- 1Ghent University Hospital, Department of Gastroenterology and Hepatology, Ghent, Belgium
- 2Ghent University, Department of Internal Medicine and Pediatrics, Ghent, Belgium
- 3University Hospitals Leuven, Department of Gastroenterology and Hepatology, Leuven, Belgium
- 4KU Leuven, Translational Research in Gastrointestinal Disorders (TARGID), Department of Chronic Diseases and Metabolism (CHROMETA), Leuven, Belgium
- 5Ghent University Hospital, Department of Dermatology, Ghent, Belgium
- 6University Hospitals Leuven, Department of Dermatology, Leuven, Belgium
- 7University Hospitals Leuven, Department of Rheumatology, Leuven, Belgium
- 8Ghent University Hospital, Department of Laboratory Medicine, Ghent, Belgium
- 9Ghent University, Department of Diagnostic Sciences, Ghent, Belgium
Background: The risks and impact of COVID19 disease and vaccination in patients with Immune Mediated Inflammatory Diseases (IMID) remain incompletely understood. IMID patients and particularly patients receiving immunosuppressive treatment were excluded from the original, registrational phase-3 COVID19 vaccination efficacy and safety trials. Real-world observational data can help to fill this gap in knowledge. The BELCOMID study aims to explore the interaction between IMIDs, immune-modulating treatment modalities and SARS-CoV-2 infection and vaccination in a real-life patient cohort.
Methods: A multidisciplinary, prospective, observational cohort study was set up. Consecutive patients with IMIDs of the gut, joints and skin followed at two high-volume referral centers were invited. Both patients under conventional treatment or targeted immune modulating therapies were included. Patient data and serological samples were collected at 3 predefined periods (before COVID19 vaccination, before booster vaccination, after booster vaccination). Primary endpoints were positive PCR-test and SARS-CoV-2 serology reflecting previous SARS-CoV-2 infection or vaccination. Associations with IMID treatment modality and IMID disease activity were assessed. Results of the first two inclusion periods (before booster vaccination) are reported.
Results: At the first inclusion period data was assessed of 2165 IMID-patients before COVID19 vaccination. At the second inclusion period, data of 2065 patients was collected of whom 1547 had received complete baseline COVID19 vaccination and 222 were partially vaccinated. SARS-CoV-2 infection rate remained low in both groups. No significant increase in IMID flare-up rate was noted in patients with prior SARS-CoV-2 infection. Multiple logistic regression analyses did not show a significant influence of IMID-treatment modality or IMID activity on SARS-CoV-2 infection risk (based on PCR positivity or N-serology). Patients treated with conventional immunomodulators, systemic steroids, and patients on advanced therapies such as biologics or small molecules, had reduced S-antibody seroconversion. S-antibody response was also lower in patients without prior SARS-CoV-2 infection and in active smokers. A subset of patients (4.1%) had no S- nor N-antibody seroconversion following complete baseline vaccination.
Conclusion: The BELCOMID study results confirm the benign course of COVID19 infection and vaccination in a large real-life IMID-population. However, our results underscore the need for repeated vaccination and smoking cessation in patients with IMIDs treated with immune-modulating therapies or systemic steroids during the pandemic.
1 Introduction
As the severe acute respiratory syndrome coronavirus 2 (SARS-CoV-2) pandemic is turning into a significant wrinkle in modern healthcare history, the exact risks and impact of COVID19 on patients with Immune Mediated Inflammatory Diseases (IMIDs) remain unclear.
SARS-CoV-2 infection may remain paucisymptomatic in up to 80% of individuals. However, COVID19 can progress to acute respiratory distress due to a cytokine storm characterized by massive production of inflammatory cytokines (1, 2). This life-threatening disease phenotype is associated with elderly age and certain comorbidities such as obesity, diabetes and arterial hypertension (1–4). Furthermore, it became clear that COVID19 can present with various extrapulmonary symptoms including gastrointestinal, dermatological and rheumatologic manifestations amongst many others (5–9).
IMIDs reflect a spectrum of disorders of the gut (Crohn’s disease, ulcerative colitis), joints (rheumatoid arthritis, psoriatic arthritis, spondylarthritis) and skin (psoriasis, hidradenitis suppurativa, atopic dermatitis). IMIDs are believed to originate from an inappropriate immune response to environmental triggers in genetically susceptible hosts. Their management has been revolutionized in the past two decades by anti-cytokine therapies, T- and B-cell targeting therapies and most recently, small molecules such as Janus Kinase inhibitors (JAKi) and PDE4 inhibitor apremilast. As the risk of infections is generally considered higher in patients under Targeted Immune-Modulating Therapies (TIMT), this could result in patients mistakenly stopping their medication, putting them at risk for flare, requiring steroid treatment and even hospitalization. Now that vaccines have been developed, it is unclear whether TIMT, and broader immunosuppression, interfere with SARS-CoV-2 serologic responses as IMID-patients were excluded from the original, registrational phase 3 COVID19 vaccine efficacy and safety studies (10–12).
As a consequence, many questions remain regarding the impact of COVID19 infection and vaccination in patients with IMIDs. We therefore explored the interaction between IMIDs, their immune modulating treatment modalities and SARS-CoV-2 in a large and real-life patient cohort.
2 Methodology
2.1 Population and design
(Detailed description of study methodology in Supplementary Materials.)
In March 2020, a cross-disciplinary consortium was set up between the University Hospitals of Leuven and Ghent. Within this consortium a multidisciplinary, prospective, observational cohort study; BELCOMID, was developed. The study was approved by the ethics committee of both hospitals (BC-08030/S64422).
Consecutive patients with IMIDs of the gut, joints and skin seen at the two hospitals were invited to participate between December 17th 2020 and February 28th 2021. Both patients under conventional treatment or TIMT were included. Conventional treatment comprised therapies without immunomodulatory effect (N-IM) and immunomodulators (IMM). N-IM included mesalazine, sulfasalazine, acitretin, metformin, zinc, antibiotics, topical treatment options and light therapy. IMM included methotrexate, ciclosporin, dimethyl fumarate, mycophenolate mofetil, leflunomide, hydroxychloroquine and thiopurines. TIMT options included biologics, JAKi and apremilast.
The study goal was twofold. The initial aim was to explore the association between COVID19 and IMIDs in a large, real-life population. This included prospective analysis of exposure to and infection with SARS-CoV-2 and relating this information to the underlying IMID disease course and treatment modalities within the study population. Secondly, as national vaccination campaigns started, the response to COVID19 vaccination in these patients depending on their different treatment modalities was studied in the same cohort.
Both clinical patient data and serial blood samples were collected at predefined registration periods between December 2020 until February 2022 with a time interval of at least 4 months between sequential sampling (see Supplementary Figure 1). Sampling period 1 ran from 17/12/2020 to 28/02/2021, prior to the national vaccination campaign. Period 2 from 01/07/2021 to 24/09/2021, prior to the start of booster vaccinations.
Data on vaccination date and type were verified by check of the Flemish database Vaccinet.
2.2 Serologic testing
For SARS-CoV-2 serologic testing, the Abbott Architect™ SARS-CoV-2 immunoglobulin G (IgG) assay (>1.4=positive) was used to detect anti-nucleocapsid antibodies (N-antibodies) and the Abbott Architect™ SARS-CoV-2 IgGII Quant assay (≥50AU/mL=seroconversion) was used to detect anti-spike protein antibodies (S-antibodies) (13, 14). Blood samples of vaccinated patients in whom no seroconversion for S- nor N-antibodies was found, were double checked with the highly sensitive and specific LIAISON®SARS-CoV-2 TrimericS IgG assay (≥33.8BAU/mL=seroconversion) (15).
2.3 Endpoints
First we explored prevalence of SARS-CoV-2 infection and serologic outcome of vaccination in our IMID cohort. Primary endpoints were therefore: positive PCR test and SARS-CoV-2 serology reflecting previous SARS-CoV-2 infection or vaccination. As a second step we explored potential associations between infection and vaccination with IMID treatment modality, IMID disease activity (using validated disease activity scores) and increased SARS-CoV-2 exposure risk. Last but not least, we performed subgroup analysis on the cohort of patients who seemed to mount a lower antibody response.
2.4 Data collection and statistics
All data was collected in a pseudonymized electronic case report form using REDCap® software. For descriptive statistics, data was exported to SPSS Statistics version 27. Analyses were performed in R version 4.0.2 with support of the Ghent University Biostatistics Unit.
Both marginal and conditional associations were examined. Marginal associations were tested using two-sided Pearson’s chi-squared tests, not taking into account potential confounders. Conditional effects were tested using adjusted binary logistic regression models and multiple logistic regression models. Due to the limited number of expected events, the number of explanatory variables that could be included in the binary logistic regression models of positive PCR test and high SARS-CoV-2 serology was restricted. Hence, to test the conditional effect of IMID treatment modality in the total population, the binary logistic regression models were adjusted for the propensity score of the respective treatment, increased exposure risk and BMI category. The propensity score was estimated by fitting a logistic regression model where treatment was the response and potential confounders were the predictors. Potential confounders of the association between treatment modality and positive PCR test or SARS-CoV-2 serology status were considered to be age, gender, smoking status, exposure risk, BMI category, IMID type, comorbidities and vaccination status.
For the continuous endpoint of S-antibody titer, linear regression analyses were performed.
All hypothesis testing was performed at the two-sided 5% significance level. No adjustment for multiple testing was made as the analyses are considered to be exploratory and hypothesis-generating. Results should be interpreted carefully and should be confirmed by other research.
3 Results
Results of data collection before the start of the national vaccination campaign (Inclusion period 1) and after onset of the vaccination campaign but before booster vaccination (Inclusion period 2) are presented.
3.1 Demographics
At baseline, 2165 IMID-patients participated. At the second inclusion period, data was collected from 1895 (87.5%) of 2165 initial patients and of 170 additional patients. Demographics were comparable for both inclusion periods (Table 1). Extensive description of IMID treatments can be found in Supplementary Table 1. Treatment patterns were comparable in both periods.
3.2 COVID19 in IMID-patients before onset of the vaccination campaign
Before onset of the vaccination campaign, almost half of participants (47.9%) were considered to have increased exposure risk to SARS-CoV-2, based on their job-related COVID19-exposure, social contacts and/or shielding behavior.
Symptoms suggestive of COVID19 (irrespective of confirmed infection) were reported by 395 (18.2%) participants. This followed the Belgian epidemiological curve (Supplementary Figure 2) (16). Hospitalization for respiratory symptoms was required in 28 patients (1.4%) of whom only one required intensive care unit (ICU) admission for invasive ventilation.
A total of 104 (5.1%) patients reported a confirmed SARS-CoV-2 infection based on positive nasopharyngeal PCR test. PCR-positivity rate was 9.9% (104/1045 tested patients). PCR-positivity rate was not influenced by flare-up rate of the underlying IMID nor by IMID treatment modality in multiple logistic regression analysis (Table 2/Figure 1).
N-antibody seroconversion and thus confirmed SARS-CoV-2 infection, was found in 3.2% of patients before vaccination. N-antibody seroconversion rate was significantly higher in patients with increased COVID19 exposure risk (RR=3.05, 95%CI 1.80-5.19, P<0.001) and in patients with prior positive PCR test (RR=62.25, 95%CI 28.92-133.96, P<0.001).
Multiple logistic regression analysis did not show significant associations between N-seroconversion rate and IMID flare-up or IMID-treatment modality (Table 2/Figure 1). However, subgroup analyses within the group of patient treated with TIMT found a significantly higher odds ratio for N-seroconversion in patients treated with anti-IL12/23 or anti-IL23 (OR 2.64, 95% CI 1.22-5.31, P=0.009). Furthermore, anti-TNF treatment led to significantly lower odds of N-seroconversion compared to patients treated with vedolizumab (OR=0.46, 95%CI 0.22-0.98, P=0.04).
The N-antibody seroconversion rate was significantly higher if the interval between positive PCR and blood withdrawal for serology analysis was shorter than 120 days (RR=4.1, 95%CI 1.64-10.24, P=0.0015).
3.3 COVID19 after onset of the vaccination campaign
At the second study sampling timepoint, 1547 of 2065 participants had received complete baseline vaccination (i.e. 2 doses of mRNA-1273, BNT162b2 or ChadOx1 nCoV-19 or 1 dose of JN78436735) and 222 were partially vaccinated. The majority (66.6%) received BNT162b2.
Symptoms suggestive for COVID19 were experienced by 7.6% of patients, positive PCR test was reported by 4.6% and test positivity rate (9.3%) was comparable to before the vaccination campaign. Only seven patients were hospitalized for COVID19 and none required ICU admission. Multiple logistic regression analyses again showed no significant influence of IMID treatment modality on PCR-positivity rate (Table 2/Figure 1).
An extensive overview of antibody seroconversion rates per vaccine can be found in Supplementary Table 2. N-antibody seroconversion was confirmed in 2.6%. IMID flare-up or treatment modality did not significantly influence N-seroconversion rates (Table 2/Figure 1).
Seroconversion rate for S-antibodies was 91.2%. Active IMID disease significantly decreased the risk of S-seroconversion (RR=0.49, 95%CI 0.36-0.66, P<0.001). In contrast to what was found for N-antibodies, IMID treatment modality interfered with the S-antibody response. The odds for S-seronegativity were significantly higher in patients using TIMT, IMM, combination treatment (IMM+TIMT) and systemic steroids (Table 2/Figure 1). Further subgroup analysis within the patient group treated with TIMT revealed that patients treated with rituximab had a significantly higher odds for S-seronegativity (OR 14.6, 95%CI 4.80-48.2, P<0.001). No significant difference was found when comparing patients treated with anti-TNF to patients on vedolizumab (OR=1.47, 95%CI: 0.84-2.71, P=0.19). However, patients on vedolizumab who received complete baseline vaccination without positive PCR-test, had significantly higher anti-S-antibody titers compared to patients treated with anti-TNF (P<0.001) (Figure 2).
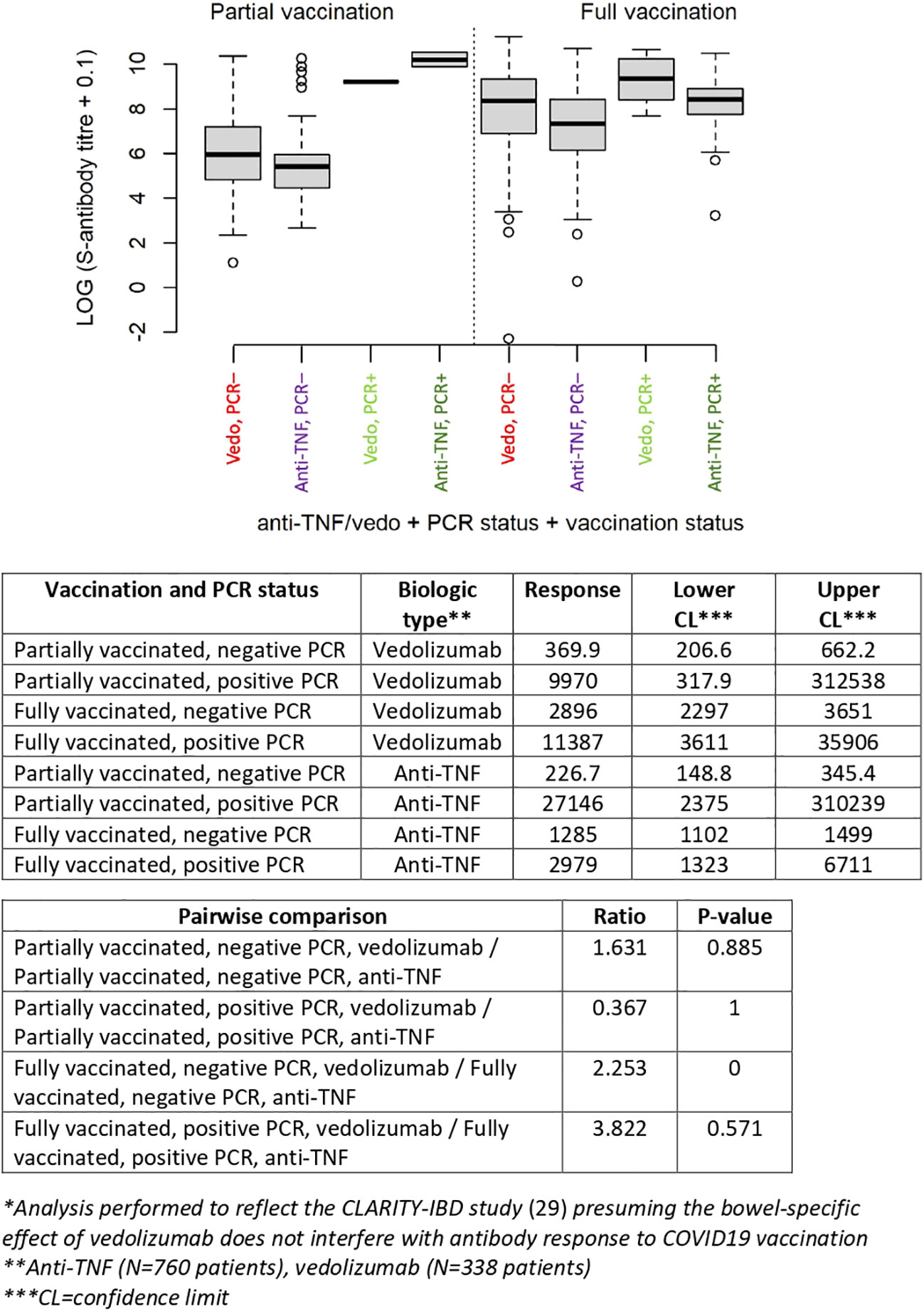
Figure 2 Anti-S-antibody titer (log-transformed) according to vaccination status, PCR status (positive or negative) and treatment modality (anti-TNF or vedolizumab*).
In fully vaccinated patients chi square comparisons did not identify significant differences in S-seroconversion rate between different vaccination types (Supplementary Table 3).
Pairwise comparisons show that S-antibody titers were higher in patients with previous PCR-positivity compared to patients with equal vaccination status without prior SARS-CoV-2 infection. Furthermore, S-antibody titers were higher in partially vaccinated patients with previous PCR-positivity compared to fully vaccinated patients without positive PCR test (Figure 3).
3.4 Lower serologic response
A particular subgroup of interest were patients within the lowest quartile of S-antibody titers (see Supplementary Table 4 for absolute values of S-antibody titers) and those with absence of seroconversion for both S- and N-antibodies following complete baseline vaccination.
Several risk factors for the lowest S-antibody quartile were identified.
Patients vaccinated with ChadOx1 nCoV-19 had higher risk compared to BNT162b2 (RR=2.00, 95%CI 1.70-2.40, P<0.001). Patients with mRNA-1273 vaccination had lower risk compared to BNT162b2 (RR=0.32, 95%CI 0.16-0.67, P=0.001).
Longer interval between vaccination and blood draw led to a significant higher risk of being in the lowest quartile of S-antibody titers (>90 days: RR=1.2, 95%CI 0.95-1.5, P=0.159; >120 days: RR=1.48, 95%CI 1.13-1.95, P=0.01).
Patients with complete baseline vaccination treated with TIMT (OR=2.12, 95%CI 1.46-3.16, P<0.001), combination of TIMT/IMM (OR=2.23, 95%CI 1.57-3.17, P<0.001) or systemic steroids (OR=2.48, 95%CI 1.54-3.97, P<0.001) had significantly higher odds of being in the lowest S-antibody quartile. Among TIMT, treatment with anti-TNF had a higher risk of being in the lowest quartile of S-antibody titer (OR=1.63, 95%CI 1.27-2.08, P<0.001). Rituximab also increased the risk of being in the lowest quartile (OR=6.82, 95%CI 2.31-23.3, P<0.001).
Lastly, patients with active IMID disease (RR=1.30, 95%CI 1.10-1.60, P=0.002) but also active smokers (RR=1.4, 95%CI 1.2-1.7, P=0.002) had a significantly higher risk of being in the lowest quartile of S-antibody titer.
On the other hand, patients with a positive PCR test in the timeframe between first inclusion period and second inclusion had significantly lower risk of ending up in the lowest quartile (RR=0.33, 95%CI 0.15-0.72, P=0.002).
In 66 of 1547 fully vaccinated IMID-patients, no seroconversion for either N- or S-antibodies was found. Following retesting with the highly sensitive and specific LIAISON®SARS-CoV-2 TrimericS IgG assay, 3 additional patients with S-seroconversion were picked up, leaving 63 patients (4.07%) with negative serology despite complete vaccination. Interestingly, 90.5% of these combined seronegative patients were treated with a biologic, of which 23 (36.5%) were on anti-TNF and 12 (19%) on rituximab. Furthermore, over half of these patients experienced IMID flare-up since previous inquiry (subgroup demographics in Supplementary Table 5).
3.5 Impact of SARS-CoV-2 infection and vaccination on IMID disease course
Before vaccination, 39.1% of patients experienced IMID flare-ups 10.7% required systemic steroids. No significant difference in flare-up rate was noted in patients with or without SARS-CoV-2 infection (RR=0.90, 95% CI 0.67-1.20, P=0.553).
At the second BELCOMID inclusion period, after onset of the vaccination campaign, IMID flare-up rate since previous inquiry was 29.1% and 5.7% of patients needed systemic steroids. Patients with positive SARS-CoV-2 PCR or N-antibody seroconversion prior to vaccination, did not experience increased IMID flare-up rates compared to patients without prior COVID19 (RR=1.10, 95%CI 0.72-1.50, P=0.907).
4 Discussion
IMID-patients may be more affected by the pandemic compared to healthy peers. Particularly patients taking immunosuppressants expressed increased concern about potential SARS-CoV-2 infection and interactions with their IMID therapy, leading to increased psychological distress and reduced quality of life (17, 18). Previous observational studies showed that IMID-patients have a higher prevalence of COVID19 compared to healthy controls (19, 20). A review of 62 studies including >300.000 patients showed a COVID19 event rate based on PCR of 0.011 and meta-analysis of seven case-controlled studies found that SARS-CoV-2 infection risk in patients with autoimmune diseases including IMIDs was significantly higher compared to controls (OR=2.19; 95%CI 1.05-4.58, P=0.038) (21).
In BELCOMID, SARS-CoV-2 infection prevalence was assessed by combination of reported PCR-positivity and measured N-seroconversion. Prior to the vaccination campaign, prevalence of COVID19 in our patients remained low. Remarkably, N-antibody seroconversion rates were lower than PCR-positivity rates. This observation might have a multifactorial basis. On the one hand it might indicate a potential attenuation of serologic responses to SARS-CoV-2 infection in IMID populations. Indeed, Simon et al. found that IMID-patients receiving cytokine inhibitors, had lower N-seroconversion rates compared to healthy individuals (22). However, in the BELCOMID cohort, multivariate logistic regression showed no clear and consistent impact of TIMT, IMM or their combination on positive PCR rate and N-seroconversion rate over both registration periods. In contrast to what was reported in the review of Akiyama et al. (21), systemic steroids also did not influence N-antibody seroconversion rate.
Another potential explanation for the discrepancy between N-seroconversion and PCR-positivity rate lies in the durability of the humoral response and the relative timing of PCR-testing and blood withdrawal for serologic analysis. Indeed several prospective and observational studies have reported progressive waning of the humoral immune response to SARS-CoV-2 infection and COVID19 vaccination over time (23–29). Following infection, antibody titers have been shown to decline from 8-9 weeks after onset of symptoms with detectable levels up to 12 weeks (30, 31). A large Italian longitudinal prospective study even revealed a 46% decay of S-antibodies in 9 months (32) and the Virus Watch prospective community cohort study in England and Wales showed that N-antibody titers started to decline onwards from 120 days after PCR testing (33). Similarly, in our cohort we demonstrated in non-vaccinated patients that the N-seroconversion rate declined if the interval between positive PCR test and blood withdrawal for N-antibody assessment exceeded 120 days.
Few studies assessed serologic responses to COVID19 vaccination in IMID-patients compared to healthy controls. IMID-patients and particularly patients receiving immunosuppressive treatment were excluded from the registrational COVID19 vaccination trials. Real-world observational data therefore helps to fill this gap in knowledge. A prospective study in patients with acquired or inherited immune disorders showed variable immune responses to vaccination with BNT162b2 (34). A meta-analysis focusing on studies in IMID-patients showed that a significantly smaller proportion of IMID-patients seroconverted after mRNA vaccination compared to healthy controls (OR=0.086; 95%CI 0.04–0.21; P<0.001) (35). In our study the S-antibody seroconversion rate was high (91.2%), but we identified several factors that influenced S-antibody response.
First, we found that TIMT, with or without combined IMM and systemic steroid treatment were associated with significantly reduced S-seroconversion rates and increased odds of being in the lowest S-antibody titer quartile. Subgroup analyses within the TIMT group, revealed anti-TNF and rituximab as risk factors for lower S-titers. This is in line with Garcillàn et al, who found that corticosteroids, B-cell depleting therapies and JAKi substantially affect vaccine immunogenicity (36) and with the VIP-study that revealed lower antibody levels in IBD patients on infliximab with or without thiopurines and tofacitinib (37). Importantly, we confirmed findings from the CLARITY-IBD study, showing that the blunting effect of anti-TNF on S-antibody response is more pronounced than that of the gut-selective vedolizumab (38, 39).
Next, vaccination characteristics seem to impact S-serology. Pairwise comparisons in our broader IMID cohort again confirmed the CLARITY-IBD study findings (39) by demonstrating higher S-antibody titers in partially vaccinated patients with previous PCR-positivity compared to fully vaccinated patients without positive PCR test. Studies comparing efficacy of different vaccination types in IMID-patients are limited. In our cohort, the risk of being in the lowest S-antibody quartile was more pronounced in patients who received ChadOx1 nCoV-19 compared to other vaccine types. This is similar to the findings of a Taiwanese, single-center study reporting higher positive rates of anti-S IgG and higher titers of anti-S IgG after two doses of mRNA-1273 or BNT162b2 compared to ChAdOx1 (40). In a British IBD population, the highest antibody response was also seen in patients receiving BNT162b2 vaccination (41).
Thirdly, just as for the N-antibodies, our results suggest a decline of S-antibody titer over time as an interval of more than 120 days between last vaccine dose and serology analysis led to a significantly increased risk of being in the lowest quartile of S-antibody titers. However, the same time interval was not associated with a significant difference in S-antibody seroconversion rate which might be related to a longer durability of S-antibody response. Indeed other observational and prospective studies have shown a faster and higher rate of decline in N-antibodies compared to S-antibodies (29, 31, 42).
Remarkably, our study identified 63 patients with combined seronegativity after complete vaccination. A potential explanation for this observation could be that this subgroup consist of patients with more refractory IMID disease. This is suggested by the high rate of TIMT and combination of TIMT/IMM use. Furthermore, there is a numerically higher rate of IMID flare-up leading to higher corticosteroid use in this subgroup compared to the total BELCOMID population. Therefore, based on our previously described findings, this refractory subgroup may have been prone to increased attenuation of antibody responses. A third registration timepoint is foreseen within the BELCOMID study protocol. This registration timepoint will enable to assess the interaction between IMIDs and COVID19 after booster vaccination. If absence of seroconversion persists despite booster vaccination, additional analyses including assessment of cellular immunity and genetic predisposition may provide answers to whether these patients actually do mount sufficient protection against SARS-CoV-2.
Another particular finding was the interaction between active smoking and antibody titers. Smoking might facilitate SARS-CoV-2 viral entry through epigenetic mechanisms that alter transcription of several key proteins implicated in the development of COVID19 (43). However, results of observational studies remain controversial. Some studies have shown lower seroconversion rates in active smokers whereas others identified smoking as a potential risk factor for severe COVID19 (44). In our study smokers did have significantly higher risk of being in the lowest quartile of S-antibody titers. This indicates that active smoking negatively impacts humoral response to COVID19 vaccination. The exact mechanism for this phenomenon remains unknown (44).
Our study has some limitations. First, patients were recruited during routine follow-up at their respective treatment facilities. This might have caused recruitment bias by overlooking patients that were, for example, admitted at ICU with severe COVID19 bypassing contact moments with their treating physician. Numbers of patients with severe COVID19 requiring ICU admission in our cohort were however very low. Therefore, we were unable to draw any conclusions towards the association between IMIDs and severe COVID19. Other observational studies however, confirm low rates of severe COVID19 even in unvaccinated IMID cohorts (28, 45). Pooled analysis of 3 large international registries SECURE-IBD, GRA and PsoProtect identified old age, number of comorbidities, use of systemic steroids, thiopurines or combination of anti-TNF with a thiopurine (but not anti-TNF monotherapy), methotrexate, rituximab and JAKi as risk factors for severe COVID19 (36, 46–51). These associations were again found in predominantly unvaccinated populations.
Secondly, evaluation of potential differences in effect on S-antibody response between different IMID types was not performed. This was not within the study goals and not reliably achievable with our statistical models.
Lastly, only humoral response to COVID19 was assessed and we did not report on different strains of SARS-CoV-2. Serological assays may be challenged by different viral variants. However, so far no impairment of the used Abbott® antibody assays targeting the nucleocapsid protein has been reported (52). Furthermore, previous studies have shown good correlation between humoral response and T-cell mediated immunity (53).
Strengths of our study include the multidisciplinary design which may serve as a platform for future multidisciplinary IMID-COVID19 related research initiatives. Furthermore, our study focusses on a spectrum of IMID pathologies taking into account all possible treatment modalities without interference with the routine follow-up and treatment decisions in clinical practice. Both S- and N-antibodies were analyzed and combined seronegativity after vaccination was checked with an additional LIAISON®SARS-CoV-2 TrimericS IgG assay. Last but not least, the prospective approach with repeated sampling at different timepoints throughout the several stages of the pandemic enables analysis of intra-patient variations and allows study of the IMID-COVID19 interaction before vaccination, after baseline vaccination and, in the future, after booster vaccination. We therefore believe that our large real-life study cohort can serve as a good representation of IMID-patients in general.
In conclusion, the BELCOMID study prospectively evaluated SARS-CoV-2 infection and vaccination in a real-life cohort of IMID-patients followed at two Belgian tertiary centers, with emphasis on impact of IMID treatment modality and disease course. Our results confirm the benign course of COVID19 in this population and show no significant impact of SARS-CoV-2 infection on IMID disease course.
The blunted antibody response in patients treated with systemic steroids, TIMT and/or IMM and the presumed limited long-term duration of humoral response to both SARS-CoV-2 infection and vaccination stresses the importance of complete and repeated vaccination in IMID-patients. Furthermore, given the lower S-antibody response, this study underlines the importance of smoking cessation in IMID-patients particularly during the pandemic.
Data availability statement
The raw data supporting the conclusions of this article will be made available by the authors, upon reasonable request.
Ethics statement
The studies involving human participants were reviewed and approved by the Ethics Committees of University Hospital of Ghent (BC-08030) and University Hospitals of Leuven (S64422). The patients/participants provided their written informed consent to participate in this study.
Author contributions
Within the BELCOMID study group all members played a substantial role in set-up, design, data collection and data processing. Statistical analyses were done by JG, TL, MT, and SV with support of the Ghent University Biostatistics Unit. Manuscript was drafted by JG and then reviewed and adapted by all members of the BELCOMID study group. All authors contributed to the article and approved the submitted version.
Funding
BELCOMID received research grants from: Almirall, Roche, Janssen, Pfizer, Eli Lilly, Amgen, Biogen, Mylan, LEO Pharma. These grants were used to finance sample collection, study coordinators and support of the Ghent University Biostatistics Unit. The afore mentioned companies had no role in study design, conduct nor reporting of results.
Acknowledgments
Special thanks goes to all study coordinators of both participating centers and the Ghent University Biostatistics Unit
Conflict of interest
The BELCOMID study group received research grants from following pharmaceutical companies: Almirall, Roche, Janssen, Pfizer, Eli Lilly, Amgen, Biogen, Mylan, LEO Pharma. These companies had no role in study design, conduct nor reporting of results. JG has served as advisory board member or speaker for Arena, Janssen and Galapagos. JS received speaker’s fees from Pfizer, Abbvie, Ferring, Falk, Takeda, Janssen, and Fresenius. JS received consultancy fees from Janssen, Ferring, Fresenius, Abbvie, Galapagos, Celltrion, Pharmacosmos, and Pharmanovia. Research support: Galapagos and Viatris. JS is supported by a Senior Clinical researcher grant from the Research foundation – Flanders. MF reports being a consultant or speaker for AbbVie, Amgen, Biogen, Boehringer Ingelheim, Celgene, Celltrion, Dr Falk, Ferring, Janssen-Cilag, Lamepro, Eli Lilly, Medtronic, Merck Sharp & Dohme MSD, Mylan, Pfizer, Regeneron, Samsung Bioepis, Sandoz, Takeda, Thermo Fisher Scientific, and Truvion Healthcare; and received research grants from AbbVie, Amgen, Biogen, Janssen, Pfizer, Takeda, and Viatris. JL has received recent grants/speaker fees of and did consulting for AbbVie, Almirall, Bristol Myers Squibb, Celtrion, Janssen, Eli Lilly, Novartis, UCB Pharma – not personal but paid to an institutional university account. HL reports receiving consultancy or speaker fees from AbbVie, Almirall, Amgen, Leo Pharma, Pfizer, – not personal but paid to an institutional university hospital account. TH has received grants and consulting and/or speaking fees from: AbbVie, Almirall, Amgen, Biogen, Bristol Myers Squibb, Celgene, Janssen, LEO Pharma, Eli Lilly, Novartis, Pfizer Inc, Sandoz, Sanofi, UCB Pharma. AL reports being a consultant or speaker for: AbbVie, Bayer, Falk Pharma, Janssen, Eli Lilly, Novartis, Pfizer Inc, Sanofi, UCB Pharma. KV reports being a consultant or speaker for Abbvie, Eli Lilly, Novartis,Pfizer, MSD, Acelyrin, UCB Pharma, Leo Pharma, Amgen and has received financial research support from UCB Pharma and Celgene. PV reports being a consultant or speaker for Abbvie, Eli Lilly, Galapagos, Gilead, MSD, Nordic Pharma, Roularta, Sidekick Health and has received financial research support from Pfizer. EP has received a consultant of speaker’s fees/travel grants on institutional account from Hologic, bioMerieux, DiaSorin, Novosanis. TL has received financial support for research from Abbvie, Mylan, MSD, Mundipharma, Biogen, Janssen, Pfizer and Takeda, Speaker fees fromFerring, MSD, Abbvie, Janssen, Amgen, Fresenius Kabi, Galapagos and Takeda and Consultancy fee from Janssen, Galapagos, Amgen, Bristol Myers Squibb, Fresenius Kabi and Takeda. SV has received grants from AbbVie, J&J, Pfizer, Takeda and Galapagos; and consulting and/or speaking fees from: AbbVie, AbolerIS Pharma, AgomAb, Alimentiv, Arena Pharmaceuticals, AstraZeneca, Avaxia, BMS, Boehringer Ingelheim, Celgene, CVasThera, Cytoki Pharma, Dr Falk Pharma, Ferring, Galapagos, Genentech-Roche, Gilead, GSK, Hospira, Imidomics, Janssen, J&J, Lilly, Materia Prima, MiroBio, Morphic, MrMHealth, Mundipharma, MSD, Pfizer, Prodigest, Progenity, Prometheus, Robarts Clinical Trials, Second Genome, Shire, Surrozen, Takeda, Theravance, Tillots Pharma AG, Zealand Pharma.
The remaining author declares that the research was conducted in the absence of any commercial or financial relationships that could be construed as a potential conflict of interest.
Publisher’s note
All claims expressed in this article are solely those of the authors and do not necessarily represent those of their affiliated organizations, or those of the publisher, the editors and the reviewers. Any product that may be evaluated in this article, or claim that may be made by its manufacturer, is not guaranteed or endorsed by the publisher.
Supplementary material
The Supplementary Material for this article can be found online at: https://www.frontiersin.org/articles/10.3389/fimmu.2023.1126351/full#supplementary-material
References
1. Zhou F, Yu T, Du R, Fan G, Liu Y, Liu Z, et al. Clinical course and risk factors for mortality of adult inpatients with COVID-19 in wuhan, China: a retrospective cohort study. Lancet (2020) 395(10229):1054–62. doi: 10.1016/S0140-6736(20)30566-3
2. Guan W, Ni Z, Hu Y, Liang W, Ou C, He J, et al. Clinical characteristics of coronavirus disease 2019 in China. N Engl J Med (2020) 382(18):1708–20. doi: 10.1056/NEJMoa2002032
3. Grasselli G, Zangrillo A, Zanella A, Antonelli M, Cabrini L, Castelli A, et al. Baseline characteristics and outcomes of 1591 patients infected with SARS-CoV-2 admitted to ICUs of the Lombardy region, Italy. Jama (2020) 323(16):1574–81. doi: 10.1001/jama.2020.5394
4. Wang D, Hu B, Hu C, Zhu F, Liu X, Zhang J, et al. Clinical characteristics of 138 hospitalized patients with 2019 novel coronavirus-infected pneumonia in wuhan, China. JAMA (2020) 323(11):1061–9. doi: 10.1001/jama.2020.1585
5. Gupta A, Madhavan MV, Sehgal K, Nair N, Mahajan S, Sehrawat TS, et al. Extrapulmonary manifestations of COVID-19. Nat Med (2020) 26(7):1017–32. doi: 10.1038/s41591-020-0968-3
6. Ciaffi J, Meliconi R, Ruscitti P, Berardicurti O, Giacomelli R, Ursini F. Rheumatic manifestations of COVID-19: a systematic review and meta-analysis. BMC Rheumatol (2020) 4(1):65. doi: 10.1186/s41927-020-00165-0
7. Mirza FN, Malik AA, Omer SB, Sethi A. Dermatologic manifestations of COVID-19: a comprehensive systematic review. Int J Dermatol (2021) 60(4):418–50. doi: 10.1111/ijd.15168
8. Gu J, Han B, Wang J. COVID-19: Gastrointestinal manifestations and potential fecal–oral transmission. Gastroenterology (2020) 158(6):1518–9. doi: 10.1053/j.gastro.2020.02.054
9. Patel KP, Patel PA, Vunnam RR, Hewlett AT, Jain R, Jing R, et al. Gastrointestinal, hepatobiliary, and pancreatic manifestations of COVID-19. J Clin Virol (2020) 128(April):104386. doi: 10.1016/j.jcv.2020.104386
10. Falsey AR, Sobieszczyk ME, Hirsch I, Sproule S, Robb ML, Corey L, et al. Phase 3 safety and efficacy of AZD1222 (ChAdOx1 nCoV-19) covid-19 vaccine. N Engl J Med (2021) 385(25):2348–60. doi: 10.1056/NEJMoa2105290
11. Baden LR, El Sahly HM, Essink B, Kotloff K, Frey S, Novak R, et al. Efficacy and safety of the mRNA-1273 SARS-CoV-2 vaccine. N Engl J Med (2021) 384(5):403–16. doi: 10.1056/NEJMoa2035389
12. Polack FP, Thomas SJ, Kitchin N, Absalon J, Gurtman A, Lockhart S, et al. Safety and efficacy of the BNT162b2 mRNA covid-19 vaccine. N Engl J Med (2020) 383(27):2603–15. doi: 10.1056/NEJMoa2034577
13. FDA. SARS-CoV-2 IgG 2020. (2020). Available at: https://www.fda.gov/media/137383/download.
14. Wadhwa A, Yin S, Freeman B, Hershow RB, Killerby M, Yousaf AR, et al. Comparison of the SARS-CoV-2 spike protein ELISA and the Abbott architect SARS-CoV-2 IgG nucleocapsid protein assays for detection of antibodies. PloS One (2021) 16(7):e0255208. doi: 10.1371/journal.pone.0255208
15. Bonelli F, Blocki FA, Bunnell T, Chu E, de la OA, Grenache DG, et al. Evaluation of the automated LIAISON ® SARS-CoV-2 TrimericS IgG assay for the detection of circulating antibodies. Clin Chem Lab Med (2021) 59(8):1463–7. doi: 10.1515/cclm-2021-0023/html
16. Sciensano. Belgium COVID-19 epidemiological situation. (2022), Available at: https://covid-19.sciensano.be/nl/covid-19-epidemiologische-situatie.
17. Grunert PC, Reuken PA, Stallhofer J, Teich N, Stallmach A. Inflammatory bowel disease in the COVID-19 pandemic: the patients’ perspective. J Crohn’s Colitis (2020) 14(12):1702–8. doi: 10.1093/ecco-jcc/jjaa126
18. Graff LA, Fowler S, Jones JL, Benchimol EI, Bitton A, Huang JG, et al. Crohn’s and colitis canada’s 2021 impact of COVID-19 and inflammatory bowel disease in Canada: Mental health and quality of life. J Can Assoc Gastroenterol (2021) 4(Supplement_2):S46–53. doi: 10.1093/jcag/gwab031
19. Pablos JL, Abasolo L, Alvaro-Gracia JM, Blanco FJ, Blanco R, Castrejón I, et al. Prevalence of hospital PCR-confirmed COVID-19 cases in patients with chronic inflammatory and autoimmune rheumatic diseases. Ann Rheum Dis (2020) 79(9):1170–3. doi: 10.1136/annrheumdis-2020-217763
20. Attauabi M, Poulsen A, Theede K, Pedersen N, Larsen L, Jess T, et al. Prevalence and outcomes of COVID-19 among patients with inflammatory bowel disease–a Danish prospective population-based cohort study. J Crohn’s Colitis (2021) 15(4):540–50. doi: 10.1093/ecco-jcc/jjaa205
21. Akiyama S, Hamdeh S, Micic D, Sakuraba A. Prevalence and clinical outcomes of COVID-19 in patients with autoimmune diseases: a systematic review and meta-analysis. Ann Rheum Dis (2021) 80(3):384–91. doi: 10.1136/annrheumdis-2020-218946
22. Simon D, Tascilar K, Krönke G, Kleyer A, Zaiss MM, Heppt F, et al. Patients with immune-mediated inflammatory diseases receiving cytokine inhibitors have low prevalence of SARS-CoV-2 seroconversion. Nat Commun (2020) 11(1):1–7. doi: 10.1038/s41467-020-17703-6
23. Laing ED, Weiss CD, Samuels EC, Coggins SA, Wang W, Wang R, et al. Durability of antibody response and frequency of SARS-CoV-2 infection 6 months after COVID-19 vaccination in healthcare workers. Emerg Infect Dis (2022) 28(4):828–32. doi: 10.3201/eid2804.212037
24. Venerito V, Stefanizzi P, Martinelli A, Fornaro M, Galeone MG, Tafuri S, et al. Anti-SARS-CoV-2 antibody decay after vaccination and immunogenicity of the booster dose of the BNT162b2 mRNA vaccine in patients with psoriatic arthritis on TNF inhibitors. Clin Exp Rheumatol (2022) 41(1):166–9. doi: 10.55563/clinexprheumatol/hptln9
25. Levin EG, Lustig Y, Cohen C, Fluss R, Indenbaum V, Amit S, et al. Waning immune humoral response to BNT162b2 covid-19 vaccine over 6 months. N Engl J Med (2021) 385(24):e84. doi: 10.1056/NEJMoa2114583
26. Marot S, Malet I, Leducq V, Zafilaza K, Sterlin D, Planas D, et al. Rapid decline of neutralizing antibodies against SARS-CoV-2 among infected healthcare workers. Nat Commun (2021) 12(1):844. doi: 10.1038/s41467-021-21111-9
27. Yamayoshi S, Yasuhara A, Ito M, Akasaka O, Nakamura M, Nakachi I, et al. Antibody titers against SARS-CoV-2 decline, but do not disappear for several months. EClinicalMedicine (2021) 32:100734. doi: 10.1016/j.eclinm.2021.100734
28. Simon D, Tascilar K, Kleyer A, Fagni F, Krönke G, Meder C, et al. Impact of cytokine inhibitor therapy on the prevalence, seroconversion rate, and longevity of the humoral immune response against SARS – CoV -2 in an unvaccinated cohort. Arthritis Rheumatol (2022) 74(5):783–90. doi: 10.1002/art.42035
29. Van Elslande J, Gruwier L, Godderis L, Vermeersch P. Estimated half-life of SARS-CoV-2 anti-spike antibodies more than double the half-life of anti-nucleocapsid antibodies in healthcare workers. Clin Infect Dis (2021) 73(12):2366–8. doi: 10.1093/cid/ciab219
30. Li K, Huang B, Wu M, Zhong A, Li L, Cai Y, et al. Dynamic changes in anti-SARS-CoV-2 antibodies during SARS-CoV-2 infection and recovery from COVID-19. Nat Commun (2020) 11(1):6044. doi: 10.1038/s41467-020-19943-y
31. Olmstead AD, Nikiforuk AM, Schwartz S, Márquez AC, Valadbeigy T, Flores E, et al. Characterizing longitudinal antibody responses in recovered individuals following COVID-19 infection and single-dose vaccination: A prospective cohort study. Viruses (2022) 14(11):2416. doi: 10.3390/v14112416
32. Sansone E, Bonfanti C, Sala E, Renzetti S, Terlenghi L, Matteelli A, et al. Immune responses to SARS-CoV-2 infection and vaccine in a big Italian COVID-19 hospital: An 18-month follow-up. Vaccines (2022) 11(1):8. doi: 10.3390/vaccines11010008
33. Navaratnam AMD, Shrotri M, Nguyen V, Braithwaite I, Beale S, Byrne TE, et al. Nucleocapsid and spike antibody responses following virologically confirmed SARS-CoV-2 infection: an observational analysis in the virus watch community cohort. Int J Infect Dis (2022) 123:104–11. doi: 10.1016/j.ijid.2022.07.053
34. Oyaert M, De Scheerder MA, Van Herrewege S, Laureys G, Van Assche S, Cambron M, et al. Evaluation of humoral and cellular responses in SARS-CoV-2 mRNA vaccinated immunocompromised patients. Front Immunol (2022) 13(March):1–13. doi: 10.3389/fimmu.2022.858399
35. Sakuraba A, Luna A, Micic D. Serologic response to coronavirus disease 2019 (COVID-19) vaccination in patients with immune-mediated inflammatory diseases: A systematic review and meta-analysis. Gastroenterology (2022) 162(1):88–108.e9. doi: 10.1053/j.gastro.2021.09.055
36. Garcillán B, Salavert M, Regueiro JR, Díaz-Castroverde S. Response to vaccines in patients with immune-mediated inflammatory diseases: A narrative review. Vaccines (2022) 10(2):297. doi: 10.3390/vaccines10020297
37. Alexander JL, Kennedy NA, Ibraheim H, Anandabaskaran S, Saifuddin A, Castro Seoane R, et al. COVID-19 vaccine-induced antibody responses in immunosuppressed patients with inflammatory bowel disease (VIP): a multicentre, prospective, case-control study. Lancet Gastroenterol Hepatol (2022) 7(4):342–52. doi: 10.1016/S2468-1253(22)00005-X
38. Kennedy NA, Goodhand JR, Bewshea C, Nice R, Chee D, Lin S, et al. Anti-SARS-CoV-2 antibody responses are attenuated in patients with IBD treated with infliximab. Gut (2021) 70(5):865–75. doi: 10.1136/gutjnl-2021-324388
39. Kennedy NA, Lin S, Goodhand JR, Chanchlani N, Hamilton B, Bewshea C, et al. Infliximab is associated with attenuated immunogenicity to BNT162b2 and ChAdOx1 nCoV-19 SARS-CoV-2 vaccines in patients with IBD. Gut (2021) 70(10):1884–93. doi: 10.1136/gutjnl-2021-324789
40. Tien N, Chang Y-C, Chen P-K, Lin H-J, Chang S-H, Lan J-L, et al. The immunogenicity and safety of three types of SARS-CoV-2 vaccines in adult patients with immune-mediated inflammatory diseases: A longitudinal cohort study. Biomedicines (2022) 10(4):911. doi: 10.3390/biomedicines10040911
41. Lin S, Kennedy NA, Saifuddin A, Sandoval DM, Reynolds CJ, Seoane RC, et al. Antibody decay, T cell immunity and breakthrough infections following two SARS-CoV-2 vaccine doses in inflammatory bowel disease patients treated with infliximab and vedolizumab. Nat Commun (2022) 13(1):1379. doi: 10.1038/s41467-022-28517-z
42. Lumley SF, Wei J, O’Donnell D, Stoesser NE, Matthews PC, Howarth A, et al. The duration, dynamics, and determinants of severe acute respiratory syndrome coronavirus 2 (SARS-CoV-2) antibody responses in individual healthcare workers. Clin Infect Dis (2021) 73(3):e699–709. doi: 10.1093/cid/ciab004
43. Shirvaliloo M. The unfavorable clinical outcome of COVID-19 in smokers is mediated by H3K4me3, H3K9me3 and H3K27me3 histone marks. Epigenomics (2022) 14(3):153–62. doi: 10.2217/epi-2021-0476
44. Ferrara P, Gianfredi V, Tomaselli V, Polosa R. The effect of smoking on humoral response to COVID-19 vaccines: A systematic review of epidemiological studies. Vaccines (2022) 10(2):303. doi: 10.3390/vaccines10020303
45. Sarmiento-Monroy JC, Espinosa G, Londoño M-C, Meira F, Caballol B, Llufriu S, et al. A multidisciplinary registry of patients with autoimmune and immune-mediated diseases with symptomatic COVID-19 from a single center. J Autoimmun (2021) 117:102580. doi: 10.1016/j.jaut.2020.102580
46. Ungaro RC, Brenner EJ, Gearry RB, Kaplan GG, Kissous-Hunt M, Lewis JD, et al. Effect of IBD medications on COVID-19 outcomes: Results from an international registry. Gut (2020) 70(4):725–32. doi: 10.1136/gutjnl-2020-322539
47. Brenner EJ, Ungaro RC, Gearry RB, Kaplan GG, Kissous-Hunt M, Lewis JD, et al. Corticosteroids, but not TNF antagonists, are associated with adverse COVID-19 outcomes in patients with inflammatory bowel diseases: Results from an international registry. Gastroenterology (2020) 159(2):481–491.e3. doi: 10.1053/j.gastro.2020.05.032
48. Agrawal M, Zhang X, Brenner EJ, Ungaro RC, Kappelman MD, Colombel J-F. The impact of vedolizumab on COVID-19 outcomes among adult IBD patients in the SECURE-IBD registry. J Crohn’s Colitis (2021) 15(11):1877–84. doi: 10.1093/ecco-jcc/jjab071
49. Agrawal M, Brenner EJ, Zhang X, Modesto I, Woolcott J, Ungaro RC, et al. Characteristics and outcomes of IBD patients with COVID-19 on tofacitinib therapy in the SECURE-IBD registry. Inflammation Bowel Dis (2021) 27(4):585–9. doi: 10.1093/ibd/izaa303
50. Izadi Z, Brenner EJ, Mahil SK, Dand N, Yiu ZZN, Yates M, et al. Association between tumor necrosis factor inhibitors and the risk of hospitalization or death among patients with immune-mediated inflammatory disease and COVID-19. JAMA Netw Open (2021) 4(10):e2129639. doi: 10.1001/jamanetworkopen.2021.29639
51. Sparks JA, Wallace ZS, Seet AM, Gianfrancesco MA, Izadi Z, Hyrich KL, et al. Associations of baseline use of biologic or targeted synthetic DMARDs with COVID-19 severity in rheumatoid arthritis: Results from the COVID-19 global rheumatology alliance physician registry. Ann Rheum Dis (2021) 80(9):1137–46. doi: 10.1136/annrheumdis-2021-220418
52. Galli C, Daghfal D, Averhoff F. Antibody testing for SARS-CoV-2 infection, quantitative determination, response to vaccines and viralvariability. (2021), Available at: https://cdn.pepperapps.io/diagnostics-cms/public/60dcbed551c1ff090981ed95?signature=eyJhbGciOiJkaXIiLCJlbmMiOiJBMTI4Q0JDLUhTMjU2In0..9nFGX43vdCD-Qd2XE-NzdA.e5mgnWdULSy2PGkSwfQ10kEG1UQzLUxIzkdUvU7F1xv06WNo-c47joEl46OgfiQdEoako-TvRl4CwkLYtVIVYRR7v2jcqnkBx9SFQIzw-nqvFqHkx_WlydBAcI4ZA_wEKCPydLqBtvFu7APi9pVFVDt-WE7028r1nMWpvAe5CiYb2tzNgCGlvM09-oxpfdsY.vWBKl-boWE1UxgZgSc1K6Q.
Keywords: SARS-CoV-2, COVID19, IMID, biologic, immunomodulator, real-world data
Citation: Geldof J, Truyens M, Sabino J, Ferrante M, Lambert J, Lapeere H, Hillary T, Van Laethem A, de Vlam K, Verschueren P, Padalko E, Lobaton T and Vermeire S (2023) SARS-CoV-2 infection and COVID19 vaccination across eight immune-mediated inflammatory disorders: A prospective, real-life Belgian cohort study – the BELCOMID study. Front. Immunol. 14:1126351. doi: 10.3389/fimmu.2023.1126351
Received: 17 December 2022; Accepted: 20 February 2023;
Published: 01 March 2023.
Edited by:
Donata Medaglini, University of Siena, ItalyReviewed by:
Elena Pettini, University of Siena, ItalyPatrick Michael Reeves, Massachusetts General Hospital and Harvard Medical School, United States
Copyright © 2023 Geldof, Truyens, Sabino, Ferrante, Lambert, Lapeere, Hillary, Van Laethem, de Vlam, Verschueren, Padalko, Lobaton and Vermeire. This is an open-access article distributed under the terms of the Creative Commons Attribution License (CC BY). The use, distribution or reproduction in other forums is permitted, provided the original author(s) and the copyright owner(s) are credited and that the original publication in this journal is cited, in accordance with accepted academic practice. No use, distribution or reproduction is permitted which does not comply with these terms.
*Correspondence: Jeroen Geldof, SmVyb2VuLmdlbGRvZkB1emdlbnQuYmU=