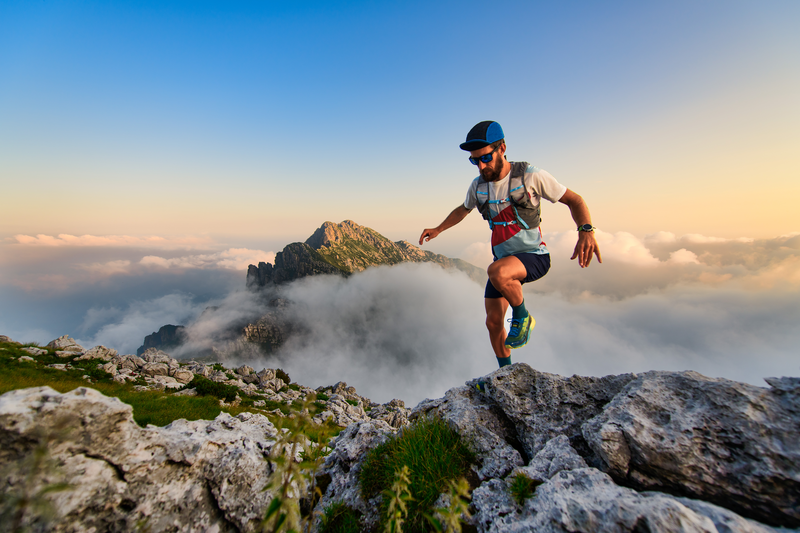
94% of researchers rate our articles as excellent or good
Learn more about the work of our research integrity team to safeguard the quality of each article we publish.
Find out more
ORIGINAL RESEARCH article
Front. Immunol. , 17 January 2023
Sec. Molecular Innate Immunity
Volume 14 - 2023 | https://doi.org/10.3389/fimmu.2023.1107063
This article is part of the Research Topic Current Challenges in Complement Diagnostics View all 27 articles
Introduction: Ficolin-2 is a serum pattern recognition molecule, involved in complement activation via the lectin pathway. This study aimed to investigate the association of ficolin-2 concentration in cord blood serum with complications related to premature birth.
Methods: 546 premature neonates were included. The concentration of ficolin-2 in cord blood serum was determined by a sandwich TRIFMA method. FCN2 genetic variants were analysed with RFLP-PCR, allele-specific PCR, Sanger sequencing or allelic discrimination using TaqMan probes method.
Findings: Cord blood serum ficolin-2 concentration correlated positively with Apgar score and inversely with the length of hospitalisation and stay at Neonatal Intensive Care Unit (NICU). Multivariate logistic regression analysis indicated that low ficolin-2 increased the possibility of respiratory distress syndrome (RDS) diagnosis [OR=2.05, 95% CI (1.24-3.37), p=0.005]. Median ficolin-2 concentration was significantly lower in neonates with RDS than in premature babies without this complication, irrespective of FCN2 gene polymorphisms localised to promoter and 3’untranslated regions: for patients born <33 GA: 1471 ng/ml vs. 2115 ng/ml (p=0.0003), and for patients born ≥33 GA 1610 ng/ml vs. 2081 ng/ml (p=0.012). Ficolin-2 level was also significantly lower in neonates requiring intubation in the delivery room (1461 ng/ml vs. 1938 ng/ml, p=0.023) and inversely correlated weakly with the duration of respiratory support (R=-0.154, p<0.001). Interestingly, in the neonates born at GA <33, ficolin-2 concentration permitted differentiation of those with/without RDS [AUC=0.712, 95% CI (0.612-0.817), p<0.001] and effective separation of babies with mild RDS from those with moderate/severe form of the disease [AUC=0.807, 95% CI (0.644-0.97), p=0.0002].
Conclusion: Low cord serum ficolin-2 concentration (especially in neonates born at GA <33 weeks) is associated with a higher risk of developing moderate/severe RDS, requiring respiratory support and intensive care.
Preterm birth is often associated with multiple pregnancies, infections and gestational diabetes, however most (even >60%) cases are unexplained (1). Common prematurity-associated complications include neonatal sepsis, necrotizing enterocolitis (NEC), intraventricular haemorrhage, patent ductus arteriosus (PDA) and retinopathy. Due to smaller surface area for gas exchange, thicker blood-gas barrier and fewer type II alveolar epithelial cells, respiratory problems are more common in preterm than in term infants (2) and are the main reasons for morbidity and mortality in premature babies. If not recognised and managed quickly, respiratory disease can escalate to respiratory failure and cardiopulmonary arrest (3). The similarities in clinical signs for common causes of respiratory distress like pneumonia, transient tachypnea of neonates (TTN), respiratory distress syndrome (RDS) and meconium aspiration syndrome (MAS) make it difficult to distinguish between them (4).
Human lung development begins between the 4th and 7th weeks of pregnancy and the formation of alveoli continues until early postnatal life (5). RDS is a multifactorial and complex disease associated with inadequate pulmonary surfactant production by immature lungs, resulting in microatelectasis, severe hypoxia, and acidosis (3, 6). The development of RDS may be caused by inadequate clearance of fetal lung liquid as well (7). Genetic susceptibility to RDS in preterm infants has also been demonstrated (8). RDS long-term complications include bronchopulmonary dysplasia (BPD) and asthma. In term and post-mature neonates, respiratory distress may be associated with meconium aspiration syndrome (MAS), and with a higher incidence of asthma in later life.
The complement system is a crucial mediator of the immune response to infection. It interacts with other innate mechanisms as well as acquired immunity, and engages in cross-talk with other endogenous cascades, like the coagulation network (9). It also contributes to cell homeostasis (10), tissue development and repair (11, 12), and reproduction (13). Low activity of classical, lectin or alternative routes of complement in sera of premature babies may enhance their vulnerability to infections due to impaired clearance of pathogens via opsonophagocytosis or direct lysis of pathogens. The lectin pathway (LP) of complement activated by microbial glycoconjugates may play a crucial role in the protection of neonates from infections due to their poor response to T-independent polysaccharide antigens and limited transplacental transfer of maternal antibodies [reviewed in (14)].
Knowledge concerning associations of LP with complications related to prematurity is still limited. Several reports have documented correlations of LP-specific factors in serum with gestational age [reviewed in (14)]. One of the pattern-recognition molecules initiating the lectin pathway of complement is ficolin-2. In a large cohort of neonates (recruited consecutively, including approx. 18% preterms), relative ficolin-2 insufficiency was shown to be associated with prematurity, low birthweight and perinatal infections (15). Ficolin-2 was also demonstrated to contribute to the clearance of serotype III group B streptococci – a common cause of neonatal sepsis (16), often clinically and radiographically indistinguishable from RDS (17). In women diagnosed with pre-eclampsia, serum ficolin-2 concentration was significantly lower in comparison with uncomplicated pregnancies (18, 19). Moreover, deposition of ficolin-2 on apoptotic trophoblasts in preeclampsia was observed (18).
So far, published reports have not demonstrated a strong relationship between prematurity-associated complications and the frequency of promoter or exon 8 FCN2 gene variants, known to markedly influence ficolin-2 level/activity (20). Recently we analysed 15 polymorphisms localised to the FCN2 3’untranslated region (3’UTR) in a large group of preterm babies. Our data revealed that reconstructed diplotypes, including both the G variant at rs4521835 and the C variant at rs73664188 (classified into proposed group VI) were associated with a significantly lower ficolin-2 concentration in cord serum (21).
Ficolin-2 is considered to be an important factor of innate immunity. Several reports suggested its association not only with infections but also with non-infectious diseases and its potential application as a prognostic marker (22–24). In this study, we examined ficolin-2 levels in cord-blood serum of preterm neonates in the context of prematurity-associated complications and its possible usefulness for their diagnosis. Moreover, the genetically determined origin of the differences in ficolin-2 levels observed was verified via analysis of FCN2 gene polymorphisms.
Cord blood samples from 546 Polish preterm neonates, including 118 born at gestational age (GA) <33rd weeks (range 24-32 weeks), and 428 born between 33rd and 37th weeks of pregnancy were obtained from the Department of Newborns’ Infectious Diseases (Poznań University of Medical Sciences, Poland), Department of Neonatology (Medical University of Gdańsk, Poland) and Department of Perinatology (Medical University of Łódź, Poland). This cohort included 504 subjects investigated previously (21).
The basic clinical data are presented in Table 1. The clinical data for neonates separated into the subgroups born at GA <33 and GA ≥33 are presented in Supplementary Table 1. The study was approved by the corresponding local ethics committees (Bioethics Committee of The Karol Marcinkowski Poznań University of Medical Sciences, Independent Bioethics Committee for Scientific Research at Medical University of Gdańsk, Bioethics Committee of The Medical University of Łódź). Written informed parental consent was obtained. This work conforms to the provisions of the Declaration of Helsinki.
Cord samples were taken at birth into sodium citrate (for DNA isolation) and clot activator (for serum) containing tubes. Isolated serum was kept at -80°C. DNA was isolated using GeneMATRIX Quick Blood Purification Kit (EURx Ltd. Gdańsk, Poland), according to the manufacturer’s protocol.
Ficolin-2 concentrations in 481 cord blood serum samples were determined by TRIFMA, as described by Świerzko et al. (25). Briefly, anti-ficolin-2 mAb (ABS 005-16, BioPorto Diagnostics, Denmark) for 384 HB Optiplate coating, whereas biotinylated mAb (GN4, Hycult Biotech, The Netherlands) and Eu3+-labelled streptavidin (Perkin Elmer, USA) for protein detection were used. The 25th percentile (determined for the whole group or for subgroups of neonates born at GA <33 and at GA ≥33) was taken as an indicator of low ficolin-2 concentration. Although the influence of tubes used for blood collection on concentration of ficolin-2 determined in serum was evidenced (26, 27), tubes of the same type were used in all three clinics collecting samples what makes all data fully comparable.
The differences in ficolin-2 levels were analysed in the context of the FCN2 gene promoter haplotype GGCA [corresponding to single nucleotide polymorphisms at positions: -986 (rs3124952); -602 (rs3124953); -64 (rs7865453); -4 (rs17514136)] (28) and 3’UTR group VI diplotype (21), both associated with low ficolin-2 concentration.
Briefly, promoter polymorphisms at positions -986 and -602 were investigated by PCR-RFLP analysis in 503 DNA samples, according to the procedures published by Metzger et al. (29). SNP at positions -64 and -4 were determined using allele-specific PCR or PCR-RFLP, respectively, as described by Szala et al. (30), with minor modifications. Polymorphisms in the FCN2 3’UTR were determined via Sanger sequencing or allelic discrimination using TaqMan probes (21). Haplotypes and diplotypes were created using Haploview 4.2 and PHASE software.
To determine whether continuous variables had a normal distribution, the Shapiro–Wilk test was conducted. The ficolin-2 concentrations were compared using Mann-Whitney U test or Kruskal-Wallis test with post-hoc Dunn test, depending on the number of compared groups. Spearman’s rank correlation test assessed the correlations. The frequencies of genotypes/clinical complications were compared by Fischer’s exact or χ2 test when appropriate. Odds ratios were calculated using online MedCalc software (https://www.medcalc.org). Clinical associations of low ficolin-2 concentration were verified using multiple logistic regression analysis. The predictive power of ficolin-2 was evaluated by receiver operating characteristics (ROC) curves and area under the curve (AUC) analysis. The Statistica (version 13.3, TIBCO Software) and SigmaPlot (version 12, Systat Software) software were used for data management and statistical calculations. P values <0.05 were considered statistically significant.
Median ficolin-2 concentrations differed markedly between neonates born before the 33rd week of gestation and those born at GA ≥33 (median 1639 ng/ml vs 2036 ng/ml, p=0.0024) (Supplementary Figure 1A). Moreover, in neonates with very low birthweight (VLBW, <1500 g), ficolin-2 was significantly lower than in babies with higher birthweight (median 1496 ng/ml vs 2018 ng/ml, p=0.0001) (Supplementary Figure 1B).
Weak but significant correlations of ficolin-2 concentration with Apgar 1’ and Apgar 5’ scores were observed (R=0.18, p=0.0002 and R=0.24, p<0.0001, respectively). The median ficolin-2 level for babies with Apgar 1’ score <7 (1559 ng/ml) was lower than that for those with Apgar 1’ score ≥7 (2014 ng/ml, p=0.01) (Supplementary Figure 1C). In the case of Apgar 5’, medians were 1409 ng/ml and 2042 ng/ml, respectively (p=0.0098) (Supplementary Figure 1D).
Moreover, ficolin-2 inversely correlated with the length of hospitalisation (R=-0,2, p<0,0001) and the length of stay at the neonatal intensive care unit (NICU) (R=-0.13, p=0.007). In neonates who stayed in the hospital for >2 weeks, the median ficolin-2 concentration was significantly lower (1646 ng/ml) than in babies, who stayed in the hospital for a shorter time (2094 ng/ml, p=0.0001) (Supplementary Figure 1E). In the group of neonates with ficolin-2 levels below 1295 ng/ml (<25th percentile), the length of stay at the neonatal ward was generally longer (mean 18 days, range 2-78 days) than in those with higher ficolin-2 (mean 14 days, range 1-91 days, p=0.02). Furthermore, ficolin-2 concentration was significantly lower (1749 ng/ml) in neonates who stayed at least 5 days at NICU in comparison with babies who stayed there for up to 4 days or did not need intensive care (2018 ng/ml, p=0.022) (Supplementary Figure 1F). It is worth noting that all neonates with ficolin-2 concentration <25th percentile and born at GA <33 required hospitalisation at NICU and their stay was generally longer than that of newborns with the same GA but with higher ficolin-2 levels [22.8 days (range 4-61 days) vs. 14.9 days (range 0-84 days), p=0.002).
The data concerning relationships between low (<25th percentile) ficolin-2 concentration in the cord blood and selected prenatal clinical complications of prematurity in the whole cohort are presented in Table 2. Additionally, FCN2 gene promoter haplotype GGCA and 3’UTR diplotype group VI were included in the analysis. Corresponding analyses between subgroups depending on gestational age (<33 weeks and ≥33 weeks, in the context of a wider panel of complications) are presented in Supplementary Table 2.
Table 2 Univariate and multivariate1 regression analysis of potential factors for low ficolin-2 concentration (below 25th percentile)2 in the whole cohort.
Logistic univariate regression analysis revealed associations of low ficolin-2 with VLBW, FCN2 promoter GGCA haplotype and group VI 3’UTR diplotype (Table 2). In contrast, premature rupture of membranes (pPROM) was associated with higher ficolin-2 concentrations. Multivariate analysis confirmed that low birthweight [OR=2.14, 95%CI (1.14-4.02), p=0.018] and GGCA haplotype [OR=2.57, 95%CI (1.26-5.26), p=0.0098], (but not the group VI 3’UTR diplotype) independently predicted low ficolin-2 concentration (Table 2).
Next, the univariate and multivariate logistic regression analysis for RDS as the dependent variable was performed (Table 3). According to the final model, the likelihood of RDS development was increased in neonates with low ficolin-2 cord blood serum concentration [OR=2.05, 95% CI (1.24-3.37), p=0.0051]. It was also associated with shorter GA [OR=7.64, 95% CI: (4.34-13.43), p<0.0001], very low birthweight [OR=3.2, 95% CI (1.46-7.03), p=0.0037], and PDA [OR=11.04, 95% CI (1.87-65.02), p=0.008].
Table 3 Univariate and multivariate logistic regression analysis1 of potential factors for RDS development.
Median ficolin-2 concentration was significantly lower in newborns diagnosed with RDS compared with neonates without this complication (for patients born <33 GA: 1471 vs. 2115 ng/ml, p=0.0003; for patients born ≥33 GA: 1610 vs. 2081 ng/ml; p=0.012) (Figure 1A). For thirty-eight neonates with RDS born at GA <33, data about disease severity were available. Kruskal-Wallis test revealed a significant difference in ficolin-2 levels among groups of babies with no RDS, those with mild and more advanced RDS (p=0.015) (medians: 2115 ng/ml, 2002 ng/ml and 1275 ng/ml, respectively). The post-hoc analysis showed significantly lower ficolin-2 in cord blood serum in neonates diagnosed with more advanced compared with those with mild RDS (p=0.0017) or without this complication (p=0.0084) (Figure 1B).
Figure 1 The comparison of ficolin-2 cord blood serum concentration in preterm neonates with and without respiratory distress syndrome (RDS), depending on gestational age (A) and disease severity in neonates delivered at GA <33 (B). The horizontal lines mark median values. Medians were compared with the use of Mann-Whitney U test (A) and Kruskal-Wallis test (p=0.015) with post-hoc Dunn test (B).
The management of RDS includes exogenous surfactant therapy (by intubation in the delivery room) as well as assisted ventilation. Ficolin-2 concentrations were lower in babies requiring intubation in the delivery room than in those who did not require it (1461 vs. 1938 ng/ml, p=0.023) (Figure 2A). The duration of respiratory support (mechanical ventilation and/or continuous positive airway pressure, CPAP) inversely correlated with ficolin-2 concentration in cord serum (R=-0.154, p<0.001).
Figure 2 Comparison of ficolin-2 concentration in neonates requiring and not requiring intubation at delivery room (A) and/or respiratory support (mechanical ventilation, CPAP, or both) (B). The horizontal lines mark median values. Medians were compared with the use of Mann-Whitney U test (A) and Kruskal-Wallis test (p=0.001) with post-hoc Dunn test (B).
Furthermore, Kruskal-Wallis analysis showed a statistically significant difference in the level of ficolin-2 across three groups: without respiratory support, with respiratory support duration <10 days and with respiratory support ≥10 days (p=0.001) (Figure 2B). Patients who did not require respiratory support had higher median ficolin-2 concentration (median 2089 ng/ml) compared with patients requiring ≥10 days of respiratory support (median 1447 ng/ml, post-hoc Dunn test p=0.0017) but compared with neonates on <10 days of respiratory support the difference was not statistically significant (median 1782 ng/ml, p=0.208).
The potential of ficolin-2 to discriminate between patients with and without RDS was also tested. When all samples were analysed, the selective power of ficolin-2 appeared rather low [AUC=0.643, 95% CI (0.588-0.699), p<0.0001] (Figure 3A). When analysis was performed for neonates born at GA <33 only, it increased to AUC=0.712 [95% CI (0.612-0.817), p=0.0003] (Figure 3B). Then, we developed a simple logistic regression model with GA and birthweight (≥/<1500 g) as independent variables. This model yielded AUC of 0.75 [95% CI (0.70-0.81, p<0.001] with a sensitivity 59.5% and specificity 88.0%. Ficolin-2 level incorporation into the model significantly increased its performance up to AUC 0.80 [95% CI (0.74-0.85), p=0.011) (Figure 3C). Importantly, sensitivity of this model reached 69.1% with preserved specificity (79.8%). Furthermore, ficolin-2 concentration could better differentiate between mild and moderate/severe RDS in newborns born at GA <33 [AUC=0.807, 95% CI (0.644-0.97), p=0.0002] with sensitivity of 75% and specificity of 91% at cut off 1469 ng/ml (Figure 3D). It should however be remembered that the number of samples with corresponding data concerning disease severity was rather small.
Figure 3 The potency of ficolin-2 to differentiate between babies with and without RDS in whole cohort (A), between neonates with and without RDS, born at GA <33 (B), between neonates with and without RDS, depending on GA (<33 vs. ≥33) and BW (<1500g vs. ≥1500g) (C) and between babies born at GA <33 with mild (I) and moderate/severe (II+III) RDS (D).
The main goal of this work was to investigate the association of ficolin-2 concentration at birth with the risk of complications related to prematurity. We demonstrated that low (<25th percentile) ficolin-2 is associated with a lower Apgar score, prolonged hospitalisation and stay at NICU. Moreover, a strong relationship with increased probability of RDS was found (Table 3). Therefore, immediately after birth preterms with low ficolin-2 levels are generally in poorer condition than those with higher concentrations of this protein.
RDS was originally described as hyaline membrane disease- (HMD)-associated vascular disruption leading to the leakage of plasma into the alveolar spaces and layering of fibrin and necrotic cells arising from type II pneumocytes along the alveolar ductus (6). It develops at or within 24 h after birth, and causes hypoxia in association with a lack of surfactant. Untreated disease leads to severe hypoxia resulting in multiple organ failure and even death.
Lung injury and progression to pulmonary edema are also suspected to be consequences of the activation of complement and its cross-talk with the coagulation system (31, 32). Several reports have described lower concentrations of complement factors and higher levels of complement activation products in neonates with RDS, especially in the subgroup poorly responding to surfactant treatment compared with good responders (33). Elevated C3a was also proposed to differentiate RDS from RDS accompanied by perinatal asphyxia (34). Knowledge concerning the role of lectin pathway factors in RDS is limited to a single report by Dogan et al. (35), showing significantly higher frequency of MBL2 genotypes associated with low concentration of MBL in affected neonates. Our results showing increased probability of development of RDS in preterms with low ficolin-2 confirm an association of the complement system with this complication. Interestingly, we found no significant relationship between FCN2 gene polymorphisms and RDS.
Respiratory distress syndrome may be prevented by corticosteroid administration and its treatment usually requires mechanical or non-invasive ventilation and transbronchial exogenous surfactant application. However, it may still result in the development of neonatal chronic lung disease and possibly severe long-term lung damage. Selection of specific and sensitive markers of RDS could be helpful in the diagnostic process and in choosing appropriate treatment. Despite intensive studies, so far no such marker is available. Our results may be helpful in this context since they suggest that ficolin-2 insufficiency has the potential to differentiate between preterms with and without RDS as well as those with mild or more severe disease, at least in the subgroup born at GA<33 (Figure 3). Moreover, we reported that low ficolin-2 in cord blood may predict a need for intubation in the delivery room as well as a requirement for intensive respiratory support.
Several reports evidenced association of low ficolin-2 concentration with diseases of the respiratory system. For example, relative ficolin-2 deficiency was shown to predispose to the development of bronchiectasis (36) and to predict disease progression in patients with idiopathic pulmonary fibrosis (37). Although, no association of ficolin-2 with susceptibility to community-acquired pneumonia was shown (38), its low concentration may increase the risk of infection with Mycobacterium tuberculosis or M. avium complex (39, 40). Median ficolin-2 level was also demonstrated to be significantly lower in paediatric patients with asthma and/or allergic rhinitis suffering from recurrent respiratory infections than in controls (41). Interestingly, Schaubel et al. (42) reported that neonatal RDS (with and without BPD) significantly enhances the risk of asthma in pre-school-aged children. Although ficolin-2 is synthesised by hepatocytes and secreted into the circulation, it can be present in the lung as well (43, 44). Interestingly, very low FCN2 mRNA was found in the fetal lung (45).
Low circulating ficolin-2 in RDS patients may be inborn or be a consequence of its consumption or leakage into the air space due to impaired function of the alveolar-capillary barrier. It may lead not only to complement activation, but also to activation of the coagulation system, via complexed MASP (MBL-associated serine proteases). Purified ficolin-2-MASP complexes were shown to release fibrinopeptides from fibrinogen, activate factor XIII and in consequence, to form a clot (46). It is also possible that ficolin-2 interacts with lung surfactant components like cholesterol crystals which constitute up to 8% of native lung surfactant. Ficolin-2-MASP complex deposition, followed by complement C4 activation on cholesterol crystals was documented by Pilely et al. (47). On the other hand, cholesterol is a major component of meconium - involved also in respiratory distress pathology and mentioned among factors responsible for surfactant inactivation (48).
Apoptosis was proposed to contribute to the pathogenesis of RDS, since numerous apoptotic cells were detected mainly in the respiratory epithelium in lungs of affected infants (49). Late apoptotic/necrotic cells may be also a target for ficolin-2/ficolin-2-MASP complexes resulting in complement activation and enhanced phagocytosis (50, 51).
Our data confirmed association of the FCN2 gene promoter polymorphisms and ficolin-2 concentration, published previously by others and ourselves (20, 28, 52). We previously reported also the relationship of 3’UTR diplotypes (group VI) with low ficolin-2 (21). That was confirmed here in univariate analysis, however lost statistical significance after multivariate logistic regression. It has to be remembered as well that prenatal steroid administration might affect ficolin-2 concentration in cord serum. We found a difference between babies born at GA ≥33 to mothers treated with steroids vs. those of mothers who were not receiving such a treatment, which however was not confirmed in multivariate regression analysis (Supplementary Table 2B).
We report here for the first time possible involvement of ficolin-2 insufficiency in neonatal RDS development. However, it is not clear whether low concentration in cord blood is a cause or consequence of disease progression. It can be assumed that cord blood ficolin-2 <1500 ng/ml may enhance the probability of moderate/severe RDS and the need for surfactant therapy and assisted ventilation. Determination of ficolin-2 concentration in cord serum may be considered a new early prognostic factor in RDS development, helpful to distinguish RDS from other prematurity-associated respiratory disorders and thus facilitating the choice of appropriate treatment. That, however, should be confirmed by an independent study, ideally taking into account responsiveness to treatment and changes of ficolin-2 levels during the course of RDS.
The raw data supporting the conclusions of this article will be made available by the authors, without undue reservation.
The studies involving human participants were reviewed and approved by Bioethics Committee of The Karol Marcinkowski Poznań University of Medical Sciences, Independent Bioethics Committee for Scientific Research at Medical University of Gdańsk, Bioethics Committee of The Medical University of Łódź. Written informed consent to participate in this study was provided by the participants’ legal guardian/next of kin.
GG and AŚ determined ficolin-2 concentrations; AS-P, GG, AŚ, and DJ determined FCN2 gene promoter polymorphisms. AŚ coordinated project realisation, designed the study, planned and supervised experimental work; DJ sequenced FCN2 gene 3’UTR; PK, KC, MK-B, and KS were responsible for patients’ qualification, taking and collecting samples as well as collecting clinical data; ID-P, JM, and JK supervised qualification and recruitment of patients and analysis of clinical data; MM and HS provided anti-ficolin-2 mAbs for initial experiments, contributed to modification of the procedure and discussed corresponding data; DM, JC, WF, and MC performed statistical analysis; MC contributed to the study design, supervision of experimental work and analysed promoter haplotypes. The draft manuscript was written by AŚ and MC, discussed, corrected and finally approved by all authors. All authors contributed to the article and approved the submitted version.
This work was funded by National Science Centre, Poland, grant 2015/17/B/NZ6/04250 and Institute of Medical Biology, Polish Academy of Sciences.
The authors are very grateful to David C. Kilpatrick for reviewing and editing the first draft of the article.
The authors declare that the research was conducted in the absence of any commercial or financial relationships that could be construed as a potential conflict of interest.
All claims expressed in this article are solely those of the authors and do not necessarily represent those of their affiliated organizations, or those of the publisher, the editors and the reviewers. Any product that may be evaluated in this article, or claim that may be made by its manufacturer, is not guaranteed or endorsed by the publisher.
The Supplementary Material for this article can be found online at: https://www.frontiersin.org/articles/10.3389/fimmu.2023.1107063/full#supplementary-material
1. Vogel JP, Chawanpaiboon S, Moller AB, Watananirun K, Bonet M, Lumbiganon P. The global epidemiology of preterm birth. Best Pract Res Clin Obstet Gynaecol (2018) 52:3–12. doi: 10.1016/j.bpobgyn.2018.04.003
2. Moss TJM, Westover AJ. Inflammation-induced lung maturation: lessons from animal experimentation. Paediatr Respir Rev (2017) 23:72–7. doi: 10.1016/j.prrv.2016.10.004
3. Reuter S, Moser C, Baack M. Respiratory distress in the newborn. Pediatr Rev (2014) 35:417–28. doi: 10.1542/pir.35.10.417
4. Sweet LR, Keech C, Klein NP, Marshall HS, Tagbo BN, Quine D, et al. Respiratory distress in the neonate: Case definition & guidelines for data collection, analysis, and presentation of maternal immunization safety data. Vaccine (2017) 35:6506–17. doi: 10.1016/j.vaccine.2017.01.046
5. Gao Y, Cornfield DN, Stenmark KR, Thebaud B, Abman SH, Raj JU. Unique aspects of the developing lung circulation: Structural development and regulation of vasomotor tone. Pulm Circ (2016) 6:407–25. doi: 10.1086/688890
6. Locci G, Fanos V, Gerosa C, Faa G. Hyaline membrane disease (HMD): the role of the perinatal pathologist. J Pediatr Neonatal Ind Med (2014) 3:e030255. doi: 10.7363/030255
7. O’Brodovich H. Fluid clearance from the lungs of newborns, infants and children. Paediatr Respir Rev (2006) 7(suppl. 1):S62–3. doi: 10.1016/j.prrv.2006.04.173
8. Levit O, Jiang Y, Bizzarro MJ, Hussain N, Buhimschi C, Gruen JR, et al. The genetic susceptibility to respiratory distress syndrome. Pediatr Res (2009) 66:693–7. doi: 10.1203/PDR.0b013e3181bbce86
9. Dzik S. Complement and coagulation: cross talk trough time. Transfus Med Rev (2019) 33:199–206. doi: 10.1016/j.tmrv.2019.08.004
10. Hahjishengallis G, Reis ES, Mastellos DC, Ricklin D, Lambris JD. Novel mechanisms and functions of complement. Nat Immunol (2017) 18:1288–98. doi: 10.1038/ni.3858
11. Mastellos D, Gemenis AE, Lambris JD. Complement: and inflammatory pathway fulfilling multiple roles at the interface of innate immunity and development. Curr Drug Targets Inflamm Allergy (2005) 4:125–7. doi: 10.2174/1568010053622993
12. DeAngelis RA, Markiewski MM, Kourtzelis I, Rafail S, Syriga M, Sandor A, et al. A complement – IL-4 regulatory circuits controls liver regeneration. J Immunol (2012) 188:641–8. doi: 10.4049/jimmunol.1101925
13. Riley-Vargas RC, Lanzendorf S, Atkinson JP. Targeted and restricted complement activation on acrosome-reacted spermatozoza. J Clin Invest (2005) 115:1241–9. doi: 10.1172/JCI23213
14. Cedzyński M, Świerzko A.St., Kilpatrick DC. Factors of the lectin pathway of complement activation and their clinical associations in neonates. J Biomed Biotechnol (2012) 2012:363246. doi: 10.1155/2012/363246
15. Swierzko AS, Atkinson APM, Cedzynski M, Macdonald SL, Szala A, Domzalska-Popadiuk I, et al. Two factors of the lectin pathway of complement, l-ficolin and mannan-binding lectin, and their association with prematurity, low birthweight and infections in a large cohort of polish neonates. Mol Immunol (2009) 46:551–8. doi: 10.1016/j.molimm.2008.07.025
16. Aoyagi Y, Adderson EE, Min JG, Matsushita M, Fujita T, Takahashi S, et al. Role of l-ficolin/mannose-binding lectin-associated serine protease complexes in the oposonophagocytosis of type III group b streptococci. J Immunol (2005) 174:418–25. doi: 10.4049/jimmunol.174.1.418
17. Yadav S, Lee B, Kamity R. Neonatal respiratory distress syndrome. In: StatPearls. Treasure Island (FL: StatPearls Publishing (2022).
18. Wang CC, Yim KW, Poon TCW, Choy KW, Chu CY, Lui WT, et al. Innate immune response by ficolin binding in apoptotic placenta is associated with the clinical syndrome of preeclampsia. Clin Chem (2007) 53:42–52. doi: 10.1373/clinchem.2007.074401
19. Halmos A, Rigo J, Szijarto J, Fust G, Prohaszka Z, Molvares A. Circulating ficolin-2 and ficolin-3 in normal pregnancy and pre-eclampsia. Clin Exp Immunol (2012) 169:49–56. doi: 10.1111/j.1365-2249.2012.04590.x
20. Kilpatrick DC, Swierzko A, Matsushita M, Domzalska-Popadiuk I, Borkowska-Kłos M, Szczapa J, et al. The relationship between FCN2 genotypes and serum ficolin-2 (L-ficolin) proteins concentrations from a large cohort of neonates. Hum Immunol (2013) 74:867–71. doi: 10.1016/j.humimm.2013.04.011
21. Świerzko AS, Jarych D, Gajek G, Chojnacka K, Kobiela P, Kufelnicka-Babout M, et al. Polymorphisms of the FCN2 gene 3’UTR region and their associations in preterm newborns., front. Immunol (2021) 12:741140. doi: 10.3389/fimmu.2021.741140
22. Carbone F, Valente A, Perego C, Bertolotto M, Pane B, Spienella G, et al. Ficolin-2 serum levels predict the occurrence of acute coronary syndrome in patients with severe carotid artery stenosis. Pharmacol Res (2021) 166:1054462. doi: 10.1016/j.phrs.2021.105462
23. Giraudi PJ, Salvoza N, Bonazza D, Saitta C, Lombardo D, Casagranda B, et al. Ficolin-2 plasma level assesses liver fibrosis in non-alcoholic fatty liver disease. Int J Mol Sci (2022) 23:2813. doi: 10.3390/ijms23052813
24. Turek-Jakubowska A, Dębski J, Jakubowski M, Szahidewicz-Krupska E, Gawryś J, Gawryś K, et al. New candidates for biomarkers and drug targets of ischemic stroke-a first dynamic LC-MS human serum proteomic study. J Clin Med (2022) 11:339. doi: 10.3390/jcm11020339
25. Świerzko AS, Michalski M, Sokołowska A, Nowicki M, Szala-Poździej A, Eppa Ł, et al. Associations of ficolins with haematological malignancies in patients receiving high-dose chemotherapy and autologous hematopoietic stem cell transplantations. Front Immunol (2020) 10:3097. doi: 10.3389/fimmu.2019.03097
26. Hein E, Bay JT, Munthe-Fog L, Garred P. Ficolin-2 reveals different analytical and biological properties dependent on different sample handling procedures. Mol Immunol (2013) 56:406–12. doi: 10.1016/j.molimm.2013.05.233
27. Brady AM, Spencer BL, Falsey AR, Nahm MH. Blood collection tubes influence serum ficolin-1 and ficolin-2 levels. Clin Vaccine Immunol (2014) 21:51–5. doi: 10.1128/CVI.00607-13
28. Szala-Poździej A, Świerzko AS, Gajek G, Kufelnicka-Babout M, Chojnacka K, Kobiela P, et al. Association of the FCN2 gene promoter region polymorphisms with very low birthweight in preterm neonates. Int J Mol Sci (2022) 23:15336. doi: 10.3390/ijms232315336
29. Metzger ML, Michelfelder I, Goldacker S, Mekaoui K, Liztman J, Guzman D, et al. Low ficolin-2 levels in common variable immunodeficiency patients with bronchiectasis. Clin Exp Immunol (2015) 179:256–64. doi: 10.1111/cei.12459
30. Szala A, Swierzko A, Cedzynski M. Cost-effective procedures for genotyping of human FCN2 gene single nucleotide polymorphisms. Immunogenetics (2013) 65:439–46. doi: 10.1007/s00251-013-0696-7
31. Jeffries AL, Coates G, O’Brodovich H. Pulmonary epithelial permeability in hyaline membrane disease. N Engl J Med (1984) 311:1075–80. doi: 10.1056/NEJM198410253111703
32. Yurdakok M, Korkmaz A, Kirazli S, Aygun C, Yigit S. Global fibrinolytic capacity in early respiratory distress syndrome: A pilot study. Am J Hematol (2002) 69:255–7. doi: 10.1002/ajh.10060
33. Wagner MH, Sonntag J, Strauss E, Obladen M. Complement and contact activation related to surfactant response in respiratory distress syndrome. Pediatr Res (1999) 45:14–8. doi: 10.1203/00006450-199901000-00004
34. Schrod L, Frauendienst-Egger G, von Stockhausen HB, Kirschfink M. Complement fragment C3a in plasma of asphyxiated neonates. Eur J Pediatr (1992) 151:688–92. doi: 10.1007/BF01957575
35. Dogan P, Ozkan H, Koksal N, Oral HB, Bagci O, Varal IG. Mannose-binding lectin gene polymorphism and its effect on short term outcomes in preterm infants. J Pediatr (Rio J) (2020) 96:520–6. doi: 10.1016/j.jped.2019.03.001
36. Kilpatrick DC, Chalmers JD, MacDonald SL, Murray M, Ali M, Hart SP, et al. Stable bronchiesctasis is associated with low serum l-ficolin concentrations. Clin Respir J (2009) 3:29–33. doi: 10.1111/j.1752-699X.2008.00105.x
37. Ashley SL, Xia M, Murray S, O’Dwyer DN, Grant E, White ES, et al. Six-SOMAmer index relating to immune, protease and angiogenic functions predicts progression in IPF. PloS One (2016) 11:e0159878. doi: 10.1371/journal.pone.0159878
38. Chalmers JD, Fleming GB, Rutherford J, Matsushita M, Kilpatrick DC, Hill AT. Serum ficolin-2 in hospitalized patients with community-acquired pneumonia. Inflammation (2014) 37:1635–41. doi: 10.1007/s10753-014-9891-4
39. Luo F, Sun X, Wang Y, Wang Q, Wu Y, Pan Q, et al. Ficolin-2 defends against virulent Mycobacterium tuberculosis infection in vivo, and its insufficiency is associated with infections in humans. PloS One (2013) 8:e73859. doi: 10.1371/journal.pone.0073859
40. Kobayashi T, Kuronuma K, Saito A, Ikeda K, Ariki S, Saitou A, et al. Insufficient serum l-ficolin is associated with disease presence and extent of pulmonary Mycobacterium avium complex disease. Resp Res (2019) 20:224. doi: 10.1186/s12931-019-1185-9
41. Cedzynski M, Atkinson APM, Swierzko A, MacDonald SL, Szala A, Zeman K, et al. L-ficolin (ficolin-2) insufficiency is associated with combined allergic and infectious respiratory disease in children. Mol Immunol (2009) 47:415–9. doi: 10.1016/j.molimm.2009.08.028
42. Schaubel D, Johansen H, Dutta M, Desmeules M, Becker A, Mao Y. Neonatal characteristics as risk factors for preschool asthma. J Asthma (1996) 33:255–64. doi: 10.3109/02770909609055366
43. Bidula S, Sexton DW, Abdolrasouli A, Shah A, Reed A, Armstrong-Jones D, et al. The serum opsonin l-ficolin is detected in lungs of human transplant recipients following fungal infections and modulates inflammation and killing of Aspergillus fumigatus. J. Infect Dis (2015) 212:234–46. doi: 10.1093/infdis/jiv027
44. Vogt S, Trendelenburg M, Tamm M, Stolz D, Hostettker KE, Osthoff M. Local and systemic recognition receptors of the lectin pathway of complement in a cohort of patients with interstitial lung diseases. Front Immunol (2020) 11:562564. doi: 10.3389/fimmu.2020.562564
45. Hummelshoj T, Munthe Fog L, Madsen HO, Sim RB, Garred P. Comparative study of the human ficolins reveals unique features of ficolin-3 (Hakata antigen). Mol Immunol (2008) 45:1623–32. doi: 10.1016/j.molimm.2007.10.006
46. Gulla K, Gupta K, Krarup A, Gal P, Schwaeble WJ, Sim RB, et al. Activation of mannan-binding lectin-associated serine proteases leads to generation of a fibrin clot. Immunology (2010) 129:482–95. doi: 10.1111/j.1365-2567.2009.03200.x
47. Pilely K, Rosbjerg A, Genster N, Gal P, Pal G, Halvorsen B, et al. Cholesterol crystals activate the lectin complement pathway via ficolin-2 and mannose-binding lectin: implications for the progression of atherosclerosis. J Immunol (2016) 196:5064–74. doi: 10.4049/jimmunol.1502595
48. Krafft MP. Overcoming inactivation of the lung surfactant by serum proteins: a potential role of fluorocarbons. Soft Matter (2015) 11:5982–94. doi: 10.1039/C5SM00926J
49. Jensen ML, Honore C, Hummelshoj T, Hansen BE, Madsen HO, Garred P. Ficolin-2 recognizes DNA and participates in the clearance of dying host cells. Mol Immunol (2007) 44:856–65. doi: 10.1016/j.molimm.2006.04.002
50. Lukkarinen HP, Laine J, Kaapa PO. Lung epithelial cells undergo apoptosis in neonatal respiratory distress syndrome. Pediatr Res (2003) 53:254–9. doi: 10.1203/00006450-200302000-00009
51. Kuraya M, Ming Z, Liu X, Matsushita M, Fujita T. Specific binding of l-ficolin and h-ficolin to apoptotic cells leads to complement activation. Immunobiology (2005) 209:689–97. doi: 10.1016/j.imbio.2004.11.001
Keywords: FCN2, ficolin-2, newborn, prematurity, preterm, respiratory distress syndrome (RDS)
Citation: Gajek G, Świerzko AS, Jarych D, Mikulski D, Kobiela P, Chojnacka K, Kufelnicka-Babout M, Szala-Poździej A, Chrzanowski J, Sobczuk K, Fendler W, Matsushita M, Domżalska-Popadiuk I, Mazela J, Kalinka J, Sekine H and Cedzyński M (2023) Association of low ficolin-2 concentration in cord serum with respiratory distress syndrome in preterm newborns. Front. Immunol. 14:1107063. doi: 10.3389/fimmu.2023.1107063
Received: 24 November 2022; Accepted: 02 January 2023;
Published: 17 January 2023.
Edited by:
Erik J.M. Toonen, Hycult Biotech, NetherlandsReviewed by:
Moon H. Nahm, University of Alabama at Birmingham, United StatesCopyright © 2023 Gajek, Świerzko, Jarych, Mikulski, Kobiela, Chojnacka, Kufelnicka-Babout, Szala-Poździej, Chrzanowski, Sobczuk, Fendler, Matsushita, Domżalska-Popadiuk, Mazela, Kalinka, Sekine and Cedzyński. This is an open-access article distributed under the terms of the Creative Commons Attribution License (CC BY). The use, distribution or reproduction in other forums is permitted, provided the original author(s) and the copyright owner(s) are credited and that the original publication in this journal is cited, in accordance with accepted academic practice. No use, distribution or reproduction is permitted which does not comply with these terms.
*Correspondence: Anna S. Świerzko, YXN3aWVyemtvQGNibS5wYW4ucGw=
†These authors have contributed equally to this work and share first authorship
Disclaimer: All claims expressed in this article are solely those of the authors and do not necessarily represent those of their affiliated organizations, or those of the publisher, the editors and the reviewers. Any product that may be evaluated in this article or claim that may be made by its manufacturer is not guaranteed or endorsed by the publisher.
Research integrity at Frontiers
Learn more about the work of our research integrity team to safeguard the quality of each article we publish.