- 1Department Outpatient Clinic, ASL8 Outpatient Clinic Quartu Sant’Elena, Cagliari, Italy
- 2Centro Servizi di Ateneo per gli Stabulari (CeSaSt), University of Cagliari, Monserrato, Italy
- 3Department of Biomedical Science, University of Cagliari, Monserrato, Italy
- 4Department of Pediatrics, Clinic of Pediatric and Rare Diseases, Microcitemico Pediatric Hospital, A.Cao, ASL8, Cagliari, Italy
- 5Department of Medical Science and Public Health, University of Cagliari, Monserrato, Italy
- 6Department of Biomedical Sciences, Institute for Genetic and Biomedical Research, Monserrato, Italy
- 7Department of Pediatric, Diabetologic Unit, Microcitemico Pediatric Hospital, A.Cao, ASL8, Cagliari, Italy
- 8Department of Biomedical Science, University of Sassari, Sassari, Italy
Introduction: T cell reactivity against pancreatic autoantigens is considered one of the main contributors to the destruction of insulin-producing cells in type 1 diabetes (T1D). Over the years, peptide epitopes derived from these autoantigens have been described in NOD mice and in both HLA class II transgenic mice and humans. However, which ones are involved in the early onset or in the progressive phases of the disease is still unclear.
Methods: In this work we have investigated, in early-onset T1D pediatric patients and HLA-matched controls from Sardinia, the potential of preproinsulin (PPI) and glutamate decarboxylase 65 (GAD65)-derived peptides to induce spontaneous T cell proliferation responses of peripheral blood mononuclear cells (PBMCs).
Results: Significant T cell responses against PPI1-18, PPI7-19 and PPI31-49, the first two belonging to the leader sequence of PPI, and GAD65271-285 and GAD65431-450, were found in HLA-DR4, -DQ8 and -DR3, -DQ2 T1D children.
Conclusions: These data show that cryptic epitopes from the leader sequence of the PPI and GAD65271-285 and GAD65431-450 peptides might be among the critical antigenic epitopes eliciting the primary autoreactive responses in the early phases of the disease. These results may have implications in the design of immunogenic PPI and GAD65 peptides for peptide-based immunotherapy.
1 Introduction
Type 1 diabetes mellitus (T1D) is the result of a slow progressive multistep autoimmune destruction of pancreatic insulin-producing cells (1–3). Early studies in European and derived populations have shown that susceptibility to T1D is strongly associated with HLA-DR3, -DQ2 and HLA-DR4, -DQ8 haplotypes, while protection is associated with HLA-DR2, -DQ6 haplotypes (4, 5). Subsequent studies have shown that within these haplotypes certain HLA-DQB1, and -DRB1 alleles and residues confer susceptibility, while others provide resistance to the disease (6–11). For instance, several studies have shown that differences of a few amino acid residues in HLA-DRB1*04 alleles are per se sufficient to modify the risk of developing T1D conferred by the high-risk HLA-DQB1*03:02 allele (12–15). Indeed, the spectrum of HLA-DQB1 and -DRB1 association, which constitutes the major component of T1D risk, is more complex than initially outlined and can be primarily grouped into very high risk, intermediate and very low risk haplotypes which, in turn, are the result of the structure of the peptide-binding pockets (15, 16).
In recent years, the intricacy and the molecular nature of immunogenic T cell epitopes of pancreatic autoantigens have been further elucidated (17). It is generally believed that T cell responses against autoantigens may become less evident with the progressive destruction of the islets of Langerhans (18, 19). Moreover, the spreading of T cell reactivity to other not primarily involved regions, of preproinsulin (PPI) or glutamate decarboxylase 65 (GAD65) autoantigens or to other pancreatic proteins/neoantigens generated from post-translational modifications may be an important confounding factor complicating the identification of pathogenically relevant T cells (19–22). Secondly, autoreactive immune responses are not completely disease-specific, since T cell reactivity against autoantigenic proteins is detected also in control subjects who carry disease-associated HLA-DR and -DQ molecules (18, 23, 24).
A useful tool to identify immunodominant T cell epitopes from pancreatic autoantigens is the use of humanized HLA transgenic mice (25). Indeed, numerous PPI and GAD65 T cell epitopes have been identified in triple HLA, human CD4 (hCD4) and IA knock-out transgenic mice (25–29) and confirmed in T1D patients (30–32). The majority of these studies have been conducted using triple HLA-DRB1*04:01, hCD4, IA knock-out mice (25–29), in HLA-DQ8 transgenic mice (33), whilst literature regarding studies on patients carrying different HLA-DR4 subtypes, such as the HLA-DRB1*04:05-DQA1*03:01-DQB1*03:02 haplotype is lacking. Interestingly, this is the most frequent HLA-DR4 haplotype found in Sardinian T1D patients (13, 16, 34).
Thus, we tested in children with recent-onset T1D and HLA-matched healthy controls from Sardinia a set of PPI and GAD65 peptides derived from previous studies in both HLA-DR4 and -DQ8 transgenic mice and humans (26–28, 33, 35).
2 Materials and methods
2.1 Sample selection
Twelve HLA-DR4, -DQ8 positive and four HLA-DR4, -DQ8 negative T1D patients with recent onset disease have been studied. In all T1D patients the diagnosis was performed 3 days before enrollment. Fourteen HLA-DR4, -DQ8 positive and four HLA-DR4, -DQ8 negative healthy blood donors without history of autoimmune diseases served as controls. The 18 healthy individuals were HLA typed in connection with other studies (36). All T1D patients with recent-onset disease were recruited from our clinic in the Microcitemico Hospital A. Cao, ASL8, Cagliari. The mean age was 7.4 ± 3.3 years, in line with the mean age of T1D onset in Sardinia (37). The mean age of healthy controls was 43.6 ± 5.4. Table 1 shows the HLA-DR, -DQ typing of T1D patients and controls. An ethics committee approval was obtained for this study (authorization no. PG/2016/7815).
2.2 HLA class II typing and haplotype analysis
HLA-DRB1, -DQA1 and -DQB1 genotypes were determined by polymerase chain reaction with sequence-specific primers (SSP-PCR) using Olerup SSP typing kits (Olerup SSP AB, Stockholm, Sweden). The HLA class II haplotypes were predicted on the basis of the known linkage disequilibrium in Sardinians (12, 13).
2.3 Proliferation assays
Blood was drawn from recent-onset T1D patients within two to three days after diagnosis. Peripheral blood mononuclear cells (PBMCs) were isolated by density gradient. Blood was diluted 1:1 with complete medium (RPMI 1640, Life Technologies Italia, Monza, Italy) containing 2% heat-inactivated fetal calf serum, 2 mM L-glutamine, 100 U/ml penicillin, 100 μg/ml of streptomycin, 50 μM 2-mercaptoethanol and HEPES. Diluted blood was then layered 1:1 on a Lymphoprep™ gradient (Stem Cell Technologies, Monza, Italy) and centrifuged at 400 g for 30 min at room temperature.
Cells were harvested and washed in complete medium containing 10-15% autologous human serum, and 2 x 105 cells/200 µl per well were incubated in the presence or absence of 20 µg/ml of PPI or GAD65 peptides. Proliferative responses against antigens were determined in 6 replicate cultures in round-bottomed 96 well plates by [3H]-thymidine incorporation after 6 days of culture, following a 6-hour pulse with 0.5 µCi [3H]-thymidine. T cell reactivity was attributed using a relative ratio (RR) of counts per minute (cpm) of radioactivity incorporated by PBMCs plus peptide, compared to PBMCs alone. A RR of 3 or higher was considered positive and used in the statistical calculation. T cell reactivity has been controlled by stimulation with concanavalin A (data not shown).
2.4 PPI and GAD65 peptides
Eleven peptides from PPI and 16 from GAD65 (Table 2) were purchased from Life Technologies Italia (Monza, Italy). These peptide epitopes were derived from research in humans and from previous studies in HLA-DR4 or -DQ8 transgenic mice (21, 25–33, 35). The purity of all these peptides was verified by reverse-phase HPLC and mass spectroscopic analysis. Before use, peptides were suspended in sterile PBS at a concentration of 2 mg/ml and stored at -80° C.
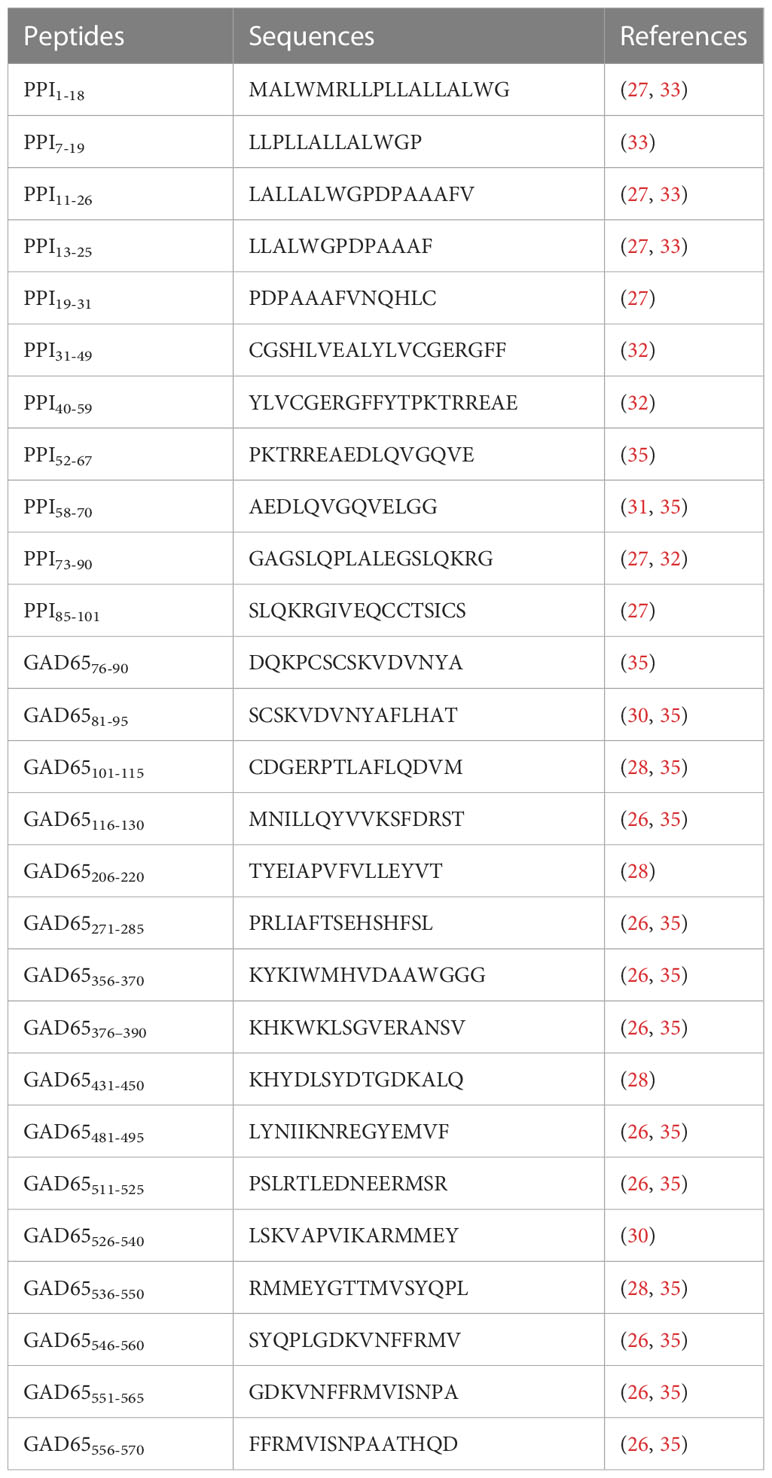
Table 2 PPI and GAD65 immunodominant epitopes identified in different HLA transgenic mice and humans used in this work.
2.5 Statistical analysis
A Chi-square test was used to compare PPI and GAD65 proliferative responses between T1D and controls. In the case of samples size lower than 5, a two-tailed Fisher’s exact test was used. A p value lower than 0.05 was considered statistically significant.
3 Results
3.1 T cell reactivity against PPI peptides
PBMCs from patients and HLA-matched healthy individuals were cultured with or without peptides and their proliferations measured. The eleven peptides tested cover the PPI protein sequence almost completely. Data were analyzed as a whole and after grouping patients and control individuals according to HLA-DR4 haplotype. Tables S1, S2 summarize the proliferative responses and RR obtained in T1D patients and controls respectively.
We have found that 75% of T1D patients and 33.3% of controls did respond to PPI peptides (p= 0.0204). Response to more than one PPI peptide was found in 62.5% of T1D patients and in 11.1% of controls (p= 0.0033).
Next, we evaluated which epitopes accounted for this reactivity by comparing the frequency of responses against specific peptides of PPI in T1D patients and controls. Figure 1A illustrates the frequency of responses against PPI peptides, while Figure 1B provides an example of responses against PPI peptides in a T1D patient (n. 11, Table S1). Tables S1, S2 summarize the proliferative responses and the RR obtained in T1D patients and controls respectively. Significant differences between patients and controls were found for PPI1-18 (p=0.0348; Figure 1A), PPI7-19 peptides (p=0.0348; Figure 1A) and PPI31-49 (p=0.0392; Figure 1A). The statistical significance for PPI peptides was lost after stratification for DR4 haplotypes.
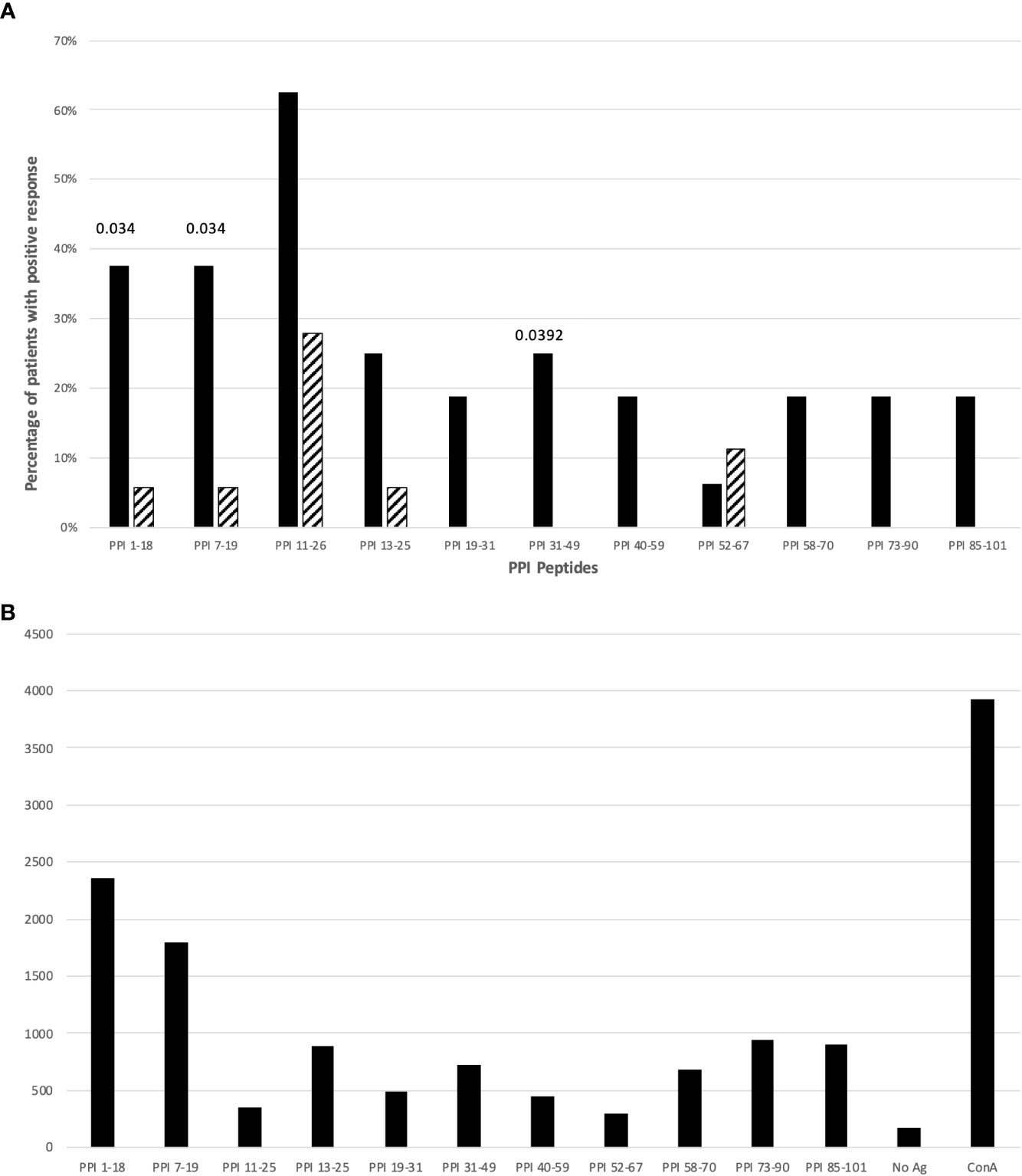
Figure 1 (A) Percentage of T1D patients (solid bars) and controls (hatched bars) responding to PPI peptides. Significant p values (<0.05) are indicated above the corresponding bars. Statistical analysis was performed using the Fisher’s exact test. (B) The figure shows cpm response with different PPI peptides in a T1D patient (n. 11, Table S1).
In terms of global reactivity, our results indicate a higher response against peptides of the PPI protein and less reactivity against GAD65 peptides. In T1D patients, we observed 46 out of 176 responses for PPI peptides versus 40 out of 224 responses for GAD65 (p=0.045).
3.2 T cell reactivity against GAD65 peptides
Sufficient PBMCs for measurement of proliferative responses against GAD65 peptides were available from 14 T1D patients and 18 control individuals. Tables S3, S4 summarize the proliferative responses and the RR obtained in T1D patients and controls respectively.
Fifty-six percent of T1D patients and 27.7% of controls did respond against GAD65 peptides (p: NS). The responses to more than one GAD65 peptide did not differ significantly between T1D patients (37.5%) and controls (16.6%). Only for GAD65271-285 and GAD65431-450 peptides a significant difference between patients and controls was observed (p=0.0278; Figure 2). The statistical significance for these two GAD65 peptides was lost after stratification for DR4 haplotypes.
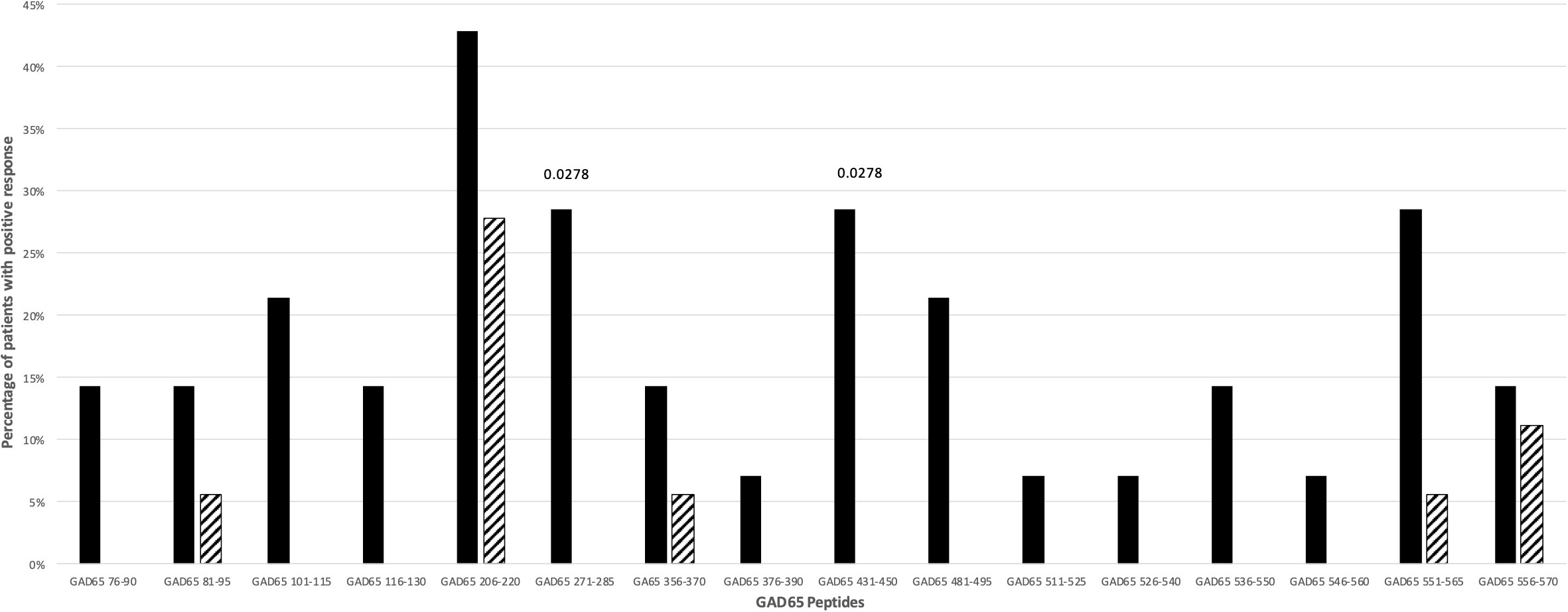
Figure 2 Percentage of T1D patients (solid bars) and controls (hatched bars) responding to GAD65 peptides. Significant p values (<0.05) are indicated above the corresponding bars. Statistical analysis was performed using the Fisher’s exact test.
4 Discussion
Here, we report the capacity of PPI and GAD65 derived peptides obtained from humans and from HLA-DR4 and -DQ8 transgenic mice (21, 25–33, 35) to induce spontaneous T cell proliferation responses in PBMCs from recent-onset T1D pediatric patients.
In terms of global reactivity, our results indicate a higher response against peptides of the PPI protein and less reactivity against GAD65 peptides.
Analysis of proliferative responses to PPI showed that reactivity is mainly directed against PPI1-18, and PPI7-19 peptides of the leader sequence. These 2 peptides are included in the immunodominant peptide PPI1-24 described as HLA-DQ8-restricted in HLA-DQ8 transgenic mice (33). However, we found responses against these 2 peptides in both HLA-DR4, -DQ8 and -DR3, -DQ2 positive T1D patients compared to HLA-matched controls (Tables S1, S2; Figure 1A).
Since we found significant responses for both peptides, we hypothesize that the sequence responsible for reactivity in T1D patients is included in the PPI7-19 shared sequence LLPLLALLALWG. Prevalent reactivity of T1D PBMCs against the leader sequence may indicate that precursors of insulin, normally confined to the ER of the β-cells, are involved in targeting T cell autoreactivity to the islets. Interestingly, the same region of PPI is recognized by CD8+ T cells from recent-onset T1D patients (38, 39), which may suggest that the leader sequence of PPI is targeted by both CD4+ and CD8+ autoreactive T cells. In addition to HLA-DQ8, the leader sequence of PPI (LALLALWGPDPAAAFV) may be presented also by HLA-DRB1*04:01 as previously shown in HLA-DRB1*04:01 transgenic mice (27). Therefore, in analogy to other autoantigens such as GAD65 (26, 28), the leader sequence of PPI may show overlapping peptide sequences that are presented by both HLA-DR and -DQ molecules.
Also for PPI31–49 peptide, including the B chain peptide PPIB9-23, reported immunodominant in both humans and NOD mice (40–42), significant proliferative responses were observed (Table S1; Figure 1A).
Interestingly, CD8+ T cells in long-standing patients have been shown to recognize the B-chain peptide PPI33–42 (38), that is also targeted by both human and NOD mice CD4+ T cells (41, 42).
Among the 16 GAD65 peptides tested, only GAD65271-285 and GAD65431-450 peptides induced significant proliferative responses in T1D patients. These patients carried both HLA-DR4 and HLA-DR3 haplotypes (Tables S3, S4; Figure 2). These findings are in line with previous data showing that GAD65271-285 was found immunodominant in HLA-DRB1*04:01 transgenic mice and humans (26), whilst a peptide similar to GAD65431-450 (GAD65431-445) is recognized by HLA-DQ8 molecules in HLA-DQ8 transgenic mice (28). Interestingly, GAD65431-450 has been also reported as an inducer of IL-13 in CD4+ T cell lines derived from HLA-DR3, -DQ2 homozygous individuals (43).
Noteworthy, the GAD65 region 245-450 that includes both GAD65271-285 and GAD65431-450 has been identified as the main target of the earlier anti-GAD65 response in pre-diabetic, healthy high-risk subjects and early onset T1D patients (44–46). Finally, in genetically at-risk T1D patients, autoantibodies against GAD65 are found mainly in HLA-DR3, -DQ2 and less commonly in HLA-DR4, -DQ8 patients (19, 47).
Our findings demonstrate the crucial importance of validating in T1D patients the prediction of immunogenic epitopes. Indeed, even epitopes that are immunodominant in HLA class II transgenic mice may be not immunogenic in humans. As an example, GAD65206-220 peptide is an immunogenic/immunodominant T cell epitope in HLA-DQ8 transgenic mice (28, 48) and in NOD mice after immunization with murine GAD65 (49) and, as such, could be considered a good pathogenic candidate for T1D in mice and humans. Instead, T cell receptor transgenic mice specific for GAD65206-220 have been demonstrated to be protected against T1D development (50). In accordance, our data show that this peptide elicits proliferative responses not only in T1D patients but also in controls (Tables S3, S4; Figure 2), making a pathogenic role highly unlikely also in humans.
Our study has several limitations that we tried to mitigate. The large number of potential T cell epitopes of pancreatic autoantigenic proteins that need to be tested challenges the relatively small amount of blood that can be drawn from children. To circumvent this limitation, we restricted the number of epitopes by prior analysis of the T cell responses in transgenic mice carrying human HLA haplotypes. Another limitation is the T cell spreading in long standing T1D patients (19–21), which is why we focused on early onset T1D pediatric patients recruited within three to four days after diagnosis. Discrepancies in the interpretation of T cell responses from PBMCs, the usage of diverse experimental methodologies among laboratories and the variability in the frequencies of HLA-DR4 subtypes in different T1D populations, may lead to different results. In this work we tried to reduce these variables taking advantage of the genetically homogenous Sardinian population, less prone to both genetic and clinical confounds that are present in more cosmopolitan collections (13, 51). Finally, a further possible limitation of this work was the significant age difference between T1D patients and controls. However, the 18 controls were healthy blood donors who were selected for a negative history of autoimmune diseases, thus making spontaneous not specific responses less likely than what could have been derived from age matched random pediatric controls attending the outpatient clinic for different disorders.
In summary, we identified for both PPI and GAD65 specific proliferative responses addressed to the PPI leader sequence and to GAD65271-285, GAD65431-450 in T1D patients. We suggest that these could be primary epitopes involved in the early phase of the clinical onset of T1D. Finally, these data hint towards the possibility that the leader sequence of PPI is targeted by both CD8+ and CD4+ T cells, with HLA class I and class II presenting slightly different overlapping peptide epitopes and thus inducing a stronger pathogenic immune response involved in the β-cell destruction.
These results may have implications in the design of immunogenic PPI and GAD65 peptides for peptide-based immunotherapy. Finally, this work could pave the way to test these epitopes in early stage T1D patients (stage 1 or 2) who have islet autoantibodies but no overt clinical diabetes requiring treatment with exogenous insulin.
Data availability statement
The original contributions presented in the study are included in the article/Supplementary Material. Further inquiries can be directed to the corresponding author.
Ethics statement
The studies involving human participants were reviewed and approved by Comitato Etico Indipendente AOUCA. Written informed consent to participate in this study was provided by the participants’ legal guardian/next of kin.
Author contributions
RJ and MC conceptualized, designed and led the research. DB, ES, SM contributed to data interpretation and drafting the manuscript. RR provided HLA typing. MMan, MMar and CC collected and processed PBMC. CR and MR provided T1D patients. FC critically revised the manuscript. MC is the guarantor of this study. All authors contributed to the article and approved the submitted version.
Funding
The research was funded by: Regione Autonoma della Sardegna (CRP-59539, MC, SM and DB); non-profit association Associazione Diabete Zero ODV supported also by Fondazione Sardegna (DB) and Thyssen Foundation, Germany (RJ).
Acknowledgments
The authors wish to thank Dr. Barbara Batetta (Department of Biomedical Science, University of Cagliari, Cagliari, Sardinia, Italy) for providing the laboratory facility to use [3H]-thymidine.
Conflict of interest
The authors declare that the research was conducted in the absence of any commercial or financial relationships that could be construed as a potential conflict of interest.
Publisher’s note
All claims expressed in this article are solely those of the authors and do not necessarily represent those of their affiliated organizations, or those of the publisher, the editors and the reviewers. Any product that may be evaluated in this article, or claim that may be made by its manufacturer, is not guaranteed or endorsed by the publisher.
Supplementary material
The Supplementary Material for this article can be found online at: https://www.frontiersin.org/articles/10.3389/fimmu.2023.1130019/full#supplementary-material
References
1. Tisch R, McDevitt H. Insulin-dependent diabetes mellitus. Cell (1996) 85:291–7. doi: 10.1016/S0092-8674(00)81106-X
2. Bluestone JA, Buckner JH, Herold KC. Immunotherapy: Building a bridge to a cure for type 1 diabetes. Science (2021) 373(6554):510–6. doi: 10.1126/science.abh1654
3. Dayan CM, Besser REJ, Oram RA, Hagopian W, Vatish M, Bendor-Samuel O, et al. Preventing type 1 diabetes in childhood. Science (2021) 373(6554):506–10. doi: 10.1126/science.abi4742
4. Svejgaard A, Christy M, Green A, Hauge M, Nerup J, Platz P, et al. HLA and diabetes. Prog Clin Biol Res (1982) 103(Pt B):55–64.
5. Thomson G. HLA DR antigens and susceptibility to insulin-dependent diabetes mellitus. Am J Hum Genet (1984) 36(6):1309–17.
6. Todd JA, Bell JI, McDevitt HO. HLA-DQ beta gene contributes to susceptibility and resistance to insulin-dependent diabetes mellitus. Nature (1987) 329(6140):599–604. doi: 10.1038/329599a0
7. Khalil I, d'Auriol L, Gobet M, Morin L, Lepage V, Deschamps I, et al. A combination of HLA-DQ beta Asp57-negative and HLA DQ alpha Arg52 confers susceptibility to insulin-dependent diabetes mellitus. J Clin Invest (1990) 85(4):1315–9. doi: 10.1172/JCI114569
8. Sheehy MJ, Scharf SJ, Rowe JR, Neme de Gimenez MH, Meske LM, Erlich HA, et al. A diabetes-susceptible HLA haplotype is best defined by a combination of HLA-DR and -DQ alleles. J Clin Invest (1989) 83(3):830–5. doi: 10.1172/JCI113965
9. Erlich HA, Zeidler A, Chang J, Shaw S, Raffel LJ, Klitz W, et al. HLA class II alleles and susceptibility and resistance to insulin dependent diabetes mellitus in Mexican-American families. Nat Genet (1993) 3(4):358–64. doi: 10.1038/ng0493-358
10. Katsarou A, Gudbjornsdottir S, Rawshani A, Dabelea D, Bonifacio E, Anderson BJ, et al. Type 1 diabetes mellitus. Nat Rev Dis Primers (2017) 3:17016. doi: 10.1038/nrdp.2017.16
11. Noble JA. Immunogenetics of type 1 diabetes: A comprehensive review. J Autoimmun (2015) 64:101–12. doi: 10.1016/j.jaut.2015.07.014
12. Cucca F, Muntoni F, Lampis R, Frau F, Argiolas L, Silvetti M, et al. Combinations of specific DRB1, DQA1, DQB1 haplotypes are associated with insulin-dependent diabetes mellitus in Sardinia. Hum Immunol (1993) 37(2):85–94. doi: 10.1016/0198-8859(93)90146-R
13. Cucca F, Lampis R, Frau F, Macis D, Angius E, Masile P, et al. The distribution of DR4 haplotypes in Sardinia suggests a primary association of type I diabetes with DRB1 and DQB1 loci. Hum Immunol (1995) 43(4):301–8. doi: 10.1016/0198-8859(95)00042-3
14. Abid Kamoun H, Hmida S, Kaabi H, Abid A, Slimane Houissa H, Maamar M, et al. HLA polymorphism in type 1 diabetes tunisians. Ann Genet (2002) 45(1):45–50. doi: 10.1016/S0003-3995(02)01104-8
15. Hu X, Deutsch AJ, Lenz TL, Onengut-Gumuscu S, Han B, Chen WM, et al. Additive and interaction effects at three amino acid positions in HLA-DQ and HLA-DR molecules drive type 1 diabetes risk. Nat Genet (2015) 47(8):898–905. doi: 10.1038/ng.3353
16. Cucca F, Lampis R, Congia M, Angius E, Nutland S, Bain SC, et al. A correlation between the relative predisposition of MHC class II alleles to type 1 diabetes and the structure of their proteins. Hum Mol Genet (2001) 10(19):2025–37. doi: 10.1093/hmg/10.19.2025
17. Di Lorenzo TP, Peakman M, Roep BO. Translational mini-review series on type 1 diabetes: Systematic analysis of T cell epitopes in autoimmune diabetes. Clin Exp Immunol (2007) 148(1):1–16. doi: 10.1111/j.1365-2249.2006.03244.x
18. Pugliese A. Autoreactive T cells in type 1 diabetes. J Clin Invest (2017) 127(8):2881–91. doi: 10.1172/JCI94549
19. Regnell SE, Lernmark A. Early prediction of autoimmune (type 1) diabetes. Diabetologia (2017) 60(8):1370–81. doi: 10.1007/s00125-017-4308-1
20. James EA, Pietropaolo M, Mamula MJ. Immune recognition of beta-cells: Neoepitopes as key players in the loss of tolerance. Diabetes (2018) 67(6):1035–42. doi: 10.2337/dbi17-0030
21. Rodriguez-Calvo T, Johnson JD, Overbergh L, Dunne JL. Neoepitopes in type 1 diabetes: Etiological insights, biomarkers and therapeutic targets. Front Immunol (2021) 12:667989. doi: 10.3389/fimmu.2021.667989
22. Ott PA, Dittrich MT, Herzog BA, Guerkov R, Gottlieb PA, Putnam AL, et al. T Cells recognize multiple GAD65 and proinsulin epitopes in human type 1 diabetes, suggesting determinant spreading. J Clin Immunol (2004) 24(4):327–39. doi: 10.1023/B:JOCI.0000029120.77824.41
23. Mannering SI, Morris JS, Stone NL, Jensen KP, PM VANE, Harrison LC. CD4+ T cell proliferation in response to GAD and proinsulin in healthy, pre-diabetic, and diabetic donors. Ann N Y Acad Sci (2004) 1037:16–21. doi: 10.1196/annals.1337.003
24. Culina S, Lalanne AI, Afonso G, Cerosaletti K, Pinto S, Sebastiani G, et al. Islet-reactive CD8(+) T cell frequencies in the pancreas, but not in blood, distinguish type 1 diabetic patients from healthy donors. Sci Immunol (2018) 3(20). doi: 10.1126/sciimmunol.aao4013
25. Sonderstrup G, Cope AP, Patel S, Congia M, Hain N, Hall FC, et al. HLA class II transgenic mice: models of the human CD4+ T-cell immune response. Immunol Rev (1999) 172:335–43. doi: 10.1111/j.1600-065X.1999.tb01377.x
26. Patel SD, Cope AP, Congia M, Chen TT, Kim E, Fugger L, et al. Identification of immunodominant T cell epitopes of human glutamic acid decarboxylase 65 by using HLA-DR(α1*0101,β1*0401) transgenic mice. Proc Natl Acad Sci USA (1997) 94(15):8082–7. doi: 10.1073/pnas.94.15.8082
27. Congia M, Patel S, Cope AP, De Virgiliis S, Sønderstrup G. T Cell epitopes of insulin defined in HLA-DR4 transgenic mice are derived from preproinsulin and proinsulin. Proc Natl Acad Sci USA (1998) 95(7):3833–8. doi: 10.1073/pnas.95.7.3833
28. Herman AE, Tisch RM, Patel SD, Parry SL, Olson J, Noble JA, et al. Determination of glutamic acid decarboxylase 65 peptides presented by the type I diabetes-associated HLA-DQ8 class II molecule identifies an immunogenic peptide motif. J Immunol (1999) 163(11):6275–82. doi: 10.4049/jimmunol.163.11.6275
29. Verhagen J, Yusuf N, Smith EL, Whettlock EM, Naran K, Arif S, et al. Proinsulin peptide promotes autoimmune diabetes in a novel HLA-DR3-DQ2-transgenic murine model of spontaneous disease. Diabetologia (2019) 62(12):2252–61. doi: 10.1007/s00125-019-04994-8
30. Cousens LP, Su Y, McClaine E, Li X, Terry F, Smith R, et al. Application of IgG-derived natural treg epitopes (IgG tregitopes) to antigen-specific tolerance induction in a murine model of type 1 diabetes. J Diabetes Res (2013) 2013:621693. doi: 10.1155/2013/621693
31. So M, Elso CM, Tresoldi E, Pakusch M, Pathiraj V, Wentworth JM, et al. Proinsulin c-peptide is an autoantigen in people with type 1 diabetes. Proc Natl Acad Sci USA (2018) 115(42):10732–7. doi: 10.1073/pnas.1809208115
32. Ihantola E-L, Ilmonen H, Kailaanmäki A, Rytkönen-Nissinen M, Azam A, Maillère B, et al. Characterization of proinsulin T cell epitopes restricted by type 1 diabetes–associated HLA class II molecules. J Immunol (2020) 204(9):2349–59. doi: 10.4049/jimmunol.1901079
33. Raju R, Munn SR, David CS. T Cell recognition of human pre-proinsulin peptides depends on the polymorphism at HLA DQ locus: A study using HLA DQ8 and DQ6 transgenic mice. Hum Immunol (1997) 58(1):21–9. doi: 10.1016/S0198-8859(97)00212-7
34. Lampis R, Morelli L, Congia M, Macis MD, Mulargia A, Loddo M, et al. The inter-regional distribution of HLA class II haplotypes indicates the suitability of the sardinian population for case-control association studies in complex diseases. Hum Mol Genet (2000) 9(20):2959–65. doi: 10.1093/hmg/9.20.2959
35. James EA, Mallone R, Kent SC, DiLorenzo TP. T-Cell epitopes and neo-epitopes in type 1 diabetes: A comprehensive update and reappraisal. Diabetes (2020) 69(7):1311–35. doi: 10.2337/dbi19-0022
36. Schirru E, Jores RD, Rossino R, Corpino M, Cucca F, Congia M. Low-risk human leukocyte antigen genes and mild villous atrophy typify celiac disease with immunoglobulin a deficiency. J Pediatr Gastroenterol Nutr (2021) 72(6):889–93. doi: 10.1097/MPG.0000000000003129
37. Songini M, Bernardinelli L, Clayton D, Montomoli C, Pascutto C, Ghislandi M, et al. The sardinian IDDM study: 1. epidemiology and geographical distribution of IDDM in Sardinia during 1989 to 1994. Diabetologia (1998) 41(2):221–7. doi: 10.1007/s001250050893
38. Luce S, Lemonnier F, Briand JP, Coste J, Lahlou N, Muller S, et al. Single insulin-specific CD8+ T cells show characteristic gene expression profiles in human type 1 diabetes. Diabetes (2011) 60(12):3289–99. doi: 10.2337/db11-0270
39. Anderson AM, Landry LG, Alkanani AA, Pyle L, Powers AC, Atkinson MA, et al. Human islet T cells are highly reactive to preproinsulin in type 1 diabetes. Proc Natl Acad Sci USA (2021) 118(41). doi: 10.1073/pnas.2107208118
40. Alleva DG, Crowe PD, Jin L, Kwok WW, Ling N, Gottschalk M, et al. A disease-associated cellular immune response in type 1 diabetics to an immunodominant epitope of insulin. J Clin Invest (2001) 107(2):173–80. doi: 10.1172/JCI8525
41. Spanier JA, Sahli NL, Wilson JC, Martinov T, Dileepan T, Burrack AL, et al. Increased effector memory insulin-specific CD4(+) T cells correlate with insulin autoantibodies in patients with recent-onset type 1 diabetes. Diabetes (2017) 66(12):3051–60. doi: 10.2337/db17-0666
42. Yang J, Chow IT, Sosinowski T, Torres-Chinn N, Greenbaum CJ, James EA, et al. Autoreactive T cells specific for insulin B:11-23 recognize a low-affinity peptide register in human subjects with autoimmune diabetes. Proc Natl Acad Sci USA (2014) 111(41):14840–5. doi: 10.1073/pnas.1416864111
43. Arif S, Gomez-Tourino I, Kamra Y, Pujol-Autonell I, Hanton E, Tree T, et al. GAD-alum immunotherapy in type 1 diabetes expands bifunctional Th1/Th2 autoreactive CD4 T cells. Diabetologia (2020) 63(6):1186–98. doi: 10.1007/s00125-020-05130-7
44. Bonifacio E, Lampasona V, Bernasconi L, Ziegler AG. Maturation of the humoral autoimmune response to epitopes of GAD in preclinical childhood type 1 diabetes. Diabetes (2000) 49(2):202–8. doi: 10.2337/diabetes.49.2.202
45. Ronkainen MS, Savola K, Knip M. Antibodies to GAD65 epitopes at diagnosis and over the first 10 years of clinical type 1 diabetes mellitus. Scand J Immunol (2004) 59(3):334–40. doi: 10.1111/j.0300-9475.2004.01402.x
46. Sohnlein P, Muller M, Syren K, Hartmann U, Bohm BO, Meinck HM, et al. Epitope spreading and a varying but not disease-specific GAD65 antibody response in type I diabetes. the childhood diabetes in Finland study group. Diabetologia (2000) 43(2):210–7. doi: 10.1007/s001250050031
47. Krischer JP, Lynch KF, Schatz DA, Ilonen J, Lernmark A, Hagopian WA, et al. The 6 year incidence of diabetes-associated autoantibodies in genetically at-risk children: The TEDDY study. Diabetologia (2015) 58(5):980–7. doi: 10.1007/s00125-015-3514-y
48. Liu J, Purdy LE, Rabinovitch S, Jevnikar AM, Elliott JF. Major DQ8-restricted T-cell epitopes for human GAD65 mapped using human CD4, DQA1*0301, DQB1*0302 transgenic IA(null) NOD mice. Diabetes (1999) 48(3):469–77. doi: 10.2337/diabetes.48.3.469
49. Chao CC, McDevitt HO. Identification of immunogenic epitopes of GAD 65 presented by Ag7 in non-obese diabetic mice. Immunogenetics (1997) 46(1):29–34. doi: 10.1007/s002510050238
50. Kim SK, Tarbell KV, Sanna M, Vadeboncoeur M, Warganich T, Lee M, et al. Prevention of type I diabetes transfer by glutamic acid decarboxylase 65 peptide 206-220-specific T cells. Proc Natl Acad Sci USA (2004) 101(39):14204–9. doi: 10.1073/pnas.0405500101
Keywords: children, type 1 diabetes mellitus, epitopes, glutamate decarboxylase, preproinsulin, human leukocyte antigen, Sardinia
Citation: Jores RD, Baldera D, Schirru E, Muntoni S, Rossino R, Manchinu MF, Marongiu MF, Caria CA, Ripoli C, Ricciardi MR, Cucca F and Congia M (2023) Peripheral blood mononuclear cells reactivity in recent-onset type I diabetes patients is directed against the leader peptide of preproinsulin, GAD65271-285 and GAD65431-450. Front. Immunol. 14:1130019. doi: 10.3389/fimmu.2023.1130019
Received: 22 December 2022; Accepted: 27 February 2023;
Published: 09 March 2023.
Edited by:
Maki Nakayama, University of Colorado, United StatesReviewed by:
Eddie A. James, Benaroya Research Institute, United StatesAaron Michels, University of Colorado, United States
Copyright © 2023 Jores, Baldera, Schirru, Muntoni, Rossino, Manchinu, Marongiu, Caria, Ripoli, Ricciardi, Cucca and Congia. This is an open-access article distributed under the terms of the Creative Commons Attribution License (CC BY). The use, distribution or reproduction in other forums is permitted, provided the original author(s) and the copyright owner(s) are credited and that the original publication in this journal is cited, in accordance with accepted academic practice. No use, distribution or reproduction is permitted which does not comply with these terms.
*Correspondence: Mauro Congia, bWF1cm9jb25naWExOTU3QGdtYWlsLmNvbQ==