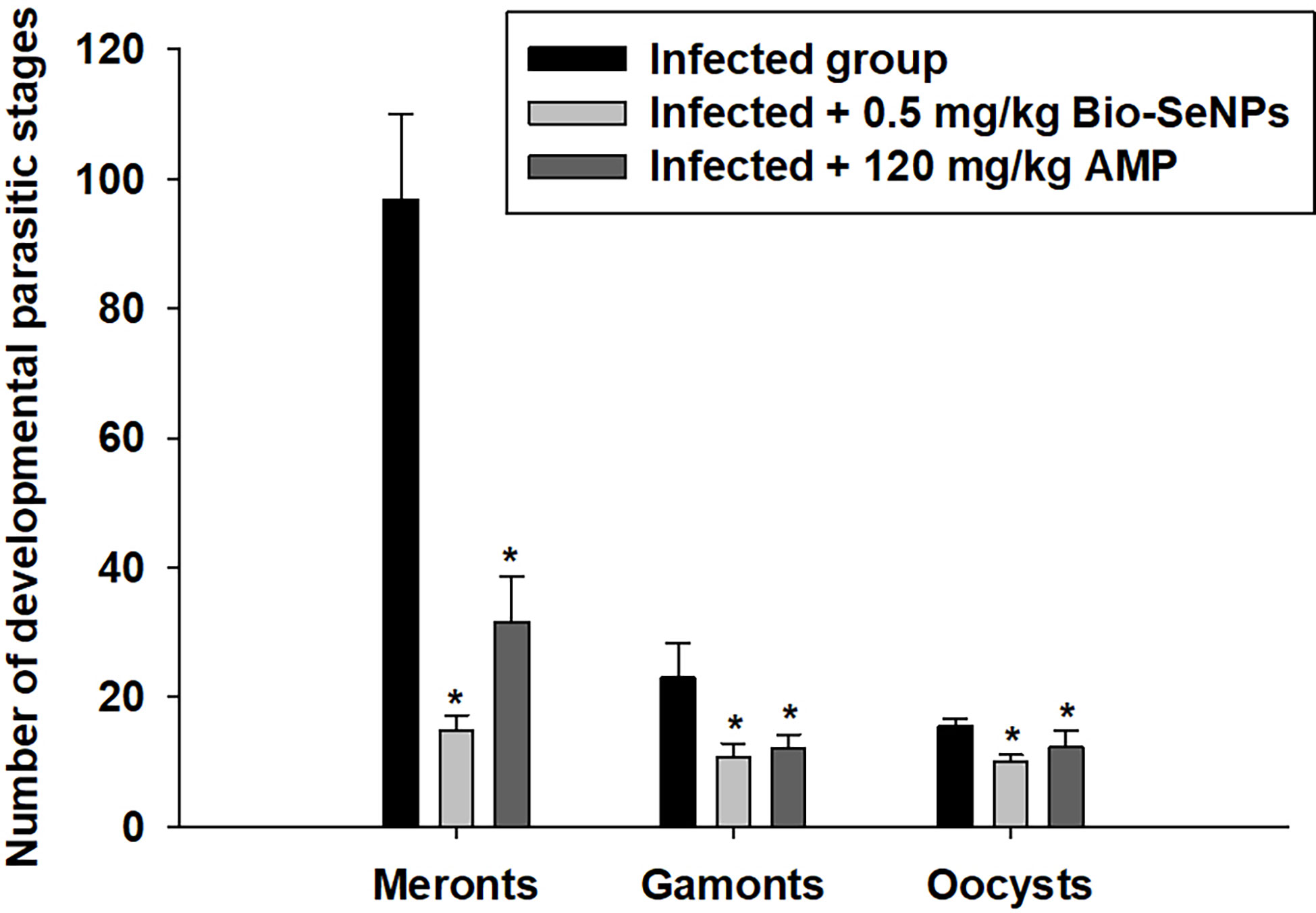
Figure 5 The number of developmental parasitic stages of Eimeria papillata in jejunum among the infected and treated groups. All values are means ± SD. * significance (P ≤ 0.05) between the infected and treated groups.
Microscopic analysis of jejunal sections stained with alcian blue (Figure 6) showed that mice infected with E. papillata had significantly fewer goblet cells (5.75 ± 0.22/VCU) than the non-infected group (9.44 ± 0.75/VCU) (Figure 7). The number of goblet cells was significantly higher in mice treated with Bio-SeNPs (7.13 ± 0.83/VCU) than in mice treated with a reference drug (6.40 ± 1.32/VCU) (Figure 7).
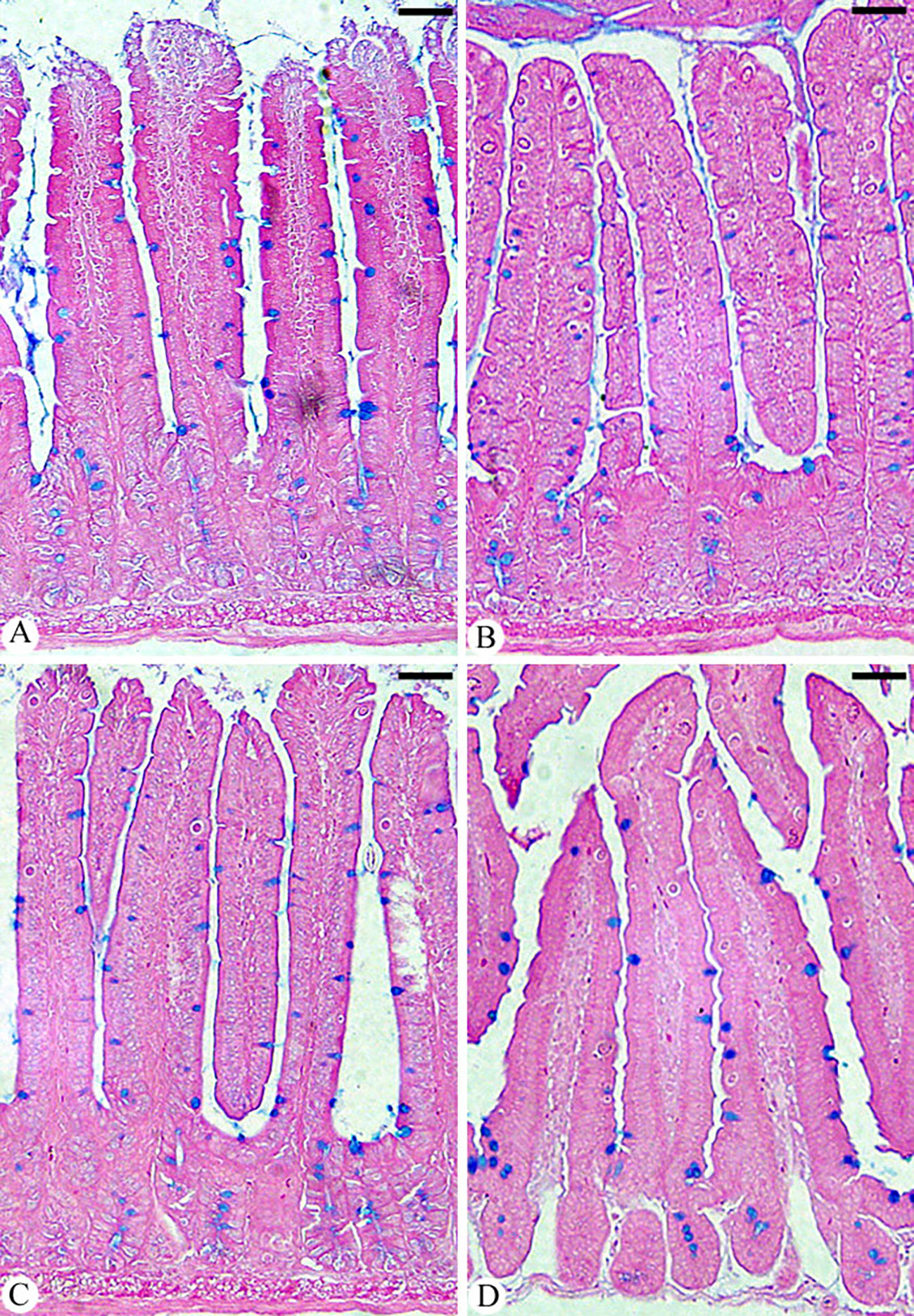
Figure 6 Jejunum sections showing goblet cells in (A) control group, (B) infected group, (C) infected group treated with Bio-SeNPs, and (D) infected group treated with AMP. Sections were stained with alcian blue and counted with eosin goblet cells in 10 well-oriented villus-crypt units (VCU). Scale bar = 100µm.
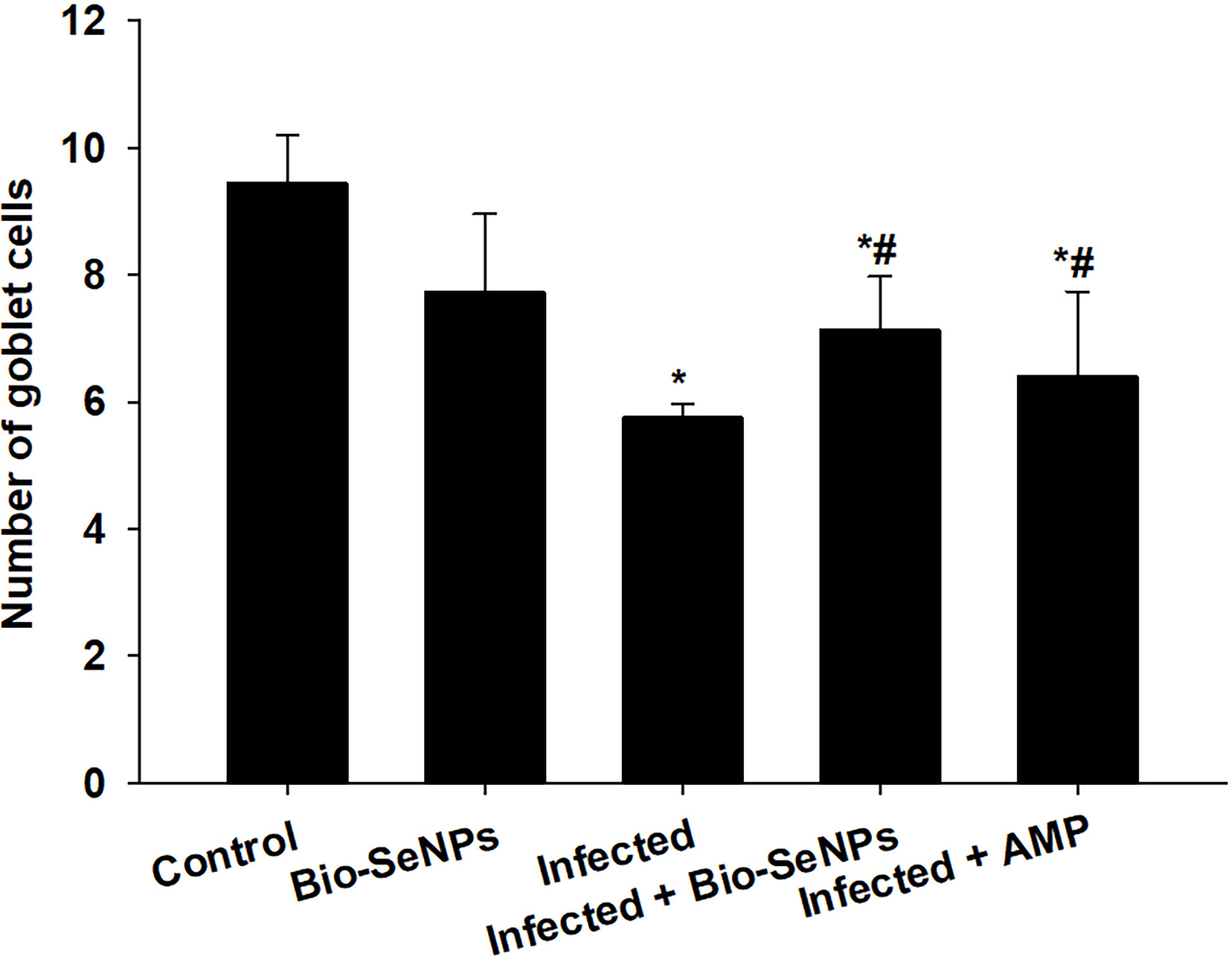
Figure 7 Changes in the number of jejunal goblet cells in the control group, infected group, treated group with Bio-SeNPs and the infected group treated with Bio-SeNPs and AMP. Values are means ± SEM. * significant change concerning the control group, # significant change concerning the infected group.
When mice were infected with E. papillata, jejunal sections from the control, infected and treated groups were stained for caspase-3 expression. This showed that the infection caused a qualitative elevation of the caspase-3 expression level compared to the non-infected group (Figure 8). After Bio-SeNPs treatment, the expression of caspase-3 significantly changed in contrast to the infected groups (Figure 8).
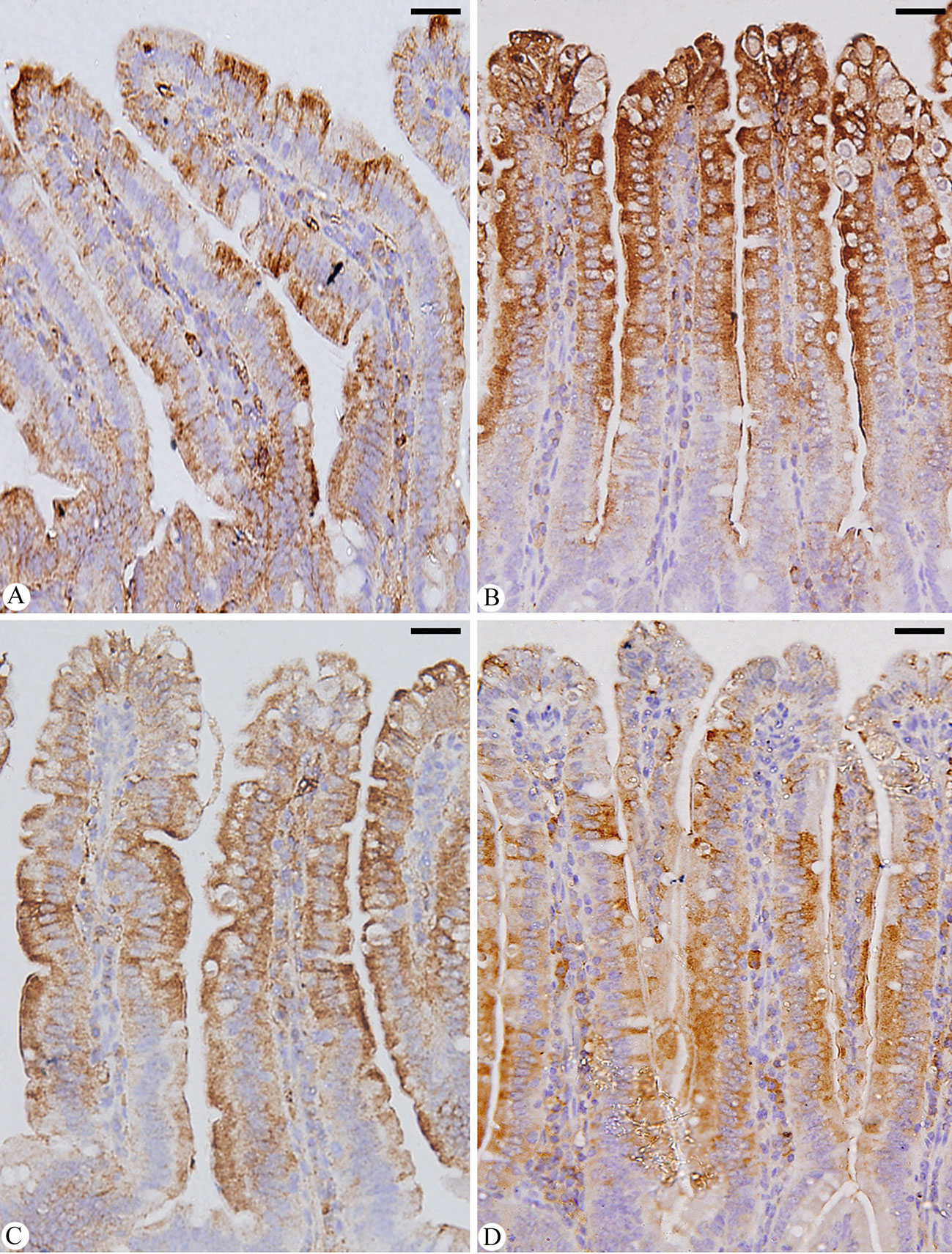
Figure 8 Immunohistochemical localization of CASP-3 in the jejuna of mice. (A) control non-infected jejunum. (B) E. papillata infected jejunum with an increased number of CASP-3 positive cells. (C, D) infected treated mouse (Bio-SeNPs and AMP, respectively) with decreased number of CASP-3 positive cells. Scale bar = 100µm.
Effect of Bio-SeNPs on oxidative stress in jejunal mice
In the infected group, the level of GSH significantly decreased from 61.37 ± 2.93 in the control group to 34.16 ± 3.49 mg/g. In contrast, mice treated with Bio-SeNPs and reference drug had increased GSH levels, reaching 59.84 ± 4.76 and 58.70 ± 5.84 mg/g, respectively (Table 1). Additionally, the level of GPx significantly decreased in the infected group from 29.82 ± 6.08 in the control group to 13.29 ± 2.49 U/g. As opposed to this, mice treated with Bio-SeNPs and reference drug had increased in their GPx levels of 21.07 ± 1.37 and 20.42 ± 1.28 U/g, respectively (Table 1).
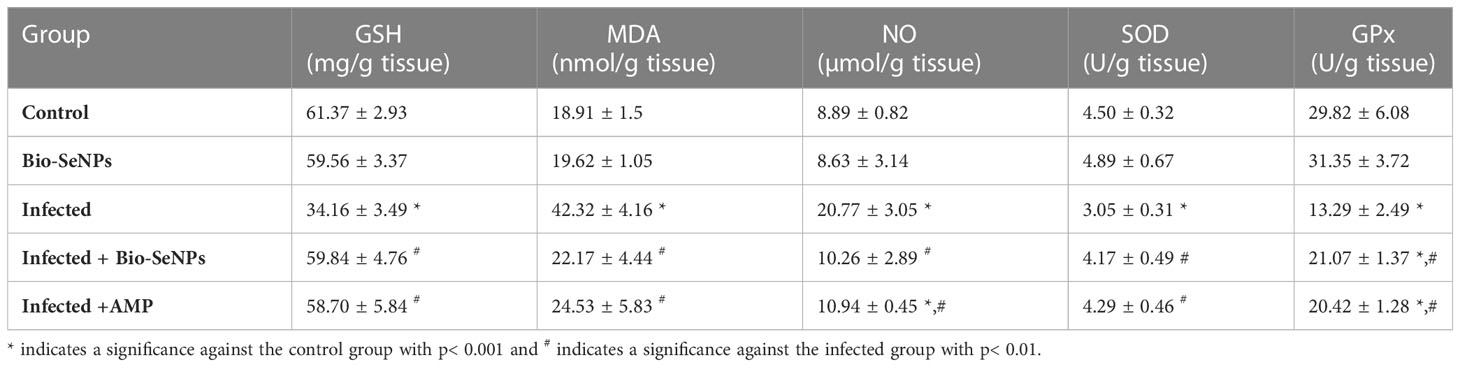
Table 1 Effect of Bio-SeNPs on the value of glutathione reduced (GSH), malondialdehyde (MDA), nitric oxide (NO), superoxide dismutase, and glutathione peroxidase (GPx) of mice infected with E. papillata.
Compared to 8.89 ± 0.82 µmol/g in the control group, the E. papillata infection caused a highly significant increase in jejunal NO to 20.77 ± 3.05. However, NO levels were significantly reduced after treatment with a dosage of Bio-SeNPs and reference drug, reduced to 10.26 ± 2.89 and 10.94 ± 0.45 µmol/g, respectively (Table 1). The MDA level was significantly increased in the infected group, going from 18.91 ± 1.59 in the control group to 42.32 ± 4.16 nmol/g. In contrast, the MDA levels of mice treated with Bio-SeNPs and reference drug were significantly reduced to 22.17 ± 4.44 and 24.53 ± 5.83 nmol/g, respectively (Table 1).
In the infected group, the SOD level significantly decreased from 4.50 ± 0.32 in the control group to 3.05 ± 0.31 U/g. In contrast, mice treated with Bio-SeNPs and reference drug had significantly lower levels of SOD, measuring 4.17 ± 0.49 and 4.29 ± 0.46 U/g, respectively (Table 1).
Effect of Bio-SeNPs on mRNA expression
The MUC2 gene’s expression level was downregulated because of Eimeria papillata infection, according to qRT-PCR (Figure 9). However, using Bio-SeNPs significantly upregulated the expression of the MUC2 gene from 0.60 to 1.50 fold (Figure 9).
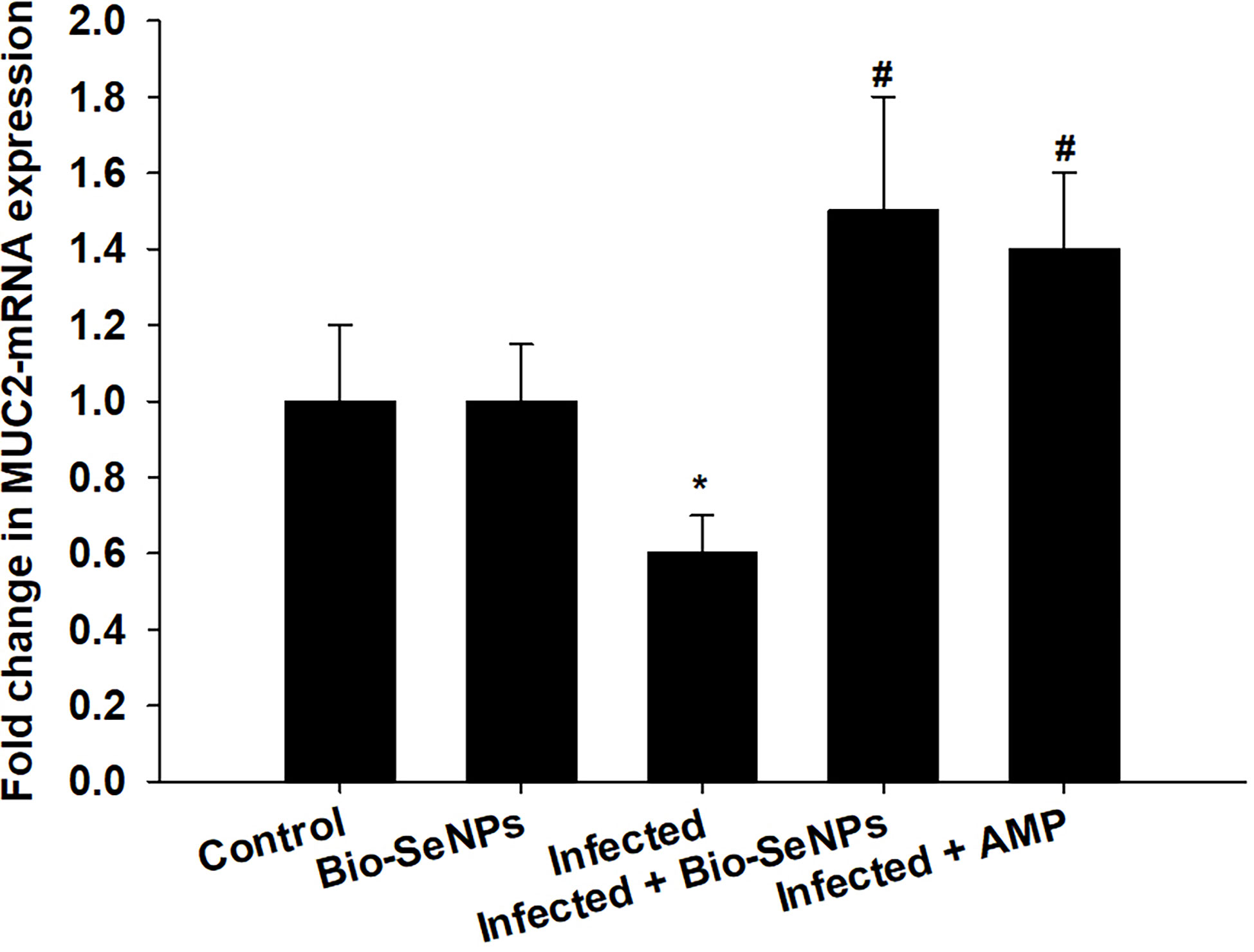
Figure 9 Effect of Bio-SeNPs on the mRNA expression of MUC2 in the jejunal samples from E. papillata-infected mice. The expression values obtained by RT-PCR analysis were normalized to the reference gene GAPDH mRNA level and are shown as fold induction (in log 2 scale) relative to the mRNA level in the control. * significance changes concerning the control group, # significance changes concerning the infected group.
BCL2 and Caspase-3 gene expression were upregulated because of the infection induced by E. papillata (Figure 10). After Bio-SeNPs treatment, caspase-3 gene expression was downregulated significantly from 3.60 to 2.10 fold, while, BCL2 gene expression was downregulated from 7.0 to 3.0 fold (Figure 10).
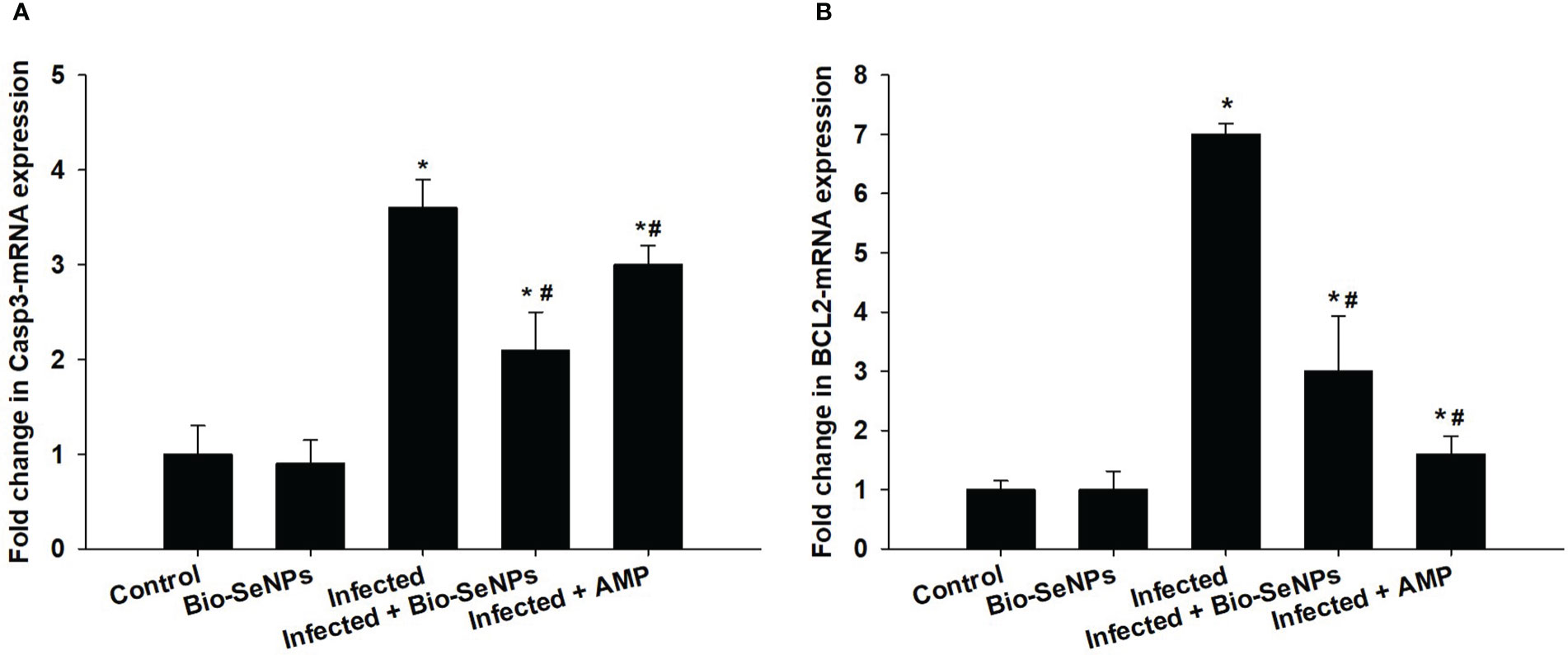
Figure 10 Effect of Bio-SeNPs on the mRNA expression of Caspase-3 (A) and BCL2 (B) in the jejunal samples from E. papillata-infected mice. The expression values obtained by RT-PCR analysis were normalized to the reference gene GAPDH mRNA level and are shown as fold induction (in log 2 scale) relative to the mRNA level in the control. * significance changes concerning the control group, # significance changes concerning the infected group.
Additionally, the expression of the IL-6 and TNF-α was upregulation because of E. papillata infection (Figure 11). When compared to the non-infected control group, this increase in mRNA expression of these genes was about 8.10 and 8.50, respectively (Figure 11). Treatment with Bio-SeNPs resulted in a 3.0 and 4.50 fold, respectively, with a significant downregulation of IL-6 and TNF-α (Figure 11).
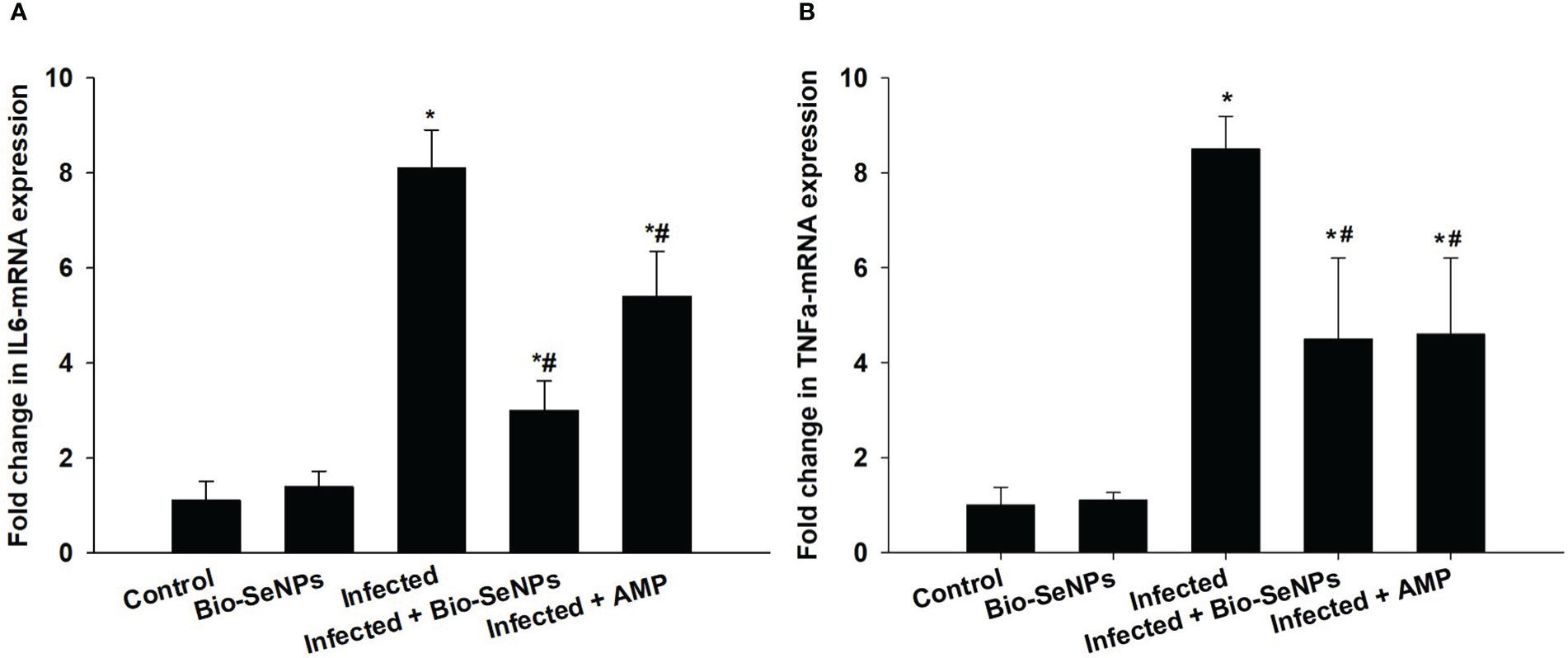
Figure 11 Effect of Bio-SeNPs on the mRNA expression of IL-6 (A) and TNF-α (B) in the jejunal samples from E. papillata-infected mice. The expression values obtained by RT-PCR analysis were normalized to the reference gene GAPDH mRNA level and are shown as fold induction (in log 2 scale) relative to the mRNA level in the control. * significance changes concerning the control group, # significance changes concerning the infected group.
Discussion
More than 30 sulfanilamide drugs have been used for the prevention and treatment of coccidiosis since the 1940s. These anticoccidial agents have an effect on Eimeria species’ cofactor synthesis, mitochondrial functions, or cell membrane function (47). The need for innovative anti-Eimeria medications is justified by growing worries about parasite resistance, consumer health, and the environmental safety of commercial drugs (11). Nanopartic les now open new opportunities for novel medical therapies to combat parasite infection (26, 48). For instance, nano-selenium (SeNPs) was used against leishmaniasis (24), schistosomiasis (28), giardiasis (29), and murine trichinosis (30). For instance, selenium at the nanoscale has several advantageous benefits on health. According to Rayman (23), SeNPs have antioxidant and anti-inflammatory properties. SeNPs are also known to have anti-leishmanial (24), and anti-coccidial (25, 26, 27) effects. They have demonstrated antimicrobial, antiviral, and anti-parasitic properties (27, 49, 50). This study investigates the potential anti-coccidiosis effects of Bio-SeNPs utilizing Azadirachta indica leaves extract, which is likely due to the extract’s composition and SeNPs’ unique properties.
In addition to having a decreasing effect on the intracellular stages of E. papillata in the jejunum, the Bio-SeNPs were an efficient agent in reducing the total number of shed oocysts on the 5th-day after infection in mice by around 97.21%. Most anti-coccidial therapies are known to cause this suppression of intracellular Eimeria stages and reducing influence on oocyst shedding rate. High levels of polyphenolic compounds found in the plant extract (51, 52) may be responsible for the anticoccidial properties. These compounds have a potent antimicrobial effect by interfering with microbial cell walls and membranes, altering their permeability for cations and water, which causes impaired membrane functions and leakage of cellular constituents and finally to cell death (53–56).
Our finding showed that E. papillata infection led to weight loss. Previous studies attributed this loss brought on by the consumption of the parasitic Eimeria stages to the intestinal epithelial carbohydrate content and the severe structural alterations that resulted (57–59). Due to the presence of bioactive compounds in the plant extract, which helped to enhance the jejunal histological architecture and restore the mice’s nutritional condition, a considerable improvement in body weight was observed after treatment.
A strong inflammatory response and oxidative damage to the mice jejunum are linked to E. papillata infection. Bio-SeNPs treatment of the infected animals demonstrated an excellent modulation of the oxidative damage of mice jejunum tissue. The strong potential effect of Bio-SeNPs is due to the antioxidant (25, 27, 60–63) and anti-inflammatory (19, 26, 64–67) activities of both SeNPs and components of the plant extract.
An imbalance between endogenous antioxidant defense and free radical production is brought on by the coccidial infection (68, 69). Our findings showed that E. papillata infection is associated with oxidative damage to the mice jejunum, which causes the antioxidant enzymes to be depleted and GSH, GPx, and SOD levels to be reduced. These oxidative parameters are crucial for shielding the animal body from free radical damage during Eimeria infection. According to Georgieva et al. (68)Dkhil et al. (, 70), and Elmahallawy et al. (71), antioxidant enzymes use nanoparticles active sites to detoxify the accumulation of the reactive oxygen species that causes destructive changes in the jejunum epithelia. Bio-SeNPs significantly prevent the infection-induced loss of these markers and upregulated their activity, which is typically decreased during oxidative damage induced by infection.
The synthesis of several carbonyl compounds, such as MDA, results from the oxidation of lipid peroxides (11, 25, 72–74). MDA levels decrease following treatment with SeNPs or neem leaf extract. Al-Quraishy et al. (36) reported that NO level upregulated as an immunological response to the presence of the infective sporozoite stages that invade the jejunal epithelial cells, causing inflammation. Furthermore, the unbalanced status led to the elevation of nitric oxide levels because of infection, which is consistent with Dominquez et al. (75). By increasing NO levels, the Bio-SeNPs could dramatically reduce the severity of the infection in the jejunum. This illustrates the potential antioxidant function of Bio-SeNPs. According to prior investigations, active compounds such as flavonoids and other derivatives were present in SeNPs and plant extracts (14, 76–78). In this study, the biological function of flavonoids is indicated by their presence in Bio-SeNPs.
One of the major intestinal immune-competent cells, goblet cells secrete mucus, which is regarded as a protective barrier (79). Multipotential stem cells can be detected in the jejunal villi. The reduced number of goblet cells could be a result of the parasite causing damage to the multipotent stem cell population at the base of the Lieberkühn crypts (80). This study demonstrated how the Eimeria-induced infection decreased the number of goblet cells in the mice jejunum. According to Yunus et al. (81), the alteration in goblet cells may affect how susceptible the Eimeria-infected host is to be limiting the parasite’s ability to grow or penetrate the local epithelium. Through the restoring functions of stem cells, Bio-SeNPs were able to increase the number of jejunal goblet cells infected with E. papillata. Additionally, goblet cells are primarily in charge of producing and maintaining the mucus barrier through the synthesis of mucin and are greatly influenced by interactions with the immune system (82, 83). This study demonstrated how E. papillata infection decreased the MUC2 gene’s expression level. According to this study by Boltin et al. (84), the MUC2 is one of the primary secreted, gel-forming mucin components that helps the mucus barrier form. Treatment with Bio-SeNPs significantly increased the expression of the MUC2 gene, supporting the findings of Gum et al. (85) and Larsson et al. (86), which suggested that an improvement in goblet cell activity may influence the regulation of mucin production.
A considerable elevation in IL-6 and TNF-α indicated the mice’s evident systemic inflammatory response to the E. papillata-induced infection. Pro-inflammatory cytokines including IL-6 and TNF-α have been found to control mucin production, according to Enss et al. (87). In contrast to the current study, cytokine inflow and mucin glycosylation downregulation were brought on by the Eimeria parasite infection. According to Hsu et al. (88), this negative association is frequently linked to a decrease in goblet cells, a thickening of the mucus, and the rate at which mucin is transported from the Golgi to secretory vesicles. Bio-SeNPs therapy dramatically reduced the systemic inflammatory response in infected mice. Our findings demonstrated that Bio-SeNPs protect host tissues by acting as anti-inflammatory agents in addition to targeting Eimeria stages within infected tissues. According to Oke et al. (52) and Abdel-Moneim et al. (63), polyphenolic chemicals may be responsible for this anti-inflammatory effect. Previous research demonstrated the immunomodulatory and anti-inflammatory properties of flavonoids as well as their capacity to reduce the production of pro-inflammatory cytokines (89, 90). Apoptosis may control the host’s response to certain intracellular parasitic diseases and aid in the removal of damaged or infected cells (91, 92). The pro-apoptotic genes BCL2 and caspase-3 significantly upregulated in this study, indicating the death of mouse jejunal cells. Previous investigations found an increase in the quantity of apoptotic cells in the jejuna of mice infected with E. papillata (6, 93). The eimeriosis-induced apoptotic alterations in jejunal cells might be improved by the Bio-SeNPs. Previous studies by Alkhudhayri et al. (27)Schumacher et al. (, 94), and Mehanna et al. (95) all reported SeNPs’ anti-apoptotic action.
Conclusion
The combined effects of Bio-SeNPs against E. papillata-induced infection in C57BL/6 male mice result in considerable anticoccidial activity, a significant improvement in the histological changes of the mice jejunum, antioxidant status, anti-apoptotic, and anti-inflammatory activities. To understand the mechanism of the Bio-SeNPs’ action on both the parasite and the host mice, more research is necessary.
Data availability statement
The original contributions presented in the study are included in the article/Supplementary Materials, further inquiries can be directed to the corresponding author/s.
Ethics statement
This research was approved by the Research Ethics Committee (REC) at King Saud University (approval number KSU-SE-22-66).
Author contributions
RA, SA and MAD designed the study. RA, MA, TA, GA, EMA, OBM, MFE, SA and MAD carried out the experiments and analyzed the data. All authors contributed to the article and approved the submitted version.
Funding
This study was supported by the Researchers Supporting Project (RSP2023R25), King Saud University, Riyadh, Saudi Arabia, and was supported by Princess Nourah bint Abdulrahman University Researchers Supporting Project number (PNURSP2023R23), Princess Nourah bint Abdulrahman University, Riyadh, Saudi Arabia.
Conflict of interest
The authors declare that the research was conducted in the absence of any commercial or financial relationships that could be construed as a potential conflict of interest.
Publisher’s note
All claims expressed in this article are solely those of the authors and do not necessarily represent those of their affiliated organizations, or those of the publisher, the editors and the reviewers. Any product that may be evaluated in this article, or claim that may be made by its manufacturer, is not guaranteed or endorsed by the publisher.
Supplementary material
The Supplementary Material for this article can be found online at: https://www.frontiersin.org/articles/10.3389/fimmu.2023.1139899/full#supplementary-material
Supplementary Figure 1 | Oocyst output in mice infected with Eimeria papillata and for infected treated groups with 0.25 mg/kg, 0.5 mg/kg, 1 mg/kg Bio-SeNPs, and 120 mg/kg amprolium (AMP) on day 5 p.i. All values are means ± SD. * significance (P ≤ 0.05) between infected and treated groups.
References
2. Burrell A, Tomley FM, Vaughan S, Marugan-Hernandez V. Life cycle stages, specific organelles and invasion mechanisms of eimeria species. Parasitology (2019) 147(3):263–78. doi: 10.1017/S0031182019001562
3. Allen PC, Fetterer RH. Recent advances in biology and immunobiology of eimeria species and in diagnosis and control of infection with these coccidian parasites of poultry. Clin Microbiol Rev (2002) 15(1):58–65. doi: 10.1128/CMR.15.1.58-65.2002
4. Dkhil MA, Khalil MF, Diab MSM, Bauomy AA, Santourlidis S, Al-Shaebi EM, et al. Evaluation of nanoselenium and nanogold activities against murine intestinal schistosomiasis. Saudi J Biol Sci (2019) 26:1468–72. doi: 10.1016/j.sjbs.2018.02.008
5. Gres V, Voza T, Chabaud A, Landau I. Coccidiosis of the wild rabbit (Oryctolagus cuniculus) in France. Parasite (2003) 10(1):51–7. doi: 10.1051/parasite/2003101p51
6. Metwaly MS, Dkhil MA, Al-Quraishy S. Anti-coccidial and anti-apoptotic activities of palm pollen grains on eimeria papillata-induced infection in mice. Biologia (2014) 69(2):254–9. doi: 10.2478/s11756-013-0297-9
7. Amer OS, Dkhil MA, Hikal WM, Al-Quraishy S. Anti-oxidant and anti-inflammatory activities of pomegranate (Punica granatum) on eimeria papillata induced infection in mice. Biomed Res Int (2015) 2015:1–7. doi: 10.1155/2015/219670
8. Williams RB. Tracing the emergence of drug-resistance in coccidia (Eimeria spp.) of commercial broiler flocks medicated with decoquinate for the first time in the united kingdom. Vet Parasitol (2006) 135(1):1–14. doi: 10.1016/j.vetpar.2005.10.012
9. Abbas RZ, Iqbal Z, Blake D, Khan MN, Saleemi MK. Anticoccidial drug resistance in fowl coccidia: the state of play revisited. World’s Poult Sci J (2011) 67(2):337–50. doi: 10.1017/S004393391100033X
10. Habibi H, Firouzi S, Nili H, Razavi M, Asadi SL, Daneshi S. Anticoccidial effects of herbal extracts on eimeria tenella infection in broiler chickens: in vitro and in vivo study. J Parasite Dis (2016) 40(2):401–7. doi: 10.1007/s12639-014-0517-4
11. Wunderlich F, Al-Quraishy S, Steinbrenner H, Sies H, Dkhil MA. Towards identifying novel anti-eimeria agents: Trace elements, vitamins, and plant-based natural products. Parasitol Res (2014) 113:3547–56. doi: 10.1007/s00436-014-4101-8
12. Latif MJ, Hassan SM, Mughal SS, Aslam A, Munir M, Shabbir N, et al. Therapeutic potential of azadirachta indica (Neem) and their active phytoconstituents against diseases prevention. J Chem Chem Sci (2020) 10(3):98–110.
13. Herrera-Calderon O, Ejaz K, Wajid M, Shehzad M, Tinco-Jayo JA, Enciso-Roca E, et al. Azadirachta indica: Antibacterial activity of neem against different strains of bacteria and their active constituents as preventive in various diseases. Pharmacogn J (2019) 11(6):1597–604. doi: 10.5530/pj.2019.11.244
14. Alzohairy MA. Therapeutics role of azadirachta indica (Neem) and their active constituents in diseases prevention and treatment. eCAM (2016) 2016:7382506. doi: 10.1155/2016/7382506
15. Sudan P, Goswami M, Singh J. Exploration of antifungal potential of azadirachta indica against microsporum gypseum. Biomed Pharmacol J (2020) 13(2):921–5. doi: 10.13005/bpj/1960
16. Habluetzel A, Pinto B, Tapanelli S, Nkouangang J, Saviozzi M, Chianese G, et al. Effects of azadirachta indica seed kernel extracts on early erythrocytic schizogony of plasmodium berghei and pro-inflammatory response in inbred mice. Malar J (2019) 18:35. doi: 10.1186/s12936-019-2671-8
17. Jagadeesh K, Srinivas K, Revankar SP. Anti inflammatory effect of azadirachta indica (Neem) in albino rats-an experimental study. IOSR J Phram (2014) 4(1):34–8. doi: 10.9790/3013-0411-34-38
18. Lee JW, Ryu HW, Park SY, Park HA, Kwon OK, Yuk HJ, et al. Protective effects of neem (Azadirachta indica a. juss.) leaf extract against cigarette smoke- and lipopolysaccharide-induced pulmonary inflammation. Inter J Mol Med (2017) 40:1932–40. doi: 10.3892/ijmm.2017.3178
19. Dkhil MA, Al-Quraishy S, Abdel Moneim AE, Delic D. Protective effect of azadirachta indica extract against eimeria papillata-induced coccidiosis. Parasitol Res (2013) 112(1):101–6. doi: 10.1007/s00436-012-3109-1
20. Gujjari L, Kalani H, Pindiprolu SK, Arakareddy BP, Yadagiri G. Current challenges and nanotechnology-based pharmaceutical strategies for the treatment and control of malaria. Parasite Epidemiol Control (2022) 17:e00244. doi: 10.1016/j.parepi.2022.e00244
21. Khandel P, Yadaw RK, Soni DK, Kanwar L, Shahi SK. Biogenesis of metal nanoparticles and their pharmacological applications: present status and application prospects. J Nanostruct Chem (2018) 8:217–54. doi: 10.1007/s40097-018-0267-4
22. Sun Y, Chen D, Pan Y, Qu W, Hao H, Wang X, et al. Nanoparticles for antiparasitic drug delivery. Drug Deliv (2019) 26(1):1206–21. doi: 10.1080/10717544.2019.1692968
23. Rayman MP. Selenium and human health. Lancet (2012) 379(9822):1256–68. doi: 10.1016/S0140-6736(11)61452-9
24. Beheshti N, Soflaei S, Shakibaie M, Yazdi MH, Ghaffarifar F, Dalimi A, et al. Efficacy of biogenic selenium nanoparticles against leishmania major: in vitro and in vivo studies. J Trace Elem Med Biol (2013) 27(3):203–7. doi: 10.1016/j.jtemb.2012.11.002
25. Dkhil MA, Abdel-Maksoud MA, Al-Quraishy S, Abdel-Baki AS, Wunderlich F. Gene expression in rabbit appendices infected with eimeria coecicola. Vet Parasitol (2012) 186(3-4):222–8. doi: 10.1016/j.vetpar.2011.11.031
26. Alkhudhayri AA, Dkhil MA, Al-Quraishy S. Nanoselenium prevents eimeriosis-induced inflammation and regulates mucin gene expression in mice jejunum. Int J Nanomed (2018) 13:1993–2003. doi: 10.2147/IJN.S162355
27. Alkhudhayri A, Al-Shaebi EM, Qasem MAA, Murshed M, Mares MM, Al-Quraishy S, et al. Antioxidant and anti-apoptotic effects of selenium nanoparticles against murine eimeriosis. An Acad Bras Cienc (2020) 92(2):e20191107. doi: 10.1590/0001-3765202020191107
28. Dkhil MA, Bauomy AA, Diab MS, Al-Quraishy S. Protective role of selenium nanoparticles against schistosoma mansoni induced hepatic injury in mice. Biomed Res (2016) 27(1):214–9.
29. Malekifard F, Tavassoli M, Vaziri K. In vitro assessment antiparasitic effect of selenium and copper nanoparticles on giardia deodenalis cyst. Iran J Parasitol (2020) 15(3):411–7. doi: 10.18502/ijpa.v15i3.4206
30. Sarhan MH, Farghaly A, Abd El-Aal NF, Farag SM, Ali AA, Farag TI. Egyptian Propolis and selenium nanoparticles against murine trichinosis: A novel therapeutic insight. J Helminthol (2022) 96:e50. doi: 10.1017/S0022149X22000359
31. Manikandan P, Letchoumy PV, Gopalakrishnan M, Nagini S. Evaluation of azadirachta indica leaf fractions for in vitro antioxidant potential and in vivo modulation of biomarkers of chemoprevention in the hamster buccal pouch carcinogenesis model. Food Chem Toxicol (2008) 46:2332–43. doi: 10.1016/j.fct.2008.03.013
32. Dkhil MA, Al-Shaebi EM, Abdel-Gaber R, Alkhudhayri A, Thagfan FA, Al-Quraishy S. Treatment of trypanosoma evansi-infected mice with eucalyptus camaldulensis led to a change in brain response and spleen immunomodulation. Front Microbiol (2022) 13:833520. doi: 10.3389/fmicb.2022.833520
33. Vahdati M, Moghadam TT. Synthesis and characterization of selenium nanoparticles-lysozyme nanohybrid system with synergistic antibacterial properties. Sci Rep (2020) 10(1):510. doi: 10.1038/s41598-019-57333-7
34. Abdel-Tawab H, Abdel-Baki AS, El-Mallah AM, Al-Quraishy S, Abdel-Haleem HM. In vivo and in vitro anticoccidial efficacy of astragalus membranaceus against eimeria papillata infection. J King Saud Uni Sci (2020) 32:2269–75. doi: 10.1016/j.jksus.2020.03.016
35. Abakar AD, Seri HI, Ismail AA, Musa HH. Comparative efficacy of selected anticoccidial drugs in ambarorow sheep naturally infected with enteric coccidia in south darfur, Sudan. Sudan J Vet Res (2005) 20:61–7.
36. Al-Quraishy S, Qasem MAA, Al-Shaebi EM, Murshed M, Mares MM, Dkhil MA. Rumex nervosus changed the oxidative status of chicken caecum infected with eimeria tenella. J King Saud Uni Sci (2020) 32(3):2207–11. doi: 10.1016/j.jksus.2020.02.034
37. Schito ML, Barta JR, Chobotar B. Comparison of four murine eimeria species in immunocompetent and immunodeficient mice. J Parasitol (1996) 82:255–62. doi: 10.2307/3284157
38. Adam H, Caihak G. Grosses zoologisches parktikum tell. arbeitsmethoden der makroskopischen und mikroskopischen anatomic. mit 283 abbildungen. Stuttgart: Gustav. Fischer Verlag (1964).
39. Dkhil MA, Abdel Moneim AE, Bauomy AA, Khalil M, Al-Shaebi EM, Al-Quraishy S. Chlorogenic acid prevents hepatotoxicity in arsenic-treated mice: role of oxidative stress and apoptosis. Mol Biol Rep (2020) 47:1161–71. doi: 10.1007/s11033-019-05217-4
40. Tsakiris S, Schulpis KH, Marinou K, Behrakis P. Protective effect of l-cysteine and glutathione on the modulated suckling rat brain na+, k+, -ATPase and Mg2+ -ATPase activities induced by the in vitro galactosaemia. Pharm Res (2004) 49:475–9. doi: 10.1016/j.phrs.2003.11.006
41. Beutler E, Duron O, Kelly BM. Improved method for the determination of blood glutathione. J Lab Clin Med (1963) 61:882–8.
42. Pagila DE, Valentine WN. Studies on the quantitative and qualitative characterization of erythrocyte glutathione peroxidase. J Lab Clin Med (1967) 70:158–69.
43. Ohkawa H, Ohishi N, Yagi K. Assay for lipid peroxides in animal tissues by thiobarbituric acid reaction. Anal Biochem (1979) 95(2):351–8. doi: 10.1016/0003-2697(79)90738-3
45. Nishikimi M, Appaji N, Yagi K. The occurrence of superoxide anion in the reaction of reduced phenazine methosulfate and molecular oxygen. Biochem Biophys Res Commun (1972) 46(2):849–54. doi: 10.1016/S0006-291X(72)80218-3
46. Livak KJ, Schmittgen TD. Analysis of relative gene expression data using real-time quantitative PCR and the 2–ΔΔCT method. Methods (2001) 25:402–8. doi: 10.1006/meth.2001.1262
47. Peek HW. Resistance to anticoccidial drugs: Alternative strategies to control coccidiosis in broilers. the Netherlands: Utrecht University (2010) p. 1–244. Doctoral Thesis.
48. Shakibaie M, Khorramizadeh MR, Faramarzi MA, Sabzevari O, Shahverdi AR. Biosynthesis and recovery of selenium nanoparticles and the effects on matrix metalloproteinase-2 expression. Biotechnol Appl Biochem (2010) 56(1):7–15. doi: 10.1042/BA20100042
49. Cermonini E, Zonaro E, Donini M, Lampis S, Lampis S, Boaretti M, et al. Biogenic selenium nanoparticles: Characterization, antimicrobial activity and effects on human dendritic cells and fibroblasts. Microb Biotechnol (2016) 9(6):758–71. doi: 10.1111/1751-7915.12374
50. Elmaaty TA, Sayed-Ahmed K, Elsisi H, Ramadan SM, Sorour H, Magdi M, et al. Novel antiviral and antibacterial durable polyester fabrics printed with selenium nanoparticles (SeNPs). Polymers (2022) 14:955. doi: 10.3390/polym14050955
51. Shewale S, Rathod V. Extraction of total phenolic content from azadirachta indica or (neem) leaves: Kinetics study. Prep Biochem Biotechnol (2018) 48(4):312–20. doi: 10.1080/10826068.2018.1431784
52. Oke EO, Adeyi O, Okolo BI, Adeyi JA, Ayanyemi J, Osoh KA, et al. Phenoilc compound extraction from Nigerian azadirachta indica leaves: Response surface and neuro-fuzzy modelling performance evaluation with cuckoo search multi objective optimization. RINENG (2020) 8:100160. doi: 10.1016/j.rineng.2020.100160
53. Maragathavalli S, Brindha S, Kaviyarasi NS, Annadurai B, Gangwar SK. Antimicrobial activity in leaf extract of neem (Azadirachta indica linn.). Inter J Sci Nat (2012) 3(1):110–3.
54. Akhter R, Sarker MW. Antimicrobial activity in leaf extract of neem in broiler. Res Agric Livest Fish (2019) 6(2):337–43. doi: 10.3329/ralf.v6i2.43063
55. Filipović N, Ušjak D, Milenković MT, Zheng K, Liverani L, Boccaccini AR, et al. Comparative study of the antimicrobial activity of selenium nanoparticles with different surface chemistry and structure. Front Bioeng Biotechnol (2021) 8:624621. doi: 10.3389/fbioe.2020.624621
56. Wylie MR, Merrell DS. The antimicrobial potential of the neem tree Azadirachta indica. Front Pharmacol (2022) 13:891535. doi: 10.3389/fphar.2022.891535
57. Anwar MI, Akhtar M, Hussain I, Haq AU, Muhammad F, Abdul Hafeez M, et al. Field evaluation of eimeria tenella (local isolates) gametocytes vaccine and its comparative efficacy with imported live vaccine, LivaCox®. Parasitol Res (2008) 104:135–43. doi: 10.1007/s00436-008-1171-5
58. Gilbert ER, Cox CM, Williams PM, Mcelroy AP, Dalloul RA, Ray K, et al. Eimeria species and genetic background influence the serum protein profile of broilers with coccidiosis. PloS One (2011) 6(1):e14636. doi: 10.1371/journal.pone.0014636
59. Qaid MM, Al-Mufarrej SI, Azzam MM, Al-Garadi MA. Anticoccidial effectivity of a traditional medicinal plant, cinnamomum verum, in broiler chickens infected with eimeria tenella. Poult Sci (2021) 100:100902. doi: 10.1016/j.psj.2020.11.071
60. Heyman L, Houri-Haddad Y, Heyman SN, Ginsburg I, Gleitman Y, Feuerstein O. Combined antioxidant effects of neem extract, bacteria, red blood cells and lysozyme: possible relation to periodontal disease. BMC complement. Altern Med (2017) 17:399. doi: 10.1186/s12906-017-1900-3
61. Nabi F, Arain MA, Hassan F, Umar M, Rajput N, Alagawany M. Nutraceutical role of selenium nanoparticles in poultry nutrition: a review. World’s Poult Sci J (2020) 76(3):459–71. doi: 10.1080/00439339.2020.1789535
62. Onyiche TE, Gotep JG, Tanko JT, Ochigbo GO, Ozoani HA, Viyoff VZ, et al. Azadirachta indica aqueous leaf extracts ameliorates coccidiosis in broiler chickens experimentally infected with eimeria oocyts. Sci Afri (2021) 13:e00851. doi: 10.1016/j.sciaf.2021.e00851
63. Abdel-Moneim AME, El-Saadony MT, Shehata AM, Saad AM, Aldhumri SA, Ouda SM, et al. Antioxidant and antimicrobial activities of spirulina platensis extracts and biogenic selenium nanoparticles against selected pathogenic bacteria and fungi. Saudi J Biol Sci (2022) 29:1197–209. doi: 10.1016/j.sjbs.2021.09.046
64. Qudoos A, Iqbal A, Ahmed SS, Khan MS, Bayram I. Effects of some alternative plant extracts used as natural coccidiostat for pigeons. JASP (2020) 3(1):20–31.
65. Ajideh R, Pourmand MR, Faramarzi MA, Sepehrizadeh Z, Pourmand G, Hassanzadeh SM, et al. Potentiation of the therapeutic effect of intravesical BCG through synthetic and biogenic selenium nanoparticles in a nitrosamine-induced bladder cancer mouse model. Adv Cancer Biol Metastasis (2022) 6:100061. doi: 10.1016/j.adcanc.2022.100061
66. Ishaq R, Chand N, Khan RU, Saeed M, Laudadio V, Tufarelli V. Methanolic extract of neem (Azadirachta indica) leaves mitigates experimentally induced coccidiosis challenge in Japanese quails. J Appl Anim Res (2022) 50(1):498–503. doi: 10.1080/09712119.2022.2096037
67. Morad MY, El-Sayed H, Elhenawy AA, Korany SM, Aloufi AS, Ibrahim AM. Myco-synthesized molluscicidal and larvicidal selenium nanoparticles: A new strategy to control biomphalaria alexandrina snails and larvae of schistosoma mansoni with an in silico study on induced oxidative stress. J Fungi (2022) 8:262. doi: 10.3390/jof8030262
68. Georgieva NV, Koinarski V, Gadjeva V. Antioxidant status during the course of eimeria tenella infection in broiler chickens. Vet J (2006) 172(2):488–92. doi: 10.1016/j.tvjl.2005.07.016
69. Surai PF, Kochish II, Fisinin VI, Kidd MT. Antioxidant defence systems and oxidative stress in poultry biology: An update. Antioxid (Basel) (2019) 8(7):235. doi: 10.3390/antiox8070235
70. Dkhil MA, Abdel-Baki AS, Wunderlich F, Sies H, Al-Quraishy S. Anticoccidial and anti-inflammatory activity of garlic in murine eimeria papillata infections. Vet Parasitol (2011) 175(1-2):66–72. doi: 10.1016/j.vetpar.2010.09.009
71. Elmahallawy EK, Fehaid A, El-Shewehy DMM, Ramez AM, Alkaldi AAM, Mady R, et al. S-methylcysteine ameliorates the intestinal damage induced by eimeria tenella infection via targeting oxidative stress and inflammatory modulators. Front Vet Sci (2021) 8:754991. doi: 10.3389/fvets.2021.754991
72. Gotep JG, Tanko JT, Forcados GE, Muraina IA, Ozele N. Therapeutic and safety evaluation of combined aqueous extracts of azadirachta indica and khaya senegalensis in chickens experimentally infected with eimeria oocysts. J Parasitol Res (2016) 2016:1–9. doi: 10.1155/2016/4692424
73. Hassan RA, Soliman ES, Hamad RT, El-Borady OM, Ali AA, Helal MS. Selenium and nano-selenium ameliorations in two breeds of broiler chickens exposed to heat stress. S. Afr J Anim Sci (2020) 50(2):215–32. doi: 10.4314/sajas.v50i2.5
74. Michalak I, Dziergowska K, Alagawany M, Farag MR, El-Shall NA, Tuli HS, et al. The effect of metal-containing nanoparticles on the health, performance and production of livestock animals and poultry. Vet Q (2022) 42(1):68–94. doi: 10.1080/01652176.2022.2073399
75. Dominquez PA, Pro-Martinez A, Narciso-Gaytán C, Hernández-Cázares A, Sosa-Montes E, Perez-Hernandez P, et al. Concurrent supplementation of arginine and antioxidant vitamins e and c reduces oxidative stress in broiler chickens after a challenge with eimeria spp. Can J Anim Sci (2015) 95:143–53. doi: 10.4141/cjas-2014-083
76. Aslam F, Rehman K, Asghar M, Sarwar M. Antibacterial activity of various phytoconstituents of neem. Pak J Agric Sci (2009) 46(3):209–13.
77. Hossain MA, Al-Toubi WAS, Weli AM, Al-Riyami QA, Al-Sabahi JN. Identification and characterization of chemical compounds in different crude extracts from leaves of omani neem. J Taibah Uni Sci (2013) 7:181–8. doi: 10.1016/j.jtusci.2013.05.003
78. Seriana I, Akmal M, Darusman D, Wahyuni S, Khairan K, Sugito S. Neem leaf (Azadirachta indica a. juss) ethanolic extract on the liver and kidney function of rats. Sci World J (2021) 2021:7970424. doi: 10.1155/2021/7970424
79. Grondin JA, Kwon YH, Far PM, Haq S, Khan W. Mucins in intestinal mucosal defense and inflammation: Learning from clinical and experimental studies. Front Immunol (2020) 11:2054. doi: 10.3389/fimmu.2020.02054
80. Umar S. Intestinal stem cells. Curr Gastroenterol Rep (2011) 12(5):340–8. doi: 10.1007/s11894-010-0130-3
81. Yunus M, Horii Y, Makimura S, Smith AL. Murine goblet cell hypoplasia during eimeria pragensis infection is ameliorated by clindamycin treatment. J Vet Sci (2005) 67(3):311–5. doi: 10.1292/jvms.67.311
82. Kim JJ, Khan WI. Goblet cells and mucins: Role in innate defense in enteric infections. Pathogens (2013) 2(1):55–70. doi: 10.3390/pathogens2010055
83. Yang S, Yu M. Role of goblet cells in intestinal barrier and mucosal immunity. J Inflamm Res (2022) 12:3171–83. doi: 10.2147/JIR.S318327
84. Boltin D, Perets TT, Vilkin A, Niv Y. Mucin function in inflammatory bowel disease, an update. J Clin Gastroenterol (2013) 47:106–11. doi: 10.1097/mcg.0b013e3182688e73
85. Gum JR, Hicks JW, Gillespie AM, Carlson EJ, Kömüves L, Karnik S, et al. Goblet cell-specific expression mediated by the MUC2 mucin gene promoter in the intestine of transgenic mice. Am J Physiol (1999) 276:G666–76. doi: 10.1152/ajpgi.1999.276.3.G666
86. Larsson JM, Karlsson H, Crespo JG, Johansson ME, Eklund L, Sjovall H, et al. Altered O-glycosylation profile of MUC2 mucin occurs in active ulcerative colitis and is associated with increased inflammation. Inflamm Bowel Dis (2011) 17:2299–307. doi: 10.1002/ibd.21625
87. Enss ML, Cornberg M, Wagner S, Gebert A, Henrichs M, Eisenblätter R, et al. Proinflammatory cytokines trigger MUC gene expression and mucin release in the intestinal cancer cell line LS180. Inflamm Res (2000) 49(4):162–9. doi: 10.1007/s000110050576
88. Hsu HP, Lai MD, Lee JC, Yen MC, Weng TY, Chen WC, et al. Mucins 2 silencing promotes colon cancer metastasis through interleukin-6 signaling. Sci Rep (2017) 7:5823. doi: 10.1038/s41598-017-04952-7
89. Dudov IA, Morenets AA, Artyukh VP, Starodub NF. Immunomodulatory effect of honeybee flower pollen load. Ukr Biokhim Zh (1994) 66(6):91–3.
90. Kempuraj D, Madhappan B, Christodoulou S, Boucher W, Cao J, Papadopoulou N, et al. Flavonols inhibit proinflammatory mediator release, intracellular calcium ion levels and protein kinase c theta phosphorylation in human mast cells. Br J Pharmacol (2005) 145(7):934–44. doi: 10.1038/sj.bjp.0706246
91. Lüder CG, Gross U, Lopes MF. Intracellular protozoan parasites and apoptosis: Diverse strategies to modulate parasite-host interactions. Trends Parasitol (2001) 17(10):480–6. doi: 10.1016/S1471-4922(01)02016-5
92. Balamurugan K, Rajaram R, Ramasami T, Narayanan S. Chromium (III)-induced apoptosis of lymphocytes: Death decision by ROS and src-family tyrosine kinases. Free Radic Biol Med (2002) 33(2):1622–40. doi: 10.1016/s0891-5849(02)01115-2
93. Dkhil MA. Anti-coccidial, anthelmintic and antioxidant activities of pomegranate (Punica granatum) peel extract. Parasitol Res (2013) 112(7):2639–46. doi: 10.1007/s00436-013-3430-3
94. Schumacher M, Cerella C, Reuter S, Dicato M, Diederich M. Anti-inflammatory, pro-apoptotic, and anti-proliferative effects of a methanolic neem (Azadirachta indica) leaf extract are mediated via modulation of the nuclear factor-kB pathway. Genes Nutr (2011) 6(2):149–60. doi: 10.1007/s12263-010-0194-6
Keywords: coccidiosis, mice, nanoparticles, Azadirachta indica, oxidative stress, apoptosis, inflammation
Citation: Abdel-Gaber R, Hawsah MA, Al-Otaibi T, Alojayri G, Al-Shaebi EM, Mohammed OB, Elkhadragy MF, Al-Quraishy S and Dkhil MA (2023) Biosynthesized selenium nanoparticles to rescue coccidiosis-mediated oxidative stress, apoptosis and inflammation in the jejunum of mice. Front. Immunol. 14:1139899. doi: 10.3389/fimmu.2023.1139899
Received: 07 January 2023; Accepted: 08 February 2023;
Published: 17 February 2023.
Edited by:
Mohd Adnan, University of Hail, Saudi ArabiaReviewed by:
Saeed El-Ashram, Foshan University, ChinaShawky Mohamed Aboelhadid, Beni-Suef University, Egypt
Copyright © 2023 Abdel-Gaber, Hawsah, Al-Otaibi, Alojayri, Al-Shaebi, Mohammed, Elkhadragy, Al-Quraishy and Dkhil. This is an open-access article distributed under the terms of the Creative Commons Attribution License (CC BY). The use, distribution or reproduction in other forums is permitted, provided the original author(s) and the copyright owner(s) are credited and that the original publication in this journal is cited, in accordance with accepted academic practice. No use, distribution or reproduction is permitted which does not comply with these terms.
*Correspondence: Mohamed A. Dkhil, bW9oYW1lZGRraGlsQHlhaG9vLmNvbQ==