- III. Department of Medicine, Division of Translational Immunology, University Medical Hospital Center Hamburg-Eppendorf, Hamburg, Germany
The discovery of tissue-resident memory T cells (TRM cells) reinterpreted the potential of human tissue-specific immunity. Following T cell receptor (TCR) activation and clonal expansion, effector T cells migrate to peripheral tissues where they remain long-term and differentiate to TRM cells after antigen clearance. This allows for prompt immunological responses upon antigen re-encounter. In addition to their protective properties in acute infections, recent studies have revealed that TRM cells might lead to aggravation of autoimmune diseases, such as lupus nephritis (LN) and anti-neutrophil cytoplasmic antibody (ANCA)-associated glomerulonephritis (GN). These diseases present as proliferative and crescentic glomerulonephritis (cGN), which is a life-threatening condition leading to end-stage renal disease (ESRD) if left untreated. A better understanding of renal TRM cells might lead to identifying new therapeutic targets for relapsing autoimmune diseases of the kidney. In this review, we summarize the current knowledge of renal TRM cells and discuss their potential pathophysiological roles in renal autoimmune diseases.
Introduction
Tissue-resident memory T cells (TRM cells) are a recently identified lymphocyte subset that resides in peripheral organs for long time periods (1, 2). These cells have been detected and analyzed in numerous non-lymphoid tissues, especially barrier-organs. Mice studies have proven that TRM cells have superior effector functions including rapid chemokine and cytokine production. Hence, together with circulating memory T cells (e.g., effector memory T cells and central memory T cells), TRM cells are critical for efficient pathogen clearance, providing an antigen-specific immediate frontline defense in peripheral organs (1, 2).
The kidney is a non-barrier organ, but still frequently gets challenged by diverse pathogens, including bacteria, viruses, and fungi, which drive renal TRM cell generation (Figure 1A) (2, 5, 11–14). Due to limited access, exploring precise immune cell populations of healthy human kidneys had been challenging. In the last few years, however, detailed analysis of the immune landscape in healthy renal tissue has been implemented using flow cytometry and single-cell RNA sequencing (scRNA-seq) (2, 5, 8, 15–17). In particular, the combined analysis of mRNA expression and cell surface marker via cellular indexing of transcriptomes and epitopes by sequencing (CITE-seq) has provided a completely new insight into cell heterogeneity. Consequently, CD4+ and CD8+ T cells with a TRM cell phenotype were identified in the healthy human kidney, representing the most abundant tissue-resident immune cell population (8, 16, 17).
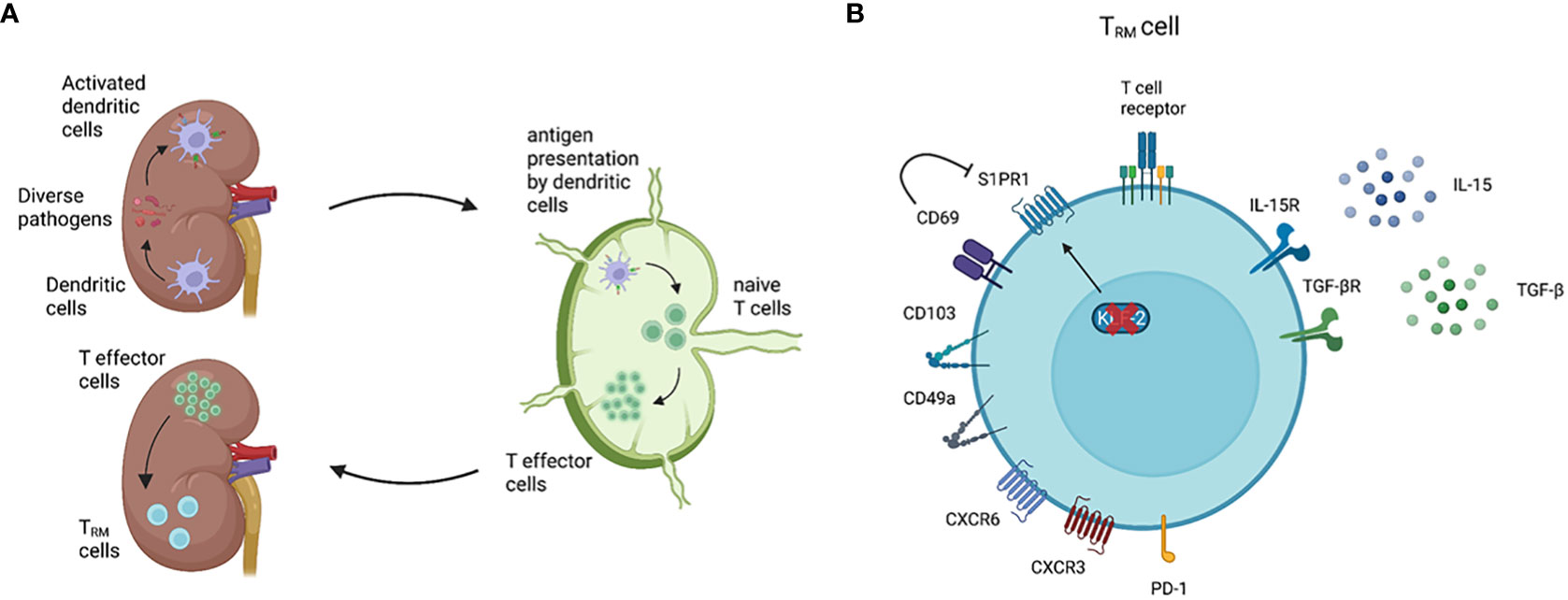
Figure 1 General features of TRM cells. Renal TRM cell development and phenotype. (A) As classical antigen-presenting cells (APC), dendritic cells (DCs) constantly surveil their microenvironment for pathogens and microbes. Once DCs in the kidney are activated, these cells migrate to the renal draining lymph nodes to present the processed antigen via the major histocompatibility complex (MHC) to naïve T cells (3). Upon activation, naïve T cells become effector T cells and migrate to the inflamed kidney via a chemokine gradient (4). While most of the effector T cells undergo apoptosis following the resolution of inflammation, a small subset of the effector T cells become TRM cells. TRM cells are the most abundant immune cell population in the healthy human kidney (5). (B) A TRM cell marker CD69 downregulates the expression of S1PR1, which is a receptor required for tissue egress. The expression of transcription factor KLF-2, which upregulates S1PR1 expression, is downregulated in TRM cells (6). CD103 and CD49a are parts of the integrins αEβ7 and α1β1, respectively. CD103 interacts with E-cadherin on epithelial cells, whereas CD49a binds to collagen IV (2, 7). Almost all renal TRM cells express the chemokine receptor CXCR3 and, to a lower degree, CXCR6 (8). Inhibitory receptors, especially PD-1, are commonly expressed on TRM cells compared with circulating memory T cells. IL-15 and TGF-β are required to induce and maintain renal TRM cells in mice (9, 10). Created with BioRender.com
Glomerulonephritis (GN) is a group of immune-mediated kidney diseases, either in the context of systemic or kidney-specific autoimmune disorders, and lead to the damage of the glomeruli and other compartments of the kidney (18). The most severe form is crescentic GN (cGN) or rapid-progressive glomerulonephritis (RPGN), which is mainly observed in autoimmune kidney diseases such as lupus nephritis (LN) and ANCA-associated GN. The histopathological features of cGN are multilayered cells in the Bowman’s capsule due to epithelial hyperplasia (crescents) and infiltration of various immune cells in the periglomerular area (19). Importantly, T cells are one of the most abundant immune cell populations in the inflamed kidney tissue. Most of the renal T cells have a tissue-resident phenotype (17, 20, 21), and the number of tissue-resident T cells correlates with disease severity (17, 22–25). Moreover, recent studies reported that TRM cells might be associated with the development and/or relapse of autoimmune diseases (2, 17, 26). For these reasons, it is of great importance to summarize and discuss the current understanding of renal TRM cells. From the next section, we will shortly explain the current knowledge of TRM cells and then discuss the role of TRM cells in renal autoimmune diseases.
General features of TRM cells
TRM cells are particularly characterized by two features – tissue residency and longevity – which are identified by distinct surface markers and transcriptional profiles (2, 14, 27). Similar to other non-lymphoid organs, tissue-residency of renal TRM cells is mainly assessed by the expression of CD69 and resident-integrins such as CD103 and CD49a, and the downregulation of transcription factor KLF-2, all of which contribute to preventing tissue egress of TRM cells (Figure 1B) (2, 6, 7, 14, 28). The longevity of TRM cells is mainly regulated by a transcriptional network which enables adaptation to a certain tissue environment with a constant supply of cytokines and nutrients. Mice studies showed that IL-15 and TGF-β are important for the development and survival of renal TRM cells after viral infection (9, 10, 29). Regarding nutrition, TRM cells in the skin utilize exogenous free fatty acids (FFA) for oxidative phosphorylation (OXPHOS) to generate ATP (30). Given that the human kidney is a highly hypoxic organ, which might inhibit efficient OXPHOS (31), further tissue-specific analysis is crucial to determine the FFA-dependency of renal TRM cells.
TRM cells can be both protective and pathogenic. In the skin, pathogen- or sterile inflammation-triggered TRM cells show protective properties leading to enhanced pathogen clearance upon antigen re-encounter (32, 33). On the other hand, auto-antigen specific TRM cells can be constantly exposed to self-antigens, contributing to chronic inflammation in autoimmune skin diseases (34). Because of the emerging pathophysiological role in relapsing autoimmune skin diseases, TRM cells have been attracting attention in the field of nephrology as well (2, 17, 26). In human kidneys, the presence of TRM cells specific to systemic virus infections (Epstein-Barr virus, cytomegalovirus, influenza A, and BK virus) has been reported (2, 5, 14), although their functional relevance such as accelerated host defense still needs to be clarified.
TRM cells in lupus nephritis
Systemic lupus erythematosus (SLE) is a systemic and chronic autoimmune disease, which typically manifests in several organs and tissues. The etiology of SLE is still not fully understood, but genetic susceptibility, environmental influences, hormones, and dysregulation of the innate and adaptive immune system have been implicated (35, 36). In SLE, up to 50% of patients develop LN (37). The main pathophysiological driver of renal tissue injury is the loss of self-tolerance, which leads to the production of autoantibodies against nuclear antigens and the accumulation of immune complexes within the glomeruli (38). These immune complexes activate the complement system, inducing a massive tissue infiltration of immune cells.
T cells are the most abundant immune cells in biopsies of patients suffering from LN as well as in lupus-prone mice kidneys (21, 39, 40). T cells localize mainly in the interstitial and periglomerular regions in LN biopsies (39–41). Depletion of infiltrating T cells by antibody injection into MRL/lpr mice significantly reduced renal inflammation and tissue damage, underlining the pathophysiologic role of T cells in LN. Furthermore, T cell infiltration correlates with clinical parameters such as proteinuria, serum creatinine levels, and histopathological grading (22–25).
Flow cytometric analyses of human LN biopsies and MRL/lpr mice revealed that most of the kidney-infiltrating T cells have TRM cell phenotype, such as the expression of CD69 and/or CD103. In addition, the numbers of CD4+ and CD8+ TRM cells in LN kidney are significantly higher compared with healthy control tissue (20, 21). Recent studies using scRNA-seq determined the immune cell composition and transcription profiles of the kidney-infiltrating immune cells during LN (26, 42). The analysis of human LN biopsies revealed the presence of different T cell clusters in the kidney and showed that feature genes of CD8+ TRM cells include ZNF683 (HOBIT), ITGAE, ITGA1, and XCL1 (26).
Renal TRM cells in LN might have enhanced effector functions. CD8+ CD103+ TRM cells from MRL/lpr mice kidneys showed the superior production of cytokines and cytotoxic molecules, such as TNF-α, IFN-γ, perforin, and granzyme B, in comparison to CD103- non-TRM cells (20). However, another study revealed contrasting results, showing an impaired cytokine production in association with the expression of inhibitory receptors, such as PD-1 and Tim3, which are generally observed during T cell exhaustion (21). A scRNA-seq analysis of the murine lupus model showed an exhaustion signature in both CD4+ and CD8+ renal T cells, suggesting that kidney-infiltrating T cells gradually develop T cell exhaustion (42). Trajectory analyses revealed that CD4+ and CD8+ T cells might go through several developmental stages. They first exhibit effector functions during migration to the tissue, and then upregulate hypoxia-inducible genes which finally lead to the T cell exhaustion (42, 43).
T cell clonality analysis is of great importance for better understanding adaptive immunity in health and disease. In autoimmune diseases, autoreactive T cells clonally expand and accumulate in inflamed organs (44–46). TCR ß-chain sequencing combined with laser-capture microdissection from kidney biopsies revealed the predominance of clonally expanded CD4+ and CD8+ T cells in periglomerular regions, suggesting both T cells play roles in the progression of LN (46). In addition, a recent study reported that the frequency of nuclear antigen-reactive CD4+ T cells correlated with disease severity (47). This study revealed the presence of CD4+ T cells specific to canonical nuclear antigens SmD1, RNP70, histone, Ro, and La in patients with LN.
During kidney inflammation, leukocytes including T cells can be found in the urine (48–50). Mass cytometry analysis of urinary T cells from LN patients revealed the expression of CD69, indicating that even T cells with a tissue-resident phenotype can be observed in the urine (50). Furthermore, the amount of T cells in the urine correlates with disease activity as well as severity, thus can be used as a biomarker in LN (49). Interestingly, nuclear antigen-reactive T cells are enriched in the urine of LN patients compared with the blood. This indicates that urinary T cells might, to some extent, reflect T cell clonality in the inflamed kidney tissue during LN (47).
TRM cells in ANCA-associated GN
ANCA-associated vasculitis (AAV) is a group of autoimmune diseases affecting small-sized vessels, encompassing microscopic polyangiitis (MPA), granulomatosis with polyangiitis (GPA), and eosinophilic granulomatosis with polyangiitis (EGPA) (51–53). Similar to SLE, the etiology of AAV is still not fully understood, but generally considered to be the result of multiple factors, including genetic susceptibility (e.g., MHC II genotypes) and environmental factors, such as pathogens and drugs (54–57). All AAV can affect the kidney and induce cGN. Each disease is commonly characterized by auto-antibodies directed against specific antigens, namely myeloperoxidase (MPO) and proteinase 3 (PR3). In general, MPO-ANCA is common in MPA, whereas PR3-ANCA is associated with GPA. Both antigens are proteins located within neutrophils and are unleashed from the cells following activation (53). Upon bacterial infections, neutrophils are activated by pro-inflammatory cytokines as well as direct contact with the pathogen, and initiate neutrophil extracellular traps (NETs) formation. Under physiological circumstances, NETs are cleared in a timely manner by specific enzymes, such as DNase I. In AAV patients, the degradation of NETs is impaired, leading to the persistent NET formation and disrupted tolerance to auto-antigens, such as MPO and PR3, resulting in the production of auto-antibodies MPO- and PR3-ANCA. ANCAs bind to neutrophils, leading to changes in adhesion molecule expression, the release of reactive oxygen species, cytokines, and lytic enzymes, and eventually induction of necrotizing vasculitis (58).
In ANCA-associated GN, the accumulation of T cells in the inflamed kidney tissue is observed (59). The number of CD4+ and CD8+ T cells correlates with kidney function. Furthermore, in the glomeruli with segmental necrosis and cellular crescents, active glomerular lesions correlate with intraglomerular T cells (59). In AAV patients, MPO- and PR3-specific CD4+ T cells were identified, showing a decreased TCR diversity and clonal expansion (55–57). These autoreactive T cells show memory T cell phenotype and secrete proinflammatory cytokines including IL-17A (55, 60).
A scRNA-seq analysis of T cells from ANCA-associated GN biopsies showed a variety of T cell populations in the inflamed kidney tissue. Those T cells include CD4+ TRM cells with different transcriptome profiles, such as type1 or typ3 immunity-associated gene expression (17). The number of CD69+ TRM cells was significantly higher in ANCA-associated GN biopsies compared to healthy renal tissue, indicating the recruitment of autoreactive T cells into the kidneys of patients (17). As observed in LN, the abundance of TRM cells positively correlated with disease activity. The TRM cells from ANCA-associated GN showed an upregulation of genes involved in T cell proliferation, activation, and cytokine signaling compared with healthy control (17).
While antigen-specific immunity is the central function of T cells, TCR-independent activation (bystander T cell activation) has also been reported (61). Upon activation by cytokines, bystander T cells can immediately secrete effector cytokines in the absence of antigen stimulation. This finding suggests that the induction of renal TRM cells can be harmful to the kidney tissue regardless of the antigen-specificity. In mice studies, systemic infection of Staphylococcus aureus or Candida albicans induced renal CD4+ TRM cells including TRM17 cells, which secrete IL-17A upon reactivation. The presence of TRM cells significantly aggravated the progression of experimental GN, indicating that bystander TRM cells contributed to GN independent of TCR signaling. In vitro study revealed that TRM cells can be activated in the presence of a cytokine cocktail of IL-23, IL-6, and IL-1β (17). Therefore, future analysis should focus on not only autoreactive T cells but also activated bystander T cells in autoimmune kidney diseases.
Treatment strategies for renal autoimmune diseases
After diagnosing kidney involvement in systemic autoimmune diseases such as SLE and AAV, therapeutic intervention is needed to prevent progressive kidney injury. In general, therapy can be divided into two phases – induction therapy to rapidly suppress inflammation and maintenance therapy to prevent relapse. Current treatment strategies are largely based on systemic immunosuppressants (Table 1) (62, 63).
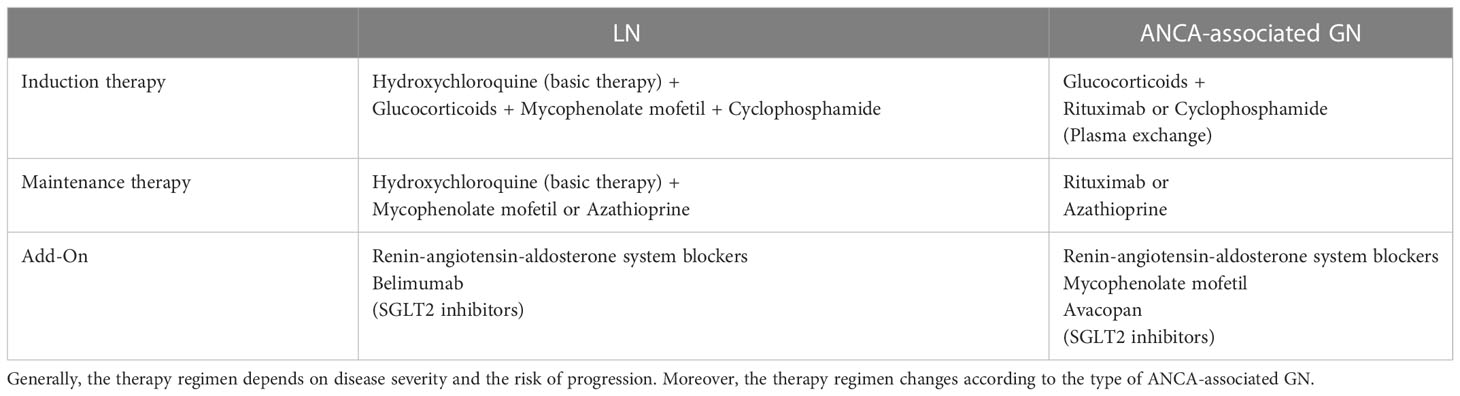
Table 1 Simplified treatment strategies in LN and ANCA-associated GN (esp. active, severe GPA/MPA) (62, 63).
Some therapeutic strategies for LN or ANCA-associated GN target B cells and/or plasma cells. The number of autoantibody-producing cells can be reduced by using rituximab, belimumab, or daratumumab, which are monoclonal antibodies against CD20, B-cell activating factor (BAFF), or CD38, respectively (63–65). In ANCA-associated GN, the complement pathway can also be targeted. Avacopan, an orally available C5a receptor antagonist which blocks neutrophil chemoattraction and activation, is noninferior to prednisone taper with respect to remission and shows better eGFR recovery (3). Despite the therapeutic progress, efficient treatment strategy targeting T cells has not been established.
Recent studies suggested that TRM cells can be targeted by diverse strategies. Firstly, TRM cell-specific metabolic pathways can be modulated. TRM cells require exogenous FFA uptake for their survival and function, and T cell-specific deletion of FABP4/5 impairs the development and function of TRM cells (30). Therefore, inhibiting FFA uptake can be an efficient treatment strategy targeting TRM cells. However, FFA-dependency and general metabolic pathways of renal TRM cells still need to be determined. Secondly, cytokines essential for long-term survival of TRM cells, e.g., IL-15, can be targeted to reduce the cells (66). CD122, which is a subunit of IL-15 and IL-2 receptor, is expressed by TRM cells as well as effector T cells (67). In vitiligo, an autoimmune disease affecting the skin, the number of TRM cells was successfully reduced by an anti-CD122 antibody therapy (67). Short-term treatment with the anti-CD122 therapy inhibited the production of cytokine by TRM cells, and long-term treatment depleted TRM cells from skin lesions. Given CD122 is expressed by TRM cells in different organs, the anti-CD122 therapy might be effective for renal TRM cells as well. Moreover, downstream of IL-15 and CD122, such as JAK-STAT pathways, can be blocked. Tofacitinib, an inhibitor of JAK1 and JAK3, impaired the survival of renal TRM cells, contributing to improved kidney function in MRL/lpr lupus mice model (20). Thirdly, autoreactive T cell-specific intervention might be possible. Diverse methods are currently under investigation to induce antigen-specific immune tolerance, and the methods include autoantigen-coated microparticles, autoantigen peptide-MHC multimers, and antigen-specific regulatory T cell transfer (68). In murine models of type I diabetes, multiple sclerosis and arthritis, disease-relevant peptide-MHC class II-nanoparticles successfully reversed established disease in association with systemic expansions of cognate CD4+ T cells displaying a type 1 regulatory T cell phenotype (68). Importantly, these peptide-MHC-based nanomedicines could suppress autoimmune diseases without suppressing systemic immunity. In order to develop TRM cell-targeting therapeutic strategies, further studies should focus on characterizing renal TRM cells and microenvironments in different disease conditions.
Conclusion
The discovery of TRM cells has significantly improved our understanding of immunology. Several studies analyzing animal models and patients with renal autoimmune diseases successfully identified CD4+ and CD8+ TRM cell populations in the kidney and showed potential clinical relevance. Autoreactive TRM cells can be clonally expanded due to self-antigen exposure, contributing to autoimmune kidney diseases (44–46). Of interest, TRM cells induced by infections also stay in the kidney and can be activated during autoimmunity (bystander T cell activation) promoting tissue damage (17). Better characterization of TRM cells and microenvironment in renal autoimmune diseases might lead to the development of TRM cell-specific therapeutic strategies.
Author contributions
PG wrote a draft. NA and UP supervised and finalized the manuscript. All authors contributed to the article and approved the submitted version.
Conflict of interest
The authors declare that the research was conducted in the absence of any commercial or financial relationships that could be construed as a potential conflict of interest.
Publisher’s note
All claims expressed in this article are solely those of the authors and do not necessarily represent those of their affiliated organizations, or those of the publisher, the editors and the reviewers. Any product that may be evaluated in this article, or claim that may be made by its manufacturer, is not guaranteed or endorsed by the publisher.
References
1. Schenkel JM, Masopust D. Tissue-resident memory T cells. Immunity (2014) 41(6):886–97. doi: 10.1016/j.immuni.2014.12.007
2. Asada N, Ginsberg P, Gagliani N, Mittrücker H-W, Panzer U. Tissue-resident memory T cells in the kidney. Semin Immunopathology (2022) 44(6):801–11. doi: 10.1007/s00281-022-00927-7
3. Jayne DRW, Merkel PA, Schall TJ, Bekker P. Avacopan for the treatment of ANCA-associated vasculitis. N Engl J Med (2021) 384(7):599–609. doi: 10.1056/NEJMoa2023386
4. Banchereau J, Steinman RM. Dendritic cells and the control of immunity. Nature (1998) 392(6673):245–52. doi: 10.1038/32588
5. Dornieden T, Sattler A, Pascual-Reguant A, Ruhm AH, Thiel LG, Bergmann YS, et al. Signatures and specificity of tissue-resident lymphocytes identified in human renal peritumor and tumor tissue. J Am Soc Nephrol (2021) 32(9):2223–41. doi: 10.1681/ASN.2020101528
6. Mackay LK, Braun A, Macleod BL, Collins N, Tebartz C, Bedoui S, et al. Cutting edge: CD69 interference with sphingosine-1-phosphate receptor function regulates peripheral T cell retention. J Immunol (2015) 194(5):2059–63. doi: 10.4049/jimmunol.1402256
7. Xu W, Bergsbaken T, Edelblum KL. The multifunctional nature of CD103 (αEβ7 integrin) signaling in tissue-resident lymphocytes. Am J Physiol Cell Physiol (2022) 323(4):C1161–c1167. doi: 10.1152/ajpcell.00338.2022
8. van der Putten C, Remmerswaal EBM, Terpstra ML, van der Bom ND, Kers J, Ten Berge IJM, et al. CD8 and CD4 T cell populations in human kidneys. Cells (2021) 10(2):288. doi: 10.3390/cells10020288
9. Ma C, Mishra S, Demel EL, Liu Y, Zhang N. TGF-beta controls the formation of kidney-resident T cells via promoting effector T cell extravasation. J Immunol (2017) 198(2):749–56. doi: 10.4049/jimmunol.1601500
10. Schenkel JM, Fraser KA, Casey KA, Beura LK, Pauken KE, Vezys V, et al. IL-15-Independent maintenance of tissue-resident and boosted effector memory CD8 T cells. J Immunol (2016) 196(9):3920–6. doi: 10.4049/jimmunol.1502337
11. Flores-Mireles AL, Walker JN, Caparon M, Hultgren SJ. Urinary tract infections: epidemiology, mechanisms of infection and treatment options. Nat Rev Microbiol (2015) 13(5):269–84. doi: 10.1038/nrmicro3432
12. Fogo AB, Lusco MA, Najafian B, Alpers CE. AJKD atlas of renal pathology: Cytomegalovirus infection. Am J Kidney Dis (2016) 68(6):e35–6. doi: 10.1053/j.ajkd.2016.10.008
13. Badiee P, Hashemizadeh Z. Opportunistic invasive fungal infections: diagnosis & clinical management. Indian J Med Res (2014) 139(2):195–204.
14. Parga-Vidal L, van Aalderen MC, Stark R, van Gisbergen K. Tissue-resident memory T cells in the urogenital tract. Nat Rev Nephrol (2022) 18(4):209–23. doi: 10.1038/s41581-021-00525-0
15. Stewart BJ, Ferdinand JR, Young MD, Mitchell TJ, Loudon KW, Riding AM, et al. Spatiotemporal immune zonation of the human kidney. Science (2019) 365(6460):1461–6. doi: 10.1126/science.aat5031
16. Park JG, Na M, Kim MG, Park SH, Lee HJ, Kim DK, et al. Immune cell composition in normal human kidneys. Sci Rep (2020) 10(1):15678. doi: 10.1038/s41598-020-72821-x
17. Krebs CF, Reimers D, Zhao Y, Paust HJ, Bartsch P, Nuñez S, et al. Pathogen-induced tissue-resident memory T(H)17 (T(RM)17) cells amplify autoimmune kidney disease. Sci Immunol (2020) 5(50):eaba4163. doi: 10.1126/sciimmunol.aba4163
18. Linke A, Tiegs G, Neumann K. Pathogenic T-cell responses in immune-mediated glomerulonephritis. Cells (2022) 11(10):1625. doi: 10.3390/cells11101625
19. Smeets B, Uhlig S, Fuss A, Mooren F, Wetzels JF, Floege J, et al. Tracing the origin of glomerular extracapillary lesions from parietal epithelial cells. J Am Soc Nephrol (2009) 20(12):2604–15. doi: 10.1681/ASN.2009010122
20. Zhou M, Guo C, Li X, Huang Y, Li M, Zhang T, et al. JAK/STAT signaling controls the fate of CD8(+)CD103(+) tissue-resident memory T cell in lupus nephritis. J Autoimmun (2020) 109:102424. doi: 10.1016/j.jaut.2020.102424
21. Tilstra JS, Avery L, Menk AV, Gordon RA, Smita S, Kane LP, et al. Kidney-infiltrating T cells in murine lupus nephritis are metabolically and functionally exhausted. J Clin Invest (2018) 128(11):4884–97. doi: 10.1172/JCI120859
22. Jabs DA, Burek CL, Hu Q, Kuppers RC, Lee B, Prendergast RA. Anti-CD4 monoclonal antibody therapy suppresses autoimmune disease in MRL/Mp-lpr/lpr mice. Cell Immunol (1992) 141(2):496–507. doi: 10.1016/0008-8749(92)90166-M
23. Jevnikar AM, Grusby MJ, Glimcher LH. Prevention of nephritis in major histocompatibility complex class II-deficient MRL-lpr mice. J Exp Med (1994) 179(4):1137–43. doi: 10.1084/jem.179.4.1137
24. Merino R, Fossati L, Iwamoto M, Takahashi S, Lemoine R, Ibnou-Zekri N, et al. Effect of long-term anti-CD4 or anti-CD8 treatment on the development of lpr CD4- CD8- double negative T cells and of the autoimmune syndrome in MRL-lpr/lpr mice. J Autoimmun (1995) 8(1):33–45. doi: 10.1006/jaut.1995.0003
25. Suárez-Fueyo A, Bradley SJ, Tsokos GC. T Cells in systemic lupus erythematosus. Curr Opin Immunol (2016) 43:32–8. doi: 10.1016/j.coi.2016.09.001
26. Arazi A, Rao DA, Berthier CC, Davidson A, Liu Y, Hoover PJ, et al. The immune cell landscape in kidneys of patients with lupus nephritis. Nat Immunol (2019) 20(7):902–14. doi: 10.1038/s41590-019-0398-x
27. Mueller SN, Mackay LK. Tissue-resident memory T cells: local specialists in immune defence. Nat Rev Immunol (2016) 16(2):79–89. doi: 10.1038/nri.2015.3
28. Carlson CM, Endrizzi BT, Wu J, Ding X, Weinreich MA, Walsh ER, et al. Kruppel-like factor 2 regulates thymocyte and T-cell migration. Nature (2006) 442(7100):299–302. doi: 10.1038/nature04882
29. Mackay LK, Rahimpour A, Ma JZ, Collins N, Stock AT, Hafon ML, et al. The developmental pathway for CD103(+)CD8+ tissue-resident memory T cells of skin. Nat Immunol (2013) 14(12):1294–301. doi: 10.1038/ni.2744
30. Pan Y, Tian T, Park CO, Lofftus SY, Mei S, Liu X, et al. Survival of tissue-resident memory T cells requires exogenous lipid uptake and metabolism. Nature (2017) 543(7644):252–6. doi: 10.1038/nature21379
31. Taylor CT, Scholz CC. The effect of HIF on metabolism and immunity. Nat Rev Nephrol (2022) 18(9):573–87. doi: 10.1038/s41581-022-00587-8
32. Mackay LK, Stock AT, Ma JZ, Jones CM, Kent SJ, Mueller SN, et al. Long-lived epithelial immunity by tissue-resident memory T (TRM) cells in the absence of persisting local antigen presentation. Proc Natl Acad Sci U.S.A. (2012) 109(18):7037–42. doi: 10.1073/pnas.1202288109
33. Gebhardt T, Wakim LM, Eidsmo L, Reading PC, Heath WR, Carbone FR. Memory T cells in nonlymphoid tissue that provide enhanced local immunity during infection with herpes simplex virus. Nat Immunol (2009) 10(5):524–30. doi: 10.1038/ni.1718
34. Owczarczyk Saczonek A, Krajewska-Włodarczyk M, Kasprowicz-Furmańczyk M, Placek W. Immunological memory of psoriatic lesions. Int J Mol Sci (2020) 21(2):625. doi: 10.3390/ijms21020625
35. Lisnevskaia L, Murphy G, Isenberg D. Systemic lupus erythematosus. Lancet (9957) 2014:1878–88:384. doi: 10.1016/S0140-6736(14)60128-8
36. Tsokos GC. Systemic lupus erythematosus. N Engl J Med (2011) 365(22):2110–21. doi: 10.1056/NEJMra1100359
37. Parikh SV, Almaani S, Brodsky S, Rovin BH. Update on lupus nephritis: Core curriculum 2020. Am J Kidney Dis (2020) 76(2):265–81. doi: 10.1053/j.ajkd.2019.10.017
38. Lech M, Anders HJ. The pathogenesis of lupus nephritis. J Am Soc Nephrol (2013) 24(9):1357–66. doi: 10.1681/ASN.2013010026
39. Alexopoulos E, Seron D, Hartley RB, Cameron JS. Lupus nephritis: correlation of interstitial cells with glomerular function. Kidney Int (1990) 37(1):100–9. doi: 10.1038/ki.1990.14
40. Couzi L, Merville P, Deminière C, Moreau JF, Combe C, Pellegrin JL, et al. Predominance of CD8+ T lymphocytes among periglomerular infiltrating cells and link to the prognosis of class III and class IV lupus nephritis. Arthritis Rheum (2007) 56(7):2362–70. doi: 10.1002/art.22654
41. Zhang T, Wang M, Zhang J, Feng X, Liu Z, Cheng Z. Association between tubulointerstitial CD8+T cells and renal prognosis in lupus nephritis. Int Immunopharmacol (2021) 99:107877. doi: 10.1016/j.intimp.2021.107877
42. Smita S, Chikina M, Shlomchik MJ, Tilstra JS. Heterogeneity and clonality of kidney-infiltrating T cells in murine lupus nephritis. JCI Insight (2022) 7(8):e156048. doi: 10.1172/jci.insight.156048
43. Sugahara M, Tanaka T, Nangaku M. Hypoxia-inducible factor and oxygen biology in the kidney. Kidney360 (2020) 1(9):1021–31. doi: 10.34067/KID.0001302020
44. Murata H, Matsumura R, Koyama A, Sugiyama T, Sueishi M, Shibuya K, et al. T Cell receptor repertoire of T cells in the kidneys of patients with lupus nephritis. Arthritis Rheum (2002) 46(8):2141–7. doi: 10.1002/art.10432
45. Massengill SF, Goodenow MM, Sleasman JW. SLE nephritis is associated with an oligoclonal expansion of intrarenal T cells. Am J Kidney Dis (1998) 31(3):418–26. doi: 10.1053/ajkd.1998.v31.pm9506678
46. Winchester R, Wiesendanger M, Zhang HZ, Steshenko V, Peterson K, Geraldino-Pardilla L, et al. Immunologic characteristics of intrarenal T cells: trafficking of expanded CD8+ T cell beta-chain clonotypes in progressive lupus nephritis. Arthritis Rheum (2012) 64(5):1589–600. doi: 10.1002/art.33488
47. Abdirama D, Tesch S, Grießbach AS, Spee-Mayer von C, Humrich JY, Stervbo U, et al. Nuclear antigen-reactive CD4(+) T cells expand in active systemic lupus erythematosus, produce effector cytokines, and invade the kidneys. Kidney Int (2021) 99(1):238–46. doi: 10.1016/j.kint.2020.05.051
48. Dolff S, Abdulahad WH, Dijk van MC, Limburg PC, Kallenberg CG, Bijl M. Urinary T cells in active lupus nephritis show an effector memory phenotype. Ann Rheum Dis (2010) 69(11):2034–41. doi: 10.1136/ard.2009.124636
49. Enghard P, Rieder C, Kopetschke K, Klocke JR, Undeutsch R, Biesen R, et al. Urinary CD4 T cells identify SLE patients with proliferative lupus nephritis and can be used to monitor treatment response. Ann Rheum Dis (2014) 73(1):277–83. doi: 10.1136/annrheumdis-2012-202784
50. Bertolo M, Baumgart S, Durek P, Peddinghaus A, Mei H, Rose T, et al. Deep phenotyping of urinary leukocytes by mass cytometry reveals a leukocyte signature for early and non-invasive prediction of response to treatment in active lupus nephritis. Front Immunol (2020) 11:256. doi: 10.3389/fimmu.2020.00256
51. Geetha D, Jefferson JA. ANCA-associated vasculitis: Core curriculum 2020. Am J Kidney Dis (2020) 75(1):124–37. doi: 10.1053/j.ajkd.2019.04.031
52. Jennette JC, Falk RJ, Bacon PA, Basu N, Cid MC, Ferrario F, et al. 2012 revised international chapel hill consensus conference nomenclature of vasculitides. Arthritis Rheum (2013) 65(1):1–11. doi: 10.1002/art.37715
53. Kitching AR, Anders HJ, Basu N, Brouwer E, Gordon J, Jayne DR, et al. ANCA-associated vasculitis. Nat Rev Dis Primers (2020) 6(1):71. doi: 10.1038/s41572-020-0204-y
54. Merkel PA, Xie G, Monach PA, Ji X, Ciavatta DJ, Byun J, et al. Identification of functional and expression polymorphisms associated with risk for antineutrophil cytoplasmic autoantibody-associated vasculitis. Arthritis Rheumatol (2017) 69(5):1054–66. doi: 10.1002/art.40034
55. Free ME, Stember KG, Hess JJ, McInnis EA, Lardinois O, Hogan SL, et al. Restricted myeloperoxidase epitopes drive the adaptive immune response in MPO-ANCA vasculitis. J Autoimmun (2020) 106:102306. doi: 10.1016/j.jaut.2019.102306
56. Chen DP, McInnis EA, Wu EY, Stember KG, Hogan SL, Hu Y, et al. Immunological interaction of HLA-DPB1 and proteinase 3 in ANCA vasculitis is associated with clinical disease activity. J Am Soc Nephrol (2022) 33(8):1517–27. doi: 10.1681/ASN.2021081142
57. Shochet L, Kitching AR. Identifying antigen-specific T cells in ANCA-associated vasculitis: A glimpse of the future? J Am Soc Nephrol (2022) 33(8):1435–7. doi: 10.1681/ASN.2022060668
58. Nakazawa D, Masuda S, Tomaru U, Ishizu A. Pathogenesis and therapeutic interventions for ANCA-associated vasculitis. Nat Rev Rheumatol (2019) 15(2):91–101. doi: 10.1038/s41584-018-0145-y
59. O’Sullivan KM, Lo CY, Summers SA, Elgass KD, McMillan PJ, Longano A, et al. Renal participation of myeloperoxidase in antineutrophil cytoplasmic antibody (ANCA)-associated glomerulonephritis. Kidney Int (2015) 88(5):1030–46. doi: 10.1038/ki.2015.202
60. Disteldorf EM, Krebs CF, Paust HJ, Turner JE, Nouailles G, Tittel A, et al. CXCL5 drives neutrophil recruitment in TH17-mediated GN. J Am Soc Nephrol (2015) 26(1):55–66. doi: 10.1681/ASN.2013101061
61. Lee HG, Cho MZ, Choi JM. Bystander CD4(+) T cells: crossroads between innate and adaptive immunity. Exp Mol Med (2020) 52(8):1255–63. doi: 10.1038/s12276-020-00486-7
62. Fanouriakis A, Kostopoulou M, Cheema K, Anders HJ, Aringer M, Bajema I, et al. 2019 update of the joint European league against rheumatism and European renal association-European dialysis and transplant association (EULAR/ERA-EDTA) recommendations for the management of lupus nephritis. Ann Rheum Dis (2020) 79(6):713–23. doi: 10.1136/annrheumdis-2020-216924
63. Chung SA, Langford CA, Maz M, Abril A, Gorelik M, Guyatt G, et al. 2021 American college of Rheumatology/Vasculitis foundation guideline for the management of antineutrophil cytoplasmic antibody-associated vasculitis. Arthritis Rheumatol (2021) 73(8):1366–83. doi: 10.1002/art.41773
64. Furie R, Rovin BH, Houssiau F, Malvar A, Teng YKO, Contreras G, et al. Two-year, randomized, controlled trial of belimumab in lupus nephritis. N Engl J Med (2020) 383(12):1117–28. doi: 10.1056/NEJMoa2001180
65. Ostendorf L, Burns M, Durek P, Heinz GA, Heinrich F, Garantziotis P, et al. Targeting CD38 with daratumumab in refractory systemic lupus erythematosus. N Engl J Med (2020) 383(12):1149–55. doi: 10.1056/NEJMoa2023325
66. van der Gracht ETI, Behr FM, Arens R. Functional heterogeneity and therapeutic targeting of tissue-resident memory T cells. Cells (2021) 10(1):164. doi: 10.3390/cells10010164
67. Richmond JM, Strassner JP, Zapata L Jr, Garg M, Riding RL, Refat MA, et al. Antibody blockade of IL-15 signaling has the potential to durably reverse vitiligo. Sci Transl Med (2018) 10(450). doi: 10.1126/scitranslmed.aam7710
Keywords: tissue-resident memory T cell, renal autoimmune disease, crescentic glomerulonephritis, lupus nephritis, ANCA-associated glomerulonephritis
Citation: Ginsberg P, Panzer U and Asada N (2023) Tissue-resident memory T cells in renal autoimmune diseases. Front. Immunol. 14:1111521. doi: 10.3389/fimmu.2023.1111521
Received: 29 November 2022; Accepted: 10 January 2023;
Published: 23 January 2023.
Edited by:
Markus Uhrberg, Heinrich Heine University of Düsseldorf, GermanyReviewed by:
Geoffrey Camirand, University of Pittsburgh, United StatesCopyright © 2023 Ginsberg, Panzer and Asada. This is an open-access article distributed under the terms of the Creative Commons Attribution License (CC BY). The use, distribution or reproduction in other forums is permitted, provided the original author(s) and the copyright owner(s) are credited and that the original publication in this journal is cited, in accordance with accepted academic practice. No use, distribution or reproduction is permitted which does not comply with these terms.
*Correspondence: Nariaki Asada, bi5hc2FkYUB1a2UuZGU=