- Department of Obstetrics, Shenzhen People’s Hospital, The Second Clinical Medical College, Jinan University, Shenzhen, China
Regulatory T (Treg) cells could be divided into thymus-derived Treg (tTreg) cells and peripherally derived Treg (pTreg) cells, and in vitro induced Treg (iTreg) cells. To date, the functions of tTreg versus pTreg and their relative contributions to maternal-fetal immune tolerance remain insufficiently defined due to a lack of a specific marker to distinguish tTreg cells from pTreg cells. In this study, we investigated the role of thymus- and extrathymus-derived Treg cells in pregnancy tolerance using transgenic ACT-mOVA, Foxp3DTR and Foxp3GFP mice, and Treg cell adoptive transfer, etc. We found that the frequencies of Treg cells in the thymus, spleen and lymph nodes (LNs) in either syngeneically- or allogeneically-mated pregnant mice were not different from non-pregnant mice. However, percentages of blood Treg cells in pregnant mice increased at mid-gestation, and percentages of decidua Treg cells in pregnant mice increased as the pregnancy progressed compared with non-pregnant mice, and were significantly higher in allogeneic mice than those in syngeneic group. Compared with syngeneic mice, levels of CCR2 and CCR6 on blood and decidua Treg cells and CCL12 in the decidua significantly increased in allogeneic mice. A surrogate fetal antigen mOVA that was recognized by naïve T cells from OT-IIFoxp3GFP mice induced the generation of pTreg cells in vivo. Transfusion of thymus and spleen Treg cells significantly decreased diphtheria toxin (DT)-increased embryo resorption rates (ERRs) and IFN-γ levels in the blood and decidua. iTreg cells also decreased ERRs and IFN-γ levels in the blood and decidua to an extent lower than thymus and spleen Treg cells. In conclusion, increased blood and decidua Treg cells in pregnancy and increased ERRs in DT-treated Foxp3DTR mice suggest an important immunosuppressive role of Treg cells in pregnancy. Elevated decidua Treg cells in pregnancy could be derived from the recruitment of tTreg cells to the decidua, or from the transformation of naïve T cells in the decidua to pTreg cells. While the immune-suppression effects of thymus and spleen Treg cells are comparable, iTreg cells might play a weaker role in maternal-fetal tolerance.
Introduction
Regulatory T (Treg) cells are a special subset of T cells with immunomodulatory function. The transcription factor forkhead box p 3 (Foxp3) is highly expressed in the nucleus of Treg cells. Foxp3 plays an important role in the differentiation and development of Treg cells and the maintenance of their immunosuppressive function (1). The important role of Foxp3 in Treg cell function was first demonstrated by Scurfy mice with Foxp3 gene mutation that led to absence of Treg cells, proliferation of T cells in multiple organs, and death of most animals 3-5 weeks after birth (2). Similarly, it has been demonstrated that human Foxp3 gene mutation causes deletion of Treg cells and autoimmune immune disorders, leading to polyendocrine adenosis, intestinal ptosis and X-linked (IPEX) syndrome (3). Foxp3+ Treg cells not only regulate autoimmune diseases and maintain the stability of the internal immune environment (4), but also play an important immunosuppressive function in allergic reactions (5) and organ transplantation (6).
Treg cells are divided into thymus-derived Treg (tTreg) cells and peripherally derived Treg (pTreg) cells in vivo according to their sources (7). Foxp3+CD4+ tTreg cells are developed from CD4+CD25+ T cells in the thymus, while pTreg cells are mainly induced from naïve CD4+ T cells after they are stimulated by antigens in peripheral lymphoid organs (8). Generation of pTreg cells requires the activation of T cell receptor (TCR), and cytokines interleukin-2 (IL-2) and transforming growth factor-β (TGF-β) (8). Both tTreg cells and pTreg cells express Foxp3, and have immunosuppressive function (9). tTreg cells inhibit autoantigens and play an important role in inhibiting the body’s autoimmune responses (10). pTreg cells target exogenous antigens in the environment, air and food, and play an important role in mucosal immune tolerance and the stability of the internal immune environment (10). In addition, induced Treg (iTreg) cells can be generated in vitro from naïve T cells in the presence of TCR activation and cytokines (11).
tTreg cells and pTreg cells are different in gene expression, phenotypic stability, and epigenetic regulation (12). The proportion of tTreg cells and pTreg cells in the total Treg cell pool of the body, their specific functions, and how to distinguish them are not yet clear. It has been proposed that tTreg cells and pTreg cells could be distinguished by Helios since it has been reported that Helios mainly expresses on tTreg cells rather than pTreg cells (12). However, subsequent study has shown that pTreg cells also express Helios (13). Human tTreg cells also include Helios- subset (14). Neuropinlin-1 has been also thought to be mainly expressed on tTreg cells rather than pTreg cells, and it has been later confirmed that Neuropinlin-1 could not distinguish tTreg cells from pTreg cells (15).
A large number of studies have shown that Treg cells may play an important role in maternal-fetal tolerance and pregnancy maintenance. Treg cells have been found to accumulate in the draining lymph nodes (LNs) of the uteri of pregnant mice and express memory and effector markers (16). Conditional removal of Treg cells from allogeneically-mated pregnant mice resulted in reduced reproductive efficiency of mice, while conditional removal of Treg cells from syngeneically-mated pregnant mice resulted in defective embryo implantation and reduced fetal size, which could be alleviated by adoptive transfer of Treg cells (17).
It has been reported that there are more inhibitory Treg cells in human decidua in early pregnancy than in peripheral blood (18). In addition, Treg cells at the maternal-fetal interface of patients with unexplained recurrent abortion were significantly reduced compared with normal pregnant women (19). Santner-nanan et al. (20) found that the number of Treg cells and the Treg/Th17 ratio in the peripheral blood of patients with preeclampsia significantly decreased compared with normal pregnant women.
Compared with syngeneically-mated pregnant mice, Treg cells in peripheral lymphoid organs but not thymus significantly increased in allogeneically-mated pregnant mice, indicating that the increase of Treg cells during pregnancy is fetal antigen specific (21). However, Aluvihare et al. (22) found that systemic amplification of Treg cells during gestation was not dependent on the stimulation of fetal antigen, but might be driven by pregnancy itself. In addition, removal of Treg cells by diphtheria toxin (DT) in both syngeneically-mated and allogeneically-mated Foxp3DTR transgenic mice resulted in embryo implantation deficiency, effector T cell infiltration, uterine inflammation and fibrosis, indicating that Treg cell-mediated pregnancy tolerance does not necessarily depend on fetus-specific antigen (23).
It has been demonstrated that Foxp3 enhancer conserved non-coding sequence 1 (CNS1) plays an important role in the generation of pTreg cells but not tTreg cells (24). Treg cells in the decidua of allogeneically-mated CNS1-/- pregnant mice significantly reduced, but embryo absorption rate increased, suggesting that fetal antigen-specific pTreg cells play a major role in pregnancy tolerance (25).
To date, the functions of tTreg versus pTreg and their relative contributions to maternal-fetal immune tolerance remain insufficiently defined due to a lack of a specific marker to distinguish tTreg cells from pTreg cells. In this study, we investigated the role of thymus- and extrathymus-derived Treg cells in pregnancy tolerance using transgenic ACT-mOVA, Foxp3DTR and Foxp3GFP mice, and Treg cell adoptive transfer, etc. We found that decidua and blood Treg cells significantly increased during pregnancy; elevated decidua Treg cells in pregnancy could stem from the transformation of naïve T cells, or from the recruitment of tTreg; while the immune-suppressive effects of Treg cells in the thymus and spleen were comparable, in vitro generated iTreg cells might also played an important but weaker role in maternal-fetal tolerance.
Materials and methods
Animals
C57BL/6 and BALB/c mice were purchased from the Experimental Animal Center of Zhongshan University (Guangzhou, China). Transgenic Foxp3DTR mice, Act-mOVA mice and OT-II mice on a C57BL/6 background were purchased from The Jackson Laboratory (Bar Harbor, ME, USA). Foxp3GFP mice on a C57BL/6 background were kindly provided by Professor Zhexiong Lian (South China University of Technology, Guangzhou, China). Mice were bred in the Laboratory Animal Center of Shenzhen People’s Hospital (Shenzhen, China) in pathogen-free conditions. Syngeneic C57BL/6 × C57BL/6 and allogeneic C57BL/6 × BALB/c mating combinations were established. Each female mouse at 10-12 weeks of age was cocaged with one male. The point at which a vaginal plug was detected was designated as embryonic day 0.5 (E0.5). Mice were sacrificed at different gestational age to investigate proportions of Treg cells in the thymi, peripheral blood, spleens, LNs or deciduae. Non-pregnant mice were set up as negative controls.
Induction of pTreg cells in vivo
Foxp3GFP females were mated to OT-II males to generate OT-IIFoxp3GFP mice. Spleen CD4+CD62L+GFP- naïve T cells were isolated from female Foxp3GFP or OT-IIFoxp3GFP mice. Female C57BL/6 mice were mated to male Act-mOVA mice to generate fetal antigen mOVA that can be recognized by CD4+ cells from OT-II mice (26). Spleen CD4+CD62L+GFP- naïve T cells (2 × 106 cells) from female Foxp3GFP mice or OT-IIFoxp3GFP mice were injected into the tail veins of pregnant C57BL/6 mice mated to Act-mOVA mice on E9.5. Pregnant mice were sacrificed on E13.5, and CD4+GFP+ Treg cells in the peripheral blood, spleens and deciduae were analyzed using flow cytometry.
Treg cell depletion and adoptive transfer of Treg cells
For Treg cell depletion, syngeneic pregnant Foxp3DTR mice were injected i.p. with DT (Sigma, Saint Louis, MO, USA) at a dosage of 60 µg/kg of body weight on E9.5, E10.5 and E11.5. Control mice received phosphatebuffered saline (PBS). For Treg cell reconstitution, 2 × 105 purified Treg cells from the thymi and spleens of C57BL/6 mice, or in vitro cultured iTreg cells were injected into the tail veins of Foxp3DTR mice 2 h before DT injection. Pregnant mice were sacrificed on E13.5. At this stage of gestation, feto-placental units undergoing resorption can be clearly distinguished from their viable counterparts on the basis of size and the presence of extensive tissue wasting and hemorrhage. The number of total embryos, resorbed and viable embryos per mouse was counted. The embryo resorption rate (ERR) was calculated as: ERR (%) = number of resorbed embryos/number of total embryos × 100. Treg cells in the thymi, peripheral blood, spleens and deciduae were analyzed using flow cytometry. Deciduae were collected for calculating Th1 and Th17 cell percentages using flow cytometry.
Isolation of thymus, spleen or LN mononuclear cells
Thymi, spleens or LNs including cervical, axillary, mesenteric and inguinal LNs were collected and mashed through a 40 μm cell strainer (Thermo Fisher Scientific, Waltham, MA, USA) to obtain single-cell suspensions. Mononuclear cells were then enriched by lysing red cells using a red cell lysis buffer (Biolegend, San Diego, CA, USA).
Isolation of peripheral blood mononuclear cells
Peripheral blood samples were collected from the vena orbitalis, heparinized, and purified by centrifugation on a Ficoll-Hypaque Premium (GE Healthcare, Pittsburgh, PA, USA).
Isolation of decidua mononuclear cells
Uterine tissues were collected, washed and cut into small pieces and digested with 1 mg/ml Dispase II (Roche, Basel, Switzerland) and 0.1 mg/ml DNase I (Roche) at 37°C for about 20 min in a shaking water bath. When single or clumps of cells were observed under the microscope, the released cells were separated from undigested tissue pieces by filtering through a 40 µm cell strainer. Mononuclear cells were purified over the Ficoll-Paque Premium by centrifugation at 400 g for 30 min at 20°C.
Isolation of spleen CD4+CD62L+ naïve T cells
Naïve T cells were isolated from the spleens of Foxp3GFP mice using a mouse CD4+CD62L+ naïve T cell isolation kit (Miltenyi Biotec, Bergisch Gladbach, Germany) according to the manufacturer’s instructions. In brief, spleen mononuclear cells were labeled with a CD4+ T Cell Biotin-Antibody Cocktail, washed and incubated with anti-biotin microbeads. Non-CD4+ T cells were then magnetically depleted using the midiMACS separator. CD4+ T cells were collected and incubated with anti-CD62L microbeads. CD4+CD62L+ naïve T cells were sorted using the miniMACS separator. The purity of isolated naïve T cells were detected using flow cytometry.
Isolation of thymus and spleen CD4+GFP+ Treg cells
Thymus and spleen mononuclear cells of Foxp3GFP mice on E12.5 were isolated. CD4+ cells were enriched using a mouse CD4+ T cell isolation kit (Miltenyi Biotec) according to the manufacturer’s instructions, and CD4+GFP+ Treg cells were sorted by a FACSAria cell sorter (Becton Dickinson, Franklin Lakes, NJ, USA). In brief, the single-cell suspensions were labeled with a CD4+ T Cell Biotin-Antibody Cocktail, washed and incubated with anti-biotin microbeads. Non-CD4+ T cells were then magnetically depleted using the midiMACS separator. Subsequently, enriched CD4+ T cells were collected, and CD4+GFP+ Treg cells were sorted using the FACS-Aria cell sorter. The purity of isolated CD4+GFP+ Treg cells were analyzed using flow cytometry.
In vitro generation of spleen iTreg cells
Spleen CD4+CD62L+GFP- naïve T cells of Foxp3GFP mice were isolated. The cells (1 × 106/ml) were then cultured in the presence of 2.5 μg/ml anti-CD3 Ab (Biolegend), 1 μg/ml anti-CD28 Ab (Biolegend), 100 U/ml IL-2 (R&D Systems, Minneapolis, MN, USA), and 10 ng/ml TGF-β (R&D Systems) for 96 h in vitro. The ratio of CD4+GFP+ iTreg cells was detected using flow cytometry, and the iTreg cells were sorted for later experiments.
Flow cytometry
For Treg cell analysis, cells were incubated with fluorescence-conjugated Abs against CD45, CD3, CD4 and CD8 for 30 min at 4°C, and then washed and fixed in a fixation buffer. Cells were re-suspended in a permeabilization wash buffer and incubated with a fluorescence-conjugated Ab against Foxp3. Isotype controls were established using matched fluorescence-labeled isotype control Abs to account for nonspecific staining. Immunostained cells were analyzed on a FACS Fortessa flow cytometer (BD Biosciences, San Jose, CA, USA) using the FlowJo software (Ashland, OR, USA). The percentages of Foxp3+ cells in the CD45+CD3+CD4+CD8- cell population were analyzed.
For Foxp3GFP mice, cells were incubated with aforementioned fluorescently labeled Abs against CD45, CD3, CD4 and CD8 for 30 min at 4°C. Cells were washed and fixed in a fixation buffer. The percentage of GFP+ cells in the CD4+GFP+ cell population was analyzed. In selected experiments, cells were incubated with fluorescently labeled Abs against CD45, CD3, CD4 and CD8, CCR2 and CCR6. The expression of CCR2 and CCR6 on CD45+CD3+CD4+CD8-GFP+ cells was detected. For naïve T cell analysis, cells were mixed with fluorescently labeled Abs against CD45, CD3, CD4 and CD8, and CD62L for 30 min at 4°C. The percentages of CD45+CD3+CD4+CD62L+ cells in CD45+CD3+CD4+CD8- cells were analyzed.
For analysing intracellular factors, cells were incubated with fluoresence-conjugated Abs against CD45, CD3, CD4 and CD8 for 30 min at 4°C. Cells were washed, fixed, re-suspended in a permeabilization wash buffer, and incubated with fluorescence-conjugated Abs against IFN-γ and IL-17. Percentages of IFN-γ+ and IL-17+ cells in the CD45+CD3+CD4+CD8- cell population were analyzed. Fluorescence-conjugated Abs, matched fluorescence-labeled isotype control Abs, fixation buffer, and permeabilization wash buffer were all purchased from BioLegend. Flow cytometry Abs were summarized in Table 1.
mRNA levels of chemokines in the decidua by real time (RT)-PCR
The uteri of the non-pregnant mice, syngeneic and allogeneic pregnant mice on E13.5 were quickly removed and placed in liquid nitrogen and transferred to the -80°C refrigerator for later use. Total RNA was extracted from the uteri using Trizol extraction, and the content and purity of total RNA were determined by spectrophotometry. cDNA was obtained according to the instructions of the PrimeScript™ RT Reagent Kit with gDNA Eraser (Perfect Real Time) (TaKaRa, Beijing, China). The mRNA levels of ccl2, ccl7, ccl12,ccl17, ccl19, ccl20, ccl21, cxcl9, cxcl10 and cxcl11 in the deciduae were detected using PowerUp™ SYBR™ Green Master Mix (Applied Biosystems, Foster City, CA, US) and QuantStudio fluorescent quantitative RT-PCR system (Thermo Fisher Scientific) with β-actin as the internal reference. The method of 2⁃△△Ct relative quantitation was used to compare the difference of target mRNA expression between groups. The primers of the chemokines were bought from Shanghai Shenggong Biological Company (Shanghai, China) and shown in the Table 2.
Protein levels of chemokines in the decidua by western blot
The uteri of the non-pregnant mice, syngeneic and allogeneic pregnant mice on E13.5 were collected and stored at -80°C. Tissue protein was extracted, and the bicinchoninic acid (BCA) Protein Quantification Kit (Thermo Fisher Scientific) was used to detect protein expression levels. The prestain protein marker and protein samples to be tested were loaded in the glue and electrophoresed, and then transferred to the PVDF membrane, and sealed by 5% skimmed milk. The membrane was then incubated with anti-mouse CCL2, CCL7, CCL12 and CCL20 Abs, washed and incubated with horseradish peroxidase (HRP)-coupled goat-anti-mouse secondary Ab IgG (H+L). The membrane was then washed, and hypersensitive chemiluminescence substrate (Thermo Fisher Scientific) was added for color exposure. Image J software (NationalnInstitutes of Health, Bethesda, MD, USA) was used to detect the gray value of the strip. The expression of target proteins CCL2, CCL7, CCL12 and CCL20 were analyzed semi-quantitatively using GAPDH as the internal reference protein. Western blot Abs were summarized in Table 1.
Chemotaxis experiments
Isolated thymus CD4+GFP+ Treg cells (1 × 105) were seeded in the upper chamber of a 24-well plate (5-μm pore; Sigma). A volume of 600 μl recombinated chemokines including 20 ng/ml CCL2, 500 ng/ml CCL7, 50 ng/ml CCL12 and 10 ng/ml CCL20 were loaded in the lower chamber, respectively. Basal culture medium in the lower chamber served as a negative control. After 24 h, cells in the lower chamber were collected. The numbers of CD4+GFP+ cells were calculated using flow cytometry. Migration index was expressed as fold change of the numbers of CD4+GFP+ cells relative to those isolated from basal culture medium controls. The assay was carried out in triplicate and repeated three times independently.
Statistical analysis
All statistical analyses were performed using SPSS 19.0 software (Chicago, IL, USA). Data were analyzed by one-way ANOVA with Bonferroni test among three or more groups, or independent Student’s t-test between two groups. Results were given as mean ± SD. A p value of < 0.05 was considered statistically significant between analyzed groups.
Results
Percentages of thymus, blood, spleen and LN Treg cells in syngeneic and allogeneic pregnancies
The percentages of thymus, spleen and LN CD4+Foxp3+ Treg cells in CD4+ T cells neither differ significantly among pregnant and non-pregnant groups, nor differ significantly between syngeneic and allogeneic mice from E4.5 to E19.5 (Figures 1A, C, D). However, as shown in Figure 1B, the proportions of blood CD4+Foxp3+ Treg cells in CD4+ T cells significantly increased in both syngeneic and allogeneic mice on E13.5 as compared with non-pregnant mice (p < 0.01 for both comparisons), while proportions of blood Treg cells at different gestational ages did not differ between syngeneic and heterogeneic pregnant mice.
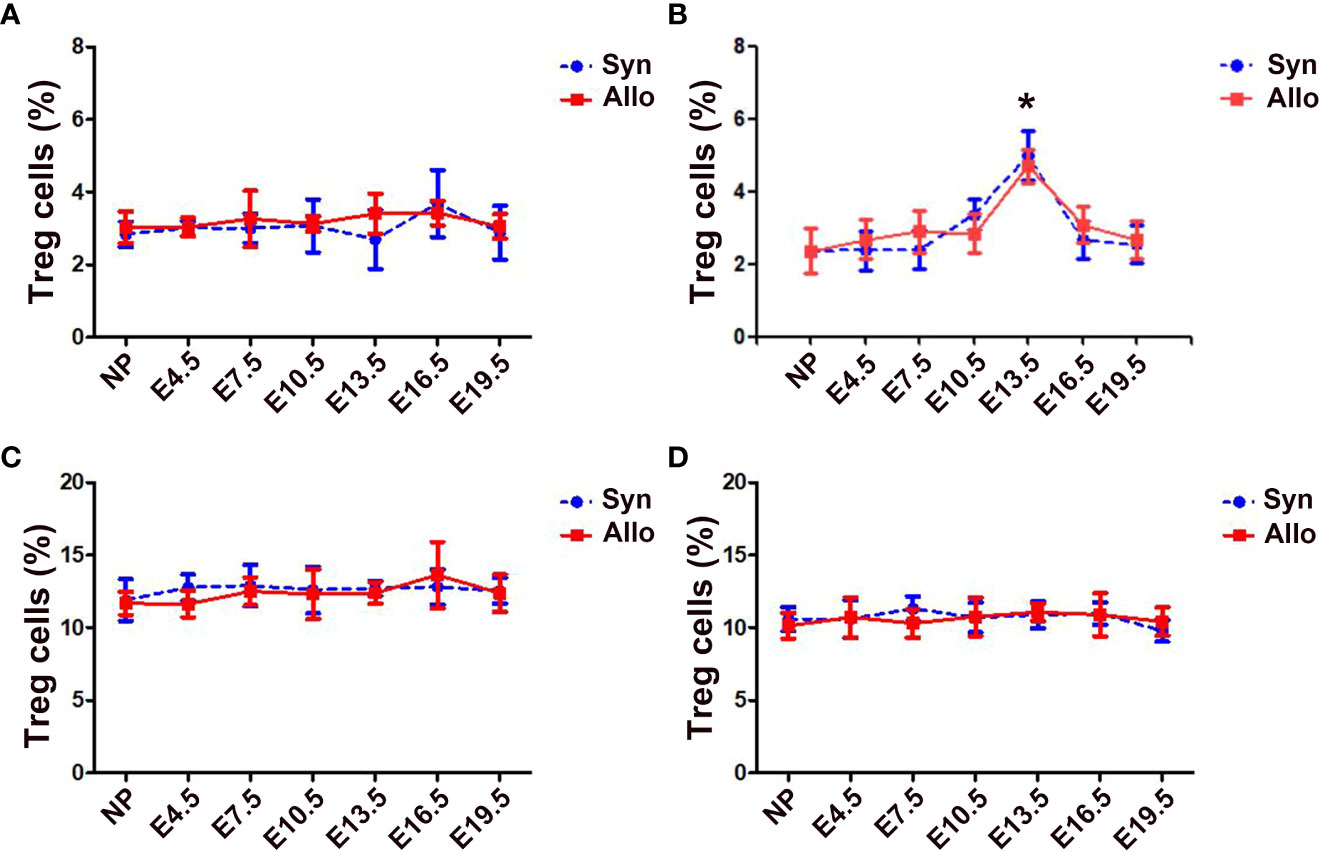
Figure 1 Percentages of thymus, blood, spleen and LN Treg cells in syngeneic and allogeneic pregnancies. A graphical summary of the percentages of thymus (A), blood (B), spleen (C) and LN (D) Treg cells is presented. Data are presented as mean ± SD (n = 6). *p < 0.01 vs the non-pregnant mice. NP, non-pregnant group; Syn, syngeneic pregnant group; Allo, allogeneic pregnant group.
Percentages of decidua Treg cells in syngeneic and allogeneic pregnancies
As shown in Figure 2, the percentages of decidua CD4+Foxp3+ Treg cells in both syngeneic and allogeneic mice significantly increased as the pregnancy progressed, peaked on E13.5, and then decreased till E19.5. The percentages of decidua Treg cells in allogeneic mice on E10.5, E13.5 and E16.5 were significantly higher than those in syngeneic mice (p < 0.01, respectively).
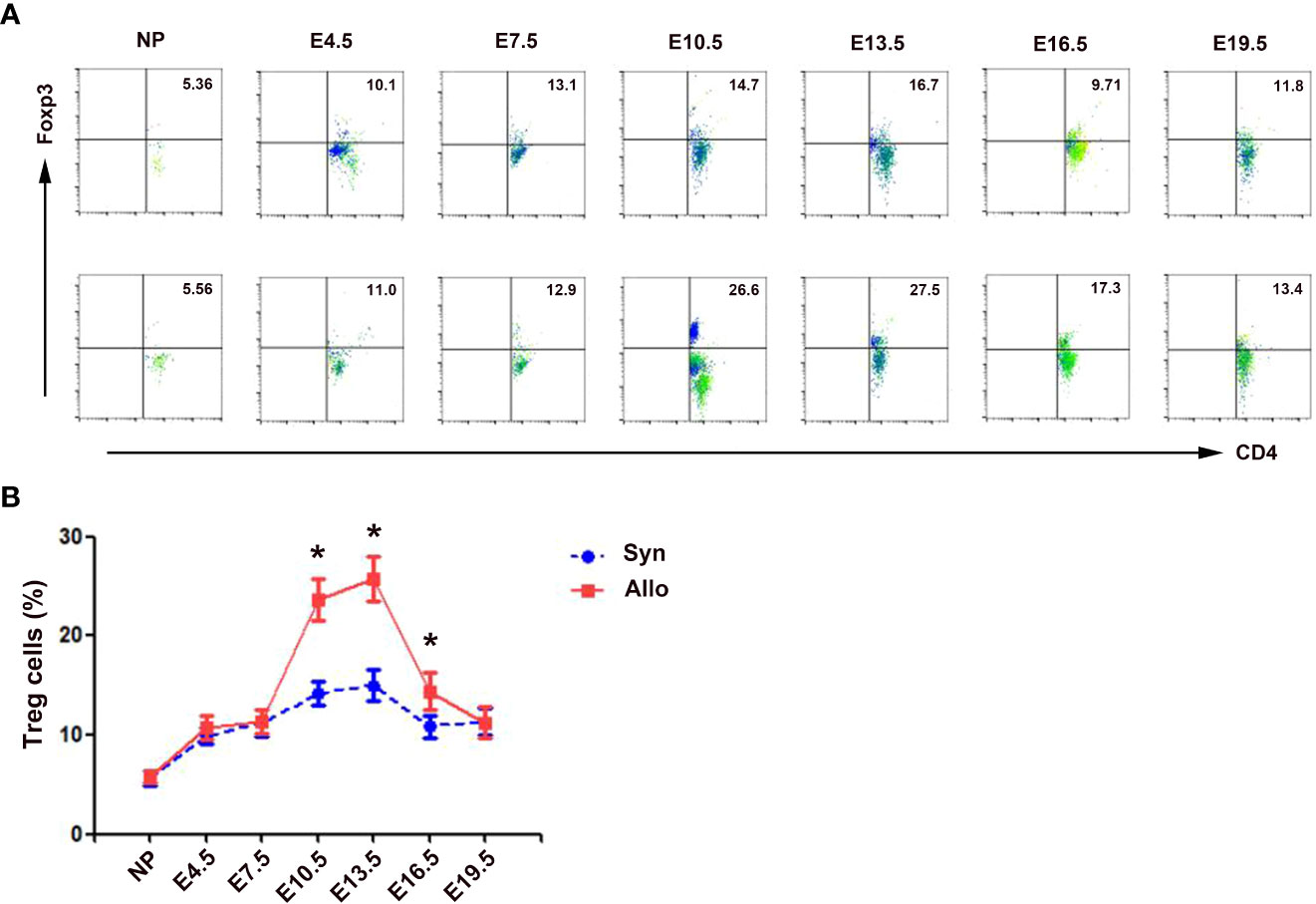
Figure 2 Percentages of decidua Treg cells in syngeneic and allogeneic pregnancies. (A) One representative experiment of the percentages of decidua Treg cells in syngeneic mice (upper) and allogeneic mice (lower) is shown. Numbers indicate the percentages of Foxp3+CD4+ cells in CD4+ T cells (%). (B) A graphical summary of the percentages of decidua Treg cells is presented. Data are presented as mean ± SD (n = 6). *p < 0.01 vs the syngeneic mice. NP, non-pregnant group; Syn, syngeneic pregnant group; Allo, allogeneic pregnant group.
mRNA and protein levels of chemokines in the deciduae of syngeneic and allogeneic mice
As shown in Figure 3A, compared with non-pregnant group, levels of CCL2, CCL7, CCL12 and CCL20 mRNA in the deciduae significantly increased in both syngeneic mice (p < 0.01, p < 0.05, p < 0.05 and p < 0.01) and in allogeneic mice (p < 0.01, respectively). In addition, levels of CCL2, CCL7, CCL12 and CCL20 mRNA in the deciduae of allogeneic mice were significantly up-regulated compared with those of syngeneic mice (p < 0.05, p < 0.05, p < 0.05 and p < 0.01). However, levels of CCL17, CCL19, CCL21, CXCL9, CXCL10 and CXCL11 mRNA in the deciduae of pregnant mice did not differ significantly from non-pregnant mice (data not shown).
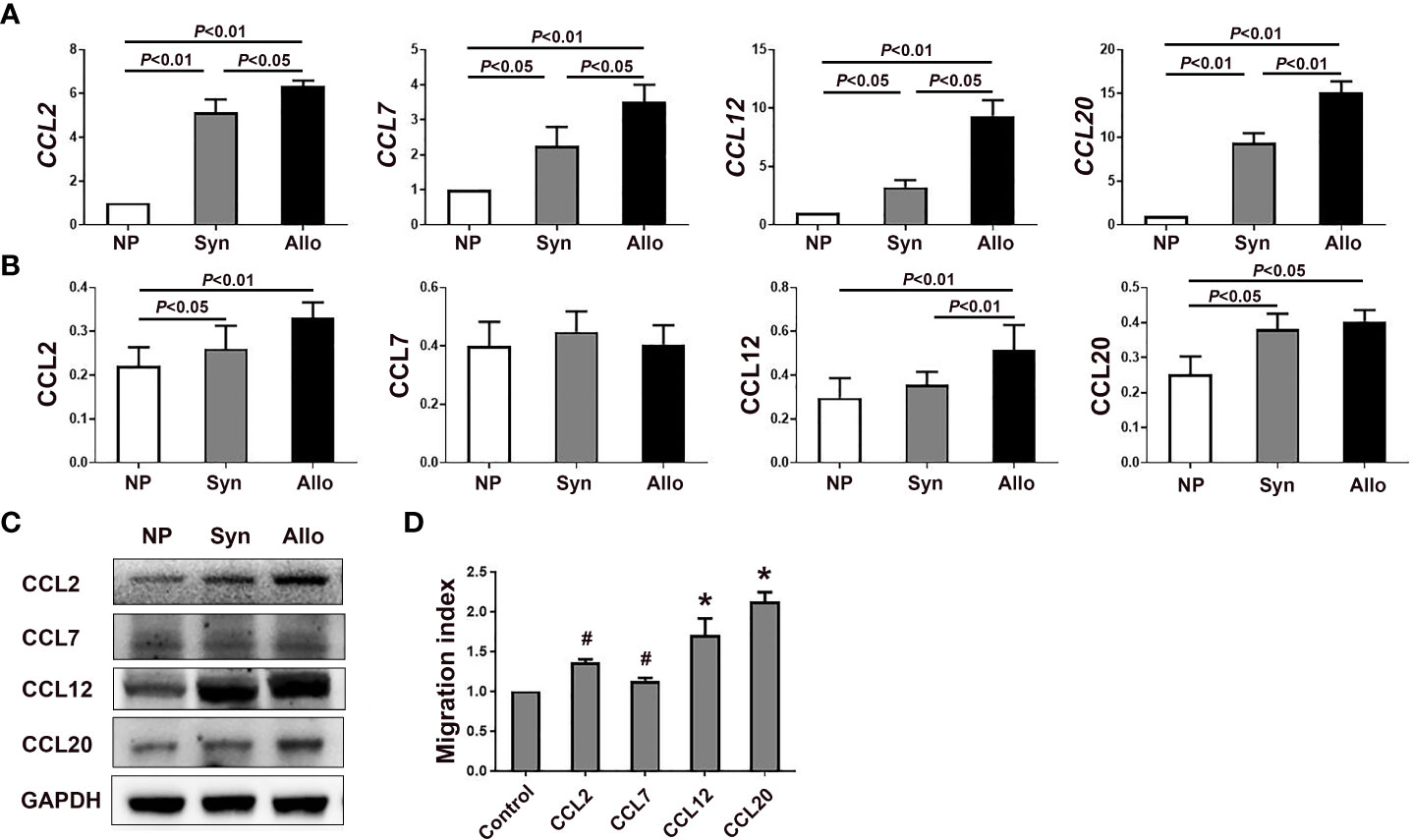
Figure 3 mRNA and protein levels of chemokines in the deciduae of syngeneic and allogeneic mice. (A) Levels of CCL2, CCL7, CCL12 and CCL20 mRNA in the deciduae of syngeneic and allogeneic mice are shown (n = 6). (B) Levels of proteins CCL2, CCL7, CCL12 and CCL20 in the deciduae of syngeneic and allogeneic mice are shown. (C) One representative western blot experiment of the protein levels of CCL2, CCL7, CCL12 and CCL20 is shown. (D) Chemotactic effects of chemokines CCL2, CCL7, CCL12 and CCL20 on thymus Treg cells. Data are presented as mean ± SD (n = 6). *p < 0.01, #p < 0.05 vs the control group.
Since mRNA levels of CCL2, CCL7, CCL12 and CCL20 significantly increased in the deciduae of pregnant mice compared with non-pregnant mice, we analyzed protein levels of those chemokines in the decidua. As shown in Figures 3B, C, protein levels of CCL2, CCL12 and CCL20 in the deciduae of syngeneic mice (p < 0.05, p < 0.01 and p < 0.05) and allogeneic mice (p < 0.01, p < 0.01 and p < 0.05) were significantly higher than those of non-pregnant mice. In addition, the level of CCL12 in the deciduae of allogeneic mice significantly increased compared with that of syngeneic mice (p < 0.01). However, we did not find significant differences in CCL7 levels among the three groups (Figures 3B, C).
Chemotactic effects of chemokines CCL2, CCL12 and CCL20 on thymus Treg cells
In the case the mRNA levels of CCL2, CCL7, CCL12 and CCL20 in the deciduae significantly increased, we wondered whether those chemokines can attract thymus Treg cells into the deciduae by chemotactic experiments in vitro. As shown in Figure 3D, the migration indexes of CCL2, CCL7, CCL12 and CCL20 groups were all significantly increased compared with the control group without chemokines (p < 0.05, p < 0.01 and p < 0.01).
Expression of chemokine receptors CCR2 and CCR6 on thymus and blood Treg cells between syngeneic and allogeneic mice
Since mRNA and protein levels of CCL2, CCL12 and CCL20 in the decidua significantly increased in pregnant mice, we wanted to know levels of the receptors CCR2 and CCR6 of those chemokines on thymus and blood Treg cells. As shown in Figures 4A-C, compared with non-pregnant mice, while the expression of CCR2 on thymus Treg cells significantly up-regulated in syngeneic and allogeneic mice (p < 0.01 for both comparisons), the expression of CCR6 on thymus Treg cells significantly up-regulated in allogeneic mice (p < 0.01). In addition, the expression of CCR2 and CCR6 on thymus Treg cells significantly increased in allogeneic mice as compared to syngeneic mice (p < 0.01 for both comparisons). Similarly, as shown in Figures 4D-F, compared with non-pregnant mice, while the expression of CCR2 on peripheral blood Treg cells significantly up-regulated in syngeneic and allogeneic mice (p < 0.01 for both comparisons), the expression of CCR6 on peripheral blood Treg cells significantly up-regulated in allogeneic mice (p < 0.01). Besides, the expression of CCR2 (p < 0.01) and CCR6 (p < 0.05) on peripheral blood Treg cells significantly increased in allogeneic mice as compared to syngeneic mice.
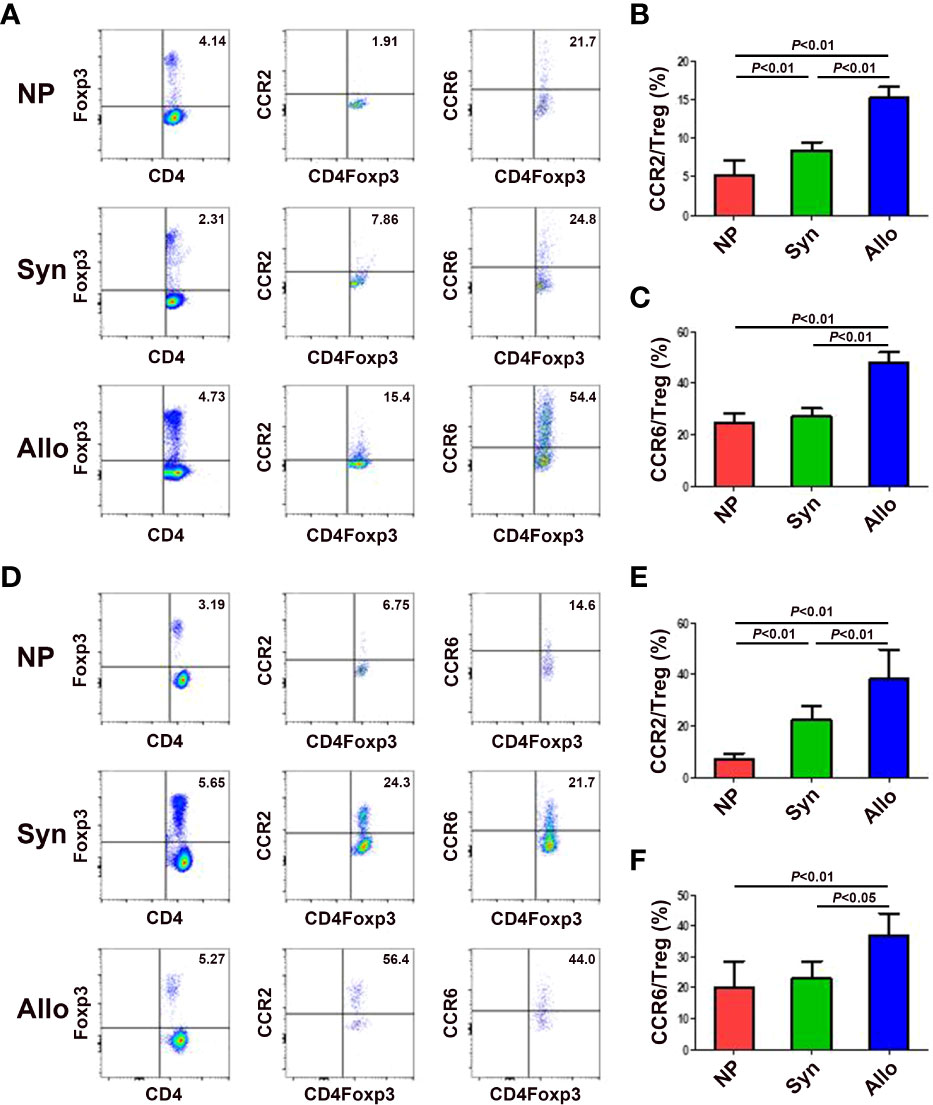
Figure 4 Expression of chemokine receptors CCR2 and CCR6 on thymus and blood Treg cells between syngeneic and allogeneic mice. (A) Representative flow cytometry experiments of the levels of CCR2 and CCR6 on thymus Treg cells are shown. Levels of CCR2 (B) and CCR6 (C) on thymus Treg cells are shown. (D) Representative flow cytometry experiments of the levels of CCR2 and CCR6 on peripheral blood Treg cells are shown. Levels of CCR2 (E) and CCR6 (F) on peripheral blood Treg cells are shown. Data are presented as mean ± SD (n = 6). NP, non-pregnant group; Syn, syngeneic pregnant group; Allo, allogeneic pregnant group.
Fetal antigen induced pTreg cell generation in vivo
In order to investigate the role of fetal antigen-specific pTreg cells in maternal-fetal tolerance, we first investigated whether pTreg could be induced from fetal antigen-specific naïve T cells using transgenic OT-II mice and fetal surrogate antigen mOVA. We demonstrated that pTreg cells were induced in vivo from mOVA-specific CD4+CD62L+GFP- naïve T cells, since transfusion of CD4+CD62L+GFP- naïve T cells that from OT-IIFoxp3GFP mice induced more CD4+GFP+ pTreg cells in the peripheral blood, spleens and deciduae of recipient mice than transfusion of naïve T cells from Foxp3GFP mice (p < 0.01, respectively) (Figure 5).
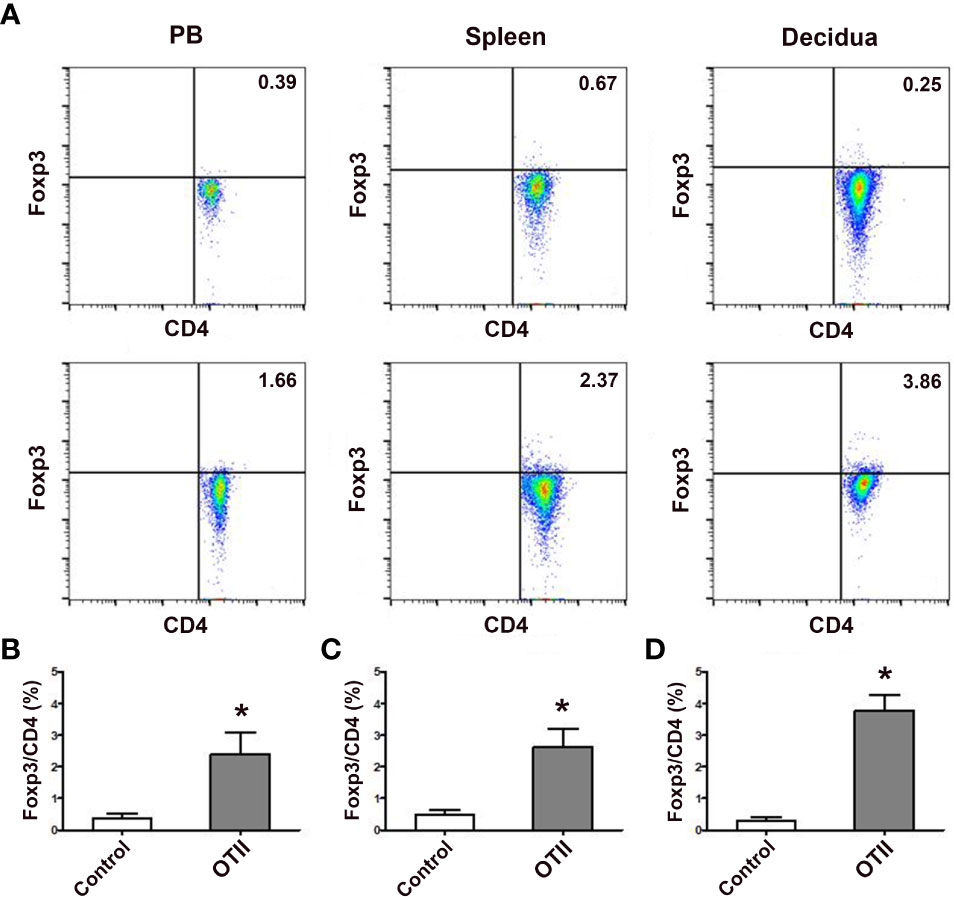
Figure 5 Fetal antigen induced pTreg cell generation in vivo. (A) Representative flow cytometry experiments of in vivo generated CD4+GFP+ Treg cells from naïve T cells of female Foxp3GFP mice (upper row) or OT-IIFoxp3GFP mice (lower row) in the peripheral blood, spleens and deciduae of pregnant C57BL/6 mice mated to Act-mOVA mice are shown. Levels of in vivo generated CD4+GFP+ Treg cells in the peripheral blood (B), spleens (C) and deciduae (D) of pregnant C57BL/6 mice mated to Act-mOVA mice are shown. Data are presented as mean ± SD (n = 6). *p < 0.01 vs the control group.
Adoptive transfer of Treg cells inhibits DT-induced pregnancy loss in Foxp3GFP mice
In order to investigate the role of Treg cells in pregnancy, pregnant Foxp3DTR mice were i.p. injected with DT to deplete Treg cells to set a murine inflammatory abortion model. Our results showed that 3 consecutive injections of DT at the dosage of 60 μg/kg weight almost completely deplete body Treg cells as demonstrated by nearly no Treg cells in the thymi, blood, LNs, spleens and deciduae without obvious maternal morbidity or mortality. Treatment with DT did not change total fetuses, but markedly increased the ERR in Foxp3DTR mice (p < 0.01) (Figure 6), suggesting an important role of Treg cells in maternal-fetal tolerance.
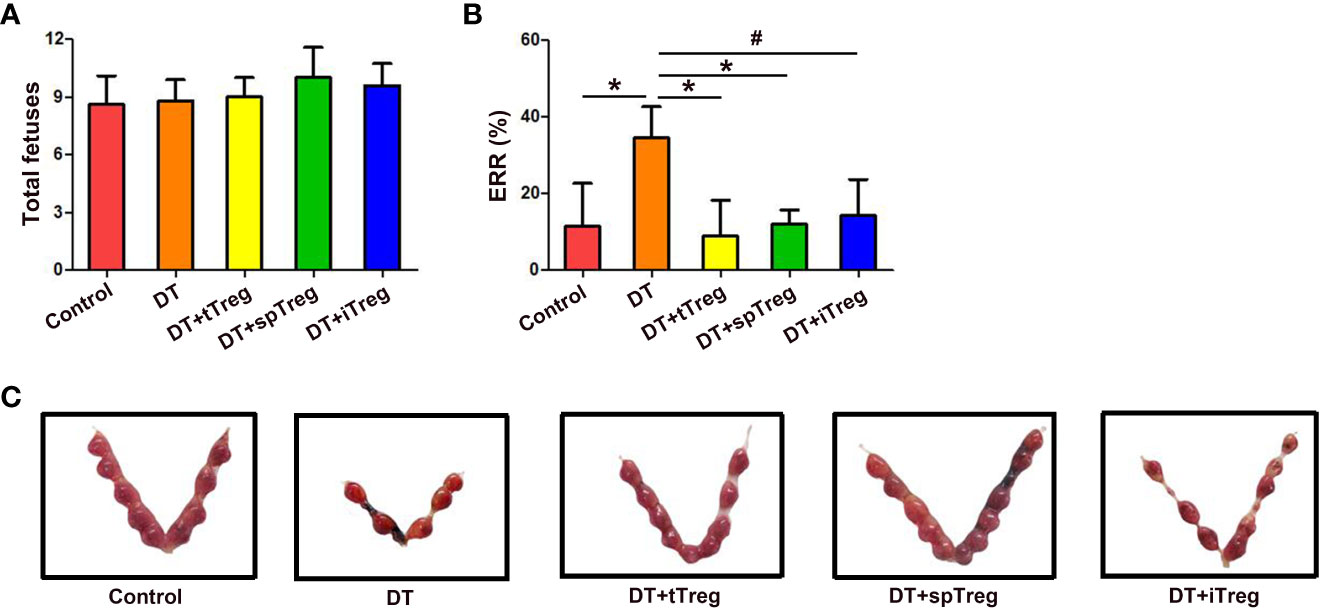
Figure 6 Adoptive transfer of Treg cells inhibits DT-induced pregnancy loss in Foxp3GFP mice. The number of total fetuses (A) and ERRs (B) were calculated. Data are presented as mean ± SD (n = 8). *p < 0.01, #p < 0.05. (C) A representative macroscopic view of fetuses from mice that received vehicle, DT, DT plus tTreg cells, DT plus spTreg cells, or DT plus iTreg cells is shown. spTreg, spleen Treg cells.
Meanwhile, we investigated whether thymus- or extrathymus-derived Treg cells play a role in pregnancy tolerance by adoptive transfer of Treg cells from different sources into DT-injected Foxp3DTR mice. As shown in Figure 6A, total fetuses did not differ among groups. Adoptive transfer of thymus (p < 0.01) or spleen CD4+GFP+ Treg cells (p < 0.01), or in vitro generated iTreg cells (p < 0.05) into DT-treated Foxp3DTR mice significantly decreased ERRs compared with DT-treated Foxp3DTR mice, which did not differ from those of vehicle-treated control mice (Figures 6B, C).
Effects of adoptive transfer of Treg cells on peripheral blood and decidua Th1 and Th17 cells in DT-treated Foxp3DTR mice
Since Th1 and Th17 cells play an important role in pregnancy loss (27), we wondered whether the immune tolerant effects by adoptive transfer of Treg cells were through inhibiting Th1 or Th17 cells. We found that treatment with DT significantly increased the percentages of peripheral blood IFN-γ+ cells and IL-17+ cells in CD45+CD3+CD4+ cells compared with controls (p < 0.01 for both comparisons, Figures 7A, B), indicating that deficiency of Treg cells increases systemic Th1 and Th17 cells. Compared with DT-treated Foxp3DTR mice, adoptive transfer of thymus or spleen CD4+GFP+ Treg cells significantly decreased the percentages of peripheral blood IFN-γ+ cells (p < 0.01 for both comparisons), which did not differ from those of vehicle-treated control mice (Figure 7A). In addition, compared with DT-treated Foxp3DTR mice, adoptive transfer of in vitro generated iTreg cells significantly decreased the percentages of peripheral blood IFN-γ+ cells (p < 0.01), which were still higher than those of vehicle-treated control mice (p < 0.01), and DT-treated Foxp3DTR mice with adoptive transfer of thymus Treg cells (p < 0.01) or spleen Treg cells (p < 0.05, Figure 7A).
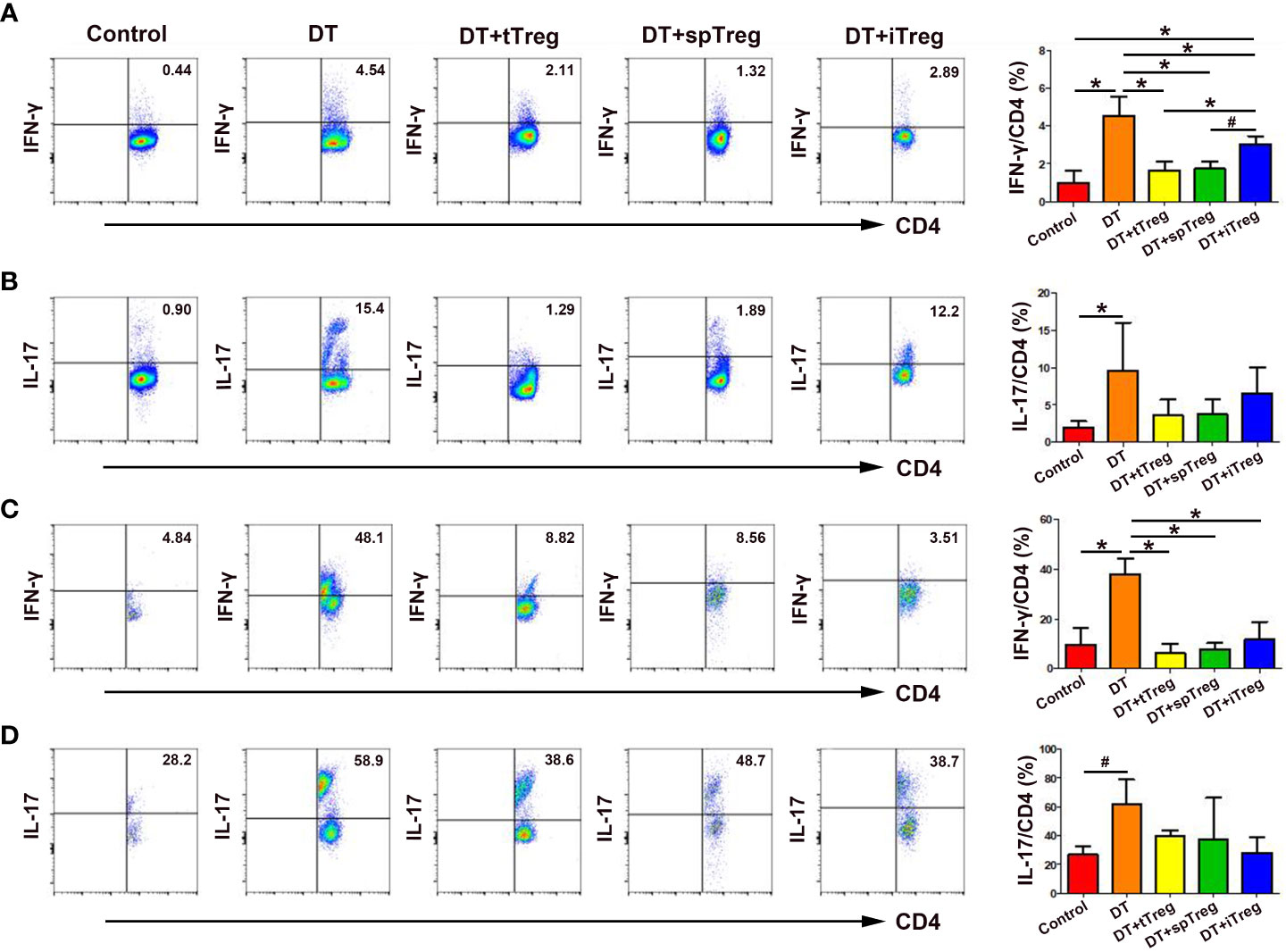
Figure 7 Effects of adoptive transfer of Treg cells on peripheral blood and decidua Th1 and Th17 cells in DT-treated Foxp3DTR mice. Representative flow cytometry experiments and levels of IFN-γ (A) and IL-17 (B) on peripheral blood CD4+ T cells are shown. Representative flow cytometry experiments and levels of IFN-γ (C) and IL-17 (D) on decidua CD4+ T cells are presented. Data are presented as mean ± SD (n = 6). *p < 0.01, #p < 0.05.
Similarly, treatment with DT significantly increased the percentages of decidua IFN-γ+ cells (p < 0.01) and IL-17+ cells (p < 0.05) in CD45+CD3+CD4+ cells compared with controls (Figures 7C, D), indicating that deficiency of Treg cells also increases maternal-fetal interface Th1 and Th17 cells. Compared with DT-treated Foxp3DTR mice, adoptive transfer of thymus and spleen Treg cells or in vitro generated iTreg cells significantly decreased the percentages of decidua IFN-γ+ cells (p < 0.01, respectively), which did not differ from those of vehicle-treated control mice (Figure 7C).Compared with DT-treated Foxp3DTR mice, adoptive transfer of thymus and spleen Treg cells or in vitro generated iTreg cells decreased the percentages of peripheral blood and decidua IL-17+ cells, but the differences did not reach statistical significance (Figures 7B, D).
Discussion
The genome of the fetus consists of both the tissue-specific antigens and paternally-inherited antigens (28). “Self”-antigens that are highly restricted to the fetus and placenta, are promiscuously expressed by medullary thymic epithelial cells under the control of Autoimmune Regulator (Aire) (29). Treg cells that circulate in mothers during pregnancy may be comprised of Tregs that are derived from the thymus as well as those are induced in the periphery. Exogenous fetal/placental antigens, including paternally-inherited major and minor histocompatibility antigens that differ between parents (30) may elicit elevated pTreg cells in the placenta. Indeed, significantly increased decidua Treg cells in allogeneic pregnant mice compared to syngeneic mice in our study suggests that at least part of increased decidua Treg cells may be derived from locally induced pTreg in response to fetus-specific paternally-inherited antigens in allogeneic mice. Similarly, scholars have reported that exogenous fetal/placental antigens induce the generation of pTreg in the decidua (25). In addition, extravillous trophoblast cells can induce Foxp3 expression in naive CD4+ T cells through a contact-independent manner, suggesting that a non-antigenic stimulation may induce pTreg cell generation in the decidua (31).
Most studies in mice agree that both syngeneic and allogeneic pregnancies involve early and local expansion of Treg cells, and an earlier and higher degree of expansion occurs in allogeneic pregnancies (16, 32, 33). In addition to locally induced pTreg cells in the decidua, up-regulated decidua Treg cells may also stem from tTreg cells since blood Treg cells in both syngeneic and allogeneic mice significantly increased at mid-gestation, and decidua Treg cells in syngeneic mice also significantly increased compared to non-pregnant mice. It has been reported that exposure of endometrium to seminal fluid induces expansion of Treg cells in the preimplantation mouse uterus (34). Accordantly, we found elevated Treg cells in the decidua as early as E4.5 in our study.
Until now, it has been difficult to directly prove the role of fetus-specific Treg cells in pregnancy tolerance due to the lack of direct manipulation of fetus-specific antigen (32). In our study, female C57BL/6 mice were mated to male Act-mOVA mice to generate a surrogate fetal antigen mOVA, which could be recognized by naïve T cells from OT-IIFoxp3GFP mice and induce the generation of pTreg cells in blood, spleens and deciduae. Similarly, Rowe et al. (35) have demonstrated that a substitute fetal antigen 2W1S55-66 induces Treg cell proliferation which remain resistant to the previous fetal antigen after delivery and rapidly expand in the second pregnancy. The progeny from C57BL/6 mice and BALB/C mice expresses peptide Eα55-66 derived from the MHC II molecule I-ED, which can be recognized by the TCR of transgenic TEa mice, and promote the transformation of T cells of TEa mice into Treg cells in a CNS1-dependent manner (25). Our results suggest that elevated decidua Treg cells could be derived from the transformation of naïve T cells in the decidua to pTreg cells after they recognize fetal antigens inherited from the father.
Part of increased decidua Treg cells may also originate from the recruitment of tTreg cells to the decidua. Elevated mRNA and protein levels of chemokines CCL2, CCL12 and CCL20 in the decidua and increased levels of chemokine receptors CCR2 and CCR6 on thymus and peripheral blood Treg cells indicates that Treg cells in the thymus could recruit to the decidua during pregnancy. We also found elevated mRNA but unchanged protein levels of CCL7 in the decidua of pregnant mice compared with non-pregnant mice in our study. The expression of CCL7 might be affected by various external and internal factors, including RNA interference, and showed no expression. In addition, our chemotaxis experiments have proved that chemokines CCL2, CCL12 and CCL20 do promote the migration of thymus Treg cells. Increased levels of decidual chemokines and chemokine receptors on thymus and peripheral blood Treg cells in allogeneic mice compared to syngeneic mice, suggests that paternally inherited antigens may also recruit tTreg cells to the decidua.
While pTreg cells play a role in maternal tolerance to the fetus (25), a possible role of central tolerance in pregnancy is less well understood and explored. Although CNS1-deleted transgenic mice were used to study the role of pTreg cells in pregnancy tolerance, deletion of the CNS1 region in Foxp3 did not completely abrogate Foxp3 induction (36). CNS1 deficiency in Foxp3 delays, but does not abrogate Treg cell selection (36). Therefore, we adoptively transferred of Treg cells from different sources into DT-injected Foxp3DTR mice to investigate their relative roles in maternal-fetal tolerance.
Interestingly, thymus Treg cells and spleen Treg cells that include thymus- and extrathymus-derived Tregs (12, 37) played a comparable role in decreasing DT- increased ERRs and IFN-γ levels in the blood and deciduae of Foxp3DTR mice. Unexpectedly, thymus Treg cells also had significant effects in suppressing immune activation in pregnancy. Indeed, it has been reported that pTreg cell deficiency induced by CNS1 depletion leads to a moderate increase of pregnancy loss in mice (25), indirectly indicating that tTreg cells may also play an important role in maternal-fetal tolerance.
Th17 cells are a newly discovered subset of Th cells different from Th1 and Th2 cells (38). They mainly secrete IL-17 and play an important role in pregnancy loss (27). In our study, transfer with thymus and spleen Treg cells, or iTreg cells decreased Th17 cells in DT-treated Foxp3DTR mice, but the differences did not reach statistical significance. Our data suggest that adoptive transfer of Treg cells may play a limited role in decreasing Th17 cells in DT-induced pregnancy loss model.
A number of studies have suggested that Foxp3+ Treg cells retain plasticity and can be “reprogrammed” into T helper cells under certain environmental conditions (39, 40). It has been demonstrated that in vitro induced iTreg cells have highly methylated Treg specific demethylation region (TSDR) and are prone to lose Foxp3 (41, 42). However, in our study, in vitro generated iTreg cells also demonstrated a role in inhibiting Treg cell deficiency-induced pregnancy loss, and blood and maternal-fetal immune activation. Since iTreg cells decreased ERRs and IFN-γ levels in the blood and decidua to an extent lower than thymus and spleen Treg cells, we consider that their effects may be weaker than those of tTreg cells and spleen Treg cells.
In conclusion, increased decidua Treg cells and DT-increased ERRs in Foxp3DTR mice suggest an immunosuppressive role of Treg cells in pregnancy. Elevated decidua Treg cells in pregnancy could be derived from the transformation of naïve T cells in the decidua to pTreg cells, or from the recruitment of tTreg cells to the decidua. The immune-suppression effects of tTreg cells and spleen Treg cells including both tTreg and pTreg cells are comparable. In vitro generated iTreg cells may also play a role in maternal-fetal tolerance, although the effects might be relatively weaker than those of tTreg cells and spleen Treg cells.
Data availability statement
The original contributions presented in the study are included in the article/supplementary material. Further inquiries can be directed to the corresponding authors.
Ethics statement
The animal study was reviewed and approved by the Animal Care and Use Committee of Jinan University.
Author contributions
ZL, XL and XC performed experiments and drafted the manuscript. YC, FW and SW analyzed and interpreted the data. LL was responsible for the conception and design of this project. LL and YL revised the manuscript and provided overall direction. All authors contributed to the article and approved the submitted version.
Funding
The work is supported by the National Nature Science Foundation of China (81871217) and Shenzhen Science and Technology Planning Project (JCYJ20220530151809021).
Acknowledgments
We thank all the members in our laboratory for their help, and thank Wei Ma for his technical support.
Conflict of interest
The authors declare that the research was conducted in the absence of any commercial or financial relationships that could be construed as a potential conflict of interest.
Publisher’s note
All claims expressed in this article are solely those of the authors and do not necessarily represent those of their affiliated organizations, or those of the publisher, the editors and the reviewers. Any product that may be evaluated in this article, or claim that may be made by its manufacturer, is not guaranteed or endorsed by the publisher.
References
1. Williams LM, Rudensky AY. Maintenance of the Foxp3-dependent developmental program in mature regulatory T cells requires continued expression of Foxp3. Nat Immunol (2007) 8(3):277–84. doi: 10.1038/ni1437
2. Brunkow ME, Jeffery EW, Hjerrild KA, Paeper B, Clark LB, Yasayko SA, et al. Disruption of a new forkhead/winged-helix protein, scurfin, results in the fatal lymphoproliferative disorder of the scurfy mouse. Nat Genet (2001) 27(1):68–73. doi: 10.1038/83784
3. Bennett CL, Christie J, Ramsdell F, Brunkow ME, Ferguson PJ, Whitesell L, et al. The immune dysregulation, polyendocrinopathy, enteropathy, X-linked syndrome (IPEX) is caused by mutations of FOXP3. Nat Genet (2001) 27(1):20–1. doi: 10.1038/83713
4. Whibley N, Gaffen SL. Brothers in arms: Th17 and treg responses in candida albicans immunity. PLoS Pathog (2014) 10(12):e1004456. doi: 10.1371/journal.ppat.1004456
5. Nadeau K, Jarvinen KM, Grishin A, Lindblad R, Sampson HA. Mechanistic correlates of clinical responses to omalizumab in the setting of oral immunotherapy for milk allergy. J Allergy Clin Immunol (2017) 140(4):1043–53.e8. doi: 10.1016/j.jaci.2017.03.028
6. Vudattu NK, Herold KC. Delayed anti-CD3 therapy in a mouse heart transplant model induced tolerance and long-term survival of allograft: achieving tolerance. Immunotherapy (2013) 5(11):1173–6. doi: 10.2217/imt.13.113
7. Abbas AK, Benoist C, Bluestone JA, Campbell DJ, Ghosh S, Hori S, et al. Regulatory T cells: recommendations to simplify the nomenclature. Nat Immunol (2013) 14(4):307–8. doi: 10.1038/ni.2554
8. Zheng SG, Wang J, Wang P, Gray JD, Horwitz DA. IL-2 is essential for TGF-β to convert naive CD4+CD25- cells to CD25+Foxp3+ regulatory T cells and for expansion of these cells. J Immunol (2007) 178(4):2018–27. doi: 10.4049/jimmunol.178.4.2018
9. Buckner JH. Mechanisms of impaired regulation by CD4+CD25+FOXP3+ regulatory T cells in human autoimmune diseases. Nat Rev Immunol (2010) 10(12):849–59. doi: 10.1038/nri2889
10. Hori S. Developmental plasticity of Foxp3+ regulatory T cells. Curr Opin Immunol (2010) 22(5):575–82. doi: 10.1016/j.coi.2010.08.004
11. Lal G, Bromberg JS. Epigenetic mechanisms of regulation of Foxp3 expression. Blood (2009) 114(18):3727–35. doi: 10.1182/blood-2009-05-219584
12. Thornton AM, Korty PE, Tran DQ, Wohlfert EA, Murray PE, Belkaid Y, et al. Expression of Helios, an ikaros transcription factor family member, differentiates thymic-derived from peripherally induced Foxp3+ T regulatory cells. J Immunol (2010) 184(7):3433–41. doi: 10.4049/jimmunol.0904028
13. Gottschalk RA, Corse E, Allison JP. Expression of Helios in peripherally induced Foxp3+ regulatory T cells. J Immunol (2012) 188(3):976–80. doi: 10.4049/jimmunol.1102964
14. Himmel ME, MacDonald KG, Garcia RV, Steiner TS, Levings MK. Helios+ and helios- cells coexist within the natural FOXP3+ T regulatory cell subset in humans. J Immunol (2013) 190(5):2001–8. doi: 10.4049/jimmunol.1201379
15. Szurek E, Cebula A, Wojciech L, Pietrzak M, Rempala G, Kisielow P, et al. Differences in expression level of Helios and neuropilin-1 do not distinguish thymus-derived from extrathymically-induced CD4+Foxp3+ regulatory T cells. PLoS One (2015) 10(10):e0141161. doi: 10.1371/journal.pone.0141161
16. Chen T, Darrasse-Jèze G, Bergot AS, Courau T, Churlaud G, Valdivia K, et al. Self-specific memory regulatory T cells protect embryos at implantation in mice. J Immunol (2013) 191(5):2273–81. doi: 10.4049/jimmunol.1202413
17. Heitmann RJ, Weitzel RP, Feng Y, Segars JH, Tisdale JF, Wolff EF. Maternal T regulatory cell depletion impairs embryo implantation which can be corrected with adoptive T regulatory cell transfer. Reprod Sci (2017) 24(7):1014–24. doi: 10.1177/1933719116675054
18. Mjösberg J, Berg G, Jenmalm MC, Ernerudh J. FOXP3+ regulatory T cells and T helper 1, T helper 2, and T helper 17 cells in human early pregnancy decidua. Biol Reprod (2010) 82(4):698–705. doi: 10.1095/biolreprod.109.081208
19. Du MR, Guo PF, Piao HL, Wang SC, Sun C, Jin LP, et al. Embryonic trophoblasts induce decidual regulatory T cell differentiation and maternal-fetal tolerance through thymic stromal lymphopoietin instructing dendritic cells. J Immunol (2014) 192(4):1502–11. doi: 10.4049/jimmunol.1203425
20. Santner-Nanan B, Peek MJ, Khanam R, Richarts L, Zhu E, Fazekas de St Groth B, et al. Systemic increase in the ratio between Foxp3+ and IL-17-producing CD4+ T cells in healthy pregnancy but not in preeclampsia. J Immunol (2009) 183(11):7023–30. doi: 10.4049/jimmunol.0901154
21. Zhao JX, Zeng YY, Liu Y. Fetal alloantigen is responsible for the expansion of the CD4+CD25+ regulatory T cell pool during pregnancy. J Reprod Immunol (2007) 75(2):71–81. doi: 10.1016/j.jri.2007.06.052
22. Aluvihare VR, Kallikourdis M, Betz AG. Regulatory T cells mediate maternal tolerance to the fetus. Nat Immunol (2004) 5(3):266–71. doi: 10.1038/ni1037
23. Teles A, Schumacher A, Kühnle MC, Linzke N, Thuere C, Reichardt P, et al. Control of uterine microenvironment by foxp3+ cells facilitates embryo implantation. Front Immunol (2013) 4:158. doi: 10.3389/fimmu.2013.00158
24. Zheng Y, Josefowicz S, Chaudhry A, Peng XP, Forbush K, Rudensky AY. Role of conserved non-coding DNA elements in the Foxp3 gene in regulatory T-cell fate. Nature (2010) 463(7282):808–12. doi: 10.1038/nature08750
25. Samstein RM, Josefowicz SZ, Arvey A, Treuting PM, Rudensky AY. Extrathymic generation of regulatory T cells in placental mammals mitigates maternal-fetal conflict. Cell (2012) 150(1):29–38. doi: 10.1016/j.cell.2012.05.031
26. Erlebacher A, Vencato D, Price KA, Zhang D, Glimcher LH. Constraints in antigen presentation severely restrict T cell recognition of the allogeneic fetus. J Clin Invest (2007) 117(5):1399–411. doi: 10.1172/JCI28214
27. Piccinni MP, Raghupathy R, Saito S, Szekeres-Bartho J. Cytokines, hormones and cellular regulatory mechanisms favoring successful reproduction. Front Immunol (2021) 12:717808. doi: 10.3389/fimmu.2021.717808
28. Ahn SH, Nguyen SL, Petroff MG. Exploring the origin and antigenic specificity of maternal regulatory T cells in pregnancy. Front Immunol (2020) 11:1302. doi: 10.3389/fimmu.2020.01302
29. Malchow S, Leventhal DS, Lee V, Nishi S, Socci ND, Savage PA. Aire enforces immune tolerance by directing autoreactive T cells into the regulatory T cell lineage. Immunity (2016) 44(5):1102–13. doi: 10.1016/j.immuni.2016.02.009
30. Linscheid C, Petroff MG. Minor histocompatibility antigens and the maternal immune response to the fetus during pregnancy. Am J Reprod Immunol (2013) 69(4):304–14. doi: 10.1111/aji.12075
31. Salvany-Celades M, van der Zwan A, Benner M, Setrajcic-Dragos V, Gomes HAB, Iyer V. Three types of functional regulatory T cells control T cell responses at the human maternal-fetal interface. Cell Rep (2019) 27(9):2537–47.e5. doi: 10.1016/j.celrep.2019.04.109
32. Xin L, Ertelt JM J, Rowe JH, TT J, JM K, Chaturvedi V, et al. Cutting edge: committed Th1 CD4+ T cell differentiation blocks pregnancy-induced foxp3 expression with antigen-specific fetal loss. J Immunol (2014) 192(7):2970–4. doi: 10.4049/jimmunol.1302678
33. Kahn DA, Baltimore D. Pregnancy induces a fetal antigen-specific maternal t regulatory cell response that contributes to tolerance. Proc Natl Acad Sci U S A (2010) 107(20):9299–304. doi: 10.1073/pnas.1003909107
34. Guerin LR, Moldenhauer LM, Prins JR, Bromfield JJ, Hayball JD, Robertson SA. Seminal fluid regulates accumulation of FOXP3+ regulatory T cells in the preimplantation mouse uterus through expanding the FOXP3+ cell pool and CCL19-mediated recruitment. Biol Reprod (2011) 85(2):397–408. doi: 10.1095/biolreprod.110.088591
35. Rowe JH, Ertelt JM, Xin L, Way SS. Pregnancy imprints regulatory memory that sustains anergy to fetal antigen. Nature (2012) 490(7418):102–6. doi: 10.1038/nature11462
36. Nutsch K, Chai JN, Ai TL, Russler-Germain E, Feehley T, Nagler CR, et al. Rapid and efficient generation of regulatory T cells to commensal antigens in the periphery. Cell Rep (2016) 17(1):206–20. doi: 10.1016/j.celrep.2016.08.092
37. Singh K, Hjort M, Thorvaldson L, Sandler S. Concomitant analysis of Helios and neuropilin-1 as a marker to detect thymic derived regulatory T cells in naïve mice. Sci Rep (2015) 5:7767. doi: 10.1038/srep07767
38. Crome SQ, Wang AY, Levings MK. Translational mini-review series on Th17 cells: function and regulation of human T helper 17 cells in health and disease. Clin Exp Immunol (2010) 159(2):109–19. doi: 10.1111/j.1365-2249.2009.04037.x
39. Bailey-Bucktrout SL, Martinez-Llordella M, Zhou X, Anthony B, Rosenthal W, Luche H, et al. Self-antigen-driven activation induces instability of regulatory T cells during an inflammatory autoimmune response. Immunity (2013) 39(5):949–62. doi: 10.1016/j.immuni.2013.10.016
40. Zhang Z, Zhang W, Guo J, Gu Q, Zhu X, Zhou X. Activation and functional specialization of regulatory T cells lead to the generation of Foxp3 instability. J Immunol (2017) 198(7):2612–25. doi: 10.4049/jimmunol.1601409
41. Ohkura N, Hamaguchi M, Morikawa H, Sugimura K, Tanaka A, Ito Y, et al. T Cell receptor stimulation-induced epigenetic changes and Foxp3 expression are independent and complementary events required for treg cell development. Immunity (2012) 37(5):785–99. doi: 10.1016/j.immuni.2012.09.010
Keywords: regulatory T (Treg) cells, tTreg cells, pTreg cells, iTreg cells, Foxp3, pregnancy, maternal-fetal tolerance
Citation: Li Z, Liang X, Chen X, Chen Y, Wang F, Wang S, Liao Y and Li L (2023) The role of thymus- and extrathymus-derived regulatory T cells in maternal-fetal tolerance. Front. Immunol. 14:1109352. doi: 10.3389/fimmu.2023.1109352
Received: 27 November 2022; Accepted: 24 January 2023;
Published: 02 February 2023.
Edited by:
Yin Tailang, Reproductive Medicine Center, Wuhan University, ChinaReviewed by:
Jie Zhou, Duke University, United StatesSurendra Sharma, Women & Infants Hospital of Rhode Island, United States
Copyright © 2023 Li, Liang, Chen, Chen, Wang, Wang, Liao and Li. This is an open-access article distributed under the terms of the Creative Commons Attribution License (CC BY). The use, distribution or reproduction in other forums is permitted, provided the original author(s) and the copyright owner(s) are credited and that the original publication in this journal is cited, in accordance with accepted academic practice. No use, distribution or reproduction is permitted which does not comply with these terms.
*Correspondence: Liping Li, doctorlipingli@foxmail.com; Yihong Liao, lyhfxr@sohu.com
†These authors have contributed equally to this work