- 1Translational and Clinical Research Institute, Newcastle University, Newcastle upon Tyne, United Kingdom
- 2Northern Centre for Cancer Care, The Newcastle upon Tyne Hospitals NHS Foundation Trust, Newcastle upon Tyne, United Kingdom
- 3Department of Gastroenterology, Newcastle upon Tyne Hospitals NHS Foundation Trust, Newcastle upon Tyne, United Kingdom
- 4Department of Dermatology, Newcastle upon Tyne Hospitals NHS Foundation Trust, Newcastle upon Tyne, United Kingdom
- 5Directorate of Musculoskeletal Services, The Newcastle upon Tyne Hospitals NHS Foundation Trust, Newcastle upon Tyne, United Kingdom
Checkpoint inhibitors (CPIs) are monoclonal antibodies which, by disrupting interactions of immune checkpoint molecules with their ligands, block regulatory immune signals otherwise exploited by cancers. Despite revolutionary clinical benefits, CPI use is associated with an array of immune-related adverse events (irAEs) that mirror spontaneous autoreactivity. Severe irAEs necessitate pausing or stopping of CPI therapy and use of corticosteroids and/or other immunomodulatory interventions. Despite increasingly widespread CPI use, irAE pathobiology remains poorly understood; its elucidation may point to targeted mitigation strategies and uncover predictive biomarkers for irAE onset in patients, whilst casting new light on mechanisms of spontaneous immune-mediated disease. This review focuses on common CPI-induced irAEs of the gut, skin and synovial joints, and how these compare to immune-mediated diseases such as ulcerative colitis, vitiligo and inflammatory arthritis. We review current understanding of the immunological changes reported following CPI therapy at the level of peripheral blood and tissue. Many studies highlight dysregulation of cytokines in irAE-affected tissue, particularly IFNγ and TNF. IrAE-affected tissues are also predominantly infiltrated by T-cells, with low B-cell infiltration. Whilst there is variability between studies, patients treated with anti-programmed cell death-1 (PD-1)/PDL-1 therapies seem to exhibit CD8+ T-cell dominance, with CD4+ T-cells dominating in those treated with anti-cytotoxic T-lymphocyte-associated protein 4 (CTLA-4) monotherapy. Interestingly, CD8+CXCR3+ T-cells have been reported to be elevated in gastrointestinal, dermatological and musculoskeletal -irAE affected tissues. These findings may highlight potential opportunities for therapeutic development or re-deployment of existing therapies to prevent and/or improve the outcome of irAEs.
1 Introduction
In health, checkpoint molecules, including cytotoxic T-lymphocyte-associated protein 4 (CTLA-4) and programmed cell death-1 (PD-1), become up-regulated following immune activation. Their ligation attenuates immune responses, mitigating unwanted or excessive host tissue damage. Cancers exploit these pathways by upregulating checkpoint molecule expression (1) to promote tumour tolerance. Checkpoint inhibitors (CPI) monoclonal antibodies are a class of drugs that block interactions between checkpoint proteins and their ligands. CPIs have transformed outcomes of many cancers (2) but cause immune-related adverse events (irAEs) of varying severity in up to 95% of patients on combination therapy (3). IrAEs can affect multiple organ systems, including but not limited to the gastrointestinal (GI) tract, skin and musculoskeletal structures; in clinical presentation they often mirror “spontaneous” immune-mediated pathologies of affected tissues. Whilst each irAE type has been linked to improved cancer survival (4–9), the events themselves are often debilitating and rarely life-threatening. IrAE severity is graded using the Common Terminology Criteria for Adverse Events (CTCAE) (10), and current clinical guidelines recommend pausing or stopping CPI therapy for ≥ grade 3 toxicity (3, 11–13). High dose corticosteroids are commonly administered in this setting, but concern about adverse cancer outcomes as a consequence of non-specific immunosuppression persists (14–16). Indeed, prompt and more targeted immunomodulation with biologic drugs may be used to combat irAEs whilst limiting corticosteroid use, but long-term safety data in controlled studies are awaited.
Although generally considered to be driven by adaptive immune mechanisms that recapitulate intended consequences of checkpoint inhibition, the precise pathobiology of irAEs remains far from understood. With increasing CPI use (17), the monitoring and treatment of their impacts represents a growing healthcare burden. Identifying robust biomarkers for predicting irAE occurrence in CPI recipients – including their tissue-specificity or responsiveness to targeted intervention(s) – remains a pressing unmet need. Insights gained may furthermore advance understanding of spontaneous immune-mediated disease mechanisms, with far-reaching benefits.
2 Gastrointestinal irAEs
2.1 Presentation
The commonest GI-irAE symptom is diarrhoea with a median time to onset of 5-8 weeks (18). Potential risk factors, including prior genetic susceptibility and the role of microbial epitopes, remain the subject of investigation. Endoscopy or cross-sectional imaging may demonstrate colitis and/or enteritis which, in more severe cases, mimics the clinical phenotype of ulcerative colitis (UC) (18). The likelihood of these complications and their severity varies with CPI regimen used (Table 1), with CTLA-4 recipients experiencing GI toxicity more frequently, and to a higher grade, compared with anti-PD-1/PD-L1 monotherapy recipients (19–22, 29). Other GI-irAEs include gastritis, oesophagitis or duodenitis, with rarer manifestations including chronic pancreatitis, hepatitis and coeliac enteropathy (18, 30). CPI enterocolitis is typically managed with oral or intravenous corticosteroids, escalated where needed to treatment with biologics including the anti-TNF monoclonal antibody infliximab or the gut-selective anti-alpha4beta7 integrin monoclonal antibody vedolizumab (18, 30, 31). However, in as many as a third of patients with diarrhoea, no endoscopic inflammation is seen (32, 33). This may be due to more proximal GI inflammation or an increasingly recognised “CPI-microscopic colitis”, where only histological inflammation is demonstrated. In contrast to the UC phenotype, this presentation is more akin to lymphocytic colitis (34), for which sufferers may benefit from orally administered corticosteroids including the beclomethasone pro-drug “clipper” (35, 36).

Table 1 Reported incidences of irAEs from a range of cancer types. Incidences shown in any grade (any), grade 1-2 (G1-2) and grade 3-4 (G3-3).
2.2 Immune dysregulation
Convincing, if complex, association between the gut microbiome and cancer outcomes in CPI recipients has emerged (37, 38). Moreover, case reports have demonstrated faecal microbiota transplant (FMT) can mitigate both anti-PD-1 tumour-resistance and treatment-refractory GI-irAEs (39, 40), and antibiotic therapy is correlated with high-grade GI-irAE incidence (41) – all consistent with a critical immune-mediating role for the microbiome in this setting that has yet to be properly understood (42).
2.2.1 T-cell dysregulation
In addition to differences in GI-irAE frequency observed between CPI treatments (Table 1), distinct immune cell infiltration patterns may further discriminate these pathologies from “spontaneous” inflammatory bowel disease (IBD). Hence, whilst prominent T-cell (but minimal B-cell) infiltration is a general observation in CPI-colitis (43), anti-CLTA-4 therapy has been particularly linked to CD4+ T-cell accumulation and anti-PD-1 with CD8+ T-cell infiltrates (43, 44). The majority of the CD8+CD103+ T-cells in the inflamed gut of CPI-colitis patients were proliferating, whereas active proliferation of CD4+ T-helper 1 (Th1) cells occurred in the peripheral blood (PB), suggesting local and systemic expansion of different T-cell subsets (45). An activated effector population of ICOS+ cells was relatively enriched amongst infiltrating CD4+ T-cells in biopsies from both anti-CTLA-4 and anti-PD1-induced colitis compared with those from IBD patients (44). A landmark study recently used single cell sequencing to compare tissue from melanoma patients who did or did not experience GI-irAEs following various CPI treatments alongside healthy tissue (46). A striking and significant enrichment for CD8+ T-cells exhibiting markers of proliferation (Ki67) and cytotoxicity (granzyme B) was seen in the lamina propria of GI-irAE patients relative to both non-irAE CPI recipients and healthy controls, alongside enhanced IFN response gene expression and a reduction in tissue resident memory (Trm) cells (46). Changes in the CD4+ T-cell compartment in the same tissue included increased frequencies of Th1 cells and increased proportions of both regulatory T-cells (Tregs) and proliferating conventional T-cells, compared with both control groups (46). Anti-CTLA-4 can deplete intra-tumoral Tregs in mice (47) and antibody-dependent cell-mediated cytotoxicity (ADCC)-mediated lysis of Tregs has been proposed as a potential mechanism of irAE development in humans (48). Mirroring other studies, however (44, 49, 50), no diminution, but rather an increase of Treg frequency was seen in GI-irAE compared with non-irAE or healthy gut tissue in the study by Luomo et al. (46), although these comparisons lacked a “spontaneous” immune-mediated colitis (IBD) control group. Conversely, Sasson et al. did find Tregs to represent a significantly lower proportion of infiltrating CD4+ T-cells in GI-irAE tissue compared to that of UC during flare (51), and a functional reduction of Treg frequency in tissue inflammation that is explicitly CPI-induced could reconcile these apparently conflicting observations.
Mucosal‐associated invariant T (MAIT) cells are innate-like, MHC class I-restricted cells enriched at mucosal sites in health, but which have been shown to accumulate in the inflamed bowel of IBD patients (52). Current studies show MAIT cells are not increased in affected tissues in gut-irAEs but those present express higher levels of granzyme B (46, 51).
2.2.2 Monocytes
Monocytic influx in CPI-colitis tissue has also been reported (45). Interestingly (and in contrast to T-cell infiltrates), monocyte enrichment was found in both inflamed and non-inflamed parts of the gut from these patients compared with healthy control tissue.
2.2.3 Cytokines
Mucosal TNF levels are high in patients with anti-CTLA-4 therapy-induced colitis, with lower levels of TNF associated with response to steroids (44). High levels of TNF signalling specifically in myeloid cells in CPI-colitis patients have also been reported (46). Baseline serum IL-17 also correlates with occurrence of colitis/diarrhoea in CPI recipients receiving anti-CTLA-4 (53) and was elevated in CPI-colitis patients compared to CPI patients who did not have an irAE (54). IL-17 levels in CPI-colitis patients reduced to similar levels to non-irAE patients upon resolution of clinical symptoms. Differences in gene expression of chemokines and their receptors have also been reported in CPI-colitis patients, with higher numbers of T-cells having increased expression of CXCR3 and CXCR6 (46).
3 Dermatological irAEs
3.1 Presentation
Dermatological toxicities from CPIs have a wide range of clinical presentations including inflammatory eruptions (drug associated maculopapular exanthem, lichenoid reactions, eczematous reactions, psoriasiform reactions and cutaneous sarcoid), vitiligo-like depigmentation rash (VLDR) and life threatening toxic epidermal necrolysis (55–58). Similar to GI-irAEs, dermatological toxicities are commoner in patients on combination CPI therapy, compared to anti-CTLA-4 or anti-PD-1 monotherapy, although grade 3-4 toxicities are rare (Table 1) (3, 23–26). Despite dermatological-irAEs being more common with CPI regimens that include anti-CTLA-4, some such as VLDR are particularly associated with anti-PD-1/PD-L1 inhibitors (59). Dermatological-irAEs can differ between cancer types, with VLDR seen more frequently in melanoma patients compared with other malignancies, where it has been associated with favourable prognostic outcomes (60–63).
3.2 Immune dysregulation
The frequently observed co-occurrence in immune-mediated skin diseases with those of the GI tract generally, coupled with recently reported links between gut dysbiosis and the development of irAEs (64) (including those of the skin), fuel interest in the concept that a “gut-skin axis” (GSA) (65) may amplify skin pathology in CPI recipients. Immune cell infiltration has been described in case reports of patients with a wide range of dermatological-irAEs including maculopapular eruptions (66), lichenoid reactions (67–70), VLDR (71) and autoimmune skin blistering conditions (bullous pemphigoid) (72, 73)
3.2.1 T-cell dysregulation
In melanoma patients with CPI-induced maculopapular eruptions a preponderance of CD4+ over CD8+ T-cells in cell infiltrates is described, with few B-cells present (66). In CPI-lichenoid reactions, infiltrating T-cells in the epidermis were predominantly CD4+, whilst CD8+ T-cells were located intradermally (67–69). One study of 5 cases of cutaneous lichenoid reactions found that, whilst the dermatopathology bore strong similarity to non-CPI lichenoid lesions, they were distinguished by increased CD163+ histocytes (67). Comparing RNA expression profiles of skin biopsies from anti-PD-1 recipients affected with mainly lichenoid-type irAEs to those derived from a range of non-CPI drug-induced toxic skin rashes, maximal overlap with Stevens-Johnsons syndrome (SJS) and toxic epidermal necrosis (TEN) was observed (70). This transcriptional profile included increased expression of 18 genes including GZMB, CXCL9 and CXCL10, seemingly linking PD-1 blockade with activation of cytotoxic T-cell responses in affected tissues. SJS/TEN reactions from CPI therapy can also occur but are extremely rare and it is thought that CD8+ T cells may play a role in the pathogenesis of SJS/TEN in CPI recipients (74–76).
In VDLR, activated CD8+CXCR3+ T-cells are observed in the vitiligo-like infiltrate of anti-PD-1 recipients. CD8+CXCR3+ T-cells may undergo clonal expansion in the periphery followed by migration into skin in dermatological-irAEs (71). CD8+ T-cells from CPI-induced vitiligo differed from healthy controls and spontaneous vitiligo in their abundant production of IFNγ and TNF, and higher circulating levels of the CXCR3 ligand CXCL10 were detected in the serum of these patients, potentially suggesting distinct mechanisms of cytotoxic cell infiltration and damage.
In summary, whilst activated (and, in particular, cytotoxic) T-cells are a dominant feature of dermatological-irAEs generally, with limited skin biopsy data, the diverse phenotypes observed remain largely unexplained. Future work, including comparative evaluations of tissue from larger, well-characterised cohorts using single cell genomics to gain more granular insights, could yet transform understanding of drug-induced and idiopathic immune-mediated skin disease alike.
4 Musculoskeletal irAEs
4.1 Presentation
The reported incidence of musculoskeletal-irAEs is about 6%, varying between 3.5 and 13.3% (Table 1) (3, 23, 27, 28). In the trial setting, CTCAE grade 1-2 adverse events have not always been reported, however, so these figures may still under-represent the overall morbidity burden. In cohorts/case series of such individuals, inflammatory arthritis manifests in over half (77, 78), and may be more likely to be the first irAE presentation in recipients of anti-PD-1/PD-L1 monotherapy (79). We here focus on musculoskeletal-irAEs that cause synovial inflammation, but polymyalgia rheumatica (PMR) syndromes are also common and there is frequently clinical overlap between the presentations (80). Patterns of joint involvement and autoantibody status at presentation are diverse but somewhat divergent from standard rheumatology practice. For example, large joint oligo- and monoarthritis (often involving the knees) seems at least as typical as small joint involvement, with features of seronegative disease including florid tenosynovitis and remitting seronegative symmetric synovitis with pitting oedema (RS3PE) being well-described.
4.2 Immune dysregulation
Unlike rheumatoid arthritis (RA), the majority of CPI-induced inflammatory arthritis (CPI-IA) patients are seronegative for circulating anti-citrullinated and/or rheumatoid factor (RF) autoantibodies (78, 80, 81).
4.2.1 T-cell dysregulation
A number of lines of evidence point to dysregulated T-cell homeostasis as a driver of CPI-IA (82–86). Kim et al. recently used a single cell sequencing approach coupled with multi-parameter flow cytometry to compare PB and synovial fluid (SF) cellular compartments of CPI recipients who developed irAEs (82). A wide range of cell subsets were relatively enriched in SF, including proliferating T-cells, an activated, CXCR3hi effector CD8 T-cell subset, Tregs and a Th1 like subset (in anti-PD1 recipients), all recalling observations in GI-irAE tissue described earlier. Enriched IL-17-expressing subsets of both CD4+ and cytotoxic CD8+ T-cells (Th17 and Tc17 cells, respectively) were also observed in this compartment amongst combination CPI recipients, albeit not reaching statistical significance for Tc17s. The latter is nonetheless of interest given recent descriptions of Tc17 expansion in SF of psoriatic arthritis (PsA) patients – another autoantibody seronegative subset of spontaneous inflammatory arthritis, raising the possibility of shared disease pathways between these conditions (87). Two additional cell subsets, MAIT γδ T-cells and a CD38hi CD127- CD8+ effector subset, were relatively enriched in SF – a finding that has since been replicated in a separate study showing these subsets underwent clonal proliferation in the joint and distinguished CPI-IA SF from that of both RA and PsA control patients (86). Diversification of the T-cell repertoire as a consequence of anti-CTLA-4 treatment was previously noted, with more extensive TCR Vβ CDR3 clonotype expansion linked to an increased likelihood of immune-related adverse events in general (88). Sharing of expanded CD8+ clones with CX3CR1hi effector and CXCR3hiCXCR6lo effector phenotypes in the SF and PB of CPI-IA patients, respectively, suggests drug-induced, systemic expansion of CD8 clusters in PB may precede their migration to the joint at irAE onset. Another study also points towards clonal expansion of specific CD8+ T-cells, with some TCR clones being shared across patients (89). Potentially mirroring observations in both “spontaneous” RA and GI-irAEs, current evidence suggests Tregs are not depleted in joints of patients who develop a joint-irAE (82, 85). They are enriched in SF of joint-irAE patients where they are apparently more suppressive than those from PB of both joint-irAE and non-irAE CPI recipients (82).
4.2.2 B-cell dysregulation
B-cells play an undisputed role in the pathogenesis of seropositive RA but, as in the case of GI-irAEs, minimal B-cell infiltration has been reported in the joints of CPI-IA patients, with one report of no detectable B-cells in SF at all (83). However, enhanced systemic B-cell reactivity during CPI treatment amongst melanoma patients who develop irAEs has been observed, with expansion and clonal diversification of a normally rare, CD21lo B-cell population reminiscent of age-associated B-cells whose potential role in RA pathogenesis is under investigation (90). Further work may yet shed light onto their potential relevance in CPI-IA development.
It is important to note that the majority of data arising from joints of CPI-IA patients described here describes characteristics of SF, and not synovial tissue. Complimentary and, arguably, more relevant data from a pathobiological perspective is expected to emerge from the study of synovial tissue biopsy samples in the near future (86).
4.2.3 Cytokines
Several cytokines have been reported to be dysregulated in patients with joint-irAEs. TNF has been reported to be highly expressed in joints of joint-irAE patients (83). Meanwhile IL-17 was shown to be elevated in a recurrent case of pseudogout at each flare (85). Other cytokines such as IL-6, IL-1β and IFNγ have also been seen to be increased in SF of joint-irAE patients (82).
5 Conclusion and therapeutic considerations
IrAEs are common, and increasing use of CPIs for an ever-widening range of tumour types and disease stages will inevitably render them more so. Based on disparate studies employing conventional histological techniques, and limited single cell sequencing of disaggregated tissues, certain commonalities and differences between immune dysregulation in dermatological, gastrointestinal and musculoskeletal irAEs are apparent (Figure 1). For example, prominent CXCR3+ T-cells (cytotoxic and/or Th1) infiltration appears to be a general feature of affected tissues. Peripheral expansion of T-cells followed by migration into tissues may underpin this facet of irAE development (46, 71, 82) such that enumerating specific PB T-cell subsets could conceivably aid in early irAE detection – a critical consideration for the future. However, as with most putative predictive toxicity biomarkers reported to date, large studies will be needed to validate preliminary observations (91). Mechanisms by which toxicities display varying tissue predilections between individuals remain largely unknown, with genetic variation, the host microbiome, pre-existing immune dysregulation and stromal factors potentially contributing. Beyond irAE development in general, unravelling this complexity could ultimately yield biomarkers that predict tissue specificity and severity. With underpinning data only now beginning to accumulate, we expect this picture to evolve rapidly over the coming years in a fast-moving field that promises important implications for irAE mitigation strategies to support sustained anti-cancer treatment for affected CPI recipients.
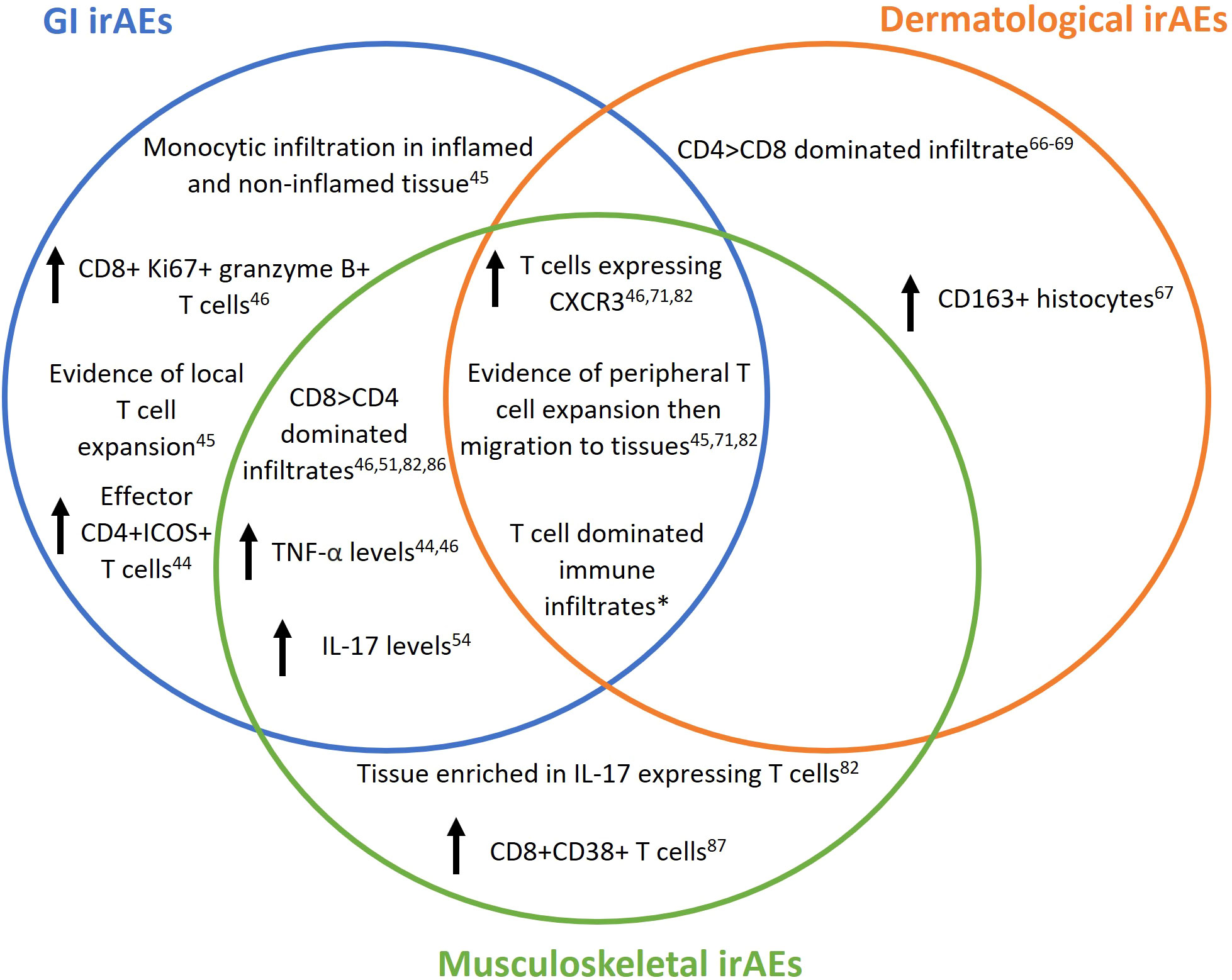
Figure 1 Reported immunological changes in patients on checkpoint inhibitor (CPI) therapy developing immune related adverse events (irAEs). IrAEs are common with CPI use and can affect multiple organs systems including but not limited to gut, skin and joints. Multiple risk factors may contribute to irAE development and/or their localisation to the represented tissues, including genetic variation, microbiome, pre-existing immune-mediated disease and stromal characteristics. Venn diagram depicts immune changes reported in human gut, skin and joint tissue following CPI therapy reported so far in the literature and covered by this review. *All research articles containing peripheral blood or tissue immune characterisation covered in this article.
Currently, and in keeping with published management guidelines, glucocorticoids remain the first-line therapeutic intervention for moderate to severe irAEs affecting the gut, skin and synovial joint, with doses in excess of 1mg/kg prednisolone advocated in some circumstances (3). The impact of this approach on tumour responses remains unclear: most studies are retrospective such that known association between irAE occurrence and improved tumour outcome may mask any blunting of CPI efficacy as a result of corticosteroid use. On the other hand, whilst the need for irAE treatment with immunosuppression within two months of CPI initiation has been suggested to be deleterious for cancer outcome (92), the confounding impact of premature CPI cessation in this context also remains unclear. The same important caveat applies to data suggesting escalation of immunosuppression, to include targeted interventions including biologic drugs on top of glucocorticoids (93). It should also be remembered that high-dose glucocorticoid use is itself associated with considerable morbidity (94–96). Controlled prospective trials coupled with experimental medicine approaches are needed to properly evaluate these issues, their design informed by emerging pathophysiological insight, should herald more personalised approaches to irAE management (97). For example, clinical trial is currently underway assessing the value of anti-TNFs alongside CPI therapy (NCT03293784).
High levels of proinflammatory cytokines including TNF in irAE-affected gut and synovial tissue suggest strong prima facie rationale for anti-TNF as the most widely deployed targeted therapy for these toxicities (80, 98). The role of this cytokine in tumour biology remains controversial, however (99–101), and some concern about the impact of the approach on cancer outcomes persists. For example, enhanced Treg activity reported with anti-TNF use in RA – of potential concern in the context of a tumour microenvironment – was specifically linked to monoclonal antibody use rather than that of a receptor fusion protein (102), emphasising that the mechanism of action of targeted therapy may be as important as the molecular targets themselves in this setting. Similar considerations could apply in respect of IL-6 targeting, another increasingly popular approach (103). B-cell depletion with the anti-CD20 monoclonal antibody rituximab has shown some promise for dermatological irAEs, with no apparent adverse impact on survival (104), and observations in inflammatory arthritis reviewed above arguably support interest in the approach. This should, however, be balanced against the general dearth of infiltrating B-cells in irAE tissues described herein, together with very recent data indicating that regulatory B-cells (Bregs), which would also be targeted by an anti-CD20 strategy, may play a critical role in the prevention of irAEs (105). A deficiency of this cell type in the circulation of CPI recipients was indeed predictive of subsequent toxicity in this study.
Finally, targeting the microbiome of CPI recipients continues to garner interest as a potential route to a more favourable balance between tumour response and irAE development. The use of probiotics as microbial modulators may offer a more streamlined strategy than FMT – which is itself not without risk – but whether the absence of microbial metabolites from such interventions limits efficacy remains to be explored. Multiple early phase trials investigating probiotic supplements alongside CPI therapies are underway, with results set to be available as early as 2024 (106).
In summary, a recent acceleration in studies evaluating irAE target tissue amongst CPI recipients is set to continue, together with data from alternative target tissues not covered in this review, will doubtless inform management strategies of the future. Combined with prospective data from ongoing and planned clinical trials and experimental medicine approaches, these endeavours should yield improved survival outcomes and quality of life for cancer patients.
Author contributions
All authors contributed to the article and approved the submitted version.
Funding
KCW’s work is supported by a studentship funded by the JGW Patterson Foundation; AG’s work is funded by a Clinical Training Fellowship supported by Cancer Research UK, and Newcastle researchers receive infrastructural support from the NIHR Newcastle Biomedical Research Centre; the views expressed are those of the author(s) and not necessarily those of the NIHR or the Department of Health and Social Care.
Conflict of interest
The authors declare that the research was conducted in the absence of any commercial or financial relationships that could be construed as a potential conflict of interest.
Publisher’s note
All claims expressed in this article are solely those of the authors and do not necessarily represent those of their affiliated organizations, or those of the publisher, the editors and the reviewers. Any product that may be evaluated in this article, or claim that may be made by its manufacturer, is not guaranteed or endorsed by the publisher.
References
1. Ohaegbulam KC, Assal A, Lazar-Molnar E, Yao Y, Zang X. Human cancer immunotherapy with antibodies to the pd-1 and pd-L1 pathway. Trends Mol Med (2015) 21(1):24–33. doi: 10.1016/j.molmed.2014.10.009
2. Bagchi S, Yuan R, Engleman EG. Immune checkpoint inhibitors for the treatment of cancer: Clinical impact and mechanisms of response and resistance. Annu Rev Pathol (2021) 16:223–49. doi: 10.1146/annurev-pathol-042020-042741
3. Haanen J, Carbonnel F, Robert C, Kerr KM, Peters S, Larkin J, et al. Management of toxicities from immunotherapy: Esmo clinical practice guidelines for diagnosis, treatment and follow-up. Ann Oncol (2017) 28(suppl_4):iv119–iv42. doi: 10.1093/annonc/mdx225
4. Wang Y, Abu-Sbeih H, Mao E, Ali N, Ali FS, Qiao W, et al. Immune-checkpoint inhibitor-induced diarrhea and colitis in patients with advanced malignancies: Retrospective review at md Anderson. J ImmunoTher Cancer (2018) 6(1):37. doi: 10.1186/s40425-018-0346-6
5. Abu-Sbeih H, Ali FS, Qiao W, Lu Y, Patel S, Diab A, et al. Immune checkpoint inhibitor-induced colitis as a predictor of survival in metastatic melanoma. Cancer Immunol Immunother (2019) 68(4):553–61. doi: 10.1007/s00262-019-02303-1
6. Weingarden AR, Gubatan J, Singh S, Balabanis TC, Patel A, Sharma A, et al. Immune checkpoint inhibitor-mediated colitis is associated with cancer overall survival. World J Gastroenterol (2022) 28(39):5750–63. doi: 10.3748/wjg.v28.i39.5750
7. Gulati N, Donnelly D, Qian Y, Moran U, Johannet P, Zhong J, et al. Revisiting the association between skin toxicity and better response in advanced cancer patients treated with immune checkpoint inhibitors. J Trans Med (2020) 18(1):430. doi: 10.1186/s12967-020-02612-5
8. Maeda A, Takase-Minegishi K, Kirino Y, Hamada N, Kunishita Y, Yoshimi R, et al. Immune checkpoint inhibitor–induced arthralgia is tightly associated with improved overall survival in cancer patients. Rheumatology (2022) keac519. doi: 10.1093/rheumatology/keac519
9. Liew DFL, Leung JLY, Liu B, Cebon J, Frauman AG, Buchanan RRC. Association of good oncological response to therapy with the development of rheumatic immune-related adverse events following pd-1 inhibitor therapy. Int J Rheum Dis (2019) 22(2):297–302. doi: 10.1111/1756-185X.13444
10. National Cancer Institute. Common terminology criteria for adverse events (2021). Available at: https://ctep.cancer.gov/protocoldevelopment/electronic_applications/ctc.htm#ctc_60.
11. Schneider BJ, Naidoo J, Santomasso BD, Lacchetti C, Adkins S, Anadkat M, et al. Management of immune-related adverse events in patients treated with immune checkpoint inhibitor therapy: Asco guideline update. J Clin Oncol (2021) 39(36):4073–126. doi: 10.1200/JCO.21.01440
12. Brahmer JR, Abu-Sbeih H, Ascierto PA, Brufsky J, Cappelli LC, Cortazar FB, et al. Society for immunotherapy of cancer (Sitc) clinical practice guideline on immune checkpoint inhibitor-related adverse events. J ImmunoTher Cancer (2021) 9(6):e002435. doi: 10.1136/jitc-2021-002435
13. Thompson JA, Schneider BJ, Brahmer J, Andrews S, Armand P, Bhatia S, et al. Nccn guidelines insights: Management of immunotherapy-related toxicities, version 1.2020. J Natl Compr Canc Netw (2020) 18(3):230–41. doi: 10.6004/jnccn.2020.0012
14. Schmitt AM, Spain L, Larkin J. Hitting the sweet spot: Optimal use of corticosteroids for immune checkpoint inhibitor side-effects. Lancet Oncol (2022) 23(9):1123–4. doi: 10.1016/S1470-2045(22)00296-0
15. Faje AT, Lawrence D, Flaherty K, Freedman C, Fadden R, Rubin K, et al. High-dose glucocorticoids for the treatment of ipilimumab-induced hypophysitis is associated with reduced survival in patients with melanoma. Cancer (2018) 124(18):3706–14. doi: 10.1002/cncr.31629
16. Scott SC, Pennell NA. Early use of systemic corticosteroids in patients with advanced nsclc treated with nivolumab. J Thorac Oncol (2018) 13(11):1771–5. doi: 10.1016/j.jtho.2018.06.004
17. National Health Service. National cancer drugs fund list (2022). Available at: https://www.england.nhs.uk/wp-content/uploads/2017/04/national-cdf-list-v1.236.pdf.
18. Powell N, Ibraheim H, Raine T, Speight RA, Papa S, Brain O, et al. British Society of gastroenterology endorsed guidance for the management of immune checkpoint inhibitor-induced enterocolitis. Lancet Gastroenterol Hepatol (2020) 5(7):679–97. doi: 10.1016/S2468-1253(20)30014-5
19. Hodi FS, Chiarion-Sileni V, Gonzalez R, Grob JJ, Rutkowski P, Cowey CL, et al. Nivolumab plus ipilimumab or nivolumab alone versus ipilimumab alone in advanced melanoma (Checkmate 067): 4-year outcomes of a multicentre, randomised, phase 3 trial. Lancet Oncol (2018) 19(11):1480–92. doi: 10.1016/S1470-2045(18)30700-9
20. Hellmann MD, Ciuleanu TE, Pluzanski A, Lee JS, Otterson GA, Audigier-Valette C, et al. Nivolumab plus ipilimumab in lung cancer with a high tumor mutational burden. N Engl J Med (2018) 378(22):2093–104. doi: 10.1056/NEJMoa1801946
21. Ascierto PA, Del Vecchio M, Robert C, Mackiewicz A, Chiarion-Sileni V, Arance A, et al. Ipilimumab 10 Mg/Kg versus ipilimumab 3 Mg/Kg in patients with unresectable or metastatic melanoma: A randomised, double-blind, multicentre, phase 3 trial. Lancet Oncol (2017) 18(5):611–22. doi: 10.1016/S1470-2045(17)30231-0
22. Borghaei H, Paz-Ares L, Horn L, Spigel DR, Steins M, Ready NE, et al. Nivolumab versus docetaxel in advanced nonsquamous non-Small-Cell lung cancer. N Engl J Med (2015) 373(17):1627–39. doi: 10.1056/NEJMoa1507643
23. Larkin J, Chiarion-Sileni V, Gonzalez R, Grob JJ, Cowey CL, Lao CD, et al. Combined nivolumab and ipilimumab or monotherapy in untreated melanoma. N Engl J Med (2015) 373(1):23–34. doi: 10.1056/NEJMoa1504030
24. Ferris RL, Blumenschein G Jr., Fayette J, Guigay J, Colevas AD, Licitra L, et al. Nivolumab for recurrent squamous-cell carcinoma of the head and neck. N Engl J Med (2016) 375(19):1856–67. doi: 10.1056/NEJMoa1602252
25. Postow MA, Chesney J, Pavlick AC, Robert C, Grossmann K, McDermott D, et al. Nivolumab and ipilimumab versus ipilimumab in untreated melanoma. N Engl J Med (2015) 372(21):2006–17. doi: 10.1056/NEJMoa1414428
26. Hodi FS, O'Day SJ, McDermott DF, Weber RW, Sosman JA, Haanen JB, et al. Improved survival with ipilimumab in patients with metastatic melanoma. N Engl J Med (2010) 363(8):711–23. doi: 10.1056/NEJMoa1003466
27. Brahmer JR, Lacchetti C, Thompson JA. Management of immune-related adverse events in patients treated with immune checkpoint inhibitor therapy: American society of clinical oncology clinical practice guideline summary. J Oncol practice/American Soc Clin Oncol (2018) 14(4):247–9. doi: 10.1200/JOP.18.00005
28. Spain L, Diem S, Larkin J. Management of toxicities of immune checkpoint inhibitors. Cancer Treat Rev (2016) 44:51–60. doi: 10.1016/j.ctrv.2016.02.001
29. Yamada K, Sawada T, Nakamura M, Yamamura T, Maeda K, Ishikawa E, et al. Clinical characteristics of gastrointestinal immune-related adverse events of immune checkpoint inhibitors and their association with survival. World J Gastroenterol (2021) 27(41):7190–206. doi: 10.3748/wjg.v27.i41.7190
30. Dougan M, Wang Y, Rubio-Tapia A, Lim JK. Aga clinical practice update on diagnosis and management of immune checkpoint inhibitor colitis and hepatitis: Expert review. Gastroenterology (2021) 160(4):1384–93. doi: 10.1053/j.gastro.2020.08.063
31. Alexander JL, Ibraheim H, Sheth B, Little J, Khan MS, Richards C, et al. Clinical outcomes of patients with corticosteroid refractory immune checkpoint inhibitor-induced enterocolitis treated with infliximab. J Immunother Cancer (2021) 9(7):e002742. doi: 10.1136/jitc-2021-002742
32. Abu-Sbeih H, Ali FS, Luo W, Qiao W, Raju GS, Wang Y. Importance of endoscopic and histological evaluation in the management of immune checkpoint inhibitor-induced colitis. J Immunother Cancer (2018) 6(1):95. doi: 10.1186/s40425-018-0411-1
33. Geukes Foppen MH, Rozeman EA, van Wilpe S, Postma C, Snaebjornsson P, van Thienen JV, et al. Immune checkpoint inhibition-related colitis: Symptoms, endoscopic features, histology and response to management. ESMO Open (2018) 3(1):e000278. doi: 10.1136/esmoopen-2017-000278
34. Choi K, Abu-Sbeih H, Samdani R, Nogueras Gonzalez G, Raju GS, Richards DM, et al. Can immune checkpoint inhibitors induce microscopic colitis or a brand new entity? Inflammation Bowel Dis (2019) 25(2):385–93. doi: 10.1093/ibd/izy240
35. Hughes MS, Molina GE, Chen ST, Zheng H, Deshpande V, Fadden R, et al. Budesonide treatment for microscopic colitis from immune checkpoint inhibitors. J Immunother Cancer (2019) 7(1):292. doi: 10.1186/s40425-019-0756-0
36. Alexander JL, Ibraheim H, Richards C, Shum B, Pavlidis P, Hunter N, et al. Oral beclomethasone dipropionate is an effective treatment for immune checkpoint inhibitor induced colitis. J ImmunoTher Cancer (2022) 10(9):e005490. doi: 10.1136/jitc-2022-005490
37. Lee KA, Thomas AM, Bolte LA, Bjork JR, de Ruijter LK, Armanini F, et al. Cross-cohort gut microbiome associations with immune checkpoint inhibitor response in advanced melanoma. Nat Med (2022) 28(3):535–44. doi: 10.1038/s41591-022-01695-5
38. Gopalakrishnan V, Spencer CN, Nezi L, Reuben A, Andrews MC, Karpinets TV, et al. Gut microbiome modulates response to anti-Pd-1 immunotherapy in melanoma patients. Science (2018) 359(6371):97–103. doi: 10.1126/science.aan4236
39. Davar D, Dzutsev AK, McCulloch JA, Rodrigues RR, Chauvin JM, Morrison RM, et al. Fecal microbiota transplant overcomes resistance to anti-Pd-1 therapy in melanoma patients. Science (2021) 371(6529):595–602. doi: 10.1126/science.abf3363
40. Chen M, Liu M, Li C, Peng S, Li Y, Xu X, et al. Fecal microbiota transplantation effectively cures a patient with severe bleeding immune checkpoint inhibitor-associated colitis and a short review. Front Oncol (2022) 12:913217. doi: 10.3389/fonc.2022.913217
41. Mohiuddin JJ, Chu B, Facciabene A, Poirier K, Wang X, Doucette A, et al. Association of antibiotic exposure with survival and toxicity in patients with melanoma receiving immunotherapy. J Natl Cancer Inst (2021) 113(2):162–70. doi: 10.1093/jnci/djaa057
42. Zhou G, Zhang N, Meng K, Pan F. Interaction between gut microbiota and immune checkpoint inhibitor-related colitis. Front Immunol (2022) 13:1001623. doi: 10.3389/fimmu.2022.1001623
43. Bamias G, Delladetsima I, Perdiki M, Siakavellas SI, Goukos D, Papatheodoridis GV, et al. Immunological characteristics of colitis associated with anti-Ctla-4 antibody therapy. Cancer Invest (2017) 35(7):443–55. doi: 10.1080/07357907.2017.1324032
44. Coutzac C, Adam J, Soularue E, Collins M, Racine A, Mussini C, et al. Colon immune-related adverse events: Anti-Ctla-4 and anti-Pd-1 blockade induce distinct immunopathological entities. J Crohns Colitis (2017) 11(10):1238–46. doi: 10.1093/ecco-jcc/jjx081
45. Nahar KJ, Marsh-Wakefield F, Rawson RV, Gide TN, Ferguson AL, Allen RO, et al. Distinct pre-treatment innate immune landscape and post-treatment T cell responses underlie immunotherapy-induced colitis. JCI Insight (2022) 7:e157839. doi: 10.1172/jci.insight.157839
46. Luoma AM, Suo S, Williams HL, Sharova T, Sullivan K, Manos M, et al. Molecular pathways of colon inflammation induced by cancer immunotherapy. Cell (2020) 182(3):655–71 e22. doi: 10.1016/j.cell.2020.06.001
47. Simpson TR, Li F, Montalvo-Ortiz W, Sepulveda MA, Bergerhoff K, Arce F, et al. Fc-dependent depletion of tumor-infiltrating regulatory T cells Co-defines the efficacy of anti-Ctla-4 therapy against melanoma. J Exp Med (2013) 210(9):1695–710. doi: 10.1084/jem.20130579
48. Romano E, Kusio-Kobialka M, Foukas PG, Baumgaertner P, Meyer C, Ballabeni P, et al. Ipilimumab-dependent cell-mediated cytotoxicity of regulatory T cells ex vivo by nonclassical monocytes in melanoma patients. Proc Natl Acad Sci U.S.A. (2015) 112(19):6140–5. doi: 10.1073/pnas.1417320112
49. Sharma A, Subudhi SK, Blando J, Scutti J, Vence L, Wargo J, et al. Anti-Ctla-4 immunotherapy does not deplete Foxp3(+) regulatory T cells (Tregs) in human cancers. Clin Cancer Res (2019) 25(4):1233–8. doi: 10.1158/1078-0432.CCR-18-0762
50. Lord JD, Hackman RC, Moklebust A, Thompson JA, Higano CS, Chielens D, et al. Refractory colitis following anti-Ctla4 antibody therapy: Analysis of mucosal Foxp3+ T cells. Dig Dis Sci (2010) 55(5):1396–405. doi: 10.1007/s10620-009-0839-8
51. Sasson SC, Zaunders JJ, Nahar K, Munier CML, Fairfax BP, Olsson-Brown A, et al. Mucosal-associated invariant T (Mait) cells are activated in the gastrointestinal tissue of patients with combination ipilimumab and nivolumab therapy-related colitis in a pathology distinct from ulcerative colitis. Clin Exp Immunol (2020) 202(3):335–52. doi: 10.1111/cei.13502
52. Haga K, Chiba A, Shibuya T, Osada T, Ishikawa D, Kodani T, et al. Mait cells are activated and accumulated in the inflamed mucosa of ulcerative colitis. J Gastroenterol Hepatol (2016) 31(5):965–72. doi: 10.1111/jgh.13242
53. Tarhini AA, Zahoor H, Lin Y, Malhotra U, Sander C, Butterfield LH, et al. Baseline circulating il-17 predicts toxicity while tgf-Beta1 and il-10 are prognostic of relapse in ipilimumab neoadjuvant therapy of melanoma. J Immunother Cancer (2015) 3:39. doi: 10.1186/s40425-015-0081-1
54. Callahan MK, Yang A, Tandon S, Xu Y, Subudhi SK, Roman RA, et al. Evaluation of serum il-17 levels during ipilimumab therapy: Correlation with colitis. J Clin Oncol (2011) 29(15_suppl):2505–. doi: 10.1200/jco.2011.29.15_suppl.2505
55. Curry JL, Tetzlaff MT, Nagarajan P, Drucker C, Diab A, Hymes SR, et al. Diverse types of dermatologic toxicities from immune checkpoint blockade therapy. J Cutan Pathol (2017) 44(2):158–76. doi: 10.1111/cup.12858
56. Gault A, Anderson AE, Plummer R, Stewart C, Pratt AG, Rajan N. Cutaneous immune-related adverse events in patients with melanoma treated with checkpoint inhibitors. Br J Dermatol (2021) 185(2):263–71. doi: 10.1111/bjd.19750
57. Ellis SR, Vierra AT, Millsop JW, Lacouture ME, Kiuru M. Dermatologic toxicities to immune checkpoint inhibitor therapy: A review of histopathologic features. J Am Acad Dermatol (2020) 83(4):1130–43. doi: 10.1016/j.jaad.2020.04.105
58. Ma B, Anandasabapathy N. Immune checkpoint blockade and skin toxicity pathogenesis. J Invest Dermatol (2022) 142(3 Pt B):951–9. doi: 10.1016/j.jid.2021.06.040
59. Kumar V, Chaudhary N, Garg M, Floudas CS, Soni P, Chandra AB. Corrigendum: Current diagnosis and management of immune related adverse events (Iraes) induced by immune checkpoint inhibitor therapy. Front Pharmacol (2017) 8:311. doi: 10.3389/fphar.2017.00311
60. Hofmann L, Forschner A, Loquai C, Goldinger SM, Zimmer L, Ugurel S, et al. Cutaneous, gastrointestinal, hepatic, endocrine, and renal side-effects of anti-Pd-1 therapy. Eur J Cancer (2016) 60:190–209. doi: 10.1016/j.ejca.2016.02.025
61. Uenami T, Hosono Y, Ishijima M, Kanazu M, Akazawa Y, Yano Y, et al. Vitiligo in a patient with lung adenocarcinoma treated with nivolumab: A case report. Lung Cancer (2017) 109:42–4. doi: 10.1016/j.lungcan.2017.04.019
62. Yun SJ, Oh IJ, Park CK, Kim YC, Kim HB, Kim HK, et al. Vitiligo-like depigmentation after pembrolizumab treatment in patients with non-small cell lung cancer: A case report. Transl Lung Cancer Res (2020) 9(4):1585–90. doi: 10.21037/tlcr-20-386
63. Lolli C, Medri M, Ricci M, Schepisi G, Filograna A, De Giorgi U, et al. Vitiligo-like lesions in a patient treated with nivolumab for renal cell carcinoma. Med (Baltimore) (2018) 97(52):e13810. doi: 10.1097/MD.0000000000013810
64. Williams N, Hoyd R, Wheeler CE, Lynn M, Bibi A, Gray S, et al. The effect of the microbiome on immune checkpoint inhibitor toxicity in patients with melanoma. J Clin Oncol (2022) 40(16_suppl):9568–. doi: 10.1200/JCO.2022.40.16_suppl.9568
65. Sinha S, Lin G, Ferenczi K. The skin microbiome and the gut-skin axis. Clin Dermatol (2021) 39(5):829–39. doi: 10.1016/j.clindermatol.2021.08.021
66. Perret RE, Josselin N, Knol AC, Khammari A, Cassecuel J, Peuvrel L, et al. Histopathological aspects of cutaneous erythematous-papular eruptions induced by immune checkpoint inhibitors for the treatment of metastatic melanoma. Int J Dermatol (2017) 56(5):527–33. doi: 10.1111/ijd.13540
67. Schaberg KB, Novoa RA, Wakelee HA, Kim J, Cheung C, Srinivas S, et al. Immunohistochemical analysis of lichenoid reactions in patients treated with anti-Pd-L1 and anti-Pd-1 therapy. J Cutan Pathol (2016) 43(4):339–46. doi: 10.1111/cup.12666
68. Joseph RW, Cappel M, Goedjen B, Gordon M, Kirsch B, Gilstrap C, et al. Lichenoid dermatitis in three patients with metastatic melanoma treated with anti-Pd-1 therapy. Cancer Immunol Res (2015) 3(1):18–22. doi: 10.1158/2326-6066.CIR-14-0134
69. Shi VJ, Rodic N, Gettinger S, Leventhal JS, Neckman JP, Girardi M, et al. Clinical and histologic features of lichenoid mucocutaneous eruptions due to anti-programmed cell death 1 and anti-programmed cell death ligand 1 immunotherapy. JAMA Dermatol (2016) 152(10):1128–36. doi: 10.1001/jamadermatol.2016.2226
70. Goldinger SM, Stieger P, Meier B, Micaletto S, Contassot E, French LE, et al. Cytotoxic cutaneous adverse drug reactions during anti-Pd-1 therapy. Clin Cancer Res (2016) 22(16):4023–9. doi: 10.1158/1078-0432.CCR-15-2872
71. Larsabal M, Marti A, Jacquemin C, Rambert J, Thiolat D, Dousset L, et al. Vitiligo-like lesions occurring in patients receiving anti-programmed cell death-1 therapies are clinically and biologically distinct from vitiligo. J Am Acad Dermatol (2017) 76(5):863–70. doi: 10.1016/j.jaad.2016.10.044
72. Kwon CW, Land AS, Smoller BR, Scott G, Beck LA, Mercurio MG. Bullous pemphigoid associated with nivolumab, a programmed cell death 1 protein inhibitor. J Eur Acad Dermatol Venereol (2017) 31(8):e349–e50. doi: 10.1111/jdv.14143
73. Naidoo J, Schindler K, Querfeld C, Busam K, Cunningham J, Page DB, et al. Autoimmune bullous skin disorders with immune checkpoint inhibitors targeting pd-1 and pd-L1. Cancer Immunol Res (2016) 4(5):383–9. doi: 10.1158/2326-6066.CIR-15-0123
74. Ito J, Fujimoto D, Nakamura A, Nagano T, Uehara K, Imai Y, et al. Aprepitant for refractory nivolumab-induced pruritus. Lung Cancer (2017) 109:58–61. doi: 10.1016/j.lungcan.2017.04.020
75. Lee O, Masood M, Nutan F. Case series of stevens-Johnson syndrome and toxic epidermal necrolysis with nivolumab and nivolumab/ ipilimumab combination therapy in metastatic melanoma. J Drugs Dermatol (2022) 21(5):529–30. doi: 10.36849/JDD.6559
76. Molina GE, Yu Z, Foreman RK, Reynolds KL, Chen ST. Generalized bullous mucocutaneous eruption mimicking stevens-Johnson syndrome in the setting of immune checkpoint inhibition: A multicenter case series. J Am Acad Dermatol (2020) 83(5):1475–7. doi: 10.1016/j.jaad.2020.03.029
77. Kostine M, Rouxel L, Barnetche T, Veillon R, Martin F, Dutriaux C, et al. Rheumatic disorders associated with immune checkpoint inhibitors in patients with cancer-clinical aspects and relationship with tumour response: A single-centre prospective cohort study. Ann Rheum Dis (2018) 77(3):393–8. doi: 10.1136/annrheumdis-2017-212257
78. Steven NM, Fisher BA. Management of rheumatic complications of immune checkpoint inhibitor therapy - an oncological perspective. Rheumatology (2019) 58(Suppl 7):vii29–39. doi: 10.1093/rheumatology/kez536
79. Cappelli LC, Brahmer JR, Forde PM, Le DT, Lipson EJ, Naidoo J, et al. Clinical presentation of immune checkpoint inhibitor-induced inflammatory arthritis differs by immunotherapy regimen. Semin Arthritis Rheum (2018) 48(3):553–7. doi: 10.1016/j.semarthrit.2018.02.011
80. Kostine M, Finckh A, Bingham CO, Visser K, Leipe J, Schulze-Koops H, et al. Eular points to consider for the diagnosis and management of rheumatic immune-related adverse events due to cancer immunotherapy with checkpoint inhibitors. Ann Rheum Dis (2021) 80(1):36–48. doi: 10.1136/annrheumdis-2020-217139
81. Cappelli LC, Gutierrez AK, Bingham CO 3rd, Shah AA. Rheumatic and musculoskeletal immune-related adverse events due to immune checkpoint inhibitors: A systematic review of the literature. Arthritis Care Res (Hoboken) (2017) 69(11):1751–63. doi: 10.1002/acr.23177
82. Kim ST, Chu Y, Misoi M, Suarez-Almazor ME, Tayar JH, Lu H, et al. Distinct molecular and immune hallmarks of inflammatory arthritis induced by immune checkpoint inhibitors for cancer therapy. Nat Commun (2022) 13(1):1970. doi: 10.1038/s41467-022-29539-3
83. Murray-Brown W, Wilsdon TD, Weedon H, Proudman S, Sukumaran S, Klebe S, et al. Nivolumab-induced synovitis is characterized by florid T cell infiltration and rapid resolution with synovial biopsy-guided therapy. J Immunother Cancer (2020) 8(1):e000281. doi: 10.1136/jitc-2019-000281
84. Medina HA, Eickhoff J, Edison JD. Thinking inside the box: Synovial tissue biopsy in immune checkpoint inhibitor-induced arthritis. J Clin Rheumatol (2021) 27(8S):S537–S40. doi: 10.1097/RHU.0000000000001088
85. Kim ST, Bittar M, Kim HJ, Neelapu SS, Zurita AJ, Nurieva R, et al. Recurrent pseudogout after therapy with immune checkpoint inhibitors: A case report with immunoprofiling of synovial fluid at each flare. J Immunother Cancer (2019) 7(1):126. doi: 10.1186/s40425-019-0597-x
86. Wang R, Singaraju A, Marks KE, Shakib L, Dunlap G, Cunningham-Bussel A, et al. Clonally expanded Cd38hi cytotoxic Cd8 T cells define the T cell infiltrate in checkpoint inhibitor-associated arthritis. bioRxiv (2021), 464961. doi: 10.1101/2021.10.19.464961
87. Steel KJA, Srenathan U, Ridley M, Durham LE, Wu SY, Ryan SE, et al. Polyfunctional, proinflammatory, tissue-resident memory phenotype and function of synovial interleukin-17a+Cd8+ T cells in psoriatic arthritis. Arthritis Rheumatol (2020) 72(3):435–47. doi: 10.1002/art.41156
88. Oh DY, Cham J, Zhang L, Fong G, Kwek SS, Klinger M, et al. Immune toxicities elicted by ctla-4 blockade in cancer patients are associated with early diversification of the T-cell repertoire. Cancer Res (2016) 77(6):1322–30. doi: 10.1158/0008-5472.CAN-16-2324
89. Reynolds G, Nasrallah M, Smith N, Thomas M, Zubiri L, Tirard A, et al. 441 checkpoint inhibitor-associated inflammatory arthritis is comprised of multiple clinical endotypes characterized by distinct transcriptional programs. J ImmunoTher Cancer (2022) 10(Suppl 2):A462–A. doi: 10.1136/jitc-2022-SITC2022.0441
90. Das R, Bar N, Ferreira M, Newman AM, Zhang L, Bailur JK, et al. Early b cell changes predict autoimmunity following combination immune checkpoint blockade. J Clin Invest (2018) 128(2):715–20. doi: 10.1172/JCI96798
91. Glehr G, Riquelme P, Yang Zhou J, Cordero L, Schilling HL, Kapinsky M, et al. External validation of biomarkers for immune-related adverse events after immune checkpoint inhibition. Front Immunol (2022) 13:1011040. doi: 10.3389/fimmu.2022.1011040
92. Maslov DV, Tawagi K, Kc M, Simenson V, Yuan H, Parent C, et al. Timing of steroid initiation and response rates to immune checkpoint inhibitors in metastatic cancer. J Immunother Cancer (2021) 9(7):e001161. doi: 10.1136/jitc-2020-002261
93. van Not OJ, Verheijden RJ, van den Eertwegh AJM, Haanen JBAG, Aarts MJB, van den Berkmortel FWPJ, et al. Association of immune-related adverse event management with survival in patients with advanced melanoma. JAMA Oncol (2022)8:1794–1801. doi: 10.1001/jamaoncol.2022.5041
94. Oh TK, Song IA. Trends in long-term glucocorticoid use and risk of 5-year mortality: A historical cohort study in south Korea. Endocrine (2020) 69(3):634–41. doi: 10.1007/s12020-020-02382-6
95. del Rincon I, Battafarano DF, Restrepo JF, Erikson JM, Escalante A. Glucocorticoid dose thresholds associated with all-cause and cardiovascular mortality in rheumatoid arthritis. Arthritis Rheumatol (2014) 66(2):264–72. doi: 10.1002/art.38210
96. Einarsdottir MJ, Ekman P, Molin M, Trimpou P, Olsson DS, Johannsson G, et al. High mortality rate in oral glucocorticoid users: A population-based matched cohort study. Front Endocrinol (Lausanne) (2022) 13:918356. doi: 10.3389/fendo.2022.918356
97. Martins F, Sykiotis GP, Maillard M, Fraga M, Ribi C, Kuntzer T, et al. New therapeutic perspectives to manage refractory immune checkpoint-related toxicities. Lancet Oncol (2019) 20(1):e54–64. doi: 10.1016/S1470-2045(18)30828-3
98. Lesage C, Longvert C, Prey S, Maanaoui S, Dreno B, Machet L, et al. Incidence and clinical impact of anti-tnfalpha treatment of severe immune checkpoint inhibitor-induced colitis in advanced melanoma: The mecolit survey. J Immunother (2019) 42(5):175–9. doi: 10.1097/CJI.0000000000000268
99. Feinberg B, Kurzrock R, Talpaz M, Blick M, Saks S, Gutterman JU. A phase I trial of intravenously-administered recombinant tumor necrosis factor-alpha in cancer patients. J Clin Oncol (1988) 6(8):1328–34. doi: 10.1200/JCO.1988.6.8.1328
100. Moore RJ, Owens DM, Stamp G, Arnott C, Burke F, East N, et al. Mice deficient in tumor necrosis factor-alpha are resistant to skin carcinogenesis. Nat Med (1999) 5(7):828–31. doi: 10.1038/10552
101. Wadstrom H, Frisell T, Askling J, Anti-Rheumatic Therapy in Sweden Study G. Malignant neoplasms in patients with rheumatoid arthritis treated with tumor necrosis factor inhibitors, tocilizumab, abatacept, or rituximab in clinical practice: A nationwide cohort study from Sweden. JAMA Intern Med (2017) 177(11):1605–12. doi: 10.1001/jamainternmed.2017.4332
102. Nguyen DX, Ehrenstein MR. Anti-tnf drives regulatory T cell expansion by paradoxically promoting membrane tnf-Tnf-Rii binding in rheumatoid arthritis. J Exp Med (2016) 213(7):1241–53. doi: 10.1084/jem.20151255
103. Campochiaro C, Farina N, Tomelleri A, Ferrara R, Lazzari C, De Luca G, et al. Tocilizumab for the treatment of immune-related adverse events: A systematic literature review and a multicentre case series. Eur J Intern Med (2021) 93:87–94. doi: 10.1016/j.ejim.2021.07.016
104. Brown AM, Masterson W, Lo J, Patel AB. Systemic treatment of cutaneous adverse events after immune checkpoint inhibitor therapy: A review. Dermatitis (2021). doi: 10.1097/DER.0000000000000776
105. Patel AJ, Willsmore ZN, Khan N, Richter A, Naidu B, Drayson MT, et al. Regulatory b cell repertoire defects predispose lung cancer patients to immune-related toxicity following checkpoint blockade. Nat Commun (2022) 13(1):3148. doi: 10.1038/s41467-022-30863-x
Keywords: checkpoint inhibitors (CPI), immune-related adverse events, autoimmunity, dermatological, gastrointestinal, musculoskeletal, pathobiology
Citation: Williams KC, Gault A, Anderson AE, Stewart CJ, Lamb CA, Speight RA, Rajan N, Plummer R and Pratt AG (2023) Immune-related adverse events in checkpoint blockade: Observations from human tissue and therapeutic considerations. Front. Immunol. 14:1122430. doi: 10.3389/fimmu.2023.1122430
Received: 12 December 2022; Accepted: 16 January 2023;
Published: 26 January 2023.
Edited by:
Thomas Skripuletz, Hannover Medical School, GermanyReviewed by:
Kathryn Bollin, Scripps Clinic, United StatesJustin Moyers, UC Irvine Medical Center, United States
Celine Boutros, Gustave Roussy Cancer Campus, France
Copyright © 2023 Williams, Gault, Anderson, Stewart, Lamb, Speight, Rajan, Plummer and Pratt. This is an open-access article distributed under the terms of the Creative Commons Attribution License (CC BY). The use, distribution or reproduction in other forums is permitted, provided the original author(s) and the copyright owner(s) are credited and that the original publication in this journal is cited, in accordance with accepted academic practice. No use, distribution or reproduction is permitted which does not comply with these terms.
*Correspondence: Arthur G. Pratt, arthur.pratt@newcastle.ac.uk