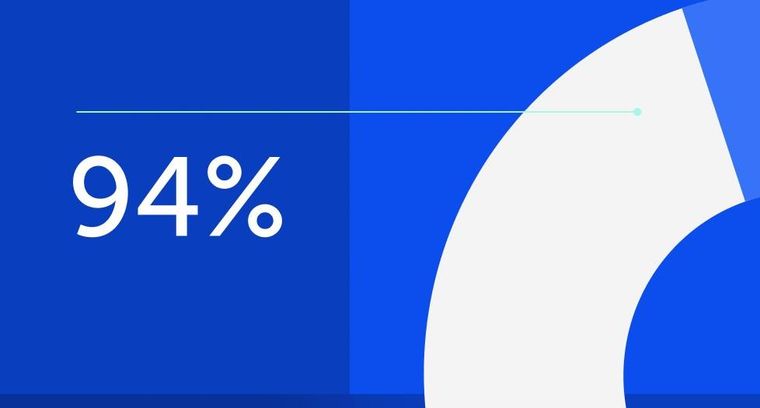
94% of researchers rate our articles as excellent or good
Learn more about the work of our research integrity team to safeguard the quality of each article we publish.
Find out more
ORIGINAL RESEARCH article
Front. Immunol., 08 February 2023
Sec. Autoimmune and Autoinflammatory Disorders : Autoimmune Disorders
Volume 14 - 2023 | https://doi.org/10.3389/fimmu.2023.1108116
This article is part of the Research TopicAutoimmune Complications of Modern Cancer TherapiesView all 8 articles
Background: Neurological immune-related adverse events (irAE-n) are severe and potentially fatal toxicities of immune checkpoint inhibitors (ICI). To date, the clinical significance of neuronal autoantibodies in irAE-n is poorly understood. Here, we characterize neuronal autoantibody profiles in patients with irAE-n and compare these with ICI-treated cancer patients without irAE-n.
Methods: In this cohort study (DRKS00012668), we consecutively collected clinical data and serum samples of 29 cancer patients with irAE-n (n = 2 pre-ICI, n = 29 post-ICI) and 44 cancer control patients without irAE-n (n = 44 pre- and post-ICI). Using indirect immunofluorescence and immunoblot assays, serum samples were tested for a large panel of neuromuscular and brain-reactive autoantibodies.
Results: IrAE-n patients and controls received ICI treatment targeting programmed death protein (PD-)1 (61% and 62%), programmed death ligand (PD-L)1 (18% and 33%) or PD-1 and cytotoxic T-lymphocyte-associated protein (CTLA-)4 (21% and 5%). Most common malignancies were melanoma (both 55%) and lung cancer (11% and 14%). IrAE-n affected the peripheral nervous system (59%), the central nervous system (21%), or both (21%). Prevalence of neuromuscular autoantibodies was 63% in irAE-n patients, which was higher compared to ICI-treated cancer patients without irAE-n (7%, p <.0001). Brain-reactive autoantibodies targeting surface (anti-GABABR, -NMDAR, -myelin), intracellular (anti-GFAP, -Zic4, -septin complex), or unknown antigens were detected in 13 irAE-n patients (45%). In contrast, only 9 of 44 controls (20%) presented brain-reactive autoantibodies before ICI administration. However, seven controls developed de novo brain-reactive autoantibodies after ICI initiation, therefore, prevalence of brain-reactive autoantibodies was comparable between ICI-treated patients with and without irAE-n (p = .36). While there was no clear association between specific brain-reactive autoantibodies and clinical presentation, presence of at least one of six selected neuromuscular autoantibodies (anti-titin, anti-skeletal muscle, anti-heart muscle, anti-LRP4, anti-RyR, anti-AchR) had a sensitivity of 80% (95% CI 0.52-0.96) and a specificity of 88% (95% CI 0.76-0.95) for the diagnosis of myositis, myocarditis, or myasthenia gravis.
Conclusion: Neuromuscular autoantibodies may serve as a feasible marker to diagnose and potentially predict life-threatening ICI-induced neuromuscular disease. However, brain-reactive autoantibodies are common in both ICI-treated patients with and without irAE-n, hence, their pathogenic significance remains unclear.
Targeting immune checkpoints with monoclonal antibodies against programmed death protein 1 (PD-1), programmed death-ligand 1 (PD-L1) or cytotoxic T-lymphocyte-associated protein 4 (CTLA-4) has been a breakthrough in the treatment of many malignancies (1–3). However, the benefits of immune checkpoint inhibitors (ICIs) are often mitigated by the development of autoimmune phenomena, referred to as immune-related adverse events (irAEs) (4, 5).
In particular, neurological irAEs (irAE-n) are an increasingly recognized complication (6) with mortality rates up to 35% and long-term sequelae in 40-68% of ICI-treated cancer patients (7–11). Common manifestations include encephalitis, myositis, myasthenia gravis (MG) and neuropathies, but every part of the nervous system can be affected (10–15). While the clinical phenotypes of irAE-n are well-described, little is known about the immunological mechanisms and potential biomarkers.
It has been proposed that ICI-induced immune activation may trigger paraneoplastic neurological disorders (PNDs) (16–18). In PNDs, autoantibodies are directed against antigens shared by the tumor and neural tissue and thereby cause – directly or mediated by cytotoxic T cells – off-target reactivity (19). However, it is unknown whether cross-reactivity is the only mechanisms to elicit ICI-induced neurotoxicity (11). As irAE-n can resemble classical, antibody-mediated neurological autoimmune disorders such as MG (20, 21), ICI-induced disruption of immune tolerance may be another decisive factor in the development of irAE-n.
If irAE-n were (partly) antibody-mediated diseases, screening of autoantibodies could help diagnosing and potentially predicting irAE-n. Moreover, treating irAE-n with B cell depletion or plasma exchange could be a valuable addition to the current standard therapy with corticosteroids (22). To further investigate the clinical significance of neuronal autoantibodies in irAE-n, we characterized neuronal autoantibody profiles in cancer patients with irAE-n in comparison to ICI-treated cancer patients without irAEs.
This cohort study was registered (DRKS00012668) and approved by the Ethics Committee of Charité Universitätsmedizin Berlin (EA1/099/17 and EA4/219/21). Written informed consent to participate in this study was obtained from all patients prior to any study procedures. The study was conducted at the Charité Universitätsmedizin Berlin between September 2017 and January 2022.
We recruited all consecutive patients with irAE-n that met the following inclusion criteria: Cancer patients over 18 years old that (1) received ICI treatment and (2) were diagnosed with an irAE-n according to the consensus criteria of “probable” or “definite” irAE-n as defined previously (23). One additional patient was included who had preexisting MG which deteriorated to a myasthenic crisis after ICI treatment initiation. In one patient, treatment was double-blinded for ICI therapy versus (vs.) placebo, but the patient developed multiple autoimmune phenomena, hence, ICI treatment was highly probable. Three investigators (LMJ, SK, PH) confirmed the diagnosis of irAE-n.
Age-, sex-, ICI- and tumor-matched adult cancer patients that were scheduled for ICI treatment were enrolled as controls. We excluded control patients with previous ICI treatment within the last six months and – as most immunotoxicities occur early after ICI treatment onset - patients that developed any severe (Common Terminology Criteria for Adverse Events [CTCAE] grade ≥3) neurological or non-neurological irAEs within the first three months of treatment. A CONSORT diagram of the study is shown in Figure 1.
Figure 1 CONSORT diagram. Between September 2017 and January 2022, a total of 86 cancer patients were enrolled for the study. After application of inclusion and exclusion criteria, 73 patients were included. As two patients that were originally allocated to the control cohort developed irAE-n, a total of 29 irAE-n patients and 44 cancer control patients were analyzed. CSF, cerebrospinal fluid; ICI, immune checkpoint inhibitor; irAEs, immune related adverse events; irAE-n, neurological immune related adverse event.
We collected the following clinical variables: Demographics (age, sex), tumor entity, neurological comorbidities, brain metastases (yes or no), ICI type, ICI cycle, type of irAE-n, treatment of irAE-n, other irAE and rechallenge of ICI. Charts and routine care data of all patients were reviewed to score the following outcome parameters retrospectively: CTCAE grade of irAE-n at three months after symptom onset (grade 1 to 5) and steroid treatment at three months after symptom onset (yes or no).
Blood serum samples of all patients with irAE-n were collected during the acute disease stage. In addition, nine cerebrospinal fluid (CSF) samples that were assessed during the routine diagnostic workup were included. From controls, blood serum samples were collected before (baseline) and six weeks (IQR, 6-8) after ICI treatment initiation. At the time of the second study visit, patients had received a median of two ICI infusions (IQR, 2-2). As irAE-n are rare and neurological consultation usually takes place when neurological symptoms are already present, assessment of pretreatment samples from irAE-n patients is difficult. However, two patients that were originally allocated to our control cohort developed neurotoxicities (2/46, 4%), therefore, two pretreatment irAE-n samples could be analyzed.
All serum samples were tested for brain-reactive IgG autoantibodies targeting the following antigens: α-amino-3-hydroxy-5-methyl-4-isoxazolepropionic acid receptor 1/2 (AMPAR1/2), amphiphysin, aquaporin 4 (AQP4), RhoGTPase-activating protein 26 (ARHGAP26), ATP1A3, carbonic anhydrase related proteins VIII (CARP VIII), contactin-associated protein-like 2 (CASPR2), collapsin response-mediator protein 5 (CV2/CRMP5), dipeptidyl-peptidase-like protein 6 (DPPX), Flotillin1/2, gamma-aminobutyric-acid A receptor (GABAAR), gamma-aminobutyric-acid B receptor (GABABR), glutamic acid decarboxy lase 65 (GAD65), glial fibrillary acidic protein (GFAP), glutamate receptor delta 2 (GluD2), glycine receptor (GlyR), Homer protein homolog 3 (Homer-3), Hu (Anna-1), immunoglobulin LON5 (IgLON5), inositol 1,4,5-trisphosphate receptor 1 (ITPR-1), leucine-rich glioma-inactivated 1 (LGI1), Ma2, metabotropic glutamate receptor 1 (mGluR1), metabotropic glutamate receptor 5 (mGluR5), myelin oligodendrocyte glycoprotein (MOG), myelin, anti-neuroendothelium, neurexin, neurochondrin, N-methyl-D-aspartate receptor (NMDAR), recoverin, Ri (Anna-2), septin complex, Tr (DNER), Yo (PCA-1), and zinc finger 4 (Zic4). In addition, IgA and IgM NMDAR autoantibodies were tested.
During clinical routine, eight of nine available CSF samples were analyzed for IgG autoantibodies directed against the following antigens: Amphiphysin, AQP4, CASPR2, DPPX, GAD65, GABABR, AMPAR1/2, NMDAR, mGluR5, GlyR, LGI1, myelin, CV2/CRMP5, Hu, Ma2, Ri, Tr, Yo. One CSF sample was only tested for eight of the above-mentioned IgG autoantibodies as only small amounts of CSF were available (amphiphysin, CV2/CRMP5, GAD65, Hu, Ma2, Ri, Tr, Yo).
Twenty-four of 29 and 41 of 44 patients with and without irAE-n, respectively, were additionally tested for IgG neuromuscular autoantibodies targeting the following antigens: Acetylcholine receptor (AchR), heart muscle, skeletal muscle, lipoprotein receptor-related protein 4 (LRP4, only tested in n = 40 controls), myelin-associated glycoprotein (MAG), muscle-specific tyrosine kinase (MuSK), P/Q-type voltage-gated calcium channel (P/Q VGCC, only tested in n = 40 controls), ryanodine receptor (RyR, only tested in n = 40 controls), SRY-related HMG-box 1 (SOX1), and titin. Specifications of all analyzed autoantibodies are described in Supplemental Table 1.
Detection of brain-reactive autoantibodies was performed using commercial assays (all EUROIMMUN Medizinische Labordiagnostika AG, Germany) including cell-based assays (CBA) and immunohistochemistry (IHC) of frozen brain tissue (rat hippocampus, rat cerebellum, and monkey cerebellum). To that end, indirect immunofluorescence using BIOCHIP mosaics™ was performed as described previously (24). To confirm CBA or IHC results for intracellular antigens, immunoblot assays (EUROLINE) were additionally performed. If autoantibodies were detected via IHC, but CBA and EUROLINE were negative, they were considered autoantibodies of unknown reactivity.
Neuromuscular autoantibodies were tested with the following commercial assays (all Labor Berlin GmbH, Germany): Anti-titin and anti-SOX1 autoantibodies using line assays; anti-LPR4, anti-skeletal muscle and anti-heart muscle autoantibodies using indirect immunofluorescence; anti-MAG, anti-MuSK, and anti-AchR autoantibodies using enzyme linked immunosorbent assays; anti-RyR autoantibodies using western blot and anti-P/Q VGCC autoantibodies using radioimmunoassays. To enhance sensitivity, we considered weakly positive or borderline positive results as positive.
Continuous and categorical variables were reported as median (interquartile range, [IQR]) and numbers (percentage), respectively. Group differences of unpaired categorical data were analyzed using the Chi-squared test or Fisher’s exact test. Comparison of paired categorical data (baseline vs. six weeks after ICI treatment onset) was conducted using the McNemar test. Group differences of continuous variables were calculated using the Mann-Whitney U test. Sensitivity, specificity, and 95% confidence intervals (CI) were calculated using the “EpiR” R package to investigate the diagnostic accuracy for specific autoantibodies. We considered an alpha-level of ≤ 0.05 as statistically significant. Graphpad Prism (version 7) and RStudio (version 2022.02.3 + 492 “Prairie Trillium”) were used for graph illustration and statistical analysis.
Twenty-nine cancer patients who were diagnosed with irAE-n and 44 ICI-treated cancer patients without high-grade (CTCAE ≥ 3) irAEs were included. Patients’ demographics and clinical characteristics are summarized in Table 1.
Prevalence of neuromuscular autoantibodies was 17% (7/41 tested patients) in control patients before ICI treatment (Figure 2A). Of these, three were only borderline positive; one for anti-PQ VGCC autoantibodies (31 pmol/l; upper reference limit, 30 pmol/l) and two for anti-AchR autoantibodies (0.4 nmol/l; upper reference limit, 0.4 nmol/l). In contrast, six weeks after ICI treatment initiation (equal to two ICI infusions) neuromuscular autoantibodies were detected in only three controls (7%, p = <.0001): In t wo of the seven patients that were initially tested positive a nd in one additional patient with de novo anti-LRP4 autoantibodies.
Figure 2 Serum autoantibody profiles in cancer patients with irAE-n and controls. (A) Frequency and specification of neuromuscular autoantibodies of 41 cancer patients before (upper left) and six weeks after (middle left) ICI treatment initiation who did not develop high-grade (= CTCAE ≥ 3) irAEs and 24 ICI-treated cancer patients with irAE-n (lower left). (B) Frequency and specification of brain-reactive autoantibodies of 44 cancer patients before (upper right) and six weeks after (middle right) ICI treatment initiation who did not develop high-grade (CTCAE ≥ 3) irAEs and 29 ICI-treated cancer patients with irAE-n (lower right). Ab, autoantibody; AchR, acetylcholine receptor; CASPR2, contactin-associated protein-like 2; GABABR, gamma-aminobutyric-acid B receptor; GFAP, glial fibrillary acidic protein; Homer-3, Homer protein homolog 3; ICI, immune checkpoint inhibitor; irAEs, immune related adverse events; irAE-n, neurological immune related adverse event; ITPR1, inositol 1,4,5-trisphosphate receptor 1; LRP4, low-density lipoprotein receptor-related protein 4; MuSK, muscle-specific tyrosine kinase; NMDAR, N-methyl-D-aspartate receptor; P/Q VGCC, P/Q-type voltage gated calcium channel; RyR, ryanodine receptor; Zic4, zinc finger 4.
Interestingly, one of these patients presented anti-titin and anti-RyR autoantibodies pre- and post-ICI treatment and reported “muscle weakness” and “exhaustion” at the last routine follow up. Unfortunately, the patient was in palliative care, therefore no further examinations were initiated.
Before ICI treatment, nine of 44 control patients (20%) were tested positive for brain-reactive autoantibodies (Figure 2B). In two patients two different brain-reactive autoantibodies were detected (anti-Zic4 [1:32000] and anti-CV2/CRMP5 [1:32]; anti-Homer-3 [1:1000] and IgA anti-NMDAR [1:100]). Two of nine patients with brain-reactive autoantibodies had diagnosed brain metastases, none had history of herpetic encephalitis or autoimmune disease of the central nervous system (CNS) as potential triggers of brain-reactive autoantibodies.
Interestingly, we noticed a de novo development of brain-reactive autoantibodies after ICI treatment initiation: Seven patients who were initially tested negative had developed brain-reactive autoantibodies after two ICI infusions (Figure 2B). In contrast, only one patient with brain-reactive autoantibodies at baseline (ITPR3, 1:320) was tested negative at the second visit, so the prevalence of brain-reactive autoantibodies was higher after ICI treatment initiation compared to baseline (34%, p =.002). Of patients with de novo autoantibodies, one (14%) had autoantibodies against intracellular antigens (anti-GFAP [1:320]), four (57%) had autoantibodies against surface antigens (IgM anti-NMDAR [1:320]; anti-CASPR2 [1:32]; anti-neuroendothelial autoantibodies [1:1000 and 1:320]) and two (29%) had autoantibodies of unknown reactivity. One additional patient showed seroconversion from IgM anti-NMDAR (1:10) to IgA anti-NMDAR (1:10). De novo Development of brain-reactive autoantibodies was not associated with a specific neoplasm (four cases of melanoma, two cases of non-small cell lung cancer [NSCLC], one case of hepatocellular carcinoma), ICI type (four cases with nivolumab treatment [PD-1 inhibitor], three cases with atezolizumab treatment [PD-L1 inhibitor]), or neurological symptoms.
Of 29 patients, irAE-n affected the CNS in six (encephalitis, hypophysitis), the peripheral nervous system (PNS) in 17 (neuropathy, myositis, MG) and both in another six patients (Table 1). A detailed cohort description can be found in Supplemental Table 2.
The prevalence of neuromuscular autoantibodies was 63% (15/24; Figure 2A) in patients with any irAE-n and 80% (12/15) in patients with either ICI-induced myositis, myocarditis, or MG. Compared to controls, neuromuscular autoantibodies were significantly more common in patients with irAE-n (63% vs. 7%; p = <.0001).
Detected autoantibodies in patients with myositis, myocarditis or MG were anti-heart muscle (six cases), anti-skeletal muscle (six cases), anti-titin (five cases), anti-RyR (four cases), anti-AchR (three cases), and anti-LRP4 (one case) autoantibodies. Eight patients (67%) had more than one neuromuscular autoantibody (Supplemental Table 2).
As two patients that were originally allocated to our control cohort developed irAE-n (2/46, 4%), two pretreatment irAE-n samples could be analyzed. One sample revealed preexisting, high-titer anti-titin, anti-heart muscle and anti-skeletal muscle autoantibodies in an 86-year-old female patient with melanoma, who died of fulminant myocarditis and myositis after one ICI infusion (patient no. 11 in Supplemental Table 2). Autopsy was performed and demonstrated invasion of predominantly macrophages and cytotoxic T cells as well as an overexpression of major histocompatibility complex class I and II in necrotic cardiac (Figures 3A-C) and skeletal muscle tissues (Figures 3D-F). The other sample was from a 52-year-old female, who was diagnosed with peripheral facial nerve palsy. Pre-ICI treatment no neuromuscular autoantibodies were detected, but after two ICI cycles the patient was tested positive for low-titer anti-AchR autoantibodies (patient no. 29 in Supplemental Table 2).
Figure 3 Histopathological and clinical findings in patients with ICI-induced myositis. (A-F) Hematoxylin-eosin (HE) and immunohistochemical staining in a female patient with fatal ICI-induced myositis and myocarditis and positive anti-titin, anti-heart muscle and anti-skeletal muscle autoantibodies. Frozen sections of HE-stained (A) cardiac muscle (D) and skeletal muscle showing necrotic myofibers and lymphocyte infiltration (original magnification x 100). CD8 (large panel, original magnification x 200) and major histocompatibility complex (MHC) class I (inset, original magnification x 100) staining revealing cytotoxic T cell invasion and sarcolemmal overexpression of MHC class I by (B) cardiac and (E) skeletal muscle fibers. CD68 (large panel, original magnification x 200) and MHC class II (inset, original magnification x 100) staining showing macrophage invasion and sarcolemmal as well as sarcoplasmic overexpression of MHC class II by (C) cardiac and (F) skeletal muscle fibers. (G) Comparison of peripheral blood creatine kinase (CK) levels in patients with ICI-induced myositis shows a trend towards higher CK levels in patients with multiple neuromuscular autoantibodies compared to patients without neuromuscular autoantibodies. (H) Sagittal and (I) axial contrast-enhanced magnetic resonance imaging of the lumbar spine with T1-weighted turbo spin echo sequence showing paravertebral contrast enhancement (arrows) as a sign of muscle edema in a patient with ICI-induced myositis and myasthenia. Ab, autoantibody; ICI, immune checkpoint inhibitor; U/l, units per liter.
To investigate the diagnostic accuracy of neuromuscular autoantibodies in patients with ICI-induced myositis, myocarditis, or MG, we selected the six most abundant autoantibodies (anti-heart muscle, anti-titin, anti-skeletal muscle, anti-RyR, anti-AchR and anti-LRP4) and compared detection of either of these autoantibodies between cancer patients with and without ICI-induced neuromuscular disease. Sensitivity and specificity were high with 80% (95% CI 0.52-0.96) and 88% (95% CI 0.76-0.95), respectively. The positive and negative predictive values were 67% (95% CI 0.41-0.87) and 94% (95% CI 0.82-0.99), respectively. Six of 65 ICI-treated cancer patients (9%) had positive neuromuscular autoantibodies but no diagnosed myositis, myocarditis, or MG. Three of these six patients had ICI-induced neuropathy (patient no. 23, no. 28, and no. 29 in Supplemental Table 2). Conversely, in three patients with myopathy no neuromuscular autoantibodies were detected. However, all of them presented only mild symptoms; one with normal creatine kinase [CK] levels and all with only discrete myopathic changes in the electromyographic activity.
Interestingly, CK levels tended to be higher in patients with myositis or myocarditis and multiple neuromuscular autoantibodies compared to patients with none or only one neuromuscular autoantibody (Figure 3G). Regarding clinical characteristics such as age, sex, neoplasm, or ICI type, we did not observe differences between irAE-n patients with and without neuromuscular autoantibodies (Table 1 and Supplemental Table 3).
The frequency of brain-reactive autoantibodies in patients with irAE-n was 45% (13/29), which was only slightly higher compared to ICI-treated cancer patients without high-grade irAEs (45% vs. 34%, p = .36; Figure 2B and Supplemental Table 2). In four patients two different brain-reactive autoantibodies were detected (IgG anti-NMDAR [1:10] and anti-GABABR [1:10]; anti-Zic4 [1:32000] and anti-GABABR [1:10, Figures 4A, B]; IgA anti-NMDAR [1:32] and IgM anti-NMDAR [1:100]; IgA anti-NMDAR [1:320] and IgM anti-NMDAR [1:10]). We did not find any brain-reactive autoantibodies in nine additionally tested CSF samples (Supplemental Table 2).
Figure 4 Brain-directed autoantibodies in ICI-treated cancer patients with irAE-n. (A, B) Cell-based assays of fixed recombinant HEK293-cells (original magnification x 200) expressing single neuronal antigens showing anti-Zic4 autoantibodies (1:100 dilution; A) and anti-GABABR autoantibodies (1:10, B) in a patient with small cell lung cancer and Guillain-Barré syndrome (GBS) after pembrolizumab treatment. However, anti-ganglioside autoantibodies, which are associated with GBS, were tested negative during clinical routine. (C) Tissue-based assay using indirect immunofluorescence screening on monkey cerebellum sections (original magnification x 200) showing anti-GFAP autoantibodies (1:100 dilution) in a melanoma patient with encephalitis after treatment with ipilimumab and nivolumab. GABABR, gamma-aminobutyric-acid B receptor; GFAP, glial fibrillary acidic protein; ICI, immune checkpoint inhibitor; irAE-n, neurological immune related adverse event; Zic4, zinc finger 4.
The two analyzed pretreatment samples revealed de novo anti-GABABR autoantibodies in a female patient with peripheral facial nerve palsy (while IgG anti-NMDAR autoantibodies were detected both pre- and post-ICI treatment; patient no. 29 in Supplemental Table 2) and no brain-reactive autoantibodies (neither pre- nor post-ICI treatment) in a female patient with myositis (patient no. 11 in Supplemental Table 2).
Comparison of clinical syndrome and autoantibody type indicated a low correlation: Of seven patients with ICI-induced encephalitis three were tested positive for brain-reactive autoantibodies (43%), but only one patient (14%) presented symptoms that matched the autoantibody-associated syndrome. This 81-year-old male patient (patient no. 4 in Supplemental Table 2) presented with disorientation, confusion and memory deficits and was tested positive for anti-GFAP autoantibodies in the serum (1:320; Figure 4C). CSF showed mononuclear pleocytosis with 51 cells per microliter and CSF protein of 1234 mg/l. Brain MRI was normal. The other two patients with encephalitis had IgA anti-NMDAR autoantibodies (patient no. 7 in Supplemental Table 2) and IgA and IgM anti-NMDAR autoantibodies (patient no. 9 in Supplemental Table 2). All other patients with irAE-n and positive autoantibodies had PNS manifestation or hypophysitis without any association to brain-reactive autoantibodies. There were no significant differences between patients with and without brain-reactive autoantibodies regarding age, sex, neoplasm, or ICI type (Table 1 and Supplemental Table 3).
Three patients (10%) died of the irAE-n (Table 1). Median CTCAE grade at three months after symptom onset was 2 (IQR, 2-3). Of 24 patients with available data, 22 (92%) still received steroid treatment three months after symptom onset. Unfavorable outcome (defined as CTCAE ≥ 3 at three months after symptom onset) was observed in 13 patients in total (45%), in four of 13 patients with brain-reactive autoantibodies (31%) and in nine of 16 patients without brain-reactive autoantibodies (56%, p =.17). Likewise, presence of neuromuscular autoantibodies was not associated with unfavorable outcome (53% vs. 44% for patients with and without neuromuscular autoantibodies, respectively, p = .67). Two patients with myositis and/or MG (patient no. 3 and patient no. 22 in Supplemental Table 2 and Figures 3H, I) were rechallenged with ICI treatment during follow-up, both developed a flare of myositis.
In this study, we characterized neuronal autoantibody profiles of 73 cancer patients with and without ICI-induced irAE-n and thereby provide new insights into ICI-induced autoimmunity: (1) Neuronal autoantibodies are common in patients with irAE-n, (2) sensitivity and specificity of neuromuscular autoantibodies is high for the diagnosis of ICI-induced myositis, myocarditis and MG, (3) ICI treatment may induce the production of brain-reactive autoantibodies, however, (4) development of brain-reactive autoantibodies is not necessarily associated with neurological symptoms.
With an incidence of 0.7-1% and a mortality rate of up to 35%, ICI-induced myositis and MG are among the most common and most threatening irAE-n (10, 14). Clinical presentation may be confusing with symptoms typically attributable to different disease entities (e.g., dropped head syndrome), complicating diagnosis and treatment (25, 26). Anti-AchR autoantibodies and striational autoantibodies (anti-titin, anti-heart muscle, and anti-skeletal muscle autoantibodies) have been described in both ICI-induced MG and myositis (21, 26–30), while myositis-specific autoantibodies (e.g., anti-Jo-1, anti-Mi-2 or anti-signal recognition particle autoantibodies) are mostly negative (31, 32). In a cohort of 24 patients with ICI-induced myositis, striational autoantibodies were found to be most common (8/17 [47%] striational autoantibodies vs. 3/17 [18%] for anti-AchR autoantibodies and 0/13 [0%] for myositis-specific autoantibodies, respectively) (32). We detected an even higher prevalence (80%) of neuromuscular autoantibodies in patients with ICI-induced neuromuscular disease and demonstrate that these autoantibodies are rare (7%) in ICI-treated patients without neurotoxicity.
Nevertheless, the immunological relevance of neuromuscular autoantibodies in these fulminant toxicities is still poorly understood. While anti-AchR autoantibodies are directly pathogenic in classical MG (33, 34), striational autoantibodies are more likely to be an immunological epiphenomenon because of their intracellular localization (35, 36). In fact, in our patient with fatal myositis and preexisting striational autoantibodies, CD8+ T cell infiltration and enhanced major histocompatibility complex expression was detected in both cardiac and skeletal muscle tissue. Others, too, reported invasion of cytotoxic T-cells and macrophages into muscular tissue of patients with anti-PD-1/PD-L1-associated myopathy and discovered expression of muscle-specific transcripts in tumor specimens via whole-transcriptome sequencing (31, 37). Intriguingly, Axelrod et al. recently identified α-myosin as one autoantigen which is targeted by self-reactive T cells in ICI-induced myocarditis (38). Consequently, it is possible that in patients with ICI-induced neuromuscular disease and striational autoantibodies, T cell-mediated mechanisms dominate, while in those with anti-AchR autoantibodies the autoantibody itself is pathogenic. As this may have therapeutical implications (T cell targeted therapies vs. B cell depletion or plasma exchange), future studies are warranted to investigate optimal treatment approaches in patients with ICI-induced autoantibody-positive neuromuscular disease.
Even though the pathogenicity of neuromuscular autoantibodies is only partly elucidated, their potential as diagnostic markers is evident. Whether autoantibodies are also feasible to predict irAE-n, however, is unclear. ICI-induced myositis and MG may occur early after treatment onset, with some patients developing symptoms after the first ICI infusion (27, 31). In our cohort, one patient presented myositis within one week after first ICI cycle. Early occurrence of irAE-n could point towards a preexisting subclinical autoimmune condition, as the priming of antigen-specific T cells requires days to weeks (39, 40). This hypothesis is supported by the detection of preexisting striational autoantibodies in four other patients with ICI-induced myositis, which all developed symptoms after the first ICI infusion (n = 1) or within 15 days after ICI treatment initiation (n = 1 after 8 days, n = 2 after 15 days), respectively (27, 41). Considering other irAEs, a recent study with 137 patients reported a strong association of preexisting rheumatic autoantibodies and anti-thyroid autoantibodies with the development of irAEs and improved tumor response (42). A study that systematically investigates the diagnostic performance of common rheumatic autoantibodies (antinuclear autoantibodies, rheumatoid factor, and antineutrophil cytoplasmic autoantibodies) to predict irAEs is currently recruiting (43). As neuromuscular irAE-n are particularly threatening immunotoxicities, screening of neuromuscular autoantibodies prior to ICI treatment may also be beneficial.
In addition, identifying clinical risk factors that are associated with ICI-induced neuromuscular disease is paramount. In our cohort, neuromuscular autoantibodies occurred in the context of both PD-1/PD-L1 monotherapy and ICI combination treatment and was not associated with specific malignancies or outcome. However, development of self-reactive T cells may be dependent on patient-specific characteristics such as tumor antigen repertoire and HLA-type (44, 45), so future research should emphasize histopathological and genetic studies.
We detected brain-reactive autoantibodies in 45% of ICI-treated cancer patients with irAE-n. Similarly, in a clinical cohort of 13 patients with irAE-n, 54% of patients had detectable neuronal autoantibodies (30). In contrast, recent studies showed a significantly lower prevalence of brain-reactive autoantibodies in cancer patients overall (25%) and healthy controls (2.5%) (46). However, most autoantibody-positive patients in our cohort did not develop the clinical syndrome typically associated with the detected autoantibody. Only one patient had symptoms consistent with GFAP-associated autoimmunity. Likewise, Sechi et al. reported that the minority of autoantibody-positive patients with irAE-n (6 of 31) presented as “classical” PNDs (30).
On the other hand, ICI-induced PNDs with typical clinical presentation and autoantibodies have been described (18) and it is known that preexisting PNDs worsen after ICI administration (30). Therefore, the pathogenicity of brain-reactive autoantibodies and the role of cancer neoantigens in patients with irAE-n of the CNS is being discussed controversially (11, 16).
In our cohort, 11 of 13 patients with irAE-n and detectable autoantibodies had autoantibodies against myelin, septin complex, NMDAR (IgA or IgM isotype), or an unknown antigen. These autoantibodies are not tested regularly in commercially available assays and their significance is unclear. In fact, in a large cohort of 300 patients, only IgG anti-NMDAR autoantibodies were associated with anti-NMDAR encephalitis, while IgA and IgM anti-NMDAR autoantibodies occurred nonspecifically in diseases like stroke or dementia (47). Otherwise, IgA anti-NMDAR autoantibodies have been linked to progressive cognitive dysfunction, a relevant decrease in NMDAR levels, and prominent changes in NMDAR-mediated currents (48). A strong association of cognitive impairment and brain-reactive autoantibodies – including IgA anti-NMDAR autoantibodies – has been shown in patients with lung cancer and melanoma (24, 46). As the patients in our cohort did not receive systematic neuropsychological testing, it is possible that more subtle cognitive impairment was missed or attributed to comorbidities within primary care.
Interestingly, we discovered a de novo development of brain-reactive but not neuromuscular autoantibodies in seven patients after anti-PD-1 or anti-PD- L1 administration. Even though four of seven patients (57%) developed surface-reactive autoantibodies, which can have a direct pathogenic effect on neuronal antigens, none of the patients were later diagnosed with irAE-n. Even though the specific mechanisms remain elusive, these findings indicate ICI-induced and possibly organ-specific disruption of immune tolerance also in ICI-treated patients without clinical signs of autoimmunity. Future studies with larger cohorts are needed to systematically assess cognitive function in antibody-positive patients and to identify risk factors for the clinical emergence of PNDs.
The mortality of irAE-n was 10% in our cohort. Ninety-two percent of patients still received steroids at three months after onset, implying a high risk for long-term adverse events like Cushing’s syndrome, secondary hypopituitarism and reduced antitumor immunity. A recent study with 387 melanoma patients reported chronic irAEs in 43% of patients (49). Together with endocrinopathies, neurotoxicities were most likely to transition to chronic illness. Hence, clinicians should be aware of irAE-n and initiate careful neurological evaluation in all cases of new-onset muscle weakness, dysphagia or dysarthria, hypo- or dysesthesia, disorientation, or cognitive impairment in ICI-treated patients.
In our cohort, every third patient with irAE-n had multiple neurotoxicities. Neuromuscular autoantibodies were also detected in patients with ICI-induced neuropathy. It is possible, that irAE-n - and in particular irAE-n of the PNS - constitute a disease continuum, so clinicians should be vigilant about overlap syndromes with potentially inferior prognosis. In contrast to a previous study on ICI-induced encephalitis (50), however, we and others (30) did not observe an association between neuronal autoantibodies and poor outcome. Taking into account that autoantibody-positive irAE-n might respond to non-steroid treatment approaches (e.g., plasma exchange, intravenous immune globulins, or rituximab), which are less likely to mitigate antitumor response (7, 20, 22), positive autoantibody status may in fact be associated with better outcome in certain cases.
To our knowledge, this is the first systematic assessment of a large panel of neuronal autoantibodies in cancer patients with and without irAE-n before and after ICI treatment initiation, providing valuable information for clinicians and researchers. Together with others (27, 42), our findings provide the groundwork for pretreatment autoantibody screening to improve ICI safety.
However, several limitations must be acknowledged. First, the small sample size does not allow for definite conclusions on the prevalence of neuronal autoantibodies in ICI-treated cancer patients. Second, our observation time was only three months. We do not know whether controls developed irAE-n beyond that period. Although most irAE-n occur early, some patients develop symptoms after one year of ICI treatment (21, 51), hence, the short follow up time is a weakness. Third, irAE-n are still a diagnosis of exclusion. Even though we used the most comprehensive diagnostic criteria (22), misdiagnosis or false-negative cases that were excluded are possible. Lastly - but most importantly - only two pretreatment samples from patients with irAE-n were available, which is explained by the low frequency of these adverse events. Therefore, we provide evidence regarding the diagnostic capacity of neuronal autoantibodies in irAE-n, but scarcely regarding their value to predict irAE-n. As the identification of patients at risk is particularly important, long-term and multicenter studies are warranted to further investigate the significance of preexisting subclinical autoimmune conditions in irAE-n.
Neuromuscular autoantibodies may serve as a feasible marker to diagnose and potentially predict ICI-induced myositis, myocarditis, and myasthenia gravis. In contrast, brain-reactive autoantibodies are common in both ICI-treated patients with and without irAE-n, hence, their pathogenic significance needs further investigation.
The original contributions presented in the study are included in the article/Supplementary Material. Further inquiries can be directed to the corresponding author.
The studies involving human participants were reviewed and approved by Institutional ethics Committee Charité Universitätsmedizin Berlin. The patients/participants provided their written informed consent to participate in this study (EA1/ 099/17 and EA4/219/21).
LMJ, SK, WB, PH and ME contributed to the conception and design of the study. LMJ, LGR, CS, SM, SK, CU, and RM enrolled the participants. LMJ, CS, SM, and LGR processed the blood samples, KR performed the experimental analysis. LMJ and PH performed the statistical analysis. LMJ wrote the first draft of the manuscript. LMJ, SK, WB, PH and ME acquired funding. All authors contributed to manuscript revision, read, and approved the submitted version.
The project was funded by the Deutsche Forschungsgemeinschaft (DFG, German Research Foundation) under Germany´s Excellence Strategy (EXC-2049 – 390688087 to ME) as well as the Berlin Institute of Health SPARK program (to WB). PH receives funding from the Else-Kröner-Fresenius-Stiftung (2020_EKEA.80). LMJ, CS, SK and PH are participants of the BIH Charité (Junior) Clinician Scientist Program funded by the Charité - Universitätsmedizin Berlin and the Berlin Institute of Health at Charité (BIH). PH is the recipient of a Rahel-Hirsch stipend by Charité Universitätsmedizin Berlin. ME received funding from DFG (Collaborative Research Center ReTune TRR 295-424778381), BMBF, DZNE, DZHK, EU, Corona Foundation, and Fondation Leducq, all outside the scope of this work.
The authors thank Petra Loge for excellent technical assistance processing the blood samples.
Author KR is employed by the Clinical Immunological Laboratory Prof. Dr. med. Winfried Stöcker. The authors declare that this study received funding from EUROIMMUN AG. The funder had the following involvement in the study: Provision of autoantibody assays for brain reactive autoantibodies. WB and PH have received lecture fees from Bristol-Meyers Squibb WB and NOGGO eV. WB and PH. ME reports grants from Bayer and fees paid to the Charité from Abbot, Amgen, AstraZeneca, Bayer, Boehringer Ingelheim, BMS, Daiichi Sankyo, Sanofi, Novartis, Pfizer, all outside the submitted work.
All claims expressed in this article are solely those of the authors and do not necessarily represent those of their affiliated organizations, or those of the publisher, the editors and the reviewers. Any product that may be evaluated in this article, or claim that may be made by its manufacturer, is not guaranteed or endorsed by the publisher.
The Supplementary Material for this article can be found online at: https://www.frontiersin.org/articles/10.3389/fimmu.2023.1108116/full#supplementary-material
1. Wolchok JD, Kluger H, Callahan MK, Postow MA, Rizvi NA, Lesokhin AM, et al. Nivolumab plus ipilimumab in advanced melanoma. N Engl J Med (2013) 369(2):122–33. doi: 10.1056/nejmoa1302369
2. Motzer RJ, Escudier B, McDermott DF, George S, Hammers HJ, Srinivas S, et al. Nivolumab versus everolimus in advanced renal-cell carcinoma. N Engl J Med (2015) 373(19):1803–13. doi: 10.1056/nejmoa1510665
3. Vaddepally RK, Kharel P, Pandey R, Garje R, Chandra AB. Review of indications of FDA-approved immune checkpoint inhibitors per NCCN guidelines with the level of evidence. Cancers (Basel) (2020) 12(3):1–19. doi: 10.3390/cancers12030738
4. Weber JS, Hodi FS, Wolchok JD, Topalian SL, Schadendorf D, Larkin J, et al. Safety profile of nivolumab monotherapy: A pooled analysis of patients with advanced melanoma. J Clin Oncol (2017) 35(7):785–92. doi: 10.1200/JCO.2015.66.1389
5. Postow MA, Sidlow R, Hellmann MD. Immune-related adverse events associated with immune checkpoint blockade. N Engl J Med (2018) 378(2):158–68. doi: 10.1056/nejmra1703481
6. Ruggiero R, Stelitano B, Fraenza F, di Mauro G, Scavone C, Sportiello L, et al. Neurological manifestations related to immune checkpoint inhibitors: Reverse translational research by using the European real-world safety data. Front Oncol (2022) 12:824511. doi: 10.3389/fonc.2022.824511
7. Janssen JBE, Leow TYS, Herbschleb KH, Gijtenbeek JMM, Boers-Sonderen MJ, Gerritsen WR, et al. Immune checkpoint inhibitor-related Guillain-Barré syndrome: A case series and review of the literature. J Immunother (2021) 44(7):276–82. doi: 10.1097/CJI.0000000000000364
8. Johnson DB, Nebhan CA, Moslehi JJ, Balko JM. Immune-checkpoint inhibitors: Long-term implications of toxicity. Nat Rev Clin Oncol (2022) 19(4):254–67. doi: 10.1038/s41571-022-00600-w
9. Zimmer L, Goldinger SM, Hofmann L, Loquai C, Ugurel S, Thomas I, et al. Neurological, respiratory, musculoskeletal, cardiac and ocular side-effects of anti-PD-1 therapy. Eur J Cancer (2016) 60:210–25. doi: 10.1016/j.ejca.2016.02.024
10. Mikami T, Liaw B, Asada M, Niimura T, Zamami Y, Green-LaRoche D, et al. Neuroimmunological adverse events associated with immune checkpoint inhibitor: A retrospective, pharmacovigilance study using FAERS database. J Neurooncol (2021) 152(1):135–44. doi: 10.1007/s11060-020-03687-2
11. Dubey D, David WS, Reynolds KL, Chute DF, Clement NF, Cohen JV, et al. Severe neurological toxicity of immune checkpoint inhibitors: Growing spectrum. Ann Neurol (2020) 87(5):659–69. doi: 10.1002/ana.25708
12. Kao JC, Liao B, Markovic SN, Klein CJ, Naddaf E, Staff NP, et al. Neurological complications associated with anti–programmed death 1 (PD-1) antibodies. JAMA Neurol (2017) 74(10):1216–22. doi: 10.1001/jamaneurol.2017.1912
13. Müller-Jensen L, Zierold S, Versluis JM, Boehmerle W, Huehnchen P, Endres M, et al. Characteristics of immune checkpoint inhibitor-induced encephalitis and comparison with HSV-1 and anti-LGI1 encephalitis: A retrospective multicentre cohort study. Eur J Cancer (2022) 175:224–35. doi: 10.1016/j.ejca.2022.08.009
14. Marini A, Bernardini A, Gigli GL, Valente M, Muñiz-Castrillo S, Honnorat J, et al. Neurologic adverse events of immune checkpoint inhibitors: A systematic review. Neurology (2021) 96(16):754–66. doi: 10.1212/WNL.0000000000011795
15. Müller-Jensen L, Zierold S, Versluis JM, Boehmerle W, Huehnchen P, Endres M, et al. Dataset of a retrospective multicenter cohort study on characteristics of immune checkpoint inhibitor-induced encephalitis and comparison with HSV-1 and anti-LGI1 encephalitis. Data Brief (2022) 45:108649. doi: 10.1016/j.dib.2022.108649
16. Vogrig A, Muñiz-Castrillo S, Honnorat J. Value of onconeural antibodies in checkpoint inhibitor-related toxicities. Ann Neurol (2020) 88(1):199–200. doi: 10.1002/ana.25764
17. Yshii LM, Gebauer CM, Pignolet B, Mauré E, Quériault C, Pierau M, et al. CTLA4 blockade elicits paraneoplastic neurological disease in a mouse model. Brain (2016) 139(11):2923–34. doi: 10.1093/brain/aww225
18. Vogrig A, Fouret M, Joubert B, Picard G, Rogemond V, Pinto AL, et al. Increased frequency of anti-Ma2 encephalitis associated with immune checkpoint inhibitors. Neurol Neuroimmunol Neuroinflamm (2019) 6(6):e604. doi: 10.1212/NXI.0000000000000604
19. Dalmau J, Rosenfeld MR. Paraneoplastic syndromes of the CNS. Lancet Neurol (2008) 7(4):327–40. doi: 10.1016/S1474-4422(08)70060-7
20. Safa H, Johnson DH, Trinh VA, Rodgers TE, Lin H, Suarez-Almazor ME, et al. Immune checkpoint inhibitor related myasthenia gravis: Single center experience and systematic review of the literature. J Immunother Cancer (2019) 7(1):319. doi: 10.1186/s40425-019-0774-y
21. Johansen A, Christensen SJ, Scheie D, Højgaard JLS, Kondziella D. Neuromuscular adverse events associated with anti-PD-1 monoclonal antibodies: Systematic review. Neurology (2019) 92(14):663–74. doi: 10.1212/WNL.0000000000007235
22. Schneider BJ, Naidoo J, Santomasso BD, Lacchetti C, Adkins S, Anadkat M, et al. Management of immune-related adverse events in patients treated with immune checkpoint inhibitor therapy: ASCO guideline update. J Clin Oncol (2021) 39(36):4073–126. doi: 10.1200/JCO.21.01440
23. Guidon AC, Burton LB, Chwalisz BK, Hillis J, Schaller TH, Amato AA, et al. Consensus disease definitions for neurologic immune-related adverse events of immune checkpoint inhibitors. J Immunother Cancer (2021) 9(7):e002890. doi: 10.1136/jitc-2021-002890
24. Bartels F, Wandrey MM, Aigner A, Strönisch T, Farmer K, Rentzsch K, et al. Association between neuronal autoantibodies and cognitive impairment in patients with lung cancer. JAMA Oncol (2021) 7(9):1302–10. doi: 10.1001/jamaoncol.2021.2049
25. Kamo H, Hatano T, Kanai K, Aoki N, Kamiyama D, Yokoyama K, et al. Pembrolizumab-related systemic myositis involving ocular and hindneck muscles resembling myasthenic gravis: a case report. BMC Neurol (2019) 19(1):184. doi: 10.1186/s12883-019-1416-1.2019:1-5
26. Suzuki S, Ishikawa N, Konoeda F, Seki N, Fukushima S, Takahashi K, et al. Nivolumab-related myasthenia gravis with myositis and myocarditis in Japan. Neurology (2017) 89(11):1127–34. doi: 10.1212/WNL.0000000000004359
27. Mammen AL, Rajan A, Pak K, Lehky T, Casciola-Rosen L, Donahue RN, et al. Pre-existing antiacetylcholine receptor autoantibodies and b cell lymphopaenia are associated with the development of myositis in patients with thymoma treated with avelumab, an immune checkpoint inhibitor targeting programmed death-ligand 1. Ann Rheum Dis (2019) 78(1):150–2. doi: 10.1136/annrheumdis-2018-213777
28. Fazel M, Jedlowski PM. Severe myositis, myocarditis, and myasthenia gravis with elevated anti-striated muscle antibody following single dose of ipilimumab-nivolumab therapy in a patient with metastatic melanoma. Case Rep Immunol (2019) 2019:2539493. doi: 10.1155/2019/2539493
29. Onda A, Miyagawa S, Takahashi N, Gochi M, Takagi M. Pembrolizumab-induced ocular myasthenia gravis with anti-titin antibody and necrotizing myopathy. Intern Med (2019) 58(11):1635–8. doi: 10.2169/internalmedicine
30. Sechi E, Markovic SN, McKeon A, Dubey D, Liewluck T, Lennon VA, et al. Neurologic autoimmunity and immune checkpoint inhibitors: Autoantibody profiles and outcomes. Neurology (2020) 95(17):e2442–52. doi: 10.1212/WNL.0000000000010632
31. Touat M, Maisonobe T, Knauss S, Ben O, Salem H. Immune checkpoint inhibitor-related myositis and myocarditis in patients with cancer. Neurology (2018) 91(10):e985–94. doi: 10.1212/WNL.0000000000006124
32. Shelly S, Triplett JD, Pinto MV, Milone M, Diehn FE, Zekeridou A, et al. Immune checkpoint inhibitor-associated myopathy: A clinicoseropathologically distinct myopathy. Brain Commun (2020) 2(2):fcaa181. doi: 10.1093/braincomms/fcaa181
33. Patrick J, Lindstrom J. Autoimmune response to acetylcholine receptor. Science (1973) 180(4088):871–2. doi: 10.1126/science.180.4088.871
34. Drachmann DB, Angus CW, Adams RN, Michelson JD, Hoffman GJ. Myasthenic antibodies cross-link acetylcholine receptors to accelerate degradation. N Engl J Med (1978) 298(20):1116–22. doi: 10.1056/NEJM197805182982004
35. Fichtner ML, Jiang R, Bourke A, Nowak RJ, O'Connor KC. Autoimmune pathology in myasthenia gravis disease subtypes is governed by divergent mechanisms of immunopathology. Front Immunol (2020) 11:776. doi: 10.3389/fimmu.2020.00776
36. Williams CL, Lennon VA. Thymic b lymphocyte clones from patients with myasthenia gravis secrete monoclonal striational autoantibodies reacting with myosin, alpha actinin, or actin. J Exp Med (1986) 164(4):1043–59. doi: 10.1084/jem.164.4.1043
37. Johnson DB, Balko JM, Compton ML, Chalkias S, Gorham J, Xu Y, et al. Fulminant myocarditis with combination immune checkpoint blockade. N Engl J Med (2016) 375(18):1749–55. doi: 10.1056/nejmoa1609214
38. Axelrod ML, Meijers WC, Screever EM, Qin J, Carroll MG, Sun X, et al. T Cells specific for α-myosin drive immunotherapy-related myocarditis. Nature (2022) 611(7937):818–26. doi: 10.1038/s41586-022-05432-3
39. Ciabattini A, Pettini E, Medaglini D. CD4(+) T cell priming as biomarker to study immune response to preventive vaccines. Front Immunol (2013) 4:421. doi: 10.3389/fimmu.2013.00421
40. Bentebibel SE, Lopez S, Obermoser G, Schmitt N, Mueller C, Harrod C, et al. Induction of ICOS+CXCR3+CXCR5+ TH cells correlates with antibody responses to influenza vaccination. Sci Transl Med (2013) 5(176):176ra32. doi: 10.1126/scitranslmed.3005191
41. Bilen MA, Subudhi SK, Gao J, Tannir NM, Tu S, Sharma P. Acute rhabdomyolysis with severe polymyositis following ipilimumab-nivolumab treatment in a cancer patient with elevated anti-striated muscle antibody. J Immunother Cancer (2016) 4:36. doi: 10.1186/s40425-016-0139-8
42. Toi Y, Sugawara S, Sugisaka J, Ono H, Kawashima Y, Aiba T, et al. Profiling preexisting antibodies in patients treated with anti-PD-1 therapy for advanced non-small cell lung cancer. JAMA Oncol (2019) 5(3):376–83. doi: 10.1001/jamaoncol.2018.5860
43. Les I, Pérez-Francisco I, Cabero M, Sánchez C, Hidalgo M. Prediction of immune-related adverse events induced by immune checkpoint inhibitors with a panel of autoantibodies: Protocol of a multicenter, prospective, observational cohort study. Front Pharmacol (2022) 13:894550. doi: 10.3389/fphar.2022.894550
44. Hommes JW, Verheijden RJ, Suijkerbuijk KPM, Hamann D. Biomarkers of checkpoint inhibitor induced immune-related adverse events–a comprehensive review. Front Oncol (2021) 10:585311. doi: 10.3389/fonc.2020.585311
45. Correale P, Saladino RE, Giannarelli D, Sergi A, Mazzei MA, Bianco G, et al. HLA expression correlates to the risk of immune checkpoint inhibitor-induced pneumonitis. Cells (2020) 9(9):1964. doi: 10.3390/cells9091964
46. Finke C, Bartels F, Lütt A, Prüss H, Harms L. High prevalence of neuronal surface autoantibodies associated with cognitive deficits in cancer patients. J Neurol (2017) 264(9):1968–77. doi: 10.1007/s00415-017-8582-0
47. Hara M, Martinez-Hernandez E, Ariño H, Armangu T. Clinical and pathogenic significance of IgG, IgA, and IgM antibodies against the NMDA receptor. Neurology (2018) 90(16):e1386–94. doi: 10.1212/WNL.0000000000005329
48. Prüss H, Höltje M, Maier N, Gomez A, Buchert R, Harms L, et al. IgA NMDA receptor antibodies are markers of synaptic immunity in slow cognitive impairment. Neurology (2012) 78(22):1743–53. doi: 10.1212/WNL.0b013e318258300d
49. Patrinely JR, Johnson R, Lawless AR, Bhave P, Sawyers A, Dimitrova M, et al. Chronic immune-related adverse events following adjuvant anti-PD-1 therapy for high-risk resected melanoma. JAMA Oncol (2021) 7(5):744–8. doi: 10.1001/jamaoncol.2021.0051
50. Velasco R, Villagrán M, Jové M, Simó M, Vilariño N, Alemany M, et al. Encephalitis induced by immune checkpoint inhibitors: A systematic review. JAMA Neurol (2021) 78(7):864–73. doi: 10.1001/jamaneurol.2021.0249
Keywords: immune checkpoint inhibitors, immune related adverse events, neurotoxicity, autoimmunity, neuronal autoantibodies, myositis, paraneoplastic syndromes, cancer immunotherapy
Citation: Müller-Jensen L, Knauss S, Ginesta Roque L, Schinke C, Maierhof SK, Bartels F, Finke C, Rentzsch K, Ulrich C, Mohr R, Stenzel W, Endres M, Boehmerle W and Huehnchen P (2023) Autoantibody profiles in patients with immune checkpoint inhibitor-induced neurological immune related adverse events. Front. Immunol. 14:1108116. doi: 10.3389/fimmu.2023.1108116
Received: 25 November 2022; Accepted: 23 January 2023;
Published: 08 February 2023.
Edited by:
Nora Möhn, Hannover Medical School, GermanyCopyright © 2023 Müller-Jensen, Knauss, Ginesta Roque, Schinke, Maierhof, Bartels, Finke, Rentzsch, Ulrich, Mohr, Stenzel, Endres, Boehmerle and Huehnchen. This is an open-access article distributed under the terms of the Creative Commons Attribution License (CC BY). The use, distribution or reproduction in other forums is permitted, provided the original author(s) and the copyright owner(s) are credited and that the original publication in this journal is cited, in accordance with accepted academic practice. No use, distribution or reproduction is permitted which does not comply with these terms.
*Correspondence: Wolfgang Boehmerle, wolfgang.boehmerle@charite.de
†These authors have contributed equally to this work
Disclaimer: All claims expressed in this article are solely those of the authors and do not necessarily represent those of their affiliated organizations, or those of the publisher, the editors and the reviewers. Any product that may be evaluated in this article or claim that may be made by its manufacturer is not guaranteed or endorsed by the publisher.
Research integrity at Frontiers
Learn more about the work of our research integrity team to safeguard the quality of each article we publish.