- 1Zhejiang Academy of Science & Technology for Inspection & Quarantine, Hangzhou, Zhejiang, China
- 2The Cancer Hospital of the University of Chinese Academy of Sciences (Zhejiang Cancer Hospital), Institute of Basic Medicine and Cancer (IBMC), Chinese Academy of Sciences, Hangzhou, Zhejiang, China
Renal cell carcinoma (RCC) is associated with high mortality rates worldwide and survival among RCC patients has not improved significantly in the past few years. A better understanding of the pathogenesis of RCC can enable the development of more effective therapeutic strategies against RCC. Hyaluronan (HA) is a glycosaminoglycan located in the extracellular matrix (ECM) that has several roles in biology, medicine, and physiological processes, such as tissue homeostasis and angiogenesis. Dysregulated HA and its receptors play important roles in fundamental cellular and molecular biology processes such as cell signaling, immune modulation, tumor progression and angiogenesis. There is emerging evidence that alterations in the production of HA regulate RCC development, thereby acting as important biomarkers as well as specific therapeutic targets. Therefore, targeting HA or combining it with other therapies are promising therapeutic strategies. In this Review, we summarize the available data on the role of abnormal regulation of HA and speculate on its potential as a therapeutic target against RCC.
1. Introduction
Kidney cancer represents around 3% of all cancer diagnoses and deaths worldwide, with a higher incidence being reported in developed nations (1, 2). Renal cell carcinoma (RCC) is the most common malignant tumor accounting for 80-85% of all kidney cancers (3). The three main histological subtypes of RCC are clear cell RCC (ccRCC, 70%), papillary RCC (pRCC, 10-15%) and chromophobe RCC (chRCC, 3-5%) (4, 5). Age, gender, race, geographic location (1, 6), obesity (7, 8), smoking (9, 10), and hypertension (11, 12) are associated with development of RCC, while lifestyle and dietary modifications may reduce risk of developing RCC (13). RCC is associated with high mortality rates because its poor sensitivity to therapies, and high recurrence risk after nephrectomy, providing a 60-70% 5−year survival rate (14). Metastasis is present in approximately 30% of RCC cases at initial diagnosis, which lead to poor clinical outcomes (15). Existing targeted immunotherapies and other therapeutic strategies against RCCs have limited efficacy, which has prompted interest in the development of alternative strategies (16).
Hyaluronan (HA) is a ubiquitous polyanionic glycosaminoglycan (GAG) found in the extracellular matrix (ECM) that also forms a pericellular coat surrounding cells. HA plays important roles in a variety of physiological functions, including cell motility and inflammation (17). Research has been conducted on the specific roles of HA in diseases such as cancer, rheumatoid arthritis and infectious diseases (18). Signal transduction and functions of HA depend on its molecular size. High molecular weight HA (HMW-HA; >500 kDa) promotes anti-inflammatory effects in most cases, whereas low molecular weight HA (LMW-HA; <120 kDa) acts as a pro-inflammatory “danger” signal that triggers local inflammation (19).
High levels of HA are associated with unfavorable prognosis in multiple cancers (20, 21). HA has recently emerged as a key player in nephrology and urology that plays a role in inflammation and ECM organization (22). However, there is no clear consensus on the importance of HA in RCC. Emerging evidence suggests that HA accumulation abnormally in RCC may contribute to aggressive malignancies and metastatic carcinomas, and may serve as an essential therapeutic target (23). Herein, we highlight the characteristics of HA and its main receptors in RCC, with specific focus on its abnormal regulation and potential as a therapeutic target.
2. HA biology and kidney
HA was independently identified by Meyer and Palmer in 1934, and was previously named from hyaloid and uronic acid (24). HA is a GAG synthesized by a wide range of living organisms. It consists of repeating disaccharide units of glucuronic acid (GlcA) and N-acetylglucosamine (GlcNAc) bound together (25). HA is well known for its water absorption abilities and its capacity to generate higher concentrations of gels (26). Eukaryotic cells use HA synthases (HAS1-3) to synthesize HA on their plasma membranes (Table 1, Figure 1). Among them, HAS1 is the least active enzyme, and requires a high concentration of UDP-GlcNAc to function (39), while HAS3 is the most active synthase. HAS1 and HAS2 synthesize HMW-HA, while HAS3 synthesizes LMW-HA (40). HAS2 is the primary HA synthase during development (41). In mammals, expression of HASs varies between normal and pathologic conditions based on tissue and cell types.
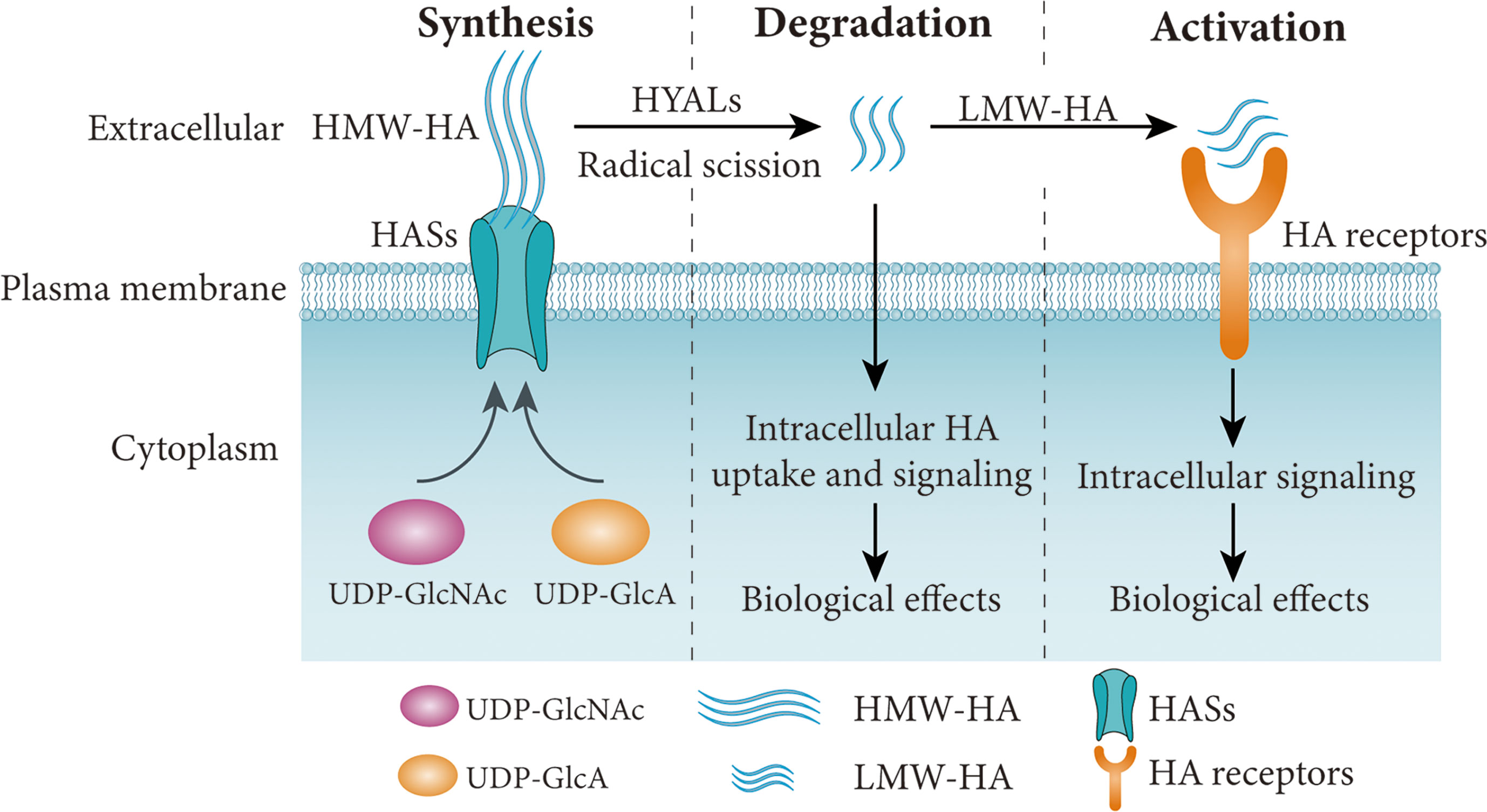
Figure 1 Simplified schematic diagram of HA synthesis, degradation and signaling pathways. HA is synthesized by HASs using UDP-GlcA and UDP-GlcNAc as substrates, and degraded into LMW-HA by HYALs or free radicals. The HA receptors are activated by LMW-HA and involved in various cellular functions. HA, hyaluronan; LMW-HA, low molecular weight HA; HASs, HA synthases; HYALs, hyaluronidases. Figure adapted from Ref (37, 38).
HA undergoes rapid turnover in the ECM, with a third of the 15g mass in an average adult human undergoing turnover each day. An increase in HA levels may be associated with higher turnover, which may reflect the pathological conditions. HA undergoes turnover and catabolism after internalization by many tissues through receptor-mediated endocytosis. HMW-HA is degraded by hyaluronidases (HYALs) (32), reactive oxygen species (ROS) (42) or ultraviolet (UV) radiation (43) (Figure 1). HA is mostly excreted in the liver each day, while only 1-2% of HA is removed in the kidney (44–47). Excretion through the kidney is limited to LMW-HA (< 12 kDa) that can pass through the glomerular barrier. In mammals, the main members of the family of HYALs include HYAL1-4, PH20 and HYALP1 (Table 1). HYAL1 cleaves HA of different molecular weights (32), while HYAL2 degrades HMW-HA into approximately 20 kDa fragments (31). HYAL3 is widely expressed, while the expression of HYAL4 is mostly in the placenta and skeletal muscle. In humans, HYALP1 is expressed as a pseudogene, but its function is unclear. PH20 plays a role in fertilization and is almost exclusively expressed in the testes (33). However, PH20 is overexpressed in other malignant tissues, such as breast (48), prostate (49) and laryngeal (50) cancers. Proteins such as TMEM2 (transmembrane protein 2) and CEMIP (cell migration-inducing protein, also called HYBID or KIAA1199), are capable of depolymerizing HA (51, 52). HA degradation products such as LMW-HA and oligosaccharides (<10 kDa) activate signaling cascades that promoting inflammation and angiogenesis and are generally associated with pathological states (Figure 1), such as cancer (19).
HA is predominantly produced in the interstitium of the renal papilla (medulla) in normal kidney, while its production in the renal cortex is very low accounting for 1-3% of the production in the medulla (53–57). The gene expression levels are HAS2 > HAS1 > HAS3 (58, 59). It is important to note that RCC mostly originates from the renal cortex. Extracellular HA is degraded by HYAL2 in all kidney regions, whereas intracellular HA is degraded by HYAL1 (60). Normal kidneys have low production of HA, with increased production of HA in the renal interstitium being linked to several renal diseases, such as acute kidney injury (61), chronic kidney diseases (62), allograft rejection (63), diabetic nephropathy (64), obstructive uropathy (65), IgA nephropathy (66), and kidney stones (67). It has been proposed that altered production of HA in papillary interstitial tissues regulates renal water handling through its effects on the matrix’s physiochemical properties and interstitial hydrostatic pressure (55, 68, 69). Ito et al. (70) demonstrated that CD44 in renal proximal tubular epithelial cells (PTCs) modulates HA-mediated regulation of cell function through TGF-β mediated mechanisms. Van den Berg et al. (71) found that glomerular endothelial HA contributes to glomerular structure and function, but whose production is lost in diabetic nephropathy. In addition, immune mediators may induce cortical fibroblasts to produce more HA (54, 72), suggesting that inflammation causes accumulation of HA in the cortex. There is need for further studies to identify the exact nature of the cells responsible for HA synthesis or the factors that contribute to its increased production in kidney diseases like RCC.
3. TME, ECM and immunity
Cancer is a complex systemic disease. The tumor microenvironment (TME) is composed of tumor cells and adjacent noncancer components, such as immune cells, fibroblasts, ECM and many others (73). Constant interactions between tumor cells and other components constitute a highly complex, dynamic and heterogenous network of the TME that supports tumor growth and invasion (74). RCC is associated with high infiltration of several immune cells, making it one of the most immunoreactive tumors (75–78). It also consists of various myofibroblasts and endothelial cells (79). Targeting cancer cells in the TME has become an appealing strategy for treating RCC (80, 81). Better understanding of the RCC may lead to the identification of specific therapeutic targets in the microenvironment, which can be used to improve the prognosis of patients.
The ECM is highly dysregulated in cancer, and may play pro-tumorigenic or anti-tumorigenic roles. During cancer progression, ECM recognizes various cell surface receptors and initiates signaling pathways that promote tumor growth (82). Unlike the ECM in healthy kidneys, RCC ECM represent is composed of a complex network of components such as GAGs, collagen, fibronectin, tenascin C, and laminins (83, 84). GAGs are regulated by altered metabolic pathways in RCC, which are associated with tumor aggressiveness and recurrence (85–87). HA is a widely produced GAG of the ECM that can have tumor promoting or tumor suppressing roles. Meanwhile, HA is mostly produced in tumor cells as well as cancer-associated fibroblasts (CAFs) in the TME (88), with the level of production varying according to the stage of the tumor. Many pro-tumorigenic effects are attributed to HA fragments (89). Under steady-state conditions, HMW-HA (>500 kDa) is the dominant HA size in most tissues and inhibits tumor progression, while LMW-HA (<120 kDa) may regulate tumor growth, invasion and metastasis through HA receptors in TME, such as CD44 and RHAMM (19, 88). Size-specific HA signaling may be related to unique conformational changes in the external receptor•HA complexes (90).
In some tumors, tumoral HA and its degradation products induce tumor angiogenesis and activate both innate and adaptive immune responses (91–93), but this association has not been studied in RCC. Tumoral HA is known to recruit tumor-associated macrophages to promote tumor neovascularization (94). LMW-HA can induce dendritic cells (DCs) activation and maturation, release proangiogenic cytokines and modulate proangiogenic properties in TME (93). Furthermore, HA has frequently been implicated in T cell trafficking and induction of cell death in activated T cells through CD44 (95, 96). Regulatory T cells (Tregs) are potent immunosuppressive cells that promote tumor angiogenesis (93) with HA binding populations being functionally more suppressive (91). In addition, interaction between natural killer (NK) cell receptor and HA on tumor cells possible to augment NK cell cytotoxicity (97). Thus, it is not surprising that HA plays significant roles in the regulation of tumor immunosuppression.
4. HA in RCC
Increased production of HA in tumor parenchyma, TME or serum is associated with tumor growth and poor outcome in cancer patients, including RCC (23), breast cancer (98), head and neck squamous cell carcinoma (99), lymphomas (100), gliomas (101), melanomas (102), lung carcinomas (103), hepatocellular carcinoma (104), and other cancers. Kaul et al. (22) reported several kidney diseases, including RCC, which are associated with changes in production of HA. Jokelainen et al. (23) revealed that 39.6% of RCC samples were HA positive. Furthermore, high cellular HA was associated with higher tumor grades and was a marker of poor prognosis in RCC patients. Thus, tumoral HA may play a role in the progression of the cancer and may act as a prognostic factor for RCC.
Zoltan-Jones et al. (105) reported that β-catenin regulated HA production in Madin-Darby canine kidney (MDCK) cells and could lead to epithelial-mesenchymal transition (EMT). Rilla et al. (106) found that induction of HAS3 expression in MDCK cells may be related to premalignant phenotypes. Moran et al. (84) reported that HAS1 regulated the migration of renal carcinoma in vitro and found no distant metastasis in mice after implanting HAS1-deficent cells. Recent evidence demonstrates that microRNA-125a may play a role in the progression of RCC through interaction with HAS1 (107), suggesting that the tumor promoting properties of HA can be explained by another mechanism.
Chi et al. (108) used Q-PCR to compare gene expression between tumor tissues and adjacent normal tissue and found that HAS1 levels were increased in ccRCC, pRCC and chRCC tissues. The expression of HYAL4 in ccRCC and pRCC was higher than in oncocytomas, while the expression of HYAL1 was lower in ccRCC than in normal kidney. There was no difference in expression between normal and tumor tissues among other members of the HA family including HAS2, HAS3, HYAL2, HYAL3, PH20, HYALP1 and CD44v. Cai et al. (109) found that the expression of HAS1-3 mRNA in human ccRCC was higher than that in adjacent normal renal samples. However, only the HAS3 protein expression was higher in ccRCC tissues at the protein level. Immunohistochemical staining showed that weak HA staining in human ccRCC tissues compared with normal adjacent samples. Similarly, Ucakturk et al. (87) used UPLC-MS analyses to show that no difference was found in HA production between RCC and normal renal samples. It is putative that HASs transcription or protein expression levels in human RCC might not reflect HA levels. Taken together, reports on the expression patterns of the HA family members in RCC are inconsistent and may be due to different experimental conditions. Also, additional mechanisms could be involved. Thus, the exact role of HA in RCC is uncertain, and further studies that are more sensitive and specific are required.
Kusmartsev et al. (110) observed an increase in HYAL2+PD-L1+ myeloid-derived suppressor cells (MDSCs) in ccRCC tumor tissue and peripheral blood. Furthermore, stroma-associated PD-L1+ myeloid cells showed significant production of HA. HYAL2+ myeloid cells indicate the occurrence of HMW-HA degradation into LMW-HA, suggesting that the relationship between myeloid cells and HA may be involved in the promotion of cancer-related inflammation and immune functions. Similarly, Dominguez-Gutierrez et al. (111) found that LMW-HA was accumulated by HYAL2+ tumor associated myeloid cells in human bladder cancer and associated with elevated production of tumor angiogenic factors. Unfortunately, it is still unclear how HA-immune interactions occur in RCC.
5. HA receptors in RCC
HA also interacts with specific proteins (Table 2) called hyaladherins (129) such as TSG-6 (130), and various cell receptors, including CD44 (131), receptor of HA-mediated motility (RHAMM) (113), layilin (132), lymphatic vessel endothelial receptor 1 (LYVE1) (118), intracellular adhesion molecule 1 (ICAM1) (117), toll like receptors (TLRs) (114), and hyaluronic acid receptor for endocytosis (HARE or Stabilin-2) (120). The receptors are activated by LMW-HA and are involved in various cellular functions including tumor metastasis and lymphocyte activation. For example, LMW-HA regulates breast cancer progression through CD44 and TLRs signaling (133). Only a few studies have investigated the cellular mechanisms underlying the role of HA receptors in RCC pathogenesis.
CD44 proteins are primary HA receptors that promote invasion and metastasis of cancer cells by modulating intracellular signaling through its interaction with RHAMM (131, 134). RHAMM regulates cell proliferation and transformation and is overexpressed in most cancers (113), the expression of RHAMM is an independent prognostic factor for RCC (135). Chi et al. (108) found that RHAMM was significantly higher in ccRCC, chRCC and pRCC than in normal kidneys. Expression of CD44s and RHAMM was also higher in ccRCC and pRCC than in oncocytomas. These findings indicate that RHAMM and CD44s expression levels in RCC tissues are potential predictors of metastasis. Furthermore, HA and proteoglycan link protein 3 (HAPLN3) is overexpressed and may promote tumor progression in ccRCC through immune cells infiltration (136).
Layilin is a HA receptor homologous to C-type lectin that has been reported to regulate cell adhesion and migration through binding to cytoskeletal proteins such as merlin and talin (137). The prognostic value of layilin in hepatocellular carcinoma was reported by Zheng et al. (121), who concluded that layilin is an unfavorable risk factor since it suppresses the functions of the CD8+ T cells in TME. In contrast, Mahuron et al. (138) reported that layilin enhanced the cytotoxic potential of melanoma. Research on mice has shown that layilin is expressed in various organs, including kidney or normal rat kidney cell line (137). Adachi et al. reported that layilin silencing prevented EMT in human ccRCC in vitro (139). These results implied that the exact function of layilin remains unclear.
In the renal papillae, HARE is localized to the endothelial cells that internalize circulating HA (140). Tissues with the highest expression of HARE are the most common targets of metastatic cancer (120). LYVE-1 is another HA-binding receptor that is found in the lymphatic vascular endothelial cells and renal tubular epithelium cells (141, 142). LYVE-1 has been used to map lymphatic vessels within and around tumor tissues to determine patient survival (143). Unfortunately, there are no reports on the roles of LYVE-1 and other hyaladherins in RCC.
6. Potential therapeutic applications of targeting HA in RCC
HA deposition persists in the TME contributes to pathophysiology through induction of high tumor interstitial pressure (tIP) and compression of tumor vessels, which results in tumor hypoxia (144, 145). The ability of HA to cover specific epitopes with enriched pericellular matrix suggests that HA could act as an immune regulator in human diseases, allowing affected cells to evade cellular immune attack (20, 146, 147). For instance, McBride et al. (148) reported that the HA pericellular matrix inhibits the formation of synapses by immune cells and killing malignant cells in vitro. In addition to formation of pericellular coats in vitro, HA can also form cables that may facilitate communication between cells (149). Breaching the HA barrier from the tumor leads to vascular permeability and improved drug delivery, monoclonal antibody (mAb), cytotoxic chemotherapy or immune cell therapeutic efficacy (Figure 2).
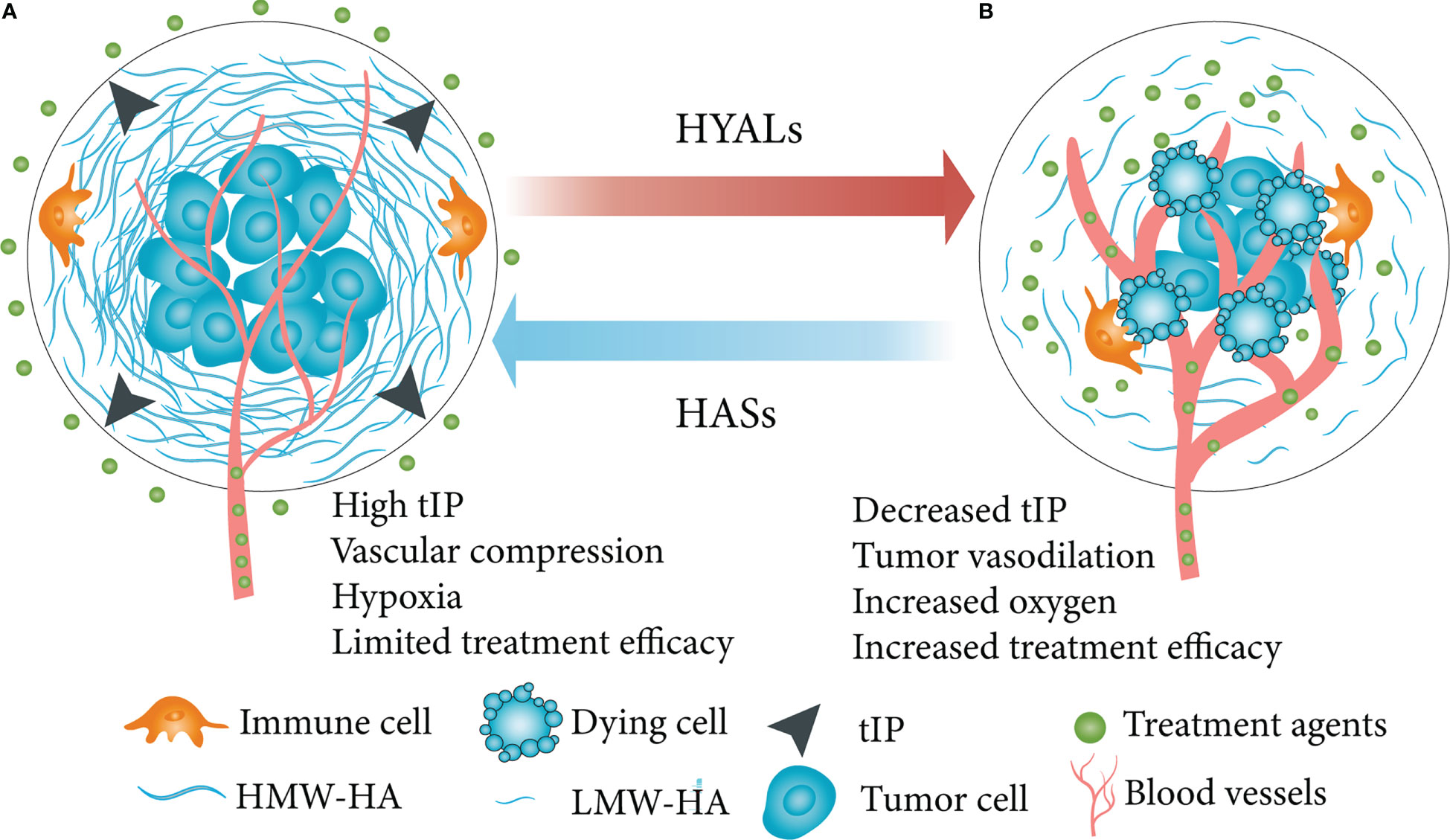
Figure 2 Simplified schematic diagram of HA depletion in a HA-enriched tumor phenotype. (A) HA swelled the TME, increased tIP, compressed tumor vasculature, caused tumor hypoxia and other effects, then limited the effectiveness of therapeutic molecules. (B) HA depletion in tumor led to vascular permeability, improved drug penetration, inhibited tumor growth and improved therapeutic efficacy. HA, hyaluronan; TME, tumor microenvironment; tIP, tumor interstitial pressure; HYALs, hyaluronidases. Figure adapted from Ref (145).
Research on HA signaling suggests that targeting HA and other members of the HA family could be used to treat cancer (150). For instance, inhibition of HAS1 induced apoptosis in bladder cancer in vitro, thus inhibiting tumor growth and angiogenesis (151). 4-methylumbelliferone (4-MU) is the best characterized chemical inhibitor of HA that inhibits HA synthesis by downregulating HAS2 and HAS3. It has been reported that 4-MU has potent anticancer effects in various tumors, including pancreatic cancer (152), breast cancer (153), esophageal cancer (154), skin cancer (155), bone cancer (156), leukemia (157), ovarian cancer (158), prostate cancer (159) and liver cancer (160). Additionally, HAS2 and HAS3 knockdown mimic the effects of 4-MU in esophageal squamous carcinoma cells (161).
Chemical compounds, such as sulfated HA (sHA), that have the ability to target HA degradation have been shown to inhibit the growth of prostate cancer cells and induce apoptosis (162). Similarly, sHA inhibits proliferation, motility, and invasion of breast cancer models (163). The depletion of HA in TME using HYALs is also being investigated as a potential cancer therapeutic strategy. PEGPH20 is human recombinant HYAL that depletes stromal HA in several animal models, and may induce reduction in tIP, increased penetration of tumors by drug as well as immune cells and inhibit the growth of tumor cells (164, 165). A variety of clinical trials are being conducted for various cancers using a combination of HYALs, chemo or radiotherapy (clinicaltrials.gov). However, whether depletion of HA could be applied to treating RCC is still unknown.
Since CD44 is a key receptor for HA, it has been targeted in different therapeutic strategies against cancer, such as vaccines, anti-CD44 antibodies, and nanoparticles that deliver CD44 siRNA (166). However, several phase I trials investigating CD44-targeted therapies showed limited clinical success in treating cancer, and the occurrence of severe side effects led to the termination of the project (167). Hence, targeting CD44 as a cancer therapeutic target requires careful evaluation. Hirose et al. (168) suggested that inhibition of HARE could be a potential strategy for preventing metastasis of melanoma to the lung in mice. Studies by Gahan et al. and Benitez et al. showed that combination of 4-MU and sorafenib inhibits the growth and motility of RCC cells by targeting RHAMM expression (169, 170), offering a potential pathway for therapeutic intervention in RCC. In combination with 4-MU, sorafenib also targets HAS3 and inhibits the growth of microvessels in RCC (171). Additionally, HA is an attractive candidate for conjugation to antitumor drugs or for use in nanoparticles (172–174). Chemotherapy drugs can be effectively delivered through HA nanomaterials. This may possibly increase the efficacy of chemotherapeutics or other therapies in tumors.
7. Conclusions and future perspectives
HA signaling pathway (HASs, HYALs, and HA receptors) is important in promoting tumor growth, metastasis, angiogenesis, and immune response. Therefore, potential therapeutic methods that can be developed include suppression of HA synthesis, clearance of the existing HA, and conjunction of HYALs and HA receptors with chemotherapy. Further studies are needed to identify the molecular mechanisms underlying the relationship between HA production and the development of cancers like RCC. There is also need to comprehensively profile the genes, proteins and metabolites involved in HA metabolism in RCC, since the whole signaling cascade is crucial to maintaining pro-cancer conditions. If these emerging strategies are clinically effective against RCC, then they could be used as adjuvant therapy in early disease to provide RCC patients with new options for the future treatment.
Author contributions
YZ led and wrote the first manuscript. CJ contributed to writing and editing of final manuscript. All authors contributed to the article and approved the submitted version.
Acknowledgments
We thank Dr. QH, JJ, WH in the Department of Nephrology, Zhejiang Provincial Hospital of Traditional Chinese Medicine for their thoughtful suggestions.
Conflict of interest
The authors declare that the research was conducted in the absence of any commercial or financial relationships that could be construed as a potential conflict of interest.
Publisher’s note
All claims expressed in this article are solely those of the authors and do not necessarily represent those of their affiliated organizations, or those of the publisher, the editors and the reviewers. Any product that may be evaluated in this article, or claim that may be made by its manufacturer, is not guaranteed or endorsed by the publisher.
References
1. Sung H, Ferlay J, Siegel RL, Laversanne M, Soerjomataram I, Jemal A, et al. Global cancer statistics 2020: GLOBOCAN estimates of incidence and mortality worldwide for 36 cancers in 185 countries. CA: A Cancer J Clin (2021) 71:209–49. doi: 10.3322/caac.21660
2. Hsieh JJ, Purdue MP, Signoretti S, Swanton C, Albiges L, Schmidinger M, et al. Renal cell carcinoma. Nat Rev Dis Primers (2017) 3:17009. doi: 10.1038/nrdp.2017.9
3. Petejova N, Martinek A. Renal cell carcinoma: Review of etiology, pathophysiology and risk factors. BioMed Pap Med Fac Univ Palacky Olomouc Czech Repub (2016) 160:183–94. doi: 10.5507/bp.2015.050
4. Ricketts CJ, De Cubas AA, Fan H, Smith CC, Lang M, Reznik E, et al. The cancer genome atlas comprehensive molecular characterization of renal cell carcinoma. Cell Rep (2018) 23:313–26. doi: 10.1016/j.celrep.2018.03.075
5. Linehan WM, Ricketts CJ. The cancer genome atlas of renal cell carcinoma: findings and clinical implications. Nat Rev Urol (2019) 16:539–52. doi: 10.1038/s41585-019-0211-5
6. Brian I Rini SCCB. Renal cell carcinoma. Lancet (2009) 373:1119–32. doi: 10.1016/S0140-6736(09)60229-4
7. Bjorge T, Tretli S, Engeland A. Relation of height and body mass index to renal cell carcinoma in two million norwegian men and women. Am J Epidemiol (2004) 160:1168–76. doi: 10.1093/aje/kwh345
8. van Dijk BAC, Schouten LJ, Kiemeney LALM, Goldbohm RA, van den Brandt PA. Relation of height, body mass, energy intake, and physical activity to risk of renal cell carcinoma: results from the Netherlands cohort study. Am J Epidemiol (2004) 160:1159–67. doi: 10.1093/aje/kwh344
9. Hunt JD, van der Hel OL, McMillan GP, Boffetta P, Brennan P. Renal cell carcinoma in relation to cigarette smoking: Meta-analysis of 24 studies. Int J Cancer (2005) 114:101–08. doi: 10.1002/ijc.20618
10. Yuan JM, Castelao JE, Gago-Dominguez M, Yu MC, Ross RK. Tobacco use in relation to renal cell carcinoma. Cancer Epidemiol. Biomarkers Prev (1998) 7:429–33.
11. McLaughlin JK, Chow WH, Mandel JS, Mellemgaard A, McCredie M, Lindblad P, et al. International renal-cell cancer study. VIII. Role diuretics other anti-hypertensive medications hypertension. Int J Cancer (1995) 63:216–21. doi: 10.1002/ijc.2910630212
12. Colt JS, Schwartz K, Graubard BI, Davis F, Ruterbusch J, DiGaetano R, et al. Hypertension and risk of renal cell carcinoma among white and black americans. Epidemiology (2011) 22:797–804. doi: 10.1097/EDE.0b013e3182300720
13. Gray RE, Harris GT. Renal cell carcinoma: diagnosis and management. Am Fam Physician (2019) 99:179–84.
14. Tostain J, Li G, Gentil-Perret A, Gigante M. Carbonic anhydrase 9 in clear cell renal cell carcinoma: A marker for diagnosis, prognosis and treatment. Eur J Cancer (2010) 46:3141–48. doi: 10.1016/j.ejca.2010.07.020
15. Li P, Wong YN, Armstrong K, Haas N, Subedi P, Davis-Cerone M, et al. Survival among patients with advanced renal cell carcinoma in the pretargeted versus targeted therapy eras. Cancer Med (2016) 5:169–81. doi: 10.1002/cam4.574
16. Posadas EM, Limvorasak S, Figlin RA. Targeted therapies for renal cell carcinoma. Nat Rev Nephrol (2017) 13:496–511. doi: 10.1038/nrneph.2017.82
17. Garantziotis S, Savani RC. Hyaluronan biology: A complex balancing act of structure, function, location and context. Matrix Biol (2019) 78-79:1–10. doi: 10.1016/j.matbio.2019.02.002
18. Simpson MA, de la Motte C, Sherman LS, Weigel PH. Advances in hyaluronan biology: signaling, regulation, and disease mechanisms. Int J Cell Biol (2015) 2015:690572. doi: 10.1155/2015/690572
19. Cyphert JM, Trempus CS, Garantziotis S. Size matters: molecular weight specificity of hyaluronan effects in cell biology. Int J Cell Biol (2015) 2015:1–08. doi: 10.1155/2015/563818
20. Caon I, Bartolini B, Parnigoni A, Caravà E, Moretto P, Viola M, et al. Revisiting the hallmarks of cancer: The role of hyaluronan. Semin Cancer Biol (2020) 62:9–19. doi: 10.1016/j.semcancer.2019.07.007
21. Sironen RK, Tammi M, Tammi R, Auvinen PK, Anttila M, Kosma V. Hyaluronan in human malignancies. Exp Cell Res (2011) 317:383–91. doi: 10.1016/j.yexcr.2010.11.017
22. Kaul A, Singampalli KL, Parikh UM, Yu L, Keswani SG, Wang X. Hyaluronan, a double-edged sword in kidney diseases. Pediatr Nephrol (2022) 37:735–44. doi: 10.1007/s00467-021-05113-9
23. Jokelainen O, Pasonen-Seppänen S, Tammi M, Mannermaa A, Aaltomaa S, Sironen R, et al. Cellular hyaluronan is associated with a poor prognosis in renal cell carcinoma. Urol Oncol (2020) 38:611–86. doi: 10.1016/j.urolonc.2020.03.029
24. Meyer K, Palmer JW. The polysaccharide of the vitreous humor. Urol Oncol (1934) 107:629–34. doi: 10.1016/S0021-9258(18)75338-6
25. Toole BP. Hyaluronan: from extracellular glue to pericellular cue. Nat Rev Cancer (2004) 4:528–39. doi: 10.1038/nrc1391
26. Stridh S, Palm F, Hansell P. Renal interstitial hyaluronan: functional aspects during normal and pathological conditions. Am J Physiol Regul Integr Comp Physiol (2012) 302:R1235–49. doi: 10.1152/ajpregu.00332.2011
27. Spicer AP, Seldin MF, Olsen AS, Brown N, Wells DE, Doggett NA, et al. Chromosomal localization of the human and mouse hyaluronan synthase genes. Genomics (1997) 41:493–97. doi: 10.1006/geno.1997.4696
28. Tammi MI, Day AJ, Turley EA. Hyaluronan and homeostasis: a balancing act. J Biol Chem (2002) 277:4581–84. doi: 10.1074/jbc.R100037200
29. Petrey AC, de la Motte CA. Hyaluronan, a crucial regulator of inflammation. Front Immunol (2014) 5:101. doi: 10.3389/fimmu.2014.00101
30. Volpi N, Schiller J, Stern R, Soltés L. Role, metabolism, chemical modifications and applications of hyaluronan. Curr Med Chem (2009) 16:1718. doi: 10.2174/092986709788186138
31. Lepperdinger G, Strobl B, Kreil G. HYAL2, a human gene expressed in many cells, encodes a lysosomal hyaluronidase with a novel type of specificity. J Biol Chem (1998) 273:22466–70. doi: 10.1074/jbc.273.35.22466
32. Csoka AB, Frost GI, Stern R. The six hyaluronidase-like genes in the human and mouse genomes. Matrix Biol (2001) 20:499–508. doi: 10.1016/S0945-053X(01)00172-X
33. Cherr GN, Meyers SA, Yudin AI, VandeVoort CA, Myles DG, Primakoff P, et al. The PH-20 protein in cynomolgus macaque spermatozoa: identification of two different forms exhibiting hyaluronidase activity. Dev Biol (1996) 175:142–53. doi: 10.1006/dbio.1996.0102
34. Yamaguchi Y, Yamamoto H, Tobisawa Y, Irie F. TMEM2: A missing link in hyaluronan catabolism identified? Matrix Biol (2019) 78-79:139–46. doi: 10.1016/j.matbio.2018.03.020
35. Spataro S, Guerra C, Cavalli A, Sgrignani J, Sleeman J, Poulain L, et al. CEMIP (HYBID, KIAA1199): structure, function and expression in health and disease. FEBS J (2022) 1-17. doi: 10.1111/febs.16600
36. Domanegg K, Sleeman JP, Schmaus A. CEMIP, a promising biomarker that promotes the progression and metastasis of colorectal and other types of cancer. Cancers (Basel) (2022) 14:5093. doi: 10.3390/cancers14205093
37. Kotla NG, Bonam SR, Rasala S, Wankar J, Bohara RA, Bayry J, et al. Recent advances and prospects of hyaluronan as a multifunctional therapeutic system. J Control Release (2021) 336:598–620. doi: 10.1016/j.jconrel.2021.07.002
38. Chanmee T, Ontong P, Itano N. Hyaluronan: A modulator of the tumor microenvironment. Cancer Lett (2016) 375:20–30. doi: 10.1016/j.canlet.2016.02.031
39. Rilla K, Oikari S, Jokela TA, Hyttinen JMT, Kärnä R, Tammi RH, et al. Hyaluronan synthase 1 (HAS1) requires higher cellular UDP-GlcNAc concentration than HAS2 and HAS3*. J Biol Chem (2013) 288:5973–83. doi: 10.1074/jbc.M112.443879
40. Itano N, Sawai T, Yoshida M, Lenas P, Yamada Y, Imagawa M, et al. Three isoforms of mammalian hyaluronan synthases have distinct enzymatic properties. J Biol Chem (1999) 274:25085–92. doi: 10.1074/jbc.274.35.25085
41. Spicer AP, Tien JL, Joo A, Bowling RJ. Investigation of hyaluronan function in the mouse through targeted mutagenesis. Glycoconj J (2002) 19:341–45. doi: 10.1023/A:1025321105691
42. Šoltés L, Mendichi R, Kogan G, Schiller J, Stankovská M, Arnhold J. Degradative action of reactive oxygen species on hyaluronan. Biomacromolecules (2006) 7:659–68. doi: 10.1021/bm050867v
43. Schmut O, Ansari AN, Faulborn J. Degradation of hyaluronate by the concerted action of ozone and sunlight. Ophthalmic Res (1994) 26:340–43. doi: 10.1159/000267499
44. Schiller S, Dorfman A. The metabolism of mucopolysaccharides in animals: iv. the influence of insulin. J Biol Chem (1957) 227:625–32. doi: 10.1016/S0021-9258(18)70742-4
45. Fraser JR, Laurent TC, Pertoft H, Baxter E. Plasma clearance, tissue distribution and metabolism of hyaluronic acid injected intravenously in the rabbit. Biochem J (1981) 200:415–24. doi: 10.1042/bj2000415
46. Fraser JR, Appelgren L, Laurent T. Tissue uptake of circulating hyaluronic acid. Cell Tissue Res (1983) 233:285–93. doi: 10.1007/BF00238296
47. Laurent TC, Lilja K, Brunnberg L, Engström-Laurent A, Laurent UB, Lindqvist U, et al. Urinary excretion of hyaluronan in man. Scand J Clin Lab Invest (1987) 47:793. doi: 10.1080/00365518709168948
48. Beech DJ, Madan AK, Deng N. Expression of PH-20 in normal and neoplastic breast tissue. J Surg Res (2002) 103:203–07. doi: 10.1006/jsre.2002.6351
49. Madan AK, Pang Y, Wilkiemeyer MB, Yu D, Beech DJ. Increased hyaluronidase expression in more aggressive prostate adenocarcinoma. Oncol Rep (1999) 6:1431. doi: 10.3892/or.6.6.1431
50. Godin DA, Fitzpatrick PC, Scandurro AB, Belafsky PC, Woodworth BA, Amedee RG, et al. PH20: a novel tumor marker for laryngeal cancer. Arch Otolaryngol Head Neck Surg (2000) 126:402–04. doi: 10.1001/archotol.126.3.402
51. Yoshida H, Okada Y. Role of HYBID (hyaluronan binding protein involved in hyaluronan depolymerization), alias KIAA1199/CEMIP, in hyaluronan degradation in normal and photoaged skin. Int J Mol Sci (2019) 20:5804. doi: 10.3390/ijms20225804
52. Tobisawa Y, Fujita N, Yamamoto H, Ohyama C, Irie F, Yamaguchi Y. The cell surface hyaluronidase TMEM2 is essential for systemic hyaluronan catabolism and turnover. J Biol Chem (2021) 297:101281. doi: 10.1016/j.jbc.2021.101281
53. Wells AF, Larsson E, Tengblad A, Fellström B, Tufveson G, Klareskog L, et al. The localization of hyaluronan in normal and rejected human kidneys. Transplantation (1990) 50:240–43. doi: 10.1097/00007890-199008000-00014
54. Johnsson C, Tufveson G, Wahlberg J, Hällgren R. Experimentally-induced warm renal ischemia induces cortical accumulation of hyaluronan in the kidney. Kidney Int (1996) 50:1224–29. doi: 10.1038/ki.1996.431
55. Hansell P, Goransson V, Odlind C, Gerdin B, Hallgren R. Hyaluronan content in the kidney in different states of body hydration. Kidney Int (2000) 58:2061–68. doi: 10.1111/j.1523-1755.2000.00378.x
56. Göransson V, Johnsson C, Nylander O, Hansell P. Renomedullary and intestinal hyaluronan content during body water excess: a study in rats and gerbils. J Physiol (2002) 542:315–22. doi: 10.1113/jphysiol.2001.014894
57. Pedagogos E, Hewitson TD, Nicholls KM, Becker GJ. Hyaluronan and rat renal fibroblasts: in vitro studies. Nephron Clin Pract (2001) 88:347–53. doi: 10.1159/000046019
58. Rügheimer L, Olerud J, Johnsson C, Takahashi T, Shimizu K, Hansell P. Hyaluronan synthases and hyaluronidases in the kidney during changes in hydration status. Matrix Biol (2009) 28:390–95. doi: 10.1016/j.matbio.2009.07.002
59. Stridh S, Kerjaschki D, Chen Y, Rügheimer L, Åstrand ABM, Johnsson C, et al. Angiotensin converting enzyme inhibition blocks interstitial hyaluronan dissipation in the neonatal rat kidney via hyaluronan synthase 2 and hyaluronidase 1. Matrix Biol (2011) 30:62–9. doi: 10.1016/j.matbio.2010.09.006
60. Colombaro V, Jadot I, Declèves A, Voisin V, Giordano L, Habsch I, et al. Hyaluronidase 1 and hyaluronidase 2 are required for renal hyaluronan turnover. Acta Histochem (2015) 117:83–91. doi: 10.1016/j.acthis.2014.11.007
61. Decleves AE, Caron N, Voisin V, Legrand A, Bouby N, Kultti A, et al. Synthesis and fragmentation of hyaluronan in renal ischaemia. Nephrol Dial Transplant (2012) 27:3771–81. doi: 10.1093/ndt/gfs098
62. Akin D, Ozmen S, Yilmaz ME. Hyaluronic acid as a new biomarker to differentiate acute kidney injury from chronic kidney disease. Iran J Kidney Dis (2017) 11:409–13.
63. Stenvinkel P, Heimbürger O, Wang T, Lindholm B, Bergström J, Elinder C. High serum hyaluronan indicates poor survival in renal replacement therapy. Am J Kidney Dis (1999) 34:1083–88. doi: 10.1016/S0272-6386(99)70014-4
64. Jones S, Jones S, Phillips AO. Regulation of renal proximal tubular epithelial cell hyaluronan generation: implications for diabetic nephropathy. Kidney Int (2001) 59:1739–49. doi: 10.1046/j.1523-1755.2001.0590051739.x
65. Johnsson C, Hallgren R, Wahlberg J, Tufveson G. Renal accumulation and distribution of hyaluronan after ureteral obstruction. Scand J Urol Nephrol (1997) 31:327–31. doi: 10.3109/00365599709030614
66. Sano N, Kitazawa K, Sugisaki T. Localization and roles of CD44, hyaluronic acid and osteopontin in IgA nephropathy. Nephron Clin Pract (2001) 89:416–21. doi: 10.1159/000046113
67. Verkoelen CF. Crystal retention in renal stone disease: a crucial role for the glycosaminoglycan hyaluronan? J Am Soc Nephrol (2006) 17:1673–87. doi: 10.1681/ASN.2006010088
68. Göransson V, Hansell P, Moss S, Alcorn D, Johnsson C, Hällgren R, et al. Renomedullary interstitial cells in culture; the osmolality and oxygen tension influence the extracellular amounts of hyaluronan and cellular expression of CD44. Matrix Biol (2001) 20:129–36. doi: 10.1016/S0945-053X(01)00129-9
69. GINETZINSKY AG. Relationship between urinary hyaluronidase and diuresis. Nature (1961) 189:235–37. doi: 10.1038/189235b0
70. Ito T, Williams JD, Fraser D, Phillips AO. Hyaluronan attenuates transforming growth factor-β1-mediated signaling in renal proximal tubular epithelial cells. Am J Pathol (2004) 164:1979–88. doi: 10.1016/s0002-9440(10)63758-3
71. van den Berg BM, Wang G, Boels M, Avramut MC, Jansen E, Sol W, et al. Glomerular function and structural integrity depend on hyaluronan synthesis by glomerular endothelium. J Am Soc Nephrol (2019) 30:1886–97. doi: 10.1681/ASN.2019020192
72. Hällgren R, Gerdin B, Tufveson G. Hyaluronic acid accumulation and redistribution in rejecting rat kidney graft. Relationship to Transplant edema. J Exp Med (1990) 171:2063–76. doi: 10.1084/jem.171.6.2063
73. Quail DF, Joyce JA. Microenvironmental regulation of tumor progression and metastasis. Nat Med (2013) 19:1423–37. doi: 10.1038/nm.3394
74. Lai Y, Tang F, Huang Y, He C, Chen C, Zhao J, et al. The tumour microenvironment and metabolism in renal cell carcinoma targeted or immune therapy. J Cell Physiol (2021) 236:1616–27. doi: 10.1002/jcp.29969
75. Nabi S, Kessler ER, Bernard B, Flaig TW, Lam ET. Renal cell carcinoma: a review of biology and pathophysiology. F1000 Res (2018) 7:307. doi: 10.12688/f1000research.13179.1
76. Nakano O, Sato M, Naito Y, Suzuki K, Orikasa S, Aizawa M, et al. Proliferative activity of intratumoral CD8+ T-lymphocytes as a prognostic factor in human renal cell carcinoma: clinicopathologic demonstration of antitumor immunity. Cancer Res (2001) 61:5132–36.
77. Díaz-Montero CM, Rini BI, Finke JH. The immunology of renal cell carcinoma. Nat Rev Nephrol (2020) 16:721–35. doi: 10.1038/s41581-020-0316-3
78. Stewart BJ, Ferdinand JR, Clatworthy MR. Using single-cell technologies to map the human immune system - implications for nephrology. Nat Rev Nephrol (2020) 16:112–28. doi: 10.1038/s41581-019-0227-3
79. Mier JW. The tumor microenvironment in renal cell cancer. Curr Opin Oncol (2019) 31:194–99. doi: 10.1097/CCO.0000000000000512
80. Heidegger I, Pircher A, Pichler R. Targeting the tumor microenvironment in renal cell cancer biology and therapy. Front Oncol (2019) 9:490. doi: 10.3389/fonc.2019.00490
81. Vuong L, Kotecha RR, Voss MH, Hakimi AA. Tumor microenvironment dynamics in clear-cell renal cell carcinoma. Cancer Discovery (2019) 9:1349–57. doi: 10.1158/2159-8290.CD-19-0499
83. Lohi J, Leivo I, Oivula J, Lehto VP, Virtanen I. Extracellular matrix in renal cell carcinomas. Histol Histopathol (1998) 13:785–96. doi: 10.14670/HH-13.785
84. Moran H, Cancel LM, Huang P, Roberge S, Xu T, Tarbell JM, et al. Glycocalyx mechanotransduction mechanisms are involved in renal cancer metastasis. Matrix Biol Plus (2022) 13:100100. doi: 10.1016/j.mbplus.2021.100100
85. Oxburgh L. The extracellular matrix environment of clear cell renal cell carcinoma. Cancers (Basel) (2022) 14:4072. doi: 10.3390/cancers14174072
86. Gatto F, Dabestani S, Bratulic S, Limeta A, Maccari F, Galeotti F, et al. Plasma and urine free glycosaminoglycans as monitoring biomarkers in nonmetastatic renal cell carcinoma–a prospective cohort study. Eur Urol Open Sci (2022) 42:30–9. doi: 10.1016/j.euros.2022.06.003
87. Ucakturk E, Akman O, Sun X, Baydar DE, Dolgun A, Zhang F, et al. Changes in composition and sulfation patterns of glycoaminoglycans in renal cell carcinoma. Glycoconj J (2016) 33:103–12. doi: 10.1007/s10719-015-9643-1
88. Tavianatou AG, Caon I, Franchi M, Piperigkou Z, Galesso D, Karamanos NK. Hyaluronan: molecular size-dependent signaling and biological functions in inflammation and cancer. FEBS J (2019) 286:2883–908. doi: 10.1111/febs.14777
89. Price Z, Lokman N, Ricciardelli C. Differing roles of hyaluronan molecular weight on cancer cell behavior and chemotherapy resistance. Cancers (Basel) (2018) 10:482. doi: 10.3390/cancers10120482
90. Weigel PH, Baggenstoss BA. What is special about 200 kDa hyaluronan that activates hyaluronan receptor signaling? Glycobiology (2017) 27:868–77. doi: 10.1093/glycob/cwx039
91. Lee-Sayer SSM, Dong Y, Arif AA, Olsson M, Brown KL, Johnson P. The where, when, how, and why of hyaluronan binding by immune cells. Front Immunol (2015) 6:150. doi: 10.3389/fimmu.2015.00150
92. Schwertfeger KL, Cowman MK, Telmer PG, Turley EA, McCarthy JB. Hyaluronan, inflammation, and breast cancer progression. Front Immunol (2015) 6:236. doi: 10.3389/fimmu.2015.00236
93. Spinelli FM, Vitale DL, Demarchi G, Cristina C, Alaniz L. The immunological effect of hyaluronan in tumor angiogenesis. Clin Transl Immunol (2015) 4:e52. doi: 10.1038/cti.2015.35
94. Kobayashi N, Miyoshi S, Mikami T, Koyama H, Kitazawa M, Takeoka M, et al. Hyaluronan deficiency in tumor stroma impairs macrophage trafficking and tumor neovascularization. Cancer Res (2010) 70:7073–83. doi: 10.1158/0008-5472.CAN-09-4687
95. Evanko SP, Potter-Perigo S, Bollyky PL, Nepom GT, Wight TN. Hyaluronan and versican in the control of human T-lymphocyte adhesion and migration. Matrix Biol (2012) 31:90–100. doi: 10.1016/j.matbio.2011.10.004
96. Ruffell B, Johnson P. Hyaluronan induces cell death in activated T cells through CD44. J Immunol (2008) 181:7044–54. doi: 10.4049/jimmunol.181.10.7044
97. Matsumoto G, Nghiem MP, Nozaki N, Schmits R, Penninger JM. Cooperation between CD44 and LFA-1/CD11a adhesion receptors in lymphokine-activated killer cell cytotoxicity. J Immunol (1998) 160:5781–89. doi: 10.4049/jimmunol.160.12.5781
98. de la Torre M, Wells AF, Bergh J, Lindgren A. Localization of hyaluronan in normal breast tissue, radial scar, and tubular breast carcinoma. Hum Pathol (1993) 24:1294–97. doi: 10.1016/0046-8177(93)90262-f
99. Wang SJ, Bourguignon LYW. Role of hyaluronan-mediated CD44 signaling in head and neck squamous cell carcinoma progression and chemoresistance. Am J Pathol (2011) 178:956–63. doi: 10.1016/j.ajpath.2010.11.077
100. Hasselbalch H, Hovgaard D, Nissen N, Junker P. Serum hyaluronan is increased in malignant lymphoma. Am J Hematol (1995) 50:231–33. doi: 10.1002/ajh.2830500402
101. Park JB, Kwak H, Lee S. Role of hyaluronan in glioma invasion. Cell Adh Migr (2008) 2:202–07. doi: 10.4161/cam.2.3.6320
102. Takabe P, Siiskonen H, Rönkä A, Kainulainen K, Pasonen-Seppänen S. The impact of hyaluronan on tumor progression in cutaneous melanoma. Front Oncol (2022) 11:811434. doi: 10.3389/fonc.2021.811434
103. Pirinen RT, Tammi RH, Tammi MI, Paakko PK, Parkkinen JJ, Agren UM, et al. Expression of hyaluronan in normal and dysplastic bronchial epithelium and in squamous cell carcinoma of the lung. Int J Cancer (1998) 79:251–55. doi: 10.1002/(sici)1097-0215(19980619)79:3<251::aid-ijc7>3.0.co;2-o
104. Li JH, Wang YC, Qin CD, Yao RR, Zhang R, Wang Y, et al. Over expression of hyaluronan promotes progression of HCC via CD44-mediated pyruvate kinase M2 nuclear translocation. Am J Cancer Res (2016) 6:509–21.
105. Zoltan-Jones A, Huang L, Ghatak S, Toole BP. Elevated hyaluronan production induces mesenchymal and transformed properties in epithelial cells. J Biol Chem (2003) 278:45801–10. doi: 10.1074/jbc.M308168200
106. Rilla K, Pasonen-Seppänen S, Kärnä R, Karjalainen HM, Törrönen K, Koistinen V, et al. HAS3-induced accumulation of hyaluronan in 3D MDCK cultures results in mitotic spindle misorientation and disturbed organization of epithelium. Histochem Cell Biol (2012) 137:153–64. doi: 10.1007/s00418-011-0896-x
107. Sun M, Guo S, Yao J, Xiao Y, Sun R, Ma W, et al. MicroRNA-125a suppresses cell migration, invasion, and regulates hyaluronic acid synthase 1 expression by targeting signal transducers and activators of transcription 3 in renal cell carcinoma cells. J Cell Biochem (2019) 120:1894–902. doi: 10.1002/jcb.27503
108. Chi A, Shirodkar SP, Escudero DO, Ekwenna OO, Yates TJ, Ayyathurai R, et al. Molecular characterization of kidney cancer. Cancer (2012) 118:2394–402. doi: 10.1002/cncr.26520
109. Cai JL, Li M, Na YQ. Correlation between hyaluronic acid, hyaluronic acid synthase and human renal clear cell carcinoma. Chin J Cancer Res (2011) 23:59–63. doi: 10.1007/s11670-011-0059-6
110. Kusmartsev S, Kwenda E, Dominguez-Gutierrez PR, Crispen PL, O’Malley P. High levels of PD-L1+ and HYAL2+ myeloid-derived suppressor cells in renal cell carcinoma. J Kidney Cancer Vhl (2022) 9:1–06. doi: 10.15586/jkcvhl.v9i2.208
111. Dominguez-Gutierrez PR, Kwenda EP, Donelan W, O’Malley P, Crispen PL, Kusmartsev S. HYAL2 expression in tumor-associated myeloid cells mediates cancer-related inflammation in bladder cancer. Cancer Res (2021) 81:648–57. doi: 10.1158/0008-5472.CAN-20-1144
112. Zöller M. CD44: can a cancer-initiating cell profit from an abundantly expressed molecule? Nat Rev Cancer (2011) 11:254–67. doi: 10.1038/nrc3023
113. Shigeishi H, Higashikawa K, Takechi M. Role of receptor for hyaluronan-mediated motility (RHAMM) in human head and neck cancers. J Cancer Res Clin Oncol (2014) 140:1629–40. doi: 10.1007/s00432-014-1653-z
114. Jiang D, Liang J, Fan J, Yu S, Chen S, Luo Y, et al. Regulation of lung injury and repair by toll-like receptors and hyaluronan. Nat Med (2005) 11:1173–79. doi: 10.1038/nm1315
115. Lundy J, Gearing LJ, Gao H, West AC, McLeod L, Deswaerte V, et al. TLR2 activation promotes tumour growth and associates with patient survival and chemotherapy response in pancreatic ductal adenocarcinoma. Oncogene (2021) 40:6007–22. doi: 10.1038/s41388-021-01992-2
116. Dang S, Peng Y, Ye L, Wang Y, Qian Z, Chen Y, et al. Stimulation of TLR4 by LMW-HA induces metastasis in human papillary thyroid carcinoma through CXCR7. Clin Dev Immunol (2013) 2013:1–11. doi: 10.1155/2013/712561
117. McCourt PA, Ek B, Forsberg N, Gustafson S. Intercellular adhesion molecule-1 is a cell surface receptor for hyaluronan. J Biol Chem (1994) 269:30081–84. doi: 10.1016/S0021-9258(18)43775-1
118. Jackson DG. Hyaluronan in the lymphatics: The key role of the hyaluronan receptor LYVE-1 in leucocyte trafficking. Matrix Biol (2019) 78-79:219–35. doi: 10.1016/j.matbio.2018.02.001
119. Jackson DG, Prevo R, Clasper S, Banerji S. LYVE-1, the lymphatic system and tumor lymphangiogenesis. Trends Immunol (2001) 22:317–21. doi: 10.1016/S1471-4906(01)01936-6
120. Harris EN, Baker E. Role of the hyaluronan receptor, stabilin-2/HARE, in health and disease. Int J Mol Sci (2020) 21:3504. doi: 10.3390/ijms21103504
121. Zheng C, Zheng L, Yoo J, Guo H, Zhang Y, Guo X, et al. Landscape of infiltrating T cells in liver cancer revealed by single-cell sequencing. Cell (2017) 169:1342–56. doi: 10.1016/j.cell.2017.05.035
122. Day AJ, Milner CM. TSG-6: A multifunctional protein with anti-inflammatory and tissue-protective properties. Matrix Biol (2019) 78-79:60–83. doi: 10.1016/j.matbio.2018.01.011
123. Liu B, Liu T, Liu Y, Feng X, Jiang X, Long J, et al. TSG-6 promotes cancer cell aggressiveness in a CD44-dependent manner and reprograms normal fibroblasts to create a pro-metastatic microenvironment in colorectal cancer. Int J Biol Sci (2022) 18:1677–94. doi: 10.7150/ijbs.69178
124. Zhuo L, Hascall VC, Kimata K. Inter-α-trypsin inhibitor, a covalent protein-glycosaminoglycan-protein complex. J Biol Chem (2004) 279:38079–82. doi: 10.1074/jbc.R300039200
125. Obayashi Y, Yabushita H, Kanyama K, Noguchi M, Zhuo L, Kimata K, et al. Role of serum-derived hyaluronan-associated protein-hyaluronan complex in ovarian cancer. Oncol Rep (2008) 19:1245. doi: 10.3892/or.19.5.1245
126. Zhang M, Li N, Liang Y, Liu J, Zhou Y, Liu C. Hyaluronic acid binding protein 1 overexpression is an indicator for disease-free survival in cervical cancer. Int J Clin Oncol (2017) 22:347–52. doi: 10.1007/s10147-016-1077-7
127. Milev P, Maurel P, Chiba A, Mevissen M, Popp S, Yamaguchi Y, et al. Differential regulation of expression of hyaluronan-binding proteoglycans in developing brain: Aggrecan, versican, neurocan, and brevican. Biochem Biophys Res Commun (1998) 247:207–12. doi: 10.1006/bbrc.1998.8759
128. Varga I, Hutóczki G, Szemcsák CD, Zahuczky G, Tóth J, Adamecz Z, et al. Brevican, neurocan, tenascin-c and versican are mainly responsible for the invasiveness of low-grade astrocytoma. Pathol Oncol Res (2012) 18:413–20. doi: 10.1007/s12253-011-9461-0
129. Day AJ, Prestwich GD. Hyaluronan-binding proteins: Tying up the giant. J Biol Chem (2002) 277:4585–88. doi: 10.1074/jbc.R100036200
130. Milner CM, Day AJ. TSG-6: a multifunctional protein associated with inflammation. J Cell Sci (2003) 116:1863–73. doi: 10.1242/jcs.00407
131. Ponta H, Sherman L, Herrlich PA. CD44: From adhesion molecules to signalling regulators. Nat Rev Mol Cell Biol (2003) 4:33–45. doi: 10.1038/nrm1004
132. Bono P, Rubin K, Higgins JM, Hynes RO, Brugge JS. Layilin, a novel integral membrane protein, is a hyaluronan receptor. Mol Biol Cell (2001) 12:891–900. doi: 10.1091/mbc.12.4.891
133. Bourguignon LYW, Wong G, Earle CA, Xia W. Interaction of low molecular weight hyaluronan with CD44 and toll-like receptors promotes the actin filament-associated protein 110-actin binding and MyD88-NFκB signaling leading to proinflammatory cytokine/chemokine production and breast tumor invasion. Cytoskeleton (Hoboken) (2011) 68:671–93. doi: 10.1002/cm.20544
134. Karousou E, Misra S, Ghatak S, Dobra K, Götte M, Vigetti D, et al. Roles and targeting of the HAS/hyaluronan/CD44 molecular system in cancer. Matrix Biol (2017) 59:3–22. doi: 10.1016/j.matbio.2016.10.001
135. Zhang H, Li L, Wang D. Increased expression of HMMR in renal cell carcinoma is an independent prognostic factor. Oncol Lett (2023) 25:28. doi: 10.3892/ol.2022.13614
136. Ding Y, Xiong S, Chen X, Pan Q, Fan J, Guo J. HAPLN3 inhibits apoptosis and promotes EMT of clear cell renal cell carcinoma via ERK and bcl-2 signal pathways. J Cancer Res Clin Oncol (2022) 149:79–90. doi: 10.1007/s00432-022-04421-3
137. Borowsky ML, Hynes RO. Layilin, a novel talin-binding transmembrane protein homologous with c-type lectins, is localized in membrane ruffles. J Cell Biol (1998) 143:429–42. doi: 10.1083/jcb.143.2.429
138. Mahuron KM, Moreau JM, Glasgow JE, Boda DP, Pauli ML, Gouirand V, et al. Layilin augments integrin activation to promote antitumor immunity. J Exp Med (2020) 217:e20192080. doi: 10.1084/jem.20192080
139. Adachi T, Arito M, Suematsu N, Kamijo-Ikemori A, Omoteyama K, Sato T, et al. Roles of layilin in TNF-α-induced epithelial-mesenchymal transformation of renal tubular epithelial cells. Biochem Biophys Res Commun (2015) 467:63–9. doi: 10.1016/j.bbrc.2015.09.121
140. Falkowski M, Schledzewski K, Hansen B, Goerdt S. Expression of stabilin-2, a novel fasciclin-like hyaluronan receptor protein, in murine sinusoidal endothelia, avascular tissues, and at solid/liquid interfaces. Histochem Cell Biol (2003) 120:361–69. doi: 10.1007/s00418-003-0585-5
141. Banerji S, Ni J, Wang SX, Clasper S, Su J, Tammi R, et al. LYVE-1, a new homologue of the CD44 glycoprotein, is a lymph-specific receptor for hyaluronan. J Cell Biol (1999) 144:789–801. doi: 10.1083/jcb.144.4.789
142. Han DH, Song HK, Lee SY, Song J, Piao SG, Yoon HE, et al. Upregulation of hyaluronan and its binding receptors in an experimental model of chronic cyclosporine nephropathy. Nephrol (Carlton) (2010) 15:216–24. doi: 10.1111/j.1440-1797.2009.01167.x
143. Karinen S, Hujanen R, Salo T, Salem A. The prognostic influence of lymphatic endothelium–specific hyaluronan receptor 1 in cancer: A systematic review. Cancer Sci (2022) 113:17–27. doi: 10.1111/cas.15199
144. Jacobetz MA, Chan DS, Neesse A, Bapiro TE, Cook N, Frese KK, et al. Hyaluronan impairs vascular function and drug delivery in a mouse model of pancreatic cancer. Gut (2012) 62:112–20. doi: 10.1136/gutjnl-2012-302529
145. Shepard HM. Breaching the castle walls: Hyaluronan depletion as a therapeutic approach to cancer therapy. Front Oncol (2015) 5:192. doi: 10.3389/fonc.2015.00192
146. Jiang D, Liang J, Noble PW. Hyaluronan as an immune regulator in human diseases. Physiol Rev (2011) 91:221–64. doi: 10.1152/physrev.00052.2009
147. Heldin P, Asplund T, Teder P, Suzuki M, Vrochides D, Papanikolaou V, et al. Regulation of hyaluronan synthesis and the interaction of hyaluronan with cells. Acta Orthopaedica Scandinavica (2009) 66:160–62. doi: 10.3109/17453679509157682
148. McBride WH, Bard JB. Hyaluronidase-sensitive halos around adherent cells. their role in blocking lymphocyte-mediated cytolysis. J Exp Med (1979) 149:507–15. doi: 10.1084/jem.149.2.507
149. Rilla K, Siiskonen H, Tammi M, Tammi R. Hyaluronan coated extracellular vesicles a novel link between hyaluronan and cancer. Adv Cancer Res (2014) 123:121–48. doi: 10.1016/B978-0-12-800092-2.00005-8
150. Lokeshwar VB, Summan M, Andre J. Targeting hyaluronic acid family for cancer chemoprevention and therapy. Adv Cancer Res (2014) 123:35–65. doi: 10.1016/B978-0-12-800092-2.00002-2
151. Golshani R, Lopez L, Estrella V, Kramer M, Iida N, Lokeshwar VB. Hyaluronic acid synthase-1 expression regulates bladder cancer growth, invasion, and angiogenesis through CD44. Cancer Res (2008) 68:483–91. doi: 10.1158/0008-5472.CAN-07-2140
152. Michl P, Gress TM. Improving drug delivery to pancreatic cancer: breaching the stromal fortress by targeting hyaluronic acid. Gut (2012) 61:1377–79. doi: 10.1136/gutjnl-2012-302604
153. Urakawa H, Nishida Y, Wasa J, Arai E, Zhuo L, Kimata K, et al. Inhibition of hyaluronan synthesis in breast cancer cells by 4-methylumbelliferone suppresses tumorigenicity in vitro and metastatic lesions of bone in vivo. Int J Cancer (2012) 130:454–66. doi: 10.1002/ijc.26014
154. Twarock S, Freudenberger T, Poscher E, Dai G, Jannasch K, Dullin C, et al. Inhibition of oesophageal squamous cell carcinoma progression by in vivo targeting of hyaluronan synthesis. Mol Cancer (2011) 10:30. doi: 10.1186/1476-4598-10-30
155. Bhattacharyya SS, Paul S, Mandal SK, Banerjee A, Boujedaini N, Khuda-Bukhsh AR. A synthetic coumarin (4-Methyl-7 hydroxy coumarin) has anti-cancer potentials against DMBA-induced skin cancer in mice. Eur J Pharmacol (2009) 614:128–36. doi: 10.1016/j.ejphar.2009.04.015
156. Hiraga T, Ito S, Nakamura H. Cancer stem-like cell marker CD44 promotes bone metastases by enhancing tumorigenicity, cell motility, and hyaluronan production. Cancer Res (2013) 73:4112–22. doi: 10.1158/0008-5472.CAN-12-3801
157. Uchakina ON, Ban H, McKallip RJ. Targeting hyaluronic acid production for the treatment of leukemia: Treatment with 4-methylumbelliferone leads to induction of MAPK-mediated apoptosis in K562 leukemia. Leuk Res (2013) 37:1294–301. doi: 10.1016/j.leukres.2013.07.009
158. Tamura R, Yokoyama Y, Yoshida H, Imaizumi T, Mizunuma H. 4-methylumbelliferone inhibits ovarian cancer growth by suppressing thymidine phosphorylase expression. J Ovarian Res (2014) 7:94. doi: 10.1186/s13048-014-0094-2
159. Lokeshwar VB, Lopez LE, Munoz D, Chi A, Shirodkar SP, Lokeshwar SD, et al. Antitumor activity of hyaluronic acid synthesis inhibitor 4-methylumbelliferone in prostate cancer cells. Cancer Res (2010) 70:2613–23. doi: 10.1158/0008-5472.CAN-09-3185
160. Piccioni F, Malvicini M, Garcia MG, Rodriguez A, Atorrasagasti C, Kippes N, et al. Antitumor effects of hyaluronic acid inhibitor 4-methylumbelliferone in an orthotopic hepatocellular carcinoma model in mice. Glycobiology (2012) 22:400–10. doi: 10.1093/glycob/cwr158
161. Twarock S, Tammi MI, Savani RC, Fischer JW. Hyaluronan stabilizes focal adhesions, filopodia, and the proliferative phenotype in esophageal squamous carcinoma cells. J Biol Chem (2010) 285:23276–84. doi: 10.1074/jbc.M109.093146
162. Benitez A, Yates TJ, Lopez LE, Cerwinka WH, Bakkar A, Lokeshwar VB. Targeting hyaluronidase for cancer therapy: antitumor activity of sulfated hyaluronic acid in prostate cancer cells. Cancer Res (2011) 71:4085–95. doi: 10.1158/0008-5472.CAN-10-4610
163. Koutsakis C, Tavianatou A, Kokoretsis D, Baroutas G, Karamanos NK. Sulfated hyaluronan modulates the functional properties and matrix effectors expression of breast cancer cells with different estrogen receptor status. Biomolecules (2021) 11:1916. doi: 10.3390/biom11121916
164. Sato N, Kohi S, Hirata K, Goggins M. Role of hyaluronan in pancreatic cancer biology and therapy: Once again in the spotlight. Cancer Sci (2016) 107:569–75. doi: 10.1111/cas.12913
165. Morosi L, Meroni M, Ubezio P, Fuso Nerini I, Minoli L, Porcu L, et al. PEGylated recombinant human hyaluronidase (PEGPH20) pre-treatment improves intra-tumour distribution and efficacy of paclitaxel in preclinical models. J Exp Clin Cancer Res (2021) 40:286. doi: 10.1186/s13046-021-02070-x
166. Toole BP. Hyaluronan-CD44 interactions in cancer: paradoxes and possibilities. Clin Cancer Res (2009) 15:7462–68. doi: 10.1158/1078-0432.CCR-09-0479
167. Weng X, Maxwell-Warburton S, Hasib A, Ma L, Kang L. The membrane receptor CD44: Novel insights into metabolism. Trends Endocrinol Metab (2022) 33:318–32. doi: 10.1016/j.tem.2022.02.002
168. Hirose Y, Saijou E, Sugano Y, Takeshita F, Nishimura S, Nonaka H, et al. Inhibition of stabilin-2 elevates circulating hyaluronic acid levels and prevents tumor metastasis. Pro Natl Acad Sci USA (2012) 109:4263–68. doi: 10.1073/pnas.1117560109
169. Gahan J, Benitez A, Yates T, Chi A, Miami F, Bird V, et al. Targeting RHAMM, a metastasis predictor, for kidney cancer therapy. J Urol (2011) 185:e156. doi: 10.1016/j.juro.2011.02.474
170. Benitez A, Yates T, Chi A, Gahan J, Bird V, Lokeshwar V. RHAMM- a potential predictor of metastasis and therapeutic target in kidney cancer. Cancer Res (2011) 71:2598. doi: 10.1158/1538-7445.AM2011-2598
171. Wang J, Jordan AR, Zhu H, Hasanali SL, Thomas E, Lokeshwar SD, et al. Targeting hyaluronic acid synthase-3 (HAS3) for the treatment of advanced renal cell carcinoma. Cancer Cell Int (2022) 22:421. doi: 10.1186/s12935-022-02818-1
172. Liu HN, Guo NN, Guo WW, Huang-Fu MY, Vakili MR, Chen JJ, et al. Delivery of mitochondriotropic doxorubicin derivatives using self-assembling hyaluronic acid nanocarriers in doxorubicin-resistant breast cancer. Acta Pharmacol Sin (2018) 39:1681–92. doi: 10.1038/aps.2018.9
173. Lu H, Zhao H, Wang K, Lv L. Novel hyaluronic acid–chitosan nanoparticles as non-viral gene delivery vectors targeting osteoarthritis. Int J Pharm (2011) 420:358–65. doi: 10.1016/j.ijpharm.2011.08.046
Keywords: hyaluronan (HA), HA receptors, HA synthases, hyaluronidases, renal cell carcinoma (RCC), therapies
Citation: Jin C and Zong Y (2023) The role of hyaluronan in renal cell carcinoma. Front. Immunol. 14:1127828. doi: 10.3389/fimmu.2023.1127828
Received: 20 December 2022; Accepted: 20 February 2023;
Published: 02 March 2023.
Edited by:
Stevan Springer, University of Prince Edward Island, CanadaReviewed by:
Abhijit Aithal, University of Nebraska Medical Center, United StatesJames McCarthy, University of Minnesota Twin Cities, United States
Eva Turley, Lawson Health Research Institute, Canada
Sergei Kusmartsev, University of Florida, United States
Copyright © 2023 Jin and Zong. This is an open-access article distributed under the terms of the Creative Commons Attribution License (CC BY). The use, distribution or reproduction in other forums is permitted, provided the original author(s) and the copyright owner(s) are credited and that the original publication in this journal is cited, in accordance with accepted academic practice. No use, distribution or reproduction is permitted which does not comply with these terms.
*Correspondence: Yunfeng Zong, em9uZ3l1bmZlbmdAemp1LmVkdS5jbg==