- 1Groupe de Recherche Action en Santé, Ouagadougou (GRAS), Ouagadougou, Burkina Faso
- 2Centre National de Recherche et de Formation sur le Paludisme (CNRFP), Ouagadougou, Burkina Faso
- 3Department of Malaria Vaccine Development, Research Institute for Microbial Diseases, Osaka University, Suita, Osaka, Japan
- 4European Vaccine Initiative (EVI), Universitäts Klinikum Heidelberg, Heidelberg, Germany
- 5Department of Molecular Protozoology, Research Institute for Microbial Diseases, Osaka University, Suita, Osaka, Japan
Background: A vaccine targeting the erythrocyte stages of Plasmodium falciparum could play a role in preventing clinical disease. BK-SE36 is a promising malaria vaccine candidate that has shown a good safety profile and immunological responses during field evaluations. It was observed that repeated natural infections could result in immune tolerance against SE36 molecule.
Methods: The primary trial was conducted to assess the safety and immunogenicity of the BK-SE36 in two cohorts of children aged 25-60 months (Cohort 1) and 12-24 months (Cohort 2). Immunization was at full dose (1.0 mL) administered at 0, 1, and 6 months. Blood samples were collected before each vaccination for immunological assessments and detection of Plasmodium falciparum infection by microscopy. Blood samples were further collected one month post each vaccination to evaluate immunogenicity.
Results: Of seventy-two (72) subjects that have received BK-SE36 vaccination, 71 had available blood smears during vaccination days. One month post Dose 2, the geometric mean of SE36 antibodies was 263.2 (95% CI: 178.9-387.1) in uninfected individuals compared to 77.1 (95% CI: 47.3-125.7) in infected participants. The same trend was observed one-month post booster dose. Participants uninfected at the time of booster vaccination had significantly higher GMTs compared to those who were infected (424.1 (95% CI: 301.9-595.8) vs. 92.8 (95% CI: 34.9-246.6), p = 0.002. There was a 14.3 (95% CI: 9.7-21.1) and 2.4 (95% CI: 1.3-4.4) fold-change, respectively, in uninfected and infected participants between one-month post Dose 2 and booster. The difference was statistically significant (p < 0.001).
Conclusion: Concomitant infection by P. falciparum during BK-SE36 vaccine candidate administration is associated with reduced humoral responses. However, it is to be noted that the BK-SE36 primary trial was not designed to investigate the influence of concomitant infection on vaccine-induced immune response and should be interpreted cautiously.
Trial registration: WHO ICTRP, PACTR201411000934120.
1 Introduction
Malaria is a huge global public health problem with around 40% of the world’s population at risk of infection. In 2020, there were approximately 241 million clinical episodes of malaria and more than 627,000 deaths (1). Most of the mortality occurs among children under 5 years of age in sub-Saharan Africa where it is estimated that a child dies from malaria every minute. Malaria is caused by five species of parasites that infect humans, and all these species belong to the genus Plasmodium: P. falciparum, P. vivax, P. ovale, P. malariae and P. knowlesi. Malaria due to P. falciparum is the deadliest form and predominates in Africa.
An effective malaria vaccine is crucial in the face of continued high malaria transmission, increasing drug and insecticide resistance, and challenges in implementing different intervention strategies, e.g. bednets and indoor residual spraying (2–6). To date, only RTS,S, a pre-erythrocytic stage vaccine, has shown partial protection and is now recommended to be used for the prevention of P. falciparum malaria in children living in regions with moderate to high transmission, as defined by WHO (7, 8). The development of next-generation more effective malaria vaccines remains a high priority and is necessary for the global eradication of malaria.
There is a strong justification for blood-stage vaccines. Indeed, unlike vaccines against the pre-erythrocytic stages, vaccines against the blood-stage aim at reducing the parasite load by preventing invasion of red blood cells or limiting parasite replication/growth after invasion, thus protecting against clinical malaria. So far, reported vaccine candidates that target the blood-stage inhibit the growth of the parasites largely by allele-specific antibodies (9).
A recombinant form of SERA5 N-terminal domain (SE36) was selected for clinical development based on (a) epidemiological studies that show correlations of high antibody titers and malaria symptoms and severe disease (10–13); (b) in vitro studies that demonstrate parasite growth inhibition or antibody-dependent complement-mediated lysis of schizonts, or antibody-dependent monocyte-mediated parasite growth inhibition (14–17); and (c) non-human primate challenge studies that demonstrate protection against P. falciparum challenge infection (10, 17, 18). The serine repeat antigen 5 (SERA5) is a highly expressed, essential blood-stage protein (19) with limited genetic differentiation and polymorphism (20). SE36 was prepared under Good Manufacturing Practice (GMP) standards and formulated with aluminum hydroxide gel (AHG) to yield BK-SE36 (10).
Phase I safety and immunogenicity trials of BK-SE36 were conducted in healthy, malaria-naïve Japanese adults (10), in malaria-exposed Ugandan volunteers aged 6- to 32-year-old (21), and in healthy 12- to 60-month-old Burkinabe children (22). These trials have demonstrated the safety and immunogenicity of BK-SE36.
In the effort to optimize vaccine induced immune response and identify factors that can confound trial endpoints, or influence vaccination schedules, in this study we aimed at understanding whether malaria infection at the time of vaccination may explain some of the heterogeneity observed in initial trials. Thus, we examined the malaria blood smears collected before each vaccination during the primary trial to explore if P. falciparum infection had interfered with the immune responses to the BK-SE36 malaria vaccine candidate in line with previously reported malaria-induced immunosuppression (23, 24).
2 Methods
2.1 Immunization and sample processing
The full study methodology as well as the trial protocol were published elsewhere (22). In brief, a double-blind, randomized, controlled, single-center study was conducted in Banfora, a place with intense and markedly seasonal malaria transmission where P. falciparum is responsible for more than 90% of all clinical malaria cases (25, 26). Coinciding with the rainy months of May-November, 60% of clinical cases are reported during the months of June-September (26). The age-de-escalating, phase Ib clinical trial with a single-blind follow-up phase enrolled 108 healthy, malaria-exposed African children. Children in both Cohort 1 (aged 25–60 months, n = 54) and Cohort 2 (aged 12–24 months, n = 54) were randomized into 3 treatment arms in a 1:1:1 ratio receiving: (a) 3 full doses of BK-SE36 by the subcutaneous route (SC), (b) 3 full doses of BK-SE36 by the intramuscular route (IM), and (c) 2 doses of the licensed Pneumococcal polysaccharide conjugate decavalent Synflorix® vaccine, alternate with 1 dose of physiological saline by the IM route (control arm). The inclusion of a third dose at Week 26 (W26; Day 182) was intended to increase the immune response and evaluate the effect of a booster dose. The first and second vaccine doses were given during the rainy season (peak of malaria transmission); the third dose during the dry (low transmission) season. In Cohort 1, the primary doses (28 days apart) were given in July-Aug 2015 and the booster dose (6 months from the first dose) given January 2016. For Cohort 2, primary vaccinations were given Oct-Nov 2015, and the booster on April 2016.
Anti-SE36 IgG antibody titers were measured by ELISA before vaccination (D0, D182), 4 weeks after each vaccination (D28, D56, D210), and at D365 and D477. ELISA was outsourced to a GLP certified testing facility (CMIC Pharma Science Co., Ltd., Japan) and used a standardized methodology. Results were expressed in titers calculated using an equilibrium line assay (10, 21).
Geometric mean titers (GMTs) were calculated at each time point. IgG1 and IgG3 subclasses were determined (27) for those with detectable anti-SE36 antibody titers 4 weeks after the second (W8/D56) and third (W30/D210) vaccinations.
Prior to each vaccination, capillary blood was also collected to prepare malaria smears. The presence of parasites was assessed by 100 x bright field microscopic examination, assuming 8,000 leukocytes/µl of blood. The count was made by species (P. falciparum, P. malariae or P. ovale), and counts for P. falciparum were made for both sexual and asexual parasites. The parasite presence and density were determined independently by two readers for the same slide; if readings were judged to be discordant, a third independent read was organized. The parasite density (parasites/μL) was calculated as the geometric mean of the two positive readings (two geometrically closest readings in the case of three positive reads). Microscopist competency was evaluated through two external quality control (EQC) programs. The first EQC was carried out by the College of American Pathology; proficiency testing with a set of 5 slides provided to each microscopist for reading thrice a year. Only a performance graded above 80% was considered satisfactory for the laboratory. The second EQC was performed by WHO (National Institute for Communicable Diseases) and involved the reading of a set of 20 slides every quarter by each microscopist. Only those with a score of at least 80%, graded as ‘competent’, were tasked in the reading of trial participants’ slides.
2.2 Statistical methods
Since the primary analysis showed no difference in terms of immune responses between the intramuscular and subcutaneous routes (22), the two arms were pooled for this study. The control arm is not of interest and was excluded from the current secondary analysis.
There were three categories depending on vaccination days and available blood smears/parasitemia data on those days. The first category (subgroup 1) includes subjects who received Dose 1 and 2 (termed “primary immunization”) and had a blood smear taken at these two occasions with results available. The subgroup considered subjects who received 2 doses since the interest is to see the effect of infection on the full vaccination status; however, the difference in GMTs between infected and non-infected subjects after one dose is provided in Supplementary Table S1. The second category (subgroup 2) is composed of subjects who received the booster (Dose 3) vaccination and had results of a blood smear taken before the booster dose. The third category (subgroup 3) includes subjects that received both primary and booster vaccinations and had results available for blood smear obtained at each of the three vaccination days.
Antibody titers and P. falciparum count are presented in terms of the geometric mean with 95% confidence interval. Because SE36 antibody titers are not normally distributed (with outliers as well as limits of detection (e.g., undetectable titers were assigned a value of “8” for statistical analyses), we use the Wilcoxon rank sum test. All the comparisons are made between infected and uninfected groups. A p-value of 0.05 was the level of statistical significance.
Statistical analyses were performed using Stata ver. 14 (StataCorp, College Station, TX, USA, www.stata.com) and GraphPad (Prism ver. 9.4.0).
2.3 Ethical and regulatory approval
The trial was conducted according to the principles of the Declaration of Helsinki and the International Conference on Harmonization (ICH) Good Clinical Practice (GCP) guidelines. The clinical trial protocol and associated documents were reviewed and approved in Burkina Faso by CNRFP institutional bioethics committee (Ref: n°2014/071/MS/SG/CNRFP/CIB, N°2016/000008/MS/SG/CNRFP/CIB), the Ministry of Health Ethical Committee for Biomedical Research (Ref: 2014-12-144) and the National Regulatory Authority (Approval for the clinical trial: (N°2015_658/MS/CAB). The trial package was also approved by the Scientific Committee/Institutional Review Committee of the Research Institute for Microbial Diseases (Ref: 26-6), Osaka University (Ref: 574) in Japan; and the London School of Hygiene and Tropical Medicine Research Ethics Committee (Ref: 9175) in United Kingdom.
3 Results
3.1 Baseline characteristics
The vaccination of Cohort 1 started on July 4, 2015 (Dose 1), and the last vaccination (Dose 3) was completed by January 11, 2016. Vaccination of Cohort 2 began on October 12, 2015 (Dose 1) and was completed by April 18, 2016 (Dose 3). In total 72 participants received 2 primary doses of BK-SE36 (22), however 1 subject in Cohort 1 had a missing blood smear during Dose 2 vaccination day and thus only 71 subjects were included in subgroup 1 analyses. For subgroup 2, all subjects who have received Dose 3 and had a blood smear before vaccination were included in the analyses (n=68). For subgroup 3, all subjects with primary and booster vaccination and a blood smear at these vaccination days were included (n=68).
The median age was 25.4 (IQR 28.2) months, and 38.9% of subjects were male.
3.2 Carriage of Plasmodium falciparum infection during immunization
The proportion of individuals carrying P. falciparum infection at the time of primary immunizations is shown in Figure 1. Infection is defined as any asexual parasitemia > 0 by microscopy. In this study, the vaccine treatment arms (SC and IM) were pooled for analysis due to the small sample size, the randomized nature of the trial, and the results indicating no difference in the vaccine induced immune response between the two routes. Therefore, it is unlikely that there are differences in malaria infection during vaccinations days and vaccine induced antibody response in the SC and IM arms. During vaccinations days there were no recorded P. malariae, P. vivax and P. ovale infections.
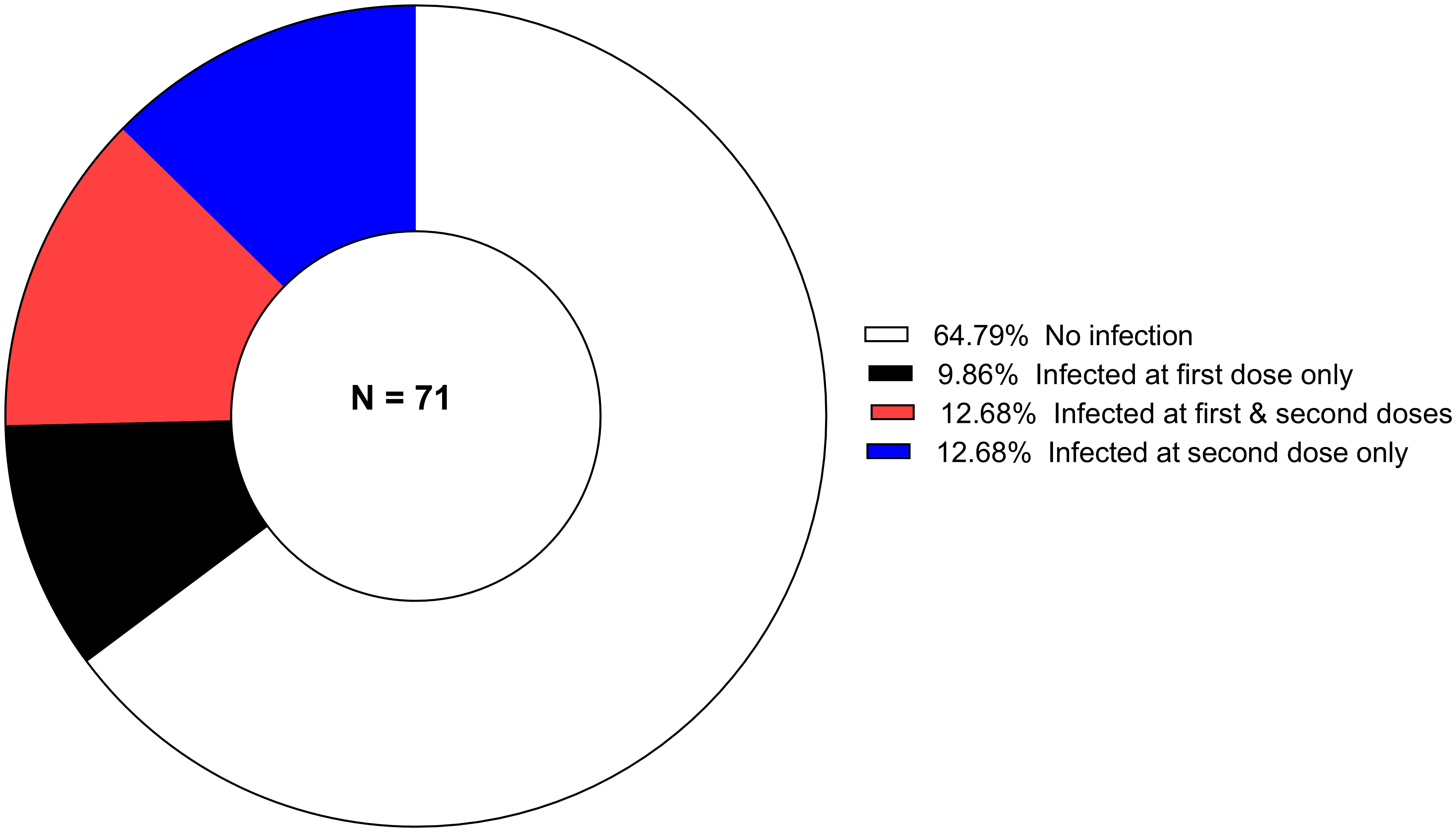
Figure 1 The proportion of P. falciparum-infected children during primary vaccination (Dose 1 and Dose 2) days.
For subgroup 1, 64.8% (46/71) participants were free of infection at both Dose 1 and 2. At either dose, 35.2% (25/71) were harboring malaria parasites. The mean age was 39.6 ( ± 14.9) and 27.9 ( ± 14.6) months in infected and uninfected children, respectively. The difference in the proportion of children infected during vaccination days was statistically significant (p = 0.002).
In subgroup 2, no P. falciparum infection was found for 83.8% (57/68) of the vaccinees at the time of the booster vaccination. The mean age was 45.2 ( ± 14.6) and 29.5 ( ± 14.9) months in infected and uninfected children, respectively. The difference was statistically significant (p = 0.001).
In total, 58.8% (40/68) receive their primary and booster doses in the absence of any P. falciparum infection (subgroup 3). The mean age was 39.7 ( ± 15.8) and 26.7 ( ± 13.8) months in infected and uninfected children, respectively. Again, the difference was statistically significant (p < 0.001).
The geometric mean of P. falciparum parasites density was 1077.6 trophozoites/µL (95% CI, 451.5-2571.9), 1811.4 trophozoites/µL (95% CI, 4716.9-4576.8) and 2359.9 trophozoites/µL (95% CI, 649.3-8577.6) respectively at Dose 1, 2 and 3. Individual P. falciparum count is provided in Supplementary Figure S1.
3.3 Immune responses and Plasmodium falciparum carriage status
Immune responses to BK-SE36 vaccination in infected and uninfected individuals are shown in Figure 2. At baseline (before Dose 1, Figure 2A), the GMT of SE36 antibody titers was 15.1 (95% CI, 11.3-20.2) in uninfected individuals compared to 17.3 (95% CI, 8.7-34.4) in infected participants (p = 0.877). One-month post-primary vaccinations (after Dose 2), the geometric mean of SE36 antibodies was 263.2 (95% CI, 178.9-387.1) in uninfected individuals compared to 77.1 (95% CI, 47.3-125.7) in infected participants. The difference was statistically significant (p < 0.001). In terms of fold change for each subject, there was a 2.2 (95% CI, 0.1.3-3.5) and 1.6 (95% CI, 1.0-2.6) fold change respectively in uninfected and infected participants from baseline to one-month post Dose 2. The difference, however, was not statistically significant (p = 0.361, Figure 2C).
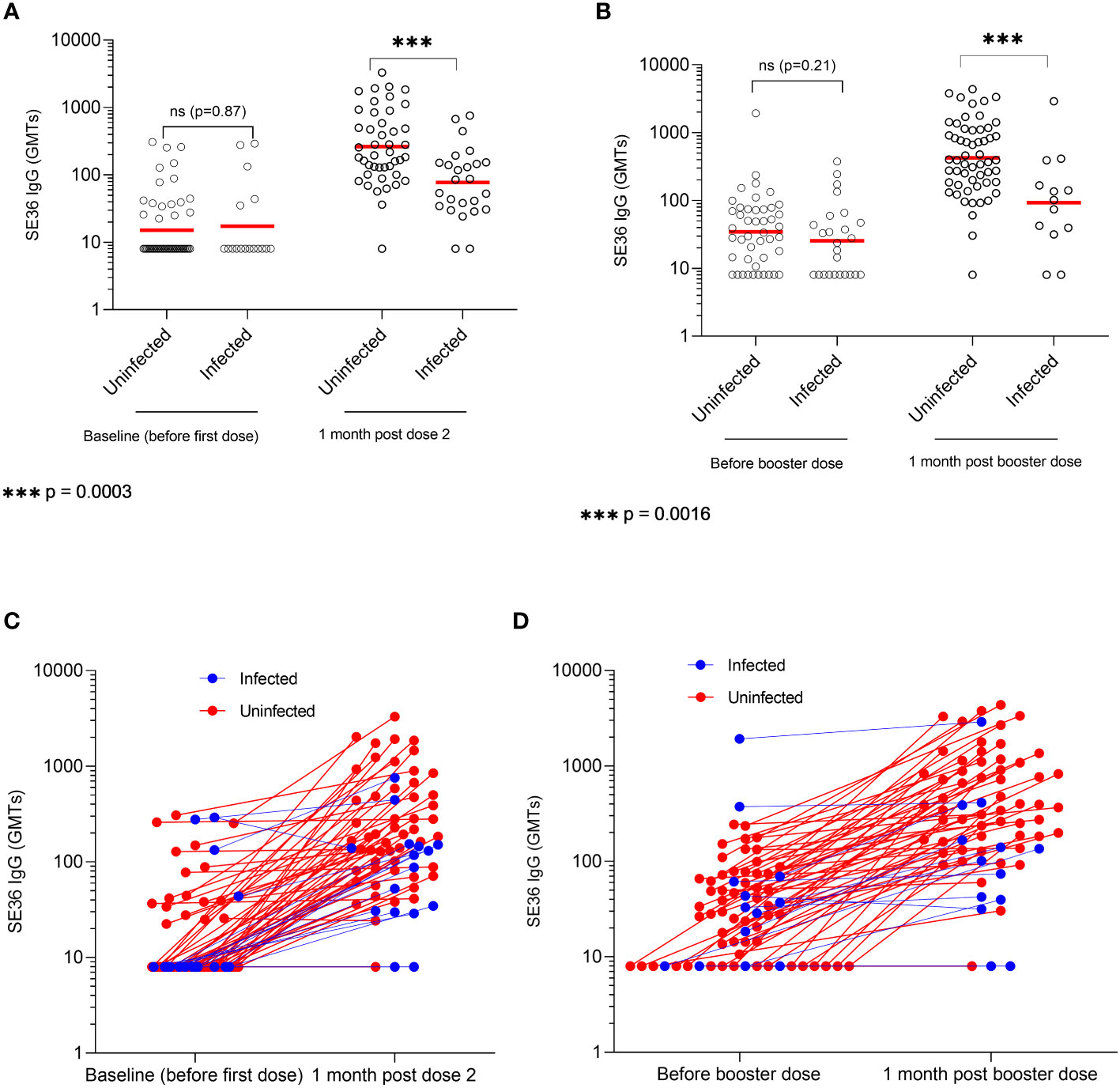
Figure 2 SE36 antibody response by infection status. Panels (A, B) show the serum anti-SE36 antibody titers (expressed as geometric means, GMT) at primary and booster vaccination, respectively. Panels (C, D) show the individual change in antibody titers one month after Dose 2 and a booster dose (Dose 3), respectively. Solid red line represents the geometric mean.
Before Dose 3 or booster dose (22 weeks post Dose 2), the GMT was 34.6 (95% CI, 24.3-49.3) in uninfected individuals compared to 25.4 (95% CI, 15.4-41.9) in infected participants. The difference was not statistically significant (p = 0.212) (Figure 2B). One month post Dose 3, participants uninfected at the time of booster vaccination had significantly higher GMTs compared to those who were infected on vaccination day (424.1 (95% CI, 301.9-595.8) vs. 92.8 (95% CI, 34.9-246.6); p = 0.002). There was a 14.3 (95% CI, 9.7-21.1) and 2.4 (95% CI, 1.3-4.4) fold change respectively in uninfected and infected participants between one-month post Dose 2 and Dose 3. The difference was statistically significant (p < 0.001, Figure 2D).
Study participants who did not have any microscopically detectable P. falciparum infection during primary and booster vaccinations had the highest GMT at one-month post Dose 3 (602.2; 95% CI, 421.5-860.3) compared to those who were found with P. falciparum infection at some point in time during vaccination days (139.2; 95% CI, 80.7-240.3). The difference between uninfected and infected subjects was statistically significant (p < 0.001). This difference was maintained towards the study end with GMTs of 101.0 (95% CI, 62.3-163.8) vs 27.21 (95% CI, 15.5-44.5) (p = 0.006) at visit 32 (D365); and 96.7 (95% CI, 59.4-157.3) vs. 25.6 (95% CI, 15.5-42.4), (p = 0.001) at visit 36 (D477) (uninfected vs infected subjects). A subgroup analysis by cohort is provided in Supplementary Table S2.
Anti-SE36 IgG antibody subclasses levels in uninfected and infected children are presented in Supplementary Figure S2. The IgG1 concentrations measured 4 weeks after Doses 2 and 3 were significantly higher in uninfected children as compared to those who had an infection during vaccination days. For IgG3, a statistically significant difference in titers between both groups was only observed post-Dose 2.
4 Discussion
In this study, we report the immune responses post-vaccination with BK-SE36 blood-stage malaria vaccine candidate in 12-60 months old children in Burkina Faso. The study findings suggest that concurrent blood-stage infection during immunization/vaccination days has reduced the magnitude of the immune responses to the vaccine. This low response to vaccination in infected individuals has also been reported for pre-erythrocyte vaccine candidates including PfSPZ (28) and ME-TRAP (29). In another phase 1 trial with a blood-stage vaccine candidate, Combination B (comprised of three asexual blood-stage antigens: merozoite surface protein 1, merozoite surface protein 2 and the ring-infected erythrocyte surface antigen), the effect of parasite clearance with sulphadoxine-pyrimethamine (SP) prior to vaccination in 5-9 years-old Papua New Guinean children was assessed (30). Antibody titers to the component antigens were not significantly modified/enhanced by SP treatment 1 week prior to vaccination although cellular response (IFN-γ) to merozoite surface protein 1 was substantially lower in the vaccinated subjects who had received SP. Additional evidence will be needed to better understand the differences observed between the BK-SE36 and Combination B vaccines. It shall also be noted that the BK-SE36 primary trial was not designed to investigate the influence of concomitant infection on vaccine-induced immune response and should be interpreted cautiously.
The malaria parasite is known to induce immunosuppression in the host during asexual/blood-stage development and this impairs the generation as well as the function of humoral and cell-mediated immunity (31). Molecular profiling has shown that asymptomatic P. falciparum malaria is characterized by an important immunoregulatory blood transcriptional signature with the upregulation of various pathways involved in the inhibition of CD4+T-cell function (32). As such, asymptomatic malaria might not support the induction of immune processes to control parasitemia or respond efficiently to malaria vaccines.
SE36 is known to tightly bind to host protein vitronectin that further binds to different host proteins in an act of molecular camouflage (33). Repeated infections and the presence of these vitronectin-bound SE36 complexes could inevitably result in immune tolerance against SE36 molecule. The reported immune tolerance is mainly due to a host response to repeated infections and takes some time to acquire (33, 34). The immunosuppression described here would rather be caused by an impaired vaccine-induced immune response consequent to a concomitant infection. It can be argued that, in the analysis, infection was defined as any asexual parasitaemia > 0 by microscopy and this definition could include new infections, those with rising parasitaemia or long-standing infections imperfectly controlled by host immune response. The primary trial was designed to have two age cohorts (22). Cohort 1 with children aged 25-60 months and a younger Cohort 2 with children aged 12-24 months. The children of Cohort 1 have presumably a longer history of exposure to repeated malaria infections, and some may represent a subgroup of children with lower SE36 responsiveness. The immune tolerance of Cohort 1 may explain why the younger children of Cohort 2 had 2-fold and 4-fold higher antibody titers than Cohort 1 after Dose 2 and Dose 3 of BK-SE36, respectively (22). In the BK-SE36 arms before vaccination, there were more children in Cohort 2 that presented high antibody titres (n=17) than in Cohort 1 (n=5). This finding may be due to chance or presence of maternal antibodies in younger cohorts [as explained in (22)]. Another observation was that more children in Cohort 1 were infected at the time of Dose 1 and 2 (52.8%) vs. 19.4% in Cohort 2, p = 0.003. Most likely, the observed lower immune response in Cohort 1 may be due to immune suppression. The response during or shortly after infection with Plasmodium spp. was reviewed by Calle et al. (31). Immunosuppression is likely a complex combination/interplay of innate and adaptive immune factors that may involve qualitative changes in B- and T-cell responses (35), e.g. expansion of atypical memory B cells (36), reduction of T-cells (37–39), activation of NK cells for early IFN-γ response (40).
Notably, not only the absolute quantity of antibody but the quality (affinity and subclass) and longevity of antibodies are also important (41, 42). Antibody levels dropped to near pre-vaccination titers 6 months after the first dose in both uninfected and infected participants (GMT 25 and 34). After Dose 3, antibody levels peaked 4-fold higher than after Dose 2, suggesting that a third dose is necessary in endemic areas to obtain the best possible immune response (a suggestion that was inferred also from the Ugandan study (21)).
Of note, a multivariate analysis showed, as expected, a negative correlation between parasite density and anti-SE36 antibody GMT. Adjusted for baseline antibody titer, age, and the interaction between age and baseline antibody response there was a 1.93%, 133.9% and 11.3% decrease in antibody GMT for each 1000 parasites/μl increase in P. falciparum density, respectively for subgroup 1, 2 and 3. Whether clearing the infections before immunization could mitigate this immunosuppression also needs further studies.
Currently, the status of P. falciparum infection was only defined based on microscopy. Owing to the detection limit of optical microscopy (~ 100 parasites/µL), we could not rule out the possibility that submicroscopic infections were missed (43), as this is a common feature in malaria-endemic settings (44–46). Moreover, because of the dynamic parasite life cycle, the time of blood sampling may also miss low-density infections even if current routine molecular diagnostic tests are used (46). While misclassifications of the subgroup may bias estimates, the observation that concomitant parasitemia (not just a history of infection) is not innocuous has implications for the scheduling and administration of the malaria blood-stage vaccine BK-SE36.
5 Conclusions
These findings confirm the immunosuppressive role of P. falciparum and the low response caused by parasite infection when the BK-SE36 malaria vaccine candidate is administered. The study also supports the need to further explore whether clearing the existing infections before vaccination could improve immune responses and potential vaccine efficacy.
Data availability statement
The original contributions presented in the study are included in the article/Supplementary Material. Further inquiries can be directed to the corresponding authors.
Ethics statement
The studies involving human participants were reviewed and approved by Burkina Faso : CNRFP institutional bioethics committee (Ref: n°2014/071/MS/SG/CNRFP/CIB, No2016/000008/MS/SG/CNRFP/CIB), the Ministry of Health Ethical Committee for Biomedical Research (Ref: 2014-12-144) and the National Regulatory Authority (Approval for the clinical trial (No2015_658/MS/CAB); Japan: the Scientific Committee/Institutional Review Committee of the Research Institute for Microbial Diseases (Ref: 26-6), Osaka University (Ref: 574); and United Kingdom: London School of Hygiene and Tropical Medicine Research Ethics Committee (Ref: 9175). Written informed consent to participate in this study was provided by the participants’ legal guardian/next of kin.
Author contributions
SS, EB, AT, IN, SH, FD, NP, and TH contributed to the conception and design of the study. AT, EB, IN, AO, SS were responsible for study implementation at the study site. AT performed the statistical analysis. NA and NP for materials/reagents/assay development and analysis tools. AT and NP wrote the first draft of the manuscript. All authors contributed to the article and approved the submitted version.
Funding
This work was supported by the Global Health Innovative Technology Fund (G2014-109; G2019-208) awarded to the Consortium; MEXT KAKENHI Grant Numbers 15651988, 24249024, Funds for Integrated Promotion of Social System Reform and Research and Development (38201103-01), Grant for Translational Research Network Program (JP20lm0203135, AMED) (C-13) awarded to TH. Grant for Cyclic Innovation for Clinical Empowerment by AMED (17nk0101206j0003) to Nobelpharma Co., Ltd (NPC, Tokyo, Japan); and Irish Aid to EVI. Additional in-kind contribution was also obtained from NPC. The funders were not involved in the study design, collection, analysis, interpretation of data, the writing of this article, or the decision to submit it for publication.
Acknowledgments
We thank study volunteers and their families for participation in the study and acknowledge the support of the URCB Centre (Unite de Recherche Clinique de Banfora), the Local Safety Monitor, and the Independent Safety Monitoring Committee for their support (Ken J. Ishii, Yoshio Hirota, Meta Roestenberg, Kwaku Poku Asante and Marcelline T. Yaméogo). Guidance of the regulatory authorities and ethics committees in Burkina Faso, UK, Japan; and the Independent Scientific Advisory Committee (Carole Long, Mahamadou Thera, Takafumi Tsuboi, and Chetan Chitnis) during the trial and follow-up study are much appreciated. We also gratefully acknowledge the clinical trial assistance of Lydia Dabré (GRAS), the quality assurance assistance of Nathalie Imbault (EVI), laboratory assistance of Hideko Yoshikawa and Sawako Itagaki (RIMD); the administration and logistical support of Nadège Kinda (GRAS), Sten Larsen Finnsson and Thorsten Kohaut (EVI), and Kumiko Tai (RIMD). Vaccine manufacture and supply were supported and undertaken by The Research Foundation for Microbial Diseases of Osaka University (BIKEN), Japan. The trial was sponsored by NPC; coordination, support, and all other activities are duly acknowledged from Masanori Osakabe, Takako Aburada, Taka Sato, Satoru Murayama, and Motomichi Kouno.
Conflict of interest
TH is the inventor of BK-SE36 and all rights have now been turned over to Nobelpharma Co., Ltd (NPC), Tokyo, Japan. NP served as a contract researcher for NPC, Apr - Sept 2017. SH and FD received support from NPC for salaries, travel, and CRO cost for clinical monitoring. EB also received support from NPC for salaries during the long-term follow-up.
The remaining authors declare that the research was conducted in the absence of any commercial or financial relationships that could be construed as a potential conflict of interest.
Publisher’s note
All claims expressed in this article are solely those of the authors and do not necessarily represent those of their affiliated organizations, or those of the publisher, the editors and the reviewers. Any product that may be evaluated in this article, or claim that may be made by its manufacturer, is not guaranteed or endorsed by the publisher.
Supplementary material
The Supplementary Material for this article can be found online at: https://www.frontiersin.org/articles/10.3389/fimmu.2023.1119820/full#supplementary-material
References
1. World Health Organization. World malaria report. (2021). Available at: https://www.who.int/teams/global-malaria-programme/reports/world-malaria-report-2021.
2. Dhiman S. Are malaria elimination efforts on right track? An analysis of gains achieved and challenges ahead. Infect Dis Poverty (2019) 8:14. doi: 10.1186/s40249-019-0524-x
3. Balikagala B, Fukuda N, Ikeda M, Katuro OT, Tachibana SI, Yamauchi M, et al. Evidence of artemisinin-resistant malaria in Africa. N Engl J Med (2021) 385:1163–71. doi: 10.1056/NEJMoa2101746
4. Nkumama IN, Osier FHA. Malaria vaccine roller coaster. Nat Microbiol (2021) 6:1345–46. doi: 10.1038/s41564-021-00982-0
5. Orok AB, Ajibaye O, Aina OO, Iboma G, Oboshi SA, Iwalokun B. Malaria interventions and control programes in Sub-Saharan Africa: A narrative review. Cogent Med (2021) 8:1. doi: 10.1080/2331205X.2021.1940639
6. Stokes BH, Ward KE, Fidock DA. Evidence of artemisinin-resistant malaria in Africa. N Engl J Med (2022) 386:1385–86. doi: 10.1056/NEJMc2117480
7. World Health Organization. WHO recommends groundbreaking malaria vaccine for children at risk, in: Historic RTS,S/AS01 recommendation can reinvigorate the fight against malaria. Available at: https://www.who.int/news/item/06-10-2021-who-recommends-groundbreaking-malaria-vaccine-for-children-at-risk (Accessed Oct 24, 2022).
8. Zavala F. RTS,S: The first malaria vaccine. J Clin Invest (2022) 132:e156588. doi: 10.1172/JCI156588
9. Cai J, Chen S, Zhu F, Lu X, Liu T, Xu W. Whole-killed blood-stage vaccine: Is it worthwhile to further develop it to control malaria? Front Microbiol (2021) 12:670775. doi: 10.3389/fmicb.2021.670775
10. Horii T, Shirai H, Jie L, Ishii KJ, Palacpac NQ, Tougan T, et al. Evidences of protection against blood-stage infection of Plasmodium falciparum by the novel protein vaccine SE36. Parasitol Int (2010) 59:380–6. doi: 10.1016/j.parint.2010.05.002
11. Okech B, Mujuzi G, Ogwal A, Shirai H, Horii T, Egwang TG. High titers of IgG antibodies against Plasmodium falciparum serine repeat antigen 5 (SERA5) are associated with protection against severe malaria in Ugandan children. Am J Trop Med Hyg (2006) 74:191–7.
12. Okech BA, Nalunkuma A, Okello D, Pang XL, Suzue K, Li J, et al. Natural human immunoglobulin G subclass responses to Plasmodium falciparum serine repeat antigen in Uganda. Am J Trop Med Hyg (2001) 65:912–7. doi: 10.4269/ajtmh.2001.65.912
13. Owalla TJ, Palacpac NM, Shirai H, Horii T, Egwang TG. Association of naturally acquired IgG antibodies against Plasmodium falciparum serine repeat antigen-5 with reduced placental parasitemia and normal birth weight in pregnant Ugandan women: A pilot study. Parasitol Int (2013) 62:237–9. doi: 10.1016/j.parint.2013.01.006
14. Alam A, Chauhan VS. Inhibitory potential of prodomain of Plasmodium falciparum protease serine repeat antigen 5 for asexual blood stages of parasite. PLoS One (2012) 7:e30452. doi: 10.1371/journal.pone.0030452
15. Aoki S, Li J, Itagaki S, Okech BA, Egwang TG, Matsuoka H, et al. Serine repeat antigen (SERA5) is predominantly expressed among the SERA multigene family of Plasmodium falciparum, and the acquired antibody titers correlate with serum inhibition of the parasite growth. J Biol Chem (2002) 277:47533–40. doi: 10.1074/jbc.M207145200
16. Arastu-Kapur S, Ponder EL, Fonović UP, Yeoh S, Yuan F, Fonović M, et al. Identification of proteases that regulate erythrocyte rupture by the malaria parasite plasmodium falciparum. Nat Chem Biol (2008) 4:203–13. doi: 10.1038/nchembio.70
17. Yagi M, Bang G, Tougan T, Palacpac NMQ, Arisue N, Aoshi T, et al. Protective epitopes of the Plasmodium falciparum SERA5 malaria vaccine reside in intrinsically unstructured n-terminal repetitive sequences. PLoS One (2014) 9:e98460. doi: 10.1371/journal.pone.0098460
18. Tougan T, Aoshi T, Coban C, Katakai Y, Kai C, Yasutomi Y, et al. TLR9 adjuvants enhance immunogenicity and protective efficacy of the SE36/AHG malaria vaccine in nonhuman primate models. Hum Vaccin Immunother (2013) 9:283–90. doi: 10.4161/hv.22950
19. Palacpac NMQ, Arisue N, Tougan T, Ishii KJ, Horii T. Plasmodium falciparum serine repeat antigen 5 (SE36) as a malaria vaccine candidate. Vaccine (2011) 29:5837–45. doi: 10.1016/j.vaccine.2011.06.052
20. Arisue N, Palacpac NMQ, Tougan T, Horii T. Characteristic features of the SERA multigene family in the malaria parasite. Parasit Vectors. (2020) 13(1):170. doi: 10.1186/s13071-020-04044-y
21. Palacpac NMQ, Ntege E, Yeka A, Balikagala B, Suzuki N, Shirai H, et al. Phase 1b randomized trial and follow-up study in Uganda of the blood-stage malaria vaccine candidate BK-SE36. PLoS One (2013) 8:e64073. doi: 10.1371/journal.pone.0064073
22. Bougouma EC, Palacpac NMQ, Tiono AB, Nebie I, Ouedraogo A, Houard S, et al. Safety and immunogenicity of BK-SE36 in a blinded, randomized, controlled, age de-escalating phase ib clinical trial in burkinabe children. Front Immunol (2022) 13:978591. doi: 10.3389/fimmu.2022.978591
23. Greenwood BM, Bradley-Moore AM, Bryceson AD, Palit A. Immunosuppression in children with malaria. Lancet (1972) 1:169–72. doi: 10.1016/s0140-6736(72)90569-7
24. Ho M, Webster HK, Looareesuwan S, Supanaranond W, Phillips RE, Chanthavanich P, et al. Antigen-specific immunosuppression in human malaria due to plasmodium falciparum. J Infect Dis (1986) 153:763–71. doi: 10.1093/infdis/153.4.763
25. Kangoye DT, Nebie I, Yaro JB, Debe S, Traore S, Ouedraogo O, et al. Plasmodium falciparum malaria in children aged 0-2 years: the role of foetal haemoglobin and maternal antibodies to two asexual malaria vaccine candidates (MSP3 and GLURP). PLoS One (2014) 9:e107965. doi: 10.1371/journal.pone.0107965
26. Tiono AB, Kangoye DT, Rehman AM, Kargougou DG, Kaboré Y, Diarra A, et al. Malaria incidence in children in south-West Burkina Faso: Comparison of active and passive case detection methods. PLoS One (2014) 9:e86936. doi: 10.1371/journal.pone.0086936
27. Ezoe S, Palacpac NMQ, Tetsutani K, Yamamoto K, Okada K, Taira M. Et al: First-in-human randomised trial and follow-up study of Plasmodium falciparum blood-stage malaria vaccine BK-SE36 with CpG-ODN(K3). Vaccine (2020) 38:7246–57. doi: 10.1016/j.vaccine.2020.09.056
28. Murphy SC, Deye GA, Sim BKL, Galbiati S, Kennedy JK, Cohen KW, et al. PfSPZ-CVac efficacy against malaria increases from 0% to 75% when administered in the absence of erythrocyte stage parasitemia: A randomized, placebo-controlled trial with controlled human malaria infection. PLoS Pathog (2021) 17:e1009594. doi: 10.1371/journal.ppat.1009594
29. Morter R, Tiono AB, Nebie I, Hague O, Ouedraogo A, Diarra A, et al. Impact of exposure to malaria and nutritional status on responses to the experimental malaria vaccine ChAd63 MVA ME-TRAP in 5-17 month-old children in Burkina Faso. Front Immunol (2022) 13:1058227. doi: 10.3389/fimmu.2022.1058227
30. Genton B, Al-Yaman F, Betuela I, Anders RF, Saul A, Baea K, et al. Safety and immunogenicity of a three-component blood-stage malaria vaccine (MSP1, MSP2, RESA) against Plasmodium falciparum in Papua new guinean children. Vaccine (2003) 22(1):30–41. doi: 10.1016/s0264-410x(03)00536-x
31. Calle CL, Mordmüller B, Singh A. Immunosuppression in malaria: Do Plasmodium falciparum parasites hijack the host? Pathogens (2021) 10:1277. doi: 10.3390/pathogens10101277
32. Studniberg SI, Ioannidis LJ, Utami RAS, Trianty L, Liao Y, Abeysekera W, et al. Molecular profiling reveals features of clinical immunity and immunosuppression in asymptomatic p. falciparum malaria. Mol Syst Biol (2022) 18:e10824. doi: 10.15252/msb.202110824
33. Tougan T, Edula JR, Takashima E, Morita M, Shinohara M, Shinohara A, et al. Molecular camouflage of Plasmodium falciparum merozoites by binding of host vitronectin to P47 fragment of SERA5. Sci Rep (2018) 8:5052. doi: 10.1038/s41598-018-23194-9
34. Shanks GD. Tolerance may be more appropriate than immunity when describing chronic malaria infections. Am J Trop Med Hyg (2019) 100(3):497–500. doi: 10.4269/ajtmh.18-0906
35. Illingworth J, Butler NS, Roetynck S, Mwacharo J, Pierce SK, Bejon P, et al. Chronic exposure to Plasmodium falciparum is associated with phenotypic evidence of b and T cell exhaustion. J Immunol (2013) 190(3):1038–47. doi: 10.4049/jimmunol.1202438
36. Weiss GE, Crompton PD, Li S, Walsh LA, Moir S, Traore B, et al. Atypical memory b cells are greatly expanded in individuals living in a malaria-endemic area. J Immunol (2009) 183(3):2176–82. doi: 10.4049/jimmunol.0901297
37. White CE, Villarino NF, Sloan SS, Ganusov VV, Schmidt NW. Plasmodium suppresses expansion of T cell responses to heterologous infections. J Immunol (2015) 194(2):697–708. doi: 10.4049/jimmunol.1401745
38. Van Braeckel-Budimir N, Kurup SP, Harty JT. Regulatory issues in immunity to liver and blood-stage malaria. Curr Opin Immunol (2016) 42:91–7. doi: 10.1016/j.coi.2016.06.008
39. Ademolue TW, Awandare GA. Evaluating antidisease immunity to malaria and implications for vaccine design. Immunology (2018) 153(4):423–34. doi: 10.1111/imm.12877
40. Obiero JM, Shekalaghe S, Hermsen CC, Mpina M, Bijker EM, Roestenberg M, et al. Impact of malaria preexposure on antiparasite cellular and humoral immune responses after controlled human malaria infection. Infect Immun (2015) 83(5):2185–96. doi: 10.1128/IAI.03069-14
41. Langhorne J, Ndungu FM, Sponaas AM, Marsh K. Immunity to malaria: more questions than answers. Nat Immunol (2008) 9:725–32. doi: 10.1038/ni.f.205
42. Cunnington AJ, Riley EM. Suppression of vaccine responses by malaria: insignificant or overlooked? Expert Rev Vaccines (2010) 9(4):409–29. doi: 10.1586/erv.10.16
43. Joanny F, Löhr SJ, Engleitner T, Lell B, Mordmüller B. Limit of blank and limit of detection of plasmodium falciparum thick blood smear microscopy in a routine setting in central Africa. Malaria J (2014) 13:234. doi: 10.1186/1475-2875-13-234
44. Frimpong A, Amponsah J, Adjokatseh AS, Agyemang D, Bentum-Ennin L, Ofori EA, et al. Asymptomatic malaria infection is maintained by a balanced pro- and anti-inflammatory response. Front Microbiol (2020) 11:559255. doi: 10.3389/fmicb.2020.559255
45. Whittaker C, Slater H, Nash R, Bousema T, Drakeley C, Ghani AC, et al. Global patterns of submicroscopic Plasmodium falciparum malaria infection: insights from a systematic review and meta-analysis of population surveys. Lancet Microbe (2021) 2:e366–74. doi: 10.1016/S2666-5247(21)00055-0
Keywords: SE36, malaria blood-stage vaccine, serine repeat antigen, SERA5, Plasmodium falciparum, immunogenicity
Citation: Tiono AB, Palacpac NMQ, Bougouma EC, Nebie I, Ouédraogo A, Houard S, Arisue N, D’Alessio F, Horii T and Sirima SB (2023) Plasmodium falciparum infection coinciding with the malaria vaccine candidate BK-SE36 administration interferes with the immune responses in Burkinabe children. Front. Immunol. 14:1119820. doi: 10.3389/fimmu.2023.1119820
Received: 09 December 2022; Accepted: 21 February 2023;
Published: 10 March 2023.
Edited by:
Katherine Rose Dobbs, Case Western Reserve University, United StatesReviewed by:
Virander Singh Chauhan, International Centre for Genetic Engineering and Biotechnology, IndiaRobert Sauerwein, TropIQ Health Sciences, Netherlands
Copyright © 2023 Tiono, Palacpac, Bougouma, Nebie, Ouédraogo, Houard, Arisue, D’Alessio, Horii and Sirima. This is an open-access article distributed under the terms of the Creative Commons Attribution License (CC BY). The use, distribution or reproduction in other forums is permitted, provided the original author(s) and the copyright owner(s) are credited and that the original publication in this journal is cited, in accordance with accepted academic practice. No use, distribution or reproduction is permitted which does not comply with these terms.
*Correspondence: Toshihiro Horii, horii@biken.osaka-u.ac.jp; Sodiomon B. Sirima, s.sirima@gras.bf
†Present address: Nobuko Arisue, Department of Hygiene and Public Health, Tokyo Women’s Medical University, Japan
‡These authors share last authorship