- 1Warren Alpert Medical School of Brown University, Providence, RI, United States
- 2Department of Diagnostic Imaging, Warren Alpert Medical School of Brown University, Providence, RI, United States
Percutaneous tumor ablation is now a widely accepted minimally invasive local treatment option offered by interventional radiology and applied to various organs and tumor histology types. It utilizes extreme temperatures to achieve irreversible cellular injury, where ablated tumor interacts with surrounding tissue and host via tissue remodeling and inflammation, clinically manifesting as post-ablation syndrome. During this process, in-situ tumor vaccination occurs, in which tumor neoantigens are released from ablated tissue and can prime one’s immune system which would favorably affect both local and remote site disease control. Although successful in priming the immune system, this rarely turns into clinical benefits for local and systemic tumor control due to intrinsic negative immune modulation of the tumor microenvironment. A combination of ablation and immunotherapy has been employed to overcome these and has shown promising preliminary results of synergistic effect without significantly increased risk profiles. The aim of this article is to review the evidence on post-ablation immune response and its synergy with systemic immunotherapies.
Introduction
Interventional radiology is an essential part of oncologic care, from its traditional roles in tissue sampling and venous access to active therapeutics in tumor-directed locoregional therapies and various palliative interventions. In addition, recent progress in immunotherapy brought particular interest to systemic and local tumor microenvironments created by locoregional interventional therapies given their potential to release tumor-specific neoantigen and other immune-stimulators via in-situ tumor destruction (1, 2).
Percutaneous tumor ablation is now a widely accepted minimally invasive local treatment option offered by interventional radiology and applied to various organs and tumor histology types (3, 4). Tissue ablative technologies utilize extreme temperatures to achieve irreversible cellular injury (3). Radiofrequency ablation (RFA) has the longest history of clinical utilization and there are now various other modalities including microwave ablation (MWA), cryoablation (CA), high-intensity focused ultrasound (HIFU), and laser ablation (LA). Another tissue ablation modality, Irreversible electroporation (IRE), differs from others as it induces non-thermal cellular injury. Regardless of specific modalities, ablated tumor interacts with surrounding tissue and host via tissue remodeling and inflammation. Clinically, this is characterized as a post-ablation syndrome, which manifests as a combination of flu-like symptoms such as fever, malaise, nausea, and others (5–7). During this process, tumor neoantigens are released from ablated tissue and prime one’s immune system which would favorably affect both local and remote site disease control (so called “abscopal effect”). Expectedly, there has been a strong interest in taking advantage of this phenomenon, especially in the form of a combination between ablation and immunotherapy. The aim of this article is to review the evidence on post-ablation immune response and its synergy with systemic immunotherapies.
Cellular injury and tumor neoantigen release after percutaneous ablative therapy
Percutaneous ablative therapies, except for IRE, induce cellular injury by exposing tumor tissue to extreme temperatures. RFA, MWA, HIFU, and LA induce hyperthermic cellular injury, while the lethal freezing temperature is used in cryoablation. Focally induced extreme temperature results in a spherical area characterized by three distinct zones: central necrotic, peripheral transitional, and normal tissue (1, 3, 4, 8) (Figure 1). The central necrotic zone is immediately adjacent to ablation probes, achieved by temperatures greater than 60°C or below -20°C (3, 8), resulting in direct and immediate cellular injury. On the other hand, the peripheral transitional zone is characterized by a band of thermal conduction between the central necrotic zone and surrounding normal tissue. A steep temperature gradient within the transitional zone results in varying degrees of cellular injury: necrotic, delayed/indirect, and reversible injury. Various processes including apoptosis, ischemia-reperfusion, and immune response are responsible for delayed or indirect cellular injury (8).
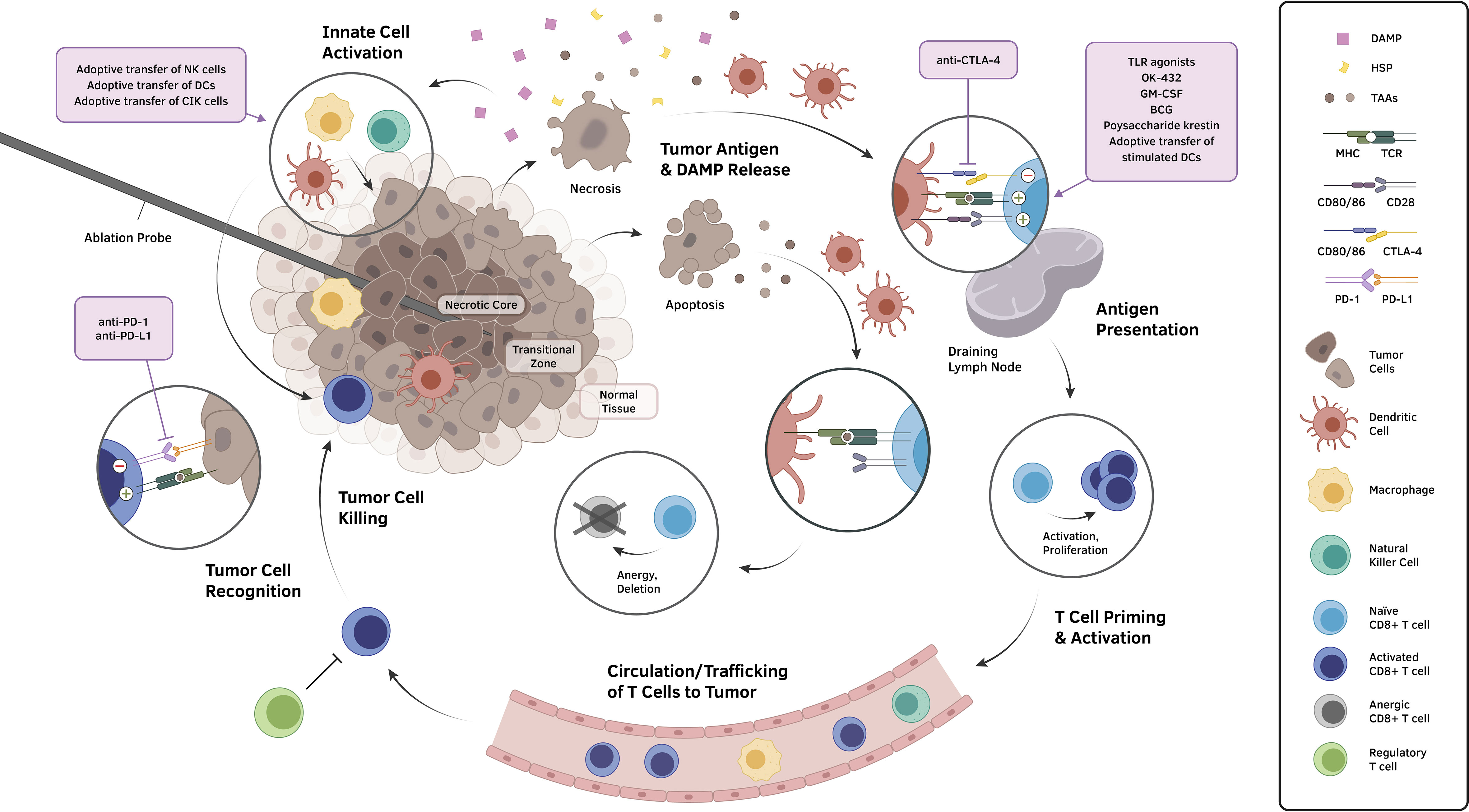
Figure 1 The application of ablative therapeutic modalities to tumors produces a biomolecular landscape that can generally be conceptualized into three concentric zones: a central necrotic zone, a transitional zone demonstrating the consequences of varying degrees of cellular injury, and an outer zone of normal tissue. Cells undergoing necrosis and other non-apoptotic forms of regulated cell death expose and release an abundance of molecules, including TAAs, tumor neoantigens, HSPs, and DAMPs, that incur the recruitment of innate cells, activation of local effector cells, and maturation of antigen presenting cells, particularly DCs. These DCs migrate to draining lymph nodes and induce the formation of tumor-specific cytotoxic lymphocytes, which subsequently circulate to the tumor site and carry out killing of tumor cells upon target antigen recognition. However, multiple negative immunomodulatory mechanisms that exist throughout the cycle, such as T cell anergy resulting from apoptotic tumor cell signaling and the induction of immunosuppressive phenotypes in the tumor microenvironment, have hindered clinically meaningful, immunologically driven anticancer effects from manifesting following ablation. Various approaches to overcome such immunosuppressive obstacles (violet boxes) have been deployed in conjunction with locoregional ablation to demonstrate prospects for a synergistic reinstatement of an immunostimulatory anti-tumor activities. Abbreviations: DAMP, damage-associated molecular pattern; DC, dendritic cell; HSP, heat shock protein; MHC, major histocompatibility complex; TAA, tumor-associated antigen; TCR, T cell receptor; TLR, toll-like receptor.
Tumor necrosis immediately releases damage-associated molecular patterns (DAMPs, such as DNAs and heat shock proteins (HSPs)) into the extracellular matrix (9). Dendritic cells (DCs) phagocytize DAMPs and subsequently promote the expression of co-stimulatory CD80/86 molecules (10, 11), present antigens on major histocompatibility complex (MHC) molecules, and promote systemic immune response (12).
Necroptosis, also called programmed necrosis, is a newly recognized form of regulated cell death with morphological features of both apoptosis and necrosis (13, 14). It is activated by death receptors, which subsequently induce RIPK3 (serine/threonine kinase 3) and activate MLKL (mixed lineage kinase domain-like pseudokinase). This ultimately leads to cell swelling and rupture as well as the release of DAMPs, hence potentially immunogenic (13, 14). Necroptosis has been observed in post-IRE environment (15, 16) but has not been reported or studied yet in other ablation modalities.
In contrast, apoptosis is an intrinsic, orchestrated process of cell death, known to be less immunogenic and inflammatory (10) than necrosis. Apoptosis also induces release of antigens that can be picked up by dendritic cells, but they do not typically release DAMPs (12). Without phagocytizing DAMPs, DCs do not induce expression of CD80 and CD86 molecules (12). Without these co-stimulators, T cell anergy or even clonal deletion may occur, thereby suppressing the immune response (12).
The relative ratio between tumor necrosis and apoptosis was hypothesized to be a critical modulator in post-ablation immunogenicity (3). Different ablation protocols and parameters have been utilized to optimize this ratio, resulting in varying degrees of post-ablation immunogenic profiles (17, 18).
Post-ablation inflammation: a double-edged sword
Ablative therapy decreases overall tumor burden and possibly leads to the reversal of immune suppression and unmasks a population of primed tumor-specific T cells that can induce protective immunity (19). Although detailed mechanism and downstream effect of the post-ablation immune response still remains elusive at large, there are many studies identifying effectors and mediators. Ablated tumors release and expose immune-potentiating tumor antigens as well as nuclear protein high mobility group B1 (HMGB1) and HSPs, which in turn induce antitumor immune response through activation of DCs (20). Studies have demonstrated tumor neoantigen released from the ablation bed yielded more effective dendritic cells in inducing CD4+ and CD8+ T cells (21). It also induces transient activation of myeloid dendritic cells (MDCs) associated with increased serum levels of TNF-α and IL-1β (22). In addition, the post-ablation environment has been shown to accelerate the migration of peripheral blood mononuclear cells (PBMC), activation of effector cells, and induction and secretion of cytokines, all of which may enhance the antitumor immune response (23).
It remains unknown how or if these post-ablation responses turn into clinically meaningful anti-tumor effects or abscopal effects. There have been anecdotal clinical case reports of the abscopal phenomenon after local ablation therapies (24–27) while the majority of post-ablation immune response remains clinically quiescent (1). Several cellular biomarkers including elevated lymphocyte-monocyte ratio (LMR) (28), decreased neutrophil-lymphocyte ratio (NLR) (29, 30) and increased cytotoxic natural killer (NK) cells (31) have been correlated with favorable post-ablation clinical outcomes.
On the contrary, there are many clinical reports with accelerated tumor progression after ablation therapy, questioning if the post-ablation environment may be in fact pro-oncogenic (32–35). There are studies showing that RFA is inferior to surgical resection due to a higher risk of recurrence and metastasis (36–39), with 5-year overall recurrence rates of 63.5% compared to 41.7% in surgical resection (40). Other studies have shown rapid tumor progression in some patients after RFA, which may be due to residual viable tumors after insufficient RFA (IRFA) (41–46). There are several suggested explanations for prooncogenic response in the post-ablation period. Rozenblum et al. showed that liver regeneration induced by RF ablation facilitates c-met/hepatocyte growth factor axis-dependent hepatocellular carcinoma (HCC) formation and blockage of IL-6 or c-met significantly reduced hepatocyte proliferation in animal models (35, 47). Another study by Kumar et al. showed that post-ablation STAT3 (signal transducer and activator of transcription 3) activation is linked to increased distant tumor stimulation in animal models (48). In addition, studies found factors such as epithelial-mesenchymal transitions (EMTs), increased number of cancer stem cells (CSCs), sustained local inflammation and hypoxic microenvironment, and heat shock response could explain rapid tumor progression after thermal ablation (49).
Ablation modality-specific immunomodulation
While all ablation techniques share eventual tissue destruction or elimination as a common endpoint, the mechanisms by which tissue is manipulated to achieve that end point differs substantially, and the resultant immunological effects might be expected to be similarly discrepant. In general, RFA is historically the most thoroughly studied and serves as the archetypal hyperthermic ablation modality.
The immunomodulatory effects of RFA are a complex interplay of both adaptive immunity activation and immunosuppressive effects. In general, in response to tumor ablation, numerous pro-inflammatory physiological pathways are activated, resulting in the upregulation of signaling molecules including IL(interleukin)-1β, IL-1α, IL-6, IL-8, IL-18, and TNFα (tumor necrosis factor α) (22, 47, 50, 51). Simultaneously, immunosuppressive molecules including IL-10 and transforming growth factor-beta are also elevated, and immunosuppressive T regulatory cells are decreased, reflecting the nuanced relationship between ablation and immune response (51, 52). Additional classes of signaling molecules, including “danger signals,” typically released by necrotic cells and implicated in the process of antigen presentation, are also affected, with extracellular heat shock protein 70 (HSP70) and high morbidity group box-1 (HMGB-1) among those studied (53–56). Further complicating our understanding of this complex interplay is the apparent heterogeneity with which these individual mechanisms are employed in any given patient, as evidenced by conflicting findings of up or down-regulation of various cytokines and regulatory T cells (47, 52, 57). At least some of this heterogeneity may be attributable to technical differences across patients, including treatment efficacy, with pro-tumoral molecules, including TNF-alpha, significantly higher among patients with subsequent tumor recurrence (58).
When compared head-to-head to alternative modalities, cryoablation has been shown to have the highest degree of immunomodulatory effects (59–62). Indeed, the well-described phenomenon of “cryoshock” whereby a hyper-activated immunological response to tumoral antigens results in a potentially lethal systemic inflammatory response represents an extreme example (63–66). Mechanistically, the relatively robust immune response following cryoablation is attributed to preservation of tumoral antigens, released from the ablation core, where necrotic cell death results from osmotic shock or physical damage, allowing spillage and subsequent uptake of these antigens by antigen presenting cells. This stands in contrast to hyperthermic ablation which results in coagulative necrosis with relatively more destruction of cellular proteins, leaving fewer viable antigens on which to predicate an immune response.
Studies of microwave ablation demonstrate the modality to have relatively fewer immunomodulatory effects; for example, serum levels of inflammatory cytokines have been shown to be lower after MWA as compared to RFA or cryoablation (59, 67). Even so, clinical reports of abscopal effects have been reported, and benchtop research has demonstrated increased tumor-specific T cell populations in HCC, as well as increased B-cell/CD4+ interactions in breast cancer (67–70). When present, these immunomodulatory effects connote an improved prognosis (68). Furthermore, “mild” microwave ablation at 48 degrees celsius has been shown to increase apoptotic rate in an in vitro and in vivo osteosarcoma model, when added to transforming growth factor (TGF) beta-1 inhibitor and heat shock protein 90 (HSP90) inhibitor, suggesting the immunomodulatory effects of microwave ablation may become increasingly appreciable in the era of immunomodulatory medications (71).
HIFU employs focused ultrasound, resulting in repeated collapse and growth of microbubbles within the target tissue, leading to mechanical disruption and boiling, with hyperthermic ablation and histotripsy as the end result (72–74). Histotripsy, which refers to cellular disruption into a liquified acellular homogenate, represents an immunological unique feature of HIFU as compared to other hyperthermic modalities. For example, in a study comparing tumor cells heated to below 55°C relative to above 80°C, there was increased dendritic cell maturation in the lower temperature group, suggesting histotripsy as an important underlying driver of the immune response, at least when allowed to predominate over coagulative necrosis (75). Additional studies have demonstrated the abscopal effects of HIFU, including showing an immunological benefit to HIFU in a melanoma model and neuroblastoma model (59, 76, 77).
The immune effects after laser ablation are relatively less well understood, as compared to other ablative modalities. One study of 13 consecutive patients undergoing percutaneous liver laser ablation demonstrated postprocedural increases in IL-6 and Tumor Necrosis Factor (TNF) Receptor 1, but not TNF-alpha, IL-1 beta, or IL-10 (78). In a murine model of colorectal liver metastases, CD3+ T cell accumulation was noted at the site of laser ablation, as well as within remote sites of disease (79). In a study of 11 patients undergoing hepatic laser ablation of colorectal metastases, the cytolytic activity of CD3+, CD4+, and CD8+ T cells was seen to increase after ablation (80).
As a younger ablative modality, the immune effects of IRE are also less well understood than RFA or cryoablation, especially as its mechanism for cell destruction - introducing nanopores into the lipid bi-layers of cell membranes - is so different from previously studied modalities (81). Early research that has been done on the immune effects of IRE show minimal inflammatory or fibrotic margins in the ablation zone, increased peripheral T lymphocytes when compared to surgical resection, and greater CD3+ T lymphocytes when compared to cryoablation (81–83). Recently, IRE’s upregulation of IFNγ has been suggested as a potential co-therapeutic target for immune regulatory medications (84).
Synergistic effect of ablation and immunotherapy
As tumor ablation has been shown to prime one’s immune system by releasing tumor neoantigens, numerous studies in recent years have investigated the combinatory effects of ablation and adjuvant immunotherapy with the hopes of demonstrating their synergistic effects (1) (Table 1). Extending beyond investigations of the mechanisms underlying the interactions between ablation and immunotherapeutic agent administration, several clinical trials and studies have been implemented in pursuit of confirming potential clinical advantages of combination therapy. Difficulties remain in directly correlating outcomes and post-treatment immune modulation, though there are many promising results.
Combinations of ablation with immune checkpoint inhibitors (ICI) in preclinical and clinical studies have offered favorable prospects. PD-1 blockade with nivolumab or pembrolizumab has been of particular interest for potential synergy with thermal ablative techniques, owing to evidence of a theoretical influence of PD-L1/PD-1 expression on RFA-induced antitumor immunity (85). One proof-of-concept clinical trial demonstrated that subtotal RFA or MWA may augment the tumor-directed immune response stimulated by these agents to increase the overall response rate and improve median overall survival (OS) in patients with HCC (86). Likewise, survival advantages have been suggested with camrelizumab (anti-PD-1) combined with RFA, shown in a propensity score matching analysis of 127 patients to improve 1-year recurrence-free survival (RFS) (87). In another pilot study, 15 patients with melanoma metastases to the liver who underwent cryoablation and pembrolizumab experienced persistently increased serum IL-6 levels immediately after cryoablation (88). At three weeks following treatment, there was a significant increase in Natural Killer (NK) cells and a decrease in regulatory T (Treg) cells. Case reports have furthermore reported successful treatment of colorectal metastases to the lung with the combination of MWA and pembrolizumab (89), as well as of metastatic clear cell RCC with the combination of cryoablation and nivolumab (90).
The use of ablation in conjunction with CTLA-4 blockade, especially by means of tremelimumab administration, may generate favorable immune processes in patients as well. In a pilot study of 12 BCLC stage C patients with metastatic HCC that did not respond to sorafenib treatment, Duffy and colleagues revealed that treatment with RFA and chemoablation therapy combined with tremelimumab led to favorable objective treatment responses, with median TTP (time to progression) of 7.4 months and median OS of 10.1 months (91), as well as a decreased volume of and increased active CD8+ cytotoxic lymphocyte (CTL) infiltration into distant lesions. The authors then went on to evaluate the efficacy of combining tremelimumab with RFA, cryoablation, or Transarterial Chemoembolization (TACE) in treating HCC (92) and with MWA in treating advanced biliary tract cancer (BTC) (93). The feasibility and safety of combination therapy were successfully demonstrated in both instances and data revealed early evidence that tremelimumab with MWA may produce differential systemic immune responses in BTC and HCC patients (93). However, all 39 HCC and 20 BTC patients studied underwent tumor ablation or TACE rendered it impossible to address the question of whether locoregional therapy enhanced the effects of ICI (92). Additionally, a pilot study assessing the feasibility of neoadjuvant treatment with another anti-CTLA-4 antibody (ipilimumab) and cryoablation in patients with operable breast cancer suggested tolerability of the combination without delay of surgical intervention, along with increased activated proliferating peripheral T cells following combination therapy compared to either treatment alone (94).
There have been promising studies looking at harnessing the potentiation of the antigen presentation process in combination with ablation therapies. Two studies investigating treatment with cryoablation and GM-CSF injection in patients with metastatic RCC (95) and prostate cancer (96) showed tumor-specific CTL response in peripheral blood. Meanwhile, the adoptive transfer of stimulated dendritic cells (DCs) themselves has also proven a candidate for use with ablation. Niu and colleagues conducted a retrospective review of 106 patients with metastatic pancreatic cancer (97), and the later at 45 patients with metastatic HCC (98), demonstrating that median survival and overall survival, respectively, were significantly improved when treated with a combination of cryoablation and adoptive transfer of GM-CSF-stimulated DCs than with cryoablation, immunotherapy, or chemotherapy alone (97, 98). A randomized phase I/II trial of 30 HCC patients investigated autologous dendritic cell tumor injection versus RFA combined with OK432-stimulated DC tumor injection (99). The study found that 5-year RFS rates of patients with significantly increased tumor-associated antigen (TAA)-specific T cell responses were much higher than those of other patients, aligned with results of previous studies showing that RFA increases the TAA-related T cell response in murine models of HCC (100). However, in another phase II study, adjuvant therapy with a TAA-pulsed DC vaccine prolonged RFS of patients with complete remission in non-RFA groups compared with those in the RFA-treated group (101), leaving the question of optimal DC treatment combinations yet to be clarified. The neoadjuvant and adjuvant potential of tumor antigen vaccines is also being explored. For instance, based on the finding that RFA tended to induce a significantly greater GPC3-specific CTL response in patients with GPC3-overexpressing HCC than TACE or surgical resection (102), the antitumor efficacy and resulting GPC3-specific CTL response following administration of a GPC3 peptide vaccine was established (103), and a phase II study of the combination of the vaccine with RFA or resection demonstrated improved 1-year recurrence rates (104), though differences between patients receiving surgical versus ablative local treatments remain unclear.
In addition to DCs, other cell types have also demonstrated signs of antitumor response when administered by adoptive transfer and hence have been interrogated for synergistic interactions with ablation. Researchers have long sought to capture the malignant cell-recognizing capability of natural killer (NK) cells, innate immune effector lymphocytes that utilize self-ligand recognition to issue rapid destructive methods against stressed cells (105), in cancer immunotherapy. In an early pilot clinical study of NK cell transfer and cryoablation in 48 patients with treatment-resistant breast cancer, effector T cells and Th1-type cytokines were increased in the peripheral blood of patients who received the combined treatment, but progression-free survival (PFS) was not significantly augmented in this group compared to those who only underwent cryoablation (106). Similar peripheral immune cell trends were seen in two prospective studies looking at this combination in 60 patients with metastatic RCC (107) and in 60 patients with non-small cell lung cancer (108), though data regarding survival were not reported. Clinical effects of NK cell immunotherapy were also studied in conjunction with IRE in primary liver cancer patients and were suggested to improve survival and elicit greater immune responses than IRE without immunotherapy (109).
Cytokine-induced killer (CIK) cells are non-major histocompatibility complex (MHC) restricted cytotoxic lymphocytes that display high proliferative and cytolytic activity (110), obtained by in vitro co-culture of human PBMCs and multiple cytokines (111). Existing data have offered substantial support for the possible benefits of CIK cell therapy in treating cancers in combination with other immunomodulatory modalities, with clinical investigations focusing mainly on implications in HCC treatment. A phase I study in ten HCC patients with chronic hepatitis B treated with MWA along with adoptive immunotherapy involving DC, CTL, and CIK infusion demonstrated the safety of this sequential combination of therapies and consequent signs of ameliorated immune suppression on peripheral blood analyses (112). Similar alterations to peripheral lymphocyte populations were seen in a prospective phase II study of 14 HCC patients treated with MWA with CIK cells alone (113), and this combination of therapies further been demonstrated in a study of 43 HCC patients to improve median OS and PFS when combined with TACE compared to MWA and TACE without immunotherapy (114). CIK cells administered in conjunction with RFA have furthermore been implicated in improving HCC treatment. OS and PFS were found to be significantly increased in a study of 30 patients with HCC treated with RFA followed by a combined infusion of CIK cells, NK cells, and γδT cells (115). In turn, when applied following a combination of RFA and TACE, CIK therapy has been revealed in meta-analysis data to be associated with higher 1-year RFS and 1- and 2-year OS than RFA plus TACE alone (116), and retrospective analyses of HCC patients treated with this triple therapy (n = 85 and n = 58, respectively) demonstrated significantly longer OS and PFS (117) as well as elevated peripheral Tregs and NK cells, lower CD8+ T cell levels, and higher general functioning scores than those who did not receive immunotherapy (118). In a prospective randomized trial, 45 HCC patients who underwent autologous CIK cell transfusion combined with RFA and TACE experienced no serious side effects and demonstrated reduced 1-year recurrence rates than those who received only RFA and TACE (9% versus 30%) (119). One multicenter, randomized phase III clinical study enrolled 230 HCC patients who had undergone surgical resection, RFA, or percutaneous ethanol injection (PEI), who were then randomly assigned to receive or not receive CIK cell infusions. The group assigned to undergo combination therapy with CIK cells had a median PFS of 44 months and had significantly longer OS and tumor-specific survival rates than the control group (101). Finally, one single-arm prospective study of 7 patients with HCC treated with RFA followed by autologous T lymphocytes stimulated with a combination of immobilized RetroNectin and anti-CD3 monoclonal antibody, which has been suggested to induce greater T cell expansion than other known methods (120, 121), demonstrated the safety of this treatment approach as well as improvements in circulating lymphocyte profiles (122), opening up avenues for the investigation of additional forms of adjuvant adoptive immunotherapy and their role in ablative cancer treatment.
Ongoing trials and future direction
As of September 2022, 60 clinical trials studying combinations of various ablation and immunotherapy are registered to be planned or underway at clinicaltrials.gov (Table 2). Among these are a few notable large, multicenter phase III trials, including EMERALD-2 and CA2099DX. Most of the clinical trial landscape, however, remains dominated by outcome-based studies of small sample sizes and great variability in treatment parameters and protocols, reflecting rapid recent advancements in both interventional oncology and immuno-oncology. Therefore, in addition to optimizing combination treatment regimens, a detailed understanding of the mechanisms and key players of synergistic interaction should be prioritized for future studies. For example, identifications of biomarkers from post-ablation tumor microenvironments will help us to select the most effective, personalized therapeutic strategy among multiple available modalities and possible combinations with immunotherapy agents.
Conclusion
Percutaneous ablation treatment offered by interventional oncology is not only effective in local tumor control but also capable of releasing tumor neoantigens in situ. Although successful in priming the immune system, this rarely turns into clinical benefits of local and systemic tumor control due to intrinsic negative immune modulation of the tumor microenvironment. A combination of ablation and immunotherapy has shown preliminary but promising results of synergistic effect without significantly increased risk profiles. Synergistic interaction between tumor ablation and immunotherapy will further our understandings of cancer generation and treatment, which will greatly benefit scientists and patients together.
Author contributions
DK contributed to conception and oversaw the entire review. NK, JY, AT, JL and DK performed literature reviews and wrote sections of the manuscript. NK and JY created tables. NK created the graphics. All authors contributed to manuscript revision, read, and approved the submitted version.
Conflict of interest
The authors declare that the research was conducted in the absence of any commercial or financial relationships that could be construed as a potential conflict of interest.
Publisher’s note
All claims expressed in this article are solely those of the authors and do not necessarily represent those of their affiliated organizations, or those of the publisher, the editors and the reviewers. Any product that may be evaluated in this article, or claim that may be made by its manufacturer, is not guaranteed or endorsed by the publisher.
References
1. Kim D, Erinjeri JP. Postablation immune microenvironment: Synergy between interventional oncology and immuno-oncology. Semin Intervent Radiol (2019) 36(4):334–42. doi: 10.1055/s-0039-1696704
2. Waitz R, Solomon SB. Can local radiofrequency ablation of tumors generate systemic immunity against metastatic disease? Radiology. (2009) 251(1):1–2. doi: 10.1148/radiol.2511082215
3. Chu KF, Dupuy DE. Thermal ablation of tumours: biological mechanisms and advances in therapy. Nat Rev Canc (2014) 14(3):199–208. doi: 10.1038/nrc3672
4. Ahmed M, Brace CL, Lee FT Jr., Goldberg SN. Principles of and advances in percutaneous ablation. Radiology. (2011) 258(2):351–69. doi: 10.1148/radiol.10081634
5. Zhong J, Bambrook J, Bhambra B, Smith J, Cartledge J, Ralph C, et al. Incidence of post-ablation syndrome following image-guided percutaneous cryoablation of renal cell carcinoma: A prospective study. Cardiovasc Intervent Radiol (2018) 41(2):270–6. doi: 10.1007/s00270-017-1811-1
6. Dodd GD 3rd, Napier D, Schoolfield JD, Hubbard L. Percutaneous radiofrequency ablation of hepatic tumors: postablation syndrome. AJR Am J Roentgenol (2005) 185(1):51–7. doi: 10.2214/ajr.185.1.01850051
7. Wah TM, Arellano RS, Gervais DA, Saltalamacchia CA, Martino J, Halpern EF, et al. Image-guided percutaneous radiofrequency ablation and incidence of post-radiofrequency ablation syndrome: prospective survey. Radiology. (2005) 237(3):1097–102. doi: 10.1148/radiol.2373042008
8. Nikfarjam M, Muralidharan V, Christophi C. Mechanisms of focal heat destruction of liver tumors. J Surg Res (2005) 127(2):208–23. doi: 10.1016/j.jss.2005.02.009
9. Sabel MS. Cryo-immunology: A review of the literature and proposed mechanisms for stimulatory versus suppressive immune responses. Cryobiology. (2009) 58(1):1–11. doi: 10.1016/j.cryobiol.2008.10.126
10. Basu S, Binder RJ, Suto R, Anderson KM, Srivastava PK. Necrotic but not apoptotic cell death releases heat shock proteins, which deliver a partial maturation signal to dendritic cells and activate the NF-kappa b pathway. Int Immunol (2000) 12(11):1539–46. doi: 10.1093/intimm/12.11.1539
11. Bottero V, Withoff S, Verma IM. NF-kappaB and the regulation of hematopoiesis. Cell Death Differ (2006) 13(5):785–97. doi: 10.1038/sj.cdd.4401888
12. Slovak R, Ludwig JM, Gettinger SN, Herbst RS, Kim HS. Immuno-thermal ablations - boosting the anticancer immune response. J Immunother Canc (2017) 5(1):78. doi: 10.1186/s40425-017-0284-8
13. Pasparakis M, Vandenabeele P. Necroptosis and its role in inflammation. Nature. (2015) 517(7534):311–20. doi: 10.1038/nature14191
14. Vanden Berghe T, Linkermann A, Jouan-Lanhouet S, Walczak H, Vandenabeele P. Regulated necrosis: the expanding network of non-apoptotic cell death pathways. Nat Rev Mol Cell Biol (2014) 15(2):135–47. doi: 10.1038/nrm3737
15. Zhang Y, Lyu C, Liu Y, Lv Y, Chang TT, Rubinsky B. Molecular and histological study on the effects of non-thermal irreversible electroporation on the liver. Biochem Biophys Res Commun (2018) 500(3):665–70. doi: 10.1016/j.bbrc.2018.04.132
16. Lopez-Alonso B, Hernaez A, Sarnago H, Naval A, Guemes A, Junquera C, et al. Histopathological and ultrastructural changes after electroporation in pig liver using parallel-plate electrodes and high-performance generator. Sci Rep (2019) 9(1):2647. doi: 10.1038/s41598-019-39433-6
17. Sabel MS, Su G, Griffith KA, Chang AE. Rate of freeze alters the immunologic response after cryoablation of breast cancer. Ann Surg Oncol (2010) 17(4):1187–93. doi: 10.1245/s10434-009-0846-1
18. Velez E, Goldberg SN, Kumar G, Wang Y, Gourevitch S, Sosna J, et al. Hepatic thermal ablation: Effect of device and heating parameters on local tissue reactions and distant tumor growth. Radiology. (2016) 281(3):782–92. doi: 10.1148/radiol.2016152241
19. Salvadori S, Martinelli G, Zier K. Resection of solid tumors reverses T cell defects and restores protective immunity. J Immunol (2000) 164(4):2214–20. doi: 10.4049/jimmunol.164.4.2214
20. Fatourou EM, Koskinas JS. Adaptive immunity in hepatocellular carcinoma: prognostic and therapeutic implications. Expert Rev Anticancer Ther (2009) 9(10):1499–510. doi: 10.1586/era.09.103
21. Schueller G, Stift A, Friedl J, Dubsky P, Bachleitner-Hofmann T, Benkoe T, et al. Hyperthermia improves cellular immune response to human hepatocellular carcinoma subsequent to co-culture with tumor lysate pulsed dendritic cells. Int J Oncol (2003) 22(6):1397–402.
22. Ali MY, Grimm CF, Ritter M, Mohr L, Allgaier HP, Weth R, et al. Activation of dendritic cells by local ablation of hepatocellular carcinoma. J Hepatol (2005) 43(5):817–22. doi: 10.1016/j.jhep.2005.04.016
23. Hansler J, Wissniowski TT, Schuppan D, Witte A, Bernatik T, Hahn EG, et al. Activation and dramatically increased cytolytic activity of tumor specific T lymphocytes after radio-frequency ablation in patients with hepatocellular carcinoma and colorectal liver metastases. World J Gastroenterol (2006) 12(23):3716–21. doi: 10.3748/wjg.v12.i23.3716
24. Kim H, Park BK, Kim CK. Spontaneous regression of pulmonary and adrenal metastases following percutaneous radiofrequency ablation of a recurrent renal cell carcinoma. Korean J Radiol (2008) 9(5):470–2. doi: 10.3348/kjr.2008.9.5.470
25. Rao P, Escudier B, de Baere T. Spontaneous regression of multiple pulmonary metastases after radiofrequency ablation of a single metastasis. Cardiovasc Intervent Radiol (2011) 34(2):424–30. doi: 10.1007/s00270-010-9896-9
26. Sanchez-Ortiz RF, Tannir N, Ahrar K, Wood CG. Spontaneous regression of pulmonary metastases from renal cell carcinoma after radio frequency ablation of primary tumor: an in situ tumor vaccine? J Urol (2003) 170(1):178–9. doi: 10.1097/01.ju.0000070823.38336.7b
27. Soanes WA, Ablin RJ, Gonder MJ. Remission of metastatic lesions following cryosurgery in prostatic cancer: immunologic considerations. J Urol (1970) 104(1):154–9. doi: 10.1016/s0022-5347(17)61690-2
28. Facciorusso A, Del Prete V, Crucinio N, Serviddio G, Vendemiale G, Muscatiello N. Lymphocyte-to-monocyte ratio predicts survival after radiofrequency ablation for colorectal liver metastases. World J Gastroenterol (2016) 22(16):4211–8. doi: 10.3748/wjg.v22.i16.4211
29. Dan J, Zhang Y, Peng Z, Huang J, Gao H, Xu L, et al. Postoperative neutrophil-to-lymphocyte ratio change predicts survival of patients with small hepatocellular carcinoma undergoing radiofrequency ablation. PloS One (2013) 8(3):e58184. doi: 10.1371/journal.pone.0058184
30. Chang X, Zhang F, Liu T, Wang W, Guo H. Neutrophil-to-lymphocyte ratio as an independent predictor for survival in patients with localized clear cell renal cell carcinoma after radiofrequency ablation: a propensity score matching analysis. Int Urol Nephrol (2017) 49(6):967–74. doi: 10.1007/s11255-017-1554-6
31. Zerbini A, Pilli M, Laccabue D, Pelosi G, Molinari A, Negri E, et al. Radiofrequency thermal ablation for hepatocellular carcinoma stimulates autologous NK-cell response. Gastroenterology. (2010) 138(5):1931–42. doi: 10.1053/j.gastro.2009.12.051
32. Ahmed M, Kumar G, Moussa M, Wang Y, Rozenblum N, Galun E, et al. Hepatic radiofrequency ablation-induced stimulation of distant tumor growth is suppressed by c-met inhibition. Radiology. (2016) 279(1):103–17. doi: 10.1148/radiol.2015150080
33. Kang TW, Lim HK, Lee MW, Kim YS, Rhim H, Lee WJ, et al. Aggressive intrasegmental recurrence of hepatocellular carcinoma after radiofrequency ablation: Risk factors and clinical significance. Radiology. (2015) 276(1):274–85. doi: 10.1148/radiol.15141215
34. Nikfarjam M, Muralidharan V, Christophi C. Altered growth patterns of colorectal liver metastases after thermal ablation. Surgery. (2006) 139(1):73–81. doi: 10.1016/j.surg.2005.07.030
35. Rozenblum N, Zeira E, Scaiewicz V, Bulvik B, Gourevitch S, Yotvat H, et al. Oncogenesis: An "Off-target" effect of radiofrequency ablation. Radiology. (2015) 276(2):426–32. doi: 10.1148/radiol.2015141695
36. Kong J, Yao C, Dong S, Wu S, Xu Y, Li K, et al. ICAM-1 activates platelets and promotes endothelial permeability through VE-cadherin after insufficient radiofrequency ablation. Adv Sci (Weinh) (2021) 8(4):2002228. doi: 10.1002/advs.202002228
37. Lee S, Kang TW, Cha DI, Song KD, Lee MW, Rhim H, et al. Radiofrequency ablation vs. surgery for perivascular hepatocellular carcinoma: Propensity score analyses of long-term outcomes. J Hepatol (2018) 69(1):70–8. doi: 10.1016/j.jhep.2018.02.026
38. Lee S, Kang TW, Song KD, Lee MW, Rhim H, Lim HK, et al. Effect of microvascular invasion risk on early recurrence of hepatocellular carcinoma after surgery and radiofrequency ablation. Ann Surg (2021) 273(3):564–71. doi: 10.1097/SLA.0000000000003268
39. Yang JD, Hainaut P, Gores GJ, Amadou A, Plymoth A, Roberts LR. A global view of hepatocellular carcinoma: trends, risk, prevention and management. Nat Rev Gastroenterol Hepatol (2019) 16(10):589–604. doi: 10.1038/s41575-019-0186-y
40. Huang J, Yan L, Cheng Z, Wu H, Du L, Wang J, et al. A randomized trial comparing radiofrequency ablation and surgical resection for HCC conforming to the Milan criteria. Ann Surg (2010) 252(6):903–12. doi: 10.1097/SLA.0b013e3181efc656
41. Koda M, Maeda Y, Matsunaga Y, Mimura K, Murawaki Y, Horie Y. Hepatocellular carcinoma with sarcomatous change arising after radiofrequency ablation for well-differentiated hepatocellular carcinoma. Hepatol Res (2003) 27(2):163–7. doi: 10.1016/s1386-6346(03)00207-9
42. Lam VW, Ng KK, Chok KS, Cheung TT, Yuen J, Tung H, et al. Incomplete ablation after radiofrequency ablation of hepatocellular carcinoma: analysis of risk factors and prognostic factors. Ann Surg Oncol Mar (2008) 15(3):782–90. doi: 10.1245/s10434-007-9733-9
43. Portolani N, Tiberio GA, Ronconi M, Coniglio A, Ghidoni S, Gaverini G, et al. Aggressive recurrence after radiofrequency ablation of liver neoplasms. Hepatogastroenterology. (2003) 50(54):2179–84.
44. Ruzzenente A, Manzoni GD, Molfetta M, Pachera S, Genco B, Donataccio M, et al. Rapid progression of hepatocellular carcinoma after radiofrequency ablation. World J Gastroenterol (2004) 10(8):1137–40. doi: 10.3748/wjg.v10.i8.1137
45. Seki T, Tamai T, Ikeda K, Imamura M, Nishimura A, Yamashiki N, et al. Rapid progression of hepatocellular carcinoma after transcatheter arterial chemoembolization and percutaneous radiofrequency ablation in the primary tumour region. Eur J Gastroenterol Hepatol (2001) 13(3):291–4. doi: 10.1097/00042737-200103000-00014
46. Takada Y, Kurata M, Ohkohchi N. Rapid and aggressive recurrence accompanied by portal tumor thrombus after radiofrequency ablation for hepatocellular carcinoma. Int J Clin Oncol (2003) 8(5):332–5. doi: 10.1007/s10147-003-0328-6
47. Rozenblum N, Zeira E, Bulvik B, Gourevitch S, Yotvat H, Galun E, et al. Radiofrequency ablation: Inflammatory changes in the periablative zone can induce global organ effects, including liver regeneration. Radiology. (2015) 276(2):416–25. doi: 10.1148/radiol.15141918
48. Kumar G, Goldberg SN, Gourevitch S, Levchenko T, Torchilin V, Galun E, et al. Targeting STAT3 to suppress systemic pro-oncogenic effects from hepatic radiofrequency ablation. Radiology. (2018) 286(2):524–36. doi: 10.1148/radiol.2017162943
49. Guo Y, Ren Y, Dong X, Kan X, Zheng C. An overview of hepatocellular carcinoma after insufficient radiofrequency ablation. J Hepatocell Carcinoma (2022) 9:343–55. doi: 10.2147/JHC.S358539
50. Evrard S, Menetrier-Caux C, Biota C, Neaud V, Mathoulin-Pelissier S, Blay JY, et al. Cytokines pattern after surgical radiofrequency ablation of liver colorectal metastases. Gastroenterol Clin Biol (2007) 31(2):141–5. doi: 10.1016/s0399-8320(07)89344-4
51. Fietta AM, Morosini M, Passadore I, Cascina A, Draghi P, Dore R, et al. Systemic inflammatory response and downmodulation of peripheral CD25+Foxp3+ T-regulatory cells in patients undergoing radiofrequency thermal ablation for lung cancer. Hum Immunol (2009) 70(7):477–86. doi: 10.1016/j.humimm.2009.03.012
52. Iwahashi S, Shimada M, Utsunomiya T, Imura S, Morine Y, Ikemoto T, et al. Epithelial-mesenchymal transition-related genes are linked to aggressive local recurrence of hepatocellular carcinoma after radiofrequency ablation. Cancer Lett (2016) 375(1):47–50. doi: 10.1016/j.canlet.2016.02.041
53. Figueiredo C, Wittmann M, Wang D, Dressel R, Seltsam A, Blasczyk R, et al. Heat shock protein 70 (HSP70) induces cytotoxicity of T-helper cells. Blood. (2009) 113(13):3008–16. doi: 10.1182/blood-2008-06-162727
54. Rai R, Richardson C, Flecknell P, Robertson H, Burt A, Manas DM. Study of apoptosis and heat shock protein (HSP) expression in hepatocytes following radiofrequency ablation (RFA). J Surg Res (2005) 129(1):147–51. doi: 10.1016/j.jss.2005.03.020
55. Schueller G, Kettenbach J, Sedivy R, Stift A, Friedl J, Gnant M, et al. Heat shock protein expression induced by percutaneous radiofrequency ablation of hepatocellular carcinoma in vivo. Int J Oncol (2004) 24(3):609–13.
56. Yang WL, Nair DG, Makizumi R, Gallos G, Ye X, Sharma RR, et al. Heat shock protein 70 is induced in mouse human colon tumor xenografts after sublethal radiofrequency ablation. Ann Surg Oncol (2004) 11(4):399–406. doi: 10.1245/ASO.2004.08.013
57. Schell SR, Wessels FJ, Abouhamze A, Moldawer LL, Copeland EM 3rd. Pro- and antiinflammatory cytokine production after radiofrequency ablation of unresectable hepatic tumors. J Am Coll Surg (2002) 195(6):774–81. doi: 10.1016/s1072-7515(02)01333-9
58. Schneider T, Sevko A, Heussel CP, Umansky L, Beckhove P, Dienemann H, et al. Serum inflammatory factors and circulating immunosuppressive cells are predictive markers for efficacy of radiofrequency ablation in non-small-cell lung cancer. Clin Exp Immunol (2015) 180(3):467–74. doi: 10.1111/cei.12596
59. Ahmad F, Gravante G, Bhardwaj N, Strickland A, Basit R, West K, et al. Changes in interleukin-1beta and 6 after hepatic microwave tissue ablation compared with radiofrequency, cryotherapy and surgical resections. Am J Surg (2010) 200(4):500–6. doi: 10.1016/j.amjsurg.2009.12.025
60. Erinjeri JP, Thomas CT, Samoilia A, Fleisher M, Gonen M, Sofocleous CT, et al. Image-guided thermal ablation of tumors increases the plasma level of interleukin-6 and interleukin-10. J Vasc Interv Radiol (2013) 24(8):1105–12. doi: 10.1016/j.jvir.2013.02.015
61. Jansen MC, van Hillegersberg R, Schoots IG, Levi M, Beek JF, Crezee H, et al. Cryoablation induces greater inflammatory and coagulative responses than radiofrequency ablation or laser induced thermotherapy in a rat liver model. Surgery. (2010) 147(5):686–95. doi: 10.1016/j.surg.2009.10.053
62. Ravindranath MH, Wood TF, Soh D, Gonzales A, Muthugounder S, Perez C, et al. Cryosurgical ablation of liver tumors in colon cancer patients increases the serum total ganglioside level and then selectively augments antiganglioside IgM. Cryobiology. (2002) 45(1):10–21. doi: 10.1016/s0011-2240(02)00102-5
63. Chapman WC, Debelak JP, Wright Pinson C, Washington MK, Atkinson JB, Venkatakrishnan A, et al. Hepatic cryoablation, but not radiofrequency ablation, results in lung inflammation. Ann Surg May (2000) 231(5):752–61. doi: 10.1097/00000658-200005000-00016
64. Seifert JK, France MP, Zhao J, Bolton EJ, Finlay I, Junginger T, et al. Large Volume hepatic freezing: association with significant release of the cytokines interleukin-6 and tumor necrosis factor a in a rat model. World J Surg (2002) 26(11):1333–41. doi: 10.1007/s00268-002-6139-5
65. Seifert JK, Morris DL. World survey on the complications of hepatic and prostate cryotherapy. World J Surg (1999) 23(2):109–13; discussion 113-4. doi: 10.1007/pl00013173
66. Washington K, Debelak JP, Gobbell C, Sztipanovits DR, Shyr Y, Olson S, et al. Hepatic cryoablation-induced acute lung injury: histopathologic findings. J Surg Res (2001) 95(1):1–7. doi: 10.1006/jsre.2000.5976
67. Ahmad F, Gravante G, Bhardwaj N, Strickland A, Basit R, West K, et al. Renal effects of microwave ablation compared with radiofrequency, cryotherapy and surgical resection at different volumes of the liver treated. Liver Int (2010) 30(9):1305–14. doi: 10.1111/j.1478-3231.2010.02290.x
68. Leuchte K, Staib E, Thelen M, Godel P, Lechner A, Zentis P, et al. Microwave ablation enhances tumor-specific immune response in patients with hepatocellular carcinoma. Cancer Immunol Immunother (2021) 70(4):893–907. doi: 10.1007/s00262-020-02734-1
69. Xu H, Sun W, Kong Y, Huang Y, Wei Z, Zhang L, et al. Immune abscopal effect of microwave ablation for lung metastases of endometrial carcinoma. J Cancer Res Ther (2020) 16(7):1718–21. doi: 10.4103/jcrt.JCRT_1399_20
70. Zhou W, Yu M, Mao X, Pan H, Tang X, Wang J, et al. Landscape of the peripheral immune response induced by local microwave ablation in patients with breast cancer. Adv Sci (Weinh) (2022) 9(17):e2200033. doi: 10.1002/advs.202200033
71. Chen L, Wang M, Lin Z, Yao M, Wang W, Cheng S, et al. Mild microwave ablation combined with HSP90 and TGFbeta1 inhibitors enhances the therapeutic effect on osteosarcoma. Mol Med Rep (2020) 22(2):906–14. doi: 10.3892/mmr.2020.11173
72. Copelan A, Hartman J, Chehab M, Venkatesan AM. High-intensity focused ultrasound: Current status for image-guided therapy. Semin Intervent Radiol (2015) 32(4):398–415. doi: 10.1055/s-0035-1564793
73. Mauri G, Nicosia L, Xu Z, Di Pietro S, Monfardini L, Bonomo G, et al. Focused ultrasound: tumour ablation and its potential to enhance immunological therapy to cancer. Br J Radiol (1083) 2018:91. doi: 10.1259/bjr.20170641
74. Roberts WW, Hall TL, Ives K, Wolf JS Jr., Fowlkes JB, Cain CA. Pulsed cavitational ultrasound: a noninvasive technology for controlled tissue ablation (histotripsy) in the rabbit kidney. J Urol (2006) 175(2):734–8. doi: 10.1016/S0022-5347(05)00141-2
75. Liu F, Hu Z, Qiu L, Hui C, Li C, Zhong P, et al. Boosting high-intensity focused ultrasound-induced anti-tumor immunity using a sparse-scan strategy that can more effectively promote dendritic cell maturation. J Transl Med (2010) 8:7. doi: 10.1186/1479-5876-8-7
76. Xing Y, Lu X, Pua EC, Zhong P. The effect of high intensity focused ultrasound treatment on metastases in a murine melanoma model. Biochem Biophys Res Commun (2008) 375(4):645–50. doi: 10.1016/j.bbrc.2008.08.072
77. Yang R, Reilly CR, Rescorla FJ, Sanghvi NT, Fry FJ, Franklin TD Jr, et al. Effects of high-intensity focused ultrasound in the treatment of experimental neuroblastoma. J Pediatr Surg (1992) 27(2):246–50. doi: 10.1016/0022-3468(92)90321-w
78. Kallio R, Sequeiros R, Surcel HM, Ohtonen P, Kiviniemi H, Syrjala H. Early cytokine responses after percutaneous magnetic resonance imaging guided laser thermoablation of malignant liver tumors. Cytokine (2006) 34(5-6):278–83. doi: 10.1016/j.cyto.2006.06.003
79. Lin WX, Fifis T, Malcontenti-Wilson C, Nikfarjam M, Muralidharan V, Nguyen L, et al. Induction of Th1Immune responses following laser ablation in a murine model of colorectal liver metastases. J Transl Med (2011) 9:83. doi: 10.1186/1479-5876-9-83
80. Vogl TJ, Wissniowski TT, Naguib NN, Hammerstingl RM, Mack MG, Munch S, et al. Activation of tumor-specific T lymphocytes after laser-induced thermotherapy in patients with colorectal liver metastases. Cancer Immunol Immunother (2009) 58(10):1557–63. doi: 10.1007/s00262-009-0663-1
81. Rubinsky B, Onik G, Mikus P. Irreversible electroporation: a new ablation modality–clinical implications. Technol Cancer Res Treat (2007) 6(1):37–48. doi: 10.1177/153303460700600106
82. Li X, Xu K, Li W, Qiu X, Ma B, Fan Q, et al. Immunologic response to tumor ablation with irreversible electroporation. PloS One (2012) 7(11):e48749. doi: 10.1371/journal.pone.0048749
83. White SB, Zhang Z, Chen J, Gogineni VR, Larson AC. Early immunologic response of irreversible electroporation versus cryoablation in a rodent model of pancreatic cancer. J Vasc Interv Radiol (2018) 29(12):1764–9. doi: 10.1016/j.jvir.2018.07.009
84. Imran KM, Nagai-Singer MA, Brock RM, Alinezhadbalalami N, Davalos RV, Allen IC. Exploration of novel pathways underlying irreversible electroporation induced anti-tumor immunity in pancreatic cancer. Front Oncol (2022) 12:853779. doi: 10.3389/fonc.2022.853779
85. Shi L, Chen L, Wu C, Zhu Y, Xu B, Zheng X, et al. PD-1 blockade boosts radiofrequency ablation-elicited adaptive immune responses against tumor. Clin Cancer Res (2016) 22(5):1173–84. doi: 10.1158/1078-0432.CCR-15-1352
86. Lyu N, Kong Y, Li X, Mu L, Deng H, Chen H, et al. Ablation reboots the response in advanced hepatocellular carcinoma with stable or atypical response during PD-1 therapy: A proof-of-Concept study. Front Oncol (2020) 10:580241. doi: 10.3389/fonc.2020.580241
87. Wang X, Liu G, Chen S, Bi H, Xia F, Feng K, et al. Combination therapy with PD-1 blockade and radiofrequency ablation for recurrent hepatocellular carcinoma: a propensity score matching analysis. Int J Hyperthermia (2021) 38(1):1519–28. doi: 10.1080/02656736.2021.1991011
88. Shen L, Qi H, Chen S, Cao F, Xie L, Wu Y, et al. Cryoablation combined with transarterial infusion of pembrolizumab (CATAP) for liver metastases of melanoma: an ambispective, proof-of-concept cohort study. Cancer Immunol Immunother (2020) 69(9):1713–24. doi: 10.1007/s00262-020-02566-z
89. Backlund M, Freedman J. Microwave ablation and immune activation in the treatment of recurrent colorectal lung metastases: A case report. Case Rep Oncol (2017) 10(1):383–7. doi: 10.1159/000468982
90. Soule E, Bandyk M, Matteo J. Percutaneous ablative cryoimmunotherapy for micrometastaic abscopal effect: No complications. Cryobiology (2018) 82:22–6. doi: 10.1016/j.cryobiol.2018.04.013
91. Duffy AG, Ulahannan SV, Makorova-Rusher O, Rahma O, Wedemeyer H, Pratt D, et al. Tremelimumab in combination with ablation in patients with advanced hepatocellular carcinoma. J Hepatol (2017) 66(3):545–51. doi: 10.1016/j.jhep.2016.10.029
92. Agdashian D, ElGindi M, Xie C, Sandhu M, Pratt D, Kleiner DE, et al. The effect of anti-CTLA4 treatment on peripheral and intra-tumoral T cells in patients with hepatocellular carcinoma. Cancer Immunol Immunother (2019) 68(4):599–608. doi: 10.1007/s00262-019-02299-8
93. Xie C, Duffy AG, Mabry-Hrones D, Wood B, Levy E, Krishnasamy V, et al. Tremelimumab in combination with microwave ablation in patients with refractory biliary tract cancer. Hepatology (2019) 69(5):2048–60. doi: 10.1002/hep.30482
94. McArthur HL, Diab A, Page DB, Yuan J, Solomon SB, Sacchini V, et al. A pilot study of preoperative single-dose ipilimumab and/or cryoablation in women with early-stage breast cancer with comprehensive immune profiling. Clin Cancer Res (2016) 22(23):5729–37. doi: 10.1158/1078-0432.CCR-16-0190
95. Thakur A, Littrup P, Paul EN, Adam B, Heilbrun LK, Lum LG. Induction of specific cellular and humoral responses against renal cell carcinoma after combination therapy with cryoablation and granulocyte-macrophage colony stimulating factor: a pilot study. J Immunother (2011) 34(5):457–67. doi: 10.1097/CJI.0b013e31821dcba5
96. Si T, Guo Z, Hao X. Combined cryoablation and GM-CSF treatment for metastatic hormone refractory prostate cancer. J Immunother (2009) 32(1):86–91. doi: 10.1097/CJI.0b013e31818df785
97. Niu L, Chen J, He L, Liao M, Yuan Y, Zeng J, et al. Combination treatment with comprehensive cryoablation and immunotherapy in metastatic pancreatic cancer. Pancreas. (2013) 42(7):1143–9. doi: 10.1097/MPA.0b013e3182965dde
98. Niu LZ, Li JL, Zeng JY, Mu F, Liao MT, Yao F, et al. Combination treatment with comprehensive cryoablation and immunotherapy in metastatic hepatocellular cancer. World J Gastroenterol (2013) 19(22):3473–80. doi: 10.3748/wjg.v19.i22.3473
99. Kitahara M, Mizukoshi E, Terashima T, Nakagawa H, Horii R, Iida N, et al. Safety and long-term outcome of intratumoral injection of OK432-stimulated dendritic cells for hepatocellular carcinomas after radiofrequency ablation. Transl Oncol (2020) 13(7):100777. doi: 10.1016/j.tranon.2020.100777
100. Mizukoshi E, Yamashita T, Arai K, Sunagozaka H, Ueda T, Arihara F, et al. Enhancement of tumor-associated antigen-specific T cell responses by radiofrequency ablation of hepatocellular carcinoma. Hepatology. (2013) 57(4):1448–57. doi: 10.1002/hep.26153
101. Lee JH, Lee JH, Lim YS, Yeon JE, Song TJ, Yu SJ, et al. Adjuvant immunotherapy with autologous cytokine-induced killer cells for hepatocellular carcinoma. Gastroenterology. (2015) 148(7):1383–91 e6. doi: 10.1053/j.gastro.2015.02.055
102. Nobuoka D, Motomura Y, Shirakawa H, Yoshikawa T, Kuronuma T, Takahashi M, et al. Radiofrequency ablation for hepatocellular carcinoma induces glypican-3 peptide-specific cytotoxic T lymphocytes. Int J Oncol (2012) 40(1):63–70. doi: 10.3892/ijo.2011.1202
103. Sawada Y, Yoshikawa T, Nobuoka D, Shirakawa H, Kuronuma T, Motomura Y, et al. Phase I trial of a glypican-3-derived peptide vaccine for advanced hepatocellular carcinoma: immunologic evidence and potential for improving overall survival. Clin Cancer Res (2012) 18(13):3686–96. doi: 10.1158/1078-0432.CCR-11-3044
104. Sawada Y, Yoshikawa T, Ofuji K, Yoshimura M, Tsuchiya N, Takahashi M, et al. Phase II study of the GPC3-derived peptide vaccine as an adjuvant therapy for hepatocellular carcinoma patients. Oncoimmunology. (2016) 5(5):e1129483. doi: 10.1080/2162402X.2015.1129483
105. Vivier E, Tomasello E, Baratin M, Walzer T, Ugolini S. Functions of natural killer cells. Nat Immunol (2008) 9(5):503–10. doi: 10.1038/ni1582
106. Liang S, Niu L, Xu K, Wang X, Liang Y, Zhang M, et al. Tumor cryoablation in combination with natural killer cells therapy and herceptin in patients with HER2-overexpressing recurrent breast cancer. Mol Immunol (2017) 92:45–53. doi: 10.1016/j.molimm.2017.10.003
107. Lin M, Xu K, Liang S, Wang X, Liang Y, Zhang M, et al. Prospective study of percutaneous cryoablation combined with allogenic NK cell immunotherapy for advanced renal cell cancer. Immunol Lett (2017) 184:98–104. doi: 10.1016/j.imlet.2017.03.004
108. Lin M, Liang SZ, Wang XH, Liang YQ, Zhang MJ, Niu LZ, et al. Clinical efficacy of percutaneous cryoablation combined with allogenic NK cell immunotherapy for advanced non-small cell lung cancer. Immunol Res (2017) 65(4):880–7. doi: 10.1007/s12026-017-8927-x
109. Yang Y, Qin Z, Du D, Wu Y, Qiu S, Mu F, et al. Safety and short-term efficacy of irreversible electroporation and allogenic natural killer cell immunotherapy combination in the treatment of patients with unresectable primary liver cancer. Cardiovasc Intervent Radiol (2019) 42(1):48–59. doi: 10.1007/s00270-018-2069-y
110. Linn YC, Hui KM. Cytokine-induced killer cells: NK-like T cells with cytotolytic specificity against leukemia. Leuk Lymphoma (2003) 44(9):1457–62. doi: 10.3109/10428190309178764
111. Gutgemann S, Frank S, Strehl J, Schmidt-Wolf IG. Cytokine-induced killer cells are type II natural killer T cells. Ger Med Sci (2007) 5:Doc07.
112. Zhou P, Liang P, Dong B, Yu X, Han Z, Xu Y. Phase clinical study of combination therapy with microwave ablation and cellular immunotherapy in hepatocellular carcinoma. Cancer Biol Ther (2011) 11(5):450–6. doi: 10.4161/cbt.11.5.14669
113. Yu MA, Liang P, Yu XL, Han ZY, Dong XJ, Wang YU, et al. Multiple courses of immunotherapy with different immune cell types for patients with hepatocellular carcinoma after microwave ablation. Exp Ther Med (2015) 10(4):1460–6. doi: 10.3892/etm.2015.2681
114. Huang ZM, Lai CX, Zuo MX, An C, Wang XC, Huang JH, et al. Adjuvant cytokine-induced killer cells with minimally invasive therapies augmented therapeutic efficacy of unresectable hepatocellular carcinoma. J Cancer Res Ther (2020) 16(7):1603–10. doi: 10.4103/jcrt.JCRT_962_19
115. Cui J, Wang N, Zhao H, Jin H, Wang G, Niu C, et al. Combination of radiofrequency ablation and sequential cellular immunotherapy improves progression-free survival for patients with hepatocellular carcinoma. Int J Canc (2014) 134(2):342–51. doi: 10.1002/ijc.28372
116. Li X, Dai D, Song X, Liu J, Zhu L, Xu W. A meta-analysis of cytokine-induced killer cells therapy in combination with minimally invasive treatment for hepatocellular carcinoma. Clin Res Hepatol Gastroenterol (2014) 38(5):583–91. doi: 10.1016/j.clinre.2014.04.010
117. Huang ZM, Li W, Li S, Gao F, Zhou QM, Wu FM, et al. Cytokine-induced killer cells in combination with transcatheter arterial chemoembolization and radiofrequency ablation for hepatocellular carcinoma patients. J Immunother (2013) 36(5):287–93. doi: 10.1097/CJI.0b013e3182948452
118. Ji Q, Fu Y, Zhu X, Wang L, Ling C. Effect of RFA and TACE combined with postoperative cytokine-induced killer cell immunotherapy in primary hepatocellular carcinoma. J BUON (2021) 26(1):235–42.
119. Weng DS, Zhou J, Zhou QM, Zhao M, Wang QJ, Huang LX, et al. Minimally invasive treatment combined with cytokine-induced killer cells therapy lower the short-term recurrence rates of hepatocellular carcinomas. J Immunother (2008) 31(1):63–71. doi: 10.1097/CJI.0b013e31815a121b
120. Muraki N, Ideno M, Nukaya I, Sagawa H, Enoki T, Kato I. T Cell expansion using RetroNectin (I): useful method to expand T cells, characterized by high portion of naive T-like cells. Yokohama, Japan: Sixty-Fifth Annual Meeting of the Japanese Cancer Association (2006).
121. Muraki N, Ideno M, Nukaya I, Sagawa H, Enoki T, Kato I. T Cell expansion using RetroNectin (II): RN-T cells contain high portion of naive T-like cells and show high ability of antigen recognition. Yokohama, Japan: Sixty-Fifth Annual Meeting of the Japanese Cancer Association (2006).
122. Ma H, Zhang Y, Wang Q, Li Y, He J, Wang H, et al. Therapeutic safety and effects of adjuvant autologous RetroNectin activated killer cell immunotherapy for patients with primary hepatocellular carcinoma after radiofrequency ablation. Cancer Biol Ther (2010) 9(11):903–7. doi: 10.4161/cbt.9.11.11697
Keywords: tumor microenvironment, immunogenicity, percutaneous ablation, antitumor immunity, tumor neoantigen
Citation: Kim NJ, Yoon JH, Tuomi AC, Lee J and Kim D (2023) In-situ tumor vaccination by percutaneous ablative therapy and its synergy with immunotherapeutics: An update on combination therapy. Front. Immunol. 14:1118845. doi: 10.3389/fimmu.2023.1118845
Received: 08 December 2022; Accepted: 21 February 2023;
Published: 08 March 2023.
Edited by:
Ming Kuang, The First Affiliated Hospital of Sun Yat-sen University, ChinaReviewed by:
Kun He, University of Pittsburgh, United StatesIsrael Barken, University of California, San Diego, United States
Xin Ye, Shandong Provincial Qianfoshan Hospital, China
Copyright © 2023 Kim, Yoon, Tuomi, Lee and Kim. This is an open-access article distributed under the terms of the Creative Commons Attribution License (CC BY). The use, distribution or reproduction in other forums is permitted, provided the original author(s) and the copyright owner(s) are credited and that the original publication in this journal is cited, in accordance with accepted academic practice. No use, distribution or reproduction is permitted which does not comply with these terms.
*Correspondence: Daehee Kim, ZGFlaGVlX2tpbUBicm93bi5lZHU=