- Department of Biomolecular Sciences, University of Urbino Carlo Bo, Urbino, Italy
Introduction: Upper respiratory tract infections (URTI) are the most common illnesses affecting athletes, causing absences from training and competition. Salivary immunoglobulin A (sIgA) is the main immune factor in saliva, and a consistent association between low concentrations of sIgA and an increased incidence of URTIs has been reported. The oral probiotic Streptococcus salivarius K12 has been suggested to have the potential to improve oral diseases and mucosal barrier function. However, the effects of this probiotic on active young subjects performing a high-intensity training (HIT) program have not been investigated.
Methods: Active young students were randomised into a treated group (S. salivarius K12) and a control (placebo) group and asked to take the product daily for 30 days. After this period, participants performed a graded exercise test and five HIT sessions, all within 3 days. They were also asked to complete the Wisconsin Upper Respiratory Symptom Survey daily to monitor URTI’s presence. Before and after the 30 days, and at 0h, 24h and 72h after the last training session, saliva samples were collected to quantify sIgA level, secretion rate, and flow. The effect of S. salivarius K12 intake on these parameters was tested using an ANOVA for repeated measures.
Results: Twenty (M = 14, F = 6) young subjects (23.5 ± 2.3 years old) participated in the study. The total accumulated training load (sRPE) in the supplementation period was similar in the two groups (treated: 4345 ± 3441 AU; control: 4969 ± 4165 AU; p > 0.05). Considering both sIgA level and secretion rate, significant time (F(4,15) = 3.38; p = 0.037; F(4,15) = 6.00; p = 0.004) and time×group interactions (F(4,15) = 2.49; p = 0.049; F(4,15) = 5.01; p = 0.009) were reported, with the treated group showing higher sIgA levels at 72h post-exercise and increased secretion rate both at 0h and 72h. The number of URTI episodes was similar in the treated and control groups (χ² = 1.83; p > 0.05).
Conclusion: This study demonstrates that relatively short-term S. salivarius K12 supplementation increased sIgA level and secretion in healthy subjects performing a demanding exercise-training programme composed of HIT sessions.
1. Introduction
Exercise stimulates an immune response, dependent on the intensity, duration, and type of the stimulus (1–3). The athletes’ immune response capacity depends on parameters such as gender, age, clinical condition, and nutritional and training status (4, 5). However, high-intensity exercises may impair immune function and increase susceptibility to infection even in apparently healthy athletes (6). Exercise is defined as high intensity when it exceeds 70% of VO2max (7). High-intensity interval training (HIT) is typically accomplished through intervals, as opposed to moderate-intensity continuous training, which is characterised by extended, continuous activity. HIT is characterised by a series of short (10 seconds) to long (5 minutes) intervals carried out at an intensity higher than the anaerobic threshold (7). HIT may temporarily perturb the immune system, triggering a decrease in immunological activity (8).
Upper respiratory tract infections (URTIs), including nose, sinuses and pharyngeals (9), are very common in athletes undergoing intense training, such as elite athletes (10, 11), which generally show a 2-6 times higher risk of URTI following exposure to the pathogen and/or environmental factors compared to non-athletes (12, 13). Intense training is acutely correlated with a reduction of several cellular and humoral elements characteristic of the immune response, including absolute salivary secretory immunoglobulin A (sIgA) concentration and secretory rate of sIgA (8, 14). This acute response seems to support the “open window” theory, the increased susceptibility to opportunistic infections from 3 to 72 hours following intense exercise (15, 16).
In the last years, oral probiotics’ use to promote oral health, salivary flow rate and sIgA contents gained increasing attention due to the possibility of preventing dental caries (17) and oral episodes of streptococcal and tonsillitis pharyngeal infections (18). This approach, based on a precision probiotics strategy (19), has also been defined as “bacterial therapy” or “bioprotic therapy” (18). A relevant example is the Streptococcus salivarius K12 microorganism isolated from the mouth of a healthy child (20). S. salivarius K12 is able to inhibit the growth of strains of Streptococcus pyogenes b-haemolytic (Lancefield group A, a common cause of tonsillitis and bacterial pharyngitis) (21, 22) and those of other pathogenic bacteria such as Micrococcus luteus, Streptococcus anginosus, Eubacterium saburreum, Micromonas micros, Streptococcus pneumonia, Haemophilus influenzae and Moraxella catarrhalis (23–25). This action is mainly due to lantibiotic cationic peptides Salivaricin A2 and Salivaricin B, encoded by the 190 kb megaplasmid found in the K12 strain (26). Notably, the S. salivarius K12 strain showed persistent colonisation of different upper respiratory tract tissues in newborns using the oral probiotic S. salivarius K12 (27, 28). Despite this recent evidence, the role of S. salivarius K12 as a precision probiotic to counteract athletes’ risk of developing URTI is still largely unknown.
In this study, twenty physically active subjects were randomised to receive either the S. salivarius K12 or placebo for 30 days and then performed a VO2max test and five HIT sessions within three days. The absolute level and secretion rate of sIgA and URTI episodes were analysed throughout the study. Here, we hypothesised that the prophylactic administration of the S. salivarius K12 might increase the salivary flow rate and sIgA levels and reduce URTI episodes in the treated group compared to the placebo group.
2. Materials and methods
2.1. Participants
Participants were recruited from students at the University of Urbino Carlo Bo, Italy. Inclusion criteria were: age between 20 and 25 years; being in good health with no chronic disease; had not taken supplements at least three months before the start of the study; having practised physical activity at least three times a week in the last year. Participants signed a written informed consent before the study. The power analysis was performed considering the repeated measures ANOVA test: considering time as a repeated measure (five measures) and group membership as a binary between factor, with a cautionary non-sphericity correction coefficient = 0.8, with effect size f = 0.68, alfa = 0.05 and 1-beta = 0.8, a total of 24 subjects must be enrolled (29). The study was conducted according to the principles stated in the Declaration of Helsinki, and it was approved by the Ethics Committee for Human Experimentation of Urbino University Carlo Bo (no. of approval 29_2020).
2.2. Experimental design
The participants were asked to take either a preparation containing the probiotic S. salivarius K12 (Bactoblis®, PharmExtracta S.p.A., Pontenure, Italy) or a placebo with the same characteristics in terms of consistency, taste, and smell. Participants were randomised using block randomisation with blocks of n = 4. The participants and staff enrolling patients were all blinded to treatment conditions. The membership was randomly assigned using a computer-generated random sequence. The compound was taken daily, starting 30 days before the phase including a graded exercise test (GXT) and HIT sessions, and throughout the protocol. Participants were asked to take the compound in the evening as a last gesture before going to sleep. In addition, participants were asked each evening to complete the Wisconsin Upper Respiratory Symptom Survey (WURSS-11) to monitor physical activity levels and health status, particularly the possible presence of URTI (30). URTI episodes were recorded through the 30 days of S. salivarius K12 supplementation, the GXT and HIT sessions, and one week after the last training session. URTI episodes were defined by answering “Yes” to the question “Do you think you have a cold?” and scoring at least 2 points on the Jackson scale, which sums the scores of eight cold symptoms, rated on a Likert scale ranging from 0 = absent to 3 = severe. Daily physical activity was monitored by asking the participant if they trained that day, and if yes, the participant indicated which sport they practised, if it was outdoor or indoor, the duration of the training session in minutes, and the perceived intensity of the whole session (on a Borg CR-10 scale). An operator reminded participants to take the product and complete the online questionnaire. On the 31st day after the start of intake, participants were invited to the laboratories in an overnight fasting state (between 8:00 and 9:00) to proceed with the start of the GXT and HIT sessions phase. The procedure involved performing the first salivary sampling, which was immediately followed by the execution of a maximal GXT to assess maximum oxygen consumption and power at the anaerobic threshold. Then all participants were recalled in the afternoon of the same day to begin HIT sessions, as described in Table 1 and Figure 1. The baseline assessment of the abundance of the genus Streptococcus in the subjects evaluated was not considered necessary since the literature already describes the colonising capacity of S. salivarius K12 both in adults (27) and children (28), against group A Streptococci (such as pyogenes) and group B Streptococci (such as agalactiae) (31). It is also reported a superiority in competitive antagonism compared to other streptococci due to the known production of salivaricins and to the adhesion capacity (32), mechanisms which overall, together with a blocking capacity of adhesion sites, were also shown to be effective against Streptococcus pneumoniae (33).
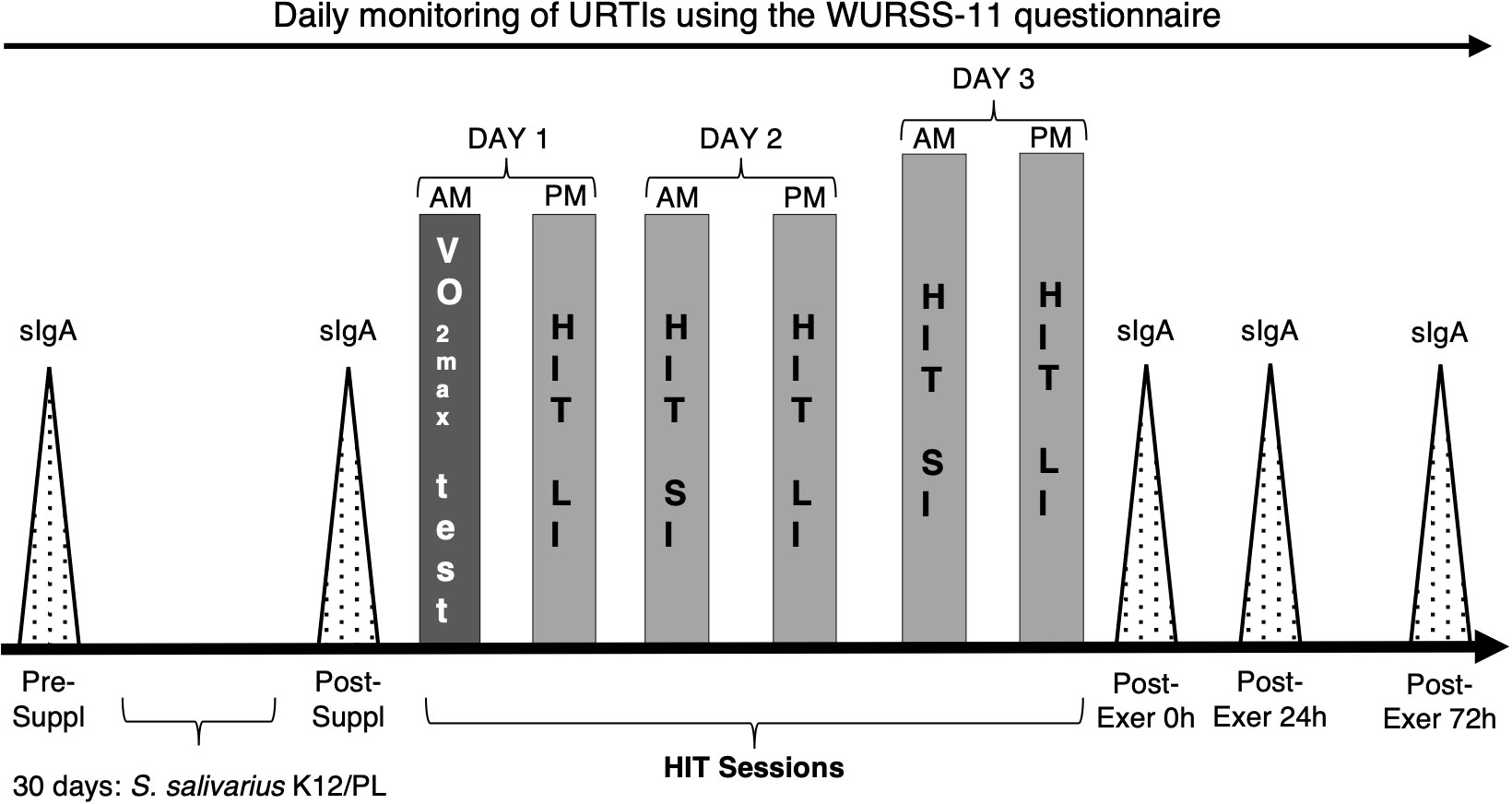
Figure 1 Experimental design. S. salivarius K12: S. salivarius supplement; PL: placebo compound; sIgA: salivary test sessions of immunoglobulin A; Pre-Suppl: salivary tests before S. salivarius K12/PL supplementation; 30 days: S. salivarius K12/PL: period of S. salivarius K12 or PL intake; Post-Suppl: salivary tests after S. salivarius K12 or PL intake; Post-Exer 0h-24h and 72h: salivary tests immediately after training sessions, after 24 and after 72h. AM, morning session; PM afternoon session. VO2max test session; HIT LI: long intervals of high-intensity training; HIT SI: short intervals of high-intensity training.
2.3. Product characteristics
The testing product (Bactoblis®), in accordance with Italian law no. 169/2004, was notified to the Minister of Health in July 2011 (registration no. 53435) and registered as a food supplement. It contains, at the time of manufacturing, 5 billion CFU/tablet of S. salivarius K12 ATCC BAA-1 024 (BLIS Technologies Ltd., New Zealand) and has been manufactured by S.I.I.T. (Trezzano sul Naviglio, Milan, Italy) in the form of a round-shaped, vanilla-flavoured, slowly dissolving tablet. The placebo product was realised in the same form, colour, consistency, solving time, and flavour but with the complete absence of probiotic bacteria.
2.4. Saliva samples collection and analysis
Saliva samples were collected in the morning (between 8:00 and 9:00), at the following moments: before and after 30 days of S. salivarius K12 administration, and immediately, 24h and 72h after the last exercise training session. Saliva samples were taken by the oral fluid collector (OFC) swab (Soma Bioscience, Wallingford, United Kingdom) following the manufacturer’s instructions (34). Briefly, the OFC swab was put on the top of the tongue until the indicator on the stem turned blue (typically after 1-4 min). During sampling, participants were asked to perform minimal orofacial movement and not suck the swab. The OFC swab collected 0.5 ml of saliva. The whole swab was then placed in the bottle containing the OFC buffer solution and gently mixed by rotation for 10 min at room temperature using a hybridisation oven/shaker (Amersham Biosciences Europe GmbH, Milan, Italy). Three drops of the saliva/buffer mix were added to the sample window of the SOMA sIgA & Cortisol lateral flow device (LFD) and incubated at room temperature for 15 min. The LFD was scanned using the SOMA Cube reader. The sIgA secretion rate was determined by multiplying the saliva flow (0.5 mL × min-1) by the sIgA concentration (µg × mL-1). All saliva samples were collected after an overnight fast, and participants were asked not to chew gum or brush their teeth before testing to reduce saliva measurement error. Furthermore, volunteers were prohibited from consuming alcoholic beverages or caffeine for 24 hours before saliva collection.
2.5. Graded exercise testing procedures
After taking the compound for 30 days and immediately after collecting saliva samples, participants performed a GXT to obtain the power value at maximum oxygen consumption (VO2max) and power value at the Ventilatory Threshold (VT2). These parameters were used to plan the training sessions using the HIT method. Specifically, the participants used the same cycle ergometer for testing and training sessions (Skillbike, Technogym SpA, Cesena, Italy). The GXT was performed with a ramped procedure in increments of 25W min-1 until exhaustion immediately after a conventional 10-minute warm-up at 50W, according to Pallares et al. (35). Polar H10 sensors (Polar Electro Oy, Kempele, Finland) recorded participants’ heart rates during the GXT. Each participant indicated their rate of perceived exertion every minute using the CR10 Scale 0–10, where 0 is defined as no exertion at all, and 10 as “maximum, strenuous” effort [according to Foster et al. (36)]. The peak power value of the last step of GXT was used to derive the values of VO2max and power at VT2.
2.6. Training protocol and training monitoring
The participants performed six sessions in three days, where the first session was the GXT. The anti-meridian (AM) sessions start between 08:30 AM and 01:00 post-meridian (PM), while the PM sessions start between 02:00 and 07:00 PM. The HIT sessions were designed with the aim of overexercising participants who had no experience with the HIT method or the twice-daily sessions. Each HIT session was performed as group cycling training (5 members per group) by projecting the day’s session schedule on the wall in real-time. Specifically, we planned two formats: short intervals, alternating intervals with power above threshold (110-150%), and recovery intervals at intensities between 30 and 40% VO2max power. On the other hand, long intervals involved intervals between 90-100% of threshold alternating with recovery intervals at intensities between 40-50% of power at VO2max. Each session began with a warm-up phase and ended with a cool-down phase. In addition, the time of the sessions was increased from 45 to 60 minutes (second to third day) by increasing the number of intervals (see Table 1). To monitor the participants’ training load, after 30 minutes of each training session, a sports scientist asked each participant to provide him or her with the perceived exertion rate of the entire session (sRPE). The CR10 scale value was multiplied by the minutes of each training session.
2.7. Statistical analysis
Descriptive statistics were reported as mean ± standard deviation (SD). Comparisons between GXT data were performed using two-sample t-tests. The effect of S. salivarius K12 supplementation, salivary flow, sIgA level and secretion rate was tested using an ANOVA for repeated measures with interaction; dependent variable levels (repeated measures; within-factor) were compared at five time-points: before the supplementation period (pre-suppl), after 30 days of supplementation (post-suppl), and immediately (0h), 24h and 72h after the last HIT session (post-exer 0h, 24h and 72h). Group (treated vs control) was a binary between-factor. Simple contrast analysis (reference category = pre-suppl category) was used to verify if salivary flow, sIgA level and secretion changed between the time points and the groups. Finally, Pearson’s correlation indexes were calculated between flux and sIgA secretion for all measurement times. Due to the small sample size and the sex distribution (14 M/6 F), it was not possible to make any reliable investigation of between-sex differences. All elaborations were performed using SPSS version 22.0 or Excel 365; significance was set at p < 0.05.
3. Results
Twenty-four healthy young adults were enrolled for the experimental protocol of the study and were randomly assigned in an equal number to the treated or control group. Twenty of them completed the study (14 males and 6 females), while four abandoned it for personal reasons. The demographic and anthropometric characteristics of the entire group of participants were: age 23.5 ± 2.3 years; height 173.5 ± 10.9 cm; weight 71.9 ± 13.1 kg; BMI: 23.7 ± 2.8 kg/m2. All subjects took the S. salivarius K12 or a placebo for 30 days, with an adherence rate of 94% (87-100%). At the end of the supplementation period, they underwent three days of acute exercise, comprising a GXT and five HIT sessions.
3.1. Graded exercise test and training protocol
Results of the GXT are reported in Table 2. No differences were detected in the recorded variables between the treated and control groups.
During the HIT sessions, the mean power was 65 ± 5% of the threshold power, and the mean heart rate was 84 ± 4% of the maximal heart rate. However, it should be noted that the mean data of the sessions also include warm-up, cool-down, and recovery phases between high-intensity intervals, so this summary statistic does not reflect the intensity of the training sessions. The mean RPE values were 7.5 ± 1.2 AU, indicating the overall effort perceived by the participants. Overall, the total accumulated training load, computed as session RPE, was 2280 ± 249 AU for the control group and 2260 ± 376 AU in the treated group; the mean training load was similar between groups (p > 0.05).
3.2. Salivary flow rate, sIgA level and secretion
Salivary flow rate, sIgA level and secretion at pre-suppl, post-suppl and post-exer 0h, 24h and 72h after the last training session are reported in Figure 2.
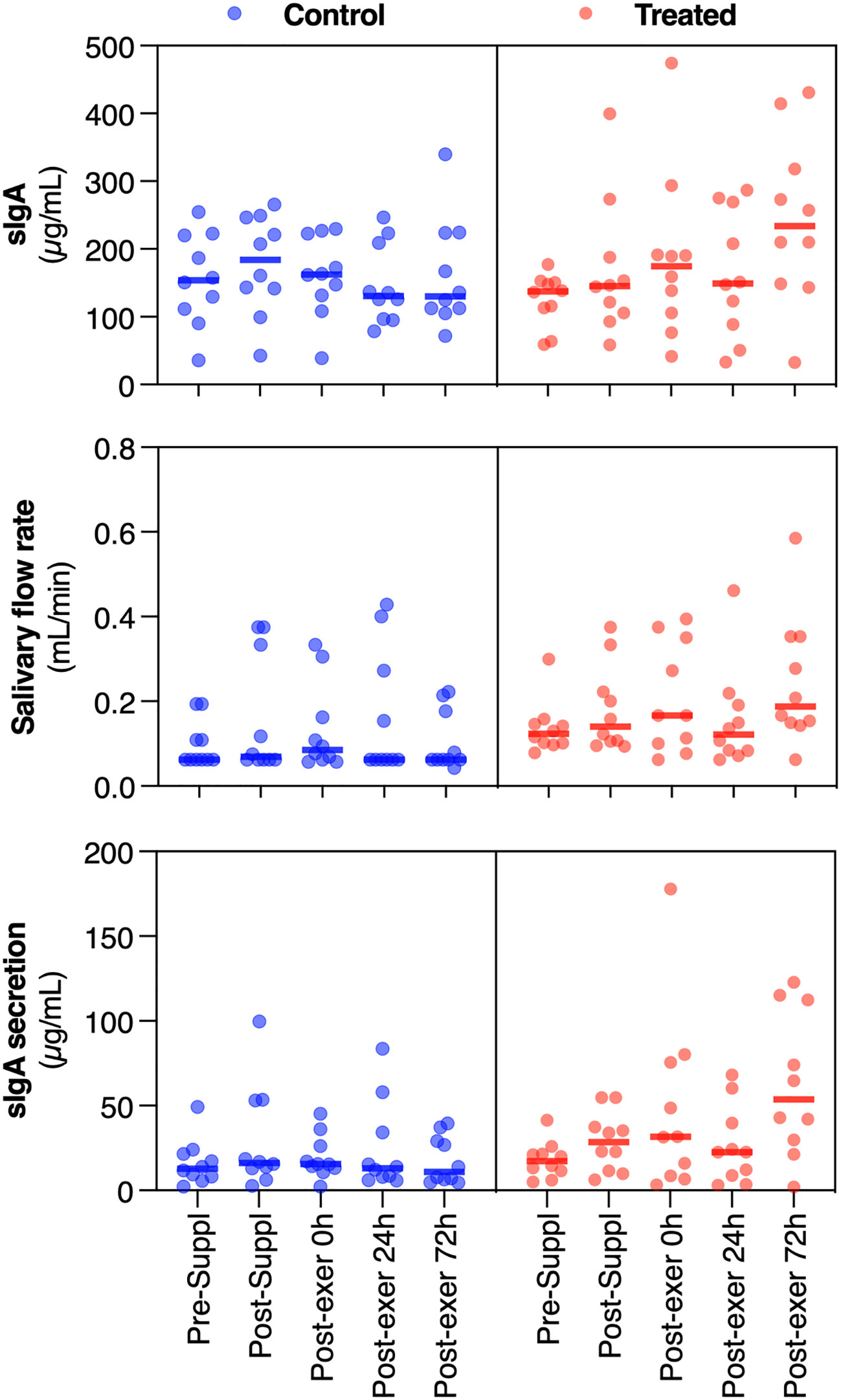
Figure 2 Dot plots of the measured variables (sIgA, salivary flow rate and sIgA secretion), for the treated and placebo groups, at the different time-points (in abscissa axis). The thick line in each set of points represents the median value. Orange dots are about the treated group, while blue dots are about the control group.
Considering salivary flux, repeated measures ANOVA revealed non-significant overall time (F(4,15)=2.41; p=0.09; =0.391), while time×group (F(4,15) = 3.81; p = 0.025; = 0.504) was significant. In particular, simple contrasts show that salivary flow remains approximately constant across groups except at post-exercise 72h, whose value was significantly higher in the treated group than in the control group.
Considering sIgA, repeated measures ANOVA revealed significant time (F(4,15) = 3.38; p = 0.037; = 0.474) and time×group (F(4,15) = 2.49; p = 0.049; = 0.122) effects. Simple contrasts analysis showed a quasi-significant increment of sIgA in the post-suppl (30 days) condition (p = 0.069) with respect to the baseline value, while a significant increase can be noted in the post-exercise 72h condition (p = 0.007). Moreover, in the post-exercise 72h condition, the treated group showed significantly higher IgA levels (p = 0.026). Relative changes (% of variation) of sIgA with respect to pre-supplementation values are reported in Figure 3.
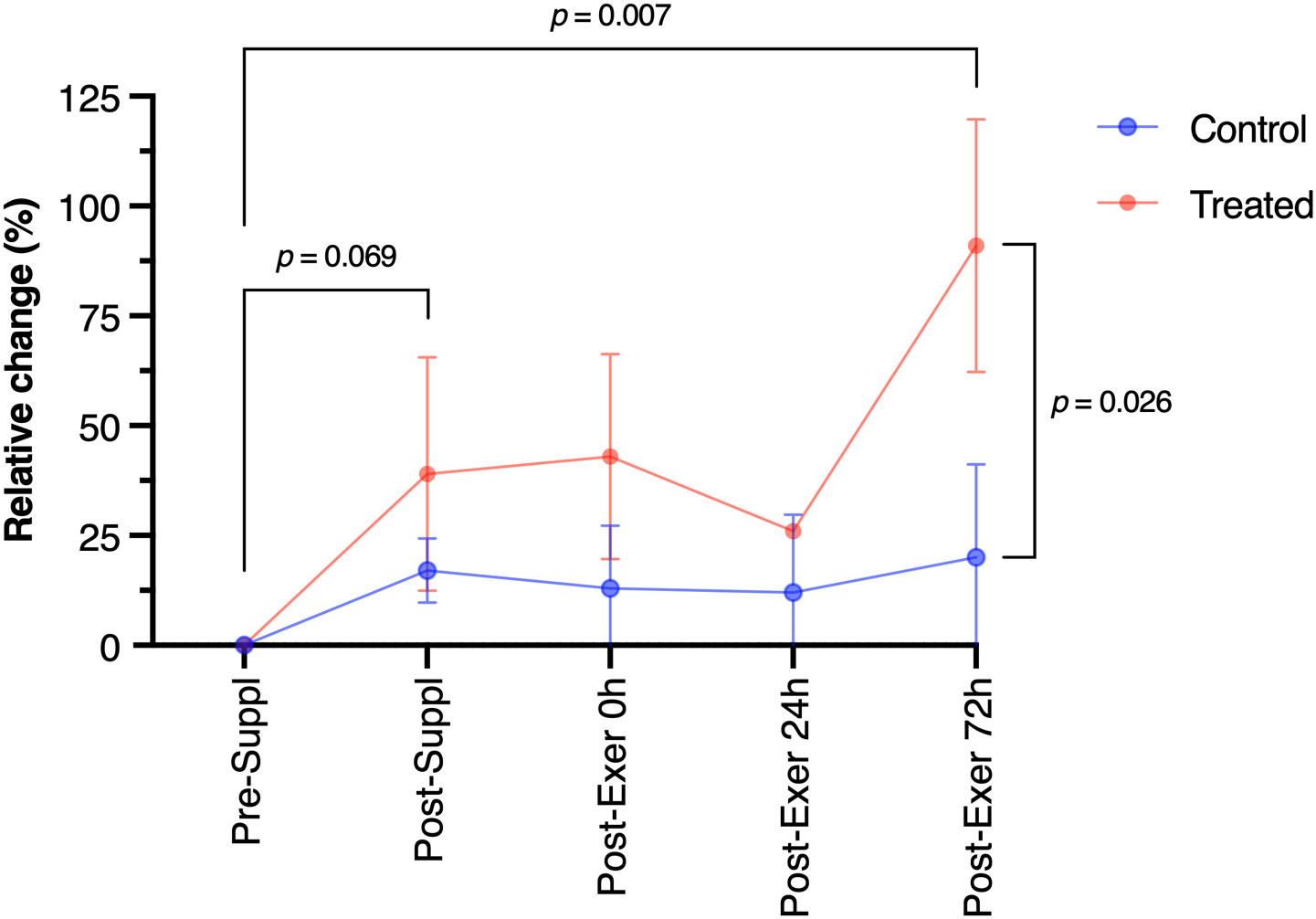
Figure 3 Immunoglobulin A (sIgA) relative change along the study period. Phase refers to before the supplementation period (pre-suppl), after 30 days of supplementation (post-suppl), immediately after the last training session (post-exer 0h), and the remaining time-points refer respectively to 24h and 72h after the last training session (post-exer 24h and 72h). Orange dots are about the treated group, while blue dots are about the control group.
Finally, considering sIgA secretion, repeated measures ANOVA revealed significant time (F(4,15) = 6.00; p = 0.004; = 0.615) and time×group (F(4,15) = 5.01; p = 0.009; = 0.572) effects. Simple contrasts revealed an increase in sIgA secretion in the treated group at 0 and 72 hours after exercise. Pearson’s R values considering between flux and IgA secretion variables were, for all times tested, >0.8, showing a significant correlation between variables.
3.3. URTI episodes
URTI episodes were recorded using the WURSS-11 questionnaire. The number of episodes in the treated and control groups is reported in Table 3. The Chi-square test did not reveal significant differences between the groups (χ² = 1.83; p > 0.05). The total accumulated training load (sRPE) in the supplementation period was roughly the same in the two groups (treated: 4345 ± 3441 AU; control: 4969 ± 4165; p > 0.05).
4. Discussion
This study aimed to investigate if 30 days of prophylactic administration of S. salivarius K12 could support the mucosal immune function of active young subjects performing HIT workouts. The main results of this study show a slight increase in sIgA level after the S. salivarius K12 supplementation and increased sIgA level and secretion rate and salivary flow in the 0-72 h post HIT compared to the placebo group. The number of URTI episodes remained low throughout the study in both groups.
The human oral cavity contains over 700 bacterial species and represents the second most complex microbiota in the human body (37). Much evidence points to an association between bacterial pathogens and oral diseases, while little attention was paid to potentially beneficial bacterial species in the oral cavity (38). S. salivarius K12, first isolated from a child with healthy oral tissues, has gained interest in paediatric care due to its effectiveness in reducing recurrent streptococcal upper respiratory tract infections (25, 39–44). Moreover, in adults with recurrent oral streptococcal pathology, 90 days of S. salivarius K12 administration reduced pharyngeal tonsillitis by about 80% throughout the study and by 60% in the six months following the use of the probiotic (18). Recent evidence suggests that the supply of S. salivarius K12 may also be effective against viral infections (40, 42, 45, 46), a finding which is also relevant in the outbreak of the COVID-19 pandemic.
URTIs are the most common illnesses affecting athletes and can cause absences from training and competition (10, 11). Athletes showed an increased incidence of URTI during periods of intense training or competition (12, 13). Several risk factors and biomarkers associated with increased URTI episodes have been identified (47). sIgA is the most studied immune parameter, and there is a strong negative correlation between the incidence of URTI and sIgA levels in athletes (46). Despite the consistent association between lower concentrations of sIgA and an increased incidence of URTI, the effect of high-intensity exercise on sIgA levels has shown conflicting results. Some studies showed a transient decrease in sIgA up to 24 hours after strenuous training sessions or competitions (6, 46, 48–51), while others were unable to confirm such findings (52–54). A recent meta-analysis by Drummond and colleagues (46) demonstrated increased levels of sIgA after acute exercise, but only in trained subjects. In the present study, at the end of the 30 days training program, the sIgA level slightly increased in both the treated and control groups, although this improvement was greater in the S. salivarius K12 supplemented subjects. Notably, the subjects in this study were exercise trained and were able to maintain their habitual physical activity patterns throughout the experimental period. Accordingly, during the 30 days of supplementation, we recorded only eight and four mild-severity URTI events in the control and treated groups, respectively. Interestingly, Babina et al. (17) recently reported that 4 weeks of S. salivarius K12 supplementation did not affect the sIgA production, secretion, and salivary flux in healthy subjects. The different modality of S. salivarius K12 supplementation between our study and that of Babina et al. (17), which included 2- weeks of probiotic washout, might at least partially explain the divergent results. Moreover, all subjects recruited in the present study were moderately trained and continued their recreational physical activities during the S. salivarius K12 supplementation period. As a result, the effect of S. salivarius K12 supplementation on sIgA may be influenced by training status. Another possibility is that S. salivarius K12 supplementation might have an adjuvant effect on sIgA, fostering a synergic effect with exercise. This hypothesis is supported by the increased sIgA level observed in the S. salivarius K12-treated group during the HIT sessions, as described subsequently. Several studies suggest that the risk of URTI can be reduced with moderate stress provided chronically, delineating a J-shaped load-URTI relationship where both insufficient and excessive activity can contribute to the increase in the risk of infection (11, 55, 56). Interestingly, Leandro et al. (57) showed that exercise intensity thresholds correlated to an immune-depressive effect with reduced production of sIgA in duration >2 h and/or intensity >80% of maximal oxygen uptake. Along this line, in this study, we also analysed the effect of six high-intensity exercise sessions performed on three consecutive days to simulate a high training load typical of a training programme of recreational and elite athletes. Here, we found that the sIgA level and secretion did not change immediately and until 72 hours after the acute exercise sessions, despite the significant training load, confirmed by the high session RPE scores. Moreover, URTI episodes did not increase within one week after the high training load exposure. Thus, our results did not support the hypothesis of general transient sIgA reduction and immunosuppression after high-intensity exercises or increased training loads in recreationally active individuals. Interestingly, we also found that the S. salivarius K12-treated group showed increased sIgA levels after 30 days of probiotic supplementation, immediately after the HIT sessions, and until 72 h post-exercise. Moreover, both sIgA secretion and salivary flux increased in the treatment group in the post-exercise period compared to the control group. Taken together, our results agree with recent evidence showing that mucosal immune function is a highly adaptable system able to respond quickly to stress, including intensified training load (52). Moreover, the controlled indoor environment of the gym during the HIT sessions might have reduced the immunosuppression risk since it is known that unfavourable ambient conditions (e.g., excessive cold or heat, humidity, and exposure to air pollutants) affect local and systemic immune responses independently from the exercise load (52).
HIT exercise sessions are commonly introduced during a training programme to maximise central and peripheral adaptation in recreational and elite athletes (52). However, in subjects with low sIgA levels and secretion, the increased training load within a short period of time might increase the URTI risk. For example, a polarised training approach based on continuous and interval training sessions increased the URTI events in recreational male endurance runners but only in those with low basal sIgA levels (58). Moreover, sIgA concentration before the training intervention strongly correlated with the number of sick days during the following 12-week training period (58). In this scenario, the ability of S. salivarius K12 to boost the sIgA level and secretion in healthy trained subjects may represent an intriguing and interesting finding. The increased salivary flow rate observed 72h after HIT sessions in the treated group might also be relevant for mucosal immune function since a higher salivary flow is associated with greater buffering capacity, salivary rinse (elimination and dilution of undesirable components) and enhancement of the antimicrobial action (59).
The mechanisms explaining the effects of S. salivarius K12 administration on mucosal IgA have been recently described in the context of SARS-CoV infection (60, 61). Interestingly, S. salivarius K12 administration increased the IgA production against the SARS-CoV-2 Spike protein RBD in mice. Moreover, patients with severe COVID-19 showed a reduction of mucosal anti-RBD IgA. Accordingly, a randomised controlled trial recently demonstrated that the S. salivarius K12 supplementation to hospitalised COVID-19 patients improved the main disease markers (60). Possible mechanisms responsible for these effects include the induction of tonic type-I IFN responses (62) and the regulation of systemic and mucosal TGF-β1 levels, with TGF-β1 as an inducer of class switch recombination antibodies in IgA (63, 64). These data outline a picture consistent with what was observed in our study. Hence, the precision probiotic approach with S. salivarius K12 seems a promising candidate to increase sIgA level and optimise mucosal microbial colonisation and mucosal barrier function.
In this study, we demonstrated that relatively short-term S. salivarius K12 supplementation increased sIgA level and secretion in healthy subjects performing regular exercise training and a demanding exercise-training programme composed of HIT sessions with short recovery time. The limited number of subjects examined, the use of a single type of training stress, the few URTI episodes recorded during the study and the unbalanced ratio of genders constitute the work’s limitations. Future studies on larger samples over longer durations and the use of other types of training stresses (managed by intensity and duration) could provide a more complete view of the potential benefits of S. salivarius K12 supplementation on URTI incidence.
Data availability statement
The raw data supporting the conclusions of this article will be made available by the authors, without undue reservation.
Ethics statement
The studies involving human participants were reviewed and approved by Ethics Committee for Human Experimentation of Urbino University Carlo Bo (no. of approval 29_2020). The patients/participants provided their written informed consent to participate in this study.
Author contributions
AB, MG, GA, and DS conceived and drew the experiment, MG and GA performed the data collection, MR, DS, and SA performed the statistical analysis, AB, MG, and GA contributed to the discussion, FP and MR revised critically the manuscript. AB, MG, GA, DS, and SA wrote the manuscript. All authors contributed to the article and approved the submitted version.
Funding
This study was supported by an unconditional grant from PharmExtracta SpA. The funder was not involved in the study design, collection, analysis, interpretation of data, the writing of this article, or the decision to submit it for publication.
Conflict of interest
The authors declare that the research was conducted in the absence of any commercial or financial relationships that could be construed as a potential conflict of interest.
The handling editor FDP declared a past collaboration with the author AB.
Publisher’s note
All claims expressed in this article are solely those of the authors and do not necessarily represent those of their affiliated organizations, or those of the publisher, the editors and the reviewers. Any product that may be evaluated in this article, or claim that may be made by its manufacturer, is not guaranteed or endorsed by the publisher.
References
1. Antrobus M, Cuttell S, Machado LR. Chapter 8 - epigenetics, exercise, and the immune system. In: Raleigh SM, editor. Epigenetics of exercise and sports, vol. 25. (Cambridge, MA, USA: Academic Press) (2021). p. 183–99.
2. Khoramipour K, Basereh A, Hekmatikar AA, Castell L, Ruhee RT, Suzuki K. Physical activity and nutrition guidelines to help with the fight against covid-19. J Sports Sci (2021) 39(1):101–7. doi: 10.1080/02640414.2020.1807089
3. Pedersen BK, Hoffman-Goetz L. Exercise and the immune system: Regulation, integration, and adaptation. Physiol Rev (2000) 80(3):1055–81. doi: 10.1152/physrev.2000.80.3.1055
4. Brodin P, Davis MM. Human immune system variation. Nat Rev Immunol (2017) 17(1):21–9. doi: 10.1038/nri.2016.125
5. Brolinson PG, Elliott D. Exercise and the immune system. Clin Sports Med (2007) 26(3):311–9. doi: 10.1016/j.csm.2007.04.011
6. Simpson RJ, Campbell JP, Gleeson M, Kruger K, Nieman DC, Pyne DB, et al. Can exercise affect immune function to increase susceptibility to infection? Exerc Immunol Rev (2020) 26:8–22.
7. Laursen PB, Jenkins DG. The scientific basis for high-intensity interval training: Optimising training programmes and maximising performance in highly trained endurance athletes. Sports Med (2002) 32(1):53–73. doi: 10.2165/00007256-200232010-00003
8. Souza D, Vale AF, Silva A, Araujo MAS, de Paula Junior CA, de Lira CAB, et al. Acute and chronic effects of interval training on the immune system: A systematic review with meta-analysis. Biol (Basel) (2021) 10(9):868. doi: 10.3390/biology10090868
9. Miko BA, Pereira MR, Safdar A. Respiratory tract infections: Sinusitis, bronchitis, and pneumonia. In: Safdar A, editor. Principles and practice of transplant infectious diseases. (New York: Springer New York) (2019). p. 339–49.
10. Cicchella A, Stefanelli C, Massaro M. Upper respiratory tract infections in sport and the immune system response. a review. Biol (Basel) (2021) 10(5):362. doi: 10.3390/biology10050362
11. Nieman DC. Risk of upper respiratory tract infection in athletes: An epidemiologic and immunologic perspective. J Athl Train (1997) 32(4):344–9.
12. Akerstrom TC, Pedersen BK. Strategies to enhance immune function for marathon runners : What can be done? Sports Med (2007) 37(4-5):416–9. doi: 10.2165/00007256-200737040-00037
13. Ronsen O. Prevention and management of respiratory tract infections in athletes. New Stud Athletics (2005) 20(3):49.
14. Campbell JP, Turner JE. Debunking the myth of exercise-induced immune suppression: Redefining the impact of exercise on immunological health across the lifespan. Front Immunol (2018) 9:648. doi: 10.3389/fimmu.2018.00648
15. Agha-Alinejad H, Ahmadi Hekmatikar AH, Ruhee RT, Shamsi MM, Rahmati M, Khoramipour K, et al. A guide to different intensities of exercise, vaccination, and sports nutrition in the course of preparing elite athletes for the management of upper respiratory infections during the covid-19 pandemic: A narrative review. Int J Environ Res Public Health (2022) 19(3):1888. doi: 10.3390/ijerph19031888
16. Pedersen BK, Bruunsgaard H. How physical exercise influences the establishment of infections. Sports Med (1995) 19(6):393–400. doi: 10.2165/00007256-199519060-00003
17. Babina K, Salikhova D, Polyakova M, Svitich O, Samoylikov R, Ahmad El-Abed S, et al. The effect of oral probiotics (Streptococcus salivarius K12) on the salivary level of secretory immunoglobulin a, salivation rate, and oral biofilm: A pilot randomized clinical trial. Nutrients (2022) 14(5):1124. doi: 10.3390/nu14051124
18. Di Pierro F, Adami T, Rapacioli G, Giardini N, Streitberger C. Clinical evaluation of the oral probiotic streptococcus salivarius K12 in the prevention of recurrent pharyngitis and/or tonsillitis caused by streptococcus pyogenes in adults. Expert Opin Biol Ther (2013) 13(3):339–43. doi: 10.1517/14712598.2013.758711
19. Veiga P, Suez J, Derrien M, Elinav E. Moving from probiotics to precision probiotics. Nat Microbiol (2020) 5(7):878–80. doi: 10.1038/s41564-020-0721-1
20. Tagg JR. Prevention of streptococcal pharyngitis by anti-streptococcus pyogenes bacteriocin-like inhibitory substances (Blis) produced by streptococcus salivarius. Indian J Med Res (2004) 119 Suppl:13–6.
21. Jack RW, Tagg JR, Ray B. Bacteriocins of gram-positive bacteria. Microbiol Rev (1995) 59(2):171–200. doi: 10.1128/mr.59.2.171-200.1995
23. Burton JP, Chilcott CN, Moore CJ, Speiser G, Tagg JR. A preliminary study of the effect of probiotic streptococcus salivarius K12 on oral malodour parameters. J Appl Microbiol (2006) 100(4):754–64. doi: 10.1111/j.1365-2672.2006.02837.x
24. Burton JP, Chilcott CN, Tagg JR. The rationale and potential for the reduction of oral malodour using streptococcus salivarius probiotics. Oral Dis (2005) 11 Suppl 1:29–31. doi: 10.1111/j.1601-0825.2005.01084.x
25. Di Pierro F, Colombo M, Giuliani MG, Danza ML, Basile I, Bollani T, et al. Effect of administration of streptococcus salivarius K12 on the occurrence of streptococcal pharyngo-tonsillitis, scarlet fever and acute otitis media in 3 years old children. Eur Rev Med Pharmacol Sci (2016) 20(21):4601–6.
26. Hyink O, Wescombe PA, Upton M, Ragland N, Burton JP, Tagg JR. Salivaricin A2 and the novel lantibiotic salivaricin b are encoded at adjacent loci on a 190-kilobase transmissible megaplasmid in the oral probiotic strain streptococcus salivarius K12. Appl Environ Microbiol (2007) 73(4):1107–13. doi: 10.1128/AEM.02265-06
27. Horz HP, Meinelt A, Houben B, Conrads G. Distribution and persistence of probiotic streptococcus salivarius K12 in the human oral cavity as determined by real-time quantitative polymerase chain reaction. Oral Microbiol Immunol (2007) 22(2):126–30. doi: 10.1111/j.1399-302X.2007.00334.x
28. Power DA, Burton JP, Chilcott CN, Dawes PJ, Tagg JR. Preliminary investigations of the colonisation of upper respiratory tract tissues of infants using a paediatric formulation of the oral probiotic streptococcus salivarius K12. Eur J Clin Microbiol Infect Dis (2008) 27(12):1261–3. doi: 10.1007/s10096-008-0569-4
29. Zhang Z, Yuan K-H. Practical statistical power analysis using webpower and r. Granger IN: ISDSA Press Notre Dame, IN, USA (2018).
30. Barrett B, Brown R, Mundt M, Safdar N, Dye L, Maberry R, et al. The Wisconsin upper respiratory symptom survey is responsive, reliable, and valid. J Clin Epidemiol (2005) 58(6):609–17. doi: 10.1016/j.jclinepi.2004.11.019
31. Patras KA, Wescombe PA, Rosler B, Hale JD, Tagg JR, Doran KS. Streptococcus salivarius K12 limits group b streptococcus vaginal colonization. Infect Immun (2015) 83(9):3438–44. doi: 10.1128/IAI.00409-15
32. Guglielmetti S, Taverniti V, Minuzzo M, Arioli S, Stuknyte M, Karp M, et al. Oral bacteria as potential probiotics for the pharyngeal mucosa. Appl Environ Microbiol (2010) 76(12):3948–58. doi: 10.1128/AEM.00109-10
33. Manning J, Dunne EM, Wescombe PA, Hale JD, Mulholland EK, Tagg JR, et al. Investigation of streptococcus salivarius-mediated inhibition of pneumococcal adherence to pharyngeal epithelial cells. BMC Microbiol (2016) 16(1):225. doi: 10.1186/s12866-016-0843-z
34. Coad S, McLellan C, Whitehouse T, Gray B. Validity and reliability of a novel salivary immunoassay for individual profiling in applied sports science. Res Sports Med (2015) 23(2):140–50. doi: 10.1080/15438627.2015.1005300
35. Pallares JG, Moran-Navarro R, Ortega JF, Fernandez-Elias VE, Mora-Rodriguez R. Validity and reliability of ventilatory and blood lactate thresholds in well-trained cyclists. PloS One (2016) 11(9):e0163389. doi: 10.1371/journal.pone.0163389
36. Foster C, Florhaug JA, Franklin J, Gottschall L, Hrovatin LA, Parker S, et al. A new approach to monitoring exercise training. J Strength Cond Res (2001) 15(1):109–15. doi: 10.1519/00124278-200102000-00019
37. Liu F, Liang T, Zhang Z, Liu L, Li J, Dong W, et al. Effects of altitude on human oral microbes. AMB Express (2021) 11(1):41. doi: 10.1186/s13568-021-01200-0
38. Li X, Liu Y, Yang X, Li C, Song Z. The oral microbiota: Community composition, influencing factors, pathogenesis, and interventions. Front Microbiol (2022) 13:895537. doi: 10.3389/fmicb.2022.895537
39. Di Pierro F, Colombo M, Zanvit A, Risso P, Rottoli AS. Use of streptococcus salivarius K12 in the prevention of streptococcal and viral pharyngotonsillitis in children. Drug Healthc Patient Saf (2014) 6:15–20. doi: 10.2147/DHPS.S59665
40. Di Pierro F, Colombo M, Zanvit A, Rottoli AS. Positive clinical outcomes derived from using streptococcus salivarius K12 to prevent streptococcal pharyngotonsillitis in children: A pilot investigation. Drug Healthc Patient Saf (2016) 8:77–81. doi: 10.2147/DHPS.S117214
41. Di Pierro F, Donato G, Fomia F, Adami T, Careddu D, Cassandro C, et al. Preliminary pediatric clinical evaluation of the oral probiotic streptococcus salivarius K12 in preventing recurrent pharyngitis and/or tonsillitis caused by streptococcus pyogenes and recurrent acute otitis media. Int J Gen Med (2012) 5:991–7. doi: 10.2147/IJGM.S38859
42. Di Pierro F, Risso P, Poggi E, Timitilli A, Bolloli S, Bruno M, et al. Use of streptococcus salivarius K12 to reduce the incidence of pharyngo-tonsillitis and acute otitis media in children: A retrospective analysis in not-recurrent pediatric subjects. Minerva Pediatr (2018) 70(3):240–5. doi: 10.23736/S0026-4946.18.05182-4
43. Gregori G, Righi O, Risso P, Boiardi G, Demuru G, Ferzetti A, et al. Reduction of group a beta-hemolytic streptococcus pharyngo-tonsillar infections associated with use of the oral probiotic streptococcus salivarius K12: A retrospective observational study. Ther Clin Risk Manag (2016) 12:87–92. doi: 10.2147/TCRM.S96134
44. Guo H, Xiang X, Lin X, Wang Q, Qin S, Lu X, et al. Oropharyngeal probiotic ent-K12 as an effective dietary intervention for children with recurrent respiratory tract infections during cold season. Front Nutr (2022) 9:900448. doi: 10.3389/fnut.2022.900448
45. Wang Q, Lin X, Xiang X, Liu W, Fang Y, Chen H, et al. Oropharyngeal probiotic ent-K12 prevents respiratory tract infections among frontline medical staff fighting against covid-19: A pilot study. Front Bioeng Biotechnol (2021) 9:646184. doi: 10.3389/fbioe.2021.646184
46. Drummond LR, Campos HO, Drummond FR, de Oliveira GM, Fernandes J, Amorim RP, et al. Acute and chronic effects of physical exercise on iga and igg levels and susceptibility to upper respiratory tract infections: A systematic review and meta-analysis. Pflugers Arch (2022) 474(12):1221–48. doi: 10.1007/s00424-022-02760-1
47. Derman W, Badenhorst M, Eken M, Gomez-Ezeiza J, Fitzpatrick J, Gleeson M, et al. Risk factors associated with acute respiratory illnesses in athletes: A systematic review by a subgroup of the ioc consensus on 'Acute respiratory illness in the athlete'. Br J Sports Med (2022) 56(11):639–50. doi: 10.1136/bjsports-2021-104795
48. Fahlman MM, Engels HJ. Mucosal iga and urti in American college football players: A year longitudinal study. Med Sci Sports Exerc (2005) 37(3):374–80. doi: 10.1249/01.mss.0000155432.67020.88
49. Gleeson M, McDonald WA, Pyne DB, Clancy RL, Cripps AW, Francis JL, et al. Immune status and respiratory illness for elite swimmers during a 12-week training cycle. Int J Sports Med (2000) 21(4):302–7. doi: 10.1055/s-2000-313
50. Neville V, Gleeson M, Folland JP. Salivary iga as a risk factor for upper respiratory infections in elite professional athletes. Med Sci Sports Exerc (2008) 40(7):1228–36. doi: 10.1249/MSS.0b013e31816be9c3
51. Pedersen BK, Kappel M, Klokker M, Nielsen HB, Secher NH. The immune system during exposure to extreme physiologic conditions. Int J Sports Med (1994) 15 Suppl 3:S116–21. doi: 10.1055/s-2007-1021125
52. Born DP, Zinner C, Sperlich B. The mucosal immune function is not compromised during a period of high-intensity interval training. is it time to reconsider an old assumption? Front Physiol (2017) 8:485. doi: 10.3389/fphys.2017.00485
53. Sari-Sarraf V, Reilly T, Doran DA. Salivary iga response to intermittent and continuous exercise. Int J Sports Med (2006) 27(11):849–55. doi: 10.1055/s-2006-923777
54. Walsh NP, Blannin AK, Clark AM, Cook L, Robson PJ, Gleeson M. The effects of high-intensity intermittent exercise on saliva iga, total protein and alpha-amylase. J Sports Sci (1999) 17(2):129–34. doi: 10.1080/026404199366226
55. Nieman DC. Exercise, infection, and immunity. Int J Sports Med (1994) 15 Suppl 3:S131–41. doi: 10.1055/s-2007-1021128
56. Nieman DC. Special feature for the olympics: Effects of exercise on the immune system: Exercise effects on systemic immunity. Immunol Cell Biol (2000) 78(5):496–501. doi: 10.1111/j.1440-1711.2000.t01-5-.x
57. Leandro CG, Ferreira ESWT, Lima-Silva AE. Covid-19 and exercise-induced immunomodulation. Neuroimmunomodulation (2020) 27(1):75–8. doi: 10.1159/000508951
58. Ihalainen JK, Schumann M, Hakkinen K, Mero AA. Mucosal immunity and upper respiratory tract symptoms in recreational endurance runners. Appl Physiol Nutr Metab (2016) 41(1):96–102. doi: 10.1139/apnm-2015-0242
59. Dodds MW, Johnson DA, Yeh CK. Health benefits of saliva: A review. J Dent (2005) 33(3):223–33. doi: 10.1016/j.jdent.2004.10.009
60. Di Pierro F, Iqtadar S, Mumtaz SU, Bertuccioli A, Recchia M, Zerbinati N, et al. Clinical effects of streptococcus salivarius K12 in hospitalized covid-19 patients: Results of a preliminary study. Microorganisms (2022) 10(10):1926. doi: 10.3390/microorganisms10101926
61. Ninnemann J, Budzinski L, Bondareva M, Witkowski M, Angermair S, Kreye J, et al. Induction of cross-reactive antibody responses against the rbd domain of the spike protein of sars-Cov-2 by commensal microbiota. bioRxiv (2021) 2021. doi: 10.1101/2021.08.08.455272
62. Bradley KC, Finsterbusch K, Schnepf D, Crotta S, Llorian M, Davidson S, et al. Microbiota-driven tonic interferon signals in lung stromal cells protect from influenza virus infection. Cell Rep (2019) 28(1):245–56.e4. doi: 10.1016/j.celrep.2019.05.105
63. Beller A, Kruglov A, Durek P, von Goetze V, Werner K, Heinz GA, et al. Specific microbiota enhances intestinal iga levels by inducing tgf-beta in T follicular helper cells of peyer's patches in mice. Eur J Immunol (2020) 50(6):783–94. doi: 10.1002/eji.201948474
Keywords: bacteriocin, IgA, Streptococcus salivarius K12, URTI, HIT
Citation: Bertuccioli A, Gervasi M, Annibalini G, Binato B, Perroni F, Rocchi MBL, Sisti D and Amatori S (2023) Use of Streptococcus salivarius K12 in supporting the mucosal immune function of active young subjects: A randomised double-blind study. Front. Immunol. 14:1129060. doi: 10.3389/fimmu.2023.1129060
Received: 21 December 2022; Accepted: 13 February 2023;
Published: 02 March 2023.
Edited by:
Francesco Di Pierro, Velleja Research S.r.l., ItalyReviewed by:
Andrey Kruglov, German Rheumatism Research Center (DRFZ), GermanyMariarosaria Matera, Azienda USL Toscana Sud Est, Italy
Qiang Wang, Wuhan University of Science and Technology, China
Copyright © 2023 Bertuccioli, Gervasi, Annibalini, Binato, Perroni, Rocchi, Sisti and Amatori. This is an open-access article distributed under the terms of the Creative Commons Attribution License (CC BY). The use, distribution or reproduction in other forums is permitted, provided the original author(s) and the copyright owner(s) are credited and that the original publication in this journal is cited, in accordance with accepted academic practice. No use, distribution or reproduction is permitted which does not comply with these terms.
*Correspondence: Davide Sisti, davide.sisti@uniurb.it