- 1Centro de Biología Molecular Severo Ochoa, Consejo Superior de Investigaciones Científicas (CSIC), Universidad Autónoma de Madrid, Madrid, Spain
- 2Hospital Universitario Ramón y Cajal, Universidad de Alcalá, IRYCIS, Madrid, Spain
- 3Department of Pediatrics, Tropical and Infectious Diseases, Hospital La Paz, and La Paz Research Institute (IdiPAZ), Translational Research Network of Pediatric Infectious Diseases (RITIP), and CIBERINFEC, Madrid, Spain
- 4Instituto Sanitario Princesa, Madrid, Spain
Background: Children are less susceptible than adults to symptomatic COVID‐19 infection, but very few studies addressed their underlying cause. Moreover, very few studies analyzed why children highly exposed to the virus remain uninfected.
Methods: We analyzed the serum levels of ACE2, angiotensin II, anti-spike and anti-N antibodies, cytokine profiles, and virus neutralization in a cohort of children at high risk of viral exposure, cohabiting with infected close relatives during the lockdown in Spain.
Results: We analyzed 40 children who were highly exposed to the virus since they lived with severe acute respiratory syndrome coronavirus-2 (SARS-CoV-2)-infected relatives during the lockdown for several months without taking preventive measures. Of those, 26 reported mild or very mild symptoms. The induced immune response to the virus was analyzed 3 months after the household infection. Surprisingly, only 15 children had IgG anti-S (IgG+) determined by a sensitive method indicative of a past infection. The rest, negative for IgG anti-N or S in various tests, could be further subdivided, according to IgM antibodies, into those having IgM anti-S and IgM anti-N (IgG−IgMhigh) and those having only IgM anti-N (IgG−IgMlow). Interestingly, those two subgroups of children with IgM antibodies have strikingly different patterns of cytokines. The IgMhigh group had significantly higher IFN-α2 and IFN-γ levels as well as IL-10 and GM-CSF than the IgMlow group. In contrast, the IgMlow group had low levels of ACE2 in the serum. Both groups have a weaker but significant capacity to neutralize the virus in the serum than the IgG+ group. Two children were negative in all immunological antibody tests.
Conclusions: A significant proportion of children highly exposed to SARS-CoV-2 did not develop a classical adaptive immune response, defined by the production of IgG, despite being in close contact with infected relatives. A large proportion of those children show immunological signs compatible with innate immune responses (as secretion of natural antibodies and cytokines), and others displayed very low levels of the viral receptor ACE2 that may have protected them from the virus spreading in the body despite high and constant viral exposure.
Introduction
Severe acute respiratory syndrome coronavirus-2 (SARS-CoV-2) is the causative agent of coronavirus disease 2019 (COVID-19) that has caused almost 580 million cases worldwide and more than 6.6 million deaths up to November 2022, according to an independent count by Johns Hopkins University (https://www.arcgis.com/apps/dashboards/bda7594740fd40299423467b48e9ecf6). SARS-CoV-2 preferentially infects the respiratory tract causing a potentially fatal disease. SARS-CoV-2 enters human cells via the receptor-binding domain (RBD) of its spike (S) protein that interacts with the angiotensin-converting enzyme 2 (ACE2) receptor (1, 2). ACE2 is a membrane-bound enzyme expressed in numerous cell types and tissues such as the lungs, arteries, heart, and intestine. ACE2 catalyzes the cleavage of angiotensin II (AngII) into angiotensin 1-7, regulating the renin–angiotensin–aldosterone system (RAS), playing a critical role in the homeostasis of tissue microcirculation and inflammation (3, 4).
Currently, it is not completely clear how altered ACE2 levels influence SARS-CoV-2 virulence and relevant COVID-19 complications [reviewed in (5)]. On the one hand, ACE2 has lung protective effects by reducing AngII-mediated pulmonary inflammation (6, 7), but reduced ACE2 levels may restrict virus infection (8). Moreover, high levels of serum ACE2 may protect from infection (9–11), acting likely as a decoy.
COVID-19 infection is usually mild in children who have a better outcome than in adults, although the reasons for this are not fully understood (12). Several theories have been proposed to explain this fact (13, 14). One of the first proposed mechanisms is based on differences in the expression and/or affinity of receptors to SARS-CoV-2 between children and adults. In particular, it was suggested that the lower expression of the viral receptor ACE2 in children in nasal epithelium and serum protects them from severe COVID-19 (15, 16).
The first defense against any pathogen is the innate immune response. After virus penetration in the respiratory tract, an innate immune response is activated in which macrophages and dendritic cells recognize the virus releasing inflammatory cytokines (such as TNF, IL‐1b, and IL‐6) and type I interferons (IFNs) (17). So, differences in antiviral IFN production may also account for those sensitivity differences between children and adults (17). SARS-CoV-2 has several strategies to alter IFN production and/or signaling pathways. Moreover, age-associated increases in the production of inflammatory cytokines have been described, implying that children may be less prone to suffer cytokine storm syndrome (12).
Furthermore, children may have a more robust innate immune response to SARS-CoV-2 due to a trained immunity, likely secondary to other viral infections and/or vaccines and allowing the early control of the disease at the site of entry in the respiratory tract (6, 18). Also, there are developmental variations in immune system function with age (12). Moreover, despite the high transmissibility of SARS-CoV-2, there are highly exposed people who have not acquired the infection. Genetic factors and other risk factors can determine the susceptibility of each individual to infection, but those are largely unknown (19).
Our study aimed to provide insight into why children with reported high-risk exposure to the virus cohabiting without protection with infected relatives remained apparently uninfected despite constant contact with the virus. Our results indicate a wide range of immune responses in children highly exposed to SARS-CoV-2.
Material and methods
Patients and sample collection
We analyzed data from children recruited at Hospital Universitario La Paz in June 2020, before the SARS-CoV-2 vaccine implementation. They had repeated high-risk exposures to SARS-CoV-2 (specifically, cohabitation with parents with confirmed COVID-19). A total of 40 human sera were obtained at least 8 weeks after the exposure from their relatives. All parents or legal guardians of the children participants provided written consent to participate in the study, which was performed according to the EU guidelines and following the ethical principles of the Declaration of Helsinki. The study was carried out at the Ramón y Cajal University Hospital in Madrid (Spain) and approved by the local Research Ethics Committee (ceic.hrc@salud.madrid.org, approval number 095/20).
Anti-S flow cytometry immunoassay
Conformational anti-S antibodies were detected by flow cytometry immunoassay (JFCI) to detect IgG and IgA isotypes as described (20, 21). Briefly, Jurkat cells stably expressing the Wuhan S variant (Jurkat-S-GFP) were incubated for 30 min on ice with a 1:50 dilution of children sera in phosphate-buffered saline (PBS), 1% bovine serum albumin (BSA, Sigma-Aldrich, Europe), and 0.02% sodium azide (Sigma-Aldrich). Cells were centrifuged 2 times for 5 min at 500g. The cell pellet was finally resuspended in a 1:300 dilution of mouse anti-human IgG1 Fc-PE (Ref.: 9054-09, Southern Biotech) and goat anti-human IgA Fc-Alexa Fluor 647 (Ref.: 2052-31, Southern Biotech). Samples were then washed and analyzed on a FACSCanto II flow cytometer (Becton-Dickinson). Data were analyzed with FlowJo software (BD). The Ig anti-S to GFP mean fluorescent intensity ratio is used as a relative quantitative value as it correlates with the titer and affinity of specific antibodies to S (20, 21). The umbral of positivity was calculated according to the negative control sera for each isotype.
ELISA
Detection of linear anti-S1/N antibodies was performed by in-home ELISA in 96‐well plates (MaxiSorp Nunc Immuno Plate) coated overnight at 4°C with S1 or N (2 µg/ml) proteins. The coated plates were incubated with the diluted sera for 1 h at room temperature. Plates were blocked with PBS + 0.05% Tween + 1% BSA for 1 h at RT. Bound antibodies were detected by incubation with mouse anti‐human IgG1 or IgM secondary antibody coupled to horseradish peroxidase (HRP; Southern Biotech) diluted 1/6,000 in 1% BSA in PBS, which was then detected using an ABTS substrate solution (Invitrogen). The OD at 415 nm was determined on an iMark microplate reader (Bio‐Rad). The specificity and sensitivity of the assays were controlled using seropositive adults with PCR+, and prepandemic sera were used as negative controls. Prepandemic sera were all below the cutoff levels.
ACE2 and AngII were measured according to the respective manufacturer’s protocol kits (ab235649 Human ACE2 simple step ELISA kit, Abcam; Human Angiotensin II ELISA kit, Reddot Biotech). The OD at 450 nm was determined on a FLUOstar OPTIMA reader (BMG Labtech).
Multiplex cytokine assay
A bead-based multiplex assay with the main cytokines released in antivirus response was measured according to the manufacturer’s protocol kit (BioLEGENDplex™ Human Anti-virus response panel). The plate was analyzed on a BD FACSCanto II High-Throughput Sampler Option. Data were analyzed using LEGENDplex™ data analysis software.
Neutralization assay with a pseudotyped virus
Lentiviral supernatants were produced from transfected HEK293T cells as described previously (20). Briefly, lentiviruses were obtained by co-transfecting plasmids pCMV (gag/pol), pHRSIN-GFP, and a truncated S envelope (pCR3.1-St) using the jetPEI transfection reagent (Polyplus-transfection). Viral supernatants were obtained after 48 h post-transfection. Polybrene (8 µg/ml) was added to the viral supernatants before the transduction of ACE2+HEK293T cells. A total of 35-50 × 103 ACE2+HEK293T cells per p48 well were seeded the day before transduction. Diluted plasma (one-fourth) was incubated with viral supernatant for 1 h at 37°C before addition to the cells. Cells were left in culture for 48 h, resuspended in PBS with 2% FBS and 5 mM of EDTA, and fixed with 2% paraformaldehyde. GFP+ cells were then analyzed on a FACSCalibur flow cytometer (Becton-Dickinson). Data were analyzed with FlowJo software (BD).
Statistical analysis
Significant differences between groups were assessed by the multiple comparisons Kruskal–Wallis test, corrected by controlling the false discovery rate using the Benjamini, Krieger, and Yekutieli test. All data and figures were analyzed and represented using the GraphPad Prism 8 software.
Results
Patient stratification
We analyzed a cohort of 40 children, some of them siblings, living at high risk of viral exposure cohabiting in a close and familiar unit with one or two infected relatives (parents with confirmed COVID-19 by PCR) during the lockdown in Spain from March to May 2020 and without taking any preventive measure at home. Since most of the children were asymptomatic or with mild and non-specific symptoms, they did not attend the hospital for analysis until the release of lockdown, and no PCR could be obtained. More than 2 months after the virus risk contact, the serum was collected. All clinical, demographic, and immunological data are reported in Table S1. Some children (62.5%) reported mild or very mild symptoms during the high-risk exposure period compatible with a possible infection by SARS-CoV-2. None of the subjects showed signs of any other pathology in the 45-60 days previous to blood collection.
The children’s immunological status was evaluated using various tests (ELISA and flow cytometry) (see Figure 1 for the flowchart). First, they were tested using a very sensitive assay, more sensitive than routine clinical tests, to measure IgG1 formation to S virus protein by flow cytometry (JFCI) (20, 21) to track past SARS-CoV-2 infection. The Ig anti-S to GFP mean fluorescent intensity ratio is used as a relative quantitative value as it correlates with the titer and affinity of specific antibodies to S. Only 15 children showed detectable antibodies by this assay (Figure 1), and eight of them were asymptomatic. Other serological assays, such as conventional ELISA IgG anti-S1 or anti-N, only detected nine (see Figure S1).
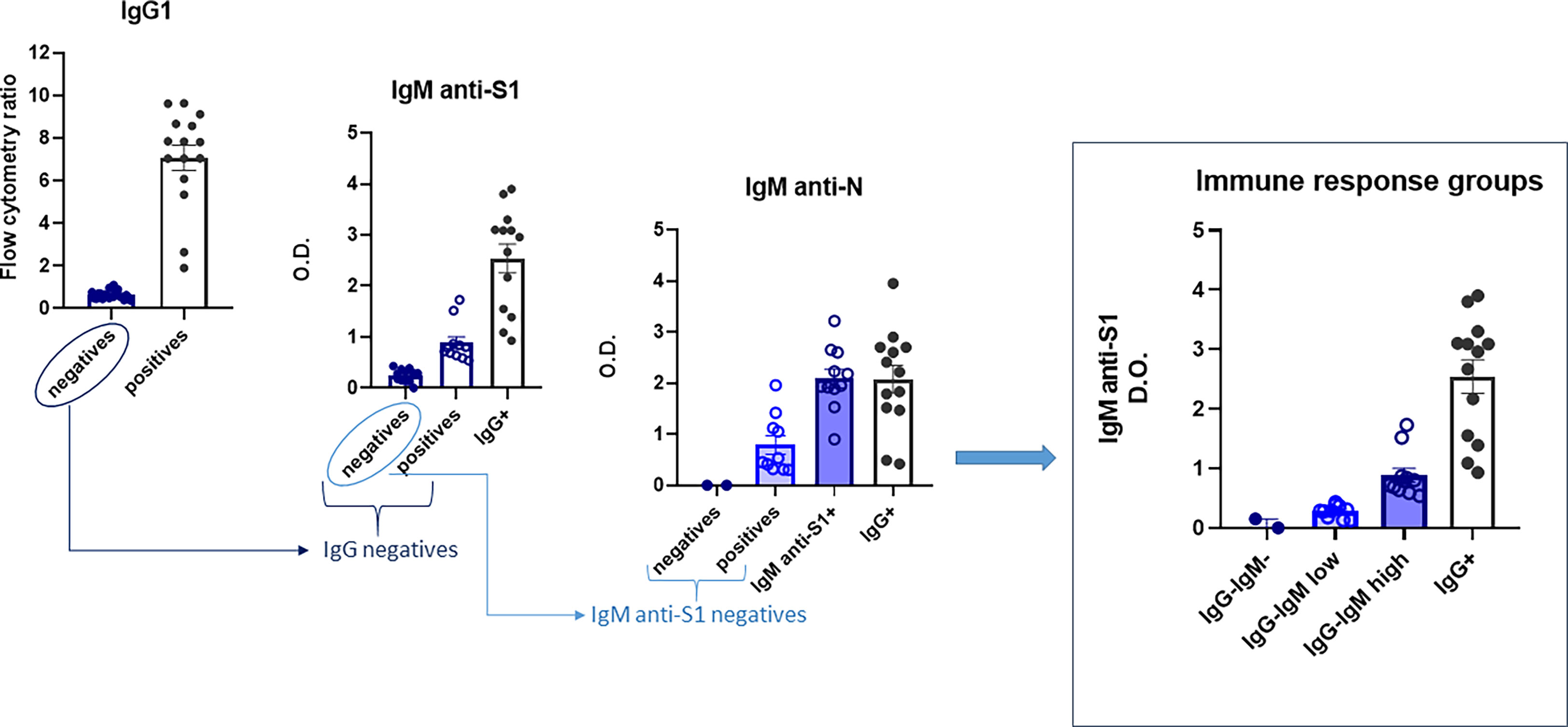
Figure 1 Flowchart of the immunoglobulin profile in children to evaluate their immunological status. Four groups were finally defined: IgG−IgM−, IgG−IgMlow, IgG−IgMhigh, and IgG+. Sera were analyzed as described in the Material and methods. Results shown are the mean values of one duplicate experiment of the three independent experiments performed with similar qualitative results.
Next, we performed a more complete serological study evaluating the presence of other isotypes against the same and/or other SARS-CoV-2 epitopes (IgM/IgA anti-S and IgM/IgG anti-N). First, the sera were checked for IgM isotype against S1 and N viral proteins by ELISA. The 15 seropositive children were also positive for IgM anti-S1. Of the 25 IgG anti-S seronegative children, all assays could be performed completely only in 20, allowing a subclassification into those with IgM antibodies against both S1 and N proteins (IgG−IgMhigh) and those negatives for IgM anti-S1 but positive for IgM antibodies against N protein (IgG−IgMlow) (Figure 1). Only two IgG-seronegative children were also negative for both IgM antibodies. Finally, we analyzed the presence of the IgA isotype against S by the JFCI test. Once again, seropositive IgG anti-S was also positive for IgA anti-S (Figure S1), while only nine in the seronegative group were positive in IgA, most of them (n = 7) belonging to the IgG−IgMlow subgroup (Figure S1).
Altogether, those assays allow us to subdivide the children according to their immunoglobulin profile into four groups:
1. IgG+: children with IgG and IgA anti-S by JFCI, IgM anti-S1 by ELISA, and most also positive for IgG anti-S and anti-N IgG by ELISA (N = 15).
2. IgG−IgMhigh: children with IgG anti-S and anti-N negative by three independent tests but medium to high levels of IgM anti-S1 and anti-N (N = 11). Two of them were also positive for IgA anti-S, determined by JFCI.
3. IgG−IgMlow: children with IgG anti-S and anti-N negative by three independent tests. IgM anti-S1 negative by ELISA but detectable levels of IgM anti-N. Most (70%) were positive for IgA anti-S. One child was eliminated for subsequent analyses due to a previous developmental pathology (N = 9).
4. Negative: IgG−IgM−IgA−. Negative in all serological tests (N = 2).
Neither association with the type of symptoms nor correlation with sex for those four groups was observed. Group IgG−IgMhigh tends to be younger, with 63.6% of children under 12 years of age, while there were only 40% and 44% in the IgG+ and IgG−IgMlow groups, respectively (Figure S2). Of note, the distribution of this immunoglobulin profile was not related to belonging to the same family unit. A total of 22 families participated in the study, 14 of them with two or more children infected. In six of those families (43%), the siblings showed a different pattern of immune response (Figure S2).
Immune and biological responses
We analyzed several immune and biological parameters in those children’s sera looking for clues on their possible innate resistance to infection. ACE2 is a virus receptor, and some reports have attributed the lower susceptibility of children to lower ACE2 levels (13). We indeed detected much lower serum ACE2 levels that we reported for adults in the adult population (9). Very interestingly, the IgG−IgMlow group had significantly much lower levels of ACE2. No differences among the other groups were observed (Figure 2).
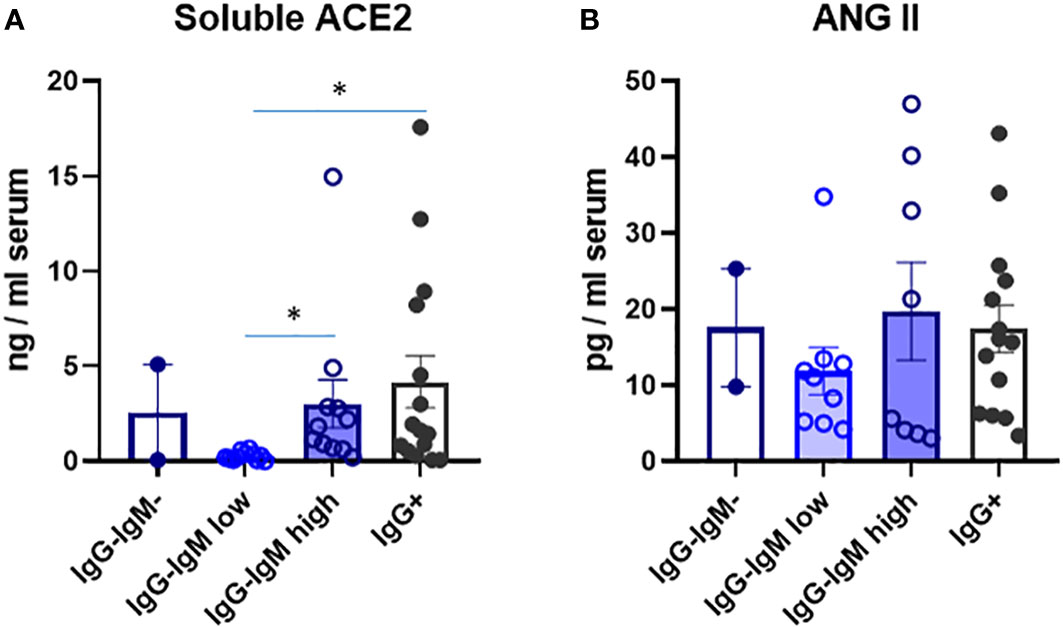
Figure 2 Levels of soluble ACE2 (A) and ANG Il (B) in serum of children according to their immunological status. Serum levels were analysed by ELISA as described in methods in the indicated four groups: IgG-IgM, IgG-IgMlow, IgG-IgMhigh, IgG+. Results shown are the mean values of one duplicate experiment of the 2 independent performed with similar qualitative results. *p-adjusted<0,05.
Several cytokines were also analyzed in serum to indirectly state the innate immune status (see Table S1). Their values considering all children as a single group are within the ranges of those reported for healthy children (i.e., IL-10, GM-CSF, IL-6, TNF) (22). Interestingly, the IgG−IgMhigh group showed significantly higher IL12p70, IL-1, IL-6, and TNF proinflammatory cytokines than the IgG−IgMlow group (Figure 3). Indeed, the IgG−IgMhigh group also had more proinflammatory cytokine TNF than IgG+ children. More remarkably, the IgG−IgMhigh group also had the highest levels of several IFNs, such as IFN-α2 and IFN-γ (Figure 3). Again, the IgG−IgMlow group showed the lowest level of IFNs, significantly lower than the other two groups. Moreover, this group showed a very similar pattern of cytokines to the negative group. Once again, a similar pattern was observed in the different groups regarding the levels of IL-10 and GM-CSF (Figure 3). Other cytokines such as IL-8 and IP-10 did not significantly differ between the groups.
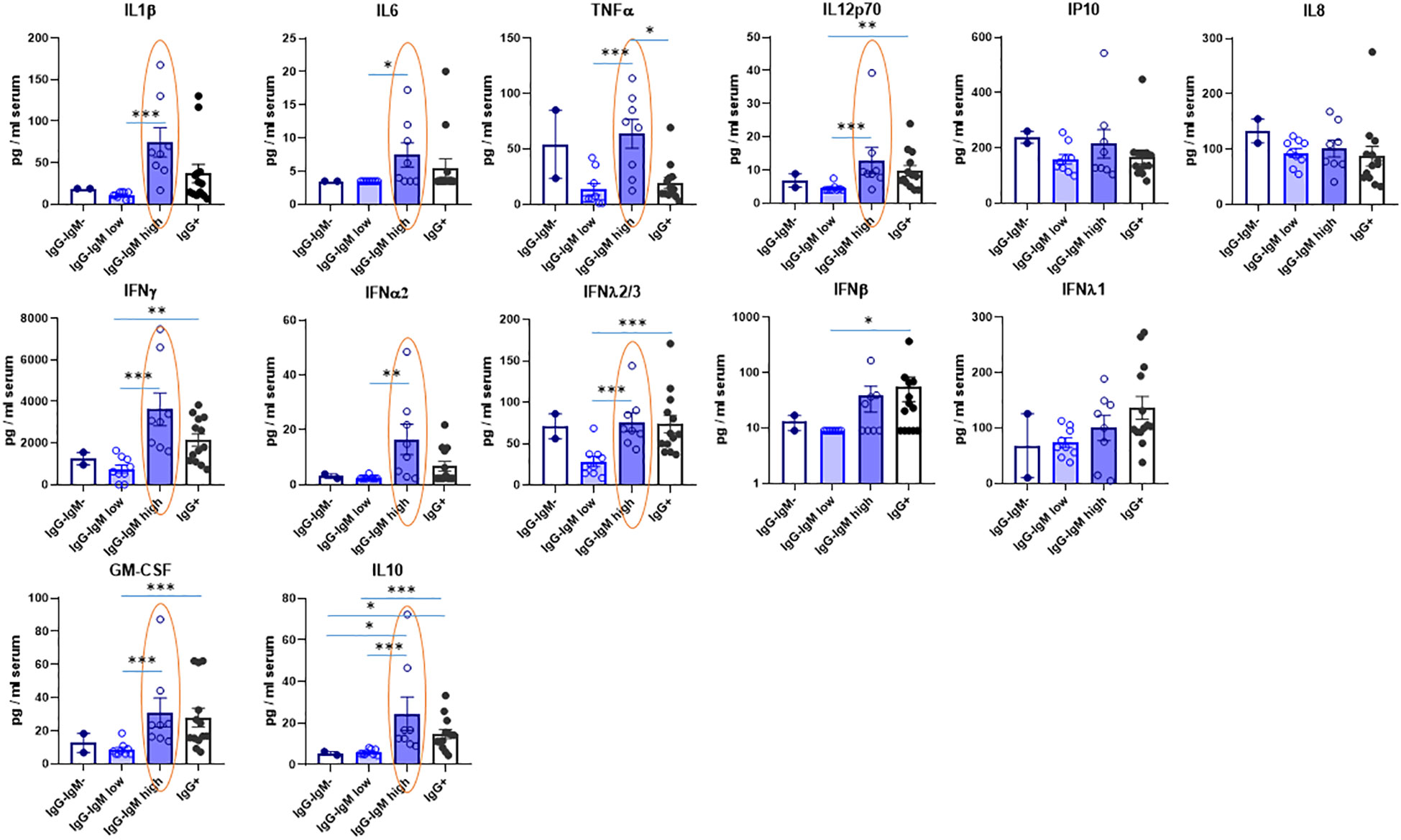
Figure 3 Levels of cytokines in serum of children according to their immunological status. Serum levels were mesured by Multiplex Cytokine Assay as described in methods in the indicated four groups: IgG-IgM-, IgG-IgMlow, IgG-IgMhigh, IgG+. The statistical differences between the IgG-IgMlow, IgG-IgMhigh and IgG. groups are indicated. *p-adjusted<0,05; **p-adjusted<0,01; ***p-adjusted<0,005.
The serum of highly exposed IgG-negative children has the capacity to neutralize SARS-CoV-2
To further explore why subjects in contact with the virus remained IgG seronegative, we investigated the ability of the lentivirus pseudotyped with the spike protein to infect ACE2-expressing cells in the presence of the sera of these subjects. As expected, all but one serum from IgG+ children neutralized the virus infection very efficiently (Figure 4). Nonetheless, despite having no IgG antibodies against the S protein, most of the sera from the IgG−IgMhigh or IgG−IgMlow groups showed a broad pattern of neutralizing activity (Figure 4). The sera from unexposed seronegative adults or prepandemic children did not neutralize [(9) and data not shown]. Considering that those groups had IgA anti-S, we performed a linear correlation. We found that in the IgG−IgMlow group, the neutralization of those sera significantly correlates with IgA anti-S1 (p = 0.0216) (Figure 4).
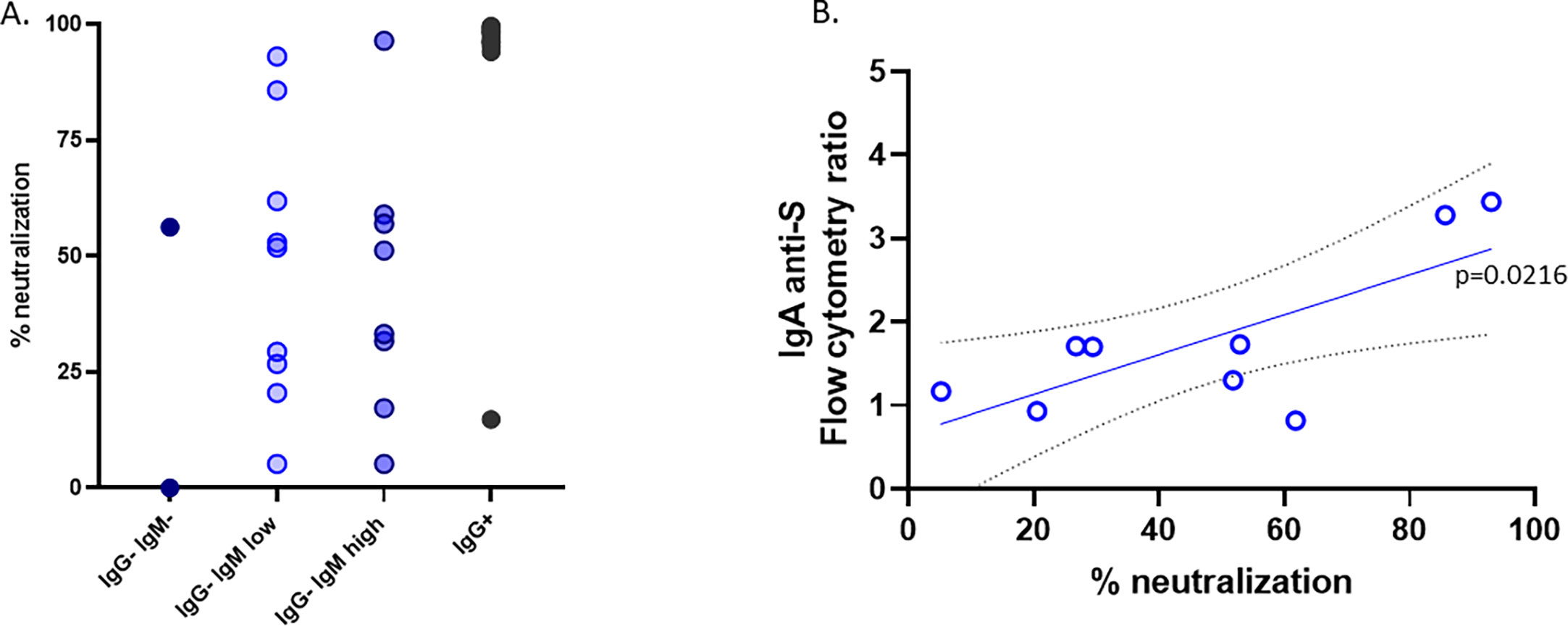
Figure 4 Neutralization. (A) The neutralization assay was carried out with the pseudotyped virus as described in the Material and methods. (B) Group IgG−IgMlow showed a significant correlation between the neutralizing activity and the levels of IgA anti-S in the serum.
Discussion
Children are less susceptible than adults to symptomatic COVID‐19 infection, but the basis for this outcome is unclear and very few studies addressed their underlying cause. Moreover, very few studies analyzed children highly exposed to the virus, who nevertheless remain apparently uninfected. Few studies, much less than in adults, have investigated the response to children (12, 13, 23, 24) but none, to our knowledge, of children cohabiting in close contact with infected patients without taking any preventive measures. Here, we have addressed this question through this descriptive, cross-sectional, and retrospective study.
Most children during the first waves were reported to be infected by close relatives (24). Surprisingly, we found that only 15/40 children in close contact with infected relatives have detectable IgG antibodies in their sera by the very sensitive JFCI test (20) indicative of a previous SARS-CoV-2 infection. Moreover, other ELISA tests against S1 or N also failed to detect IgG against the virus in those IgG-negative (by JFCI) patients. Thus, 25/40 children did not show positivity in any of those three tests despite cohabiting with an infected person(s) for a long time without taking preventive measures.
Nonetheless, a thorough analysis of immune response to track the possible SARS-CoV-2 infection indicated that all but two must have been in contact with the virus and mounted an immune response against it, since they had variable levels of IgM anti-S1 and/or anti-N protein and specific IgA antibodies against the spike protein in their serum. The IgG−IgMlow subgroup was mainly positive for IgM antibodies against the N protein. Those antibodies may have arisen due to a possible cross-reaction with the common nucleocapsid protein expressed by other coronaviruses. However, nine children in the IgG-seronegative group were positive for IgA anti-S, and most of them (n = 7) belong to the IgG−IgMlow subgroup, indicative of some kind of SARS-CoV-2 infection beyond a possible cross-reaction with another coronavirus.
Since most of the analysis of the epidemiological surveys used IgG anti-SARS-CoV-2 for defining people that pass the infection, extrapolating our data would indicate that the extent of the disease in children would be greater than reported. In this regard, whether COVID-19 is less common in children is debated. Some propose that children are less susceptible, whereas others believe that children become infected as adults but are less likely to be symptomatic (25).
More importantly, our analyses defined two different IgG− seropositive groups according to the levels of IgM, anti-S, or anti-N: IgG−IgMhigh, a combination of medium to high levels of IgM anti-S and anti-N, and IgG−IgMlow, only with IgM anti-N. Although those differences were somewhat arbitrary, the subsequent analysis demonstrated that both groups defined very different immune responses to the virus. Thus, the IgG−IgMhigh group, which has higher levels of IgM anti-S1 and anti-N than the IgG−IgMlow group, also tended to be younger. By contrast, the levels of IgA anti-S, determined by a sensitive flow cytometry test, were more frequent and higher in the IgG−IgMlow group, although lower than in the IgG+ group.
Nonetheless, in many of the other parameters analyzed, those groups have strikingly different behavior suggesting a different immunological/biological response to the virus. Thus, the IgG−IgMlow group has much lower serum ACE2 than the rest. Furthermore, an analysis of parameters indicative of potential innate responses such as the levels of serum cytokines also revealed striking differences between the groups. The IgG−IgMhigh group has the highest levels of some antiviral IFNs, such as IFN-α2, IFN-λ2/3, and IFN-γ, among all groups, whereas the IgG−IgMlow group has lower levels. Other cytokines, such as the proinflammatory IL-1/TNF/IL-6, as well as GM-CSF and IL-10, were also higher in the IgG−IgMhigh group than in the IgG−IgMlow group. Moreover, the levels of those cytokines in the IgG−IgMhigh group were even higher in many instances than in the IgG+ group.
Surprisingly, the sera from the IgG−IgMlow group, despite having lower antiviral and macrophage-activating cytokines and no detectable IgM anti-S, have a weak but detectable viral neutralization ability. Despite not having IgG anti-S, the IgG−IgMhigh and IgG−IgMlow groups had a similar neutralization capability. Indeed, in the IgG−IgMlow group, this weak neutralization correlates to IgA anti-S levels.
We do not know the reason for the differences between the two IgG-IgM+ groups, but we speculate that the IgG−IgMlow group represents a group of children with the lowest SARS-CoV-2 receptor, ACE2, which may be the reason for the reduced virus entry. The role of ACE2 expression levels on SARS-CoV-2 infection severity is still debated. Membrane-bound ACE2 is the main cellular receptor of SARS-CoV-2, and lower levels are expected to reduce infection of airway epithelial cells. In this regard, it was proposed that the lower expression of the viral receptor ACE2 in children in the nasal epithelium and serum protects them from severe COVID-19 (15, 16) and that patients with a lower expression level of ACE2 are less vulnerable to developing severe symptoms (26). Moreover, sACE2 tends to increase with age (27, 28). The presence of IgA antibodies in mucosal sites against SAR-CoV-2 with good neutralizing activity was described in IgG-seronegative patients with mild disease (29). IgA is the main immunoglobulin in the respiratory tract and contributes to virus neutralization more heavily than IgG (30, 31). Thus, neutralizing IgA anti-S antibodies in the IgG+IgMlow group could also, besides low ACE2 levels, explain their resistance to a more productive infection.
On the other hand, we propose that the IgG−IgMhigh group could include a group of children with an innate immune response. This hypothesis is based on cytokine levels, which are elevated in this group, having the highest levels of antiviral interferons, such as IFN-α2 and IFN-γ, among all the groups. The protective antiviral response in circulating immune cells of adult COVID patients is strongly associated with a specific subset of IFNs, most prominently IFN-α2 and IFN-γ, which are inversely correlated with severity (32). Interestingly, those are the ones we show elevated in IgG−IgMhigh children. Moreover, children have higher nasal IFN-α2, IFN-γ, and IL-1β cytokine levels than adults. Those cytokines had been associated with lower susceptibility, indicating a stronger mucosal innate immune response in children than in adults that may contribute to milder clinical outcomes (33).
It has been proposed that polyclonal native IgM may protect children from SARS-CoV-2 infection (14). This polyclonal IgM is abundantly present in neonates and children and can recognize viral particles or infected cells, being also able to recognize self- and altered self-antigens. Native IgM may play a role in defense against SARS-CoV-2 since it may neutralize the virus through the recognition of endogenous “danger signals” encoded by the virus (14). The IgM antibodies in the IgG−IgMhigh group may also be natural antibodies and, together with the low ACE2 levels, one of the causes of viral resistance. Aside from IgM, natural IgA production has also been described to be derived from B1 lymphocytes by a T-independent mechanism (30). In this regard, purified IgM and IgA fractions of patient sera display neutralizing activities (34). Unfortunately, we do not have data on T-cell response. Nonetheless, the fact that most of those children, despite being highly exposed, did not suffer a “classical” SARS-CoV-2 infection (defined by the generation of IgG anti-S or anti-N due to antigen presentation and T–B cooperation) may argue against a SARS-CoV-2-specific T-cell response in the IgG-seronegative children. Thus, a rather plausible scenario is that those IgA and IgM antibodies present in IgG-negative children may be natural antibodies derived from B1 lymphocytes by a T-independent mechanism. It is important to note that blood samples were obtained 2 months after the virus exposure, time enough for the IgG isotype switch to occur. B1 cells appear early in the ontogeny, decreasing with age and providing an essential link between innate and adaptive immune systems (35). Our hypothesis is supported by the fact that B1 lymphocytes have been described to be high in children and protect against respiratory viruses such as influenza (36), and it has also been proposed as a mechanism for resistance to SARS-CoV-2 (30, 37). Indeed, B1 lymphocytes secrete natural IgM, IL-10, and GM-CSF (37), and we found higher IL-10 and GM-CSF in the IgG−IgMhigh group, strongly supporting that there are B1 cells involved in the resistance of those children. Unfortunately, we do not have the cellular sample to corroborate this hypothesis.
In summary, we have identified different mechanisms that may play some role in children’s resistance to SARS-CoV-2 infection. Children with higher levels of IFNs in the airways can clear the virus faster as well as children with low levels of ACE2 receptors in which the virus does not have the opportunity to replicate to a high level in the mucosa. Both mechanisms may have prevented the spread of the virus into the body, avoiding a classical T/B response defined by the production of IgG immunoglobulin.
Data availability statement
The original contributions presented in the study are included in the article/Supplementary Material. Further inquiries can be directed to the corresponding authors.
Ethics statement
The studies involving human participants were reviewed and approved by the local Research Ethics Committee (ceic.hrc@salud.madrid.org, approval number 095/20). Written informed consent to participate in this study was provided by the participants’ legal guardian/next of kin.
Author contributions
MU, MM, PD, and LH conducted the experiments. MU acquired and analyzed the data. LG-B, CC, and TS provided human biospecimens. MU and MF analyzed and interpreted the data. TS conceived the clinical recruitment of the patients. DA and UB performed bioinformatics to analyze the data. MF and UB conceived and designed this study. MF wrote the manuscript. All authors contributed to the article and approved the submitted version.
Funding
This research was funded by grants from “Ministerio de Ciencia e Innovación” (PID-2019104760RB-100); “Comunidad de Madrid” (S2017/BMD-3671. INFLAMUNE-CM; FEDER and COTRAVI-19-CM) to MF; Consejo Superior de Investigaciones Científica, CSIC (CSIC-COV19-108, SGL210235) to MF and UB; CRUE-Supera COVID; the European Development Regional Fund “A way to achieve Europe” (ERDF); Merck, Sharp and Dohme Investigator Studies Program (code MISP# IIS 60257); and Fondo Supera COVID-19 (2020–001) to SSV. The study also received institutional grants from “Fundación Ramón Areces” and “Banco de Santander.”
Conflict of interest
The authors declare that the research was conducted in the absence of any commercial or financial relationships that could be construed as a potential conflict of interest.
Publisher’s note
All claims expressed in this article are solely those of the authors and do not necessarily represent those of their affiliated organizations, or those of the publisher, the editors and the reviewers. Any product that may be evaluated in this article, or claim that may be made by its manufacturer, is not guaranteed or endorsed by the publisher.
Supplementary material
The Supplementary Material for this article can be found online at: https://www.frontiersin.org/articles/10.3389/fimmu.2023.1105237/full#supplementary-material
References
1. Lu R, Zhao X, Li J, Niu P, Yang B, Wu H, et al. Genomic characterisation and epidemiology of 2019 novel coronavirus: implications for virus origins and receptor binding. Lancet (London England) (2020) 395(10224):565–74. doi: 10.1016/S0140-6736(20)30251-8
2. Hoffmann M, Kleine-Weber H, Schroeder S, Krüger N, Herrler T, Erichsen S, et al. SARS-CoV-2 cell entry depends on ACE2 and TMPRSS2 and is blocked by a clinically proven protease inhibitor. Cell (2020) 181(2):271–80.e8. doi: 10.1016/j.cell.2020.02.052
3. Abassi Z, Higazi AAR, Kinaneh S, Armaly Z, Skorecki K, Heyman SN. ACE2, COVID-19 infection, inflammation, and coagulopathy: Missing pieces in the puzzle. Front Physiol (2020) 11:574753. doi: 10.3389/fphys.2020.574753
4. Gaddam RR, Chambers S, Bhatia M. ACE and ACE2 in inflammation: a tale of two enzymes. Inflammation Allergy Drug Targets (2014) 13(4):224–34. doi: 10.2174/1871528113666140713164506
5. Bastolla U, Chambers P, Abia D, Garcia-Bermejo M-L, Fresno M. Is covid-19 severity associated with ACE2 degradation? Front Drug Discovery (2022) 1. doi: 10.3389/fddsv.2021.789710
6. Dhochak N, Singhal T, Kabra SK, Lodha R. Pathophysiology of COVID-19: Why children fare better than adults? Indian J Pediatr (2020) 87(7):537–46. doi: 10.1007/s12098-020-03322-y
7. Magrone T, Magrone M, Jirillo E. Focus on receptors for coronaviruses with special reference to angiotensin-converting enzyme 2 as a potential drug target - a perspective. Endocr Metab Immune Disord Drug Targets (2020) 20(6):807–11. doi: 10.2174/1871530320666200427112902
8. Bastolla U. Mathematical model of SARS-Cov-2 propagation versus ACE2 fits COVID-19 lethality across age and sex and predicts that of SARS. Front Mol Biosci (2021) 8:706122. doi: 10.3389/fmolb.2021.706122
9. Maza MDC, Ubeda M, Delgado P, Horndler L, Llamas MA, van Santen HM, et al. ACE2 serum levels as predictor of infectability and outcome in COVID-19. Front Immunol (2022) 13:836516. doi: 10.3389/fimmu.2022.836516
10. El-Shennawy L, Hoffmann AD, Dashzeveg NK, McAndrews KM, Mehl PJ, Cornish D, et al. Circulating ACE2-expressing extracellular vesicles block broad strains of SARS-CoV-2. Nat Commun (2022) 13(1):405. doi: 10.1038/s41467-021-27893-2
11. Singh B, Singh D, Verma V, Yadav R, Kumar R. Angiotensin-converting enzyme 2 as a potential therapeutic target for COVID-19: A review. J Pharm Anal (2022) 12(2):215–20. doi: 10.1016/j.jpha.2021.12.003
12. Cusenza F, Davino G, D'Alvano T, Argentiero A, Fainardi V, Pisi G, et al. Silence of the lambs: The immunological and molecular mechanisms of COVID-19 in children in comparison with adults. Microorganisms (2021) 9(2):330. doi: 10.3390/microorganisms9020330
13. Kapustova L, Petrovicova O, Banovcin P, Antosova M, Bobcakova A, Urbancikova I, et al. COVID-19 and the differences in physiological background between children and adults and their clinical consequences. Physiol Res (2021) 70(S2):S209–s25. doi: 10.33549/physiolres.934759
14. Massalska MA, Gober HJ. How children are protected from COVID-19? a historical, clinical, and pathophysiological approach to address COVID-19 susceptibility. Front Immunol (2021) 12:646894. doi: 10.3389/fimmu.2021.646894
15. Bunyavanich S, Do A, Vicencio A. Nasal gene expression of angiotensin-converting enzyme 2 in children and adults. Jama (2020) 323(23):2427–9. doi: 10.1001/jama.2020.8707
16. Pavel AB, Wu J, Renert-Yuval Y, Del Duca E, Glickman JW, Miller RL, et al. SARS-CoV-2 receptor ACE2 protein expression in serum is significantly associated with age. Allergy (2021) 76(3):875–8. doi: 10.1111/all.14522
17. Palermo E, Di Carlo D, Sgarbanti M, Hiscott J. Type I interferons in COVID-19 pathogenesis. Biol (Basel) (2021) 10(9):829. doi: 10.3390/biology10090829
18. Netea MG, Giamarellos-Bourboulis EJ, Domínguez-Andrés J, Curtis N, van Crevel R, van de Veerdonk FL, et al. Trained immunity: a tool for reducing susceptibility to and the severity of SARS-CoV-2 infection. Cell (2020) 181(5):969–77. doi: 10.1016/j.cell.2020.04.042
19. Choudhary S, Sreenivasulu K, Mitra P, Misra S, Sharma P. Role of genetic variants and gene expression in the susceptibility and severity of COVID-19. Ann Lab Med (2021) 41(2):129–38. doi: 10.3343/alm.2021.41.2.129
20. Horndler L, Delgado P, Abia D, Balabanov I, Martínez-Fleta P, Cornish G, et al. Flow cytometry multiplexed method for the detection of neutralizing human antibodies to the native SARS-CoV-2 spike protein. EMBO Mol Med (2021) 13(3):e13549. doi: 10.15252/emmm.202013549
21. Romero-Pinedo S, Quesada M, Horndler L, Alvarez-Fernandez S, Olmo A, Abia D, et al. Vaccine type-, age- and past infection-dependence of the humoral response to SARS-CoV-2 spike s protein. Front Immunol (2022) 13:809285. doi: 10.3389/fimmu.2022.809285
22. Kleiner G, Marcuzzi A, Zanin V, Monasta L, Zauli G. Cytokine levels in the serum of healthy subjects. Mediators Inflammation (2013) 2013:434010. doi: 10.1155/2013/434010
23. Chou J, Thomas PG, Randolph AG. Immunology of SARS-CoV-2 infection in children. Nat Immunol (2022) 23(2):177–85. doi: 10.1038/s41590-021-01123-9
24. Filippatos F, Tatsi EB, Michos A. Immune response to SARS-CoV-2 in children: A review of the current knowledge. Pediatr Investig (2021) 5(3):217–28. doi: 10.1002/ped4.12283
25. Sinaei R, Pezeshki S, Parvaresh S, Sinaei R. Why COVID-19 is less frequent and severe in children: a narrative review. World J Pediatr (2021) 17(1):10–20. doi: 10.1007/s12519-020-00392-y
26. Amati F, Vancheri C, Latini A, Colona VL, Grelli S, D'Apice MR, et al. Expression profiles of the SARS-CoV-2 host invasion genes in nasopharyngeal and oropharyngeal swabs of COVID-19 patients. Heliyon (2020) 6(10):e05143. doi: 10.1016/j.heliyon.2020.e05143
27. Swärd P, Edsfeldt A, Reepalu A, Jehpsson L, Rosengren BE, Karlsson MK. Age and sex differences in soluble ACE2 may give insights for COVID-19. Crit Care (London England) (2020) 24(1):221. doi: 10.1186/s13054-020-02942-2
28. Kornilov SA, Lucas I, Jade K, Dai CL, Lovejoy JC, Magis AT. Plasma levels of soluble ACE2are associated with sex, metabolic syndrome, and its biomarkers in a large cohort, pointing to a possible mechanism for increased severity in COVID-19. Crit Care (London England) (2020) 24(1):452. doi: 10.1186/s13054-020-03141-9
29. Cervia C, Nilsson J, Zurbuchen Y, Valaperti A, Schreiner J, Wolfensberger A, et al. Systemic and mucosal antibody responses specific to SARS-CoV-2 during mild versus severe COVID-19. J Allergy Clin Immunol (2021) 147(2):545–57.e9. doi: 10.1016/j.jaci.2020.10.040
30. Quinti I, Mortari EP, Fernandez Salinas A, Milito C, Carsetti R. IgA antibodies and IgA deficiency in SARS-CoV-2 infection. Front Cell Infect Microbiol (2021) 11:655896. doi: 10.3389/fcimb.2021.655896
31. Gaebler C, Wang Z, Lorenzi JCC, Muecksch F, Finkin S, Tokuyama M, et al. Evolution of antibody immunity to SARS-CoV-2. Nature (2021) 591(7851):639–44. doi: 10.1038/s41586-021-03207-w
32. Galbraith MD, Kinning KT, Sullivan KD, Araya P, Smith KP, Granrath RE, et al. Specialized interferon action in COVID-19. Proc Natl Acad Sci U.S.A. (2022) 119(11):e2116730119. doi: 10.1073/pnas.2116730119
33. Pierce CA, Sy S, Galen B, Goldstein DY, Orner E, Keller MJ, et al. Natural mucosal barriers and COVID-19 in children. JCI Insight (2021) 6(9):e148694. doi: 10.1172/jci.insight.148694
34. Klingler J, Weiss S, Itri V, Liu X, Oguntuyo KY, Stevens C, et al. Role of immunoglobulin m and a antibodies in the neutralization of severe acute respiratory syndrome coronavirus 2. J Infect Dis (2021) 223(6):957–70. doi: 10.1093/infdis/jiaa784
35. Kumar D, Romero Y, Schuck KN, Smalley H, Subedi B, Fleming SD. Drivers and regulators of humoral innate immune responses to infection and cancer. Mol Immunol (2020) 121:99–110. doi: 10.1016/j.molimm.2020.03.005
36. Smith FL, Baumgarth N. B-1 cell responses to infections. Curr Opin Immunol (2019) 57:23–31. doi: 10.1016/j.coi.2018.12.001
Keywords: COVID-19, children, immune response, protection, cytokines
Citation: Úbeda M, Maza MdC, Delgado P, Horndler L, Abia D, García-Bermejo L, Serrano-Villar S, Calvo C, Bastolla U, Sainz T and Fresno M (2023) Diversity of immune responses in children highly exposed to SARS-CoV-2. Front. Immunol. 14:1105237. doi: 10.3389/fimmu.2023.1105237
Received: 22 November 2022; Accepted: 16 February 2023;
Published: 03 March 2023.
Edited by:
Yi-Hao Chan, The Rockefeller University, United StatesReviewed by:
Tesfaye Gelanew, Armauer Hansen Research Institute, EthiopiaSiew-Wai Fong, A*STAR Infectious Disease Labs, Singapore
Copyright © 2023 Úbeda, Maza, Delgado, Horndler, Abia, García-Bermejo, Serrano-Villar, Calvo, Bastolla, Sainz and Fresno. This is an open-access article distributed under the terms of the Creative Commons Attribution License (CC BY). The use, distribution or reproduction in other forums is permitted, provided the original author(s) and the copyright owner(s) are credited and that the original publication in this journal is cited, in accordance with accepted academic practice. No use, distribution or reproduction is permitted which does not comply with these terms.
*Correspondence: Manuel Fresno, bWZyZXNub0BjYm0uY3Npcy5lcw==; María Úbeda, bXViZWRhQGNibS5jc2ljLmVz