- Department of General Surgery, Affiliated Drum Tower Hospital, Nanjing University Medical School, Nanjing, China
Tissue-resident memory T cells (Trm) are a particular type of T cell subgroup, which stably reside in tissues and have been revealed to be the most abundant memory T cell population in various tissues. They can be activated in the local microenvironment by infection or tumor cells and rapidly clean them up to restore homeostasis of local immunity in gastrointestinal tissues. Emerging evidence has shown that tissue-resident memory T cells have great potential to be mucosal guardians against gastrointestinal tumors. Therefore, they are considered potential immune markers for immunotherapy of gastrointestinal tumors and potential extraction objects for cell therapy with essential prospects in clinical translational therapy. This paper systematically reviews the role of tissue-resident memory T cells in gastrointestinal tumors and looks to the future of their prospect in immunotherapy to provide a reference for clinical application.
1 Introduction
Immunotherapy has developed rapidly in the past few years and has become one of the first-line treatment strategies in gastrointestinal tumors, of which immune checkpoint inhibitors (ICIs) are the representative drug and have achieved promising therapeutic effects by activating the exhausted immune cells. T cells, especially CD8+ T cells, are the main effector cells of the anti-tumor immune response. Memory T cells are the main T cell subsets that exert anti-infection and anti-tumor immune response due to their contact with infection and/or tumor antigens (1). These cells can form long-term memory on tumor-specific antigens, which makes them reliable candidates as a target for anti-tumor therapy. Memory T cells have two subsets: circulating memory T cells and tissue-resident memory T (Trm) cells. In gastrointestinal tissues, Trm cells reside in local tissues, survive for a long time, self-renew, and initiate a rapid response when encountering infection antigens and tumor antigens. At the same time, Trm cells are also considered to be the main population responding to PD-1 inhibitors, playing an important role in immune therapy (2, 3). Therefore, a systematic review of the role of Trm in gastrointestinal tumors will provide an important theoretical basis for better understanding how it plays a positive anti-tumor immune response and optimizes existing immunotherapy strategies.
2 Overview of tissue-resident memory T cells
2.1 Phenotype of tissue-resident memory T cells
Naive T cells can differentiate into memory T cells after being stimulated by antigens. Circulating memory T cells include central memory T cells (Tcm) and effector memory T cells, circulating from circulation, secondary lymphoid organs, and in some situations non-lymphoid tissues (4). Tissue-resident memory T cells were first found in the intestinal mucosa, but were once considered as a special subset of Tem cells (5). Gebhardt et al. later confirmed and officially named this type of memory T cell subset in the skin (6), and now tissue-resident memory T cells have been found in almost all human tissues, including lymph nodes (7). Tissue-resident memory T cells and circulating memory T cells have different phenotypes, molecular characteristics and functions (8) (Figure 1): Tcm is relatively static, and mainly exists in lymphatic tissues, highly expressing lymphoid homing receptor. Tcm can be activated when stimulated by antigens, and then proliferate and differentiate into other types of memory T cells (Figure 1). Compared to Tcm, Tem mainly exists in non-lymphoid tissues or tissues with inflammation. Mostly, Tcm differentiates into Tem under stimulation. Interestingly, it has been revealed that a small number of Tem cells can differentiate into Tcm cells to maintain stability and its durable effects in the absence of antigen stimulation (1). It used to be thought that Trm cells steadily reside in local tissues to realize rapid immune response to the antigens, with typical characteristics of low homing-related molecule expression and high expression of tissue-resident-associated molecules like CD69 and CD103 (6, 9, 10). Hartana et al. afterwards found a small number of Trm cells in the peripheral blood of healthy people (11), indicating that Trm cells might enter peripheral blood. Some Trm cells can upregulate the expression of molecules such as CD36 to move out of the tissues (12). Beura et al. (13) found that Trm cells in non-lymph node tissues can migrate to lymph nodes, thus increasing the accumulation of antigen-specific Trm cells in lymph nodes, especially in draining lymph nodes. Fonseca et al. (14) also found that the reactivated Trm can re-enter the circulating pool called “ex-Trm”. And these ex-Trm cells retain strong affinity for the original tissue. Fonseca et al. pointed out that Trm cells have certain developmental plasticity and can regenerate Tcm cells and Tem cells, which allows outside-in immune responses. Behr et al. (15) proved this result and they also found that ex-Trm-derived cells showed a higher protective electrical potential than their non-ex-Trm-derived counterparts. Thus, Trm cells retain developmental plasticity and shape both local and systemic T cell responses.
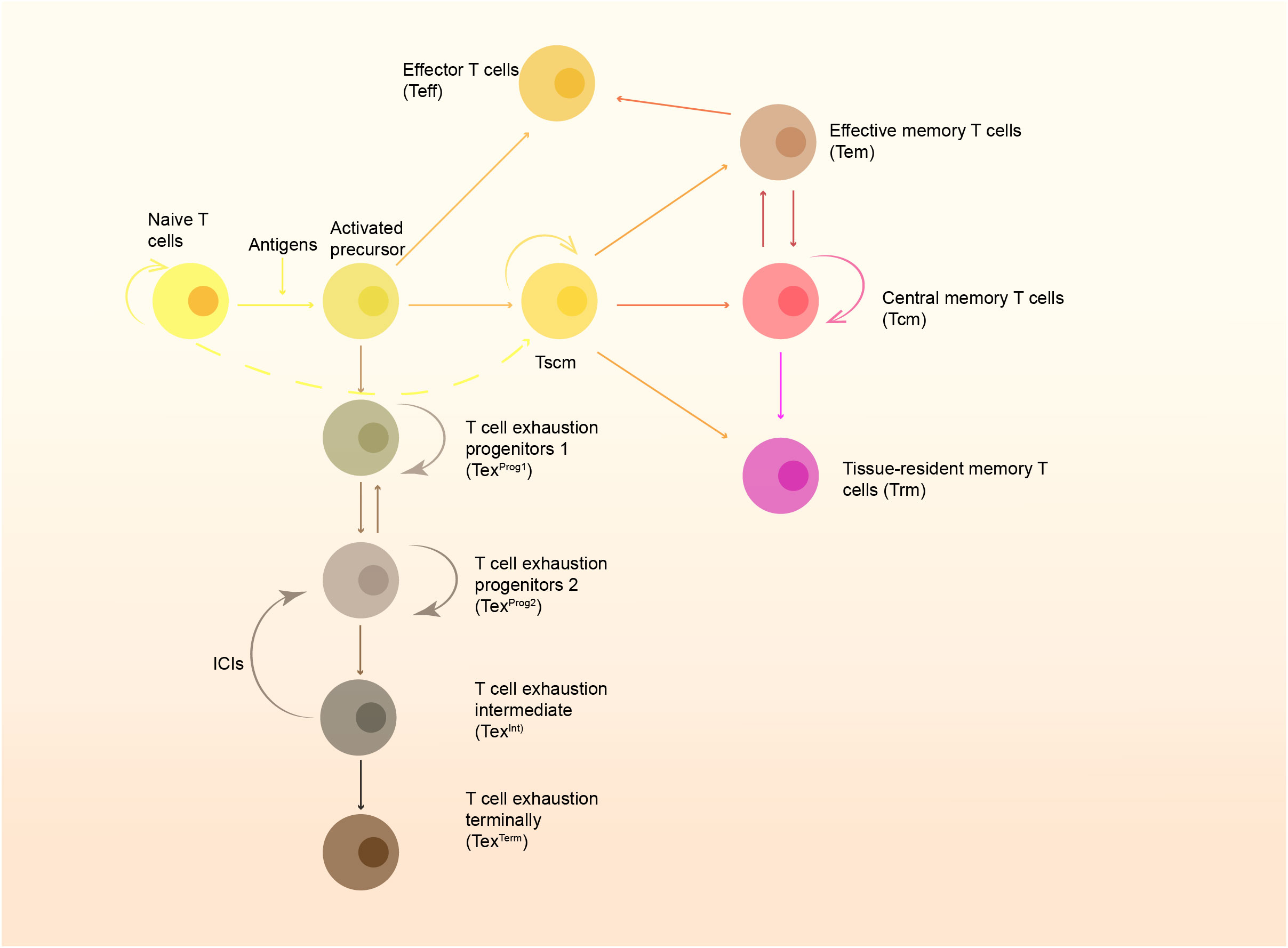
Figure 1 The formation of memory cells and the T cell exhaustion pathway. Naïve T cells are stimulated by antigens presented by APC and develop to active precursors, which further differentiate into effector T cells (Teff) and stem-like memory T cells (Tscm). Tscm can further develop to central memory T cells (Tcm), effector memory T cells (Tem) and tissue-resident memory T cells (Trm). Some of them can also become exhausted gradually, and restoration of function of these cells rely on immune checkpoint inhibitors (ICIs).
The molecular characteristics of tissue-resident memory T cells generally include high expression of CD103, CD69, and CD49a, as well as low expression of CCR7 and S1PR1, among which the down-regulation of S1PR1 is considered as the precondition for tissue residency of Trm (16). T cells have been found to circulate into the blood in response to sphingosine-1-phosphate (S1P). However, CD69 play an antagoinistic role for S1PR1 (17) by binding to S1P interferes with the transmembrane region of S1PR1 and promoting S1PR1 internalization (18). Joint regulation of CD69 and other important transcriptional pathways (described below) eventually leads to the low expression and activity of S1PR1 in Trm, which can’t respond to S1P. The synergistic effect of low CD62L and high CD69 expression is to prevent the lymphatic homing and migration of Trm cells, which is one of the critical factors for Trm to settle in tissues. Thus tissue-resident memory T cells can also be simply defined as CD62L-CD69+ T cells to distinguish them from circulating memory T cells. Similarly, low expression CD62L and CCR7 effectively prevent T cells from migrating through the vascular wall (8, 19–21).
In addition, CD103 and CD49a are considered key tissue residence molecules (20). CD103 can interact with E-cadherin on the surface of epithelial cells to increase the aggregation of Trm in tissues, and induces the response of tissue-resident memory T cells to chemokines (22). Although some tissue-resident memory T cells do not express CD103, molecules with similar effects to CD103 were found in these CD103- Trm cells: in liver, CD103-Trm cells can adhere to the hepatic sinusoid epithelium by LFA-1, enabling tissue retention (23). In addition to helping T cells to reside in peripheral tissue, CD69 and CD103 played a significant role in the formation and survival of Trm (24). CD103 may also be related to lipid metabolism by inducing CD36, CD103hiTrm often has a higher expression level of CD36 and up-regulation of lipid-related metabolic pathways than CD103loTrm (25). CD49a, the very late antigen-1(VLA-1), also known as integrin α1, can form a complex with CD49d that achieves adhesion to epithelial cells through its ligands, while this adhesion can allow Trm to migrate along the epithelial basement membrane. CD49a promotes the survival of Trm and is involved in the regulation of IL-15-induced production of granzyme and perforin to maintain the functional stability of Trm (20, 26, 27). Cytokines in the immune microenvironment, such as IL-2/IL-12/IL-15/IL-7, regulate CD62L and S1PR1 and play an essential role in the formation, survival, function maintenance, and regeneration of Trm in tissues (Figure 2).
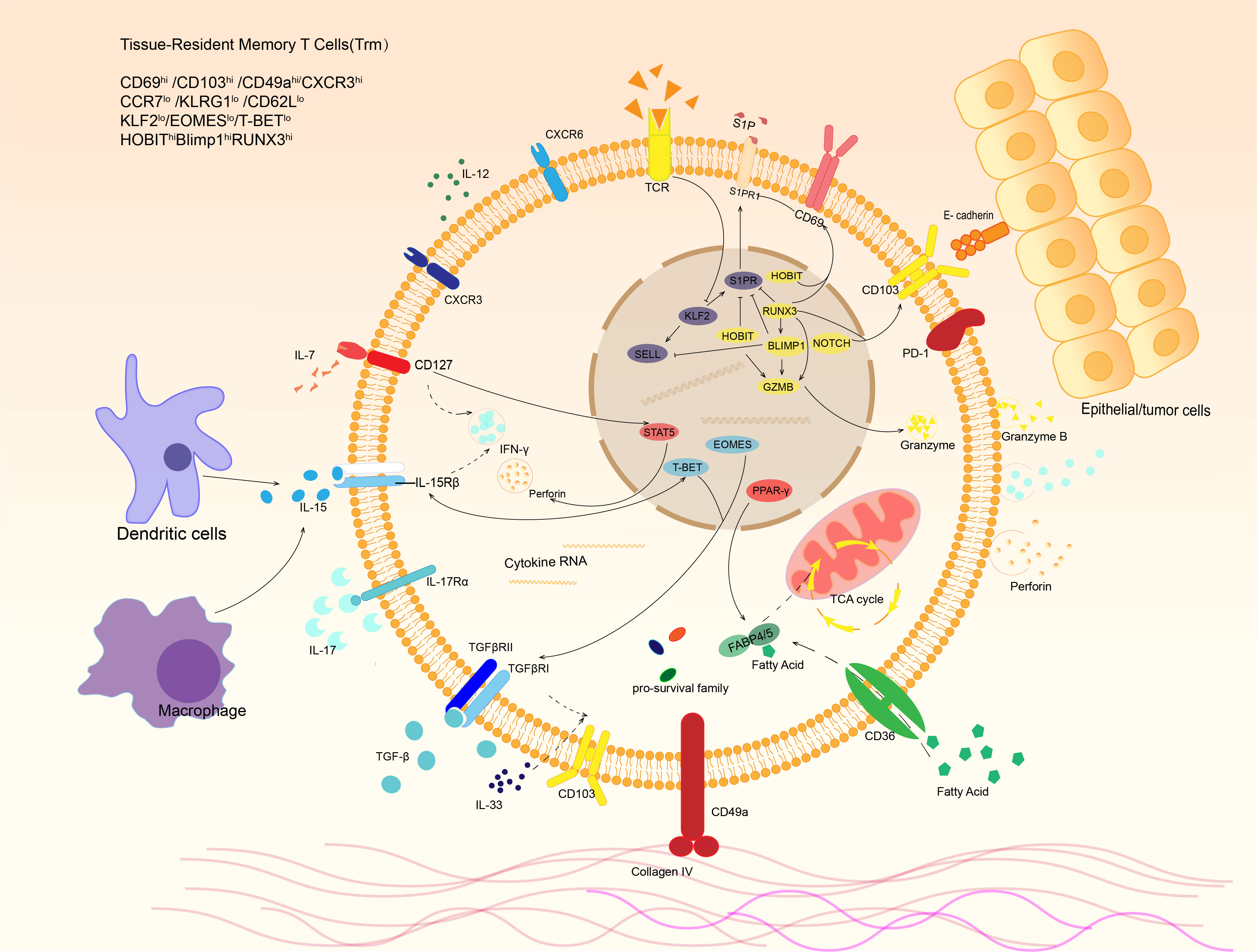
Figure 2 Phenotypes and signaling pathways of tissue-resident memory T cells. Trm cells express distinct phenotype under stimulation of various cytokines in tumor microenvironment. For example, dendritic cells and macrophages can release IL-15, which binds to IL-15 receptor and activates transcription factor T-bet, resulting in IFN-γ expression of Trm. In response to IL-7 in tumor microenvironment, STAT5 is activated and leads to IFN-γand perforin production. E-cadherin expressed by other cells and extracellular matrix like collagen IV are also able to bind the receptors expressed on Trm surface. After that, they release a variety of cytokines to kill targeted cells and regulate local immune microenvironment.
With the development of sequencing technology, the heterogeneity of Trm has been revealed, which helps us better understand how Trm cells play the role in local tissues. As mentioned above, CD103 is essential for Trm residence and maintenance. CD103+ Trm cells are the main force in gastrointestinal tissues to fight against infection and tumors, while CD103- Trm cells have a more unique function. For instance, in the intestine, CD103+CD69+CD8+ Trm cells possess high ability to produce inflammatory cytokines, while CD103-CD69+CD8+ Trm cells have a high expression of β2-integrin and are more able to produce granzyme (28). Besides, researchers have found that CD103- Trm can differentiate into CD103+ Trm to mediate immune response towards secondary infections, instead of the primarily resident CD103+ Trm (29, 30). Besides the different function, various subsets of Trm cells, including CD103+CD69+, CD103-CD69+ and CD103-CD69- Trm cells, have shown great potential to proliferate. Further exploration
Transcription factors involved in Trm formation include high expression of Runx3, Hobit, Blimp1 and Notch, with low expression of T-bet, Eomes and Klf2. In addition, Trm also expresses pro-survival family members such as Bcl-2 and various anti-apoptotic factors (19, 21, 31). The expression of Runx3 is considered a key factor for the formation of Trm. Overexpression of Runx3 during thymic T cell development promotes the formation of CD8+T cells and is also an important factor in the induction of CD103 (32). And effector T cells in tissues tend to have higher Runx3 expression (33). Runx3 can promote the expression of tissue-residency genes and molecules (CD69, Blimp-1, etc.) and suppress genes related to tissue egress and recirculation (T-bet, S1PR1, Klf2, etc.) (34, 35). Blimp-1, one of the downstream targets of Runx3, can also regulate the expression of S1PR1 and CD62L directly or indirectly through Klf2 and Sell, while Hobit, a homolog of Blimp-1, has a similar function (20, 21, 31, 36). Notch can also promote the expression of CD103 (37). In contrast, TCF-1, a transcription factor maintaining CD8+ T cell stemness, can repress CD103 expression by directly suppressing the transcription and inhibiting TGF-β-induced CD103 expression, which restricts Trm development (38). Hobit may maintain Trm production in the gut and skin in the absence of Blimp-1 (36). The downstream targets of Notch are solute carriers of nutrients, including amino acids, indicating that Notch helps maintain the nutritional metabolism of Trm (39). In addition to regulating the formation of tissue-resident phenotypes, these transcription factors also control the cytotoxicity of Trm. Runx3 promotes the differentiation of cytotoxic T lymphocytes (CTLs) and inhibits terminal differentiation, ensuring the development, growth, and longevity of CTLs (34). At the same time, Runx3, together with Blimp-1, Hobit, T-bet and others, regulates the production of granzyme B and/or perforin through its complex regulatory pathway (40). It is worth noting that Hobit is superior to Blimp-1 in long-term expression of granzyme (41). Eomes was thought to suppress Trm cell formation, but a recent study revealed that Eomes was essential for Trm maintenance in the intestine but not the colon (42). Thus, various expression of transcriptional factors determines heterogeneity and existence of Trm cells, which can be altered in case of tumor or infection. Focusing on the alteration may help us better to understand the phenotype and function changes of Trm cells and provide more ideas to regulate these cells to play an anti-tumor role.
2.2 Anti-tumor effect of tissue-resident memory T cells and T cell exhaustion
Trm cells have been confirmed to monitor of local immunity and play an important role in infectious diseases (6, 19, 43–45) and autoimmune diseases (46, 47). The significant negative correlation between ITGAE (encoding CD103) and CD69 gene expression and molecular markers of glioblastoma (48) suggests that CD8+Trm cells may have an anti-tumor effect, as demonstrated by studies in other tumors (9, 44, 49, 50). At the same time, tissue-resident memory T cells are also a key target for the regulation of immune-checkpoint blockade (ICB) therapy (22, 51, 52). The anti-tumor effect of Trm is manifested in two aspects: 1. Trm mediates local immunity, and 2. It kills tumor cells by toxicity (53). Activated Trm can identify the tumor-associated antigens and secrete cytotoxic granular proteins, CCL3, CCL4, CCL5 and other chemokines, as well as pro-inflammatory cytokines such as interferon-γ (IFN-γ) and tumor necrosis factors (TNF) (53, 54). The anti-tumor effect of tissue-resident memory T cells is closely related to their phenotypic molecules (Table 1). The interaction between CD103 and cadherin protects the survival of Trm in tumor tissues, and also causes the bidirectional signal of T cell receptor (TCR) [24], and induces TCR-dependent tumor antigen-specific killing effect. The release of TNF-γ can promote the up-regulation of vascular adhesion molecule 1(VCAM-1), recruit CD8+ circulating memory T cells and B cells, and further induce and promote local immune response (22, 43). In the mouse melanoma model, the defect or inhibition of CD103 manifests as the immune system’s imbalance in regulating tumor growth. Compared with the CD103 wild type mouse, the melanoma in the CD103 deficient mouse is large and grows faster (67). In addition, the cells with high CD103 expression are often accompanied by the expression of CD69 and PD1. CD103+Trm also has higher cytotoxicity than CD103-Trm (57, 58, 61). CD49a has a similar effect to CD103. After using CD49a inhibitors, the mice in the experimental group also showed uncontrolled tumor growth (56).
The function of tissue-resident cells is regulated by other immune cells. Dendritic cells promote the differentiation and formation of tissue-resident memory T cells (68) and play a protective role in tumors. The interaction between CXCR6+Trm and CXCL16+APC is important for maintaining tumor immunity (69). However, some immune cells play a negative regulatory role, tumor-associated fibroblasts (CAFs) are essential in tumor microenvironment and have complex relationships with tumor cells and TILs. CAFs can inhibit Trm activity by upregulating the expression of PD-1 by cytokines, and increase the risk of lymphatic metastasis (70). In addition, Treg cells can inhibit Trm cell immune activity and promote tumor growth, and they have more significant infiltration in the tumor, especially tumor matrix (71, 72). These two immune cell subsets can work together with macrophage type 2 to form an immune barrier against anti-tumor immune response by Trm (72).
Trm has an excellent anti-tumor effect, but like other T cell subsets, it will gradually lose its immune activity and convert into exhausted T cells (Tex) when continuously stimulated by antigens and/or inflammatory factors. According to the expression of Ly108 and CD69, T cells are divided into four stages (Figure 1). The intermediate Tex subset (TexInt) will gradually lose its cytotoxicity and proliferation capacity. However, T cells at this stage can regain their cytotoxic effect after PD-1 blockade. Once the cells enter the terminally exhausted subset, T cells will no longer respond to immune checkpoint inhibitors (ICIs) (73). It is now generally accepted that exhausted T cells are characterized by low antigen-stimulated proliferation, high expression of multiple immune checkpoints and progressive loss of T-cell effector function. Thus, immune checkpoints are also known as exhausted-related molecules (74). Trm cells also express multiple immune checkpoints, such as PD-1, TIM-3, and CTLA-1, etc. Interestingly, although Trm cells have multiple immune checkpoint molecules, many studies have shown that their function is not exhausted (11, 75). On the contrary, the functional Trm is more powerful and metabolically active than Tex (64). But it is shown in multiple tumor models that Trm is a group of cells with the trend of exhaustion (9, 11, 53, 64, 75). Zheng et al. (76) performed single-cell sequencing on a variety of tumor-infiltrating T cells, including gastric cancer and esophageal cancer, and found that the exhausted T cells were mainly from effector memory T cells and tissue-resident T cells. Recent study has found that the exhaustion of CD8+ T cells is accompanied by an increase in the number of Tc17 cells with poor cytolytic capacity (77).The scRNA-seq of gastric tumor also showed Tc17 exhaustion pathway originating from Trm (78). Another single-cell sequencing results showed that Trm cells could be divided into three subsets: Hobit-enriched CD103hiPD-1loTrm, Granzyme K-enriched CD103loPD-1hi Trm, and CD103hi PD-1hiCTLAhiLAG3hiTIGIThi Trm, while the last subset is considered as exhaustion-related subtype (79). Milne et al. also identified subsets of memory T cells with resident gene expression characteristic of progenitor exhaustion T (Tpex) cell (Id3hiBlimp1lo) and terminal exhaustion (80), and this study indicates that there exists a complete developmental pathway to exhaustion in Trm. In general, Trm cells may keep their function with the expression of immune checkpoints, but they still can convert into exhausted T cells. The differentiation pathways and intrinsic mechanism remain unclear, and the exploration to the pending issue may provide novel strategies for anti-tumor therapy.
Dysfunction of T cells in the advanced tumor microenvironment is related to tumor immune escape. Once it happens, the number of T cells expressing either high-level or low-level cytotoxic granulosa proteins is significantly reduced and the exhausted-related molecules are significantly overexpressed in tumor microenvironment (TME) (57). Studies have pointed out that non-terminally Trm-Tex dynamic is related to tumor prognosis (63, 64, 76). And the population with high expression of cell cycle-related genes, though less, is likely to be related to the tumor-associated antigen (TAA) (81), and replenish effector Trm cells as they become exhausted (81, 82)
3 Tissue-resident memory T cells in gastrointestinal tumors
3.1 Tissue-resident memory T cells in gastric cancer
In normal gastric tissue, lamina propria mononuclear cells (LPMCs) have a tissue-resident phenotype, with more than 70% of LPMC CD8+ T cells co-expressing CD103 and CD69, and nearly 20% expressing CD103 alone (83). CD69+CD103+ Trm also accounts for approximately 30% of TILs in the TME of gastric adenocarcinoma with high expression of PD-1, TIGIT, and CD39 (49). Like most solid tumors, Trm cells in gastric cancer have significant spatial heterogeneity. In gastric cancer tissues, more GZMB- Trm cells can be seen, and CD103- T cells infiltrate more in the tumor matrix, while CD103+ Trm cells appear more excessively in the tumor epithelium. Various types of Trm are decreased in the tumor matrix with lower activity and function (51). Besides, the infiltration of CD103+CD8+ Trm cells in advanced gastric cancer is also less than in early gastric cancer (57).
It has been found that development of gastric cancer is associated with dysfunction or declined abundance of Trm (84–86). Trm cells in the tumor microenvironment ingest free fatty acid (FFA) as the main energy source mainly through CD36-FABP4/FABP5 related metabolic pathways (25). However, gastric cancer cells also have up-regulated CD36 and lipid metabolism (87). Aoki (88) and Pan (87) found high expression of CD36 may be associated with peritoneal metastasis of gastric cancer. Furthermore, this suggested that there might be a competitive relationship on lipid uptake between gastric cancer cells and Trm. Recently, Lin et al. (49) revealed that gastric cancer cells deprive Trm of fatty acids through more aggressive competitive uptake, which leads to Trm death. They further found that blocking PD-1/PD-L1 reduced lipid metabolism of gastric cancer cells, but enhanced the lipid uptake and lipid metabolism of Trm cells.
In recent years, tertiary lymphoid structure (TLS) has been found to be associated with the prognosis or development of tumors. TLS is associated with survival and clinical outcomes in patients with immunotherapy for tumors and can serve as a potential therapeutic target for tumors (89, 90). And TLS is also associated with a better prognosis for gastric cancer. Interestingly, Mori et al. found that CD103+CD8+Trm cells were found in and around TLS, which could secrete high levels of cytolytic enzyme and IFN-γ, and IFN-γ was considered to have the function of promoting TLS formation. Consistently, patients with CD103 overexpression also tend to have more abundant TLS. Patients with CD103hiTLShi also had a better therapeutic response to nivolumab (86, 91).
In addition to the susceptibility and immune microenvironment changes caused by genetic susceptibility, pathogenic microbial infection, and microbiome alterations also play an important role in the occurrence and development of gastric cancer and affect the function of TILs. Gastric cancer is one of the few tumors related to infection, Helicobacter pylori (H. pylori.) and Epstein–Barr virus (EBV) are the main infection-related factor (92–94). H. pylori can inhibit the CD8+ CTLs by myeloid-derived suppressor cells (MDSCs) and induce the up-regulated expression of PD-L1 of tumor epithelial cells and macrophages, thereby inducing the resistance of gastric cancer to ICB therapy against PD-1/PD-L1 (95, 96). Xu et al. have shown that the induction of Trm proliferation and differentiation can enhance the local immune response to H. pylori (97). A recent study has shown that CagA-specific CD8+ Trm cells can infiltrate the gastric mucosa and control the H. pylori. infection (98). EBV infection can induce DNA demethylation of host cells, which results in expression of tumor suppressor genes, loss of tumor-related antigens, and over-expression of immune checkpoint like PD-L1 and PD-L2 and induces immune evade of Epstein–Barr virus-associated gastric cancer (EBVaGC) by PD-1/PD-L1 interactions between tumor cells and T cells (99, 100). The typical characteristics of the gastric cancer microbiota were the flora change and the decrease in microbial diversity. The mouse model showed that enhanced Methylobacterium in gastric cancer promoted tumor progression by reducing the infiltration of TGF-β+CD8+ Trm and was related to the exhaustion of Trm (101). Similarly, Yang et al. (102) showed higher CXCL13 production in CD8+ Trm, which contributed to TLS formation.
3.2 Tissue-resident memory T cells in colorectal cancer
In normal intestinal tissue, the proportion of CD69+CD103+CD8+ T cells in CD8+ T cells can even exceed 80% (28). However, they have significant functional differences in the lamina propria and epithelial layer. The tissue-resident memory T cells have active pathways that regulate cell survival and cytokine signal transduction in the lamina propria with higher expression of Runx3,NR4A2,ICOS and LITAF, while they show more obvious cytotoxic characteristics in the epithelial layer with a higher level of GZMM,LTB,GZMA and CXCR3 (103). These CD69+CD103+ Trm cells have been found to highly express CD161 and CD127 (IL-7R), which help maintain T-cell cytotoxicity. In addition, IL-7 can restore the activity of CD8+ T cells by reducing the expression of PD-1 (104).
The immune microenvironment regulates the function of CD8+ T cells and the formation of CD8+ Trm: TGF-β can inhibit the migration of CD8 T cells, which, together with IL-33, can promote T cell differentiation into Trm (105), TGF-β can induce the synthesis of CD103 and CD69 molecules in the maintenance phase of Trm cells (22, 106). Once TGF-β is blocked, the abundance of Trm cells can significantly decrease (62). Paradoxically, TGF-β has two sides to tumor immunity, and it can regulate the expression of tumor cell cycle-related proteins and inhibit cell cycle from exerting anti-tumor effects in the early stage of cancer. It becomes a spy for immune responses to advanced tumors. When the tumor enters the advanced stage, the tumor cells can secrete a large amount of TGF-β to damage T cell mitochondrial respiration and inhibit the production of IFN-γ, thereby reducing T cell activity and helping the tumor escape through the Treg cell pathway (107, 108). IL-7 appeared to inhibit the negative effects of TGF-β on suppressing Trm development (104). Intraepithelial lymphocytes (IELs) are activated by IL-15 (109), and IL-15 can mediate their proliferation and survival, and regulate the expression of IFN-γ (110, 111). IL-15 can also regulate the growth and apoptosis of colorectal cancer tumor cells, and low level of IL-15 is also associated with low-infiltrating lymphocytes in tumor microenvironment and poor prognosis of the colorectal cancer (112). Desboi et al. (113) found that IL-15 hyper-agonist receptor-linker -IL-15 (RLI) could limit tumor growth in the early stage of colorectal cancer and the combined use of PD-1 inhibitors had a higher activation rate of CD8+ TILs in colorectal cancer compared with PD-1 inhibitors or RLI alone, but had no significant inhibition or CD8+ TILs activation for advanced colorectal cancer, which was consistent with T cell exhaustion.
The prognosis of colorectal cancer (CRC) is also related to its subtypes. Microsatellite instability (MSI) is considered as one of the main carcinogenic pathways of colorectal cancer and can be used as a prognostic marker. According to the mismatch repair (MMR) and MSI, RCRs can be divided into high microsatellite instability RCRs (MSI-H)/mismatch repair system defective CRC (dMMR), low microsatellite instability RCRs (MSI-L) and microsatellite stability RCRs (MSS). Colorectal patients with MSS/MSS-L have a worse prognosis and respond to targeted PD-1/PD-L1 or CTLA-4, while the sensitivity to ICIs and prognosis of those with dMMR/MSI-H are significantly better (114–116). Compared with normal intestinal mucosa or MSS-RCR tissue, CD8+ T cells have more significant enrichment in MMR-deficient CRC tissue (117, 118). Studies have found that CD8+ Trm in colorectal cancer patients has specific demethylation of CD103 and CD39-related genes (65), CD103+CD39+ T cells have a strong MHC-dependent tumor killing ability, and the co-expression of CD103 and CD39 is also considered to be the key to T cell tumor-specific recognition characteristics and differentiation from bystander T cells, and can be used as an independent risk factor for the prognosis of colorectal cancer (65, 119).
CRC is associated with environmental factors and earlier onset age has been observed among these patients (120, 121). In most cases, the adenoma-carcinoma-metastasis process is experienced, which is associated with the accumulation of specific genetic events of “APC-KRAS-TP53”. Particular case was reported that the lung metastases in a patient with metastatic colorectal cancer disappeared or shrank after autologous isolation and culture of CD8+ T cells that had a specific effect on the mutant KRAS gene, and were still in a clinically disease-free state four months after surgery (122), which indicated the effectiveness and broad prospect of Trm as an as a potential target of colorectal cancer adoptive cell therapy.
4 Application of tissue-resident memory T cells in gastrointestinal tumors
4.1 Prognostic value and ICIs response of tissue-resident memory T cells
Tissue-resident memory T cells are associated with the prognosis of a variety of tumors (Table 1). In general, a high proportion of CD8+ Trm predicts better survival, and has better prognostic value than the total number of CD8+ T cells. Further studies show that non-terminal tissue-resident memory T cells may be more important (63); and it has also been found in ovarian cancer that its immunogenicity is determined by fewer stem cell-like tissue-resident memory T cells (82). Tissue-resident memory T cells, perhaps the highest tumor reaction potential T cells, are important participants in the anti-tumor effects, and can respond to ICIs in the early stage (123, 124). PD-1 blockade can significantly proliferate tissue-resident memory T cells with an enhancement of anti-tumor effect (2), and it also promotes the migration of Tcm into the tumor microenvironment and differentiation into Trm (125). Single-cell sequencing analysis also showed significant up-regulation of tissue presence-related phenotypes after blocking PD-1 (9). Tumor microenvironment in patients with better ICIs responses tends to be more Trm-rich (55), and it has been proved that highly infiltrated tissue-resident memory T cells can be used as a marker of benign ICB therapeutic response in colorectal cancer (65, 66), breast cancer (9, 50), melanoma (55) and other tumors (60). In addition, tissue-resident memory T cells can be highly enriched in the tumor microenvironment after chemoradiotherapy (126) and are associated with a good prognosis for patients who receive doublet chemotherapy after surgery (59), suggesting that ICIs combined with adjuvant chemoradiotherapy can achieve better anti-tumor effects. In fact, Phase III clinical trials have shown that Nivolumab combined with neoadjuvant chemotherapy significantly improved the overall survival and progression-free survival of patients with gastric cancer (127).
4.2 Enhancing activity and T cell therapy of tissue-resident memory T cells
Given the excellent anti-tumor effect and potential prognostic value, it is worth exploring how to make better use of tissue-resident memory T cells for anti-tumor therapy. And it also implies a better anti-tumor environment in the gastric cancer microenvironment because of a lower proportion of terminal-exhausted CD8+ T cells and a higher proportion of CD8+ Trm in the gastric cancer microenvironment (76). Enhancing the proliferative capacity and cytotoxicity of Trm may be a better option for gastrointestinal tumor immunotherapy by the combination of 4-1BB co-stimulation or RLI with PD-1 blockade at this stage (57, 113).
CD8+ T cells can be induced to express CD103 by reprogramming tumor-infiltrating DCs with β-glucan gel polysaccharide. CD103+CD8+ T cells generated in this way could control the growth of existing tumors (128). In addition, it has been reported that a patient with locally recurrent gastric cancer was relieved by injecting DCs into tumor for 30 months (129). HBV-specific tumor-resident T cells correlated with relaxation-free survival of hepatocellular carcinoma (63), considering the relationship between gastric cancer and infection factors, as well as the particularity of gastrointestinal flora, the induction of infection-derived specific tissue-resident memory T cells by vaccine may have exploratory significance for preventing the occurrence of gastrointestinal tumors or intervening in their development (130). Induction the formation of Trm via cancer vaccines is also conceivable (62, 131, 132)
Autologous tumor infiltrating lymphocytes adoptive therapy has observed objective regression or even complete remission of tumors in melanoma (133) and breast cancer (134) with objective tumor regression, or even complete remission. As an important subset of TILs, Trm may be able to undertake this role better. Anada and Matthewd’s research showed that the expression of Runx-3 promoted the formation of tissue-resident memory T cells, and they use melanin model revealed that the metastasis and localization of CD8+ T with high expression of Runx-3 in TME inhibited the growth of tumor (35). As mentioned above, a part of recyclable tissue-resident memory T cells does exist in the human body (14, 15), which indicate that constructing T cells by inducing or coercing the expression of genes related to tissue retention may be one of the feasible pathways for cell therapy.
Although adoptive T cell therapy has made great achievements and shown great potential, how to select more tumor antigen-specific T (Tas) cells or induce the expansion of Tas cells is still a challenge. The lack of tumor antigen specificity of CAR-T increases the potential risk of targeted tumor removal toxicity, and even serious side effects (135). As mentioned above, tissue-resident memory T cells in the gastrointestinal tumor microenvironment have a significant portion co-express CD39 and CD103 (49, 65), which is considered to be a marker of tumor antigen-specific response in solid tumors (119). In addition, the TCR of Tas cells in patients is a more personalized choice of TCR-T cell therapy. A transcriptomic profiles of neoantigen-reactive T cells from gastrointestinal tumors also revealed that most neoantigen-reactive cells co-express CXCL13 and GZMA (136). He et al. proved that CXCL13 is a unique marker of Tas cells and they found ENTPD1 (CD39) can be used as a surface marker of CD8+ Tas cells. Moreover, TCR-T cells expressing TCR from Tas cells show significant therapeutic effects on autologous patient-derived xenograft (PDX) tumors from autologous patients (137). Therefore, rational utilization of the tissue-resident property of CXCL13+ Trm may lead to more precise targeted tumor therapy. Since it is relatively difficult to extract Trm in local tissues, Trm in tumor draining lymph nodes would also be a good alternative. Trm cells have been proved to exist in not only non-lymphoid tissues but also lymphoid tissues, especially lymph node (7), and infection-associated antigen-specific CD8+ Trm within the lymph nodes can be migrated from non-lymph node tissue (13). Recent studies also showed that tumor-specific CD8+ Trm existed in draining lymph nodes and could prevent the spread of melanoma in the lymph nodes (138). Hence, the tumor-specific Trm located in lymph node can not only prevent tumor cells spreading, but also can be an alternative option for extraction and TCR repertoire recognition and following TCR-T transformation.
5 Concluding remarks
Immunotherapy for gastrointestinal tumors remains a worldwide challenge. Immunotherapy for cancer aims to identify and eliminate tumor cells by stimulating T cells with tumor-killing effects to reach the tumor microenvironment. Tumor-infiltrating tissue-resident T cells can be used as a potential target population of ICIs, and it is also a biomarker for the prognosis of gastrointestinal tumors and PD-1 blocking effect. The phenotype and function of tissue-resident memory T cells implicate their potential therapeutic value. First, Trm resides in peripheral non-lymphoid tissues, and the reoccurring immune response is much faster than that of circulating memory T cells, thus enabling Trm to participate in immune regulation of tumor more quickly. Second, CD8+ Trm can secrete a variety of cytokines to promote tumor cell lysis and death, and the related phenotypic molecules can also exercise a regulatory effect on tumor growth. Therefore, we judged that the tissue-resident memory T cells could be potential extraction targets for adoptive T cell therapy. However, as we have mentioned repeatedly above, T cell exhaustion is closely related to the function of Trm and immune escape of tumor and TexTerm has lost its immune activity. Besides, tumor tissues also contain T cell populations without tumor immune function such as bystander T cells. Therefore, in order to better utilize Trm for anti-tumor, we need more details how Trm cells’ functions are restricted by tumor and find out the cell populations with tumor antigen specificity and long-term stable cytotoxic effect among numerous subsets or produce effective targeted anti-tumor T cells by cell technology to intervene in the exhaustion of CD8+ Trm by certain means and implement more accurate regulation for this crimp.
Author contributions
ML, XW: Writing - original draft. DC: Figure drawing. XS and WG: Conceptualization, Writing – review & editing, funding acquisition. All authors contributed to the article and approved the submitted version.
Funding
This work was supported by National Natural Science Foundation of China (81970500 XS, 82172645 WG), Start-up funding for the introduction of talents in Nanjing Drum Tower Hospital (RC2022-015).
Conflict of interest
The authors declare that the research was conducted in the absence of any commercial or financial relationships that could be construed as a potential conflict of interest.
Publisher’s note
All claims expressed in this article are solely those of the authors and do not necessarily represent those of their affiliated organizations, or those of the publisher, the editors and the reviewers. Any product that may be evaluated in this article, or claim that may be made by its manufacturer, is not guaranteed or endorsed by the publisher.
Abbreviations
MSI, Microsatellite instability; CRC, Colorectal cancer; CTLs, Cytotoxic T lymphocytes; Trm, Tissue-resident memory T cells; Tcm, Central memory T cells; Tem, Effector memory T cells; Tex, Exhausted T cells; Tas, Tumor antigen-specific T cells; TAA, Tumor-associated antigen; TCR, T cell receptor; IELs, Intraepithelial lymphocytes; MDSC, Myeloid-derived suppressor cells; S1P, sphingosine-1-phosphate; TLS, Tertiary lymphoid structure; ICI, Immune checkpoint inhibitor; ICB, Immune-checkpoint blockade; VLA-1, Very late antigen-1; FFA, Free fatty acid; EBVaGC, Epstein–Barr virus-associated gastric cancer.
References
1. Muroyama Y, Wherry EJ. Memory T-cell heterogeneity and terminology. Cold Spring Harb Perspect Biol (2021) 13(10):a037929. doi: 10.1101/cshperspect.a037929
2. Pizzolla A, Keam SP, Vergara IA, Caramia F, Thio N, Wang M, et al. Tissue-resident memory T cells from a metastatic vaginal melanoma patient are tumor-responsive T cells and increase after anti-PD-1 treatment. J Immunother Cancer (2022) 10(5):e004574. doi: 10.1136/jitc-2022-004574
3. Han L, Gao Q-L, Zhou X-M, Shi C, Chen G-Y, Song Y-P, et al. Characterization of CD103 CD8 tissue-resident T cells in esophageal squamous cell carcinoma: May be tumor reactive and resurrected by anti-PD-1 blockade. Cancer Immunol Immunother CII (2020) 69:1493–504. doi: 10.1007/s00262-020-02562-3
4. Sallusto F, Geginat J, Lanzavecchia A. Central memory and effector memory T cell subsets: function, generation, and maintenance. Annu Rev Immunol (2004) 22:745–63. doi: 10.1146/annurev.immunol.22.012703.104702
5. Masopust D, Vezys V, Marzo AL, Lefrançois L. Preferential localization of effector memory cells in nonlymphoid tissue. Sci (New York NY) (2001) 291:2413–7. doi: 10.1126/science.1058867
6. Gebhardt T, Wakim LM, Eidsmo L, Reading PC, Heath WR, Carbone FR. Memory T cells in nonlymphoid tissue that provide enhanced local immunity during infection with herpes simplex virus. Nat Immunol (2009) 10:524–30. doi: 10.1038/ni.1718
7. Schenkel JM, Fraser KA, Masopust D. Cutting edge: resident memory CD8 T cells occupy frontline niches in secondary lymphoid organs. J Immunol (2014) 192:2961–4. doi: 10.4049/jimmunol.1400003
8. Martin MD, Badovinac VP. Defining memory CD8 T cell. Front Immunol (2018) 9:2692. doi: 10.3389/fimmu.2018.02692
9. Savas P, Virassamy B, Ye C, Salim A, Mintoff CP, Caramia F, et al. Single-cell profiling of breast cancer T cells reveals a tissue-resident memory subset associated with improved prognosis. Nat Med (2018) 24:986–93. doi: 10.1038/s41591-018-0078-7
10. Yang K, Kallies A. Tissue-specific differentiation of CD8(+) resident memory T cells. Trends Immunol (2021) 42:876–90. doi: 10.1016/j.it.2021.08.002
11. Hartana CA, Ahlén Bergman E, Broomé A, Berglund S, Johansson M, Alamdari F, et al. Tissue-resident memory T cells are epigenetically cytotoxic with signs of exhaustion in human urinary bladder cancer. Clin Exp Immunol (2018) 194:39–53. doi: 10.1111/cei.13183
12. Klicznik MM, Morawski PA, Höllbacher B, Varkhande SR, Motley SJ, Kuri-Cervantes L, et al. Human CD4(+)CD103(+) cutaneous resident memory T cells are found in the circulation of healthy individuals. Sci Immunol (2019) 4(37):eaav8995. doi: 10.1126/sciimmunol.aav8995
13. Beura LK, Wijeyesinghe S, Thompson EA, Macchietto MG, Rosato PC, Pierson MJ, et al. T Cells in nonlymphoid tissues give rise to lymph-Node-Resident memory T cells. Immunity (2018) 48:327–38.e5. doi: 10.1016/j.immuni.2018.01.015
14. Fonseca R, Beura LK, Quarnstrom CF, Ghoneim HE, Fan Y, Zebley CC, et al. Developmental plasticity allows outside-in immune responses by resident memory T cells. Nat Immunol (2020) 21:412–21. doi: 10.1038/s41590-020-0607-7
15. Behr FM, Parga-Vidal L, Kragten NAM, van Dam TJP, Wesselink TH, Sheridan BS, et al. Tissue-resident memory CD8+ T cells shape local and systemic secondary T cell responses. Nat Immunol (2020) 21:1070–81. doi: 10.1038/s41590-020-0723-4
16. Skon CN, Lee JY, Anderson KG, Masopust D, Hogquist KA, Jameson SC. Transcriptional downregulation of S1pr1 is required for the establishment of resident memory CD8+ T cells. Nat Immunol (2013) 14:1285–93. doi: 10.1038/ni.2745
17. Shiow LR, Rosen DB, Brdicková N, Xu Y, An J, Lanier LL, et al. CD69 acts downstream of interferon-alpha/beta to inhibit S1P1 and lymphocyte egress from lymphoid organs. Nature (2006) 440:540–4. doi: 10.1038/nature04606
18. Baeyens A, Bracero S, Chaluvadi VS, Khodadadi-Jamayran A, Cammer M, Schwab SR. Monocyte-derived S1P in the lymph node regulates immune responses. Nature (2021) 592:290–5. doi: 10.1038/s41586-021-03227-6
19. Schenkel JM, Masopust D. Tissue-resident memory T cells. Immunity (2014) 41:886–97. doi: 10.1016/j.immuni.2014.12.007
20. Crowl JT, Heeg M, Ferry A, Milner JJ, Omilusik KD, Toma C, et al. Tissue-resident memory CD8(+) T cells possess unique transcriptional, epigenetic and functional adaptations to different tissue environments. Nat Immunol (2022) 23:1121–31. doi: 10.1038/s41590-022-01229-8
21. Dijkgraaf FE, Kok L, Schumacher TNM. Formation of tissue-resident CD8(+) T-cell memory. Cold Spring Harb Perspect Biol (2021) 13(8):a038117. doi: 10.1101/cshperspect.a038117
22. Corgnac S, Boutet M, Kfoury M, Naltet C, Mami-Chouaib F. The emerging role of CD8(+) tissue resident memory T (TRM) cells in antitumor immunity: A unique functional contribution of the CD103 integrin. Front Immunol (2018) 9:1904. doi: 10.3389/fimmu.2018.01904
23. Franciszkiewicz K, Le Floc'h A, Boutet M, Vergnon I, Schmitt A, Mami-Chouaib F. CD103 or LFA-1 engagement at the immune synapse between cytotoxic T cells and tumor cells promotes maturation and regulates T-cell effector functions. Cancer Res (2013) 73:617–28. doi: 10.1158/0008-5472.CAN-12-2569
24. Mackay LK, Rahimpour A, Ma JZ, Collins N, Stock AT, Hafon ML, et al. The developmental pathway for CD103(+)CD8+ tissue-resident memory T cells of skin. Nat Immunol (2013) 14:1294–301. doi: 10.1038/ni.2744
25. Pan Y, Tian T, Park CO, Lofftus SY, Mei S, Liu X, et al. Survival of tissue-resident memory T cells requires exogenous lipid uptake and metabolism. Nature (2017) 543:252–6. doi: 10.1038/nature21379
26. Ray SJ, Franki SN, Pierce RH, Dimitrova S, Koteliansky V, Sprague AG, et al. The collagen binding α1β1 integrin VLA-1 regulates CD8 T cell-mediated immune protection against heterologous influenza infection. Immunity (2004) 20:167–79. doi: 10.1016/s1074-7613(04)00021-4
27. Bromley SK, Akbaba H, Mani V, Mora-Buch R, Chasse AY, Sama A, et al. CD49a regulates cutaneous resident memory CD8(+) T cell persistence and response. Cell Rep (2020) 32:108085. doi: 10.1016/j.celrep.2020.108085
28. FitzPatrick MEB, Provine NM, Garner LC, Powell K, Amini A, Irwin SL, et al. Human intestinal tissue-resident memory T cells comprise transcriptionally and functionally distinct subsets. Cell Rep (2021) 34:108661. doi: 10.1016/j.celrep.2020.108661
29. Fung HY, Teryek M, Lemenze AD, Bergsbaken T. CD103 fate mapping reveals that intestinal CD103- tissue-resident memory T cells are the primary responders to secondary infection. Sci Immunol (2022) 7:eabl9925. doi: 10.1126/sciimmunol.abl9925
30. von Hoesslin M, Kuhlmann M, de Almeida GP, Kanev K, Wurmser C, Gerullis A-K, et al. Secondary infections rejuvenate the intestinal CD103+ tissue-resident memory T cell pool. Sci Immunol (2022) 7:eabp9553. doi: 10.1126/sciimmunol.abp9553
31. Fonseca R, Burn TN, Gandolfo LC, Devi S, Park SL, Obers A, et al. Runx3 drives a CD8(+) T cell tissue residency program that is absent in CD4(+) T cells. Nat Immunol (2022) 23:1236–45. doi: 10.1038/s41590-022-01273-4
32. Grueter B, Petter M, Egawa T, Laule-Kilian K, Aldrian CJ, Wuerch A, et al. Runx3 regulates integrin alpha E/CD103 and CD4 expression during development of CD4-/CD8+ T cells. J Immunol (Baltimore Md 1950) (2005) 175:1694–705. doi: 10.4049/jimmunol.175.3.1694
33. Kurd NS, He Z, Louis TL, Milner JJ, Omilusik KD, Jin W, et al. Early precursors and molecular determinants of tissue-resident memory CD8(+) T lymphocytes revealed by single-cell RNA sequencing. Sci Immunol (2020) 5(47):eaaz6894. doi: 10.1126/sciimmunol.aaz6894
34. Wang D, Diao H, Getzler AJ, Rogal W, Frederick MA, Milner J, et al. The transcription factor Runx3 establishes chromatin accessibility of cis-regulatory landscapes that drive memory cytotoxic T lymphocyte formation. Immunity (2018) 48:659–74.e6. doi: 10.1016/j.immuni.2018.03.028
35. Milner JJ, Toma C, Yu B, Zhang K, Omilusik K, Phan AT, et al. Runx3 programs CD8(+) T cell residency in non-lymphoid tissues and tumours. Nature (2017) 552:253–7. doi: 10.1038/nature24993
36. Mackay LK, Minnich M, Kragten NA, Liao Y, Nota B, Seillet C, et al. Hobit and Blimp1 instruct a universal transcriptional program of tissue residency in lymphocytes. Sci (New York NY) (2016) 352:459–63. doi: 10.1126/science.aad2035
37. Backer RA, Helbig C, Gentek R, Kent A, Laidlaw BJ, Dominguez CX, et al. A central role for notch in effector CD8(+) T cell differentiation. Nat Immunol (2014) 15:1143–51. doi: 10.1038/ni.3027
38. Wu J, Madi A, Mieg A, Hotz-Wagenblatt A, Weisshaar N, Ma S, et al. T Cell factor 1 suppresses CD103+ lung tissue-resident memory T cell development. Cell Rep (2020) 31:107484. doi: 10.1016/j.celrep.2020.03.048
39. Hombrink P, Helbig C, Backer RA, Piet B, Oja AE, Stark R, et al. Programs for the persistence, vigilance and control of human CD8(+) lung-resident memory T cells. Nat Immunol (2016) 17:1467–78. doi: 10.1038/ni.3589
40. Cruz-Guilloty F, Pipkin ME, Djuretic IM, Levanon D, Lotem J, Lichtenheld MG, et al. Runx3 and T-box proteins cooperate to establish the transcriptional program of effector CTLs. J Exp Med (2009) 206:51–9. doi: 10.1084/jem.20081242
41. Kragten NAM, Behr FM, Vieira Braga FA, Remmerswaal EBM, Wesselink TH, Oja AE, et al. Blimp-1 induces and hobit maintains the cytotoxic mediator granzyme b in CD8 T cells. Eur J Immunol (2018) 48:1644–62. doi: 10.1002/eji.201847771
42. Lin YH, Duong HG, Limary AE, Kim ES, Hsu P, Patel SA, et al. Small intestine and colon tissue-resident memory CD8+ T cells exhibit molecular heterogeneity and differential dependence on eomes. Immunity (2023) 56(1):207–23.e8. doi: 10.1016/j.immuni.2022.12.007
43. Parga-Vidal L, van Aalderen MC, Stark R, van Gisbergen K. Tissue-resident memory T cells in the urogenital tract. Nat Rev Nephrol (2022) 18:209–23. doi: 10.1038/s41581-021-00525-0
44. Christo SN, Evrard M, Park SL, Gandolfo LC, Burn TN, Fonseca R, et al. Discrete tissue microenvironments instruct diversity in resident memory T cell function and plasticity. Nat Immunol (2021) 22:1140–51. doi: 10.1038/s41590-021-01004-1
45. Parga-Vidal L, Taggenbrock R, Beumer-Chuwonpad A, Aglmous H, Kragten NAM, Behr FM, et al. Hobit and blimp-1 regulate TRM abundance after LCMV infection by suppressing tissue exit pathways of TRM precursors. Eur J Immunol (2022) 52:1095–111. doi: 10.1002/eji.202149665
46. Ryan GE, Harris JE, Richmond JM. Resident memory T cells in autoimmune skin diseases. Front Immunol (2021) 12:652191. doi: 10.3389/fimmu.2021.652191
47. Zundler S, Becker E, Spocinska M, Slawik M, Parga-Vidal L, Stark R, et al. Hobit- and blimp-1-driven CD4(+) tissue-resident memory T cells control chronic intestinal inflammation. Nat Immunol (2019) 20:288–300. doi: 10.1038/s41590-018-0298-5
48. La Manna MP, Di Liberto D, Lo Pizzo M, Mohammadnezhad L, Shekarkar Azgomi M, Salamone V, et al. The abundance of tumor-infiltrating CD8(+) tissue resident memory T lymphocytes correlates with patient survival in glioblastoma. Biomedicines (2022) 10(10):2454. doi: 10.3390/biomedicines10102454
49. Lin R, Zhang H, Yuan Y, He Q, Zhou J, Li S, et al. Fatty acid oxidation controls CD8(+) tissue-resident memory T-cell survival in gastric adenocarcinoma. Cancer Immunol Res (2020) 8:479–92. doi: 10.1158/2326-6066.CIR-19-0702
50. Byrne A, Savas P, Sant S, Li R, Virassamy B, Luen SJ, et al. Tissue-resident memory T cells in breast cancer control and immunotherapy responses. Nat Rev Clin Oncol (2020) 17:341–8. doi: 10.1038/s41571-020-0333-y
51. Amsen D, van Gisbergen KPJM, Hombrink P, van Lier RAW. Tissue-resident memory T cells at the center of immunity to solid tumors. Nat Immunol (2018) 19:538–46. doi: 10.1038/s41590-018-0114-2
52. Okla K, Farber DL, Zou W. Tissue-resident memory T cells in tumor immunity and immunotherapy. J Exp Med (2021) 218(4):e20201605. doi: 10.1084/jem.20201605
53. Mami-Chouaib F, Blanc C, Corgnac S, Hans S, Malenica I, Granier C, et al. Resident memory T cells, critical components in tumor immunology. J Immunother Cancer (2018) 6:87. doi: 10.1186/s40425-018-0399-6
54. Mattei F, Andreone S, Marone G, Gambardella AR, Loffredo S, Varricchi G, et al. Eosinophils in the tumor microenvironment. Adv Exp Med Biol (2020) 1273:1–28. doi: 10.1007/978-3-030-49270-0_1
55. Edwards J, Wilmott JS, Madore J, Gide TN, Quek C, Tasker A, et al. CD103(+) tumor-resident CD8(+) T cells are associated with improved survival in immunotherapy-naïve melanoma patients and expand significantly during anti-PD-1 treatment. Clin Cancer Res (2018) 24:3036–45. doi: 10.1158/1078-0432.Ccr-17-2257
56. Murray T, Fuertes Marraco SA, Baumgaertner P, Bordry N, Cagnon L, Donda A, et al. Very late antigen-1 marks functional tumor-resident CD8 T cells and correlates with survival of melanoma patients. Front Immunol (2016) 7:573. doi: 10.3389/fimmu.2016.00573
57. Shen Y, Li XL, Li YX, Shan ZG, Zhao YL, Cheng P, et al. Distribution, phenotype, functional and clinical relevance of CD8(+)CD103(+) tissue-resident memory T cells in human gastric cancer. Cancer Immunol Immunother (2022) 71:1645–54. doi: 10.1007/s00262-021-03105-0
58. Li R, Liu H, Cao Y, Wang J, Chen Y, Qi Y, et al. Identification and validation of an immunogenic subtype of gastric cancer with abundant intratumoural CD103(+)CD8(+) T cells conferring favourable prognosis. Br J Cancer (2020) 122:1525–34. doi: 10.1038/s41416-020-0813-y
59. Mori T, Tanaka H, Deguchi S, Miki Y, Yoshii M, Tamura T, et al. CD103(+) T cells may be a useful biomarker in borrmann type 4 gastric cancer. Cancer Diagn Progn (2022) 2:384–90. doi: 10.21873/cdp.10121
60. Webb JR, Milne K, Watson P, Deleeuw RJ, Nelson BH. Tumor-infiltrating lymphocytes expressing the tissue resident memory marker CD103 are associated with increased survival in high-grade serous ovarian cancer. Clin Cancer Res (2014) 20:434–44. doi: 10.1158/1078-0432.Ccr-13-1877
61. Ganesan AP, Clarke J, Wood O, Garrido-Martin EM, Chee SJ, Mellows T, et al. Tissue-resident memory features are linked to the magnitude of cytotoxic T cell responses in human lung cancer. Nat Immunol (2017) 18:940–50. doi: 10.1038/ni.3775
62. Nizard M, Roussel H, Diniz MO, Karaki S, Tran T, Voron T, et al. Induction of resident memory T cells enhances the efficacy of cancer vaccine. Nat Commun (2017) 8:15221. doi: 10.1038/ncomms15221
63. Cheng Y, Gunasegaran B, Singh HD, Dutertre CA, Loh CY, Lim JQ, et al. Non-terminally exhausted tumor-resident memory HBV-specific T cell responses correlate with relapse-free survival in hepatocellular carcinoma. Immunity (2021) 54:1825–40.e7. doi: 10.1016/j.immuni.2021.06.013
64. Barsch M, Salié H, Schlaak AE, Zhang Z, Hess M, Mayer LS, et al. T-Cell exhaustion and residency dynamics inform clinical outcomes in hepatocellular carcinoma. J Hepatol (2022) 77:397–409. doi: 10.1016/j.jhep.2022.02.032
65. Yang R, Cheng S, Luo N, Gao R, Yu K, Kang B, et al. Distinct epigenetic features of tumor-reactive CD8+ T cells in colorectal cancer patients revealed by genome-wide DNA methylation analysis. Genome Biol (2019) 21:2. doi: 10.1186/s13059-019-1921-y
66. Luo Y, Zong Y, Hua H, Gong M, Peng Q, Li C, et al. Immune-infiltrating signature-based classification reveals CD103(+)CD39(+) T cells associate with colorectal cancer prognosis and response to immunotherapy. Front Immunol (2022) 13:1011590. doi: 10.3389/fimmu.2022.1011590
67. Malik BT, Byrne KT, Vella JL, Zhang P, Shabaneh TB, Steinberg SM, et al. Resident memory T cells in the skin mediate durable immunity to melanoma. Sci Immunol (2017) 2(10):eaam6346. doi: 10.1126/sciimmunol.aam6346
68. Enamorado M, Khouili SC, Iborra S, Sancho D. Genealogy, dendritic cell priming, and differentiation of tissue-resident memory CD8(+) T cells. Front Immunol (2018) 9:1751. doi: 10.3389/fimmu.2018.01751
69. Vella JL, Molodtsov A, Angeles CV, Branchini BR, Turk MJ, Huang YH. Dendritic cells maintain anti-tumor immunity by positioning CD8 skin-resident memory T cells. Life Sci alliance (2021) 4(10):e202101056. doi: 10.26508/lsa.202101056
70. Li X, Zhai J, Shen Y, Zhang T, Wang Y, He Y, et al. Tumor-derived IL-8 facilitates lymph node metastasis of gastric cancer via PD-1 up-regulation in CD8(+) T cells. Cancer Immunol Immunother (2022) 71:3057–70. doi: 10.1007/s00262-022-03223-3
71. Li Y, Hu X, Lin R, Zhou G, Zhao L, Zhao D, et al. Single-cell landscape reveals active cell subtypes and their interaction in the tumor microenvironment of gastric cancer. Theranostics (2022) 12:3818–33. doi: 10.7150/thno.71833
72. Farhood B, Najafi M, Mortezaee K. CD8(+) cytotoxic T lymphocytes in cancer immunotherapy: A review. J Cell Physiol (2019) 234:8509–21. doi: 10.1002/jcp.27782
73. Beltra JC, Manne S, Abdel-Hakeem MS, Kurachi M, Giles JR, Chen Z, et al. Developmental relationships of four exhausted CD8(+) T cell subsets reveals underlying transcriptional and epigenetic landscape control mechanisms. Immunity (2020) 52:825–41 e8. doi: 10.1016/j.immuni.2020.04.014
74. Wherry EJ, Ha SJ, Kaech SM, Haining WN, Sarkar S, Kalia V, et al. Molecular signature of CD8+ T cell exhaustion during chronic viral infection. Immunity (2007) 27:670–84. doi: 10.1016/j.immuni.2007.09.006
75. Wakim LM, Woodward-Davis A, Liu R, Hu Y, Villadangos J, Smyth G, et al. The molecular signature of tissue resident memory CD8 T cells isolated from the brain. J Immunol (2012) 189:3462–71. doi: 10.4049/jimmunol.1201305
76. Zheng L, Qin S, Si W, Wang A, Xing B, Gao R, et al. Pan-cancer single-cell landscape of tumor-infiltrating T cells. Science (2021) 374:abe6474. doi: 10.1126/science.abe6474
77. Kim BS, Kuen DS, Koh CH, Kim HD, Chang SH, Kim S, et al. Type 17 immunity promotes the exhaustion of CD8(+) T cells in cancer. J immunother Cancer (2021) 9(6):e002603. doi: 10.1136/jitc-2021-002603
78. Sun K, Xu R, Ma F, Yang N, Li Y, Sun X, et al. scRNA-seq of gastric tumor shows complex intercellular interaction with an alternative T cell exhaustion trajectory. Nat Commun (2022) 13:4943. doi: 10.1038/s41467-022-32627-z
79. Guo X, Zhang Y, Zheng L, Zheng C, Song J, Zhang Q, et al. Global characterization of T cells in non-small-cell lung cancer by single-cell sequencing. Nat Med (2018) 24:978–85. doi: 10.1038/s41591-018-0045-3
80. Milner JJ, Toma C, He Z, Kurd NS, Nguyen QP, McDonald B, et al. Heterogenous populations of tissue-resident CD8(+) T cells are generated in response to infection and malignancy. Immunity (2020) 52:808–24.e7. doi: 10.1016/j.immuni.2020.04.007
81. Clarke J, Panwar B, Madrigal A, Singh D, Gujar R, Wood O, et al. Single-cell transcriptomic analysis of tissue-resident memory T cells in human lung cancer. J Exp Med (2019) 216:2128–49. doi: 10.1084/jem.20190249
82. Anadon CM, Yu X, Hanggi K, Biswas S, Chaurio RA, Martin A, et al. Ovarian cancer immunogenicity is governed by a narrow subset of progenitor tissue-resident memory T cells. Cancer Cell (2022) 40:545–57 e13. doi: 10.1016/j.ccell.2022.03.008
83. Booth JS, Toapanta FR, Salerno-Goncalves R, Patil S, Kader HA, Safta AM, et al. Characterization and functional properties of gastric tissue-resident memory T cells from children, adults, and the elderly. Front Immunol (2014) 5:294. doi: 10.3389/fimmu.2014.00294
84. Li R, Liu H, Cao Y, Wang J, Chen Y, Qi Y, et al. Identification and validation of an immunogenic subtype of gastric cancer with abundant intratumoural CD103CD8 T cells conferring favourable prognosis. Br J Cancer (2020) 122:1525–34. doi: 10.1038/s41416-020-0813-y
85. Lin R, Zhang H, Yuan Y, He Q, Zhou J, Li S, et al. Fatty acid oxidation controls CD8 tissue-resident memory T-cell survival in gastric adenocarcinoma. Cancer Immunol Res (2020) 8:479–92. doi: 10.1158/2326-6066.CIR-19-0702
86. Mori T, Tanaka H, Suzuki S, Deguchi S, Yamakoshi Y, Yoshii M, et al. Tertiary lymphoid structures show infiltration of effective tumor-resident T cells in gastric cancer. Cancer Sci (2021) 112:1746–57. doi: 10.1111/cas.14888
87. Pan J, Fan Z, Wang Z, Dai Q, Xiang Z, Yuan F, et al. CD36 mediates palmitate acid-induced metastasis of gastric cancer via AKT/GSK-3beta/beta-catenin pathway. J Exp Clin Cancer Res (2019) 38:52. doi: 10.1186/s13046-019-1049-7
88. Aoki T, Kinoshita J, Munesue S, Hamabe-Horiike T, Yamaguchi T, Nakamura Y, et al. Hypoxia-induced CD36 expression in gastric cancer cells promotes peritoneal metastasis via fatty acid uptake. Ann Surg Oncol (2022) 10.1245/s10434-022-12465-5. doi: 10.1245/s10434-022-12465-5
89. Dieu-Nosjean MC, Giraldo NA, Kaplon H, Germain C, Fridman WH, Sautès-Fridman C. Tertiary lymphoid structures, drivers of the anti-tumor responses in human cancers. Immunol Rev (2016) 271(1):260–75. doi: 10.1111/imr.12405
90. Trüb M, Zippelius A. Tertiary lymphoid structures as a predictive biomarker of response to cancer immunotherapies. Front Immunol (2021) 12:674565. doi: 10.3389/fimmu.2021.674565
91. Mori T, Tanaka H, Deguchi S, Yamakoshi Y, Miki Y, Yoshii M, et al. Clinical efficacy of nivolumab is associated with tertiary lymphoid structures in surgically resected primary tumors of recurrent gastric cancer. PloS One (2022) 17:e0262455. doi: 10.1371/journal.pone.0262455
92. Correa P. Gastric cancer: Overview. Gastroenterol Clin North Am (2013) 42:211–7. doi: 10.1016/j.gtc.2013.01.002
93. Machlowska J, Baj J, Sitarz M, Maciejewski R, Sitarz R. Gastric cancer: Epidemiology, risk factors, classification, genomic characteristics and treatment strategies. Int J Mol Sci (2020) 21(11):4012. doi: 10.3390/ijms21114012
94. Yang J, Liu Z, Zeng B, Hu G, Gan R. Epstein-Barr Virus-associated gastric cancer: A distinct subtype. Cancer Lett (2020) 495:191–9. doi: 10.1016/j.canlet.2020.09.019
95. Deng R, Zheng H, Cai H, Li M, Shi Y, Ding S. Effects of helicobacter pylori on tumor microenvironment and immunotherapy responses. Front Immunol (2022) 13:923477. doi: 10.3389/fimmu.2022.923477
96. Wu J, Zhu X, Guo X, Yang Z, Cai Q, Gu D, et al. Helicobacter urease suppresses cytotoxic CD8+ T-cell responses through activating Myh9-dependent induction of PD-L1. Int Immunol (2021) 33:491–504. doi: 10.1093/intimm/dxab044
97. Xu N, Ruan G, Liu W, Hu C, Huang A, Zeng Z, et al. Vaccine-induced gastric CD4(+) tissue-resident memory T cells proliferate in situ to amplify immune response against helicobacter pylori insult. Helicobacter (2019) 24:e12652. doi: 10.1111/hel.12652
98. Koch MR, Gong R, Friedrich V, Engelsberger V, Kretschmer L, Wanisch A, et al. CagA-specific gastric CD8+ tissue-resident T cells control helicobacter pylori during the early infection phase. Gastroenterology (2022). doi: 10.1053/j.gastro.2022.12.016
99. Xing X, Guo J, Ding G, Li B, Dong B, Feng Q, et al. Analysis of PD1, PDL1, PDL2 expression and T cells infiltration in 1014 gastric cancer patients. Oncoimmunology (2018) 7:e1356144. doi: 10.1080/2162402X.2017.1356144
100. Sasaki S, Nishikawa J, Sakai K, Iizasa H, Yoshiyama H, Yanagihara M, et al. EBV-associated gastric cancer evades T-cell immunity by PD-1/PD-L1 interactions. Gastric Cancer (2019) 22:486–96. doi: 10.1007/s10120-018-0880-4
101. Peng R, Liu S, You W, Huang Y, Hu C, Gao Y, et al. Gastric microbiome alterations are associated with decreased CD8+ tissue-resident memory T cells in the tumor microenvironment of gastric cancer. Cancer Immunol Res (2022) 10:1224–40. doi: 10.1158/2326-6066.Cir-22-0107
102. Yang L, He YT, Dong S, Wei XW, Chen ZH, Zhang B, et al. Single-cell transcriptome analysis revealed a suppressive tumor immune microenvironment in EGFR mutant lung adenocarcinoma. J immunother Cancer (2022) 10(2):e003534. doi: 10.1136/jitc-2021-003534
103. Lutter L, Roosenboom B, Brand EC, Ter Linde JJ, Oldenburg B, van Lochem EG, et al. Homeostatic function and inflammatory activation of ileal CD8(+) tissue-resident T cells is dependent on mucosal location. Cell Mol Gastroenterol Hepatol (2021) 12:1567–81. doi: 10.1016/j.jcmgh.2021.06.022
104. Wang C, Kong L, Kim S, Lee S, Oh S, Jo S, et al. The role of IL-7 and IL-7R in cancer pathophysiology and immunotherapy. Int J Mol Sci (2022) 23(18):10412. doi: 10.3390/ijms231810412
105. de Andrea CE, Schalper KA, Sanmamed MF, Melero I. Immunodivergence in metastatic colorectal cancer. Cancer Cell (2018) 34:876–8. doi: 10.1016/j.ccell.2018.11.012
106. Zhang N, Bevan MJ. Transforming growth factor-beta signaling controls the formation and maintenance of gut-resident memory T cells by regulating migration and retention. Immunity (2013) 39:687–96. doi: 10.1016/j.immuni.2013.08.019
107. Lange J, Rivera-Ballesteros O, Buggert M. Human mucosal tissue-resident memory T cells in health and disease. Mucosal Immunol (2022) 15:389–97. doi: 10.1038/s41385-021-00467-7
108. Chen B, Mu C, Zhang Z, He X, Liu X. The love-hate relationship between TGF-beta signaling and the immune system during development and tumorigenesis. Front Immunol (2022) 13:891268. doi: 10.3389/fimmu.2022.891268
109. Jiang W, Wang X, Zeng B, Liu L, Tardivel A, Wei H, et al. Recognition of gut microbiota by NOD2 is essential for the homeostasis of intestinal intraepithelial lymphocytes. J Exp Med (2013) 210:2465–76. doi: 10.1084/jem.20122490
110. Patidar M, Yadav N, Dalai SK. Interleukin 15: A key cytokine for immunotherapy. Cytokine Growth factor Rev (2016) 31:49–59. doi: 10.1016/j.cytogfr.2016.06.001
111. Cui F, Qu D, Sun R, Zhang M, Nan K. NK cell-produced IFN-γ regulates cell growth and apoptosis of colorectal cancer by regulating IL-15. Exp Ther Med (2020) 19:1400–6. doi: 10.3892/etm.2019.8343
112. Bahri R, Pateras IS, D'Orlando O, Goyeneche-Patino DA, Campbell M, Polansky JK, et al. IL-15 suppresses colitis-associated colon carcinogenesis by inducing antitumor immunity. Oncoimmunology (2015) 4:e1002721. doi: 10.1080/2162402x.2014.1002721
113. Desbois M, Le Vu P, Coutzac C, Marcheteau E, Beal C, Terme M, et al. IL-15 trans-signaling with the superagonist RLI promotes Effector/Memory CD8+ T cell responses and enhances antitumor activity of PD-1 antagonists. J Immunol (Baltimore Md 1950) (2016) 197:168–78. doi: 10.4049/jimmunol.1600019
114. Bui QL, Mas L, Hollebecque A, Tougeron D, de la Fouchardiere C, Pudlarz T, et al. Treatments after immune checkpoint inhibitors in patients with dMMR/MSI metastatic colorectal cancer. Cancers (Basel) (2022) 14(2):406. doi: 10.3390/cancers14020406
115. Randrian V, Evrard C, Tougeron D. Microsatellite instability in colorectal cancers: Carcinogenesis, neo-antigens, immuno-resistance and emerging therapies. Cancers (Basel) (2021) 13(12):3063. doi: 10.3390/cancers13123063
116. De' Angelis GL, Bottarelli L, Azzoni C, De' Angelis N, Leandro G, Di Mario F, et al. Microsatellite instability in colorectal cancer. Acta BioMed (2018) 89:97–101. doi: 10.23750/abm.v89i9-S.7960
117. Guastadisegni C, Colafranceschi M, Ottini L, Dogliotti E. Microsatellite instability as a marker of prognosis and response to therapy: A meta-analysis of colorectal cancer survival data. Eur J Cancer (Oxford Engl 1990) (2010) 46:2788–98. doi: 10.1016/j.ejca.2010.05.009
118. de Vries NL, van Unen V, Ijsselsteijn ME, Abdelaal T, van der Breggen R, Farina Sarasqueta A, et al. High-dimensional cytometric analysis of colorectal cancer reveals novel mediators of antitumour immunity. Gut (2020) 69:691–703. doi: 10.1136/gutjnl-2019-318672
119. Duhen T, Duhen R, Montler R, Moses J, Moudgil T, de Miranda NF, et al. Co-Expression of CD39 and CD103 identifies tumor-reactive CD8 T cells in human solid tumors. Nat Commun (2018) 9:2724. doi: 10.1038/s41467-018-05072-0
120. Sung H, Ferlay J, Siegel RL, Laversanne M, Soerjomataram I, Jemal A, et al. Global cancer statistics 2020: GLOBOCAN estimates of incidence and mortality worldwide for 36 cancers in 185 countries. CA Cancer J Clin (2021) 71:209–49. doi: 10.3322/caac.21660
121. Baidoun F, Elshiwy K, Elkeraie Y, Merjaneh Z, Khoudari G, Sarmini MT, et al. Colorectal cancer epidemiology: Recent trends and impact on outcomes. Curr Drug Targets (2021) 22:998–1009. doi: 10.2174/1389450121999201117115717
122. Tran E, Robbins PF, Lu YC, Prickett TD, Gartner JJ, Jia L, et al. T-Cell transfer therapy targeting mutant KRAS in cancer. N Engl J Med (2016) 375:2255–62. doi: 10.1056/NEJMoa1609279
123. Edwards J, Wilmott JS, Madore J, Gide TN, Quek C, Tasker A, et al. CD103+ tumor-resident CD8+ T cells are associated with improved survival in immunotherapy-naïve melanoma patients and expand significantly during anti–PD-1 treatment. Clin Cancer Res (2018) 24:3036–45. doi: 10.1158/1078-0432.Ccr-17-2257
124. Luoma AM, Suo S, Wang Y, Gunasti L, Porter CBM, Nabilsi N, et al. Tissue-resident memory and circulating T cells are early responders to pre-surgical cancer immunotherapy. Cell (2022) 185:2918–35.e29. doi: 10.1016/j.cell.2022.06.018
125. Enamorado M, Iborra S, Priego E, Cueto FJ, Quintana JA, Martínez-Cano S, et al. Enhanced anti-tumour immunity requires the interplay between resident and circulating memory CD8(+) T cells. Nat Commun (2017) 8:16073. doi: 10.1038/ncomms16073
126. Lauret Marie Joseph E, Kirilovsky A, Lecoester B, El Sissy C, Boullerot L, Rangan L, et al. Chemoradiation triggers antitumor Th1 and tissue resident memory-polarized immune responses to improve immune checkpoint inhibitors therapy. J immunother Cancer (2021) 9(7):e002256. doi: 10.1136/jitc-2020-002256
127. Janjigian YY, Shitara K, Moehler M, Garrido M, Salman P, Shen L, et al. First-line nivolumab plus chemotherapy versus chemotherapy alone for advanced gastric, gastro-oesophageal junction, and oesophageal adenocarcinoma (CheckMate 649): a randomised, open-label, phase 3 trial. Lancet (London England) (2021) 398:27–40. doi: 10.1016/s0140-6736(21)00797-2
128. Wu TC, Xu K, Banchereau R, Marches F, Yu CI, Martinek J, et al. Reprogramming tumor-infiltrating dendritic cells for CD103+ CD8+ mucosal T-cell differentiation and breast cancer rejection. Cancer Immunol Res (2014) 2:487–500. doi: 10.1158/2326-6066.Cir-13-0217
129. Kobayashi M, Sakabe T, Chiba A, Nakajima A, Okamoto M, Shimodaira S, et al. Therapeutic effect of intratumoral injections of dendritic cells for locally recurrent gastric cancer: a case report. World J Surg Oncol (2014) 12:390. doi: 10.1186/1477-7819-12-390
130. Rosato PC, Wijeyesinghe S, Stolley JM, Nelson CE, Davis RL, Manlove LS, et al. Virus-specific memory T cells populate tumors and can be repurposed for tumor immunotherapy. Nat Commun (2019) 10:567. doi: 10.1038/s41467-019-08534-1
131. Sandoval F, Terme M, Nizard M, Badoual C, Bureau MF, Freyburger L, et al. Mucosal imprinting of vaccine-induced CD8+ T cells is crucial to inhibit the growth of mucosal tumors. Sci Trans Med (2013) 5:172ra20. doi: 10.1126/scitranslmed.3004888
132. Cafri G, Gartner JJ, Zaks T, Hopson K, Levin N, Paria BC, et al. mRNA vaccine-induced neoantigen-specific T cell immunity in patients with gastrointestinal cancer. J Clin Invest (2020) 130:5976–88. doi: 10.1172/jci134915
133. Besser MJ, Shapira-Frommer R, Treves AJ, Zippel D, Itzhaki O, Hershkovitz L, et al. Clinical responses in a phase II study using adoptive transfer of short-term cultured tumor infiltration lymphocytes in metastatic melanoma patients. Clin Cancer Res (2010) 16:2646–55. doi: 10.1158/1078-0432.Ccr-10-0041
134. Zacharakis N, Huq LM, Seitter SJ, Kim SP, Gartner JJ, Sindiri S, et al. Breast cancers are immunogenic: Immunologic analyses and a phase II pilot clinical trial using mutation-reactive autologous lymphocytes. J Clin Oncol (2022) 40:1741–54. doi: 10.1200/jco.21.02170
135. Morgan RA, Yang JC, Kitano M, Dudley ME, Laurencot CM, Rosenberg SA. Case report of a serious adverse event following the administration of T cells transduced with a chimeric antigen receptor recognizing ERBB2. Mol Ther (2010) 18:843–51. doi: 10.1038/mt.2010.24
136. Zheng C, Fass JN, Shih YP, Gunderson AJ, Sanjuan Silva N, Huang H, et al. Transcriptomic profiles of neoantigen-reactive T cells in human gastrointestinal cancers. Cancer Cell (2022) 40:410–23.e7. doi: 10.1016/j.ccell.2022.03.005
137. He J, Xiong X, Yang H, Li D, Liu X, Li S, et al. Defined tumor antigen-specific T cells potentiate personalized TCR-T cell therapy and prediction of immunotherapy response. Cell Res (2022) 32:530–42. doi: 10.1038/s41422-022-00627-9
Keywords: tissue resident memory T, gastrointestinal tumor, anti-tumor response, cancer immunotherapy, tumor immune microenvironment
Citation: Liang M, Wang X, Cai D, Guan W and Shen X (2023) Tissue-resident memory T cells in gastrointestinal tumors: turning immune desert into immune oasis. Front. Immunol. 14:1119383. doi: 10.3389/fimmu.2023.1119383
Received: 08 December 2022; Accepted: 27 February 2023;
Published: 09 March 2023.
Edited by:
Fengyin Li, University of Science and Technology of China, ChinaReviewed by:
Oscar Junhong Luo, Jinan University, ChinaLianjun Zhang, Suzhou Institute of Systems Medicine (ISM), China
Hongwei Yao, Beijing Friendship Hospital, China
Copyright © 2023 Liang, Wang, Cai, Guan and Shen. This is an open-access article distributed under the terms of the Creative Commons Attribution License (CC BY). The use, distribution or reproduction in other forums is permitted, provided the original author(s) and the copyright owner(s) are credited and that the original publication in this journal is cited, in accordance with accepted academic practice. No use, distribution or reproduction is permitted which does not comply with these terms.
*Correspondence: Xiaofei Shen, dg1535058@smail.nju.edu.cn; Wenxian Guan, 15850502391@163.com
†These authors have contributed equally to this work