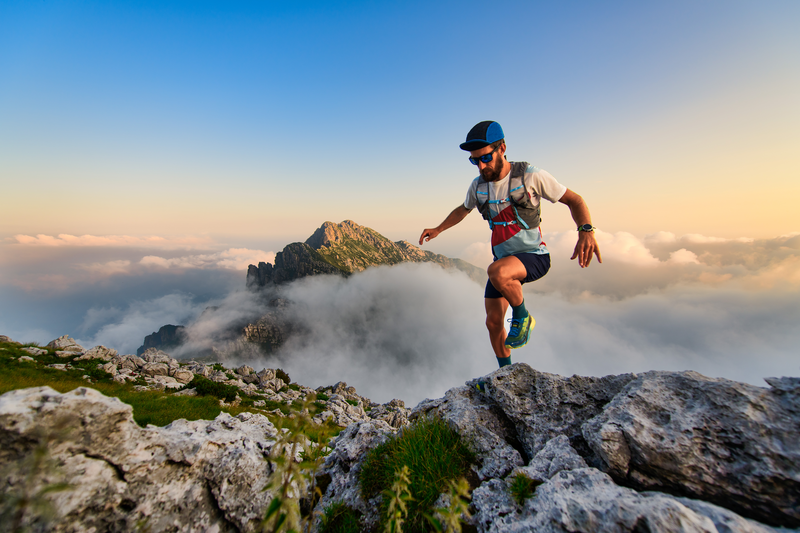
95% of researchers rate our articles as excellent or good
Learn more about the work of our research integrity team to safeguard the quality of each article we publish.
Find out more
ORIGINAL RESEARCH article
Front. Immunol. , 01 March 2023
Sec. Comparative Immunology
Volume 14 - 2023 | https://doi.org/10.3389/fimmu.2023.1110696
This article is part of the Research Topic Aquatic Nutrition and Intestine Immunity View all 18 articles
Introduction: In an effort to minimize the usage of fishmeal in aquaculture, novel protein diets, including Tenebrio molitor, cottonseed protein concentrate, Clostridium autoethanogenum, and Chlorella vulgaris were evaluated for their potential to replace fishmeal. Nevertheless, comprehensive examinations on the gut health of aquatic animals under an alternate feeding strategy when fed novel protein diets are vacant.
Methods: Five isonitrogenous and isolipidic diets containing various proteins were manufactured, with a diet consisting of whole fishmeal serving as the control and diets containing novel proteins serving as the experimental diets. Largemouth bass (Micropterus salmoides) with an initial body weight of 4.73 ± 0.04g employed as an experimental animal and given these five diets for the first 29 days followed by a fishmeal diet for the next 29 days.
Results: The results of this study demonstrated that the growth performance of novel protein diets in the second stage was better than in the first stage, even though only the C. vulgaris diet increased antioxidant capacity and the cottonseed protein concentrate diet decreased it. Concerning the intestinal barriers, the C. autoethanogenum diet lowered intestinal permeability and plasma IL-1β/TNF-α. In addition, the contents of intestinal immunological factors, namely LYS and sIgA-like, were greater in C. vulgaris than in fishmeal. From the data analysis of microbiome and metabolome, the levels of short chain fatty acids (SCFAs), anaerobic bacteria, Lactococcus, and Firmicutes were significantly higher in the C. autoethanogenum diet than in the whole fishmeal diet, while the abundance of Pseudomonas, aerobic bacteria, Streptococcus, and Proteobacteria was lowest. However, no extremely large differences in microbiota or short chain fatty acids were observed between the other novel protein diets and the whole fishmeal diet. In addition, the microbiota were strongly connected with intestinal SCFAs, lipase activity, and tight junctions, as shown by the Mantel test and Pearson’s correlation.
Discussion: Taken together, according to Z-score, the ranking of advantageous functions among these protein diets was C. autoethanogenum diet > C. vulgaris diet > whole fishmeal diet > cottonseed protein concentrate > T. molitor diet. This study provides comprehensive data illustrating a mixed blessing effect of novel protein diets on the gut health of juvenile largemouth bass under an alternate feeding strategy.
Aquaculture provides humans with enormous amounts of high-quality proteins. Both oceanic and freshwater aquaculture need vast quantities of fishmeal, a high-quality protein for aquaculture (1). However, fishmeal is unsustainable, from 2020 FAO report, the yearly output of fishmeal in the next decade may rise by no more than 1% compared to 2018, while the price of fishmeal will climb by 30%, putting a significant strain on diets prices and worldwide fishmeal stock capacity (2). Consequently, it is crucial to explore high-quality fishmeal substitutes in aquatic practices.
Chlorella vulgaris diet, Clostridium autoethanogenum protein diet, cottonseed protein concentrate diet, and Tenebrio molitor diet are developed to ease the shortage of fishmeal in feed industry. In recent years, researchers have concentrated on these novel proteins owing to their abundant fat/fatty acids, low price, high content of protein, mineral, balanced amino acid profiles, and vitamin content (3–6). Earlier studies have examined the impacts of these proteins on Cyprinus carpio var. specularis, Litopenaeus vannamei, and Micropterus salmoides (4, 7–9). Nonetheless, these studied mainly focus on partial substitution strategy of fishmeal, and explore their functions on muscle quality, growth performance and intestinal health. Few studies have been carried out to investigate in depth the impacts of novel proteins on fish growth performance and intestinal immunity from the perspective of alternate feeding strategy while comparing the relative substitution potential of each novel protein diet.
Alternate feeding strategy is an effective method to conserve high-value or resource-limited feed ingredients. Some studies have extensively conducted for fish oil based diet substitution with terrestrially derived oil or plant-sourced oil diets without compromising fish fatty acid composition (10), fish growth performance (11), or their physio-biochemical performance (12, 13) in Scophthalmus maximus, Gadus morhua, Dicentrarchus labrax, and Acanthopagrus schlegelii. These studies showed that using an alternate feeding strategy could reduce the use of fish oil. However, few studies have focused on replacing fishmeal with novel proteins under an alternate feeding strategy. The intestine is the primary location for the absorption and digestion of fish nutrients, and its health is favorable to the correct execution of fish physiological processes (14). It is commonly accepted that the intestinal barriers include of immunological, microbial, physical, and chemical barriers (15), which play key roles in fish growth, nutritional status, immunology, and resistance to illness.
Largemouth bass (M. salmoides), which is one of the most cultivated species in China, was selected as a research model owing to its high fishmeal consumption in commercial diets, which ranges between 35 and 50 percent (16). In order to explore novel protein diets that might potentially replace fishmeal in fish diets, this research aims to evaluate the effects of novel protein diets on the gut health and growth performance of juvenile largemouth bass under an alternate feeding approach. Compared to previously published research on these proteins, the present study displayed a comprehensive view of the interaction between novel proteins and gut health and growth performance, with a focus on the connection between gut microbiota, their metabolites, and physiochemistry biomarkers under an alternate feeding strategy, as illustrated in Figure 1.
Figure 1 The work flow of this study. Whole fishmeal diet, C. vulgaris diet, cottonseed protein concentrate, C. autoethanogenum diet, and T. molitor diet, referred as FM/FM, ChM/FM, CSM/FM, CAP/FM, and TM/FM, respectively.
The methodologies employed in this research properly adhered to the management Rule of Laboratory Animals (Chinese order no. 676 of the State Council, revised 1 March, 2017). The largemouth bass were provided by Hubei Zhenghao Fish Fry farm. Cottonseed protein concentrate, Fishmeal, C. autoethanogenum, T. molitor and C. vulgaris were formulated to five isonitrogenous and isolipidic expanded pellet diets. The approximate composition was shown in Supplementary Table 1. Each feed was molded into 2 mm floating pellets and air-dried for two days at room temperature. All experimental diets were kept at a temperature of -20°C. Anterior to the start of the growth trial, the five diets were pooled and supplied to juvenile largemouth bass for 21 days to prevent the unpalatability and to acclimatize them to the experimental surroundings. Five groups of largemouth bass (starting body weight: 4.73 ± 0.04g) with three replicates per treatment were allocated to 15 blue cylindrical acrylic tanks (Water volume: 400 L) at random, containing 35 fish per tank in a recirculation system. The experiment consisted of two stages, in which fish were initially given novel proteins for 29 days, then fishmeal for another 29 days, and all were fed twice daily at 9:00-9:30 am and 5:00-5:30 pm until a level of apparent satiety. The bulk body weight of fish was recorded every week. Throughout the duration of the growth trial, about 1/7 of the water was replenished every day to remove faeces buildup. During the growth trial, the water quality was maintained as follows: 27-30°C water temperature, ammonia-N<0.2 mg L-1, nitrite-N<0.025 mg L-1, and dissolved oxygen>5.0 mg L-1, pH at approximately 7.5, and 10L:14D photoperiod with illumination from 8:00 am to 6:00 pm.
In order to examine the gut microbiota and short chain fatty acids (SCFAs), seven fish from each treatment were randomly taken after a 6-hour fast on day 58 and anesthesia with 75 mg L-1 MS-222 (Aladdin, Shanghai, China). The method for sacrificing fish was previously described (17). The entire contents of the intestine were collected in sterile centrifuge tubes, which were immediately frozen in liquid nitrogen and stored at -80°C. Three samples from each treatment, with similar bacterial structures and compositions, were analyzed for their SCFAs contents. On day 58, additional fish were starved for 24 hours, anesthetized with the same amount of MS-222, slaughtered, and dissected as described above. The middle intestine of nine fish from each treatment was rapidly frozen in liquid nitrogen and stored at -80°C for investigation of intestinal biochemical indexes and associated gene expression. For morphological study, the distal intestines of nine fish from each treatment group were preserved in 4% paraformaldehyde. For intestinal permeability investigations, serum was taken from nine fish per treatment, allowed to rest at 4°C for overnight, and the supernatant was collected. The initial body weight (IBW), final body weight (FBW), average daily gain (ADC), Feed conversion ratio (FCR), protein efficiency ratio (PER), specific growth rate (SGR), and survival rate (SR) were calculated using the following formulae:
The intestinal tissue slice slides were made by Service bio-Company (Wuhan, China). Briefly, the middle gut was washed in sterile saline, fixed for 48 hours in 4% paraformaldehyde, and then rinsed in 70% ethanol. 5μm thick slices of paraffin-embedded, aematoxylin-eosin-stained intestines. Using an Olympus DP72 microscope paired with a Nikon E600 camera and Olympus CellSens Standard software to acquire images. At 10 sites on a single slide, the height/width of each villi, the muscle layer thickness and the number of goblet cells, were assessed. In addition, in order to eliminate the effects from body length, the quantification results from morphology were calibrated. Using commercial Elisa kits, the serum concentrations of lipopolysaccharide (LPS), lysozyme (LYS), Interleukin 1 beta (IL-1β), and tumor necrosis factor (TNF-α) were measured (Enzyme-linked Biotechnology, Shanghai, China). The required operation was conducted in accordance with the instructions.
Approximately 100 mg of intestinal tissue was mixed with cold saline to generate a 10% homogenate (Tissue: Saline=1:9). After centrifugation (4500 rpm/min) for 15 minutes, the supernatant was collected and analyzed. Employing commercial test kits (Nanjing jiancheng Bioengineering Institute, Nanjing, China), the activities of superoxide dismutase (SOD), catalase (CAT), and malondialdehyde (MDA) were determined. Utilizing commercial Elisa kits, the level of glutathione peroxidase (GSH-Px) and mucin 2 (MUC2), lysozyme (LYS), immunoglobulin M (IgM), immunoglobulin G-like (IgG-like), and secretory immunoglobulin-like (sIgA-like) were determined (Enzyme-linked Biotechnology, Shanghai, China). The required operation was conducted in conformity with the instructions.
RT-qPCR was conducted as previously described (17). Briefly, total RNA of middle intestine was extracted, and RNA templates were used to transcribe cDNAs. The cDNAs were amplified by PCR using the PrimeScript RT reagent Kit (Takara, Japan). With the primers provided in Supplementary Table 2, the RT-qPCR was conducted on a CFX96™ Real-Time System (bio-RAD, USA). β-actin was chosen as a housekeeping gene due to its stable expression. The primers, i.e., occludin, claudin-1, zo-1, claudin-4, sod, cat, gpx, keap-1, and nrf-2, were selected and exhibited amplification efficiencies ranging from 91% to 115%. The results of real-time RT-qPCR were analyzed by the 2−ΔΔCT method.
Following the manufacturer’s instructions, total bacterial DNA was extracted from the intestinal contents using an OMEGA DNA Kit (D5625-01) (Omega Bio-Tek, Norcross, GA, USA). PCR amplification and Illumina MiSeq sequencing (Shanghai, China) were used to study the V3-V4 region of the bacterial 16S rRNA gene. Refer to the additional materials for more information.
The detection of intestinal contents short chain fatty acids was accomplished by the previously described technique of gas chromatography-mass spectrometry (GC-MS) (17). See supplementary materials for details.
SPSS 25.0 (IBM, Armonk, NY, USA) were used to analyze the data. For data not normally distributed, the nonparametric Krustal-Wallis test followed by the Dunn’s multiple comparison test was applied. One-way ANOVA was used to evaluate normally distributed data, following with least significant differences for multiple treatments comparisons. P<0.05 was considered statistical differences. Illustrations were created using R 3.6.1 ggplot2 package as well as GraphPad Prism 8.3.0. In addition, we supported the idea that statistical differences should not serve as the exclusive criteria for assessing data discrepancies (18), and we wish to apply multiple criterion to study data discrepancies among experimental treatments fully. Consequently, z-score value was employed to standardize the data and it was used to assess the complex data and their complicated association owing to the huge amount of differently classified physiological indicators utilized in our study. In addition, Z value is negative once the original value is below the mean and positive when the original value is above the mean. This Z value is independent of the original unit of measurement.
As demonstrated in Figures 2A, B, FBW, ADC, SGR, and PER were reduced in diets containing novel proteins, although the SR, intestinal morphology, villus structure, muscular layer thickness, and goblet cells were unaltered. However, significant changes in FCR were not seen in ChM/FM, CSM/FM, and CAP/FM. Figure 2C demonstrates that the slopes of K of 0-4 weeks were less than the 4-8 weeks, which showed that growth performance of treatments containing novel protein diets in the second stage outperformed the first stage.
Figure 2 The growth performance and intestinal morphology of juvenile largemouth bass under an alternate feeding strategy. Growth performance heatmap (A);The distal intestines of FM/FM, ChM/FM, CSM/FM, CAP/FM, and TM/FM protein diet under 100 x magnification and the intestinal quantitative data. (B); Wight gain growth curve (C). Data are also expressed as mean (S.E.M). Values on each column/heatmap with different superscripts typified statistical differences (P<0.05).
The levels of CAT, T-SOD, MDA, GSH-Px, cat, gpx, sod, nrf-2, and keap-1 were evaluated in the intestine (Figures 3A–J). With the greatest GSH-Px activity in the ChM/FM group and the lowest CAT activity in the CSM/FM group, there were no dramatically discrepancies in the levels of MDA and T-SOD between diets containing novel proteins and FM/FM. In comparison to other diets, the levels of gpx and sod were significantly reduced in the ChM/FM and CSM/FM groups. Although the expression patterns of nrf-2 and keap-1 were distinct, the ratio of keap-1/nrf-2 did not alter substantially between diets containing novel proteins and FM/FM. These non-significant variations were also discovered in the level of cat.
Figure 3 The antioxidant enzymes and genes expression of juvenile largemouth bass intestines under an alternate feeding strategy were shown as follows: CAT (A), GSH-Px (B), T-SOD (C), MDA (D), keap-1/nrf-2 (E), cat (F), gpx (G), sod (H), nrf-2 (I), and keap-1 (J). Data are expressed as mean (S.E.M). Values on each column with different superscripts typified statistical differences (P<0.05).
The contents of LYS, sIgA-like, IgG-like, IgM, TNF-α, IL-1β, amylopsin, lipase, pepsase, and mucin 2 in the gut were examined to further investigate the impacts of alternate feeding of diets containing novel proteins on immunochemistry barriers (Figures 4A–K). Except for sIgA-like, no statistically significant alterations were seen in these biochemical indices. However, the plasma concentrations of TNF-α and IL-1β were lower in CAP/FM than in FM/FM (P=0.074). Moreover, the greatest levels of two crucial immunological markers, LYS and sIgA-like, were seen in the ChM/FM group.
Figure 4 The immunological and chemical barriers of juvenile largemouth bass under an alternate feeding strategy were shown as follows: LYS (A), sIgA-like (B), IgG-like (C), IgM (D), plasma TNF-α (E), plasma IL-1β (F), LYS (G), amylopsin (H), lipase (I), pepsase (J), and mucin 2 (K). Data are expressed as mean (S.E.M). Values on each column with different superscripts typified statistical differences (P<0.05).
The intestinal permeability was measured to assess the intestinal physical barrier’s integrity (Figures 5A–F). The level of claudin-1 and zo-1 did not differ statistically between CAP/FM and FM/FM, however the expression of these genes in other protein diets was considerably reduced in comparison to FM/FM (P<0.05). Intriguingly, the level of claudin-4 was substantially greater in CAP/FM than in FM/FM (P<0.05), and the transcription of occludin was significantly lower in TM/FM (P<0.05). In addition, the plasma LPS concentrations of all diets containing novel proteins were lower than FM/FM, and the intestinal LPS content of CAP/FM was lower than CSM/FM (P=0.057) and ChM/FM (P=0.051), while there were no significant differences amongst the five protein diets.
Figure 5 The intestinal physical barrier of juvenile largemouth bass under an alternate feeding strategy were shown as follows: claudin-1 (A), zo-1 (B), occludin (C), claudin-4 (D), intestinal LPS (E), and plasma LPS (F). Data are expressed as mean (S.E.M). Values on each column with different superscripts typified statistical differences (P<0.05).
To better comprehend the impact of new protein diets on the intestinal microbiome of juvenile largemouth bass under an alternative feeding strategy, the microbial diversity, functions, and compositions were investigated. Figures 6A–C demonstrate that the β-diversity of CAP/FM is substantially greater than that of other diets (P<0.05). Similarly, the α-diversity of CAP/FM exhibited a greater Simpson index than FM/FM (P<0.05). The bugbase function and phenotypic predictions (Figure 6D) revealed that ChM/FM had more stress tolerance than all other diets. Additionally, the quantity of anaerobic bacteria in CAP/FM exceeded that of the other protein diets, but the abundance of aerobic bacteria was the lowest. Figures 6E, F illustrate the intestinal composition at both the phylum and genus levels. Firmicutes, a SCFAs-producing phylum, and Proteobacteria, a phylum of opportunistic pathogens, changed significantly. Accordingly, the quantity of probiotics (Lactococcus) and pathogens (Pseudomonas) in the five protein diets was highly variable at the genus level. Specifically, Figures 6G, H depict the comparison of abundance of microbiota. The CAP/FM diet included the greatest abundance of Lactococcus and Firmicutes and the lowest abundance of Pseudomonas and Proteobacteria. In addition, CAP/FM showed a greater abundance of Plesiomonas than both ChM/FM and FM/FM (P<0.05). In contrast, these two protein diets included a greater number of Actinobacteria, Streptococcus, and Cyanobacteria than the CAP/FM diet. However, other microbiota did not differ considerably between diets containing novel proteins and FM/FM.
Figure 6 The intestinal microbiome of juvenile largemouth bass intestinal contents under an alternate feeding strategy were shown as follows: β-diversity (A), abundance comparison of β-diversity (B), α-diversity (C), bugbase analysis (D), top 10 microbiota at genus level (E), Top 10 microbiota at phylum level (F), abundance comparison of top ten microbiota at genus level (G), and abundance comparison of top 10 microbiota at phylum level (H).The statistical differences were presented as *P<0.05,**P<0.01, and ***P<0.001.
SCFAs have crucial roles in maintaining intestinal health and preventing occurrence of disease; thus, their presence in the five protein diets were considered. Figure 7A is a chord diagram depicting the contents of intestinal SCFAs in each protein diet. Seven SCFAs were discovered, with the greatest acetic acid concentration and the lowest isobutyric acid level. The heatmap in Figure 7B reveals that the CAP/FM diet included a greater proportion of SCFAs than other protein diets. In contrast, the concentration of SCFAs in TM/FM was the lowest. The bar graphs clearly (Figures 7C–J) demonstrate the considerable variation in SCFAs levels across all protein diets (Figure 7C). The lowest levels of total SCFAs, acetic acid, butyric acid, and propionic acid were found in the TM/FM diet, whereas the highest levels were found in the CAP/FM diet. Comparatively, the concentrations of isovaleric acid and isobutyric acid in the CAP/FM diet were considerably greater than in the FM/FM diet (P<0.05), although these two acids were significantly lower in other diets containing novel proteins (P<0.05). Additionally, no considerable differences were identified between diets containing novel proteins and FM/FM for caproic acid and valeric acid.
Figure 7 The intestinal metabolome of juvenile largemouth bass intestinal contents under an alternate feeding strategy were shown as follows: Distribution of SCFAs in each diet (A), relative abundance of SCFAs in each diet (B), total SCFAs contents (C), acetic acid content (D), butyric acid content (E), propionic acid content (F), caproic acid content (G), valeric acid content (H), isovaleric acid content (I), and isobutyric acid content (J). Data are expressed as mean (S.E.M). Values on each column with different superscripts typified statistical differences (P<0.05).
Pearson’s correlation was used to further study the link between microbiota and physiological indicators (Figure 8A). Shannon index, Simpson index, Firmicutes, Lactococcus, Anaerobic bacteria, Fusobacteriam, Plesiomonas, Cetobacterium, and Aeromonas were positively correlated with SCFAs (acetic acid, butyric acid, isovaleric acid, isovaleric acid, and propionic acid) and claudin-4. In contrast, these two categories of physiological indicators were adversely associated with pathogens, including Proteobacteria, Pseudomonas, and Aerobic bacteria. Similarly, Cyanobacteria, Actinobacteria, and Streptococcus also had significant positive correlations with ADC, SGR, and occludin (P<0.05). Inversely, the Verrucomicrobia had adverse correlations with ADC, SGR, and PER (P<0.05) although a positive correlation with FCR was observed (P<0.05). Surprisingly, the intestinal structure biomarkers were associated with Weissella (P<0.05).
Figure 8 Combined analysis and scoring of all data obtained from various protein treatments: (A) Heat map of Pearson’s correlation analysis (|r|>0.6, P 0.05) of bacterial phenotype, abundance at the genus/phylum level, SCFAs, and physiological biomarkers. On the heat map, the red square indicates a positive association, while the blue square indicates a negative correlation. (B) The Mantel test was performed on the core microbiota, intestinal SCFAs, and physiological indicators. The size of the square in the matrix symbolizes the coefficient value, with red squares representing positive correlations and blue squares negative correlations. The lines outside of the matrix reveal the relationship between the core microbiota as well as intestinal SCFAs and physiological markers, with thicker lines indicating stronger associations. (C) Heat map of the z-score analysis was clustered as the intestinal antioxidant①, intestinal chemical barrier②, intestinal immunological barrier③, intestinal physical barrier④, intestinal SCFAs contents⑤, and intestinal microbiota barrier⑥. The red-highlighted indexes indicated their negative relationship with fish intestinal health, whereas the black-highlighted indicated their positive relationship with fish intestinal health. (D) On the basis of the Z-score calculated from panel (C), a multidimensional radar map analysis of the effects of five protein treatments on the intestinal health of juvenile largemouth bass was presented. The statistical differences were presented as *P<0.05,**P<0.01, and ***P<0.001..
The Mantel test revealed the connections between microbiota/SCFAs, and physiological indicators (Figure 8B). Outside of the matrix, claudin-4 had a significant influence on the abundance of SCFAs and the top ten bacteria at the phylum level (P<0.05). In addition, the lipase activity may have a major impact on the top ten microbiota at the genus or phylum level. Within the matrix, the Mantel test revealed the negative association between CAT and villus structure/muscular layer thickness (P<0.05), FCR and PER/SGR/occludin (P<0.05), lipase and MDA, trypsin and claudin-1, LYS and mucin 2 (P<0.05). On the other hand, there were positive correlations between villus structure and muscular layer thickness, ADC and zo-1/occludin/SGR/PER/LPS (P<0.05), PER and SGR/zo-1/occludin (P<0.05), goblet cells and LYS/digestive enzymes, CAT and immune globulin/digestive enzymes, GSH-Px and sIgA-like/LYS/digestive enzymes, sIgA-like and digestive enzymes, pepsase and immune globulin/LYS (P<0.05), sIgA-like and LYS (P<0.05), claudin-1 and zo-1 (P<0.05).
Next, the Z-score was then computed in Figure 8C and presented in Figure 8D for a full understanding of the rank of functions for each diet. Briefly, the Z-score value were microbiota barrier: CAP/FM (4.87) > CSM/FM (0.89) > TM/FM (-1.29) > FM/FM (-1.36) > ChM/FM (-3.14); physical barrier: CAP/FM (4.95) > FM/FM (1.29) > CSM/FM (-0.48) > ChM/FM(-0.68)> TM/FM(-3.3); immunological barrier: ChM/FM (2.98)> CAP/FM (1.11) > TM/FM (0.66) > FM/FM (-0.98) > CSM/FM (-3.76); chemical barrier ChM/FM (1.68) > CAP/FM (0.62) > TM/FM (-0.27) > CSM/FM (0.91) > FM/FM (-1.11); antioxidant capacity: ChM/FM (2.69) > CAP/FM (2.32) > CSM/FM (1.06) > FM/FM (-1.05) > TM/FM (-1.83); SCFAs contents: CAP/FM (8.8) > FM/FM (0.12) > ChM/FM(0.11)> CSM/FM (-2.97) > TM/FM (-6.08).
It is well known that growth performance is an essential criteria for evaluating fishmeal alternatives. Even though a great deal of research has been conducted on alternate feeding strategy in fish (10, 11, 19), the impacts of new proteins on the growth performance of fish under alternate feeding strategy have not been investigated. In the present research, a substantial decrease in growth performance was detected when comparing diets containing novel proteins to whole fish meal diet; nevertheless, the most surprising discovery was that the growth rate in the second 29 days outperformed the first 29 days. This might be linked to fish-compensatory growth, in which fish that have suffered nutritional deficits instantly undergo a high rate of synthesis upon re-feeding, rapidly raising metabolic levels and enhancing food conversion rates (20). Therefore, the improved growth performance in the second stage of the current study was enhanced, and this was similar to studies done by Zhu et al., 2022, Bi et al., 2021, and Yılmaz et al., 2016 (10, 12, 13), which found the re-feeding of fish oil can also improve the decreased growth performance as well as n-3 ∑PUFA caused by linseed oil, beef tallow-based diets, and canola oil in A. schlegelii, S. maximus, and D. labrax respectively. Moreover, growth performance is possibly related with intestinal bacteria. On the basis of Pearson’s correlation analysis, the number of Cyanobacteria, Acitinobacteria, and Streptococcus was positively connected with weight increment; however, the abundance of these bacteria was lower in new protein diets than in whole fishmeal diet. Furthermore, the occludin was identified as a biomarker for growth performance by the Mantel test, and the lower occludin in diets containing novel proteins compared with the complete fishmeal diet may be responsible for the reduced growth performance. These joint analysis may provide new insight into the decreased growth performance in fish. Although lower growth performance was seen throughout the present investigation, the non-significant changes in intestinal structure and villus height/width demonstrated the growth potential of novel protein diets in the long-term alternate feeding.
Antioxidant enzymes are essential parts of the antioxidant defense system and help to reduce oxidative damage. MDA, an indication of oxidative stress injury in animals, is a lipid peroxidation product (21). In addition, it is well known that the nrf-2/keap-1 pathway protects cells from oxidative stress, and the activation of nrf2 might up-regulate downstream antioxidant genes that serve as a key cellular defense against the cytotoxic consequences of oxidative stress (22). The present research discovered a decrease in the level of keap-1, thus the GSH-Px activity in algae protein C. vulgaris diet increased. This was similar to the findings of Mohsen et al. (3), Hassnaa et al. (23), and Li et al. (19) when feeding Oreochromis niloticus and M. salmoides with a meal containing C. vulgaris observing the increased antioxidant capacity. This may be attributed to antioxidant bioactive components, which are pigments and vitamin C contained in this diet (24, 25), thus these factors promoted the colonization of oxidative stress tolerant bacteria ultimately leading to an increase in antioxidant capacity of C. vulgaris diet. According to several studies (26, 27), cottonseed protein concentrate, in which free-gossypol content was about 243.94mg/kg is detrimental to the intestinal health in grass carp and Nile tilapia. These fish were believed to tolerate anti-nutritional factors better than carnivorous fish. Our study observed decreased CAT activity in this plant protein diet, and this is largely owing to the poor resistance of carnivorous fish species to free-gossypol content in cottonseed protein concentrate diet (19), which caused intestinal inflammation when its level beyond 13.98mg/kg in a carnivorous fish, Sillago sihama Forsskál (28). Surprisingly, gpx and sod was dramatically reduced in algae diet C. vulgaris and cottonseed protein concentrate diets compared to whole fishmeal diet, although their GSH-Px as well as T-SOD enzyme activity was unaffected. In addition, the keap/nrf-2 ratio did not differ between the diets containing novel proteins and the whole fishmeal diet. These results indicated that the antioxidant system may inhibit the expression of these genes through feedback control (29).
The intestinal tight junction genes (claudin-1, zo-1, occludin, and claudin-4) and serum IL-1β, TNF-α and LPS can affect intestinal permeability in largemouth bass (17, 30), thus can reflect the intestinal cell-cell integrity. In the current study, the expression of tight-junction genes was suppressed in the cottonseed protein concentrate, algae protein C. vulgaris, and insect protein T. molitor diets, despite no considerable change in plasma LPS concentration. While the level of claudin-4 was up-regulated in the bacterial protein C. autoethanogenum diet. Additionally, this diet had the lowest LPS level in plasma and intestine compared to other protein diets. However, these results contradicted prior research that claimed the improved physical barrier was detected in yellow worm meal, bacterial protein C. autoethanogenum, and algal protein Chlorella diets (17, 19). Possible explanation is that the Gram-negative bacterium, i.e. Pseudomonas, which could disrupt the intestinal physical barrier and cause diseases in freshwater fish (31), was most prevalent in whole fishmeal. Therefore, the favorable effects that T. molitor, bacterial protein C. autoethanogenum, and algal protein C. vulgaris diets had were removed during the second fishmeal stage.
Immunity in fish is reliant on the immunological response, which is intimately connected to innate and adaptive immune components such as immunoglobulin (32). In addition, MUC2, LYS, and digestive enzyme can prevent intestine from being invaded by exogenous pathogens (15, 17). These parameters form a critical component of the intestinal immunochemical barrier and play a vital role in maintaining intestinal homeostasis. Our earlier investigation confirmed that a 56-day administration of novel protein diets might increase chemical and immunological intestinal barriers in largemouth bass (17), however the present study did not find any overall changes. Only the content of LYS and sIgA-like rose on the algae protein C. vulgaris diet compared with whole fishmeal diet. Similar findings were made by Maliwat et al. (33), Mohsen et al. (3), and Chen et al. (34), who discovered that feeding Chlorella to Macrobrachium rosenbergii, O. niloticus, and Oncorhynchus mykiss increased their immunity. These may be attributed to water-soluble polysaccharides (35), D-Lactic acid (36), and water-soluble alpha-glucans (37) contained in algae protein C. vulgaris diet. For other diets containing novel proteins, generally, it seems that the enhanced physiological functions from diets containing novel proteins were overshadowed by the second 29-day fishmeal stage. Also, it is interesting that there was a high correlation between lipase activity and the composition of the microbiota, although there were no significant differences between diets containing novel proteins and whole fishmeal. This is verified in the finding by Yang et al. (38), who also found that supplemental Aspergillus lipase can change the microbiota composition in rats. This may be due to the intestine’s enhanced hydrolysis of undigested macronutrients such as proteins and lipids into nutrients useable by the intestinal flora.
The intestinal microbiota may generate toxic compounds that result in barrier abnormality and disease processes, but it could also generate favorable metabolites like SCFAs, which have anti-inflammatory, anti-oxidant, and enteric-epithelial-repair functions that could impact animal wellness and disease progression (39). Thus, the structure and composition of the intestinal microbiota are regarded as crucial for intestinal health, since they improve host physiology and immunity in the intestine and affect body’s immunological response (40). Usually, the dominant bacteria play important role in the health status, and the most abundant bacteria in this research were Lactococcus, Firmicutes, Pseudomonas, and Protebacteria. Protebacteria, Pseudomonas, and Streptococcus, which have been recognized as detrimental to aquatic organisms, were shown to be less prevalent in the bacterial protein C. autoethanogenum diet. In contrast, a rise in beneficial bacteria, namely Lactococcus and Firmicutes, was seen in this diet. Consequently, a significant quantity of acetic acid, butyric acid, and propionic acid were generated. In agreement with the current findings, earlier research has revealed that feeding a diet containing C. autoethanogenum increased Firmicutes and decreased Protebacteria in largemouth bass (17, 19, 41). These were also confirmed by the bugbase functional predictions, which indicated that this diet included the greatest concentration of anaerobic bacteria and the lowest concentration of aerobic bacteria. This may be due to the fact that C. autoethanogenum contains a significant amount of carbohydrates, lipids, and vitamins that may regulate gut microbiota and enhance intestinal health (6, 42). However, no significant differences in microbiota were identified across other diets containing novel proteins and whole fishmeal diet when using this alternate feeding strategy. A possible explanation for these results may be that the other three protein diets were not as well adapted to the alternate feeding strategy as the bacterial protein C. autoethanogenum diet.
SCFAs were recognized to supply energy to intestinal mucosal cells, boost cell metabolism and development, as well as decrease the expansion of pathogens and prevent intestinal dysfunction. The recent research discovered very favorable associations between SCFAs and Shannon/Simpson. These indicators were used to assess the intestinal health (9, 43, 44) in earlier investigations. In addition, our findings were confirmed by a study conducted on mice, which indicated that increasing α-diversity linked to higher levels of short-chain fatty acids (45). Our discovery provides new insight into the elevated intestinal SCFAs in fish, albeit with further study for underlying mechanism. According to the Mantel test, claudin-4 is important for SCFAs contents, and lower expression was found in yellow meal worm diet, algae protein Chlorella diet, and cottonseed protein concentrate, resulting in lower SCFAs contents in these diets. This finding broadly supported our previous work, which found that tight junctions may affect the production of SCFAs (17).
Pearson’s correlation analysis revealed favorable associations between SCFAs/claudin-4 and probiotics, suggesting that these bacteria may aid in the production of SCFAs and the maintenance of intestinal barrier integrity. In contrast, the association between Proteobacteria/Pseudomonas/Aerobic bacteria and SCFAs yielded contradictory results. These pathogens were previously discovered to cause death or intestinal inflammation in numerous fish, including juvenile hybrid grouper (46) and largemouth bass (17, 30). Nonetheless, significant relationships were also discovered between SCFAs and possible pathogens (Plesiomonas/Aeromonas). The reason for this is not clear, but it may have something to do with the synergy between microbes, as Ding et al. (47) found the probiotic-treated diet could improve the health status of juvenile Megalobrama amblycephala while simultaneously increasing the abundance of both intestinal beneficial bacteria and harmful bacteria.
Despite the fact that physiological and microbial indicators were not significantly altered in the cottonseed protein concentrate diets, yellow worm meal diet, and algae protein C. vulgaris diet, compared to the whole fishmeal diet, we sought to assess their function holistically. Therefore, Z-score value of each diet from different physiological biomarkers were computed and summarized. Based on the amount of area occupied by each diet, the order is as follows: bacterial protein C. autoethanogenum diet (CAP/FM) > algae protein C. vulgaris diet > (ChM/FM) > whole fishmeal diet (FM/FM) > cottonseed protein concentrate diet (CSM/FM) > insect protein T. molitor diet (TM/FM). This result is inconsistent with our previous research (17), which indicated that the ranking functions in the algae protein C. vulgaris diet (ChM) were the highest, followed by the bacterial protein C. autoethanogenum diet (CAP), the insect protein yellow worm meal diet (TM), the fishmeal diet (FM), and the cottonseed protein concentrate diet (CSM). This discrepancy could be attributed to the different feeding strategies and adaptations of juvenile largemouth bass to different novel protein diets. It can thus be suggested that, compared with the whole fishmeal diet, the bacterial protein C. autoethanogenum diet and the algae protein C. vulgaris diet have better intestinal health under this alternate feeding strategy, which is an effective strategy to feed largemouth bass. However, This alternate feeding technique may not be acceptable for the insect protein T. molitor diet or the cottonseed protein concentrate diet.
This study set out to evaluate the functions of novel protein diets and a whole fishmeal diet under alternate feeding strategy. From the schematic model of this study (Figure 9), the growth performance of all diets containing novel proteins was elevated. In addition, the C. autoethanogenum diet and the C. vulgaris diet improved intestinal health. However, our comprehensive evaluation of the T. molitor diet and the cottonseed protein concentrate diet using the Z-score value revealed that intestinal health performance was inferior to that of the whole fishmeal diet. This work contributes to the existing application of novel protein diets by providing an alternate feeding strategy. Nonetheless, the alternate feeding strategy should be introduced with caution when fed novel proteins, since not all diets performed better than a fishmeal diet when employing this strategy. Future investigation and experimentation into the C. autoethanogenum diet as well as the C. vulgaris diet, when introduced with alternate feeding, is strongly recommended.
Figure 9 A schematic model showing the mechanism of the effects of novel protein diets on the intestinal health of juvenile largemouth bass under an alternate feeding strategy.
The data presented in the study are deposited in the NCBI repository, accession number PRJNA932585.
The animal study was reviewed and approved by Chinese order no. 676 of the state Council revised 1 March, 2017. Written informed consent was obtained from the owners for the participation of their animals in this study.
LL: conceptualization, data curation, formal analysis, investigation, methodology, writing - original draft. YW: sampling, resources, and software. YH: funding. CW: supervision, project administration, visualization writing, editing. All people who made significant contributions to the work presented in the article, including those who offered editing and writing assistance but are not authors, are acknowledged in the paper’s Acknowledgments section and have given their written permission to be included. All authors contributed to the article and approved the submitted version.
This work was financially supported by the National Key Research and Development Program of China (2019YFD0900200) and Fundamental Research Funds for the Central Universities (grant number: 2662020SCPY005).
Thanks to reviewers for their constructive comments to help improve the paper. Dr. Xuemei Xiong, Dr. Jie Wang, Dr. Gang Yang, and Ms. Wenyu Feng were thanked for their assistance in improving the quality of our work. Prof. Kenichi Tsuda and his team members were also thanked for their gracious assistance in supplying lab equipment and space for experiments during our lab's summer 2022 power cut.
The authors declare that the research was conducted in the absence of any commercial or financial relationships that could be construed as a potential conflict of interest.
All claims expressed in this article are solely those of the authors and do not necessarily represent those of their affiliated organizations, or those of the publisher, the editors and the reviewers. Any product that may be evaluated in this article, or claim that may be made by its manufacturer, is not guaranteed or endorsed by the publisher.
The Supplementary Material for this article can be found online at: https://www.frontiersin.org/articles/10.3389/fimmu.2023.1110696/full#supplementary-material
1. Zhang W, Belton B, Edwards P, Henriksson PJG, Little DC, Newton R, et al. Aquaculture will continue to depend more on land than sea. Nature (2022) 603:E2–4. doi: 10.1038/s41586-021-04331-3
2. FAO. The state of world fisheries and aquaculture. Rome: Food and agricultural organization (2020). Available at: http://www.fao.org/3/ca9229en/ca9229en.pdf.
3. Abdel-Tawwab M, Mousa MAA, Mamoon A, Abdelghany MF, Abdel-Hamid EAA, Abdel-Razek N, et al. Dietary Chlorella vulgaris modulates the performance, antioxidant capacity, innate immunity, and disease resistance capability of Nile tilapia fingerlings fed on plant-based diets. Anim Feed Sci Technol (2022) 283:115181. doi: 10.1016/j.anifeedsci.2021.115181
4. Li H, Hu Z, Liu S, Sun J, Ji H. Influence of dietary soybean meal replacement with yellow mealworm (Tenebrio molitor) on growth performance, antioxidant capacity, skin color, and flesh quality of mirror carp (Cyprinus carpio var. specularis). Aquaculture (2022) 561:738686. doi: 10.1016/j.aquaculture.2022.738686
5. Shen J, Liu H, Tan B, Dong X, Yang Q, Chi S, et al. Effects of replacement of fishmeal with cottonseed protein concentrate on the growth, intestinal microflora, haematological and antioxidant indices of juvenile golden pompano (Trachinotus ovatus). Aquac Nutr (2020) 26:1119–30. doi: 10.1111/anu.13069
6. Simpson S, Allen W, Conrado R, Molloy SJUS. United states patent publication, gas fermentation for the production of protein or feed. (2016).
7. Chen J, Wang H, Yuan H, Hu N, Zou F, Li C, et al. Effects of dietary Clostridium autoethanogenum protein on the growth, disease resistance, intestinal digestion, immunity and microbiota structure of Litopenaeus vannamei reared at different water salinities. Front Immunol (United States: Patent Application Publication). (2022) 13:1034994. doi: 10.3389/fimmu.2022.1034994
8. Wang M, Chen Z, Wang Y, Zou J, Li S, Guo X, et al. Largemouth bass (Micropterus salmoides) exhibited better growth potential after adaptation to dietary cottonseed protein concentrate inclusion but experienced higher inflammatory risk during bacterial infection. Front Immunol (2022) 13:997985. doi: 10.3389/fimmu.2022.997985
9. Zhang Z, Xi L, Liu H, Jin J, Yang Y, Zhu X, et al. High replacement of fishmeal by chlorella meal affects intestinal microbiota and the potential metabolic function in largemouth bass (Micropterus salmoides). Front Microbiol (2022) 13:1016662. doi: 10.3389/fmicb.2022.1016662
10. Bi Q, Liao Z, Wei Y, Liang M, Xu H. Effects of alternate feeding between fish oil- and terrestrially sourced oil-based diets on fatty acid composition of different tissues of turbot. Aquac Res (2021) 52:3475–82. doi: 10.1111/are.15154
11. Bjornevik M, Imsland AKD, Hanssen H, Roth B, Vikingstad E, Foss A. The effect of alternate-day feeding on growth and feed conversion in Atlantic cod gadus morhua. Aquac Nutr (2021) 27:1206–11. doi: 10.1111/anu.13260
12. Yilmaz HA, Corraze G, Panserat S, Eroldogan OT. Effects of alternate feeding with different lipid sources on fatty acid composition and bioconversion in European sea bass (Dicentrarchus labrax). Aquaculture (2016) 464:28–36. doi: 10.1016/j.aquaculture.2016.06.013
13. Zhu T, Shen Y, Li X, Pan T, Luo J, Lu J, et al. Effects of an alternating linseed oil-fish oil feeding strategy on growth, fatty acid restoration and expression of lipid related genes in black seabream (A. schlegelii). Aquaculture (2022) 547:737456. doi: 10.1016/j.aquaculture.2021.737456
14. Dawood MAO. Nutritional immunity of fish intestines: important insights for sustainable aquaculture. Rev Aquac (2021) 13:642–63. doi: 10.1111/raq.12492
15. Seo K, Seo J, Yeun J, Choi H, Kim Y-I, Chang S-Y. The role of mucosal barriers in human gut health. Arch Pharm Res (2021) 44:325–41. doi: 10.1007/s12272-021-01327-5
16. He M, Li X, Poolsawat L, Guo Z, Yao W, Zhang C, et al. Effects of fish meal replaced by fermented soybean meal on growth performance, intestinal histology and microbiota of largemouth bass (Micropterus salmoides). Aquac Nutr (2020) 26:1058–71. doi: 10.1111/anu.13064
17. Li L, Wang Y, Zhang Z, Wang C. Microbiomic and metabonomic analysis provide new insights into the enhanced intestinal health in large-size largemouth bass (Micropterus salmoides) when fed novel proteins: Novel proteins are promising foods for future aquaculture. Aquaculture (2023) 563:739019. doi: 10.1016/j.aquaculture.2022.739019
18. Amrhein V, Greenland S, McShane B. Retire statistical significance. Nature (2019) 567:305–7. doi: 10.1038/d41586-019-00857-9
19. Li L, Liu X, Wang Y, Huang Y, Wang C. Effects of alternate feeding between fish meal and novel protein diets on the intestinal health of juvenile largemouth bass (Micropterus salmoides). Aquac Rep (2022) 23:101023. doi: 10.1016/j.aqrep.2022.101023
20. Bavcevic L, Klanjscek T, Karamarko V, Anicic I, Legovic T. Compensatory growth in gilthead sea bream (Sparus aurata) compensates weight, but not length. Aquaculture (2010) 301:57–63. doi: 10.1016/j.aquaculture.2010.01.009
21. Martínez-Álvarez RM, Morales AE, Sanz A. Antioxidant defenses in fish: Biotic and abiotic factors. Rev Fish Biol Fish (2005) 15:75–88. doi: 10.1007/s11160-005-7846-4
22. Lee DY, Song MY, Kim EH. Role of oxidative stress and Nrf2/KEAP1 signaling in colorectal cancer: Mechanisms and therapeutic perspectives with phytochemicals. Antioxidants (2021) 10:743. doi: 10.3390/antiox10050743
23. Saleh HA, Gaber HS, El-Khayat HMM, Abdel-Motleb A, Mohammed WA-A, Okasha H. Influences of dietary supplementation of chlorella vulgaris and Spirulina platensis on growth-related genes expression and antioxidant enzymes in Oreochromis niloticus fish exposed to heavy metals. Aquacult Stud (2022) 22:AQUAST793. doi: 10.4194/aquast793
24. Mahmoud EA, El-Sayed BM, Mahsoub YH, El-Murr AEI, Neamat-Allah ANF. Effect of Chlorella vulgaris enriched diet on growth performance, hemato-immunological responses, antioxidant and transcriptomics profile disorders caused by deltamethrin toxicity in Nile tilapia (Oreochromis niloticus). Fish Shellfish Immunol (2020) 102:422–9. doi: 10.1016/j.fsi.2020.04.061
25. Raji AA, Alaba PA, Yusuf H, Abu Bakar NH, Taufek NM, Muin H, et al. Fishmeal replacement with Spirulina platensis and Chlorella vulgaris in African catfish (Clarias gariepinus) diet: Effect on antioxidant enzyme activities and haematological parameters. Res Vet Sci (2018) 119:67–75. doi: 10.1016/j.rvsc.2018.05.013
26. Li W-J, Zhang L, Wu H-X, Li M, Wang T, Zhang W-B, et al. Intestinal microbiota mediates gossypol-induced intestinal inflammation, oxidative stress, and apoptosis in fish. J Agric Food Chem (2022) 70:6688–97. doi: 10.1021/acs.jafc.2c01263
27. Wang K-Z, Feng L, Jiang W-D, Wu P, Liu Y, Jiang J, et al. Dietary gossypol reduced intestinal immunity and aggravated inflammation in on-growing grass carp (Ctenopharyngodon idella). Fish Shellfish Immunol (2019) 86:814–31. doi: 10.1016/j.fsi.2018.12.014
28. Liu H, Dong X, Tan B, Du T, Zhang S, Yang Y, et al. Effects of fish meal replacement by low-gossypol cottonseed meal on growth performance, digestive enzyme activity, intestine histology and inflammatory gene expression of silver sillago (Sillago sihamaForsskal) (1775). Aquac Nutr (2020) 26:1724–35. doi: 10.1111/anu.13123
29. Zhang Y, Liang X-F, He S, Chen X, Wang J, Li J, et al. Effects of high carbohydrate diet-modulated microbiota on gut health in Chinese perch. Front Microbiol (2020) 11:575102. doi: 10.3389/fmicb.2020.575102
30. Zhou Y-L, He G-L, Jin T, Chen Y-J, Dai F-Y, Luo L, et al. High dietary starch impairs intestinal health and microbiota of largemouth bass. Micropterus salmoides Aquacult (2021) 534:736261. doi: 10.1016/j.aquaculture.2020.736261
31. Shi Y, Cao X, Ye Z, Xu Y, Wang Y, Li Z, et al. Role of dietary Schizochytrium sp. in improving disease resistance of zebrafish metabolic and microbial. Aquaculture (2021) 539:736631. doi: 10.1016/j.aquaculture.2021.736631
32. Uribe C, Folch H, Enriquez R, Moran G. Innate and adaptive immunity in teleost fish: A review. Vet Med-cezch (2011) 56:486–503. doi: 10.17221/3294-VETMED
33. Maliwat GCF, Velasquez SF, Buluran SMD, Tayamen MM, Ragaza JA. Growth and immune response of pond-reared giant freshwater prawn Macrobrachium rosenbergii post larvae fed diets containing Chlorella vulgaris. Aquacult Fish (2021) 6:465–70. doi: 10.1016/j.aaf.2020.07.002
34. Chen W, Gao S, Huang Y, Chang K, Zhao X. Addition of chlorella sorokiniana meal in the diet of juvenile rainbow trout (Oncorhynchus mykiss): Influence on fish growth, gut histology, oxidative stress, immune response, and disease resistance against Aeromonas salmonicida. Fish Shellfish Immunol (2022) 129:243–50. doi: 10.1016/j.fsi.2022.08.062
35. Hsu H-Y, Jeyashoke N, Yeh C-H, Song Y-J, Hua K-F, Chao LK. Immunostimulatory bioactivity of algal polysaccharides from Chlorella pyrenoidosa activates macrophages via toll-like receptor 4. J Agric Food Chem (2010) 58:927–36. doi: 10.1021/jf902952z
36. Lee S-M, Kim S-K, Lee N, Ahn C-Y, Ryu C-M. D-lactic acid secreted by Chlorella fusca primes pattern-triggered immunity against Pseudomonas syringae in arabidopsis. Plant J (2020) 102:761–78. doi: 10.1111/tpj.14661
37. Tabarsa M, Shin I-S, Lee JH, Surayot U, Park W, You S. An immune-enhancing water-soluble alpha-glucan from Chlorella vulgaris and structural characteristics. Food Sci Biotechnol (2015) 24:1933–41. doi: 10.1007/s10068-015-0255-0
38. Yang Y, Kumrungsee T, Kato N, Fukuda S, Kuroda M, Yamaguchi S. Supplemental Aspergillus lipase and protease preparations display powerful bifidogenic effects and modulate the gut microbiota community of rats. Fermentation (2021) 7:294. doi: 10.3390/fermentation7040294
39. Wang A, Ran C, Wang Y, Zhang Z, Ding Q, Yang Y, et al. Use of probiotics in aquaculture of China-a review of the past decade. Fish Shellfish Immunol (2019) 86:734–55. doi: 10.1016/j.fsi.2018.12.026
40. Egerton S, Culloty S, Whooley J, Stanton C, Ross RP. The gut microbiota of marine fish. Front Microbiol (2018) 9:873. doi: 10.3389/fmicb.2018.00873
41. Ma S, Liang X, Chen P, Wang J, Gu X, Qin Y, et al. A new single-cell protein from Clostridium autoethanogenum as a functional protein for largemouth bass (Micropterus salmoides). Anim Nutr (2022) 10:99–110. doi: 10.1016/j.aninu.2022.04.005
42. Wang J, Chen L, Xu J, Ma S, Liang X, Wei Z, et al. C1 gas protein: A potential protein substitute for advancing aquaculture sustainability. Rev Aquac (2022). doi: 10.1111/raq.12707
43. Huang J-N, Zhang Y, Xu L, He K-X, Wen B, Yang P-W, et al. Microplastics: A tissue-specific threat to microbial community and biomarkers of discus fish (Symphysodon aequifasciatus). J Hazard Mater (2022) 424:127751. doi: 10.1016/j.jhazmat.2021.127751
44. Zhang Y, Wen B, Meng L-J, Gao J-Z, Chen Z-Z. Dynamic changes of gut microbiota of discus fish (Symphysodon haraldi) at different feeding stages. Aquaculture (2021) 531:735912. doi: 10.1016/j.aquaculture.2020.735912
45. Fu Y-P, Li C-Y, Peng X, Wangensteen H, Inngjerdingen KT, Zou Y-F. Pectic polysaccharides from Aconitum carmichaelii leaves protects against DSS-induced ulcerative colitis in mice through modulations of metabolism and microbiota composition. BioMed Pharmacother (2022) 155:113767. doi: 10.1016/j.biopha.2022.113767
46. Ye G, Dong X, Yang Q, Chi S, Liu H, Zhang H, et al. Low-gossypol cottonseed protein concentrate used as a replacement of fish meal for juvenile hybrid grouper (Epinephelus fuscoguttatus female x Epinephelus lanceolatus male) effects on growth performance, immune responses and intestinal microbiota. Aquaculture (2020) 524:735309. doi: 10.1016/j.aquaculture.2020.735309
47. Ding Z, Wang X, Liu Y, Zheng Y, Li H, Zhang M, et al. Dietary mannan oligosaccharides enhance the non-specific immunity, intestinal health, and resistance capacity of juvenile blunt snout bream (Megalobrama amblycephala) against aeromonas hydrophila. Front Immunol (2022) 13:863657. doi: 10.3389/fimmu.2022.863657
Keywords: intestinal health, alternate feeding strategy, intestinal barrier, novel protein diets, largemouth bass, SCFAs (short chain fatty acids)
Citation: Li L, Wang Y, Huang Y and Wang C (2023) Multi-omics approach to study the dual effects of novel proteins on the intestinal health of juvenile largemouth bass (Micropterus salmoides) under an alternate feeding strategy. Front. Immunol. 14:1110696. doi: 10.3389/fimmu.2023.1110696
Received: 29 November 2022; Accepted: 13 February 2023;
Published: 01 March 2023.
Edited by:
Liansheng Wang, Heilongjiang River Fisheries Research Institute (CAFS), ChinaCopyright © 2023 Li, Wang, Huang and Wang. This is an open-access article distributed under the terms of the Creative Commons Attribution License (CC BY). The use, distribution or reproduction in other forums is permitted, provided the original author(s) and the copyright owner(s) are credited and that the original publication in this journal is cited, in accordance with accepted academic practice. No use, distribution or reproduction is permitted which does not comply with these terms.
*Correspondence: Chunfang Wang, Y2Z3YW5nQG1haWwuaHphdS5lZHUuY24=; Yanqing Huang, aHlxcmljaEAxNjMuY29t
Disclaimer: All claims expressed in this article are solely those of the authors and do not necessarily represent those of their affiliated organizations, or those of the publisher, the editors and the reviewers. Any product that may be evaluated in this article or claim that may be made by its manufacturer is not guaranteed or endorsed by the publisher.
Research integrity at Frontiers
Learn more about the work of our research integrity team to safeguard the quality of each article we publish.