- 1Department of Plastic Surgery, Shandong Provincial Qianfoshan Hospital, School of Basic Medical Sciences, Weifang Medical University, Weifang, Shandong, China
- 2Shandong Provincial Key Laboratory for Rheumatic Disease and Translational Medicine, The First Affiliated Hospital of Shandong First Medical University & Shandong Provincial Qianfoshan Hospital, Jinan, China
- 3Department of Clinical Laboratory Medicine, Shandong Public Health Clinical Center, Shandong University, Jinan, China
The peptidyl arginine deiminase (PADI) family is a calcium ion-dependent group of isozymes with sequence similarity that catalyze the citrullination of proteins. Histones can serve as the target substrate of PADI family isozymes, and therefore, the PADI family is involved in NETosis and the secretion of inflammatory cytokines. Thus, the PADI family is associated with the development of inflammatory autoimmune diseases and cancer, reproductive development, and other related diseases. In this review, we systematically discuss the role of the PADI family in the pathogenesis of various diseases based on studies from the past decade to provide a reference for future research.
Highlights
1. SNP of PADI family can endow disease susceptibility.
2. The citrullination of PADI family makes it participate in different physiological processes.
3. The role of PADI family in various physiological processes makes it participate in the occurrence of human diseases.
4. PADI family can be used as the target of disease treatment for drug development.
Introduction
The peptidyl arginine deiminase (PADI) family is composed of isozymes with sequence similarity and is located on human chromosome 1p36.13. Its family members include PADI1-4 and PADI6. The PADI family is a class of calcium ion-dependent enzymes that can catalyze the citrullination of proteins. It converts positively charged arginine into neutrally charged citrulline, thereby changing the structure and function of proteins. Studies have shown that PADI family members have unique subcellular localization and tissue distribution, which determine the functional specificity of each family member (Table 1).
As shown in Table 1, the specific distribution of the PADI family in tissues determines that the PADI family can participate in different physiological processes, which was further validated in single-cell studies of the PADI family. Downregulation of PADI1 and PADI3 in the skin of the extremities leads to alterations in filaggrin and keratin. PADI2 is associated with multiple sclerosis (MS) and posttreatment Lyme disease. PADI4 can induce arteriosclerosis by mediating the formation of NETs or promote tumor growth and metastasis by altering the tumor microenvironment. Furthermore, PADI6 is associated with the ovarian reserve (OR), oocyte maturation and early embryonic development (27–32).
SNP analysis of the PADI family showed that genetic variations in the PADI gene were significantly associated with susceptibility to multiple diseases. This allows the PADI family to participate in the development of multiple diseases, as shown in Table 2.
Therefore, PADI family members may be associated with the occurrence of diseases by participating in different physiological processes. The current research shows that the PADI family mainly participates in the occurrence of inflammatory autoimmune diseases, cancer and reproductive development-related diseases by participating in gene expression regulation, NETosis, the secretion of inflammatory cytokines, energy metabolism and the release of extracellular vesicles. The pathological mechanisms by which the PADI family acts in various diseases has never been systematically described before. In this paper, we reviewed the progress of research on the PADI family in the past decade to provide a reference for further study on the role of the PADI family in diseases, thereby promoting the application of the PADI family in the clinical treatment of human diseases.
Physiological processes involving the PADI family
Gene expression regulation
First, the PADI family can regulate chromatin status through the citrullination of histones to activate transcription. PADI2 can prevent the degradation of androgen receptor (AR), mediate H3R26Cit, promote the binding AR and its target gene, and promote the transcriptional activation of the target gene (55).
Second, the PADI family mainly regulates the state of chromatin by crosstalk between the citrullination of histones and the methylation of histones, thus controlling gene transcription. During the activation of estrogen receptor (ER) target genes, ER recruits PADI2 to the promoter of the target gene, and PADI2 catalyzes H3R26Cit to agglutinate chromatin. Thereafter, H3K27 demethylase is recruited to chromatin, leading to the transcriptional activation of ER target genes (56–58). It has also been shown that H3R26Cit mediated by PADI4 interacts with H3K27me3 (59). When PADI4 regulates the expression of p53 target genes, the interaction between PADI4 and p53 leads to the recruitment of PADI4 to the p21 promoter. This increases histone citrullination and reduces histone Arg methylation, thereby inhibiting the expression of p21, cell cycle arrest and apoptosis (60). It has also been shown that PADI2/3 can inhibit the premature differentiation of mouse trophoblastic stem cells by maintaining key DNA methylation sites (61).
In addition, the PADI family can also regulate gene transcription by acting on enzymes involved in the transcription process. The PADI2-mediated citrullination of RNA polymerase II C-terminal domain (RNAP2-CTD) R1810 facilitates RNAP2 pause release and the efficient transcription of RNAP2 (62). However, the citrullination of DNA methyltransferase DNMT3A by PADI4 increases the level of DNA methyltransferase 3A (DNMT3A). This leads to the hypermethylation of certain gene promoters, thus affecting transcription (63).
Neutrophil extracellular trap formation
NETosis is a type of reticular DNA structure that contains histones and cytotoxic proteins that are formed by neutrophils through the penetration of the plasma membrane, the decomposition of the cytoskeleton and nuclear membrane, the concentration of chromatin, and the assembly of antibacterial proteins on chromatin scaffolds when the body is stimulated by foreign invaders. Research shows that PADI4 can participate in all aspects of neutrophil extracellular traps (NETs) (64). First, PADI2 and PADI4 can cause chromatin deagglutination through citrullinated histone H3 (CitH3) and promote the release of DNA out of cells (13–16, 22, 23). Second, PADI4 can also mediate the degradation of laminin and HMGB1 through a synergistic effect with calpain, leading to nuclear membrane rupture (65). In addition, PADI4 can also participate in the decomposition of nuclear and plasma membranes by promoting the assembly of NLRP3 inflammatory bodies (66). Therefore, PADI2 and PADI4 in the PADI family can promote NETosis (Figure 1).
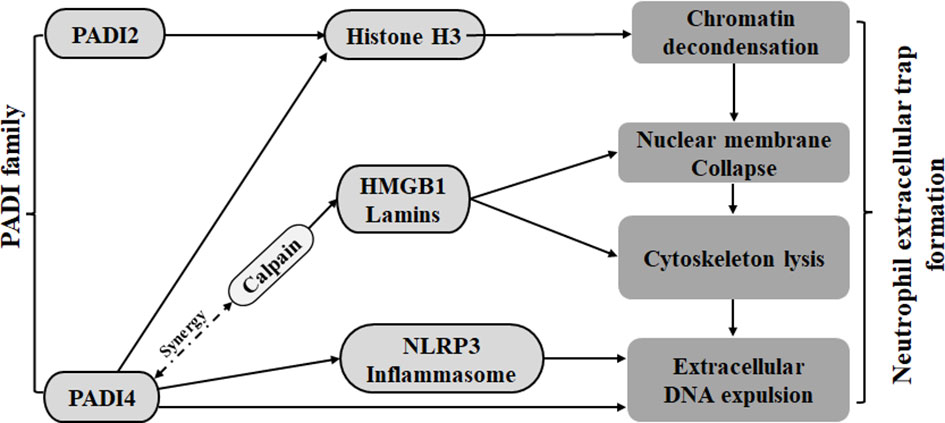
Figure 1 The PADI family promotes the formation of neutrophil traps. Both PADI2 and PADI4 can cause chromatin depolymerization by acting on histone H3. PADI4 can cooperate with calpain on HMGB1 and lamins to cause nuclear membrane disruption. PADI4 can also mediate nuclear membrane disruption and cytoskeleton fragmentation by promoting nucleosome assembly.
Secretion of inflammatory cytokines
First, the PADI family can affect cytokine secretion by participating in the differentiation and apoptosis of immune cells. In activated Jurkat cells, overexpression of PADI2 citrullinates the surface vimentin, thereby inducing the apoptosis of activated Jurkat cells (67). During the differentiation of Th cells, PADI2 can inhibit the differentiation of Th2 cells through citrullinated GATA3, thereby inhibiting the secretion of interleukin 4 (IL-4), IL-5 and IL-13. PADI2 can also promote the differentiation of Th17 cell ROR through the citrullination of RORγt, thereby promoting the secretion of IL-17A and IL-17F (68). In macrophages, PADI2 can promote the expression of IL-1β, IL-6 and TNF-α through the citrullination of NF-κB p65 (69). PADI4 can positively regulate TNF-α and CCL2 (70). PADI2 coordinates with PADI4 to regulate the assembly of the NLRP3 inflammasome to promote IL-1β release (71). In the process of macrophage differentiation, PADI2 and PADI4 lead to citrullinated PAI-2, separating PAI-2 from PSMB1. This leads to the upregulation of PAI-2 to promote the secretion of TNFα and IL-1β (72).
Second, the PADI family regulates the secretion of inflammatory cytokines by affecting nonimmune cells. In bone marrow mesenchymal stem cells, PADI2 can increase the level of IL-6 by mediating H3R26Cit (73). PADI4 can be used as an epigenetic coactivator of Tal1 to activate the expression of IL6ST, a target gene of Tal1/PADI4. This promotes cytokine signal transduction, including that of IL-6 (74). PADI4 can promote the binding of bromodomain containing protein 4 (BRD4) and the cytokine gene promoter E2F-1 through citrullination and promote the secretion of TNFα, CCL3 and IL-1β (75). PADI4 can also promote the expression of IL-1β and TNFα through the citrullination of NF-κB p65 (76). Therefore, PADI2 and PADI4 of the PADI family can promote the secretion of inflammatory cytokines (Figure 2).
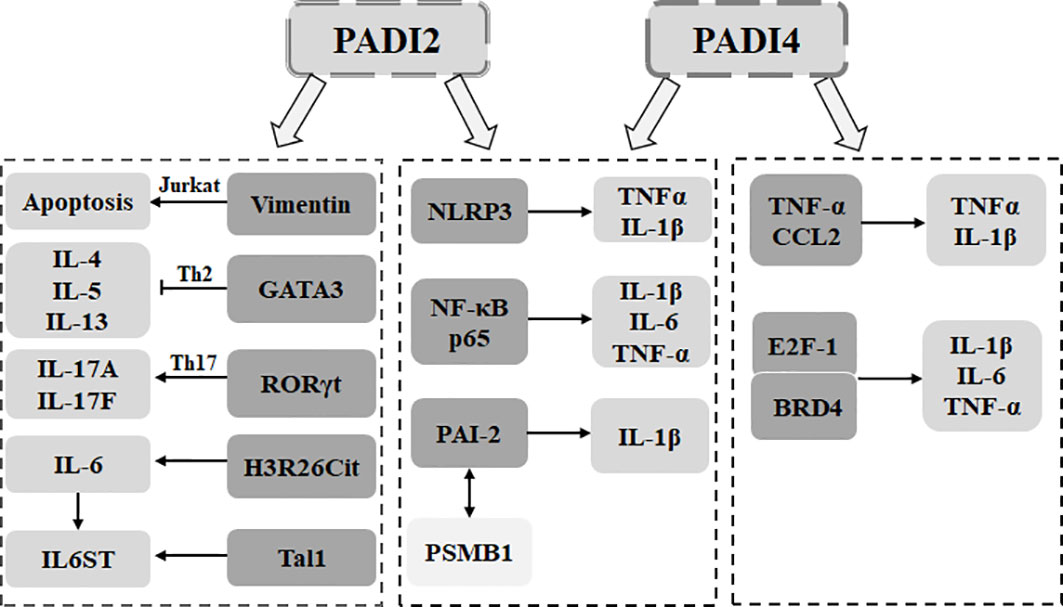
Figure 2 The PADI family is involved in the secretion of inflammatory cytokines. In immune cells, PADI2 can mediate Jurkat apoptosis through the citrullination of vimentin. PADI2 can be used to citrullinate GATA3. RORγt determines the differentiation direction of Th cells and affects the secretion of inflammatory cytokines. PADI4 can directly affect the secretion of inflammatory cytokines in macrophages. PADI2 can cooperate with PADI4 to affect the assembly of macrophage inflammatory bodies, mediate the dissociation of PAI-2 and PSMB1, and promote the secretion of inflammatory cytokines. In nonimmune cells, PADI2 can promote the secretion of inflammatory cytokines by targeting histones and can also act as an epigenetic coactivator to promote the signal transduction of inflammatory cytokines. PADI4 can promote the binding of BRD4 and E2F-1 and promote the secretion of inflammatory cytokines. PADI4 can also target NF-κB p65 to promote the secretion of inflammatory cytokines.
Energy metabolism and extracellular vesicle release
Studies have shown that the PADI family can participate in energy metabolism. PADI1 and PADI3 can promote glycolysis through citrulline pyruvate kinase M2 (PKM2) arginine 106, leading to the proliferation of cancer cells (77, 78). In addition, TINCR promotes de novo lipid biosynthesis and histone H3K27 acetylation by preventing the ubiquitination and degradation of ACLY, leading to the accumulation of acetyl-CoA in cells. Additionally, it mediates the drug resistance of tumors through the PADI-MAPK-MMP2/9 pathway (79). Therefore, the PADI family can participate in intracellular energy metabolism.
Extracellular vesicles (EVs) are a heterogeneous group of vesicles; they contain various proteins, lipids and nucleic acids. Studies have shown that PADI2, PADI3 and PADI4 in the PADI family promote the carcinogenic microenvironment by mediating the formation of EVs, leading to tumor invasion (80, 81). Therefore, the PADI family can participate in the formation of extracellular vesicle release.
Recent research has shown that the PADI family can participate in the occurrence of diseases by participating in various physiological processes. Next, we will discuss the molecular mechanisms by which the PADI family acts in various diseases.
The PADI family and inflammatory autoimmune diseases
Studies have shown that the PADI family can mediate the formation of autoantibodies through epigenetic modifications, protein posttranslational modifications, NETosis and cytokine production, thus participating in the occurrence of inflammatory autoimmune diseases such as arthritis, neurodegenerative diseases, atherosclerosis and thrombosis, systemic lupus erythematosus, and infection (Figure 3).
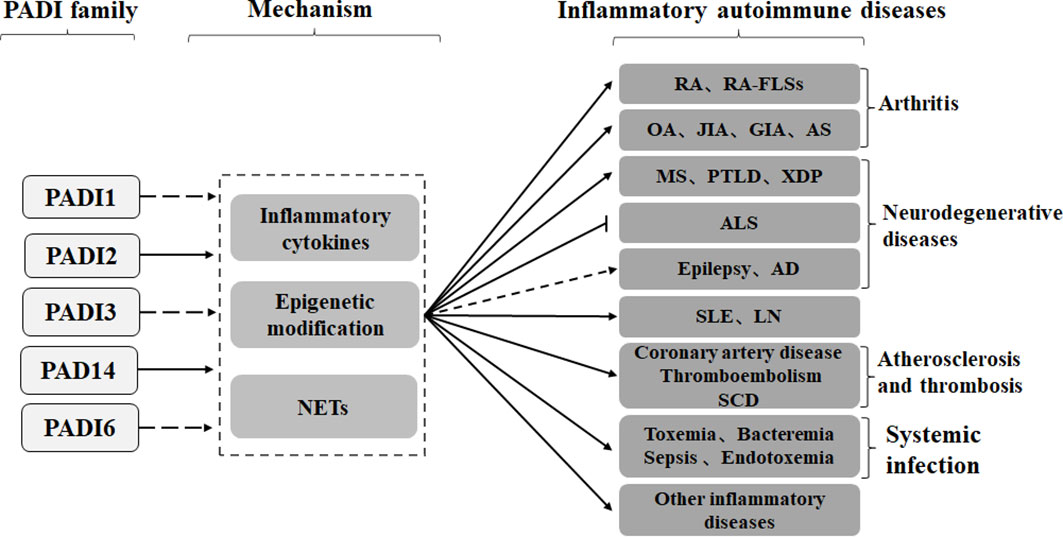
Figure 3 PADI2 and PADI4 in the PADI family can promote inflammatory autoimmune diseases, such as arthritis, neurodegeneration, arteriosclerosis and thrombosis, systemic lupus erythematosus and infection, through cytokines, epigenetics and NETs. No studies have shown that other members of the PADI family, other than PADI2 and PADI4, are involved in the development of inflammatory autoimmune diseases. Recent studies have shown that the PADI family can inhibit the development of ALS, but the role of the PADI family in epilepsy and AD is still controversial.
Arthritis
Arthritis is a general term that can be applied to numerous conditions. It is an inflammatory disease that occurs in the joints and surrounding tissues of the human body. Studies have shown that the PADI family is an important participant in the occurrence of arthritis (82).
The PADI family promotes the occurrence and exacerbation of rheumatoid arthritis (RA) by acting on fibroblast-like synovial cells (FLSs). Moreover, PADI4-mediated NETosis results in the release of high levels of PADI into the spinal fluid (SF) of RA patients (83). However, hypoxic conditions in RA can further upregulate the levels of PADI2 and PADI4, leading to an increase in citrullinated fibrinogen in FLSs (84, 85). Since PADI4 can provide more citrullinated epitopes than PADI2, high titers of anti-citrullinated protein antibodies (ACPAs) preferentially bind to citrullinated fibrinogen catalyzed by PADI4, which also leads to the preferential binding of ACPAs to histone H3 mediated by PADI4 (86, 87).
The binding of PADI4 to histone H3 in the p21 promoter region leads to the inhibition of p21 transcription and apoptosis in RA-FLSs. This promotes hypoxia-induced autophagy and proliferation and exacerbates the malignant progression of RA (85, 88). PADI4 can also increase H3 citrullination in CD14hi monocytes through TLR and induce the expression of TNFα, MIP1β, IFNα, and IL-12 and the formation of monocyte extracellular traps (METs). Therefore, inflammation and the production of ACPAs are promoted and RA is exacerbated (89). The citrullination of fibrin mediated by PADI2 can induce the expression of the proinflammatory cytokines IL-6 and IL-8 through the TLR4 pathway. This leads to an inflammatory response in RA synovial fibroblasts (RASFs) and promotes the occurrence of RA (90). Furthermore, the dysregulation of PTPN22 can also lead to the aggravation of RA, because PTPN22 can inhibit the high citrullination mediated by PADI2 and PADI4 (91, 92).
In addition, the PADI family can exacerbate RA through other mechanisms. In synoviocytes, the interaction of PADI4 with SYVN1 may induce cell proliferation by inhibiting the p53 pathway and apoptosis and may also trigger RA by mimicking the state of endoplasmic reticulum stress inhibition (93). Citrullination of fibronectin (FN) by PADI4 leads to the upregulation of the proteolytic activity of ADAMTS4, which promotes the erosion of ADAMTS4 in joints. This causes the destruction of cartilage and the aggravation of RA (94). Furthermore, individual genetic polymorphisms of PADI2 and PADI4 can increase susceptibility to RA (35–37, 41, 43, 46, 47, 95–97). In ACPA RA, PADI4 can help HLA-DRB1 bind to ACPA (98, 99). Studies have shown that the upregulation of PADI2 and citrullination of the protein are associated with RA-related interstitial lung disease (RA-ILD), but the specific mechanism still needs further study (100, 101).
The PADI family also plays an important role in other types of arthritis. PADI4 polymorphisms confer susceptibility to OA and juvenile idiopathic arthritis (JIA) (40, 102, 103). Additionally, PADI4 can promote the progression of glucose 6-phosphate isomerase-induced arthritis (GIA) by mediating NETosis, increasing the number of CD4+ T and Th17 cells, and upregulating the level of IL-6 (104). In ankylosing spondylitis (AS), the upregulation of PADI4 mediates TNF-α-induced proliferation and the osteogenic differentiation of human mesenchymal stem cells (hMSCs), aggravating the progression of AS (105).
In summary, the current study shows that PADI2 and PADI4 of the PADI family play a role in promoting arthritis, but whether other members of the PADI family are associated with arthritis remains to be elucidated.
Neurodegenerative diseases
Neurodegenerative disease is a general term for a class of diseases in which a large loss of neurons and/or their myelin sheaths leads to neural dysfunction, including epilepsy, Alzheimer’s disease (AD), Parkinson’s disease (PD), and amyotrophic lateral sclerosis (ALS). Studies have shown that the PADI family can influence neurodegenerative disease progression through epigenetic modifications and autoantibodies.
On the one hand, the PADI family promotes the occurrence of neurodegenerative diseases. In the hippocampus of AD patients, upregulation of PADI2 leads to the abnormal accumulation of citrullinated GFAP, promoting AD progression (106). Moreover, high PADI2 expression in the CNS leads to the exacerbation of MS and posttreatment Lyme disease (PTLD) (28). In prion-infected astrocytes, the upregulation of PADI2 activates the overexpression of citrullinated proteins. This leads to a functional change in enolase and promotes the malignant progression of prions (107). Moreover, X-linked dystonia Parkinson’s disease (XDP) is aggravated by increased levels of PADI2, PADI4, CitH3 and inflammation in the prefrontal cortex (PFC) and its derived fibroblasts (108).
On the other hand, the PADI family inhibits the development of neurodegenerative diseases. First, PADI family members can prevent disease by maintaining nerve cell homeostasis. The citrullination of proteins by PADI2 promotes chromatin decondensation. This leads to the upregulation of oligodendrocyte differentiation genes, ensures normal oligodendrocyte differentiation, myelination, and motor function, and prevents motor dysfunction (109). Moreover, in human neural stem cells (hNSCs), PADI3 binds to apoptosis-inducing factor (AIF) and translocates it to the nucleus to induce apoptosis (110). Second, the aberrant expression of the PADI family under pathological conditions prevents disease progression. In AD, PADI4 mediates autophagy and inhibits phosphorylation of the Akt/mTOR pathway, thereby increasing cell viability, inhibiting apoptosis and senescence, and delaying AD progression (111). In ALS, PADI4 citrullinates the RGG motif of the FET protein, inhibiting the aggregation of the FET protein and reducing susceptibility to ALS (112).
In addition, some studies have noted that the PADI family can participate in the occurrence of epilepsy through epigenetics and autoantibodies, but its specific mechanism of action requires further study (113).
Overall, the PADI family can both promote and inhibit the occurrence of neurodegenerative diseases. However, there are few studies on the PADI family and neurodegeneration, and further research is needed to elucidate the mechanisms of the PADI family in specific neurodegenerative diseases.
Atherosclerosis and thrombosis
Atherosclerosis is a condition in which plaque-like deposits of lipids (atheromas or atherosclerotic plaques) form in the arterial walls of medium or large arteries, reducing or blocking blood flow. Studies have shown that the accumulation of PADI4-mediated NETosis at the site of intimal injury destroys the integrity of the vascular intima and promotes the occurrence of atherosclerosis, which is associated with coronary artery disease (CAD) (114, 115).
Intimal damage from atherosclerosis leads to thrombus formation, which is promoted by NETosis that is mediated by the upregulation of PADI4. In carotid arteries, PADI4 can promote plaque instability through NETs (116). During vaso-occlusive crisis (VOC), the upregulation of PADI4 promotes immune thrombosis through NETosis, leading to venous thromboembolism and sickle cell disease (SCD) (117). In heparin-induced thrombocytopenia (HIT) mice, PADI4 enhances neutrophil-endothelial cell adhesion and neutrophil clot infiltration through NETosis, thereby promoting the formation and progression of venous thrombosis (118). In placentation, PADI4 can promote an inflammatory response and thrombosis by mediating NETosis, increasing the susceptibility to miscarriage (119). PADI4 can also exacerbate anti-neutrophil cytoplasmic antibody-associated vasculitis (ANCA-AAV) (120).
Taken together, these results indicate that the PADI family can promote atherosclerosis and thrombosis, leading to the occurrence of related diseases.
Systemic lupus erythematosus
Systemic lupus erythematosus (SLE) is an autoimmune disease characterized by many autoantibodies in serum and involves multiple organs. Studies have shown that the PADI family may be involved in SLE through different mechanisms.
In TLR7-induced lupus, PADI2 and PADI4 promote the induction of TLR7 in lupus through innate and adaptive immunity (121). Moreover, the upregulation of PADI4 caused by the stimulation of TLR7 promoted the phosphorylation of p38 MAPK and upregulated the expression of the P38 MAPK scaffold protein JLP. Thus, the renal invasion of neutrophils was promoted, and lupus was exacerbated (122). Mutations in the deubiquitinase domain of TNFAIP3 (A20) led to the upregulation of PADI4. This promoted protein citrullination and NETosis formation, leading to increased susceptibility to SLE (123). PADI4 polymorphisms also confer susceptibility to SLE and lupus nephritis (LN) (49).
Systemic infection
Systemic infection is caused by a weakened immune function of the body, which leads to the infection of pathogenic bacteria and their toxic metabolites through lymphatic vessels or directly into the bloodstream. Systemic infection can be divided into toxemia, bacteraemia, sepsis, and endotoxemia. Studies have shown that the expression of the PADI family, the concentration of H3, and NETosis are positively correlated with the severity of sepsis, septic shock, and toxemia. This observation is a result of the upregulation of PADI2 and PADI4 when the body is infected. Additionally, CitH3 can promote NETosis and increase the inflammatory response, thereby promoting the aggravation of various infections (13, 14, 16, 124–127). Moreover, in sepsis, PADI2 can promote Caspase-11-dependent pyroptosis and reduce the antibacterial activity of macrophages, thereby exacerbating sepsis (15). In conclusion, the current study shows that the PADI family acts as a facilitator of systemic infection.
Other inflammatory autoimmune diseases
First, the PADI family can promote the occurrence of other inflammatory autoimmune diseases by mediating NETosis. After renal ischemia-reperfusion (IR) injury, PADI4 is upregulated in proximal renal tubules. Moreover, PADI4 can promote tubular NF-κB activity and inflammation by citrullinating nuclear factor kappa B essential modulator (NEMO); it can also aggravate tubular inflammation and injury after IR by increasing neutrophil infiltration, neutrophil trap formation, apoptosis and the secretion of inflammatory factors (128–131). In hidradenitis suppurativa (HS), increased NETs mediated by the PADI family promote immune dysregulation and lead to inflammation (132). Respiratory syncytial virus (RSV) induces NETosis through histone citrullination by PADI1-4, leading to the development of RSV bronchiolitis inflammation in infants and young children (133). When chronic eyelid inflammation (blepharitis) occurs in the eye, PADI4-mediated aggregated NETs block the meibomian gland (MG), leading to meibomian gland dysfunction (MGD) (134). In diabetes, the upregulation of PADI4 in neutrophils increases CitH3 levels, promotes NETosis, and inhibits wound healing (135). PADI4 also promotes gallstone formation through NETosis (136).
Second, the PADI family can also promote the occurrence of inflammatory autoimmune diseases through other mechanisms. In periodontitis, increased expression of PADI2, PADI4, and citrullinated proteins accompanies the exacerbation of periodontitis (137–139). In hepatic stellate cells (HSCs), the PADI2-mediated abnormal accumulation of citrullinated GFAP promotes liver fibrosis (140). During human rhinovirus (HRV) infection, the upregulation of PADI2 in human bronchial epithelial cells leads to the citrullination of human cathelicidin LL-37. This reduces its antiviral activity against HRV and allows HRV to escape the immune response (141). Moreover, citrullinated fibrinogen (cFBG) has been detected in patients with inflammatory diseases. cFBG produced by PADI2 not only inhibits fibrin polymerization but also damages fibrin fibre properties, leading to adverse effects on hemostasis (142).
The PADI family and cancer
Studies have shown that in different cancer types, the PADI family can participate in tumorigenesis as an epigenetic modifier. It can also play a role in tumor cell proliferation, migration, invasion, angiogenesis, and drug resistance by regulating different signaling pathways, and as a result, it can act as an oncogene or tumor suppressor gene in cancer (Figure 4). At present, PADI2 and PADI4 are the most frequently studied members of the PADI family in tumors. In this article, we elaborate on the molecular mechanisms by which each PADI family member participates in tumors.
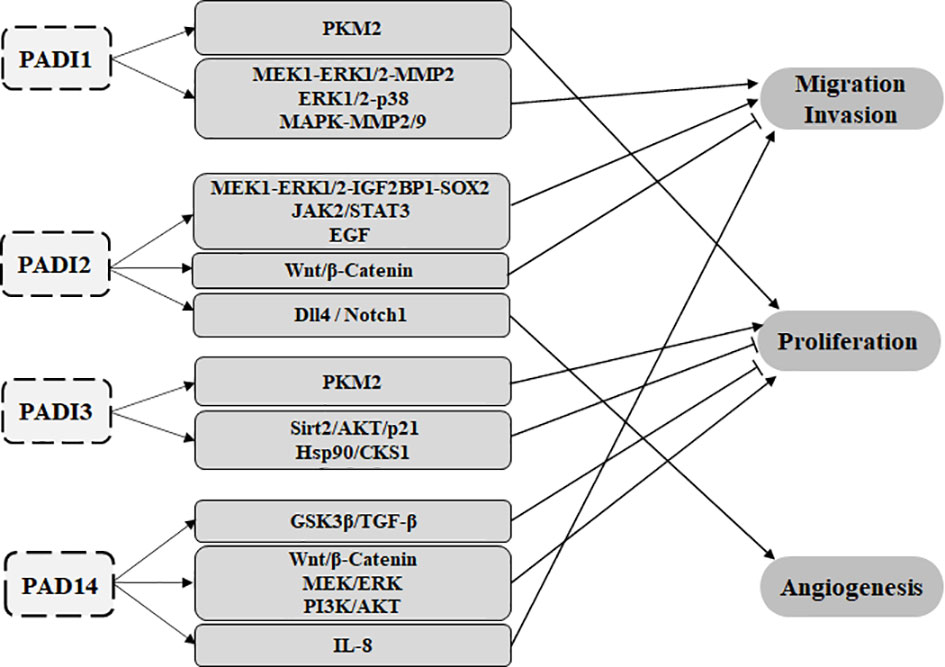
Figure 4 The PADI family can participate in tumour proliferation, migration, invasion and angiogenesis through various signalling pathways. PADI1 and PADI3 can promote glycolysis through the citrullination of PKM2, leading to the proliferation of tumour cells. PADI1 can promote tumour migration and invasion through MEK1-ERK1/2-MMP2, ERK1/2-p38 and MAPK-MMP2/9. PADI2 can promote tumour migration through the MEK1-ERK1/2-IGF2BP1-SOX2, JAK2/STAT3 and EGF signalling pathways. PADI2 also affects Wnt/β-catenin to inhibit tumour metastasis. PADI2 can promote tumour angiogenesis through the Dll4/Notch1 signalling pathway. PADI3 can inhibit tumour proliferation through Sirt2/AKT/p21 and Hsp90/CKS1. PADI4 can act on IL-8 to promote tumour cell metastasis. PADI2 can also affect Wnt/β-Catenin, MEK/ERK and PI3K/AKT to promote the proliferation of tumour cells. PADI4 can also inhibit the proliferation of tumour cells through GSK3β/TGF-β. At present, there is no report on PADI6 and tumours.
PADI1
First, PADI1 can affect the proliferation of tumor cells by participating in energy metabolism. The citrullination of Arg 10 in PKM2 by PADI1 can lead to an increase in glycolysis, thus promoting the proliferation of cancer cells (77, 78). Second, PADI1 can promote EMT and metastasis of triple-negative breast cancer cells by regulating MEK1-ERK1/2-MMP2 signal transduction (143). In pancreatic ductal adenocarcinoma (PAAD), PADI1 can activate ERK1/2-p38 signal transduction, thereby promoting cell migration and invasion (140). PADI1 can also mediate the proliferation, metastasis and cisplatin resistance of nasopharyngeal carcinoma (NPC) through the TINCR-ALY-PADI1-MAPK-MMP2/9 axis (144). However, although some studies have shown that PADI1 is a poor prognostic factor of artificial cancer in pancreatic cancer and clear renal cell carcinoma and that PADI1 can also participate in the progression of laryngeal squamous cell carcinoma (LSCC), its specific mechanism of action still needs further study (145–147). Therefore, PADI1 mainly acts as an oncogene.
PADI2
First, PADI2 can affect the proliferation of tumor cells by regulating gene expression. When normal breast cells are transformed into malignant tumors, the upregulated expression of PADI2 affects the expression of cell cycle genes, such as p21, GADD45α and Ki67, which are related to tumor progression (148). This may be because PADI2 can affect gene expression through epigenetic modifications in breast cancer. For example, in estrogen receptor ER+ breast cancer, PADI2 can not only promote susceptibility but can also citrullinate histone H3R26, change the structure of nucleosomes, promote the binding of ER and DNA, lead to the activation of ER target genes, and increase the survival rate of ER+ breast cancer patients (57). PADI2 can also citrullinate R1810 (cit1810) at RNAP2-CTD (RNA polymerase II), promote the interaction with the P-TEFb (positive transcription elongation factor b) complex, lead to the release of RNAP2, and promote the transcription of cell cycle genes and the proliferation of breast cancer cells (62). In prolactinomas and growing prolactinomas, PADI2 and PADI4 can promote the upregulation of the HMGA1, N-MYC and IGF-1 oncogenes by catalyzing the citrullination of histones, thus promoting the proliferation of cancer cells (149). In hepatocellular carcinoma, the downregulation of PADI2 inhibits EPO expression and promotes the proliferation and migration of hepatocellular carcinoma cells (150). PADI2 can also inhibit the proliferation of colon cancer cells by inducing G1 phase arrest in colon cancer cells through its citrullination effect (151).
Second, PADI2 can mediate the migration and invasion of tumor cells. PADI2 can participate in tumor cell migration and invasion by regulating gene expression. The overexpression of PADI2 can upregulate the expression of ACSL4 and BIRC3, downregulate the expression of CA9, promote abnormal lipid metabolism and tumor cell invasion, and lead to the abnormal migration of breast tumor cells (152). The downregulation of PADI2 can lead to the low expression of CXCR2, thus inhibiting the proliferation and migration of gastric cancer cells and promoting apoptosis (150). In bone marrow mesenchymal stem cells (BMMSCs) from multiple myeloma (MM), the overexpression of PADI2 promotes the expression of interleukin-6 (IL-6) through the citrullination of histone H3R26. It also mediates the drug resistance of MM and leads to the malignant progression of MM (73, 153). In skin cancer, the overexpression of PADI2 can lead to the malignant progression of tumors by promoting the inflammatory microenvironment (154, 155).
PADI2 can also participate in the migration and invasion of tumor cells by mediating the transmission of some signaling pathways. In endometrial carcinoma, PADI2 can citrullinate MEK1 arginine 113/189, promote the phosphorylation of extracellular signal regulated protein kinase 1/2 (ERK1/2), activate insulin-like growth factor II binding protein 1 (IGF2BP1), and prevent the degradation of SOX2 mRNA. This causes the abnormal accumulation of SOX2 and leads to the malignant progression of EC (156). In ovarian cancer, PADI2 can lead to metastasis and invasion by promoting the JAK2/STAT3 signaling pathway (157). In colorectal cancer (CRC), PADI2 can citrullinate β-catenin, leading to its degradation. Thus, Wnt signaling is inactivated, and the progression of CRC is inhibited (158). In breast cancer, PADI2 can mediate cell migration by promoting the EGF signaling pathway (159). However, studies have shown that the overexpression of PADI2 can promote liver metastasis of CRC (160).
PADI2 is also involved in tumor cell migration and invasion by mediating EVs. High expression of PADI2 and PADI3 can reduce the expression of moesin, increase extracellular vesicles (EVs) to release tumor-promoting factors, reduce EV tumor suppressors, and increase the invasiveness of tumor cells, thereby promoting the malignant progression of PDAC (80). The specific mechanisms of action of PADI2 in the metastasis and invasion of bladder cancer still needs further study (161).
In addition, PADI2 can mediate tumor angiogenesis. In malignant glioma, hypoxia can induce the upregulation of the PADI family (PADI1, 2, 3 and 4), and PADI2 can citrullinate vascular endothelial growth factor receptor 2 (162). Studies have shown that PADI2 is an angiogenesis-regulating gene that can promote angiogenesis through Dll4/Notch1 signaling (163, 164).
It has been reported that the overexpression of PADI2 can increase tamoxifen resistance in breast cancer cells (165).
As mentioned above, the role of PADI2 in tumors is still controversial. However, because PADI2 can participate in tumor progression by mediating tumor proliferation, migration and invasion, tumor angiogenesis and drug resistance, it has become an important target in tumor treatment.
PADI3
As previously mentioned, PADI3 can promote glycolysis through the citrullination of PKM2, leading to the proliferation of cancer cells. PADI3 can inhibit the development of colon cancer by inhibiting the expression of Sirt2 and upregulating p21. These effects lead to a reduction in AKT phosphorylation and the downregulation of Snail, thereby inducing cell cycle arrest and inhibiting cell proliferation (166). PADI3 can also exert its antitumor activity by inhibiting the expression of Hsp90 and CKS1 (167). PADI3 can also increase the invasiveness of tumor cells by mediating EVs, thereby promoting the malignant progression of PDAC (80). Therefore, PADI3 could play a role as both an oncogene and tumor suppressor gene in tumors.
PADI4
First, PADI4 can mediate the occurrence of cancer. PADI4 can downregulate the expression of NANOG and OCT4, the two main transcription factors of stem cells, by reducing H3R17me2a, leading to a reduction in breast cancer stem cell activity (168). PADI4-mediated NETs can help pancreatic cancer cells cross cell cycle checkpoints and promote the occurrence of PDAC (169).
Second, PADI4 can mediate the proliferation and metastasis of cancer cells. PADI4 can participate in the proliferation and metastasis of cancer cells by regulating gene expression. In oral squamous cell carcinoma (OSCC), PADI4 can upregulate its target HIST1H1B through epigenetic modifications, promoting the loss of cell differentiation and leading to the progression of OSCC (170). In esophageal squamous cell carcinoma (ESCC), PADI4 can stimulate the growth of ESCC cells and upregulate CA9 to promote ESCC metastasis (171). PADI4 synergizes with B-cell-specific Moloney leukemia virus insertion site 1 (Bmi-1) to promote the carcinogenesis and progression of ESCC (172). In prolactinomas and growing prolactinomas, PADI2 and PADI4 can promote the proliferation of cancer cells by catalyzing the citrullination of histones and upregulating the oncogenes HMGA1, N-MYC and IGF-1 (149). In lung cancer, the upregulation of PADI4 can inhibit the expression of IRF5 and CD86 and promote the expression of CD163 and CD206. This leads to the activation of macrophages and their pro-tumor effect, thereby promoting epithelial-mesenchymal transition (EMT) in lung cancer and inhibiting cell apoptosis (173, 174).
PADI4 can mediate the proliferation and metastasis of cancer cells by promoting NETs. In breast cancer, PADI4 can also mediate the formation of cancer extracellular chromatin networks (CECNs) and promote lung metastasis of breast cancer (175). In PDAC, PADI4 activates pancreatic stellate cells through DNA released by neutrophils in NETs, promoting PDAC proliferation and metastasis (176).
PADI4 can participate in the proliferation and metastasis of cancer cells through certain signaling pathways. The overexpression of PADI4 increases the level of nuclear GSK3β protein, thereby inhibiting the epithelial-mesenchymal transition induced by TGF-β signaling (177). In gastric cancer, PADI4 can accelerate GC metastasis by promoting IL-8 and can also promote proliferation (178, 179). In osteosarcoma cells, PADI4 can also stimulate Wnt/β-Catenin and MEK/ERK signaling and promote proliferation (180). In nasopharyngeal carcinoma, PADI4 can activate the PI3K/AKT pathway to promote proliferation (181).
In addition, PADI4 can mediate tumor resistance. In non-small cell lung cancer (NSCLC), the overexpression of PADI4 can downregulate the expression of ETS domain protein (Elk1) and inhibit EMT, thereby reducing the drug resistance of NSCLC. In HCC cells, the overexpression of PADI4 can mediate protective autophagy, leading to resistance to chemotherapeutic drugs (182). In NPC cells, PADI4 overexpression can inhibit DNA damage. Additionally, PADI4 can downregulate the expression of p21 and activate the mTOR signaling pathway to induce radiation resistance (183–185). In breast cancer cells, PADI4 can also reverse multidrug resistance (MDR) by activating GSK3β/p53. In CRC cells, PADI can promote the migration and growth of GSK3β by promoting the nuclear transport of nuclear cyclin-dependent kinase inhibitor 1 (CDKN1A) ubiquitin-dependent proteasome degradation (186).
PADI4 can also mediate tumor-associated angiogenesis. In breast cancer and liver cancer, PADI4 can promote angiogenesis and tumor growth (187). In gastric cancer, PADI4 promotes gastric tumorigenesis and angiogenesis by upregulating CXCR2, KRT14, and TNF-α expression (53).
Therefore, PADI4 acts as an oncogene or tumor suppressor gene in tumors mainly by mediating tumorigenesis, proliferation, migration, angiogenesis and drug resistance.
In summary, the members of the PADI family (at present, there is no report on the relationship between PADI6 and tumors) could participate in tumor progression through various mechanisms. Thus, it plays an important role in tumor pathology and provides a target for tumor treatment.
The PADI family and reproductive development-related diseases
Studies have shown that the expression of the PADI family in reproductive organs and germ cells is crucial for reproductive developments. In Sertoli cells, the specific expression of PADI2 is involved in testis development by mediating the regulation of target genes by SOX9 regulation (12). PADI6 is associated with the ovarian reserve (OR) in the primordial follicle pool (31). During early embryonic development, PADI1 transactivates the early embryonic genome by catalyzing histone tail citrullination. PADI6 ensures an adequate ribosome supply by promoting oocyte cytoplasmic lattice (CPL) formation and promoting the progression of early embryonic development (7, 188).
However, the abnormal expression of PADI family members can trigger reproductive development-related diseases. First, polymorphisms in PADI6 are associated with sexual developmental disorders (189). Second, PADI6 is one of the genes encoding the subcortical maternal complex (SCMC), which is necessary for oocyte maturation and early embryonic development. Therefore, the loss and mutation of PADI6 destabilizes SCMC, resulting in abnormal oocyte maturation, fertilization failure, early embryonic developmental arrest, multilocus imprinting disorder, molar pregnancy, miscarriage, and female infertility (32, 190–207).
In conclusion, the PADI family is an important regulator that ensures the normal progression of reproductive development.
The PADI family and other disorders
First, the PADI family can participate in the differentiation of the epidermis and hair follicles. In the process of epidermal differentiation, PADI1 and/or PADI3 can promote keratinization of the epidermis through autophagy and promote the preservation of keratinocytes through citrullinated keratin to enhance the ability of the body to adapt to different environments (27, 208). In the differentiation of hair follicles, PADI1 promotes the ability of β-catenin to stimulate hair follicle differentiation, and PADI2 is involved in the development of hair follicles (209, 210). Moreover, PADI3 is involved in hair shaft formation, and its loss causes morphological changes in hair, leading to the development of uncombable hair syndrome (UHS) and central centrifugal cicatricial alopecia (CCCA) (211).
In addition to the above, the PADI family may also be involved in the occurrence of other types of diseases. In the heart, the citrullination of myofilament proteins by the PADI family results in cardiac contractility impairment and reduced cellular sensitivity to Ca2+, leading to heart failure (HF) (212). In the retina, PADI2 and PADI4 are associated with age-related macular degeneration (AMD) in the human retina through their citrullination of proteins (213, 214). In addition, PADI2 can promote the formation of elastic fibers by citrullinating Fibulin-5 (FBLN5). PADI2 can also inhibit the senescence-related secretory phenotype (SASP) by inhibiting the NFκB signaling pathway, thereby delaying the senescence of osteoblasts, increasing resistance to pneumonia and improving environmental adaptability (215–217). Moreover, PADI4 can reduce susceptibility to tuberculosis (218).
Application of the PADI family in disease treatment
As mentioned above, the PADI family is mainly involved in inflammatory autoimmune diseases, cancer and reproductive development-related diseases and plays an important role in the occurrence of these diseases. Therefore, in recent years, an increasing number of researchers have explored the possibility of the PADI family as a target in the develop of drugs, as shown in Table 3.
Discussion
Studies have shown that the calcium ion dependent enzyme PADI family targets protein substrates through its citrullination. First, there is crosstalk between PADI family members and methylation by targeting histones, and thus they play a role as epigenetic modifying enzymes. Secondly, the PADI family can regulate gene expression and signal transduction by targeting non-histone proteins. Therefore, the PADI family can participate in various physiological processes through the citrullination of proteins, which is associated with the occurrence of diseases (Figure 5).
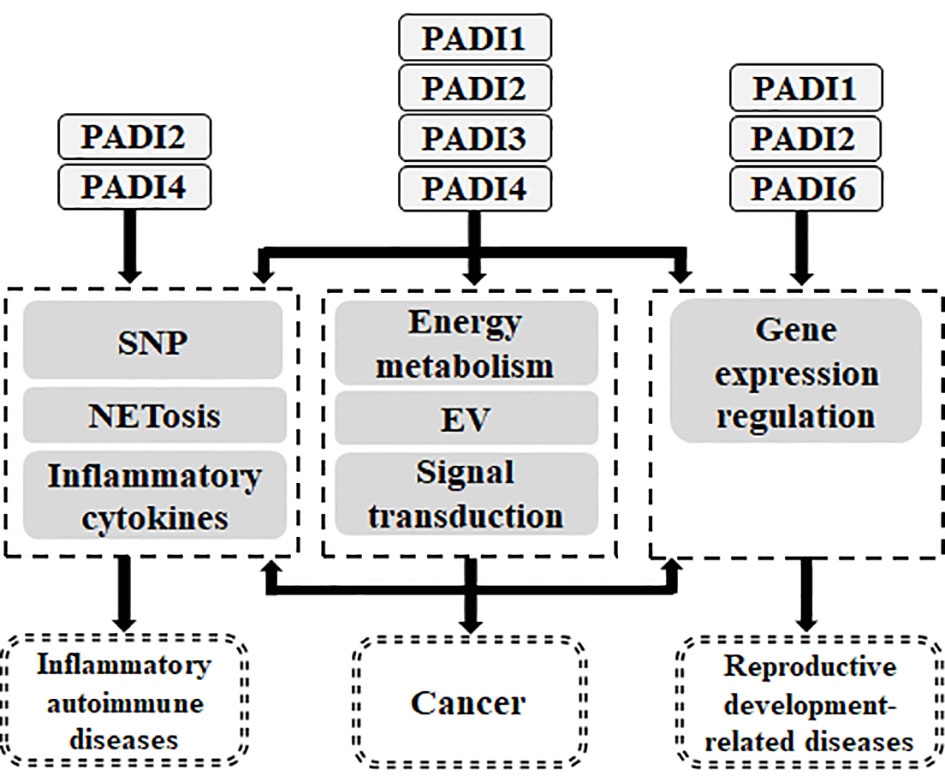
Figure 5 Relationship between PADI family and human diseases. The genetic variation of PADI family will endow with the susceptibility of inflammatory autoimmune diseases and cancer. PADI family can participate in inflammatory autoimmune diseases through NETosis and inflammatory cytokines. PADI family can participate in cancer through NETosis, inflammatory cytokines, energy metabolism, EV, signal transduction and gene expression regulation. PADI family can participate in reproductive and development-related diseases through gene expression regulation.
In arthritis, the genetic variation of PADI2 and PADI4 can confer RA susceptibility, and they can aggravate arthritis by promoting the secretion of NETosis and inflammatory cytokines. In neurodegenerative diseases, the high expression of PADI2 leads to the abnormal accumulation of citrullinated proteins and promotes their malignant progression, while PADI4 can lead to inflammation through citrullinated histone H3, thus promoting the progression of neurodegenerative diseases. However, PADI2, PADI3 and PADI4 can also inhibit the progression of neurodegenerative diseases by participating in the differentiation, apoptosis and senescence of nerve cells. In atherosclerosis and thrombosis, PADI4 can lead to an inflammatory response and thrombosis by promoting NETosis. In SLE, PADI2 and PADI4 can promote its progression through the immune response. In addition, the genetic variation of PADI4 can confer SLE susceptibility and promote its deterioration by promoting NETosis. In systemic infection, PADI2 and PADI4 can lead to the deterioration of infection by promoting NETosis, and PADI2 can also lead to the deterioration of infection by inducing cell apoptosis. Therefore, in inflammatory autoimmune diseases, the PADI family mainly participates in disease progression through PADI2 and PADI4.
In cancer, PADI1 can promote tumor proliferation, migration, invasion and drug resistance by promoting energy metabolism and signal transduction (MEK1-ERK1/2-MMP2, ERK1/2-p38 and PADI1-MAPK-MMP2/9), thus it acts as oncogene in cancer. PADI2 can regulate gene expression, energy metabolism, inflammatory response and signal transduction (MEK1/ERK1/2/IGF2BP1/SOX2, JAK2/STAT3, Wnt/β-Catenin, EGF, Dll4/Notch1) to mediate the proliferation, migration, invasion and drug resistance of tumor cells, thus acting as an oncogene and tumor suppressor gene. PADI3 can affect the proliferation and invasion of tumor cells by regulating energy metabolism, EV and signal transduction (Sirt2/p21/AKT/Snail, Hsp90/CKS1), thereby inhibiting or promoting cancer. PADI4 can affect tumorigenesis by regulating cell activity, cell cycle progression and differentiation. PADI4 can also regulate gene expression, NETs and signal transduction (GSK3β/TG-β、Wnt/β-Catenin、MEK/ERK、PI3K/AKT、GSK3β/P53) to affect the proliferation, migration, invasion, drug resistance and angiogenesis of tumor cells. Therefore, PADI4 can promote and inhibit cancer. As mentioned earlier, the role of the PADI family (except PADI6) in cancer is still under debate. PADI family members can function as oncogenes and as tumor suppressors.
Among the diseases related to reproductive development, PADI1, PADI2 and PADI6 are necessary to ensure normal reproductive development. However, current research shows that the abnormal expression of PADI6 can lead to abnormal oocyte maturation, fertilization failure, early embryo development stagnation, multiple site imprinting disorder, hydatidiform mole, abortion and female infertility, and the genetic variation of PADI6 is related to sexual development disorder.
In summary, PADI family members can play different roles in different disease types. On one hand, they can ensure the normal progression of various physiological activities in the body and prevent the occurrence of diseases. On the other hand, the abnormal expression of PADI family members can lead to disorders in physiological processes in the body and promote the occurrence of diseases. However, there is no doubt that due to the unique function and physiological roles of PADI family members, their potential as therapeutic targets in disease will receive increasing attention.
As mentioned above, PADI2 and PADI4 are the most studied members of the PADI family. They are mainly involved in the development of inflammatory autoimmune diseases and cancer, and they can be used as targets of disease treatment for drug development (Table 3).
At present, the mechanism by which the PADI family is involved in human physiological processes and diseases is still being investigated. Among its members, PADI2 and PADI4 are the most studied. They are mainly involved in the development of inflammatory autoimmune diseases and cancer, and they are used as targets of disease treatment for drug development (Table 3). In recent years, the important role of the PADI family in diseases has attracted people’s attention. In the future, clarifying the functions of PADI family members and the molecular mechanism of their role in various diseases will be the focus of research.
In this study, we systematically describe the function of PADI family members and their specific mechanism and research progress in various diseases, hoping to provide a reference for the study of the PADI family and promote the application of PADI family members as therapeutic targets for clinical diseases. The names of all proteins involved in this article are shown in Table 4.
Conclusion
In this review, firstly we discussed the difference between five PADI family members, which showed that they have different localization, expression patterns and functional specificity. Secondly, we discussed the genetic variation of PADI family members (PADI2 and PADI4) is related to disease susceptibility. In addition, the PADI family can participate in the regulation of gene expression, the formation of neutrophil extracellular traps, the secretion of inflammatory cytokines, energy metabolism and the release of extracellular vesicles and other important physiological processes, so that it can participate in disease progression (including inflammatory autoimmune diseases, cancer, and reproductive development-related diseases).
Author contributions
CZ, ZC and CL prepared the Manuscript, Tables and Figures, CL and ZC provided fundings. All authors read and approved the final manuscript.
Funding
This study was supported by the Natural Science Foundation of Shandong Province (ZR2021LSW018, ZR2021MH162), Jinan Science and Technology Development Program (Nos. 202019192, 201907116 and 202019180), Shandong Medical and Health Science and Technology Development Plan Project (202202080963), National Natural Science Foundation of China (Nos. 82271803, 81802422, 81904243 and 82001735), Shandong Provincial Key R&D Program (No. 2019GSF108115).
Acknowledgments
We are grateful to Na Liang, Jianguo Xu, Hongtao Lv and Bing Xu, for their help in the process of preparing the manuscript and funding support.
Conflict of interest
The authors declare that the research was conducted in the absence of any commercial or financial relationships that could be construed as a potential conflict of interest.
Publisher’s note
All claims expressed in this article are solely those of the authors and do not necessarily represent those of their affiliated organizations, or those of the publisher, the editors and the reviewers. Any product that may be evaluated in this article, or claim that may be made by its manufacturer, is not guaranteed or endorsed by the publisher.
Glossary
References
1. Ying S, Kojima T, Kawada A, Nachat R, Serre G, Simon M, et al. An intronic enhancer driven by NF-kappaB contributes to transcriptional regulation of peptidylarginine deiminase type I gene in human keratinocytes. J Invest Dermatol (2010) 130(11):2543–52. doi: 10.1038/jid.2010.179
2. Dong S, Ying S, Kojima T, Shiraiwa M, Kawada A, Mechin MC, et al. Crucial roles of MZF1 and Sp1 in the transcriptional regulation of the peptidylarginine deiminase type I gene (PADI1) in human keratinocytes. J Invest Dermatol (2008) 128(3):549–57. doi: 10.1038/sj.jid.5701048
3. Senshu T, Kan S, Ogawa H, Manabe M, Asaga H. Preferential deimination of keratin K1 and filaggrin during the terminal differentiation of human epidermis. Biochem Biophys Res Commun (1996) 225(3):712–9. doi: 10.1006/bbrc.1996.1240
4. Schwab BL, Guerini D, Didszun C, Bano D, Ferrando-May E, Fava E, et al. Cleavage of plasma membrane calcium pumps by caspases: A link between apoptosis and necrosis. Cell Death Differ (2002) 9(8):818–31. doi: 10.1038/sj.cdd.4401042
5. Vossenaar ER, Radstake TR, van der Heijden A, van Mansum MA, Dieteren C, Rooij DJ, et al. Expression and activity of citrullinating peptidylarginine deiminase enzymes in monocytes and macrophages. Ann Rheum Dis (2004) 63(4):373–81. doi: 10.1136/ard.2003.012211
6. Hewitt SC, Deroo BJ, Hansen K, Collins J, Grissom S, Afshari CA, et al. Estrogen receptor-dependent genomic responses in the uterus mirror the biphasic physiological response to estrogen. Mol Endocrinol (2003) 17(10):2070–83. doi: 10.1210/me.2003-0146
7. Zhang X, Liu X, Zhang M, Li T, Muth A, Thompson PR, et al. Peptidylarginine deiminase 1-catalyzed histone citrullination is essential for early embryo development. Sci Rep (2016) 6:38727. doi: 10.1038/srep38727
8. Jang B, Shin HY, Choi JK, Nguyen PT, Jeong BH, Ishigami A, et al. Subcellular localization of peptidylarginine deiminase 2 and citrullinated proteins in brains of scrapie-infected mice: nuclear localization of PAD2 and membrane fraction-enriched citrullinated proteins. J Neuropathol Exp Neurol (2011) 70(2):116–24. doi: 10.1097/NEN.0b013e318207559e
9. Cherrington BD, Morency E, Struble AM, Coonrod SA, Wakshlag JJ. Potential role for peptidylarginine deiminase 2 (PAD2) in citrullination of canine mammary epithelial cell histones. PloS One (2010) 5(7):e11768. doi: 10.1371/journal.pone.0011768
10. Dong S, Kojima T, Shiraiwa M, Mechin MC, Chavanas S, Serre G, et al. Regulation of the expression of peptidylarginine deiminase type II gene (PADI2) in human keratinocytes involves Sp1 and Sp3 transcription factors. J Invest Dermatol (2005) 124(5):1026–33. doi: 10.1111/j.0022-202X.2005.23690.x
11. Arandjelovic S, McKenney KR, Leming SS, Mowen KA. ATP induces protein arginine deiminase 2-dependent citrullination in mast cells through the P2X7 purinergic receptor. J Immunol (2012) 189(8):4112–22. doi: 10.4049/jimmunol.1201098
12. Tsuji-Hosokawa A, Kashimada K, Kato T, Ogawa Y, Nomura R, Takasawa K, et al. Peptidyl arginine deiminase 2 (Padi2) is expressed in sertoli cells in a specific manner and regulated by SOX9 during testicular development. Sci Rep (2018) 8(1):13263. doi: 10.1038/s41598-018-31376-8
13. Wu Z, Deng Q, Pan B, Alam HB, Tian Y, Bhatti UF, et al. Inhibition of PAD2 improves survival in a mouse model of lethal LPS-induced endotoxic shock. Inflammation (2020) 43(4):1436–45. doi: 10.1007/s10753-020-01221-0
14. Deng Q, Pan B, Alam HB, Liang Y, Wu Z, Liu B, et al. Citrullinated histone H3 as a therapeutic target for endotoxic shock in mice. Front Immunol (2019) 10:2957. doi: 10.3389/fimmu.2019.02957
15. Tian Y, Qu S, Alam HB, Williams AM, Wu Z, Deng Q, et al. Peptidylarginine deiminase 2 has potential as both a biomarker and therapeutic target of sepsis. JCI Insight (2020) 5(20):e138873. doi: 10.1172/jci.insight.138873
16. Pan B, Li Y, Liu Y, Wang W, Huang G, Ouyang Y. Circulating CitH3 is a reliable diagnostic and prognostic biomarker of septic patients in acute pancreatitis. Front Immunol (2021) 12:766391. doi: 10.3389/fimmu.2021.766391
17. Moscarello MA, Mastronardi FG, Wood DD. The role of citrullinated proteins suggests a novel mechanism in the pathogenesis of multiple sclerosis. Neurochem Res (2007) 32(2):251–6. doi: 10.1007/s11064-006-9144-5
18. Dong S, Kanno T, Yamaki A, Kojima T, Shiraiwa M, Kawada A, et al. NF-y and Sp1/Sp3 are involved in the transcriptional regulation of the peptidylarginine deiminase type III gene (PADI3) in human keratinocyte. Biochem J (2006) 397(3):449–59. doi: 10.1042/BJ20051939
19. Abbas AK, Le K, Pimmett VL, Bell DA, Cairns E, Dekoter RP. Negative regulation of the peptidylarginine deiminase type IV promoter by NF-kappaB in human myeloid cells. Gene (2014) 533(1):123–31. doi: 10.1016/j.gene.2013.09.108
20. Tanikawa C, Ueda K, Nakagawa H, Yoshida N, Nakamura Y, Matsuda K. Regulation of protein citrullination through p53/PADI4 network in DNA damage response. Cancer Res (2009) 69(22):8761–9. doi: 10.1158/0008-5472.CAN-09-2280
21. Dong S, Zhang Z, Takahara H. Estrogen-enhanced peptidylarginine deiminase type IV gene (PADI4) expression in MCF-7 cells is mediated by estrogen receptor-alpha-promoted transfactors activator protein-1, nuclear factor-y, and Sp1. Mol Endocrinol (2007) 21(7):1617–29. doi: 10.1210/me.2006-0550
22. Wang Y, Li M, Stadler S, Correll S, Li P, Wang D, et al. Histone hypercitrullination mediates chromatin decondensation and neutrophil extracellular trap formation. J Cell Biol (2009) 184(2):205–13. doi: 10.1083/jcb.200806072
23. Leshner M, Wang S, Lewis C, Zheng H, Chen XA, Santy L, et al. PAD4 mediated histone hypercitrullination induces heterochromatin decondensation and chromatin unfolding to form neutrophil extracellular trap-like structures. Front Immunol (2012) 3:307. doi: 10.3389/fimmu.2012.00307
24. Choi M, Lee OH, Jeon S, Park M, Lee DR, Ko JJ, et al. The oocyte-specific transcription factor, nobox, regulates the expression of Pad6, a peptidylarginine deiminase in the oocyte. FEBS Lett (2010) 584(16):3629–34. doi: 10.1016/j.febslet.2010.07.037
25. Xia X, Yan C, Wu W, Zhou Y, Hou L, Zuo B, et al. Characterization of the porcine peptidylarginine deiminase type VI gene (PADI6) promoter: Sp1 regulates basal transcription of the porcine PADI6. Gene (2016) 575(2 Pt 2):551–8. doi: 10.1016/j.gene.2015.09.042
26. Liu X, Morency E, Li T, Qin H, Zhang X, Zhang X, et al. Role for PADI6 in securing the mRNA-MSY2 complex to the oocyte cytoplasmic lattices. Cell Cycle (2017) 16(4):360–6. doi: 10.1080/15384101.2016.1261225
27. Merleev AA, Le ST, Alexanian C, Toussi A, Xie Y, Marusina AI, et al. Biogeographic and disease-specific alterations in epidermal lipid composition and single-cell analysis of acral keratinocytes. JCI Insight (2022) 7(16):e159762. doi: 10.1172/jci.insight.159762
28. Kim Y, Rebman AW, Johnson TP, Wang H, Yang T, Colantuoni C, et al. Peptidylarginine deiminase 2 autoantibodies are linked to less severe disease in multiple sclerosis and post-treatment Lyme disease. Front Neurol (2022) 13:874211. doi: 10.3389/fneur.2022.874211
29. Zhai M, Gong S, Luan P, Shi Y, Kou W, Zeng Y, et al. Extracellular traps from activated vascular smooth muscle cells drive the progression of atherosclerosis. Nat Commun (2022) 13(1):7500. doi: 10.1038/s41467-022-35330-1
30. Zhu D, Lu Y, Gui L, Wang W, Hu X, Chen S, et al. Self-assembling, pH-responsive nanoflowers for inhibiting PAD4 and neutrophil extracellular trap formation and improving the tumor immune microenvironment. Acta Pharm Sin B (2022) 12(5):2592–608. doi: 10.1016/j.apsb.2021.11.006
31. Liu L, Liu B, Li K, Wang C, Xie Y, Luo N, et al. Identification of biomarkers for predicting ovarian reserve of primordial follicle via transcriptomic analysis. Front Genet (2022) 13:879974. doi: 10.3389/fgene.2022.879974
32. Capalbo A, Buonaiuto S, Figliuzzi M, Damaggio G, Girardi L, Caroselli S, et al. Maternal exome analysis for the diagnosis of oocyte maturation defects and early embryonic developmental arrest. Reprod BioMed Online (2022) 45(3):508–18. doi: 10.1016/j.rbmo.2022.05.009
33. Too CL, Murad S, Dhaliwal JS, Larsson P, Jiang X, Ding B, et al. Polymorphisms in peptidylarginine deiminase associate with rheumatoid arthritis in diverse Asian populations: evidence from MyEIRA study and meta-analysis. Arthritis Res Ther (2012) 14(6):R250. doi: 10.1186/ar4093
34. Massarenti L, Enevold C, Damgaard D, Hansen PR, Frisch M, Odum N, et al. Peptidylarginine deiminase 2 gene polymorphisms in subjects with periodontitis predispose to rheumatoid arthritis. Int J Mol Sci (2022) 23(17):9536. doi: 10.3390/ijms23179536
35. Guzman-Guzman IP, Ramirez-Velez CI, Falfan-Valencia R, Navarro-Zarza JE, Gutierrez-Perez IA, Zaragoza-Garcia O, et al. PADI2 polymorphisms are significantly associated with rheumatoid arthritis, autoantibodies serologic status and joint damage in women from southern Mexico. Front Immunol (2021) 12:718246. doi: 10.3389/fimmu.2021.718246
36. Chang X, Xia Y, Pan J, Meng Q, Zhao Y, Yan X. PADI2 is significantly associated with rheumatoid arthritis. PloS One (2013) 8(12):e81259. doi: 10.1371/journal.pone.0081259
37. Massarenti L, Enevold C, Damgaard D, Odum N, Garred P, Frisch M, et al. PADI4 polymorphisms confer risk of anti-CCP-Positive rheumatoid arthritis in synergy with HLA-DRB1*04 and smoking. Front Immunol (2021) 12:707690. doi: 10.3389/fimmu.2021.707690
38. Du Y, Liu X, Guo JP, Liu X, Li R, Zhao Y, et al. Association between PADI4 gene polymorphisms and anti-cyclic citrullinated peptide antibody positive rheumatoid arthritis in a large Chinese han cohort. Clin Exp Rheumatol (2014) 32(3):377–82.
39. Bashir M, Mateen W, Khurshid S, Mehmood Malik J, Agha Z, Khan F, et al. A common missense variant rs874881 of PADI4 gene and rheumatoid arthritis: Genetic association study and in-silico analysis. Gene (2022) 854:147123. doi: 10.1016/j.gene.2022.147123
40. Mukhtar M, Sheikh N, Batool A, Khawar MB, Fatima N, Mehmood R. Novel functional polymorphism on PADI-4 gene and its association with arthritis onset. Saudi J Biol Sci (2022) 29(2):1227–33. doi: 10.1016/j.sjbs.2021.09.037
41. Mergaert AM, Bawadekar M, Nguyen TQ, Massarenti L, Holmes CL, Rebernick R, et al. Reduced anti-histone antibodies and increased risk of rheumatoid arthritis associated with a single nucleotide polymorphism in PADI4 in north americans. Int J Mol Sci (2019) 20(12):3093. doi: 10.3390/ijms20123093
42. Shaker OG, El Boghdady NA, El Sayed AE. Association of MiRNA-146a, MiRNA-499, IRAK1 and PADI4 polymorphisms with rheumatoid arthritis in Egyptian population. Cell Physiol Biochem (2018) 46(6):2239–49. doi: 10.1159/000489592
43. Banos-Hernandez CJ, Navarro-Zarza JE, Parra-Rojas I, Vazquez-Villamar M, Padilla-Gutierrez Ramon J, Valle Y, et al. PADI4 polymorphisms and the functional haplotype are associated with increased rheumatoid arthritis susceptibility: A replication study in a southern Mexican population. Hum Immunol (2017) 78(9):553–8. doi: 10.1016/j.humimm.2017.05.005
44. Goh LL, Yong MY, See WQ, Chee EYW, Lim PQ, Koh ET. NLRP1, PTPN22 and PADI4 gene polymorphisms and rheumatoid arthritis in ACPA-positive Singaporean Chinese. Rheumatol Int (2017) 37(8):1295–302. doi: 10.1007/s00296-017-3762-x
45. Lee YH, Bae SC. Association between susceptibility to rheumatoid arthritis and PADI4 polymorphisms: a meta-analysis. Clin Rheumatol (2016) 35(4):961–71. doi: 10.1007/s10067-015-3098-4
46. Hashemi M, Zakeri Z, Taheri H, Bahari G, Taheri M. Association between peptidylarginine deiminase type 4 rs1748033 polymorphism and susceptibility to rheumatoid arthritis in zahedan, southeast Iran. Iran J Allergy Asthma Immunol (2015) 14(3):255–60.
47. Yang XK, Liu J, Liu J, Liang Y, Xu WD, Leng RX, et al. Associations between PADI4 gene polymorphisms and rheumatoid arthritis: An updated meta-analysis. Arch Med Res (2015) 46(4):317–25. doi: 10.1016/j.arcmed.2015.05.011
48. Cheng J, Zhang H, Zhuang C, Liu R. Peptidylarginine deiminase type 4 and methyl-CpG binding domain 4 polymorphisms in Chinese patients with rheumatoid arthritis. J Rheumatol (2012) 39(6):1159–65. doi: 10.3899/jrheum.120007
49. Massarenti L, Enevold C, Damgaard D, Odum N, Nielsen CH, Jacobsen S. Peptidylarginine deiminase-4 gene polymorphisms are associated with systemic lupus erythematosus and lupus nephritis. Scand J Rheumatol (2019) 48(2):133–40. doi: 10.1080/03009742.2018.1488273
50. Zhou Y, Liu X. PADI4 and IL-33 gene polymorphisms associated with differential susceptibility to juvenile-onset systemic lupus erythematosus and juvenile idiopathic arthritis in Chinese children. Med (Baltimore) (2022) 101(50):e31598. doi: 10.1097/MD.0000000000031598
51. Chang X, Hou X, Pan J, Fang K, Wang L, Han J. Investigating the pathogenic role of PADI4 in oesophageal cancer. Int J Biol Sci (2011) 7(6):769–81. doi: 10.7150/ijbs.7.769
52. Sawicka B, Borysewicz-Sanczyk H, Wawrusiewicz-Kurylonek N, Aversa T, Corica D, Goscik J, et al. Analysis of polymorphisms rs7093069-IL-2RA, rs7138803-FAIM2, and rs1748033-PADI4 in the group of adolescents with autoimmune thyroid diseases. Front Endocrinol (Lausanne) (2020) 11:544658. doi: 10.3389/fendo.2020.544658
53. Zheng Y, Zhao G, Xu B, Liu C, Li C, Zhang X, et al. PADI4 has genetic susceptibility to gastric carcinoma and upregulates CXCR2, KRT14 and TNF-alpha expression levels. Oncotarget (2016) 7(38):62159–76. doi: 10.18632/oncotarget.11398
54. Wang L, Gu H, Long T, Pan H, Lv L, Shi Y, et al. PADI4 rs2240337 G>A polymorphism is associated with susceptibility of esophageal squamous cell carcinoma in a Chinese population. Oncotarget (2017) 8(55):93655–71. doi: 10.18632/oncotarget.20675
55. Wang L, Song G, Zhang X, Feng T, Pan J, Chen W, et al. PADI2-mediated citrullination promotes prostate cancer progression. Cancer Res (2017) 77(21):5755–68. doi: 10.1158/0008-5472.CAN-17-0150
56. Clancy KW, Russell AM, Subramanian V, Nguyen H, Qian Y, Campbell RM, et al. Citrullination/Methylation crosstalk on histone H3 regulates ER-target gene transcription. ACS Chem Biol (2017) 12(6):1691–702. doi: 10.1021/acschembio.7b00241
57. Guertin MJ, Zhang X, Anguish L, Kim S, Varticovski L, Lis JT, et al. Targeted H3R26 deimination specifically facilitates estrogen receptor binding by modifying nucleosome structure. PloS Genet (2014) 10(9):e1004613. doi: 10.1371/journal.pgen.1004613
58. Zhang X, Bolt M, Guertin MJ, Chen W, Zhang S, Cherrington BD, et al. Peptidylarginine deiminase 2-catalyzed histone H3 arginine 26 citrullination facilitates estrogen receptor alpha target gene activation. Proc Natl Acad Sci U.S.A. (2012) 109(33):13331–6. doi: 10.1073/pnas.1203280109
59. Song S, Xiang Z, Li J, Ji J, Yan R, Zhu Z, et al. A novel citrullinated modification of histone 3 and its regulatory mechanisms related to IPO-38 antibody-labeled protein. Front Oncol (2019) 9:304. doi: 10.3389/fonc.2019.00304
60. Li P, Yao H, Zhang Z, Li M, Luo Y, Thompson PR, et al. Regulation of p53 target gene expression by peptidylarginine deiminase 4. Mol Cell Biol (2008) 28(15):4745–58. doi: 10.1128/MCB.01747-07
61. Ballasy NN, Bering EA, Kokorudz C, Radford BN, Zhao X, Dean W, et al. Padi2/3 deficiency alters the epigenomic landscape and causes premature differentiation of mouse trophoblast stem cells. Cells (2022) 11(16):2466. doi: 10.3390/cells11162466
62. Sharma P, Lioutas A, Fernandez-Fuentes N, Quilez J, Carbonell-Caballero J, Wright RHG, et al. Arginine citrullination at the c-terminal domain controls RNA polymerase II transcription. Mol Cell (2019) 73(1):84–96 e7. doi: 10.1016/j.molcel.2018.10.016
63. Deplus R, Denis H, Putmans P, Calonne E, Fourrez M, Yamamoto K, et al. Citrullination of DNMT3A by PADI4 regulates its stability and controls DNA methylation. Nucleic Acids Res (2014) 42(13):8285–96. doi: 10.1093/nar/gku522
64. Thiam HR, Wong SL, Qiu R, Kittisopikul M, Vahabikashi A, Goldman AE, et al. NETosis proceeds by cytoskeleton and endomembrane disassembly and PAD4-mediated chromatin decondensation and nuclear envelope rupture. Proc Natl Acad Sci U.S.A. (2020) 117(13):7326–37. doi: 10.1073/pnas.1909546117
65. Gosswein S, Lindemann A, Mahajan A, Maueroder C, Martini E, Patankar J, et al. Citrullination licenses calpain to decondense nuclei in neutrophil extracellular trap formation. Front Immunol (2019) 10:2481. doi: 10.3389/fimmu.2019.02481
66. Munzer P, Negro R, Fukui S, Meglio L, Aymonnier K, Chu L, et al. NLRP3 inflammasome assembly in neutrophils is supported by PAD4 and promotes NETosis under sterile conditions. Front Immunol (2021) 12:683803. doi: 10.3389/fimmu.2021.683803
67. Hsu PC, Liao YF, Lin CL, Lin WH, Liu GY, Hung HC. Vimentin is involved in peptidylarginine deiminase 2-induced apoptosis of activated jurkat cells. Mol Cells (2014) 37(5):426–34. doi: 10.14348/molcells.2014.2359
68. Sun B, Chang HH, Salinger A, Tomita B, Bawadekar M, Holmes CL, et al. Reciprocal regulation of Th2 and Th17 cells by PAD2-mediated citrullination. JCI Insight (2019) 4(22):e129687. doi: 10.1172/jci.insight.129687
69. Yu HC, Tung CH, Huang KY, Huang HB, Lu MC. The essential role of peptidylarginine deiminases 2 for cytokines secretion, apoptosis, and cell adhesion in macrophage. Int J Mol Sci (2020) 21(16):5720. doi: 10.3390/ijms21165720
70. Cheng Y, Si Y, Wang L, Ding M, Yu S, Lu L, et al. The regulation of macrophage polarization by hypoxia-PADI4 coordination in rheumatoid arthritis. Int Immunopharmacol (2021) 99:107988. doi: 10.1016/j.intimp.2021.107988
71. Mishra N, Schwerdtner L, Sams K, Mondal S, Ahmad F, Schmidt RE, et al. Cutting edge: Protein arginine deiminase 2 and 4 regulate NLRP3 inflammasome-dependent IL-1beta maturation and ASC speck formation in macrophages. J Immunol (2019) 203(4):795–800. doi: 10.4049/jimmunol.1800720
72. Lai NS, Yu HC, Tung CH, Huang KY, Huang HB, Lu MC. Increased peptidylarginine deiminases expression during the macrophage differentiation and participated inflammatory responses. Arthritis Res Ther (2019) 21(1):108. doi: 10.1186/s13075-019-1896-9
73. McNee G, Eales KL, Wei W, Williams DS, Barkhuizen A, Bartlett DB, et al. Citrullination of histone H3 drives IL-6 production by bone marrow mesenchymal stem cells in MGUS and multiple myeloma. Leukemia (2017) 31(2):373–81. doi: 10.1038/leu.2016.187
74. Kolodziej S, Kuvardina ON, Oellerich T, Herglotz J, Backert I, Kohrs N, et al. PADI4 acts as a coactivator of Tal1 by counteracting repressive histone arginine methylation. Nat Commun (2014) 5:3995. doi: 10.1038/ncomms4995
75. Ghari F, Quirke AM, Munro S, Kawalkowska J, Picaud S, McGouran J, et al. Citrullination-acetylation interplay guides E2F-1 activity during the inflammatory response. Sci Adv (2016) 2(2):e1501257. doi: 10.1126/sciadv.1501257
76. Sun B, Dwivedi N, Bechtel TJ, Paulsen JL, Muth A, Bawadekar M, et al. Citrullination of NF-kappaB p65 promotes its nuclear localization and TLR-induced expression of IL-1beta and TNFalpha. Sci Immunol (2017) 2(12):eaal3062. doi: 10.1126/sciimmunol.aal3062
77. Coassolo S, Davidson I. Regulation of glycolysis and cancer cell proliferation by PKM2 citrullination. Mol Cell Oncol (2021) 8(4):1927446. doi: 10.1080/23723556.2021.1927446
78. Coassolo S, Davidson G, Negroni L, Gambi G, Daujat S, Romier C, et al. Citrullination of pyruvate kinase M2 by PADI1 and PADI3 regulates glycolysis and cancer cell proliferation. Nat Commun (2021) 12(1):1718. doi: 10.1038/s41467-021-21960-4
79. Zheng ZQ, Li ZX, Guan JL, Liu X, Li JY, Chen Y, et al. Long noncoding RNA TINCR-mediated regulation of acetyl-CoA metabolism promotes nasopharyngeal carcinoma progression and chemoresistance. Cancer Res (2020) 80(23):5174–88. doi: 10.1158/0008-5472.CAN-19-3626
80. Uysal-Onganer P, S, Mortoglou M, Kraev I, Lange S. Peptidylarginine deiminase inhibitor application, using cl-amidine, PAD2, PAD3 and PAD4 isozyme-specific inhibitors in pancreatic cancer cells, reveals roles for PAD2 and PAD3 in cancer invasion and modulation of extracellular vesicle signatures. Int J Mol Sci (2021) 22(3):1396. doi: 10.3390/ijms22031396
81. Uysal-Onganer P, MacLatchy A, Mahmoud R, Kraev I, Thompson PR, Inal JM, et al. Peptidylarginine deiminase isozyme-specific PAD2, PAD3 and PAD4 inhibitors differentially modulate extracellular vesicle signatures and cell invasion in two glioblastoma multiforme cell lines. Int J Mol Sci (2020) 21(4):1495. doi: 10.3390/ijms21041495
82. Fukui S, Gutch S, Fukui S, Cherpokova D, Aymonnier K, Sheehy CE, et al. The prominent role of hematopoietic peptidyl arginine deiminase 4 in arthritis: Collagen- and granulocyte colony-stimulating factor-induced arthritis model in C57BL/6 mice. Arthritis Rheumatol (2022) 74(7):1139–46. doi: 10.1002/art.42093
83. Spengler J, Lugonja B, Ytterberg AJ, Zubarev RA, Creese AJ, Pearson MJ, et al. Release of active peptidyl arginine deiminases by neutrophils can explain production of extracellular citrullinated autoantigens in rheumatoid arthritis synovial fluid. Arthritis Rheumatol (2015) 67(12):3135–45. doi: 10.1002/art.39313
84. Yu R, Li C, Sun L, Jian L, Ma Z, Zhao J, et al. Hypoxia induces production of citrullinated proteins in human fibroblast-like synoviocytes through regulating HIF1alpha. Scand J Immunol (2018) 87(4):e12654.
85. Fan T, Zhang C, Zong M, Fan L. Hypoxiainduced autophagy is inhibited by PADI4 knockdown, which promotes apoptosis of fibroblastlike synoviocytes in rheumatoid arthritis. Mol Med Rep (2018) 17(4):5116–24.
86. Blachere NE, Parveen S, Frank MO, Dill BD, Molina H, Orange DE. High-titer rheumatoid arthritis antibodies preferentially bind fibrinogen citrullinated by peptidylarginine deiminase 4. Arthritis Rheumatol (2017) 69(5):986–95. doi: 10.1002/art.40035
87. Damgaard D, Bawadekar M, Senolt L, Stensballe A, Shelef MA, Nielsen CH. Relative efficiencies of peptidylarginine deiminase 2 and 4 in generating target sites for anti-citrullinated protein antibodies in fibrinogen, alpha-enolase and histone H3. PloS One (2018) 13(8):e0203214. doi: 10.1371/journal.pone.0203214
88. Fan L, Zong M, Gong R, He D, Li N, Sun LS, et al. PADI4 epigenetically suppresses p21 transcription and inhibits cell apoptosis in fibroblast-like synoviocytes from rheumatoid arthritis patients. Int J Biol Sci (2017) 13(3):358–66. doi: 10.7150/ijbs.16879
89. Okamato Y, Ghosh T, Okamoto T, Schuyler RP, Seifert J, Charry LL, et al. Subjects at-risk for future development of rheumatoid arthritis demonstrate a PAD4-and TLR-dependent enhanced histone H3 citrullination and proinflammatory cytokine production in CD14(hi) monocytes. J Autoimmun (2021) 117:102581. doi: 10.1016/j.jaut.2020.102581
90. Sanchez-Pernaute O, Filkova M, Gabucio A, Klein M, Maciejewska-Rodrigues H, Ospelt C, et al. Citrullination enhances the pro-inflammatory response to fibrin in rheumatoid arthritis synovial fibroblasts. Ann Rheum Dis (2013) 72(8):1400–6. doi: 10.1136/annrheumdis-2012-201906
91. Chang HH, Liu GY, Dwivedi N, Sun B, Okamoto Y, Kinslow JD, et al. A molecular signature of preclinical rheumatoid arthritis triggered by dysregulated PTPN22. JCI Insight (2016) 1(17):e90045. doi: 10.1172/jci.insight.90045
92. Chang HH, Dwivedi N, Nicholas AP, Ho IC. The W620 polymorphism in PTPN22 disrupts its interaction with peptidylarginine deiminase type 4 and enhances citrullination and NETosis. Arthritis Rheumatol (2015) 67(9):2323–34. doi: 10.1002/art.39215
93. Aratani S, Fujita H, Yagishita N, Yamano Y, Okubo Y, Nishioka K, et al. Inhibitory effects of ubiquitination of synoviolin by PADI4. Mol Med Rep (2017) 16(6):9203–9. doi: 10.3892/mmr.2017.7764
94. Yan X, Yin L, Wang Y, Zhao Y, Chang X. The low binding affinity of ADAMTS4 for citrullinated fibronectin may contribute to the destruction of joint cartilage in rheumatoid arthritis. Clin Exp Rheumatol (2013) 31(2):201–6.
95. Song ST, Kim SS, Kim JY, Lee SY, Kim K, Kwon IS, et al. Association of single nucleotide polymorphisms of PADI4 and HLA-DRB1 alleles with susceptibility to rheumatoid arthritis-related lung diseases. Lung (2016) 194(5):745–53. doi: 10.1007/s00408-016-9916-x
96. Guo ZY, Zhang JX, Wu M, Mei YF, Lin XJ, Bu C, et al. Meta-analysis of the association between PADI4 -92C/G polymorphism and rheumatoid arthritis in the Chinese population. Braz J Med Biol Res (2017) 50(10):e6115. doi: 10.1590/1414-431x20176115
97. Gong LL, Chang J, Yang YM. Association between peptidyl arginine deiminase 4 (PADI4)-104C/T polymorphism and rheumatoid arthritis: A meta-analysis in the Chinese population. Genet Mol Res (2016) 15(3):1–8. doi: 10.4238/gmr.15038750
98. Roudier J, Balandraud N, Auger I. How RA associated HLA-DR molecules contribute to the development of antibodies to citrullinated proteins: The hapten carrier model. Front Immunol (2022) 13:930112. doi: 10.3389/fimmu.2022.930112
99. Balandraud N, Auger I, Roudier J. Do RA associated HLA-DR molecules bind citrullinated peptides or peptides from PAD4 to help the development of RA specific antibodies to citrullinated proteins? J Autoimmun (2021) 116:102542. doi: 10.1016/j.jaut.2020.102542
100. Tsoyi K, Esposito AJ, Sun B, Bowen RG, Xiong K, Poli F, et al. Syndecan-2 regulates PAD2 to exert antifibrotic effects on RA-ILD fibroblasts. Sci Rep (2022) 12(1):2847. doi: 10.1038/s41598-022-06678-7
101. Samara KD, Trachalaki A, Tsitoura E, Koutsopoulos AV, Lagoudaki ED, Lasithiotaki I, et al. Upregulation of citrullination pathway: From autoimmune to idiopathic lung fibrosis. Respir Res (2017) 18(1):218. doi: 10.1186/s12931-017-0692-9
102. Ali MA, Abdelaziz A, Ali M, Abonar A, Hanafy M, Hussein H, et al. PADI4 (rs2240340), PDCD1 (rs10204525), and CTLA4 (231775) gene polymorphisms and polyarticular juvenile idiopathic arthritis. Br J BioMed Sci (2020) 77(3):123–8. doi: 10.1080/09674845.2020.1730626
103. Hisa K, Yanagimachi MD, Naruto T, Miyamae T, Kikuchi M, Hara R, et al. PADI4 and the HLA-DRB1 shared epitope in juvenile idiopathic arthritis. PloS One (2017) 12(2):e0171961. doi: 10.1371/journal.pone.0171961
104. Seri Y, Shoda H, Suzuki A, Matsumoto I, Sumida T, Fujio K, et al. Peptidylarginine deiminase type 4 deficiency reduced arthritis severity in a glucose-6-phosphate isomerase-induced arthritis model. Sci Rep (2015) 5:13041. doi: 10.1038/srep13041
105. Yang Y, Dai M. Expression of PADI4 in patients with ankylosing spondylitis and its role in mediating the effects of TNF-alpha on the proliferation and osteogenic differentiation of human mesenchymal stem cells. Int J Mol Med (2015) 36(2):565–70. doi: 10.3892/ijmm.2015.2248
106. Ishigami A, Masutomi H, Handa S, Nakamura M, Nakaya S, Uchida Y, et al. Mass spectrometric identification of citrullination sites and immunohistochemical detection of citrullinated glial fibrillary acidic protein in alzheimer’s disease brains. J Neurosci Res (2015) 93(11):1664–74. doi: 10.1002/jnr.23620
107. Jang B, Ishigami A, Maruyama N, Carp RI, Kim YS, Choi EK. Peptidylarginine deiminase and protein citrullination in prion diseases: strong evidence of neurodegeneration. Prion (2013) 7(1):42–6. doi: 10.4161/pri.22380
108. Petrozziello T, Mills AN, Vaine CA, Penney EB, Fernandez-Cerado C, Legarda GPA, et al. Neuroinflammation and histone H3 citrullination are increased in X-linked dystonia parkinsonism post-mortem prefrontal cortex. Neurobiol Dis (2020) 144:105032. doi: 10.1016/j.nbd.2020.105032
109. Falcao AM, Meijer M, Scaglione A, Rinwa P, Agirre E, Liang J, et al. PAD2-mediated citrullination contributes to efficient oligodendrocyte differentiation and myelination. Cell Rep (2019) 27(4):1090–1102.e10. doi: 10.1016/j.celrep.2019.03.108
110. U KP, Subramanian V, Nicholas AP, Thompson PR, Ferretti P. Modulation of calcium-induced cell death in human neural stem cells by the novel peptidylarginine deiminase-AIF pathway. Biochim Biophys Acta (2014) 1843(6):1162–71.
111. Shen S, Wang X, Lv H, Shi Y, Xiao L. PADI4 mediates autophagy and participates in the role of ganoderic acid a monomers in delaying the senescence of alzheimer’s cells through the Akt/mTOR pathway. Biosci Biotechnol Biochem (2021) 85(8):1818–29. doi: 10.1093/bbb/zbab054
112. Tanikawa C, Ueda K, Suzuki A, Iida A, Nakamura R, Atsuta N, et al. Citrullination of RGG motifs in FET proteins by PAD4 regulates protein aggregation and ALS susceptibility. Cell Rep (2018) 22(6):1473–83. doi: 10.1016/j.celrep.2018.01.031
113. Buono RJ, Bradfield JP, Wei Z, Sperling MR, Dlugos DJ, Privitera MD, et al. Genetic variation in PADI6-PADI4 on 1p36.13 is associated with common forms of human generalized epilepsy. Genes (Basel) (2021) 12(9):1441. doi: 10.3390/genes12091441
114. Molinaro R, Yu M, Sausen G, Bichsel CA, Corbo C, Folco EJ, et al. Targeted delivery of protein arginine deiminase-4 inhibitors to limit arterial intimal NETosis and preserve endothelial integrity. Cardiovasc Res (2021) 117(13):2652–63. doi: 10.1093/cvr/cvab074
115. Shi Y, Yang S, Luo M, Zhang WD, Ke ZP. Systematic analysis of coronary artery disease datasets revealed the potential biomarker and treatment target. Oncotarget (2017) 8(33):54583–91. doi: 10.18632/oncotarget.17426
116. Shimonaga K, Matsushige T, Takahashi H, Hashimoto Y, Yoshiyama M, Ono C, et al. Peptidylarginine deiminase 4 as a possible biomarker of plaque instability in carotid artery stenosis. J Stroke Cerebrovasc Dis (2021) 30(7):105816. doi: 10.1016/j.jstrokecerebrovasdis.2021.105816
117. Hounkpe BW, Chenou F, Domingos IF, Cardoso EC, Sobreira Costa MJV, Araujo AS, et al. Neutrophil extracellular trap regulators in sickle cell disease: Modulation of gene expression of PADI4, neutrophil elastase, and myeloperoxidase during vaso-occlusive crisis. Res Pract Thromb Haemost (2021) 5(1):204–10. doi: 10.1002/rth2.12463
118. Gollomp K, Kim M, Johnston I, Hayes V, Welsh J, Arepally GM, et al. Neutrophil accumulation and NET release contribute to thrombosis in HIT. JCI Insight (2018) 3(18):e99445. doi: 10.1172/jci.insight.99445
119. Erpenbeck L, Chowdhury CS, Zsengeller ZK, Gallant M, Burke SD, Cifuni S, et al. PAD4 deficiency decreases inflammation and susceptibility to pregnancy loss in a mouse model. Biol Reprod (2016) 95(6):132. doi: 10.1095/biolreprod.116.140293
120. Wang ZL, Shang JC, Li CM, Li M, Xing GQ. Significance of serum peptidylarginine deiminase type 4 in ANCA-associated vasculitis. Beijing Da Xue Bao Yi Xue Ban (2014) 46(2):200–6.
121. Liu Y, Lightfoot YL, Seto N, Carmona-Rivera C, Moore E, Goel R, et al. Peptidylarginine deiminases 2 and 4 modulate innate and adaptive immune responses in TLR-7-dependent lupus. JCI Insight (2018) 3(23):e124729. doi: 10.1172/jci.insight.124729
122. Hanata N, Shoda H, Hatano H, Nagafuchi Y, Komai T, Okamura T, et al. Peptidylarginine deiminase 4 promotes the renal infiltration of neutrophils and exacerbates the TLR7 agonist-induced lupus mice. Front Immunol (2020) 11:1095. doi: 10.3389/fimmu.2020.01095
123. Odqvist L, Jevnikar Z, Riise R, Oberg L, Rhedin M, Leonard D, et al. Genetic variations in A20 DUB domain provide a genetic link to citrullination and neutrophil extracellular traps in systemic lupus erythematosus. Ann Rheum Dis (2019) 78(10):1363–70. doi: 10.1136/annrheumdis-2019-215434
124. Leppkes M, Maueroder C, Hirth S, Nowecki S, Gunther C, Billmeier U, et al. Externalized decondensed neutrophil chromatin occludes pancreatic ducts and drives pancreatitis. Nat Commun (2016) 7:10973. doi: 10.1038/ncomms10973
125. Tian Y, Russo RM, Li Y, Karmakar M, Liu B, Puskarich MA, et al. Serum citrullinated histone H3 concentrations differentiate patients with septic verses non-septic shock and correlate with disease severity. Infection (2021) 49(1):83–93. doi: 10.1007/s15010-020-01528-y
126. Costa NA, Gut AL, Azevedo PS, Polegato BF, Magalhaes ES, Ishikawa LLW, et al. Peptidylarginine deiminase 4 concentration, but not PADI4 polymorphisms, is associated with ICU mortality in septic shock patients. J Cell Mol Med (2018) 22(10):4732–7. doi: 10.1111/jcmm.13717
127. Colon DF, Wanderley CW, Franchin M, Silva CM, Hiroki CH, Castanheira FVS, et al. Neutrophil extracellular traps (NETs) exacerbate severity of infant sepsis. Crit Care (2019) 23(1):113. doi: 10.1186/s13054-019-2407-8
128. Du M, Yang L, Gu J, Wu J, Ma Y, Wang T. Inhibition of peptidyl arginine deiminase-4 prevents renal ischemia-Reperfusion-Induced remote lung injury. Mediators Inflamm 2020 (2020) p:1724206. doi: 10.1155/2020/1724206
129. Rabadi MM, Han SJ, Kim M, V, Lee HT. Peptidyl arginine deiminase-4 exacerbates ischemic AKI by finding NEMO. Am J Physiol Renal Physiol (2019) 316(6):F1180–90. doi: 10.1152/ajprenal.00089.2019
130. Rabadi M, Kim M, Li H, Han SJ, Choi Y, D'Agati V. ATP induces PAD4 in renal proximal tubule cells via P2X7 receptor activation to exacerbate ischemic AKI. Am J Physiol Renal Physiol (2018) 314(2):F293–305. doi: 10.1152/ajprenal.00364.2017
131. Ham A, Rabadi M, Kim M, Brown KM, Ma Z, D'Agati V, et al. Peptidyl arginine deiminase-4 activation exacerbates kidney ischemia-reperfusion injury. Am J Physiol Renal Physiol (2014) 307(9):F1052–62. doi: 10.1152/ajprenal.00243.2014
132. Byrd AS, Carmona-Rivera C, O'Neil LJ, Carlucci PM, Cisar C, Rosenberg AZ, et al. Neutrophil extracellular traps, B cells, and type I interferons contribute to immune dysregulation in hidradenitis suppurativa. Sci Transl Med (2019) 11(508):eaav5908. doi: 10.1126/scitranslmed.aav5908
133. Muraro SP, De Souza GF, Gallo SW, Da Silva BK, De Oliveira SD, Vinolo MAR, et al. Respiratory syncytial virus induces the classical ROS-dependent NETosis through PAD-4 and necroptosis pathways activation. Sci Rep (2018) 8(1):14166. doi: 10.1038/s41598-018-32576-y
134. Mahajan A, Hasikova L, Hampel U, Gruneboom A, Shan X, Herrmann I, et al. Aggregated neutrophil extracellular traps occlude meibomian glands during ocular surface inflammation. Ocul Surf (2021) 20:1–12. doi: 10.1016/j.jtos.2020.12.005
135. Wong SL, Demers M, Martinod K, Gallant M, Wang Y, Goldfine AB, et al. Diabetes primes neutrophils to undergo NETosis, which impairs wound healing. Nat Med (2015) 21(7):815–9. doi: 10.1038/nm.3887
136. Munoz LE, Boeltz S, Bilyy R, Schauer C, Mahajan A, Widulin N, et al. Neutrophil extracellular traps initiate gallstone formation. Immunity (2019) 51(3):443–450.e4. doi: 10.1016/j.immuni.2019.07.002
137. Engstrom M, Eriksson K, Lee L, Hermansson M, Johansson A, Nicholas AP, et al. Increased citrullination and expression of peptidylarginine deiminases independently of p. gingivalis and a. actinomycetemcomitans in gingival tissue of patients with periodontitis. J Transl Med (2018) 16(1):214.
138. Akkaya HU, Yilmaz HE, Narin F, Saglam M. Evaluation of galectin-3, peptidylarginine deiminase-4, and tumor necrosis factor-alpha levels in gingival crevicular fluid for periodontal health, gingivitis, and stage III grade c periodontitis: A pilot study. J Periodontol (2022) 93(1):80–8. doi: 10.1002/JPER.21-0137
139. Harvey GP, Fitzsimmons TR, Dhamarpatni AA, Marchant C, Haynes DR, Bartold PM. Expression of peptidylarginine deiminase-2 and -4, citrullinated proteins and anti-citrullinated protein antibodies in human gingiva. J Periodontal Res (2013) 48(2):252–61. doi: 10.1111/jre.12002
140. Kim SE, Park JW, Kim MJ, Jang B, Jeon YC, Kim HJ, et al. Accumulation of citrullinated glial fibrillary acidic protein in a mouse model of bile duct ligation-induced hepatic fibrosis. PloS One (2018) 13(8):e0201744. doi: 10.1371/journal.pone.0201744
141. Casanova V, Sousa FH, Shakamuri P, Svoboda P, Buch C, D'Acremont M, et al. Citrullination alters the antiviral and immunomodulatory activities of the human cathelicidin LL-37 during rhinovirus infection. Front Immunol (2020) 11:85. doi: 10.3389/fimmu.2020.00085
142. Damiana T, Damgaard D, Sidelmann JJ, Nielsen CH, Maat MPM, Munster AB, et al. Citrullination of fibrinogen by peptidylarginine deiminase 2 impairs fibrin clot structure. Clin Chim Acta (2020) 501:6–11. doi: 10.1016/j.cca.2019.10.033
143. Qin H, Liu X, Li F, Miao L, Li T, Xu B, et al. PAD1 promotes epithelial-mesenchymal transition and metastasis in triple-negative breast cancer cells by regulating MEK1-ERK1/2-MMP2 signaling. Cancer Lett (2017) 409:30–41. doi: 10.1016/j.canlet.2017.08.019
144. Ji T, Ma K, Chen L, Cao T. PADI1 contributes to EMT in PAAD by activating the ERK1/2-p38 signaling pathway. J Gastrointest Oncol (2021) 12(3):1180–90. doi: 10.21037/jgo-21-283
145. Chen Y, Xu R, Ruze R, Yang J, Wang H, Song J, et al. Construction of a prognostic model with histone modification-related genes and identification of potential drugs in pancreatic cancer. Cancer Cell Int (2021) 21(1):291. doi: 10.1186/s12935-021-01928-6
146. Zhang Z, Lin E, Zhuang H, Xie L, Feng X, Liu J, et al. Construction of a novel gene-based model for prognosis prediction of clear cell renal cell carcinoma. Cancer Cell Int (2020) 20:27. doi: 10.1186/s12935-020-1113-6
147. Wang J, Liu D, Gu Y, Zhou H, Li H, Shen X, et al. Potential prognostic markers and significant lncRNA-mRNA co-expression pairs in laryngeal squamous cell carcinoma. Open Life Sci (2021) 16(1):544–57. doi: 10.1515/biol-2021-0052
148. McElwee JL, Mohanan S, Griffith OL, Breuer HC, Anguish LJ, Cherrington BD, et al. Identification of PADI2 as a potential breast cancer biomarker and therapeutic target. BMC Cancer (2012) 12:500. doi: 10.1186/1471-2407-12-500
149. DeVore SB, Young CH, Li G, Sundararajan A, Ramaraj T, Mudge J, et al. Histone citrullination represses MicroRNA expression, resulting in increased oncogene mRNAs in somatolactotrope cells. Mol Cell Biol (2018) 38(19):e00084–18. doi: 10.1128/MCB.00084-18
150. Guo W, Zheng Y, Xu B, Ma F, Li C, Zhang X, et al. Investigating the expression, effect and tumorigenic pathway of PADI2 in tumors. Onco Targets Ther (2017) 10:1475–85. doi: 10.2147/OTT.S92389
151. Funayama R, Taniguchi H, Mizuma M, Fujishima F, Kobayashi M, Ohnuma S, et al. Protein-arginine deiminase 2 suppresses proliferation of colon cancer cells through protein citrullination. Cancer Sci (2017) 108(4):713–8. doi: 10.1111/cas.13179
152. Wang H, Xu B, Zhang X, Zheng Y, Zhao Y, Chang X. PADI2 gene confers susceptibility to breast cancer and plays tumorigenic role via ACSL4, BINC3 and CA9 signaling. Cancer Cell Int (2016) 16:61. doi: 10.1186/s12935-016-0335-0
153. Tanday S. Targeting PADI2 could stop the progression of myeloma. Lancet Oncol (2016) 17(8):e325. doi: 10.1016/S1470-2045(16)30314-X
154. Mohanan S, Horibata S, Anguish LJ, Mukai C, Sams K, McElwee JL, et al. PAD2 overexpression in transgenic mice augments malignancy and tumor-associated inflammation in chemically initiated skin tumors. Cell Tissue Res (2017) 370(2):275–83. doi: 10.1007/s00441-017-2669-x
155. McElwee JL, Mohanan S, Horibata S, Sams KL, Anguish LJ, McLean D, et al. PAD2 overexpression in transgenic mice promotes spontaneous skin neoplasia. Cancer Res (2014) 74(21):6306–17. doi: 10.1158/0008-5472.CAN-14-0749
156. Xue T, Liu X, Zhang M, E Q, Liu S, Zou M, et al. PADI2-catalyzed MEK1 citrullination activates ERK1/2 and promotes IGF2BP1-mediated SOX2 mRNA stability in endometrial cancer. Adv Sci (Weinh) (2021) 8(6):2002831. doi: 10.1002/advs.202002831
157. Liu L, Zhang Z, Zhang G, Wang T, Ma Y, Guo W. Down-regulation of PADI2 prevents proliferation and epithelial-mesenchymal transition in ovarian cancer through inhibiting JAK2/STAT3 pathway in vitro and in vivo, alone or in combination with olaparib. J Transl Med (2020) 18(1):357. doi: 10.1186/s12967-020-02528-0
158. Qu Y, Olsen JR, Yuan X, Cheng PF, Levesque MP, Brokstad KA, et al. Small molecule promotes beta-catenin citrullination and inhibits wnt signaling in cancer. Nat Chem Biol (2018) 14(1):94–101. doi: 10.1038/nchembio.2510
159. Horibata S, Rogers KE, Sadegh D, Anguish LJ, McElwee JL, Shah P, et al. Role of peptidylarginine deiminase 2 (PAD2) in mammary carcinoma cell migration. BMC Cancer (2017) 17(1):378. doi: 10.1186/s12885-017-3354-x
160. Chen D, Sun Q, Zhang L, Zhou X, Cheng X, Zhou D, et al. The lncRNA HOXA11-AS functions as a competing endogenous RNA to regulate PADI2 expression by sponging miR-125a-5p in liver metastasis of colorectal cancer. Oncotarget (2017) 8(41):70642–52. doi: 10.18632/oncotarget.19956
161. Gao BS, Rong CS, Xu HM, Sun T, Hou J, Xu Y. Peptidyl arginine deiminase, type II (PADI2) is involved in urothelial bladder cancer. Pathol Oncol Res (2020) 26(2):1279–85. doi: 10.1007/s12253-019-00687-0
162. Sase T, Arito M, Onodera H, Omoteyama K, Kurokawa MS, Kagami Y, et al. Hypoxia-induced production of peptidylarginine deiminases and citrullinated proteins in malignant glioma cells. Biochem Biophys Res Commun (2017) 482(1):50–6. doi: 10.1016/j.bbrc.2016.10.154
163. Bai J, Khajavi M, Sui L, Fu H, Tarakkad Krishnaji S, Birsner AE, et al. Angiogenic responses in a 3D micro-engineered environment of primary endothelial cells and pericytes. Angiogenesis (2021) 24(1):111–27. doi: 10.1007/s10456-020-09746-6
164. Khajavi M, Zhou Y, Birsner AE, Bazinet L, Sant Di Rosa A, Schiffer AJ, et al. Identification of Padi2 as a novel angiogenesis-regulating gene by genome association studies in mice. PloS Genet (2017) 13(6):e1006848. doi: 10.1371/journal.pgen.1006848
165. Li F, Miao L, Xue T, Qin H, Mondal S, Thompson PR, et al. Inhibiting PAD2 enhances the anti-tumor effect of docetaxel in tamoxifen-resistant breast cancer cells. J Exp Clin Cancer Res (2019) 38(1):414. doi: 10.1186/s13046-019-1404-8
166. Chang X, Chai Z, Zou J, Wang H, Wang Y, Zheng Y, et al. PADI3 induces cell cycle arrest via the Sirt2/AKT/p21 pathway and acts as a tumor suppressor gene in colon cancer. Cancer Biol Med (2019) 16(4):729–42. doi: 10.20892/j.issn.2095-3941.2019.0065
167. Chai Z, Wang L, Zheng Y, Liang N, Wang X, Zheng Y, et al. PADI3 plays an antitumor role via the Hsp90/CKS1 pathway in colon cancer. Cancer Cell Int (2019) 19:277. doi: 10.1186/s12935-019-0999-3
168. Moshkovich N, Ochoa HJ, Tang B, Yang HH, Yang Y, Huang J, et al. Peptidylarginine deiminase IV regulates breast cancer stem cells via a novel tumor cell-autonomous suppressor role. Cancer Res (2020) 80(11):2125–37. doi: 10.1158/0008-5472.CAN-19-3018
169. Zhang Y, Chandra V, Sanchez Riquelme E, Dutta P, Quesada PR, Rakoski A, et al. Interleukin-17-induced neutrophil extracellular traps mediate resistance to checkpoint blockade in pancreatic cancer. J Exp Med (2020) 217(12):e20190354. doi: 10.1084/jem.20190354
170. Mohanty V, Subbannayya Y, Patil S, Puttamallesh VN, Najar MA, Datta KK, et al. Molecular alterations in oral cancer using high-throughput proteomic analysis of formalin-fixed paraffin-embedded tissue. J Cell Commun Signal (2021) 15(3):447–59. doi: 10.1007/s12079-021-00609-3
171. Liu C, Tang J, Li C, Pu G, Yang D, Chang X. PADI4 stimulates esophageal squamous cell carcinoma tumor growth and up-regulates CA9 expression. Mol Carcinog (2019) 58(1):66–75. doi: 10.1002/mc.22907
172. Wang W, Ji HJ, Sun NB, Chang XT, Xu B, Wang Y, et al. B-cell specific moloney leukemia virus insert site 1 and peptidyl arginine deiminase IV positively regulate carcinogenesis and progression of esophageal squamous cell carcinoma. Oncol Lett (2017) 13(6):4349–56. doi: 10.3892/ol.2017.6001
173. Gu W, Zhang M, Gao F, Niu Y, Sun L, Xia H, et al. Berberine regulates PADI4-related macrophage function to prevent lung cancer. Int Immunopharmacol (2022) 110:108965. doi: 10.1016/j.intimp.2022.108965
174. Liu M, Qu Y, Teng X, Xing Y, Li D, Li C, et al. PADI4mediated epithelialmesenchymal transition in lung cancer cells. Mol Med Rep (2019) 19(4):3087–94.
175. Shi L, Yao H, Liu Z, Xu M, Tsung A, Wang Y. Endogenous PAD4 in breast cancer cells mediates cancer extracellular chromatin network formation and promotes lung metastasis. Mol Cancer Res (2020) 18(5):735–47. doi: 10.1158/1541-7786.MCR-19-0018
176. Miller-Ocuin JL, Liang X, Boone BA, Doerfler WR, Singhi AD, Tang D, et al. DNA Released from neutrophil extracellular traps (NETs) activates pancreatic stellate cells and enhances pancreatic tumor growth. Oncoimmunology (2019) 8(9):e1605822. doi: 10.1080/2162402X.2019.1605822
177. Stadler SC, Vincent CT, Fedorov VD, Patsialou A, Cherrington BD, Wakshlag JJ, et al. Dysregulation of PAD4-mediated citrullination of nuclear GSK3beta activates TGF-beta signaling and induces epithelial-to-mesenchymal transition in breast cancer cells. Proc Natl Acad Sci U.S.A. (2013) 110(29):11851–6. doi: 10.1073/pnas.1308362110
178. Chang XT, Wu H, Li HL, Li HL, Zheng YB. PADI4 promotes epithelial-mesenchymal transition(EMT) in gastric cancer via the upregulation of interleukin 8. BMC Gastroenterol (2022) 22(1):25. doi: 10.1186/s12876-022-02097-0
179. Xin J, Song X. Role of peptidylarginine deiminase type 4 in gastric cancer. Exp Ther Med (2016) 12(5):3155–60. doi: 10.3892/etm.2016.3798
180. Guo J, Yin L, Zhang X, Su P, Zhai Q. Factors associated with promoted proliferation of osteosarcoma by peptidylarginine deiminase 4. BioMed Res Int (2021) 2021:5596014. doi: 10.1155/2021/5596014
181. Chen H, Wei L, Luo M, Wang X, Zhu C, Huang H, et al. LINC00324 suppresses apoptosis and autophagy in nasopharyngeal carcinoma through upregulation of PAD4 and activation of the PI3K/AKT signaling pathway. Cell Biol Toxicol (2022) 38(6):995–1011. doi: 10.1007/s10565-021-09632-x
182. Zhang C, Fan L, Fan T, Wu D, Gao L, Ling Y, et al. Decreased PADI4 mRNA association with global hypomethylation in hepatocellular carcinoma during HBV exposure. Cell Biochem Biophys (2013) 65(2):187–95. doi: 10.1007/s12013-012-9417-3
183. Chen H, Wei L, Luo M, Wang X, Zhan Y, Mao Y, et al. PAD4 inhibitor promotes DNA damage and radiosensitivity of nasopharyngeal carcinoma cells. Environ Toxicol (2021) 36(11):2291–301. doi: 10.1002/tox.23342
184. Chen H, Luo M, Wang X, Liang T, Huang C, Huang C, et al. Correction to: Inhibition of PAD4 enhances radiosensitivity and inhibits aggressive phenotypes of nasopharyngeal carcinoma cells. Cell Mol Biol Lett (2021) 26(1):55. doi: 10.1186/s11658-021-00303-7
185. Wei LN, Luo M, Wang XP, Liang T, Huang CJ, Chen H. PADI4, negatively regulated by miR-335-5p, participates in regulating the proliferation, migration, invasion and radiosensitivity of nasopharyngeal carcinoma cells. J Biol Regul Homeost Agents (2021) 35(1):117–29.
186. Luo X, Chang S, Xiao S, Peng Y, Gao Y, Hu F, et al. PAD4-dependent citrullination of nuclear translocation of GSK3beta promotes colorectal cancer progression via the degradation of nuclear CDKN1A. Neoplasia (2022) 33:100835. doi: 10.1016/j.neo.2022.100835
187. Wang Y, Lyu Y, Tu K, Xu Q, Yang Y, Salman S, et al. Histone citrullination by PADI4 is required for HIF-dependent transcriptional responses to hypoxia and tumor vascularization. Sci Adv (2021) 7(35):eabe3771. doi: 10.1126/sciadv.abe3771
188. Qian J, Nguyen NMP, Rezaei M, Huang B, Tao Y, Zhang X, et al. Biallelic PADI6 variants linking infertility, miscarriages, and hydatidiform moles. Eur J Hum Genet (2018) 26(7):1007–13. doi: 10.1038/s41431-018-0141-3
189. Nowacka-Woszuk J, Stachowiak M, Szczerbal I, Szydlowski M, Szabelska-Beresewicz A, Zyprych-Walczak J, et al. Whole genome sequencing identifies a missense polymorphism in PADI6 associated with testicular/ovotesticular XX disorder of sex development in dogs. Genomics (2022) 114(4):110389. doi: 10.1016/j.ygeno.2022.110389
190. Dong J, Fu J, Yan Z, Li L, Qiu Y, Zeng Y, et al. Novel biallelic mutations in PADI6 in patients with early embryonic arrest. J Hum Genet (2022) 67(5):285–93. doi: 10.1038/s10038-021-00998-8
191. Fei CF, Zhou LQ. Gene mutations impede oocyte maturation, fertilization, and early embryonic development. Bioessays (2022) 44(10):e2200007. doi: 10.1002/bies.202200007
192. Tong X, Jin J, Hu Z, Zhang Y, Fan HY, Zhang YL, et al. Mutations in OOEP and NLRP5 identified in infertile patients with early embryonic arrest. Hum Mutat (2022) 43(12):1909–20. doi: 10.1002/humu.24448
193. Xu Y, Wang R, Pang Z, Wei Z, Sun L, Li S, et al. Novel homozygous PADI6 variants in infertile females with early embryonic arrest. Front Cell Dev Biol (2022) 10:819667. doi: 10.3389/fcell.2022.819667
194. Tannorella P, Calzari L, Daolio C, Mainini E, Vimercati A, Gentilini D, et al. Germline variants in genes of the subcortical maternal complex and multilocus imprinting disturbance are associated with miscarriage/infertility or beckwith-wiedemann progeny. Clin Epigenet (2022) 14(1):43. doi: 10.1186/s13148-022-01262-2
195. Eggermann T, Yapici E, Bliek J, Pereda A, Begemann M, Russo S, et al. Trans-acting genetic variants causing multilocus imprinting disturbance (MLID): common mechanisms and consequences. Clin Epigenet (2022) 14(1):41. doi: 10.1186/s13148-022-01259-x
196. Huang B, Zhao Y, Zhou L, Gong T, Feng J, Han P, et al. PADI6 regulates trophoblast cell migration-invasion through the Hippo/YAP1 pathway in hydatidiform moles. J Inflammation Res (2021) 14:3489–500. doi: 10.2147/JIR.S313422
197. Liu J, Tan Z, He J, Jin T, Han Y, Hu L, et al. Two novel mutations in PADI6 and TLE6 genes cause female infertility due to arrest in embryonic development. J Assist Reprod Genet (2021) 38(6):1551–9. doi: 10.1007/s10815-021-02194-1
198. Rezaei M, Suresh B, Bereke E, Hadipour Z, Aguinaga M, Qian J, et al. Novel pathogenic variants in NLRP7, NLRP5, and PADI6 in patients with recurrent hydatidiform moles and reproductive failure. Clin Genet (2021) 99(6):823–8. doi: 10.1111/cge.13941
199. Sang Q, Zhou Z, Mu J, Wang L. Genetic factors as potential molecular markers of human oocyte and embryo quality. J Assist Reprod Genet (2021) 38(5):993–1002. doi: 10.1007/s10815-021-02196-z
200. Eggermann T, Kadgien G, Begemann M, Elbracht M. Biallelic PADI6 variants cause multilocus imprinting disturbances and miscarriages in the same family. Eur J Hum Genet (2021) 29(4):575–80. doi: 10.1038/s41431-020-00762-0
201. Zheng W, Hu H, Dai J, Zhang S, Gu Y, Dai C, et al. Expanding the genetic and phenotypic spectrum of the subcortical maternal complex genes in recurrent preimplantation embryonic arrest. Clin Genet (2021) 99(2):286–91. doi: 10.1111/cge.13858
202. Cubellis MV, Pignata L, Verma A, Sparago A, Prete Del R, Monticelli M, et al. Loss-of-function maternal-effect mutations of PADI6 are associated with familial and sporadic beckwith-wiedemann syndrome with multi-locus imprinting disturbance. Clin Epigenet (2020) 12(1):139. doi: 10.1186/s13148-020-00925-2
203. Zheng W, Chen L, Dai J, Dai C, Guo J, Lu C, et al. New biallelic mutations in PADI6 cause recurrent preimplantation embryonic arrest characterized by direct cleavage. J Assist Reprod Genet (2020) 37(1):205–12. doi: 10.1007/s10815-019-01606-7
204. Robbins SM, Thimm MA, Valle D, Jelin AC. Genetic diagnosis in first or second trimester pregnancy loss using exome sequencing: a systematic review of human essential genes. J Assist Reprod Genet (2019) 36(8):1539–48. doi: 10.1007/s10815-019-01499-6
205. Begemann M, Rezwan FI, Beygo J, Docherty LE, Kolarova J, Schroeder C, et al. Maternal variants in NLRP and other maternal effect proteins are associated with multilocus imprinting disturbance in offspring. J Med Genet (2018) 55(7):497–504. doi: 10.1136/jmedgenet-2017-105190
206. Wang X, Song D, Mykytenko D, Kuang Y, Lv Q, Li B, et al. Novel mutations in genes encoding subcortical maternal complex proteins may cause human embryonic developmental arrest. Reprod BioMed Online (2018) 36(6):698–704. doi: 10.1016/j.rbmo.2018.03.009
207. Xu Y, Shi Y, Fu J, Yu M, Feng R, Sang Q, et al. Mutations in PADI6 cause female infertility characterized by early embryonic arrest. Am J Hum Genet (2016) 99(3):744–52. doi: 10.1016/j.ajhg.2016.06.024
208. Cau L, Takahara H, Thompson PR, Serre G, Mechin MC, Simon M. Peptidylarginine deiminase inhibitor cl-amidine attenuates cornification and interferes with the regulation of autophagy in reconstructed human epidermis. J Invest Dermatol (2019) 139(9):1889–1897.e4. doi: 10.1016/j.jid.2019.02.026
209. Hu L, Bikle DD, Oda Y. Reciprocal role of vitamin d receptor on beta-catenin regulated keratinocyte proliferation and differentiation. J Steroid Biochem Mol Biol (2014) 144 Pt A:237–41. doi: 10.1016/j.jsbmb.2013.11.002
210. Jin M, Lu J, Fei X, Lu Z, Quan K, Liu Y, et al. Genetic signatures of selection for cashmere traits in Chinese goats. Anim (Basel) (2020) 10(10):1905. doi: 10.3390/ani10101905
211. Malki L, Sarig O, Romano MT, Mechin MC, Peled A, Pavlovsky M, et al. Variant PADI3 in central centrifugal cicatricial alopecia. N Engl J Med (2019) 380(9):833–41. doi: 10.1056/NEJMoa1816614
212. Fert-Bober J, Giles JT, Holewinski RJ, Kirk JA, Uhrigshardt H, Crowgey EL, et al. Citrullination of myofilament proteins in heart failure. Cardiovasc Res (2015) 108(2):232–42. doi: 10.1093/cvr/cvv185
213. Hollingsworth TJ, Radic MZ, Beranova-Giorgianni S, Giorgianni F, Wang Y, Iannaccone A. Murine retinal citrullination declines with age and is mainly dependent on peptidyl arginine deiminase 4 (PAD4). Invest Ophthalmol Vis Sci (2018) 59(10):3808–15. doi: 10.1167/iovs.18-24118
214. Bonilha VL, Shadrach KG, Rayborn ME, Li Y, Pauer GJ, Hagstrom SA, et al. Retinal deimination and PAD2 levels in retinas from donors with age-related macular degeneration (AMD). Exp Eye Res (2013) 111:71–8. doi: 10.1016/j.exer.2013.03.017
215. Sun B, Tomita B, Salinger A, Tilvawala RR, Li L, Hakami H, et al. PAD2-mediated citrullination of fibulin-5 promotes elastogenesis. Matrix Biol (2021) 102:70–84. doi: 10.1016/j.matbio.2021.07.001
216. Kim HJ, Kim WJ, Shin HR, Yoon HI, Moon JI, Lee E, et al. ROS-induced PADI2 downregulation accelerates cellular senescence via the stimulation of SASP production and NFkappaB activation. Cell Mol Life Sci (2022) 79(3):155.
217. Cao YH, Xu SS, Shen M, Chen ZH, Gao L, Lv FH, et al. Historical introgression from wild relatives enhanced climatic adaptation and resistance to pneumonia in sheep. Mol Biol Evol (2021) 38(3):838–55. doi: 10.1093/molbev/msaa236
218. Lim MK, Shim TS, Park M, Lee SK, Sohn YH, Sheen DH, et al. Heterozygote genotypes for PADI4_89 were protectively associated with susceptibility to tuberculosis in koreans. Rheumatol Int (2015) 35(4):651–5. doi: 10.1007/s00296-014-3119-7
219. Sun YN, Ma YN, Jia XQ, Yao Q, Chen JP, Li H. Inducement of ER stress by PAD inhibitor BB-Cl-Amidine to effectively kill AML cells. Curr Med Sci (2022) 42(5):958–65. doi: 10.1007/s11596-022-2637-x
220. Hemmati-Dinarvand M, Kheirandish S, Khodadadian A, Mostafazadeh M, Seghatoleslam A. Blockage of wnt/beta-catenin signaling pathway in colorectal cancer resistant cells by nitazoxanide effects on peptidylarginine deiminases expression. Asian Pac J Cancer Prev (2022) 23(9):3215–22. doi: 10.31557/APJCP.2022.23.9.3215
221. Zhao X, Gu C, Wang Y. PAD4 selective inhibitor TDFA protects lipopolysaccharide-induced acute lung injury by modulating nuclear p65 localization in epithelial cells. Int Immunopharmacol (2020) 88:106923. doi: 10.1016/j.intimp.2020.106923
222. Tejeda EJC, Bello AM, Wasilewski E, Koebel A, Dunn S, Kotra LP. Noncovalent protein arginine deiminase (PAD) inhibitors are efficacious in animal models of multiple sclerosis. J Med Chem (2017) 60(21):8876–87. doi: 10.1021/acs.jmedchem.7b01102
223. Park B, Yim JH, Lee HK, Kim BO, Pyo S. Ramalin inhibits VCAM-1 expression and adhesion of monocyte to vascular smooth muscle cells through MAPK and PADI4-dependent NF-kB and AP-1 pathways. Biosci Biotechnol Biochem (2015) 79(4):539–52. doi: 10.1080/09168451.2014.991681
Keywords: PADI, citrullination, autoimmune, cancer, inflammatory
Citation: Zhu C, Liu C and Chai Z (2023) Role of the PADI family in inflammatory autoimmune diseases and cancers: A systematic review. Front. Immunol. 14:1115794. doi: 10.3389/fimmu.2023.1115794
Received: 04 December 2022; Accepted: 08 February 2023;
Published: 20 March 2023.
Edited by:
Steven O’Reilly, STipe Therapeutics, DenmarkReviewed by:
Xiaotian Chang, The Affiliated Hospital of Qingdao University, ChinaShicheng Guo, University of Wisconsin-Madison, United States
Copyright © 2023 Zhu, Liu and Chai. This is an open-access article distributed under the terms of the Creative Commons Attribution License (CC BY). The use, distribution or reproduction in other forums is permitted, provided the original author(s) and the copyright owner(s) are credited and that the original publication in this journal is cited, in accordance with accepted academic practice. No use, distribution or reproduction is permitted which does not comply with these terms.
*Correspondence: Chunyan Liu, bGl1Y2h1bnlhbjIwMThAMTI2LmNvbQ==; Zhengbin Chai, Y2hhaXpoZW5nYmluQDEyNi5jb20=