- 1Key Laboratory of Radiation Oncology of Taizhou, Radiation Oncology Institute of Enze Medical Health Academy, Department of Radiation Oncology, Taizhou Hospital Affiliated to Wenzhou Medical University, Taizhou, Zhejiang, China
- 2Department of Radiation Oncology, Xi’an No. 3 Hospital, The Affiliated Hospital of Northwest University, Xi’an, Shaanxi, China
The high primary resistance incidence and unavoidable secondary resistance are the major clinical obstacle to lasting long-term benefits in Non-small-cell lung cancer (NSCLC) patients treated with immunotherapy. The mechanisms of immunotherapy resistance in NSCLC are complex, mainly involving tumor cells and tumor microenvironment (TME) infiltrating immune cells, including TAMs, B cells, NK cells, and T cells. The selection of clinical strategies for NSCLC progression after immunotherapy resistance should depend on the progressive mode. The progression pattern of NSCLC patients after immunotherapy resistance can be divided into oligo-progression and systemic/multiple progression, which should be considered for further treatment selection. In the future, it needs to explore how to optimize the combined therapy and explore strategies to reprogram infiltrating immune cells under various genetic backgrounds of tumor cells and timely reshape TME during antitumor treatments.
1 Introduction
Lung cancer is one of the malignant tumors with the high incidence rate and mortality in the world (1). Non-small-cell lung cancer (NSCLC) accounts for 80% of lung cancer, which mainly includes squamous cell carcinoma (LUSC) and adenocarcinoma (LUAD) (2). The development of immunotherapy profoundly initiates a new era of antitumor treatment, and single or combination immunotherapy has been applicated as the first- and second-line treatment strategies for NSCLC. Inhibitors against programmed death protein 1 (PD-1)/its ligand (PD-L1) or cytotoxic T lymphocyte antigen 4 (CTLA-4) are the most classical and widely applicated immune checkpoint inhibitors (ICIs). Although ICIs profoundly improve overall survival in NSCLC patients with I-IV stages (3–7), there is a non-all-patient response to immunotherapy. Oncologists and NSCLC patients inevitably face the challenge of immunotherapy primary-, secondary-resistance, and progression after treatment discontinuation. As recommended by the first meeting of the SITC Immunotherapy Resistance Taskforce, patients with primary resistance showed that the tumor evaluation after <6 weeks of immunotherapy was progress disease (PD) or stable disease (SD), while secondary resistance was defined as that tumor response to immunotherapy reached complete response (CR), partial response(PR), or SD ≥6 months, and then PD confirmed by imaging scan (8). Explore resolution strategies for improving tumor response to immunotherapy will bring a new leap in NSCLC prognosis. The mechanism of immunotherapy resistance is complex, dynamic, and interdependent. A prerequisite for a clinical response to immunotherapy is a normal cancer-immunity cycle(CIC), which comprises the release of cancer cell antigen, cancer antigen presentation by dendritic cells/APCs, priming and activation of APCs and T cells, trafficking of T cells to tumors, infiltration of T cells into tumors, recognition of cancer cells by T cells, and immune-mediated cancer cells killing (9). ICIs application can block the inhibitory signal of T cell activation, which is only one step of completed CIC. One or more steps of the CIC are interrupted to enable tumors to evade immunosurveillance, and immunotherapy fails to activate effective antitumor immunity (10). During the CIC process, the regulation in the recruitment and infiltration of T cells and cancer cell antigen releasement contribute to the remodeling tumor microenvironment (TME). It also plays a pivotal role in antitumor therapeutic efficacy, except in affecting tumor progression (11). TME is a complex and dynamic changed microenvironment comprising endothelial cells, fibroblasts, immune cells, etc., infiltrating with cytokines, growth factors, hormones, extracellular matrix, etc., and nourishing by the surrounding tumor vascular (11). The TME may dynamically converse between the immunosuppressive TME and the immune-active TME. In addition, the inflammatory status, gut microbiome, diet, etc. of tumor hosts have been demonstrated to be associated with primary and secondary resistance to ICIs (12). The immunological condition of hosts also can affect cells within TME through systemic or local ways (12). Therefore, focusing on the mechanism and drug of TME modulation may be the breakthrough point for reversing immunotherapy resistance. In this review, we focus on the current clinical predicament of immunotherapy resistance in NSCLC, the role of CIC, especially TME, in immunomodulation, and potential strategies to reverse immunotherapy resistance.
2 The characteristics of immunotherapy resistance for NSCLC
2.1 Primary resistance to immunotherapy
Phase III randomized trials (CheckMate 017 and CheckMate 057) were designed to compare Nivolumab with chemotherapy in the second-line treatment of NSCLC patients with progression after standard first-line treatment (13). The best overall response (BOR) analysis showed that patients with PD accounted for over 40%, and these patients could be defined as primary resistance to ICIs (13). OAK trial also showed that accounting for 44% of NSCLC patients treated with atezolizumab as second-line treatment developed PD (14). For ICI alone or combined with another ICI as first-line treatment, 21%~27% of NSCLC patients have primary resistance to immunotherapy (15–17). For ICI combined with chemotherapy as first-line treatment, the incidence of primary resistance to ICIs in NSCLC patients was around 10% (18–20). As shown in Figure 1, the incidence of primary resistance to immunotherapy in NSCLC patients previously treated with standard chemotherapy was higher than that in NSCLC patients treated with ICIs as first-line treatment. Moreover, a lower incidence of primary resistance to ICIs appeared in NSCLC patients treated with immunotherapy plus chemotherapy. This phenomenon may result from the fact that various chemotherapeutic agents can induce immunogenic cell death (ICD) (21). As we know, ICD can enhance the release of cancer cell antigens, a step of a normal cancer-immunity cycle, to improve an anticancer immune response (21, 22).
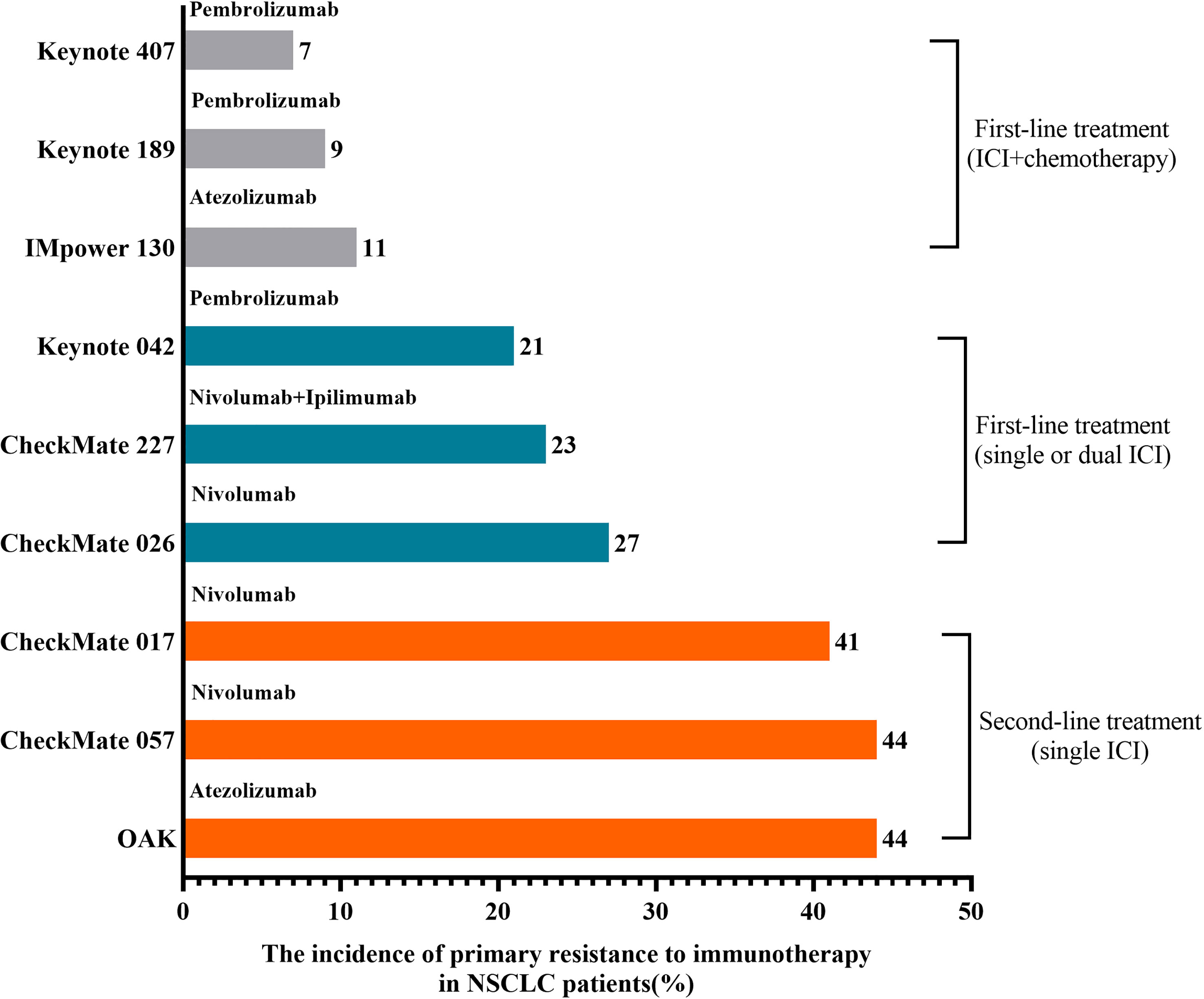
Figure 1 The incidence of primary resistance to immunotherapy in NSCLC patients. The highest incidence of primary resistance to ICIs was found in patients treated with ICIs as second-line treatment after chemotherapy failure, while the lowest incidence was found in NSCLC patients previously treated with immunotherapy plus chemotherapy.
2.2 Secondary resistance to immunotherapy
Acquired resistance was defined as having an initial response to ICIs over a while and ultimately happening to disease progression (23). A pooled analysis from clinical studies in advanced NSCLC patients treated with nivolumab showed that up to 65% of initial responders developed the progressive disease at 4 years of follow-up (24). As shown in Figure 2, ICIs applied as first- and second-line treatment for NSCLC patients with rates of secondary resistance was 52%-57% and 32%-64%, respectively (25). In addition, a retrospective study of 1201 NSCLC patients treated with PD-1 inhibitors at Memorial Sloan Kettering Cancer Center (MSKCC) found that 78% of 243 cases acquired an initial response to immunotherapy and further developed secondary resistance (26). With the prolonged response time or progression-free survival (PFS), the occurrence rate of secondary resistance declined with the duration of remission in NSCLC patients treated with ICIs (26). The 1-year incidence rate was 53%, with 37% at 1-2 years and 10% after 2 years (26). Compared to systematic progression, the most common pattern of secondary resistance to ICIs in NSCLC patients was oligo progression, defined as ≤ 2 progressed disease sites (26–28). And NSCLC patients with oligo progression after treating PD-1 inhibitors occurred secondary resistance later than patients with systematic progression and had a better survival prognosis (26). In the second-line and above treatment for NSCLC patients, an earlier or higher objective response rate (ORR) of a single ICI was accompanied by a lower incidence of secondary resistance and more significant long-term survival benefits (24, 25).
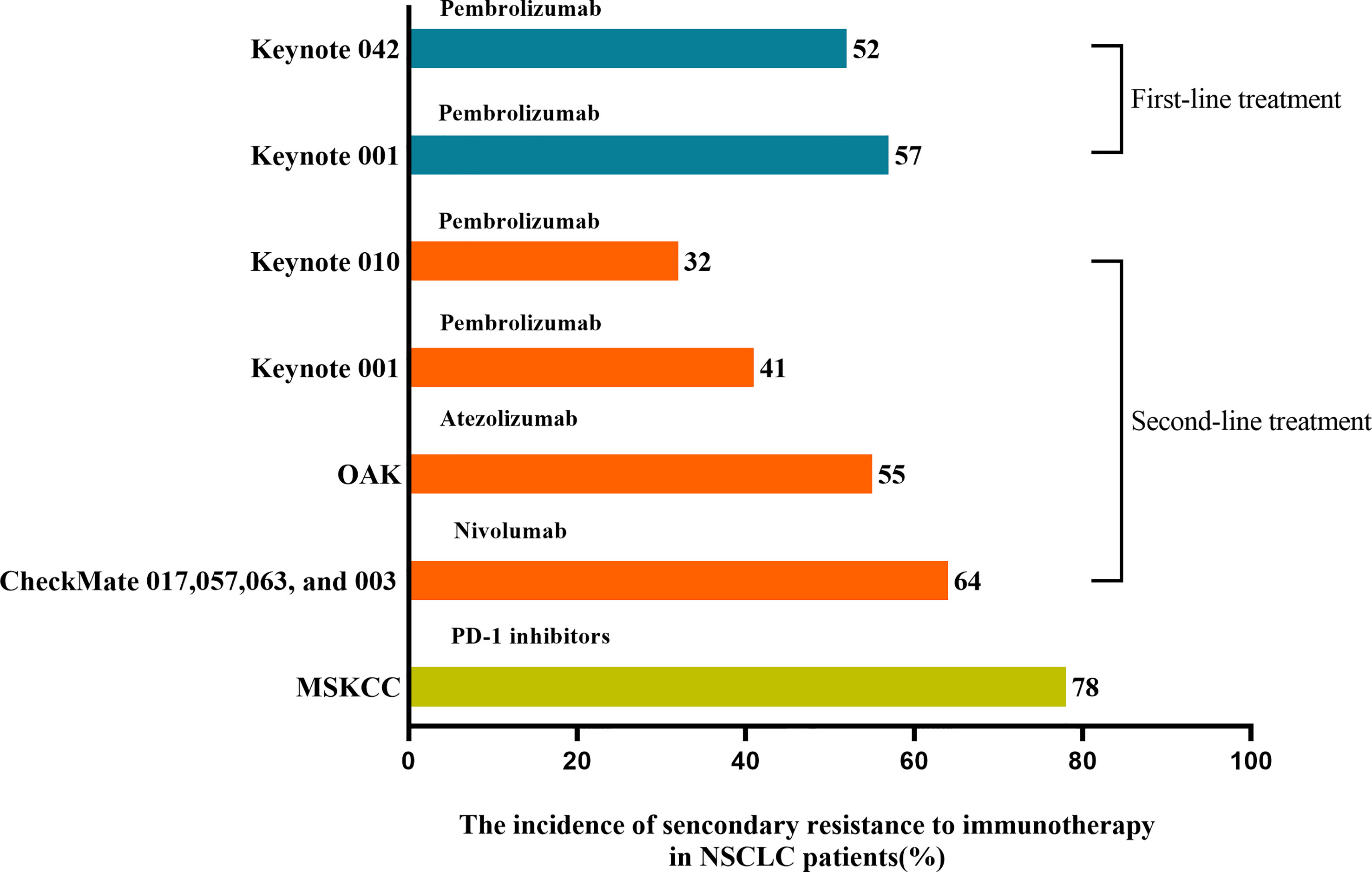
Figure 2 The incidence of secondary resistance to immunotherapy in NSCLC patients. ICIs applied as first- and second-line treatment in treating NSCLC patients resulted in 52%-57% and 32%-64% rates of secondary resistance, respectively. MSKCC found that 78% of patients who acquired an initial response to PD-1 inhibitors further developed secondary resistance.
In summary, tumors in primary resistance NSCLC patients may consist of no or only a few sensitive tumor cells and resistive tumor cells to immunotherapy, which may present no active immune response(PD) or activate antitumor immune response followed by swiftly submerging by intricated mechanism (SD<6 months). When tumors are comprised of no or only a few resistive tumor cells and sensitive tumor cells to immunotherapy, NSCLC patients will show a favorable and lasting response to immunotherapy(CR, PR, SD>6 months). However, some will form secondary resistance when tumor cells lose active response to immunotherapy by complicated mechanisms (Figure 3).
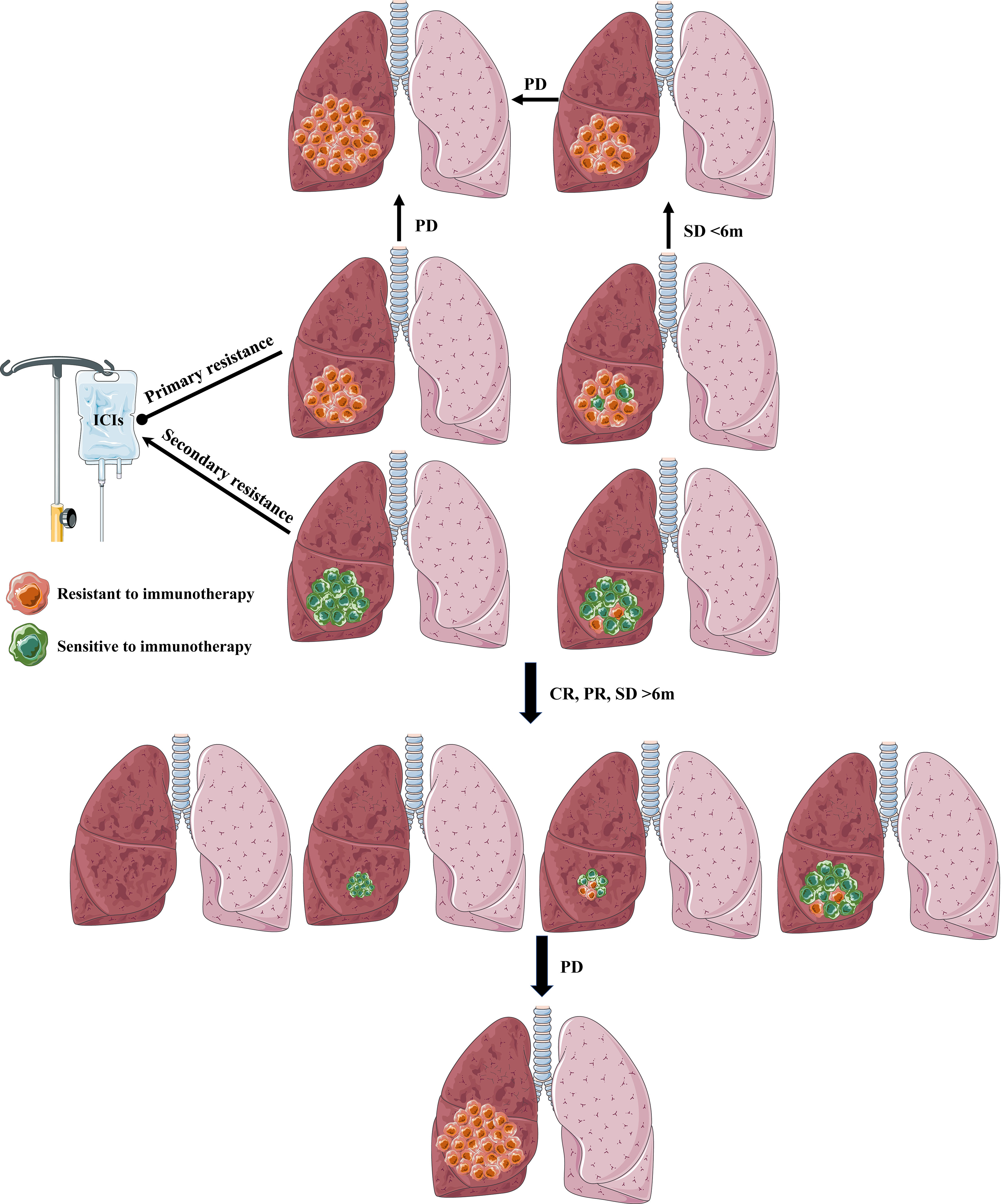
Figure 3 The definition and explanation of primary resistance and secondary resistance. Primary resistance: tumors in primary resistance NSCLC patients may contain no or only a few sensitive tumor cells to immunotherapy, which may present no active immune response (PD) or activate antitumor immune response followed by swiftly submerging by intricated mechanism (SD<6 months). Secondary resistance: When tumors contain no or only a few resistive tumor cells to immunotherapy, NSCLC patients will show a favorable and lasting response (CR, PR, SD) to immunotherapy (>6 months). However, some will form secondary resistance when tumor cells lose active response to immunotherapy by complicated mechanisms.
3 Mechanisms of immunotherapy resistance in NSCLC
A normal CIC, a prerequisite for response to immunotherapy, can be summarized in four steps: recognizable tumor antigen release (within tumor cells); immune cells identify, transmit tumor antigen, and then are activated (system of the tumor-bearing host); recruiting, trafficking, and infiltration of immune cells into the TME (outside of tumor cells); Finally, triggering immune-mediated cancer cell killing(TME, host). A deficit in any one of these four steps will contribute to varying degrees of immunotherapy resistance (Figure 4).
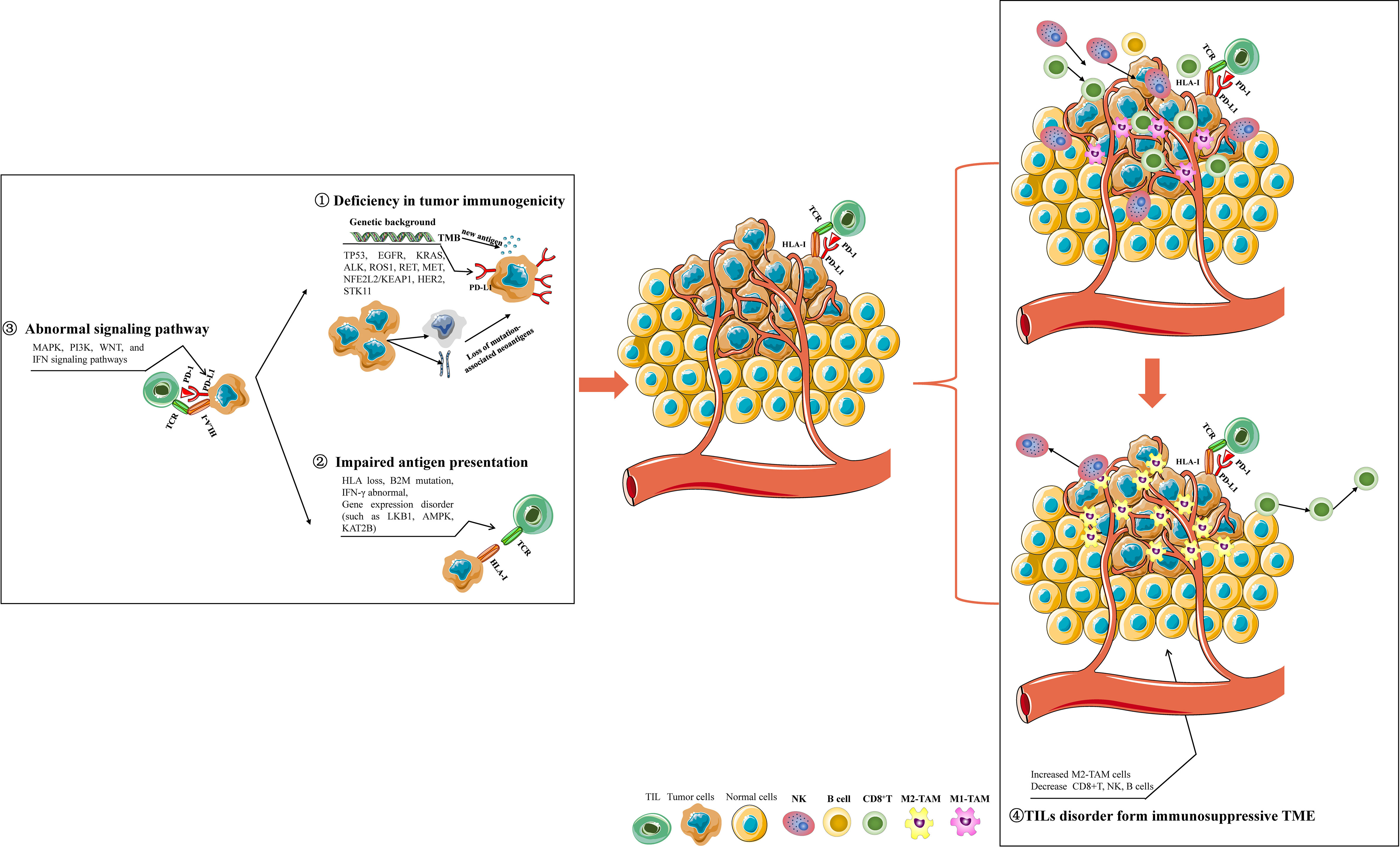
Figure 4 The mechanisms involved in immunotherapy resistance of NSCLC. Deficiency in tumor immunogenicity: various drive gene mutations affect PD-L1 expression, TMB, and tumor-specific neoantigen formation; Impaired antigen presentation: HLA loss, B2M mutation, IFN-γ signaling dysregulation, and some genes disorder; Abnormal signaling pathway: abnormal MAPK, PI3K, WNT, and IFN signaling pathways may influence tumor immunogenicity and antigen presentation; Abnormal activity and infiltration of immune cells in NSCLC TME: NSCLC TME enriched with increased M2 macrophages, decreased B cells, and NK cells form an immunosuppressive TME to resist the initiation of antitumor immunity. Some gene expression disorders or mutations in NSCLC cells can impair the activity, tumor-cell-killing function, proliferation, and infiltration of CD8+ T-cells in TME, which contribute to exhausted CD8+ T-cells for immunotherapy resistance.
3.1 Mechanisms involved within NSCLC cells
3.1.1 Deficiency in tumor immunogenicity
PD-L1 expression and tumor mutation burden (TMB) are the most popularly applied biomarkers for predicting immunotherapy response. NSCLC patients with a high expression of PD-L1 usually obtain better benefits from anti-PD-1/PD-L1 therapy (29, 30). NSCLC patients with a higher tumor tissue TMB (tTMB) are correlated with a more effective response to PD-1/PD-L1 inhibitors by leading to tumor-specific neoantigen formation to elevate tumor immunogenicity (31–34). Therefore, any intrinsic and extrinsic factors affecting the expression of PD-L1 or/and TMB in tumor cells may be the mechanism for resistance to immunotherapy. Next-generation sequencing (NGS) applications found that innate drive gene mutations affect PD-L1 expression and TMB, which may contribute to primary or adaptive resistance to immunotherapy in NSCLC. EGFR is a well-studied drive gene in NSCLC patients, the mutation status of which can induce PD-L1 expression (35, 36). In EGFR-mutated NSCLC cells, activated EGFR was found to elevate PD-L1 expression via the IL-6/JAK/STAT3 signaling or p-ERK1/2/p-c-Jun pathway (35, 36). Moreover, NSCLC patients with uncommon EGFR mutation, including G719X, L861Q, S768I, and Ex20 ins, had more abundant PD-L1 expression accompanied by CD8+ tumor-infiltrating lymphocytes(TILs) infiltration (37). In addition, mutations of RET and HER2 attenuated PD-L1 expression, while mutations of ALK, ROS, and MET enhanced PD-L1 expression but downregulated TMB and TIL infiltration leading to resistance to ICIs (38–41). TP53 or NFE2L2/KEAP1 mutations could increase TMB and PD-L1 expression to enhance the sensibility to immunotherapy (42, 43). However, NSCLC subsets with co-occurrence of EGFR, HER2, ALK, ROS1, RET, and MET mutations get minimal benefit from ICIs despite high PD-L1 expression. These findings confirmed a TMB/PD-L1-independent effect on response sensitivity to ICIs for specific drive genes mutations (44). KRAS mutation, another frequently mutated type in NSCLC, usually co-occurs with different gene mutations. Various KRAS mutation subtypes have distinct TMB and PD-L1 expressions, and KRAS G12C is the most common subtype with a high rate of PD-L1 positive (45). Mutated-TP53 was more prevalent in KRAS wild-type NSCLC, while mutated-STK11 was more frequently found in KRAS-mutated NSCLC (45). In KRAS-mutant lung adenocarcinoma, STK11 mutation was identified as the most common genomic background for primary resistance to PD-1/PD-L1 inhibitors (46). Interestingly, the acquired resistance after initial response to ICIs in NSCLC patients showed the landscape of genomic changes characterized by loss of putative mutation-associated neoantigens through eliminating tumor subclones or deleting specific chromosomal regions (47). Moreover, High TMB and neoantigen burden(APOBEC, IFNGR1, or VTCN1 mutation) were associated with enhanced efficacy response in NSCLC immunotherapy, but PTEN mutation was associated with non-response to immunotherapy (34).
3.1.2 Impaired antigen presentation
Impaired antigen processing and presentation have been confirmed as a mechanism for lung cancer with acquired resistance to ICIs (48, 49). Class I human leukocyte antigen (HLA-I/MHC-I) plays a leading role in neoantigen presentation to improve tumor recognition by T cell receptors. HLA gene loss damages the process of neoantigen presentation resulting in immune evasion for tumors (50). The germline HLA-I evolutionary divergence was strongly associated with the survival benefit of metastatic melanoma or NSCLC patients treated with anti-CTLA-4 or anti-PD-1/-PD-L1 (51). 40% of NSCLC carry allele-Specific HLA Loss(HLA-I LOH), which is related to a raised neoantigen burden, PD-L1 positivity, and poor response to ICIs treatment (52, 53). Loss of the B2M gene, an essential chaperone for HLA-I-mediated antigen presentation, formed an immunosuppressive TME characterized by reduced TIL infiltration and conferred resistance to ICIs in NSCLC (49, 54, 55). Interferon-gamma (IFN-γ) has also been identified to stimulate the expression of HLA on NSCLC cells (56, 57). Except for the role of IFN-γ in HLA regulation, IFN-γ signaling is also critical for the initiation of PD-L1 expression in cancer and host cells (58, 59). An analysis of gene expression profiles for pembrolizumab-treated patients found that IFN-γ-related mRNA profile could predict clinical response to PD-1 inhibitor (60). A prospective study about cytokine profiles in NSCLC patients receiving ICI treatment in the second line revealed that patients with elevated expression of IFN-γ significantly benefit from PD-1 blockers (61). Moreover, attenuated antigen presentation was also found in NSCLC with compromised LKB1 and AMPK activity (62). Low expression of KAT2B concurrent with a higher frequency of somatic genes mutation was associated with lower response efficacy to ICIs in NSCLC patients, while KAT2B was linked to IFN-γ regulation, antigen processing, and presentation (63).
3.1.3 Abnormal signaling pathway
It has been reported that the aberrations of MAPK, PI3K, WNT, and IFN signaling pathways may be implicated in the resistance mechanisms of lung cancer immunotherapy (12). As discussed above, genetic mutation EGFR influences tumor immunogenicity by regulating PD-L1 expression and TMB. MAPK and PI3K pathways were involved in the immunotherapy resistance mechanism mediated by EGFR mutation-induced PD-L1 increasing (64). MAPK and PI3K pathways were downstream of the RAS signaling, the activation of which supported intrinsic PD-L1 expression (65, 66). BRAF mutation is a rare form of NSCLC and is part of the MAPK pathway. And BRAF mutation seems to be more associated with high expression of PD-L1(PD-L1 expression ≥50%) than other subtypes (67). In addition, the activity of the MAPK pathway was significant for EGF- and IFNγ-induced PD-L1 expression, contributing to improving response to ICIs in NSCLC (68). The PI3K/AKT/mTOR pathway plays critical roles in multiple biological functions or processes of cancers, and it can be activated by the genetic mutation of EGFR or KRAS in NSCLC (69–71). Significantly, uncontrolled activation of the PI3K/AKT/mTOR pathway can modulate the response to ICIs by driving PD-L1 expression and remodeling the infiltration and function of TIL (71, 72). WNT signaling alterations in NSCLC were also associated with PD-L1 negativity but this altered PD-L1 expression without predictive value for ICIs (73). However, a recent study identified that SCD1-related fatty acids in serum were correlated with the response efficiency of NSCLC patients treated with a PD-1 inhibitor, while WNT signaling was significantly involved in the immunomodulatory function of SCD1 (74). The loss of IFN signaling in tumor cells has been highlighted as a mechanism of the primary and acquired resistance to ICIs in cancer patients (75–77). The production of IFN in TME could induce PD-L1 expression on the surface of tumor cell lines, including NSCLC (78–80). There is still a lack of evidence about the relation between the genomic alterations in IFN signaling and response to ICIs treatment in NSCLC patients.
3.2 Mechanisms involved in NSCLC TME
Recruiting, trafficking and infiltration of immune cells into the TME are essential to a normal CIC. The TME comprised TILs and stromal cells, cytokines, and vasculature, which influence response to immunotherapy by dynamically reshaping the immunogenicity of TME. Immunosuppressive TME formation is a leading mechanism of immunotherapy resistance and is a vital breach to enhance the effective response to immunotherapy. We will focus on the TILs in NSCLC TME to give insights into the immunotherapy resistance mechanisms. A landscape of the TILs in NSCLC TME identified that CD4+ T cells were the maximum T cell population, followed by CD8+ T cells, and then B cells, macrophages, natural killer (NK) cells, and dendritic cells (DCs) in order (81).
3.2.1 M2-TAMs
Macrophages within the TME are designated as tumor-associated macrophages (TAMs). TAMs are the fundamental components within the TME, which perform a crucial function in the immunity remodeling of TME and affect response to immunotherapy (82, 83). A recent single-cell RNA sequencing revealed that the temporal and spatial distribution of macrophages was diverse in the TME of NSCLC and assisted tumor immune escape by initiating regulatory T-cell response (84). There are two classically polarized phenotypes of macrophages, including M1 (immune-activated type) and M2 (immunosuppressive type), and the latter is the main type of TAMs (72). TME enriched with more M2 macrophages is significantly associated with a worse response rate and prognosis for NSCLC patients receiving immunotherapy (85). However, TAMs can be re-engineered into M1-type to increase the response to ICIs treatment (86). In NSCLC, TAMs could promote tumor cell glycolysis by TNFα secretion and facilitate tumor hypoxia by increasing AMPK and PGC-1α, leading to decreased PD-L1 of tumor cells and T-cell infiltration in TME to cause immunotherapy resistance (87). Surprisingly, NSCLC patients enriched with PD-L1+ TAM in TME represented better survival for receiving PD-1/PD-L1 blockers (88). Additionally, another study suggested that PD-L1 mainly plays an effect in forming an immunosuppressive M2-type TAM (89). However, M2-type TAM could be reprogrammed into an immune-activated type by anti-PD-L1 treatment but not anti-PD-1 (89). Many genes or signaling pathways are involved in targeting TAM recruitment, activation, and survival, which can be applied as a targeting strategy to improve tumor immunotherapy (90, 91).
3.2.2 NK cells
NK cells are powerful innate immune cells. NK cells perform a direct tumor-killing effect and indirectly enhance antitumor immunity mediated by T cells. Additionally, NK cells regulate DCs, macrophages, and neutrophils through cytotoxicity and cytokine release (92). Moreover, tumor-infiltrating NK cells could trigger T-cell-mediated immunity by stimulating the recruitment of DCs into TME, conferring improved tumor immune control (93). The infiltration of NK and plasma cells has been defined as a distinct immune subset in NSCLC, contributing to the most favorable prognosis (94). The high infiltration of NK cells in tumor tissue has been confirmed as a biomarker for predicting durable response to ICIs immunotherapy in NSCLC patients (95, 96). It is worth noting that there is a negative relationship between the density of TAMs and NK- and T-cell antitumor activities in NSCLC (97, 98).
3.2.3 B cells
Tumor-infiltrating B cells have been identified as the most differential gene between immunotherapy responders and non-responders in patients with melanoma (99). Tertiary lymphoid structures (TLS) were also used as a marker of efficient immunotherapies due to initiating and/or maintaining local and systemic T- and B-cell mediated antitumor activity (100). B cells and plasma cells were found to co-present in TLS, and the abundance of intra-tumoral B cells was also linked to the prediction in the response efficacy of anti-PD-L1 in NSCLC (101, 102). B cells have also been found to exert antigen-presenting function to CD4+ TILs in TME to influence prognosis in NSCLC immunotherapy (102).
3.2.4 CD4+ and CD8+ T cells
The presence of CD4+ T cells and CD8+ T cells in TME was associated with the objective clinical responses to anti-PD-1/PD-L1 blockade in NSCLC (103–107). And the predictive potency of CD4+ T cells and CD8+ T cells was more prominent in the PD-L1 positive sub-population. PD-1+ CD4+ T-cell was a negative predictor for immunotherapy availability in advanced NSCLC patients, while PD-1+CD8+ T-cell was a positive predictor (85, 103–105, 108). To complicate matters, multiple subsets or activity states of CD4+ T cells and CD8+ T cells have diverse effects on the response to immunotherapy. A single-cell sequencing analyzing T cell composition in NSCLC suggested that patients enriched with “pre-exhausted” CD8+ T cells (CD8-C4-GZMK), non-activated Tregs, and activated CD4+ cells had a much better prognosis than that in patients enriched with exhausted T cells (CD8-C6-LAYN and CD4-C7-CXCL13) and activated Tregs (109). Epigenetic changes-mediated high exhaustion of T cells is an important resistant mechanism to ICIs treatment (91, 110, 111), and therefore the “pre-exhausted” T cells might be alternative target for improving immunotherapy (109). Another study explored that NSCLC cells-derived exosomal circUSP7 could induce CD8+ T cell dysfunction to confer anti-PD-1 resistance (112). Recently, a phase I clinical trial confirmed the safety and feasibility of PD-1-edited T cells in NSCLC (113). Chimeric antigen receptor (CAR)-T cells, as another modified-T cell therapy, has attracted more and more interest in clinical applications as antitumor therapy for various solid tumors, including NSCLC, in recent years (114, 115). In summary, accumulated evidence validated various gene expression disorders or mutations in NSCLC cells impair the activity, tumor-cell-killing function, proliferation, and infiltration of CD8+ T-cells in TME, which contribute to exhausted CD8+ T-cells for immunotherapy resistance (91, 97, 111, 116–118).
4 Current clinical strategies for NSCLC after immunotherapy resistance
The progressive mode in NSCLC patients with immunotherapy resistance can be summarized into oligo-progression and systemic/multiple progression. The failure pattern of oligo-progression occurrence in 20% of NSCLC patients under treatment with PD-1/PD-L1 inhibitors (119). The oligo-progressive lesions are primarily involved in the brain, lung, and lymph nodes for immunotherapy-treated NSCLC patients (119). Clinical treatment selection for NSCLC progression after immunotherapy resistance should depend on the progressive mode (Figure 5).
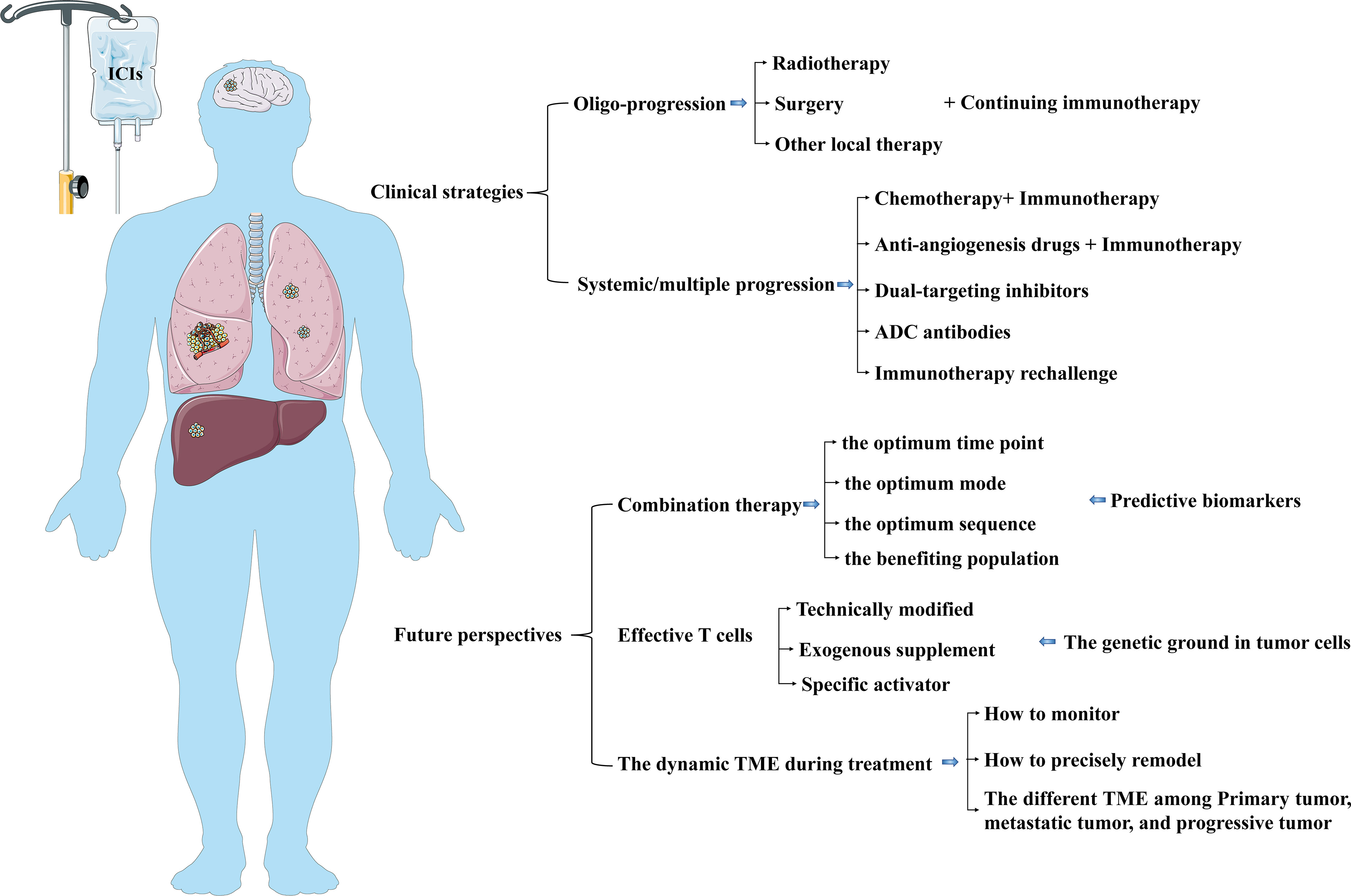
Figure 5 The current clinical strategies and future perspectives in NSCLC patients after immunotherapy resistance. The selection of clinical treatment for NSCLC progression after immunotherapy resistance should be dependent on the progressive mode. Further studies can pay attention to optimizing the combined therapy and exploring strategies to modulate infiltrating immune cells and timely reshape TME.
4.1 Oligo-progression
The general cognition of the term “oligo-progression” is that the progressive metastases are up to 3–5 lesions and limited to 1–3 organs in the premise of a well-controlled metastatic disease (120, 121). Retrospective studies suggested that radiotherapy and/or surgery treating oligo-progressive sites combined with continuing immunotherapy could improve regional control rates and overall survival benefits (122–126). Many NSCLC patients receiving immunotherapy show primary resistance. Local radiation therapy on oligo-metastases improves immunotherapy response for NSCLC patients without multiple progression after primary systemic therapy. Radiotherapy might improve the response to immunotherapy in patients treated with ICI through its immunostimulatory effects and eradicating metastatic deposits (127). Radiation-killing tumor cells promote releasing and presenting tumor antigens, up-regulating PD-L1 expression on tumor cells, increasing TME infiltration of CD8+ T cells, and enhancing T cell-mediated immune response, contributing to improved antitumor immune response (128–130). The PEMBRO-RT trial(a multicenter, randomized phase 2) and MDACC (phase 1/2) trial found that NSCLC with PD-L1-negative tumors could obtain more survival benefits from the addition of radiotherapy than that in patients receiving ICI without radiotherapy (131, 132). For advanced NSCLC patients with oligo-progression or oligo-metastases, continuing immunotherapy combined with local treatment can eliminate primary and acquired resistance to ICIs, improving local control rate(LCR) and overall survival. Except for LCR, the improved overall survival from this combination is due to the distant antitumor effect on metastatic sites out of the radiation field by the radiotherapy-mediated immunoregulator effect. However, there are still many issues to be explored, such as the optimum patient selection, the radiotherapy scheme or fractionated dose selection, the number and location selection of radiation treating metastases, the selection of specific checkpoint inhibitors, and the sequence of radiotherapy combined with immunotherapy. There are many ongoing prospective trials about the effect of radiotherapy combined with immunotherapy in NSCLC patients with limited metastases or who oligo-progressed on ICI treatment or and we are looking forward to awaiting the outcome (127, 133, 134).
4.2 Systemic/multiple progression
4.2.1 Combined therapy
Systemic combination therapies were currently clinically possible strategies for systemic/multiple progression in NSCLC treated with ICIs. BTCRC-LUN15-029(phase 2) suggested that NSCLC patients who progressed after ICIs alone or in combination with chemotherapy could benefit from pembrolizumab plus next-line chemotherapy (135). However, further research is needed to confirm the certain population that can benefit from continued immunotherapy after immunotherapy resistance. Although EGFR/ALK mutation was involved in the mechanism for ICI resistance, EGFR/ALK TKI combination with ICI usually served as a strategy for treating NSCLC patients with acquired EGFR-TKI resistance, not for patients with ICI resistance. However, limited clinical efficacy and a high incidence of treatment-related toxicities did not encourage the further application of this combination. Many clinical trials, such as CheckMate 370 (136), KEYNOTE-021 (137), GEFTREM (138), LUX-Lung-IO (139), have shown that EGFR or ALK inhibitors combined with ICIs were feasible but limited efficacy and more toxicity in treating NSCLC patients with newly diagnosed or refractory or PD after first-line therapy. Furthermore, the growing evidence supported the combination of anti-angiogenic agents and ICIs (140, 141). Ramucirumab combined with pembrolizumab showed an encouraging antitumor activity with acceptable toxicities in NSCLC patients, having progressed on one to three lines of previous therapy (142). A pooled analysis from 23 prospective studies confirmed that ICIs combined with anti-angiogenic agents with favorable antitumor activity and manageable toxic effects might be a new option for NSCLC patients, especially those who received no treatment or chemotherapy intolerance (143). However, especially for treating NSCLC patients with PD after ICI-pretreated, the application of this combination therapy is in the exploring phase. More and more prospective trials have been conducted to identify the efficiency and safety of PD-1/L1 inhibitors plus anti-angiogenesis drugs(Cabozantinib, Sitravatinib, Lenvatinib, Nintedanib) in NSCLC patients previously treated with an ICI (144–150). Cabozantinib is a multi-targeted tyrosine kinase inhibitor (TKI) and reshapes an immune-active TME by the inhibition of MET and TAM receptor kinases (TYRO3, AXL, MER) (151, 152). Sitravatinib is another multi-targeted TKI that inhibits VEGFR, TAM receptors (TYRO3, AXL, MERTK), and Split family receptors, which reduce the proliferation of immunosuppressive cells, initiate T cell infiltration into TME, decrease T cell exhaustion, promote M1 macrophage polarization (153, 154). The anti-VEGFR drug Lenvatinib was found to perform immunoregulation by reducing TAMs infiltration and increasing the activity of CD8+ T cells (155, 156). Another anti-angiogenic agent Nintedanib also plays an antitumor immunity by remodeling TME through increased tumoral infiltration of CD8+ T cells and granzyme B production (157). Anti-PD-1/PD-L1 and other non-PD-1/PD-L1 blockers are alternative therapeutic strategies for NSCLC patients with immunotherapy resistance. However, Durvalumab plus tremelimumab(anti-CTLA-4) had minimal benefits in NSCLC patients with progression after anti-PD-1 therapy (158). Other trials about combining with Vibostolimab(anti-TIGIT) (NCT02964013, NCT04725188) have not been completed. Moreover, TME modulators, such as MDM2, SEMA4D, and HDAC inhibitors, combined with ICIs were explored to apply in NSCLC patients with refractory and failure after immunotherapy, resulting in safety but limited benefits (159–162). These preliminary results need to be validated in further studies, including a larger sample, adjusted scheme, or changed time point of combined treatment. There are other ongoing clinical trials to investigate the practicability of new drugs such as AK112(dual-targeting PD-1/VEGF) or KN046(dual-targeting PD-1/CTLA-4), or SAR408701(anti-CEACAM5) in antagonizing immunotherapy resistance (163–165). Increasing clinical trials are ongoing to investigate and explore the safety and efficacy of a new combination treatment or drug in NSCLC patients with ICIs resistance (Table 1). We expect these trials’ results will provide additional therapeutic options for ICI-resistant NSCLC in the short term.
4.2.2 Immunotherapy rechallenge
Should NSCLC patients with prior anti-PD-1/PD-L1 therapy with a durable response be given a second course of ICI if their disease progresses? For PD-L+ NSCLC patients who had progression after 35 cycles/2 years of pembrolizumab, pembrolizumab retreatment showed that the disease control rate was over 77% (166). Another real-world setting also found evidence supporting that nivolumab retreatment could bring better responses in NSCLC patients who had a long-term response to first-course treatment (167). In addition, other reports also found that only limited patients could benefit from immunotherapy retreatment (168, 169), so it is necessary to explore effective predictive biomarkers to screen the optimum population.
5 Conclusion and future perspectives
Although immunotherapy improves survival prognosis in NSCLC patients, primary and acquired resistance impair long-term clinical benefits in the partial population. There is a negative relationship between the ORR of immunotherapy and the occurrence of acquired resistance. 74% of NSCLC patients with an effective initial response to immunotherapy will experience disease progression within 5 years. The mechanism of immunotherapy resistance is complex, dynamic, and interdependent, mainly involving intrinsic factors(tumor cells) and extrinsic factors (TME infiltrating immune cells). In the clinical appliance, therapeutic strategies for NSCLC progression after immunotherapy resistance is still in the exploratory stage. The progressive mode after immunotherapy resistance should be taken into consideration for further treatment. Radiotherapy, chemotherapy, anti-angiogenesis drugs, and TME modulators can synergistically enhance immunotherapy by regulating the process of the cancer-immunity cycle, including tumor antigen release, presentation, and TME infiltrating immune cells. However, these synergistic effects are uncontrollable and unpredictable. In further studies, we still face many challenges, such as how to find the optimum time point, mode, sequence of combination therapy, and the population who can obtain more benefits from the combination therapy, how to find the corresponding predictive biomarkers, how to confirm the optimum treatment for NSCLC patients characterized by distinct or multiple genetic backgrounds. A prerequisite for a clinical response to immunotherapy is a normal cancer-immunity cycle, of which effective T cells are vital policymakers and executants for antitumor immunity. Therefore, technically modified-T cell therapy attains more and more attention. In addition, also worth considering are the effect of the genetic background of tumor cells on native or artificially reprogrammed immune cells and how to monitor and precisely remodel the dynamic TME during treatment (Figure 5).
Author contributions
SZ: contribution to data collection, writing, and editing figures. HY: conception and design of the study, revising the manuscript critically. All authors contributed to the article and approved the submitted version.
Funding
This study was supported by National Natural Science Foundation of China (NSFC 81872458), and Xi’an Health Commission (2022ms11).
Conflict of interest
The authors declare that the research was conducted in the absence of any commercial or financial relationships that could be construed as a potential conflict of interest.
Publisher’s note
All claims expressed in this article are solely those of the authors and do not necessarily represent those of their affiliated organizations, or those of the publisher, the editors and the reviewers. Any product that may be evaluated in this article, or claim that may be made by its manufacturer, is not guaranteed or endorsed by the publisher.
Abbreviations
NSCLC, non-small-cell lung cancer; TME, tumor microenvironment; LUSC, lung squamous cell carcinoma; LUAD, lung adenocarcinoma; PD-1, programmed death protein 1; PD-L1, programmed death protein ligand 1; CTLA-4, cytotoxic T lymphocyte antigen 4; ICIs, immune checkpoint inhibitors; PD, progress disease; SD, stable disease; CR, complete response; PR, partial response; CIC, cancer-immunity cycle; ICD, immunogenic cell death; MSKCC, Memorial Sloan Kettering Cancer Center; PFS, progression-free survival; ORR, objective response rate; TILs, tumor-infiltrating lymphocytes; HLA-I/MHC-I, Class I human leukocyte antigen; FN-γ, Interferon-gamma; NK, natural killer; DCs, dendritic cells; TAMs, tumor-associated macrophages.
References
1. Siegel RL, Miller KD, Fuchs HE, Jemal A. Cancer statistics, 2022. CA Cancer J Clin (2022) 72(1):7–33. doi: 10.3322/caac.21708
2. Goldstraw P, Ball D, Jett JR, Le Chevalier T, Lim E, Nicholson AG, et al. Non-small-cell lung cancer. Lancet (2011) 378(9804):1727–40. doi: 10.1016/S0140-6736(10)62101-0
3. Felip E, Altorki N, Zhou C, Csoszi T, Vynnychenko I, Goloborodko O, et al. Adjuvant atezolizumab after adjuvant chemotherapy in resected stage IB-IIIA non-small-cell lung cancer (IMpower010): a randomised, multicentre, open-label, phase 3 trial. Lancet (2021) 398(10308):1344–57. doi: 10.1016/S0140-6736(21)02098-5
4. Forde PM, Spicer J, Lu S, Provencio M, Mitsudomi T, Awad MM, et al. Neoadjuvant nivolumab plus chemotherapy in resectable lung cancer. N Engl J Med (2022) 386(21):1973–85. doi: 10.1056/NEJMoa2202170
5. Antonia SJ, Villegas A, Daniel D, Vicente D, Murakami S, Hui R, et al. Overall Survival with Durvalumab after Chemoradiotherapy in Stage III NSCLC. N Engl J Med. (2018) 379(24):2342–50. doi: 10.1056/NEJMoa1809697
6. Herbst RS, Baas P, Kim DW, Felip E, Perez-Gracia JL, Han JY, et al. Pembrolizumab versus docetaxel for previously treated, PD-L1-positive, advanced non-small-cell lung cancer (KEYNOTE-010): A randomised controlled trial. Lancet (2016) 387(10027):1540–50. doi: 10.1016/S0140-6736(15)01281-7
7. Socinski MA, Jotte RM, Cappuzzo F, Orlandi F, Stroyakovskiy D, Nogami N, et al. Atezolizumab for first-line treatment of metastatic nonsquamous NSCLC. N Engl J Med (2018) 378(24):2288–301. doi: 10.1056/NEJMoa1716948
8. Kluger HM, Tawbi HA, Ascierto ML, Bowden M, Callahan MK, Cha E, et al. Defining tumor resistance to PD-1 pathway blockade: recommendations from the first meeting of the SITC immunotherapy resistance taskforce. J Immunother Cancer (2020) 8(1):e000398. doi: 10.1136/jitc-2019-000398
9. Chen DS, Mellman I. Oncology meets immunology: The cancer-immunity cycle. Immunity (2013) 39(1):1–10. doi: 10.1016/j.immuni.2013.07.012
10. Hanahan D, Weinberg RA. Hallmarks of cancer: the next generation. Cell (2011) 144(5):646–74. doi: 10.1016/j.cell.2011.02.013
11. Wu T, Dai Y. Tumor microenvironment and therapeutic response. Cancer Lett (2017) 387:61–8. doi: 10.1016/j.canlet.2016.01.043
12. Wang F, Wang S, Zhou Q. The resistance mechanisms of lung cancer immunotherapy. Front Oncol (2020) 10:568059. doi: 10.3389/fonc.2020.568059
13. Borghaei H, Gettinger S, Vokes EE, Chow LQM, Burgio MA, de Castro Carpeno J, et al. Five-year outcomes from the randomized, phase III trials CheckMate 017 and 057: Nivolumab versus docetaxel in previously treated non-Small-Cell lung cancer. J Clin Oncol (2021) 39(7):723–33. doi: 10.1200/JCO.20.01605
14. Rittmeyer A, Barlesi F, Waterkamp D, Park K, Ciardiello F, von Pawel J, et al. Atezolizumab versus docetaxel in patients with previously treated non-small-cell lung cancer (OAK): A phase 3, open-label, multicentre randomised controlled trial. Lancet (2017) 389(10066):255–65. doi: 10.1016/S0140-6736(16)32517-X
15. Carbone DP, Reck M, Paz-Ares L, Creelan B, Horn L, Steins M, et al. First-line nivolumab in stage IV or recurrent non-Small-Cell lung cancer. N Engl J Med (2017) 376(25):2415–26. doi: 10.1056/NEJMoa1613493
16. Hellmann MD, Paz-Ares L, Bernabe Caro R, Zurawski B, Kim SW, Carcereny Costa E, et al. Nivolumab plus ipilimumab in advanced non-small-cell lung cancer. N Engl J Med (2019) 381(21):2020–31. doi: 10.1056/NEJMoa1910231
17. Mok TSK, Wu YL, Kudaba I, Kowalski DM, Cho BC, Turna HZ, et al. Pembrolizumab versus chemotherapy for previously untreated, PD-L1-expressing, locally advanced or metastatic non-small-cell lung cancer (KEYNOTE-042): a randomised, open-label, controlled, phase 3 trial. Lancet (2019) 393(10183):1819–30. doi: 10.1016/S0140-6736(18)32409-7
18. West H, McCleod M, Hussein M, Morabito A, Rittmeyer A, Conter HJ, et al. Atezolizumab in combination with carboplatin plus nab-paclitaxel chemotherapy compared with chemotherapy alone as first-line treatment for metastatic non-squamous non-small-cell lung cancer (IMpower130): A multicentre, randomised, open-label, phase 3 trial. Lancet Oncol (2019) 20(7):924–37. doi: 10.1016/S1470-2045(19)30167-6
19. Gandhi L, Rodriguez-Abreu D, Gadgeel S, Esteban E, Felip E, De Angelis F, et al. Pembrolizumab plus chemotherapy in metastatic non-Small-Cell lung cancer. N Engl J Med (2018) 378(22):2078–92. doi: 10.1056/NEJMoa1801005
20. Paz-Ares L, Luft A, Vicente D, Tafreshi A, Gumus M, Mazieres J, et al. Pembrolizumab plus chemotherapy for squamous non-Small-Cell lung cancer. N Engl J Med (2018) 379(21):2040–51. doi: 10.1056/NEJMoa1810865
21. Wang YJ, Fletcher R, Yu J, Zhang L. Immunogenic effects of chemotherapy-induced tumor cell death. Genes Dis (2018) 5(3):194–203. doi: 10.1016/j.gendis.2018.05.003
22. Vanmeerbeek I, Sprooten J, De Ruysscher D, Tejpar S, Vandenberghe P, Fucikova J, et al. Trial watch: chemotherapy-induced immunogenic cell death in immuno-oncology. Oncoimmunology (2020) 9(1):1703449. doi: 10.1080/2162402X.2019.1703449
23. Schoenfeld AJ, Antonia SJ, Awad MM, Felip E, Gainor J, Gettinger SN, et al. Clinical definition of acquired resistance to immunotherapy in patients with metastatic non-small-cell lung cancer. Ann Oncol (2021) 32(12):1597–607. doi: 10.1016/j.annonc.2021.08.2151
24. Antonia SJ, Borghaei H, Ramalingam SS, Horn L, De Castro Carpeno J, Pluzanski A, et al. Four-year survival with nivolumab in patients with previously treated advanced non-small-cell lung cancer: a pooled analysis. Lancet Oncol (2019) 20(10):1395–408. doi: 10.1016/S1470-2045(19)30407-3
25. Schoenfeld AJ, Hellmann MD. Acquired resistance to immune checkpoint inhibitors. Cancer Cell (2020) 37(4):443–55. doi: 10.1016/j.ccell.2020.03.017
26. Schoenfeld A, Rizvi H, Memon D, Luo J, Preeshagul I, Sauter J, et al. Acquired resistance to PD-1 blockade in NSCLC. J OF Clin Oncol (2020) 38(Supplement 15):9621. doi: 10.1200/JCO.2020.38.15_suppl.9621
27. Heo JY, Yoo SH, Suh KJ, Kim SH, Kim YJ, Ock CY, et al. Clinical pattern of failure after a durable response to immune check inhibitors in non-small cell lung cancer patients. Sci Rep (2021) 11(1):2514. doi: 10.1038/s41598-021-81666-x
28. Hosoya K, Fujimoto D, Morimoto T, Kumagai T, Tamiya A, Taniguchi Y, et al. Clinical factors associated with shorter durable response, and patterns of acquired resistance to first-line pembrolizumab monotherapy in PD-L1-positive non-small-cell lung cancer patients: a retrospective multicenter study. BMC Cancer (2021) 21(1):346. doi: 10.1186/s12885-021-08048-4
29. Garon EB, Hellmann MD, Rizvi NA, Carcereny E, Leighl NB, Ahn MJ, et al. Five-year overall survival for patients with advanced non Small-cell lung cancer treated with pembrolizumab: Results from the phase I KEYNOTE-001 study. J Clin Oncol (2019) 37(28):2518–27. doi: 10.1200/JCO.19.00934
30. Middleton G, Brock K, Savage J, Mant R, Summers Y, Connibear J, et al. Pembrolizumab in patients with non-small-cell lung cancer of performance status 2 (PePS2): A single arm, phase 2 trial. Lancet Respir Med (2020) 8(9):895–904. doi: 10.1016/S2213-2600(20)30033-3
31. Turajlic S, Litchfield K, Xu H, Rosenthal R, McGranahan N, Reading JL, et al. Insertion-and-deletion-derived tumour-specific neoantigens and the immunogenic phenotype: a pan-cancer analysis. Lancet Oncol (2017) 18(8):1009–21. doi: 10.1016/S1470-2045(17)30516-8
32. Hellmann MD, Ciuleanu TE, Pluzanski A, Lee JS, Otterson GA, Audigier-Valette C, et al. Nivolumab plus ipilimumab in lung cancer with a high tumor mutational burden. N Engl J Med (2018) 378(22):2093–104. doi: 10.1056/NEJMoa1801946
33. Alborelli I, Leonards K, Rothschild SI, Leuenberger LP, Savic Prince S, Mertz KD, et al. Tumor mutational burden assessed by targeted NGS predicts clinical benefit from immune checkpoint inhibitors in non-small cell lung cancer. J Pathol (2020) 250(1):19–29. doi: 10.1002/path.5344
34. Chen H, Chong W, Teng C, Yao Y, Wang X, Li X. The immune response-related mutational signatures and driver genes in non-small-cell lung cancer. Cancer Sci (2019) 110(8):2348–56. doi: 10.1111/cas.14113
35. Chen N, Fang W, Zhan J, Hong S, Tang Y, Kang S, et al. Upregulation of PD-L1 by EGFR activation mediates the immune escape in EGFR-driven NSCLC: Implication for optional immune targeted therapy for NSCLC patients with EGFR mutation. J Thorac Oncol (2015) 10(6):910–23. doi: 10.1097/JTO.0000000000000500
36. Zhang N, Zeng Y, Du W, Zhu J, Shen D, Liu Z, et al. The EGFR pathway is involved in the regulation of PD-L1 expression via the IL-6/JAK/STAT3 signaling pathway in EGFR-mutated non-small cell lung cancer. Int J Oncol (2016) 49(4):1360–8. doi: 10.3892/ijo.2016.3632
37. Chen K, Cheng G, Zhang F, Zhu G, Xu Y, Yu X, et al. PD-L1 expression and T cells infiltration in patients with uncommon EGFR-mutant non-small cell lung cancer and the response to immunotherapy. Lung Cancer (2020) 142:98–105. doi: 10.1016/j.lungcan.2020.02.010
38. Guisier F, Dubos-Arvis C, Vinas F, Doubre H, Ricordel C, Ropert S, et al. Efficacy and safety of anti-PD-1 immunotherapy in patients with advanced NSCLC with BRAF, HER2, or MET mutations or RET translocation: GFPC 01-2018. J Thorac Oncol (2020) 15(4):628–36. doi: 10.1016/j.jtho.2019.12.129
39. Offin M, Guo R, Wu SL, Sabari J, Land JD, Ni A, et al. Immunophenotype and response to immunotherapy of RET-rearranged lung cancers. JCO Precis Oncol (2019) 3:PO.18.00386. doi: 10.1200/PO.18.00386
40. Sabari JK, Leonardi GC, Shu CA, Umeton R, Montecalvo J, Ni A, et al. PD-L1 expression, tumor mutational burden, and response to immunotherapy in patients with MET exon 14 altered lung cancers. Ann Oncol (2018) 29(10):2085–91. doi: 10.1093/annonc/mdy334
41. Mazieres J, Drilon A, Lusque A, Mhanna L, Cortot AB, Mezquita L, et al. Immune checkpoint inhibitors for patients with advanced lung cancer and oncogenic driver alterations: results from the IMMUNOTARGET registry. Ann Oncol (2019) 30(8):1321–8. doi: 10.1093/annonc/mdz167
42. Biton J, Mansuet-Lupo A, Pecuchet N, Alifano M, Ouakrim H, Arrondeau J, et al. TP53, STK11, and EGFR mutations predict tumor immune profile and the response to anti-PD-1 in lung adenocarcinoma. Clin Cancer Res (2018) 24(22):5710–23. doi: 10.1158/1078-0432.CCR-18-0163
43. Xu X, Yang Y, Liu X, Cao N, Zhang P, Zhao S, et al. NFE2L2/KEAP1 mutations correlate with higher tumor mutational burden Value/PD-L1 expression and potentiate improved clinical outcome with immunotherapy. Oncologist (2020) 25(6):e955–63. doi: 10.1634/theoncologist.2019-0885
44. Negrao MV, Skoulidis F, Montesion M, Schulze K, Bara I, Shen V, et al. Oncogene-specific differences in tumor mutational burden, PD-L1 expression, and outcomes from immunotherapy in non-small cell lung cancer. J Immunother Cancer (2021) 9(8):e002891. doi: 10.1136/jitc-2021-002891
45. Judd J, Abdel Karim N, Khan H, Naqash AR, Baca Y, Xiu J, et al. Characterization of KRAS mutation subtypes in non-small cell lung cancer. Mol Cancer Ther (2021) 20(12):2577–84. doi: 10.1158/1535-7163.MCT-21-0201
46. Skoulidis F, Goldberg ME, Greenawalt DM, Hellmann MD, Awad MM, Gainor JF, et al. STK11/LKB1 mutations and PD-1 inhibitor resistance in KRAS-mutant lung adenocarcinoma. Cancer Discovery (2018) 8(7):822–35. doi: 10.1158/2159-8290.CD-18-0099
47. Anagnostou V, Smith KN, Forde PM, Niknafs N, Bhattacharya R, White J, et al. Evolution of neoantigen landscape during immune checkpoint blockade in non-small cell lung cancer. Cancer Discovery (2017) 7(3):264–76. doi: 10.1158/2159-8290.CD-16-0828
48. Gettinger S, Choi J, Hastings K, Truini A, Datar I, Sowell R, et al. Impaired HLA class I antigen processing and presentation as a mechanism of acquired resistance to immune checkpoint inhibitors in lung cancer. Cancer Discovery (2017) 7(12):1420–35. doi: 10.1158/2159-8290.CD-17-0593
49. Thompson JC, Davis C, Deshpande C, Hwang WT, Jeffries S, Huang A, et al. Gene signature of antigen processing and presentation machinery predicts response to checkpoint blockade in non-small cell lung cancer (NSCLC) and melanoma. J Immunother Cancer (2020) 8(2):e000974. doi: 10.1136/jitc-2020-000974
50. Campoli M, Ferrone S. HLA antigen changes in malignant cells: Epigenetic mechanisms and biologic significance. Oncogene (2008) 27(45):5869–85. doi: 10.1038/onc.2008.273
51. Chowell D, Krishna C, Pierini F, Makarov V, Rizvi NA, Kuo F, et al. Evolutionary divergence of HLA class I genotype impacts efficacy of cancer immunotherapy. Nat Med (2019) 25(11):1715–20. doi: 10.1038/s41591-019-0639-4
52. McGranahan N, Rosenthal R, Hiley CT, Rowan AJ, Watkins TBK, Wilson GA, et al. Allele-specific HLA loss and immune escape in lung cancer evolution. Cell (2017) 171(6):1259–71. doi: 10.1016/j.cell.2017.10.001
53. Montesion M, Murugesan K, Jin DX, Sharaf R, Sanchez N, Guria A, et al. Somatic HLA class I loss is a widespread mechanism of immune evasion which refines the use of tumor mutational burden as a biomarker of checkpoint inhibitor response. Cancer Discovery (2021) 11(2):282–92. doi: 10.1158/2159-8290.CD-20-0672
54. Springer S. Transport and quality control of MHC class I molecules in the early secretory pathway. Curr Opin Immunol (2015) 34:83–90. doi: 10.1016/j.coi.2015.02.009
55. Zhao Y, Cao Y, Chen Y, Wu L, Hang H, Jiang C, et al. B2M gene expression shapes the immune landscape of lung adenocarcinoma and determines the response to immunotherapy. Immunology (2021) 164(3):507–23. doi: 10.1111/imm.13384
56. Yano T, Sugio K, Yamazaki K, Kase S, Yamaguchi M, Ondo K, et al. Direct IFNluence of interferon-gamma on proliferation and cell-surface antigen expression of non-small cell lung cancer cells. Lung Cancer (2000) 30(3):169–74. doi: 10.1016/s0169-5002(00)00136-7
57. Wroblewski JM, Bixby DL, Borowski C, Yannelli JR. Characterization of human non-small cell lung cancer (NSCLC) cell lines for expression of MHC, co-stimulatory molecules and tumor-associated antigens. Lung Cancer (2001) 33(2-3):181–94. doi: 10.1016/s0169-5002(01)00210-0
58. Bellucci R, Martin A, Bommarito D, Wang K, Hansen SH, Freeman GJ, et al. Interferon-gamma-induced activation of JAK1 and JAK2 suppresses tumor cell susceptibility to NK cells through upregulation of PD-L1 expression. Oncoimmunology (2015) 4(6):e1008824. doi: 10.1080/2162402X.2015.1008824
59. Zhou S, Xie J, Huang Z, Deng L, Wu L, Yu J, et al. Anti-PD-(L)1 immunotherapy for brain metastases in non-small cell lung cancer: Mechanisms, advances, and challenges. Cancer Lett (2021) 502:166–79. doi: 10.1016/j.canlet.2020.12.043
60. Ayers M, Lunceford J, Nebozhyn M, Murphy E, Loboda A, Kaufman DR, et al. IFN-gamma-related mRNA profile predicts clinical response to PD-1 blockade. J Clin Invest (2017) 127(8):2930–40. doi: 10.1172/JCI91190
61. Kauffmann-Guerrero D, Kahnert K, Kiefl R, Sellmer L, Walter J, Behr J, et al. Systemic inflammation and pro-inflammatory cytokine profile predict response to checkpoint inhibitor treatment in NSCLC: a prospective study. Sci Rep (2021) 11(1):10919. doi: 10.1038/s41598-021-90397-y
62. Gao Y, Paivinen P, Tripathi S, Domenech-Moreno E, Wong IPL, Vaahtomeri K, et al. Inactivation of AMPK leads to attenuation of antigen presentation and immune evasion in lung adenocarcinoma. Clin Cancer Res (2022) 28(1):227–37. doi: 10.1158/1078-0432.CCR-21-2049
63. Zhou X, Wang N, Zhang Y, Yu H, Wu Q. KAT2B is an immune infiltration-associated biomarker predicting prognosis and response to immunotherapy in non-small cell lung cancer. Invest New Drugs (2022) 40(1):43–57. doi: 10.1007/s10637-021-01159-6
64. Peng S, Wang R, Zhang X, Ma Y, Zhong L, Li K, et al. EGFR-TKI resistance promotes immune escape in lung cancer via increased PD-L1 expression. Mol Cancer (2019) 18(1):165. doi: 10.1186/s12943-019-1073-4
65. Sumimoto H, Takano A, Teramoto K, Daigo Y. RAS-Mitogen-Activated protein kinase signal is required for enhanced PD-L1 expression in human lung cancers. PloS One (2016) 11(11):e0166626. doi: 10.1371/journal.pone.0166626
66. Ferrer I, Zugazagoitia J, Herbertz S, John W, Paz-Ares L, Schmid-Bindert G. KRAS-mutant non-small cell lung cancer: From biology to therapy. Lung Cancer (2018) 124:53–64. doi: 10.1016/j.lungcan.2018.07.013
67. Dudnik E, Peled N, Nechushtan H, Wollner M, Onn A, Agbarya A, et al. BRAF mutant lung cancer: Programmed death ligand 1 expression, tumor mutational burden, microsatellite instability status, and response to immune check-point inhibitors. J Thorac Oncol (2018) 13(8):1128–37. doi: 10.1016/j.jtho.2018.04.024
68. Stutvoet TS, Kol A, de Vries EG, de Bruyn M, Fehrmann RS. Terwisscha van scheltinga AG et al. MAPK pathway activity plays a key role in PD-L1 expression of lung adenocarcinoma cells. J Pathol (2019) 249(1):52–64. doi: 10.1002/path.5280
69. Mossmann D, Park S, Hall MN. mTOR signalling and cellular metabolism are mutual determinants in cancer. Nat Rev Cancer (2018) 18(12):744–57. doi: 10.1038/s41568-018-0074-8
70. Hollander MC, Maier CR, Hobbs EA, Ashmore AR, Linnoila RI, Dennis PA. Akt1 deletion prevents lung tumorigenesis by mutant K-ras. Oncogene (2011) 30(15):1812–21. doi: 10.1038/onc.2010.556
71. Lastwika KJ, Wilson W 3rd, Li QK, Norris J, Xu H, Ghazarian SR, et al. Control of PD-L1 expression by oncogenic activation of the AKT-mTOR pathway in non-small cell lung cancer. Cancer Res (2016) 76(2):227–38. doi: 10.1158/0008-5472.CAN-14-3362
72. Giannone G, Ghisoni E, Genta S, Scotto G, Tuninetti V, Turinetto M, et al. Immuno-metabolism and microenvironment in cancer: Key players for immunotherapy. Int J Mol Sci (2020) 21(12):4414. doi: 10.3390/ijms21124414
73. Schoenfeld AJ, Rizvi H, Bandlamudi C, Sauter JL, Travis WD, Rekhtman N, et al. Clinical and molecular correlates of PD-L1 expression in patients with lung adenocarcinomas. Ann Oncol (2020) 31(5):599–608. doi: 10.1016/j.annonc.2020.01.065
74. Katoh Y, Yaguchi T, Kubo A, Iwata T, Morii K, Kato D, et al. Inhibition of stearoyl-CoA desaturase 1 (SCD1) enhances the antitumor T cell response through regulating beta-catenin signaling in cancer cells and ER stress in T cells and synergizes with anti-PD-1 antibody. J Immunother Cancer (2022) 10(7):e004616. doi: 10.1136/jitc-2022-004616
75. Gao J, Shi LZ, Zhao H, Chen J, Xiong L, He Q, et al. Loss of IFN-gamma pathway genes in tumor cells as a mechanism of resistance to anti-CTLA-4 therapy. Cell (2016) 167(2):397–404. doi: 10.1016/j.cell.2016.08.069
76. Benci JL, Xu B, Qiu Y, Wu TJ, Dada H, Twyman-Saint Victor C, et al. Tumor interferon signaling regulates a multigenic resistance program to immune checkpoint blockade. Cell (2016) 167(6):1540–54. doi: 10.1016/j.cell.2016.11.022
77. Zaretsky JM, Garcia-Diaz A, Shin DS, Escuin-Ordinas H, Hugo W, Hu-Lieskovan S, et al. Mutations associated with acquired resistance to PD-1 blockade in melanoma. N Engl J Med (2016) 375(9):819–29. doi: 10.1056/NEJMoa1604958
78. Morimoto Y, Kishida T, Kotani SI, Takayama K, Mazda O. Interferon-beta signal may up-regulate PD-L1 expression through IRF9-dependent and independent pathways in lung cancer cells. Biochem Biophys Res Commun (2018) 507(1-4):330–6. doi: 10.1016/j.bbrc.2018.11.035
79. Chen S, Crabill GA, Pritchard TS, McMiller TL, Wei P, Pardoll DM, et al. Mechanisms regulating PD-L1 expression on tumor and immune cells. J Immunother Cancer (2019) 7(1):305. doi: 10.1186/s40425-019-0770-2
80. Dong S, Guo X, Han F, He Z, Wang Y. Emerging role of natural products in cancer immunotherapy. Acta Pharm Sin B (2022) 12(3):1163–85. doi: 10.1016/j.apsb.2021.08.020
81. Stankovic B, Bjorhovde HAK, Skarshaug R, Aamodt H, Frafjord A, Muller E, et al. Immune cell composition in human non-small cell lung cancer. Front Immunol (2018) 9:3101. doi: 10.3389/fimmu.2018.03101
82. Najafi M, Hashemi Goradel N, Farhood B, Salehi E, Nashtaei MS, Khanlarkhani N, et al. Macrophage polarity in cancer: A review. J Cell Biochem (2019) 120(3):2756–65. doi: 10.1002/jcb.27646
83. Mantovani A, Marchesi F, Malesci A, Laghi L, Allavena P. Tumour-associated macrophages as treatment targets in oncology. Nat Rev Clin Oncol (2017) 14(7):399–416. doi: 10.1038/nrclinonc.2016.217
84. Casanova-Acebes M, Dalla E, Leader AM, LeBerichel J, Nikolic J, Morales BM, et al. Tissue-resident macrophages provide a pro-tumorigenic niche to early NSCLC cells. Nature (2021) 595(7868):578–84. doi: 10.1038/s41586-021-03651-8
85. Kim SR, Chun SH, Kim JR, Kim SY, Seo JY, Jung CK, et al. The implications of clinical risk factors, CAR index, and compositional changes of immune cells on hyperprogressive disease in non-small cell lung cancer patients receiving immunotherapy. BMC Cancer (2021) 21(1):19. doi: 10.1186/s12885-020-07727-y
86. Xia Y, Rao L, Yao H, Wang Z, Ning P, Chen X. Engineering macrophages for cancer immunotherapy and drug delivery. Adv Mater (2020) 32(40):e2002054. doi: 10.1002/adma.202002054
87. Jeong H, Kim S, Hong BJ, Lee CJ, Kim YE, Bok S, et al. Tumor-associated macrophages enhance tumor hypoxia and aerobic glycolysis. Cancer Res (2019) 79(4):795–806. doi: 10.1158/0008-5472.CAN-18-2545
88. Liu Y, Zugazagoitia J, Ahmed FS, Henick BS, Gettinger SN, Herbst RS, et al. Immune cell PD-L1 colocalizes with macrophages and is associated with outcome in PD-1 pathway blockade therapy. Clin Cancer Res (2020) 26(4):970–7. doi: 10.1158/1078-0432.CCR-19-1040
89. Hartley GP, Chow L, Ammons DT, Wheat WH, Dow SW. Programmed cell death ligand 1 (PD-L1) signaling regulates macrophage proliferation and activation. Cancer Immunol Res (2018) 6(10):1260–73. doi: 10.1158/2326-6066.CIR-17-0537
90. Sedighzadeh SS, Khoshbin AP, Razi S, Keshavarz-Fathi M, Rezaei N. A narrative review of tumor-associated macrophages in lung cancer: regulation of macrophage polarization and therapeutic implications. Transl Lung Cancer Res (2021) 10(4):1889–916. doi: 10.21037/tlcr-20-1241
91. Chen X, Gao A, Zhang F, Yang Z, Wang S, Fang Y, et al. ILT4 inhibition prevents TAM- and dysfunctional T cell-mediated immunosuppression and enhances the efficacy of anti-PD-L1 therapy in NSCLC with EGFR activation. Theranostics (2021) 11(7):3392–416. doi: 10.7150/thno.52435
92. Vivier E, Raulet DH, Moretta A, Caligiuri MA, Zitvogel L, Lanier LL, et al. Innate or adaptive immunity? the example of natural killer cells. Science (2011) 331(6013):44–9. doi: 10.1126/science.1198687
93. Bottcher JP, Bonavita E, Chakravarty P, Blees H, Cabeza-Cabrerizo M, Sammicheli S, et al. NK cells stimulate recruitment of cDC1 into the tumor microenvironment promoting cancer immune control. Cell (2018) 172(5):1022–37. doi: 10.1016/j.cell.2018.01.004
94. Backman M, La Fleur L, Kurppa P, Djureinovic D, Elfving H, Brunnstrom H, et al. Infiltration of NK and plasma cells is associated with a distinct immune subset in non-small cell lung cancer. J Pathol (2021) 255(3):243–56. doi: 10.1002/path.5772
95. Frigola J, Navarro A, Carbonell C, Callejo A, Iranzo P, Cedres S, et al. Molecular profiling of long-term responders to immune checkpoint inhibitors in advanced non-small cell lung cancer. Mol Oncol (2021) 15(4):887–900. doi: 10.1002/1878-0261.12891
96. Poma AM, Bruno R, Pietrini I, Ali G, Pasquini G, Proietti A, et al. Biomarkers and gene signatures to predict durable response to pembrolizumab in non-small cell lung cancer. Cancers (Basel) (2021) 13(15):3828. doi: 10.3390/cancers13153828
97. La Fleur L, Botling J, He F, Pelicano C, Zhou C, He C, et al. Targeting MARCO and IL37R on immunosuppressive macrophages in lung cancer blocks regulatory T cells and supports cytotoxic lymphocyte function. Cancer Res (2021) 81(4):956–67. doi: 10.1158/0008-5472.CAN-20-1885
98. Hu Z, Xu X, Wei H. The adverse impact of tumor microenvironment on NK-cell. Front Immunol (2021) 12:633361. doi: 10.3389/fimmu.2021.633361
99. Helmink BA, Reddy SM, Gao J, Zhang S, Basar R, Thakur R, et al. B cells and tertiary lymphoid structures promote immunotherapy response. Nature (2020) 577(7791):549–55. doi: 10.1038/s41586-019-1922-8
100. Dieu-Nosjean MC, Giraldo NA, Kaplon H, Germain C, Fridman WH, Sautes-Fridman C. Tertiary lymphoid structures, drivers of the antitumor responses in human cancers. Immunol Rev (2016) 271(1):260–75. doi: 10.1111/imr.12405
101. Patil NS, Nabet BY, Muller S, Koeppen H, Zou W, Giltnane J, et al. Intratumoral plasma cells predict outcomes to PD-L1 blockade in non-small cell lung cancer. Cancer Cell (2022) 40(3):289–300. doi: 10.1016/j.ccell.2022.02.002
102. Bruno TC, Ebner PJ, Moore BL, Squalls OG, Waugh KA, Eruslanov EB, et al. Antigen-presenting intratumoral b cells affect CD4(+) TIL phenotypes in non-small cell lung cancer patients. Cancer Immunol Res (2017) 5(10):898–907. doi: 10.1158/2326-6066.CIR-17-0075
103. Thommen DS, Koelzer VH, Herzig P, Roller A, Trefny M, Dimeloe S, et al. A transcriptionally and functionally distinct PD-1(+) CD8(+) T cell pool with predictive potential in non-small-cell lung cancer treated with PD-1 blockade. Nat Med (2018) 24(7):994–1004. doi: 10.1038/s41591-018-0057-z
104. Zappasodi R, Budhu S, Hellmann MD, Postow MA, Senbabaoglu Y, Manne S, et al. Non-conventional inhibitory CD4(+)Foxp3(-)PD-1(hi) T cells as a biomarker of immune checkpoint blockade activity. Cancer Cell (2018) 34(4):691. doi: 10.1016/j.ccell.2018.09.007
105. Kamphorst AO, Pillai RN, Yang S, Nasti TH, Akondy RS, Wieland A, et al. Proliferation of PD-1+ CD8 T cells in peripheral blood after PD-1-targeted therapy in lung cancer patients. Proc Natl Acad Sci U S A (2017) 114(19):4993–8. doi: 10.1073/pnas.1705327114
106. Li F, Li C, Cai X, Xie Z, Zhou L, Cheng B, et al. The association between CD8+ tumor-infiltrating lymphocytes and the clinical outcome of cancer immunotherapy: A systematic review and meta-analysis. EClinicalMedicine (2021) 41:101134. doi: 10.1016/j.eclinm.2021.101134
107. Hashemi S, Fransen MF, Niemeijer A, Ben Taleb N, Houda I, Veltman J, et al. Surprising impact of stromal TIL’s on immunotherapy efficacy in a real-world lung cancer study. Lung Cancer (2021) 153:81–9. doi: 10.1016/j.lungcan.2021.01.013
108. Mildner F, Sopper S, Amann A, Pircher A, Pall G, Kock S, et al. Systematic review: Soluble immunological biomarkers in advanced non-small-cell lung cancer (NSCLC). Crit Rev Oncol Hematol (2020) 153:102948. doi: 10.1016/j.critrevonc.2020.102948
109. Guo X, Zhang Y, Zheng L, Zheng C, Song J, Zhang Q, et al. Global characterization of T cells in non-small-cell lung cancer by single-cell sequencing. Nat Med (2018) 24(7):978–85. doi: 10.1038/s41591-018-0045-3
110. Pauken KE, Sammons MA, Odorizzi PM, Manne S, Godec J, Khan O, et al. Epigenetic stability of exhausted T cells limits durability of reinvigoration by PD-1 blockade. Science (2016) 354(6316):1160–5. doi: 10.1126/science.aaf2807
111. Sanmamed MF, Nie X, Desai SS, Villaroel-Espindola F, Badri T, Zhao D, et al. A burned-out CD8(+) T-cell subset expands in the tumor microenvironment and curbs cancer immunotherapy. Cancer Discovery (2021) 11(7):1700–15. doi: 10.1158/2159-8290.CD-20-0962
112. Chen SW, Zhu SQ, Pei X, Qiu BQ, Xiong D, Long X, et al. Cancer cell-derived exosomal circUSP7 induces CD8(+) T cell dysfunction and anti-PD1 resistance by regulating the miR-934/SHP2 axis in NSCLC. Mol Cancer (2021) 20(1):144. doi: 10.1186/s12943-021-01448-x
113. Lu Y, Xue J, Deng T, Zhou X, Yu K, Deng L, et al. Safety and feasibility of CRISPR-edited T cells in patients with refractory non-small-cell lung cancer. Nat Med (2020) 26(5):732–40. doi: 10.1038/s41591-020-0840-5
114. Doroshow DB, Sanmamed MF, Hastings K, Politi K, Rimm DL, Chen L, et al. Immunotherapy in non-small cell lung cancer: Facts and hopes. Clin Cancer Res (2019) 25(15):4592–602. doi: 10.1158/1078-0432.CCR-18-1538
115. Hu Z, Zheng X, Jiao D, Zhou Y, Sun R, Wang B, et al. LunX-CAR T cells as a targeted therapy for non-small cell lung cancer. Mol Ther Oncolytics (2020) 17:361–70. doi: 10.1016/j.omto.2020.04.008
116. Gao Q, Wang S, Chen X, Cheng S, Zhang Z, Li F, et al. Cancer-cell-secreted CXCL11 promoted CD8(+) T cells infiltration through docetaxel-induced-release of HMGB1 in NSCLC. J Immunother Cancer (2019) 7(1):42. doi: 10.1186/s40425-019-0511-6
117. Rizvi NA, Hellmann MD, Snyder A, Kvistborg P, Makarov V, Havel JJ, et al. Cancer immunology. mutational landscape determines sensitivity to PD-1 blockade in non-small cell lung cancer. Science (2015) 348(6230):124–8. doi: 10.1126/science.aaa1348
118. Liu Z, Wang T, She Y, Wu K, Gu S, Li L, et al. N(6)-methyladenosine-modified circIGF2BP3 inhibits CD8(+) T-cell responses to facilitate tumor immune evasion by promoting the deubiquitination of PD-L1 in non-small cell lung cancer. Mol Cancer (2021) 20(1):105. doi: 10.1186/s12943-021-01398-4
119. Rheinheimer S, Heussel CP, Mayer P, Gaissmaier L, Bozorgmehr F, Winter H, et al. Oligoprogressive non-Small-Cell lung cancer under treatment with PD-(L)1 inhibitors. Cancers (Basel) (2020) 12(4):1046. doi: 10.3390/cancers12041046
120. Guckenberger M, Lievens Y, Bouma AB, Collette L, Dekker A, deSouza NM, et al. Characterisation and classification of oligometastatic disease: a European society for radiotherapy and oncology and European organisation for research and treatment of cancer consensus recommendation. Lancet Oncol (2020) 21(1):e18–28. doi: 10.1016/S1470-2045(19)30718-1
121. Franceschini D, De Rose F, Cozzi S, Franzese C, Rossi S, Finocchiaro G, et al. The use of radiation therapy for oligoprogressive/oligopersistent oncogene-driven non small cell lung cancer: State of the art. Crit Rev Oncol Hematol (2020) 148:102894. doi: 10.1016/j.critrevonc.2020.102894
122. Griswold CR, Kerrigan K, Patel SB. Combination of local ablative therapy and continuation of immune checkpoint inhibitor (ICI) therapy provides durable treatment response past oligometastatic progression in NSCLC: A case report. Case Rep Oncol (2019) 12(3):866–71. doi: 10.1159/000504473
123. Guisier F, Gervais R, El Husseini K, Assié J-B, Geier M, Decroisette C, et al. Local ablative treatment and treatment beyond progression for oligo-progression in stage IV non-small cell lung cancer after tumour response to anti-PD1 treatment. Ann Oncol (2019) 30(Supplement 5):v620–v621. doi: 10.1093/annonc/mdz260.034
124. Metro G, Addeo A, Signorelli D, Gili A, Economopoulou P, Roila F, et al. Outcomes from salvage chemotherapy or pembrolizumab beyond progression with or without local ablative therapies for advanced non-small cell lung cancers with PD-L1 >/=50% who progress on first-line immunotherapy: real-world data from a European cohort. J Thorac Dis (2019) 11(12):4972–81. doi: 10.21037/jtd.2019.12.23
125. Sotelo MJ, Cabezas-Camarero S, Riquelme A, Bueno C. Long-term survival of a patient with programmed death ligand 1-negative lung adenocarcinoma and oligoprogressive disease treated with nivolumab and stereotactic body radiation therapy. J Cancer Res Ther (2020) 16(4):941–5. doi: 10.4103/jcrt.JCRT_81_19
126. Tobita S, Kinehara Y, Tamura Y, Kurebe H, Ninomiya R, Utsu Y, et al. Successful continuous nivolumab therapy for metastatic non-small cell lung cancer after local treatment of oligometastatic lesions. Thorac Cancer (2020) 11(8):2357–60. doi: 10.1111/1759-7714.13539
127. Peeters STH, Van Limbergen EJ, Hendriks LEL, De Ruysscher D. Radiation for oligometastatic lung cancer in the era of immunotherapy: What do we (Need to) know? Cancers (Basel) (2021) 13(9):2132. doi: 10.3390/cancers13092132
128. Dovedi SJ, Adlard AL, Lipowska-Bhalla G, McKenna C, Jones S, Cheadle EJ, et al. Acquired resistance to fractionated radiotherapy can be overcome by concurrent PD-L1 blockade. Cancer Res (2014) 74(19):5458–68. doi: 10.1158/0008-5472.CAN-14-1258
129. Demaria S, Golden EB, Formenti SC. Role of local radiation therapy in cancer immunotherapy. JAMA Oncol (2015) 1(9):1325–32. doi: 10.1001/jamaoncol.2015.2756
130. Twyman-Saint Victor C, Rech AJ, Maity A, Rengan R, Pauken KE, Stelekati E, et al. Radiation and dual checkpoint blockade activate non-redundant immune mechanisms in cancer. Nature (2015) 520(7547):373–7. doi: 10.1038/nature14292
131. Theelen W, Peulen HMU, Lalezari F, van der Noort V, de Vries JF, Aerts J, et al. Effect of pembrolizumab after stereotactic body radiotherapy vs pembrolizumab alone on tumor response in patients with advanced non-small cell lung cancer: Results of the PEMBRO-RT phase 2 randomized clinical trial. JAMA Oncol (2019) 5(9):1276–82. doi: 10.1001/jamaoncol.2019.1478
132. Welsh J, Menon H, Chen D, Verma V, Tang C, Altan M, et al. Pembrolizumab with or without radiation therapy for metastatic non-small cell lung cancer: a randomized phase I/II trial. J Immunother Cancer (2020) 8(2):e001001. doi: 10.1136/jitc-2020-001001
133. Shang S, Liu J, Verma V, Wu M, Welsh J, Yu J, et al. Combined treatment of non-small cell lung cancer using radiotherapy and immunotherapy: challenges and updates. Cancer Commun (Lond) (2021) 41(11):1086–99. doi: 10.1002/cac2.12226
134. Prelaj A, Pircher CC, Massa G, Martelli V, Corrao G, Lo Russo G, et al. Beyond first-line immunotherapy: Potential therapeutic strategies based on different pattern progressions: Oligo and systemic progression. Cancers (Basel) (2021) 13(6):1300. doi: 10.3390/cancers13061300
135. Salous T, Shukla NA, Althouse SK, Perkins SM, Furqan M, Leal T, et al. A phase 2 trial of chemotherapy plus pembrolizumab in patients with advanced non-small cell lung cancer previously treated with a PD-1 or PD-L1 inhibitor: Big ten cancer research consortium BTCRC-LUN15-029. Cancer (2022) 129(2):264–71. doi: 10.1002/cncr.34565
136. Spigel DR, Reynolds C, Waterhouse D, Garon EB, Chandler J, Babu S, et al. Phase 1/2 study of the safety and tolerability of nivolumab plus crizotinib for the first-line treatment of anaplastic lymphoma kinase translocation - positive advanced non-small cell lung cancer (CheckMate 370). J Thorac Oncol (2018) 13(5):682–8. doi: 10.1016/j.jtho.2018.02.022
137. Yang JC, Gadgeel SM, Sequist LV, Wu CL, Papadimitrakopoulou VA, Su WC, et al. Pembrolizumab in combination with erlotinib or gefitinib as first-line therapy for advanced NSCLC with sensitizing EGFR mutation. J Thorac Oncol (2019) 14(3):553–9. doi: 10.1016/j.jtho.2018.11.028
138. Riudavets M, Naigeon M, Texier M, Dorta M, Barlesi F, Mazieres J, et al. Gefitinib plus tremelimumab combination in refractory non-small cell lung cancer patients harbouring EGFR mutations: The GEFTREM phase I trial. Lung Cancer (2022) 166:255–64. doi: 10.1016/j.lungcan.2021.11.018
139. Levy B, Barlesi F, Paz-Ares L, Bennouna J, Erman M, Felip E, et al. Phase II study of afatinib plus pembrolizumab in patients with squamous cell carcinoma of the lung following progression during or after first-line chemotherapy (LUX-Lung-IO). Lung Cancer (2022) 166:107–13. doi: 10.1016/j.lungcan.2022.01.023
140. Manegold C, Dingemans AC, Gray JE, Nakagawa K, Nicolson M, Peters S, et al. The potential of combined immunotherapy and antiangiogenesis for the synergistic treatment of advanced NSCLC. J Thorac Oncol (2017) 12(2):194–207. doi: 10.1016/j.jtho.2016.10.003
141. Moya-Horno I, Viteri S, Karachaliou N, Rosell R. Combination of immunotherapy with targeted therapies in advanced non-small cell lung cancer (NSCLC). Ther Adv Med Oncol (2018) 10:1758834017745012. doi: 10.1177/1758834017745012
142. Herbst RS, Arkenau HT, Santana-Davila R, Calvo E, Paz-Ares L, Cassier PA, et al. Ramucirumab plus pembrolizumab in patients with previously treated advanced non-small-cell lung cancer, gastro-oesophageal cancer, or urothelial carcinomas (JVDF): a multicohort, non-randomised, open-label, phase 1a/b trial. Lancet Oncol (2019) 20(8):1109–23. doi: 10.1016/S1470-2045(19)30458-9
143. Gao RL, Song J, Sun L, Wu ZX, Yi XF, Zhang SL, et al. Efficacy and safety of combined immunotherapy and antiangiogenesis with or without chemotherapy for advanced non-small-cell lung cancer: A systematic review and pooled analysis from 23 prospective studies. Front Pharmacol (2022) 13:920165. doi: 10.3389/fphar.2022.920165
144. Neal J, Lim F, Felip E, Gentzler R, Patel S, Baranda J, et al. Cabozantinib plus atezolizumab in NSCLC patients previously treated with a checkpoint inhibitor: Results from COSMIC 021. J Thorac Oncol (2021) 16(S3):S230–1. doi: 10.1016/j.jtho.2021.01.151
145. Neal J, Kundu P, Tanaka T, Enquist I, Patel S, Balestrini A, et al. CONTACT-01: A phase III, randomized study of atezolizumab plus cabozantinib versus docetaxel in patients with metastatic non-small cell lung cancer (mNSCLC) previously treated with PD-L1/PD-1 inhibitors and platinum-containing chemotherapy. J OF Clin Oncol (2021) 39(S15):TPS9134–TPS9134. doi: 10.1200/JCO.2021.39.15_suppl.TPS9134
146. Leal T, Berz D, Rybkin I, Iams W, Bruno D, Blakely C, et al. MRTX-500: Phase II trial of sitravatinib (sitra) plus nivolumab (nivo) in patients (pts) with non-squamous (NSQ) non-small cell lung cancer (NSCLC) progressing on or after prior checkpoint inhibitor (CPI) therap. Ann Oncol (2022) 33(S1):S19–20. doi: 10.1016/j.annonc.2022.01.052
147. Percent I, Reynolds C, Konduri K, Whitehurst M, Nidhiry E, Yanagihara R, et al. Phase III trial of sitravatinib plus nivolumab vs. docetaxel for treatment of NSCLC after platinum-based chemotherapy and immunotherapy (SAPPHIRE). J OF Clin Oncol (2020) 38(S15):TPS9635–TPS9635. doi: 10.1200/JCO.2020.38.15_suppl.TPS9635
148. Gao B, Ma Z, Yu X, Huang D, Zhao J, Day D, et al. Sitravatinib plus tislelizumab in patients with anti-PD-(L)1 refractory/resistant metastatic NSCLC. Ann Oncol (2021) 32(S5):S998–8. doi: 10.1016/j.annonc.2021.08.1886
149. Shalata W, Iraqi M, Bhattacharya B, Fuchs V, Roisman LC, Cohen AY, et al. Rapid response to the combination of lenvatinib and pembrolizumab in patients with advanced carcinomas (Lung adenocarcinoma and malignant pleural mesothelioma). Cancers (Basel) (2021) 13(14):3630. doi: 10.3390/cancers13143630
150. Puri S, Tanvetyanon T, Creelan B, Shafique M, Saltos A, Chiappori A, et al. Phase II study of nivolumab and ipilimumab combined with nintedanib in recurrent non-small cell lung cancer. J OF Thorac Oncol (2021) 16(S10):S924–5. doi: 10.1016/j.jtho.2021.08.176
151. El-Khoueiry AB, Hanna DL, Llovet J, Kelley RK. Cabozantinib: An evolving therapy for hepatocellular carcinoma. Cancer Treat Rev (2021) 98:102221. doi: 10.1016/j.ctrv.2021.102221
152. Maroto P, Porta C, Capdevila J, Apolo AB, Viteri S, Rodriguez-Antona C, et al. Cabozantinib for the treatment of solid tumors: a systematic review. Ther Adv Med Oncol (2022) 14:17588359221107112. doi: 10.1177/17588359221107112
153. Du W, Huang H, Sorrelle N, Brekken RA. Sitravatinib potentiates immune checkpoint blockade in refractory cancer models. JCI Insight (2018) 3(21):e124184. doi: 10.1172/jci.insight.124184
154. Msaouel P, Genovese G, Gao J, Sen S, Tannir NM. TAM kinase inhibition and immune checkpoint blockade- a winning combination in cancer treatment? Expert Opin Ther Targets (2021) 25(2):141–51. doi: 10.1080/14728222.2021.1869212
155. Voron T, Colussi O, Marcheteau E, Pernot S, Nizard M, Pointet AL, et al. VEGF-a modulates expression of inhibitory checkpoints on CD8+ T cells in tumors. J Exp Med (2015) 212(2):139–48. doi: 10.1084/jem.20140559
156. Kato Y, Tabata K, Kimura T, Yachie-Kinoshita A, Ozawa Y, Yamada K, et al. Lenvatinib plus anti-PD-1 antibody combination treatment activates CD8+ T cells through reduction of tumor-associated macrophage and activation of the interferon pathway. PloS One (2019) 14(2):e0212513. doi: 10.1371/journal.pone.0212513
157. Kato R, Haratani K, Hayashi H, Sakai K, Sakai H, Kawakami H, et al. Nintedanib promotes antitumour immunity and shows antitumour activity in combination with PD-1 blockade in mice: potential role of cancer-associated fibroblasts. Br J Cancer (2021) 124(5):914–24. doi: 10.1038/s41416-020-01201-z
158. Leighl NB, Redman MW, Rizvi N, Hirsch FR, Mack PC, Schwartz LH, et al. Phase II study of durvalumab plus tremelimumab as therapy for patients with previously treated anti-PD-1/PD-L1 resistant stage IV squamous cell lung cancer (Lung-MAP substudy S1400F, NCT03373760). J Immunother Cancer (2021) 9(8):e002973. doi: 10.1136/jitc-2021-002973
159. Tolcher A, Reeves J, McKean M, Chmielowski B, Beck J, Shaheen M, et al. Preliminary results of a phase II study of alrizomadlin (APG-115), a novel, small-molecule MDM2 inhibitor, in combination with pembrolizumab in patients (pts) with unresectable or metastatic melanoma or advanced solid tumors that have failed immuno-oncologic (I-O) drugs. J OF Clin Oncol (2021) 39(S15):2506–2506. doi: 10.1200/JCO.2021.39.15_suppl.2506
160. Shafique M, Fisher T, Evans E, Leonard J, Pastore D, Mallow C, et al. Interim results from a phase Ib/II study of pepinemab in combination with avelumab in advanced NSCLC patients following progression on prior systemic and/or anti-PDx therapies. J OF Clin Oncol (2020) 38(S5):75–75. doi: 10.1200/JCO.2020.38.5_suppl.75
161. Hellmann MD, Janne PA, Opyrchal M, Hafez N, Raez LE, Gabrilovich DI, et al. Entinostat plus pembrolizumab in patients with metastatic NSCLC previously treated with anti-PD-(L)1 therapy. Clin Cancer Res (2021) 27(4):1019–28. doi: 10.1158/1078-0432.CCR-20-3305
162. Shafique MR, Fisher TL, Evans EE, Leonard JE, Pastore DRE, Mallow CL, et al. A phase Ib/II study of pepinemab in combination with avelumab in advanced non-small cell lung cancer. Clin Cancer Res (2021) 27(13):3630–40. doi: 10.1158/1078-0432.CCR-20-4792
163. Zhao Y, Fang W, Yang Y, Chen J, Zhuang L, Du Y, et al. A phase II study of AK112 (PD-1/VEGF bispecific) in combination with chemotherapy in patients with advanced non-small cell lung cancer. J OF Clin Oncol (2022) 40(S16):9019–9019. doi: 10.1200/JCO.2022.40.16_suppl.9019
164. Zhao H, Ma Y, Zhang Y, Hong S, Yang Y, Fang W, et al. The preliminary efficacy and safety data of KN046 in patients failed on prior immune checkpoint inhibitors therapy. J OF Clin Oncol (2020) 38(S15):3020–3020. doi: 10.1200/JCO.2020.38.15_suppl.3020
165. Johnson ML, Chadjaa M, Yoruk S, Besse B. Phase III trial comparing antibody-drug conjugate (ADC) SAR408701 with docetaxel in patients with metastatic non-squamous non-small cell lung cancer (NSQ NSCLC) failing chemotherapy and immunotherapy. J Clin Oncol (2021) 38(S15):TPS9625–TPS9625. doi: 10.1200/JCO.2020.38.15_suppl.TPS9625
166. Herbst RS, Garon EB, Kim DW, Cho BC, Perez-Gracia JL, Han JY, et al. Long-term outcomes and retreatment among patients with previously treated, programmed death-ligand 1−Positive, advanced Non−Small-cell lung cancer in the KEYNOTE-010 study. J Clin Oncol (2020) 38(14):1580–90. doi: 10.1200/JCO.19.02446
167. Giaj Levra M, Cotte FE, Corre R, Calvet C, Gaudin AF, Penrod JR, et al. Immunotherapy rechallenge after nivolumab treatment in advanced non-small cell lung cancer in the real-world setting: A national data base analysis. Lung Cancer (2020) 140:99–106. doi: 10.1016/j.lungcan.2019.12.017
168. Teraoka S, Akamatsu H, Takamori S, Hayashi H, Miura S, Hata A, et al. A phase II study of nivolumab rechallenge therapy in advanced NSCLC patients who responded to prior anti-PD-1/L1 inhibitors: West Japan oncology group 9616L. Ann Oncol (2021) 32(S5):S1001–2. doi: 10.1016/j.annonc.2021.08.1893
169. Ponce Aix S, Carcereny Costa E, Bosch-Barrera J, Felip Font E, Guirado M, Coves Sarto J, et al. Pembrolizumab re-challenge in patients with relapsed non-small cell lung cancer (NSCLC): A preliminary report of the REPLAY phase II trial - cohort I. Ann Oncol (2021) 32(S7):S1450–0. doi: 10.1016/j.annonc.2021.10.179
Keywords: NSCLC, immunotherapy, TME, immune cells, clinical strategies
Citation: Zhou S and Yang H (2023) Immunotherapy resistance in non-small-cell lung cancer: From mechanism to clinical strategies. Front. Immunol. 14:1129465. doi: 10.3389/fimmu.2023.1129465
Received: 22 December 2022; Accepted: 28 March 2023;
Published: 06 April 2023.
Edited by:
Zhaohua Hou, Memorial Sloan Kettering Cancer Center, United StatesReviewed by:
Jonghwa Won, ABL Bio/Sang Hoon Lee, Republic of KoreaArpad Szoor, University of Debrecen, Hungary
Yue Chen, Cedars Sinai Medical Center, United States
Copyright © 2023 Zhou and Yang. This is an open-access article distributed under the terms of the Creative Commons Attribution License (CC BY). The use, distribution or reproduction in other forums is permitted, provided the original author(s) and the copyright owner(s) are credited and that the original publication in this journal is cited, in accordance with accepted academic practice. No use, distribution or reproduction is permitted which does not comply with these terms.
*Correspondence: Haihua Yang, yhh93181@163.com