- Immunology, Metastasis & Microenvironment Program, Ellen and Ronald Caplan Cancer Center, The Wistar Institute, Philadelphia, PA, United States
Ovarian cancer metastasis occurs primarily in the peritoneal cavity. Orchestration of cancer cells with various cell types, particularly macrophages, in the peritoneal cavity creates a metastasis-favorable environment. In the past decade, macrophage heterogeneities in different organs as well as their diverse roles in tumor settings have been an emerging field. This review highlights the unique microenvironment of the peritoneal cavity, consisting of the peritoneal fluid, peritoneum, and omentum, as well as their own resident macrophage populations. Contributions of resident macrophages in ovarian cancer metastasis are summarized; potential therapeutic strategies by targeting such cells are discussed. A better understanding of the immunological microenvironment in the peritoneal cavity will provide a stepping-stone to new strategies for developing macrophage-based therapies and is a key step toward the unattainable eradication of intraperitoneal metastasis of ovarian cancer.
Introduction
According to Globocan’s 2020 projections, the incidence rate of ovarian cancer is expected to climb from ~300,000 new cases to ~430,000 new cases worldwide by 2040 (an increase of nearly 37%), with mortality rates also projected to increase by more than 50% (1). Currently, more than 200,000 women die annually from ovarian cancer, making it the second most deadly gynecological cancer (1). Because effective screening methods for early detection have not yet been established (2), patients are often found to have advanced disease that has spread outside the ovaries at the time of diagnosis (3). Although maximum efforts should be made for tumor reduction because complete resection at the primary surgery leads to a good prognosis, complete resection is often difficult due to the spread of the disease (4). Despite advancements in molecular targeted therapies (such as the recent introduction of poly (ADP-ribose) polymerase (PARP) inhibitors and anti-vascular endothelial growth factor (VEGF) monoclonal antibodies), recurrence is inevitable for most patients and the disease eventually becomes resistant to treatment (5, 6). Efficacies of immune checkpoint therapies remain limited in ovarian cancer, which is why immunotherapies have not yet become a standard treatment option (7–10).
Ovarian cancer cases with peritoneal metastasis are very common. More than 75% of patients show intraperitoneal metastases at the time of their first surgery (11) and 75% of recurrent disease is intraperitoneal (12). Because the epithelium of the ovary or the fallopian tube (where ovarian cancer originates) is exposed in the abdominal cavity with no anatomic barriers (13), ovarian cancer cells easily detach from the primary tumor and enter the peritoneal fluid. These cells disseminate throughout the entire peritoneal cavity but preferentially metastasize to the peritoneum and omentum. Here, cancer cells thrive in a favorable tumor microenvironment for survival, engraftment, and development through various interactions with stromal cells (14, 15). Unfortunately, eradication of intra-abdominal lesions in ovarian cancer is an unresolved issue and the efficacy of intraperitoneal chemotherapy and hyperthermic intraperitoneal chemotherapy (HIPEC) is presently inconclusive (16, 17).
Macrophages in the peritoneal cavity play an important role in shaping the tumor microenvironment in ovarian cancer metastasis (18). It has been known for more than half a century that macrophages are abundant in the peritoneal fluid (19). It is now clear that multiple macrophage populations with unique characteristics are present not only in the peritoneal fluid, but also in the peritoneum and omentum in the steady state. These are called tissue-resident macrophages. Elegant lineage-tracing studies in mice in the past decade reveal that tissue-resident macrophages generally have two distinct origins, embryonic precursors prenatally and bone marrow precursors postnatally (i.e., monocyte-derived). The ratios between these two fractions vary across tissue types and are regulated by tissue-specific signals (20–25). Macrophages of different origins can exhibit hardwired differences that may not be as “plastic” as we previously thought (26). Macrophages in tumors (tumor-associated macrophages; TAMs) consist of different proportions of these resident fractions which are present before tumor formation and newly-infiltrated monocyte-derived fraction which come during tumor progression. Importantly, TAMs with different origins have been suggested to play different roles in tumor progression (27). Notably, embryonically-derived resident macrophages in the peritoneal fluid, peritoneum, and omentum have been individually shown to have tumor-promoting role in ovarian cancer (24, 25, 28). Therefore, targeting these cells may be of therapeutic interest for the development of novel anti-cancer immunotherapies.
Here, we provide an overview of the unique microenvironment and resident macrophage populations within the peritoneal fluid, peritoneum, and omentum, as well as their roles in ovarian cancer progression in mice and humans. Detailed knowledge of the intraperitoneal environment, including the origin-based diversity of macrophages, will deepen our understanding about the intraperitoneal metastasis of ovarian cancer. Furthermore, it will help overcome the limited efficacy of macrophage-targeted cancer therapy in clinical settings due to the complexity of macrophage origins, plasticity, and intra-tumor heterogeneity (29, 30), and help toward controlling the ovarian cancer progression in the peritoneal cavity.
The unique intraperitoneal environment and its contribution to ovarian cancer progression
The peritoneal cavity contains serous exudate with various components such as steroid hormones, cytokines, and growth factors at steady state (31, 32). The peritoneal fluid volume is 5-20 mL in humans, which varies widely depending on the physiological condition. For example, in females, this volume changes during the estrus cycle and reaches the maximal level after ovulation (31). Macrophages are the most abundant immune cell population in peritoneal fluid, followed by smaller populations of T cells, dendritic cells, mast cells, NK cells, and B cells (33, 34) (Figure 1A). During ovarian cancer metastasis, cancer cells detach from the primary tumor as single cells or clusters that form multicellular spheroids containing other cellular components such as macrophages and fibroblasts. Epidermal growth factor (EGF) secreted from these cells in spheroids promotes cancer cell growth and survival (Figure 1B). These conditions help metastatic cancer cells floating in the peritoneal fluid overcome anoikis (15, 35, 36).
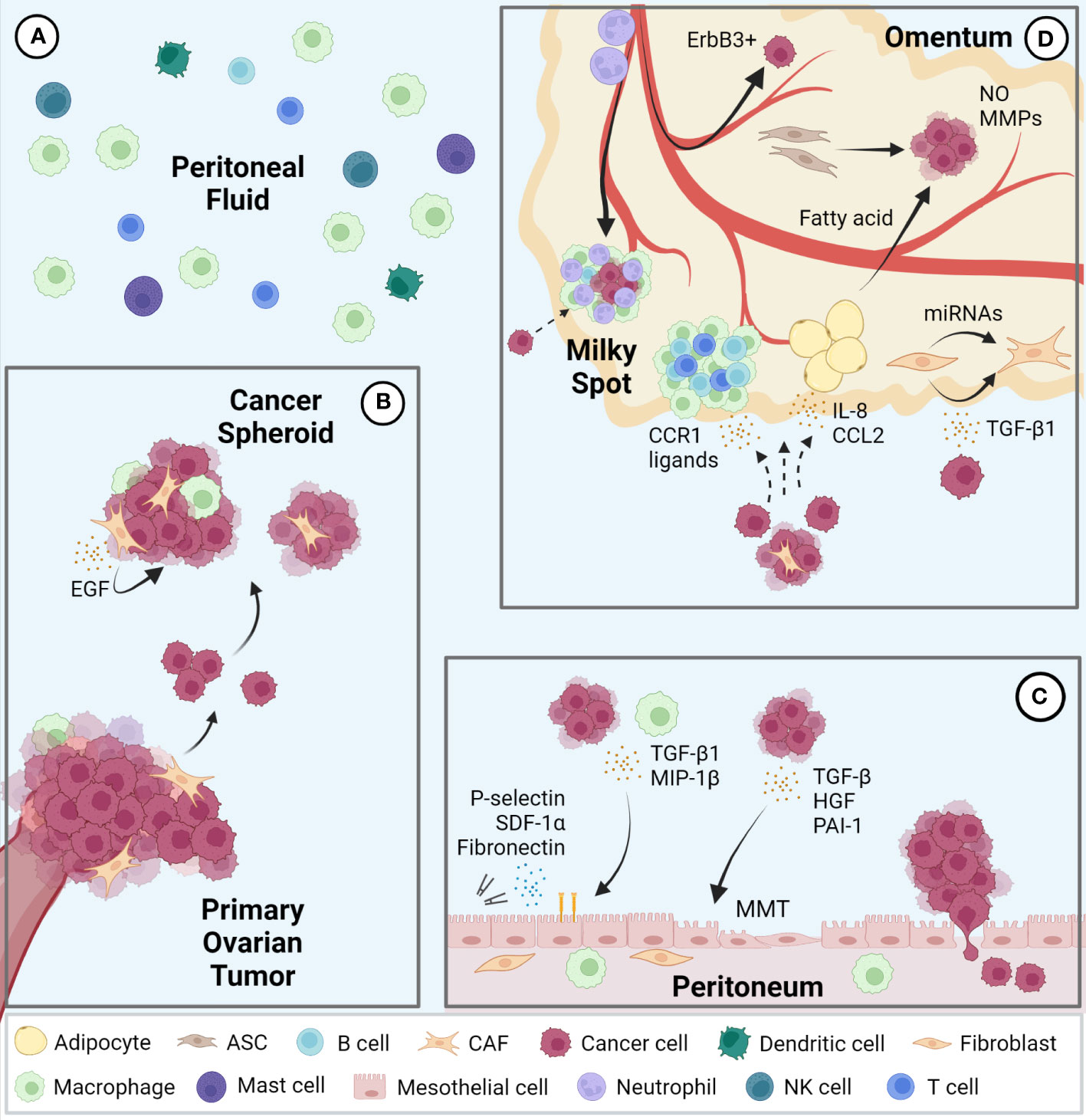
Figure 1 Intraperitoneal metastasis of ovarian cancer. (A) Immune cell populations represented in the peritoneal fluid; macrophages represent the largest population followed by T cells, dendritic cells, mast cells, NK cells, and B cells. (B) Ovarian cancer cells metastasize via detaching from the primary tumor to form multicellular spheroids containing various stromal cells in the peritoneal fluid and seed in pro-tumor secondary sites including the peritoneum (C) and omentum (D). EGF, epidermal growth factor; TGF, transforming growth factor; MIP, macrophage inflammatory protein; MMT, mesothelial-to-mesenchymal transition; SDF, stromal cell-derived factor; hepatocyte growth factor (HGF); PAI, plasminogen activator inhibitor; CCR, C-C motif chemokine receptor; CCL, C-C motif chemokine ligand; NO, nitric oxide; MMP, matrix metalloproteinase; CAF, cancer associated fibroblasts; ASC, adipose-derived stromal cells.
The peritoneal cavity is lined by a single layer of mesothelial cells, known as the mesothelium, which covers all visceral organs. The peritoneal mesothelium not only provides a non-adhesive protective surface to facilitate the movement of organs within the cavity but also serves as an important immune barrier against breaching of microorganisms (37). During ovarian cancer metastasis, mesothelial cells stimulated by cancer cell- or macrophage-derived cytokines, such as transforming growth factor beta 1 (TGF-β1) and macrophage inflammatory protein 1 beta (MIP-1β), promote cancer cell adhesion, invasion, and proliferation via expression of fibronectin, stromal cell-derived factor-1α (SDF-1α) and P-selectin (38–40). Mesothelial cells also support cancer cells by undergoing mesothelial-to-mesenchymal transition (MMT) induced by cytokines such as TGF-β, hepatocyte growth factor (HGF), and plasminogen activator inhibitor-1 (PAI-1) from cancer cells (41–43). In addition, spheroids in contact with mesothelial cells facilitate their migration and clearance, allowing cancer cells access to the sub-mesothelial environment (44) (Figure 1C).
The omentum is a central regulator of intraperitoneal homeostasis, controlling inflammation, regulating fluid exchange, promoting angiogenesis, and storing and supplying lipids (45). Underneath the mesothelial cells that cover the surface are abundant adipocytes, adipose-derived stromal cells (ASCs), fibroblasts, and immune cells (14). Milky spots are lymphoid tissues within the omentum consisting mainly of macrophages, T cells, and B cells. Importantly, the mesothelial lining in the milky spots is not continuous, which enables circulating leukocytes to migrate into the peritoneal cavity (46). Milky spots are also known to be a major implantation site of cancer cell metastasis (47). Indeed, cancer cells preferentially lodge and grow in omental milky spots rather than in other peritoneal fat depots (48). Macrophages in the milky spots promote colonization of cancer cells via secretion of C-C motif chemokine receptor 1 (CCR1) ligand (49). It was also reported that neutrophil influx to the omentum, predominantly in milky spots and through neutrophil extracellular traps (NETs), facilitated a premetastatic niche (50). In addition, a mechanism of hematogenous metastasis with a preference for the omentum via the ErbB3-neuregulin1 axis has been reported (51). Omental adipocytes increase adipokine secretion, such as Interleukin 8 (IL-8) and C-C motif chemokine ligand 2 (CCL2) to promote homing of ovarian cancer cells to the omentum (52, 53). Moreover, once cancer cells are seeded in the omentum, adipocytes transfer fatty acids to cancer cells and increases energy production through fatty acid oxidation (53, 54). ASCs in the omentum increase production of nitric oxide (NO) and matrix metalloproteinases (MMPs) in ovarian cancer cells and promote cancer cell growth and metastasis (55, 56). Finally, ovarian cancer cells can also transform omental fibroblasts into cancer associated fibroblasts (CAFs) via miRNAs and TGF-β1 to modulate the tumor microenvironment at the metastatic niche (57, 58) (Figure 1D).
In summary, there are supportive mechanisms for ovarian cancer that are specific to each of the organs of the peritoneal fluid, peritoneum, and omentum.
Identification and characterization of tissue-resident macrophages in the peritoneal cavity in mice
Peritoneal fluid contains an abundance of immune cells, of which macrophages are the most dominant, accounting for about half of the total number (59). In the steady state, macrophages do not adhere to the peritoneum but float in the peritoneal fluid (59). Fate mapping studies have shown that there are two types of resident macrophages in the peritoneal fluid in mice: Large peritoneal macrophages (LPMs) from embryonic origins and small peritoneal macrophages (SPMs) derived from circulating monocytes (22). LPMs represent approximately 90% of intraperitoneal macrophages at homeostasis and are characterized by the expression of GATA6 and GATA6-regulated peritoneal macrophage-specific genes (20, 21, 60). Retinoic acid supplied by omental adipose tissue and Wilms tumor 1 (WT1)-expressing mesothelial cells or submesothelial fibroblasts plays a central role in GATA6 expression in LPMs (60, 61). Although the composition of SPM niche is unclear, development of SPMs requires IRF4 and signals from microbiome (62). LPMs express a higher level of F4/80 and lower level of MHCII than SPMs (20), however, intracellular adhesion molecule 2 (ICAM2) and Tim-4 are specifically expressed by LPMs and CD226 in SPMs, therefore they are considered selective markers (62–65). Embryo-derived LPMs are partially replaced by monocyte-derived macrophages during aging and inflammation (66–68). Interestingly, monocyte-derived macrophages that replace the resident LPMs after inflammation exhibit a phenotype similar to embryo-derived LPMs (69, 70). LPMs phagocytose more and differentially secrete inflammatory cytokines in response to stimuli when compared to SPMs (71). Moreover, LPMs rapidly form multicellular aggregates at the site of intraperitoneal bacterial exposure or peritoneal injury to control the spread of bacterial infection and assist in wound healing respectively (59, 72, 73). In addition, LPMs are known to be involved in IgA production by B1 cells in gut-associated lymphoid tissue (GALT) (60) and play an important role in B1 cell homeostasis.
In addition to resident macrophages in the peritoneal fluid, macrophages within parietal membranes in mice have been described by Uderhardt et al. (74). Specifically, a resident macrophage population was identified in mesothelial cell layers of the mesentery and peritoneum, characterized by CD64+F4/80+LYVE1hi expression, and noted to be distinct from macrophages in the peritoneal fluid (24). These LYVE1hi membrane-associated macrophages derive primarily from embryonic progenitor cells and are regulated by colony stimulating factor 1 (CSF1), which is produced by WT1+ stromal cells. These macrophages resemble LYVE1+ macrophages present on the surface membranes of other organs (with the exception on the liver) and have a different gene expression phenotype from resident macrophages in other body compartments, such as peritoneal fluid macrophages, alveolar macrophages, and microglia (24).
In the murine omentum, there is also a dominant LYVE1+ macrophage population. Among the LYVE1+ macrophages (also CD169+), the CD163+Tim-4+ population has been shown to be the resident fraction derived from embryonic progenitor cells, while others derive from monocytes. This embryo-derived population is found in the vicinity of the milky spots and has a unique gene expression pattern that is enriched in the JAK-STAT pathway (25). LYVE1+ macrophages in the omentum during the embryonic period have been shown to regulate lymphatic permeability and function through modulation of IL-1β production (75).
Thus, tissue-specific resident macrophage fractions of embryonic or monocyte origins have been identified in the peritoneal fluid, peritoneum and omentum, revealing the origin- and compartment-based diversity of macrophages in the peritoneal cavity (Figure 2). Markers for compartment-specific resident macrophage in the peritoneal cavity are summarized in Figure 3.
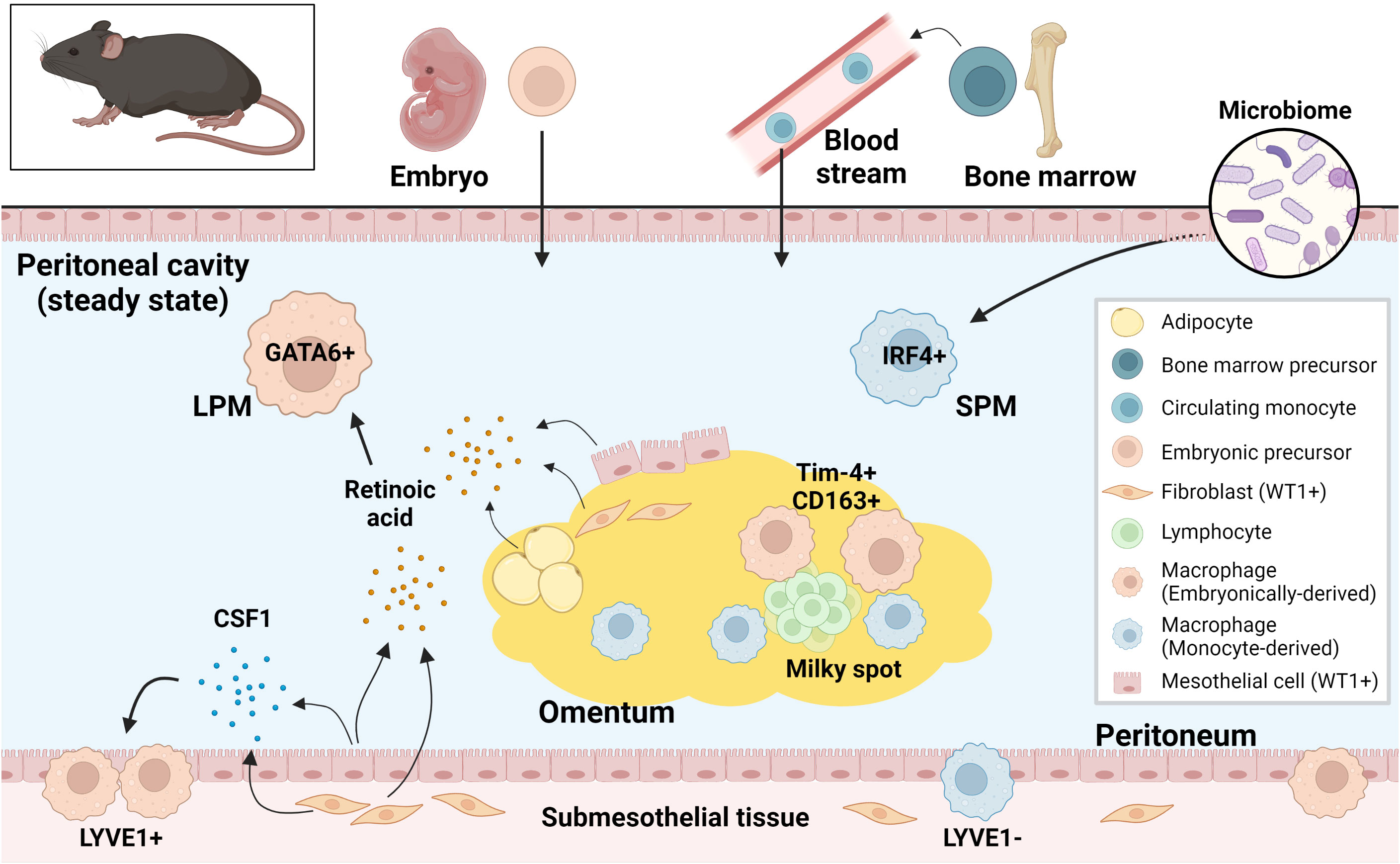
Figure 2 Murine resident peritoneal macrophages and their supporting environment at steady state. Both embryonically-derived and monocyte-derived resident macrophages are present in murine peritoneal cavity at steady state. These resident macrophages from different origins are regulated differently in the tissue-specific niche. LPM, large peritoneal macrophage; SPM, small peritoneal macrophage; CSF1, colony stimulating factor 1; WT1, Wilms tumor 1.
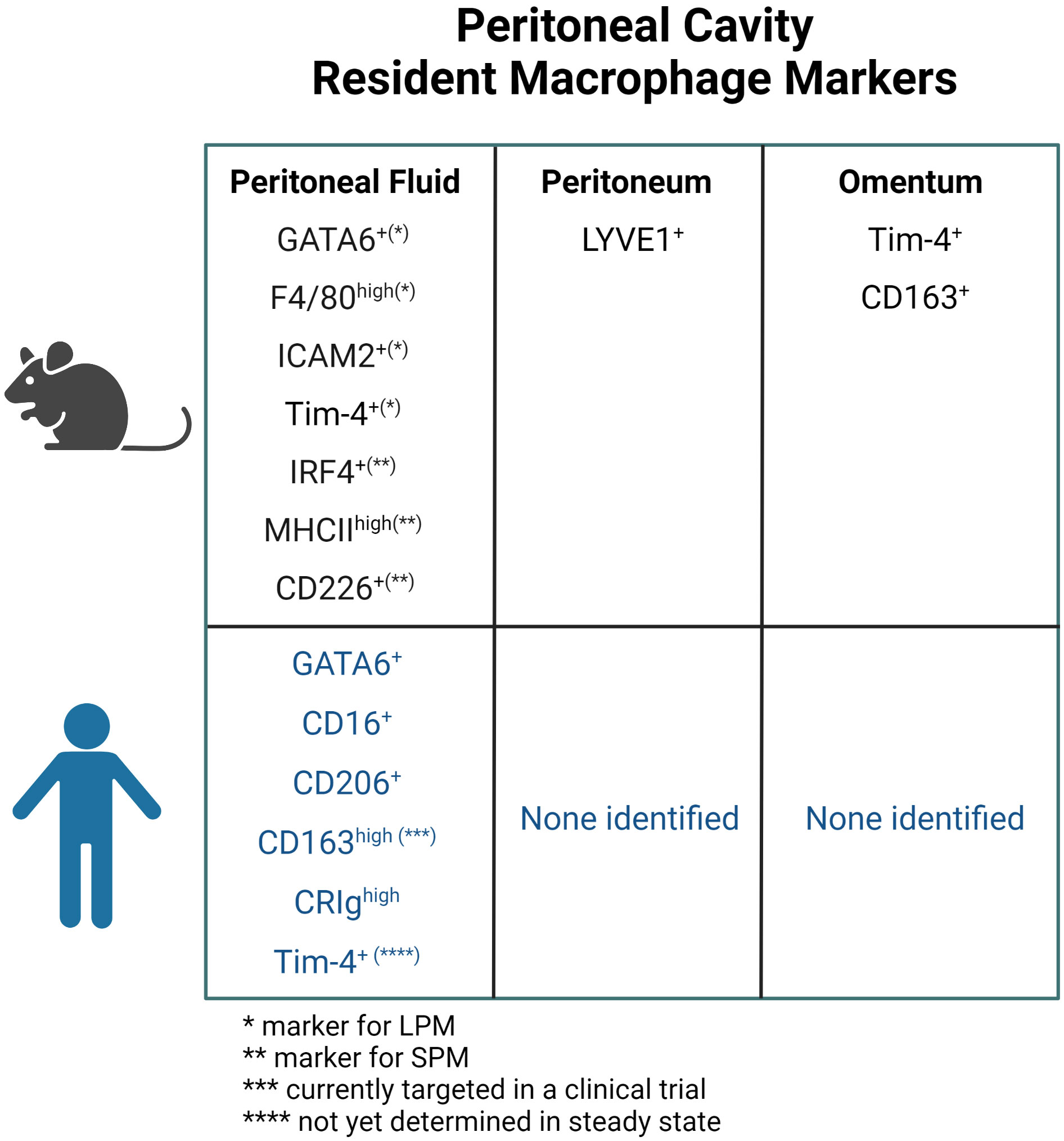
Figure 3 Markers for resident macrophage in the peritoneal fluid, peritoneum, and omentum in mice and human. ICAM, intracellular adhesion molecule; LPM, large peritoneal macrophage; SPM, small peritoneal macrophage.
The role of resident peritoneal macrophages in ovarian cancer progression in mice
Macrophages in the peritoneal cavity contribute to the intraperitoneal metastasis of ovarian cancer through spheroid formation, increased adhesion of cancer cells to mesothelial cells, and colonization to milky spots in omentum (36, 40, 49). Macrophage-mediated inflammation has also been shown to be important in the progression of ovarian cancer (76). In the past decade, it has been elucidated that macrophages of both embryonic and monocytic origins coexist in tumors in varying proportions, and that these different macrophages have distinct effects on tumorigenesis depending on tumor types (27).
First, regarding macrophages in the peritoneal fluid, Xia et al. showed that in an ID8 ovarian cancer model, macrophages in the peritoneal fluid can be divided by Tim-4 expression into the Tim-4+GATA6+ resident type and the Tim-4-GATA6- monocyte-derived type. Functionally, monocyte-derived macrophages do not contribute to ID8 tumorigenesis using CCR2 deficient murine models, whereas resident peritoneal macrophages support tumor progression. These protumor resident macrophages have high arginase 1 expression, high mitophagy activity, and decreased mTORC1 activity. Selective autophagy deficiency in myeloid cells by deleting FIP200 induces loss of Tim-4+ macrophages, enhanced T cell immunity, and suppressed ID8 intraperitoneal tumor growth in vivo (28). Casanova-Acebes et al. showed that Tim-4+GATA6+ LPMs are maintained by retinoid X receptors (RXRs). RXR deficiency 1.) reduces the survival of Tim-4+ LPMs through excess lipid accumulation, 2.) reduces LPM accumulation in early ovarian tumors, and 3.) slows primary ovarian tumor growth (65). Weiss et al. showed that the immune responsive gene 1 (IRG1)-synthesized metabolite, itaconic acid, and oxidative phosphorylation are upregulated in F4/80+ resident macrophages in intraperitoneal tumors. Interestingly, IRG1 deletion in macrophages reduces oxidative phosphorylation and subsequent reactive oxygen species (ROS) production in resident macrophages, which leads to attenuated ROS-mediated MAPK activation in tumor cells and suppressed growth of ID8 intraperitoneal tumors (77).
Embryonic LYVE1+ resident macrophages in the peritoneum are characterized by increased expression of alternatively activated macrophage genes such as Retnla (RELMα) and Mrc1 (CD206). Although LYVE1+ macrophages are also present in the omentum, removal of LYVE1+ macrophages suppressed intraperitoneal tumor growth of ID8 cells in an omentectomized mouse model, demonstrating that LYVE1+ macrophages in the peritoneal membrane promote intraperitoneal expansion of ovarian cancer independent of the omentum (24).
Etzerodt et al. showed that resection of the omentum prior to tumor cell injection delayed intraperitoneal progression in the ID8 mouse model of ovarian cancer, confirming the tumor-promoting properties of the omentum in ovarian cancer. They showed that Tim-4+CD163+ resident macrophages in the omentum contributed to the acquisition of a cancer stem cell (CSC)-like phenotype in tumor cells and increased the tumor cell number in the ascites without affecting tumor development in the omentum. Furthermore, continuous administration of CD163-targeted lipid nanoparticles (LNPs) specifically reduced the numbers of embryonic Tim-4+CD163+ and monocyte-derived Tim-4-CD163+ macrophages. This led to reduced tumor burden in both the omentum and ascites (25). These data indicate that macrophage subpopulations in the omentum may be separately involved in tumor progression. However, the mouse models used in this study cannot separate the role of omental macrophages from membrane macrophages, because both omental and membrane but not fluid macrophages express CD163 (24, 25). Further studies need to design a model that can specifically target the omental macrophage.
In summary, embryonically-derived resident macrophages in the peritoneal fluid, peritoneum, and omentum promote intraperitoneal progression of ovarian cancer. Tim-4, LYVE-1, and CD163 are some of the surface markers for the resident macrophages in the peritoneal fluid, membrane, and omentum, respectively. It remains less well understood what roles monocyte-derived macrophages play in the progression of ovarian cancer in these peritoneal compartments.
Resident peritoneal macrophages and ovarian cancer in humans
Unlike murine resident macrophages, resident peritoneal macrophages in humans are much less well-studied, mainly due to the limited sample availability, particularly in the steady state. Furthermore, it is nearly impossible to definitively determine their precise origin because of ethical concerns. Therefore, in this review, we consider human macrophages as tissue resident when they are present before detectable diseases. Whether they develop prenatally during embryogenesis or postnatally from circulating monocytes remains unknown and difficult to test. Despite such limitations, recent technical advances have led to important discoveries of some aspects of resident peritoneal macrophages in humans.
Like in mice, macrophages are the predominant immune cell component in human peritoneal fluid (78). Although it remains controversial whether resident macrophages in human peritoneal fluid require GATA6 for survival and self-renewal, Ruiz-Alcaraz et al. analyzed peritoneal fluid from patients undergoing tubal ligation or gynecological surgery for benign tumors and found that over 80% of CD14+CD16+ cells in the monocyte/macrophage fraction in humans were GATA6 positive (79). On the other hand, CD14+ macrophages in cancerous ascites did not appear to express GATA6 (80). Interestingly, resident macrophages are decreased in cancer tissues compared to normal tissues in lung cancer (81), which could potentially explain the difference in GATA6 expression in the peritoneal macrophages between healthy donors and cancer patients (67). Interestingly, the analysis of peritoneal fluid from infants to adolescents who underwent surgical procedures that were considered immunologically intact showed that the percentage of CD14+CD16high fractions was higher in infants and lower in adolescent children, which might reflect age-related changes in resident macrophages (82). This is somewhat consistent with the murine data showing that increasing proportions of resident peritoneal macrophages derive from monocyte precursors in the bone marrow as mice age (68).
Two studies have suggested CD16+CD206+ macrophages as resident macrophages in the peritoneal fluid in humans. In one study, a CD16+CD206+ macrophage fraction can be detected in patients before peritoneal dialysis, whose peritoneal environment is considered as homeostatic and physiologic. These CD16+CD206+ “mature” macrophages were abundant before dialysis, but decreased after dialysis, whereas the CD16+CD206- fraction increased after dialysis. Moreover, during acute peritonitis, the CD16+CD206+ macrophage fraction decreased and the CD16-CD206- fraction increased compared to the steady state, which is consistent with a decrease in the resident macrophage fraction during infection in mice (83). In the other study, Stengel et al. characterized macrophages in ascites from cirrhotic patients by CD206 expression and defined CD206+ cells as human LPMs and CD206- cells as human SPMs because the former was larger and more granular. Human LPMs contain a homogenous CD16+ and CCR2- population and express higher levels of CD163 and CRIg than human SPMs. Additionally, human LPMs showed higher Ki-67 positivity than human SPMs, which indicates the increased proliferative potential of human LPMs. Moreover, the proportion of human LPMs decreases in the presence of peritoneal infection, which phenocopies what has been reported in mice LPMs (84). Irvine et al. compared macrophages in human cirrhotic ascites with mouse peritoneal fluid macrophages by RNA sequencing. They showed similarities between CRIghigh human macrophages and F4/80high murine resident macrophages, as well as between CRIglow human macrophages and monocytes and F4/80low murine monocyte-derived macrophages. These hypothesize that CRIghigh and CRIglow macrophages may represent tissue-resident and monocyte-derived populations in humans, respectively (85). Importantly, recent single cell RNA sequence data confirmed expression of CD16 (FCGR3A), CD206 (MRC1), CD163, and CRIg (VSIG4) in macrophages from both peritoneal fluid at steady state and ovarian cancer ascites (33, 86).
Tim-4 is another potential marker for resident peritoneal macrophages in humans. In ovarian cancer ascites from patients, Chow et al. demonstrated the presence of Tim-4+ macrophages (which marks tissue-resident macrophages in murine peritoneal fluid), but Tim-4 was not expressed on steady-state circulating monocytes. Moreover, Tim-4 expression within tumor tissues was detected only in the remaining native tissue compartment, not in the tumor-invaded area, suggesting that Tim-4 is also a specific marker for resident macrophages in human peritoneal fluid (80). Another study by Xia et al. reported only 3% of Tim-4+ macrophages in ovarian cancer ascites. They postulated that Tim-4 may not be a marker for the resident macrophage fraction in ovarian cancer ascites, but they also acknowledged the possibility of poor sensitivity of the anti-human Tim-4 antibody they used. Using RNA seq data of macrophages in ovarian cancer ascites (87), they showed a similarity in ovarian cancer ascites between human CRIghigh macrophages and murine Tim-4+ macrophages, and that ovarian cancer patients with higher CRIghigh expression had poor prognosis (28).
Even less is known about resident macrophages in the peritoneal membrane and omentum in humans. Single-cell RNA seq of metastatic omental tumor of ovarian cancer identified a CD163+CD204+ cluster with high CD14 and CD16 expression and a NR1H2+ cluster in macrophages of all 6 cases (88). This may indicate the presence of specific macrophage subsets in the omentum. However, it is not clear whether these macrophage subsets are also present in the steady state, i.e., resident macrophages.
Thus, it has been suggested that molecules such as CD16, CD206, CD163, CRIg, and Tim-4 may be associated with resident macrophages in the human peritoneal fluid, and macrophages expressing these molecules are also found in ascites from ovarian cancer patients (Figure 3).
Therapeutic potential of resident peritoneal macrophages for ovarian cancer
There has been a significant development of therapies targeting macrophages in the tumor microenvironment. The two main approaches are 1.) reducing the number of macrophages, via CSF1-colony stimulating factor 1 receptor (CSF1R) or CCL2-CCR2 pathway inhibition, and 2.) exploiting the macrophage plasticity by reprogramming immunosuppressive macrophages to immunoreactive, as represented by successful targeting the CD47-SIRPα pathway (30). However, apart from toll-like receptor (TLR) agonists, such as imiquimod and BCG, most of the therapeutic strategies that showed efficacy in preclinical models have yet to become the standard treatment in clinical settings (89). Clinical efficacies of macrophage-targeting therapies in solid tumors have been limited due to the complexity of the origin, plasticity, and intra-tumor heterogeneity of macrophages (27, 29, 30). Recently, adoptive immunotherapy using genetically modified macrophages (chimeric antigen receptor-macrophage; CAR-M) with enhanced phagocytosis and high T cell costimulatory capability has been developed. A Phase I trial using CAR-M that recognize HER2 antigen have been initiated to verify its safety and efficacy (29, 90, 91).
The contribution of resident macrophages to tumor progression has been demonstrated not only in the peritoneal cavity, but also in the brain (92), pancreas (93), and lung (81, 94), suggesting the promising therapeutic potential of targeting resident macrophages in cancer. Below, we highlight strategies of targeting several potential markers of resident peritoneal macrophages as cancer therapies. Because resident macrophages in the omentum and the peritoneal membrane were only recently characterized in tumor models, there has been no published data on specifically targeting these two populations. The following summary only focuses on resident macrophages in the peritoneal fluid.
As previously discussed, Tim-4 is a phosphatidylserine receptor that has been identified in resident peritoneal macrophage in both mice and humans (28, 80, 95). In a murine intraperitoneal metastatic model of colorectal and lung cancer, Tim-4 blockade alone showed no significant effect on tumor progression. However, combined with an immune checkpoint inhibitor, Tim-4 blockade significantly slowed tumor progression, proposing the treatment strategy that blockade of Tim-4-mediated sequestration against phosphatidylserine+CD8+ T cells to enhance the efficacy of CD8+ T cell-based immunotherapies (80). Interestingly, targeting another phosphatidylserine receptor, MerTK, which is in the same pathway (efferocytosis) as Tim-4, in these macrophages increases anti-tumor immunity (96), suggesting that targeting the efferocytosis pathway in resident peritoneal macrophages holds a great promise in treating intraperitoneal metastasis of ovarian cancer.
Another potential cross-species marker of resident peritoneal macrophage is CRIg, also known as VSIG4. It has been characterized as a complement receptor as well as a co-inhibitory immune checkpoint molecule of T cells (97, 98). An exciting ongoing study highlights an anti-CRIg antibody (VTX-1218) by Verseau Therapeutics. Preliminary results suggest a synergistic anti-cancer effect of VTX-1218 and immune checkpoint therapy in syngeneic mouse models. Mechanistically, VTX-1218 repolarizes tumor-associated macrophages into proinflammatory phenotypes and promotes T cell-mediated tumor killing (99). Further studies using VTX-1218 or targeting CRIg in murine models of ovarian cancer is needed to validate these preclinical data targeting this pathway.
CD206 and CD163 are other candidates to target on resident peritoneal macrophages in humans. Because both molecules have garnered attention as a marker of immunosuppressive M2 macrophages within TAMs, therapeutic development targeting them is being vigorously pursued (100, 101). However, it should be noted that those molecules can also be expressed on TAMs differentiated from circulating monocytes and therefore therapies targeting them may not be specific to tissue-resident fraction in cancer setting. For example, CD206 expression can be induced on CD14+ monocytes from peripheral blood cocultured with IL-6, one of the major cytokines upregulated in ovarian cancer ascites (102, 103). Nonetheless, Zhou et al. showed that Fe3O4-based polylactic-glycolic acid (PLGA) nanoparticles, whose surface was modified with an anti-CD206 monoclonal antibody, repolarized M2 macrophages to a M1 phenotype (104). Additionally, Jaynes et al. developed a 10-mer peptide (RP-182) that selectively induces a conformational switch of CD206 from the open to the closed state. This activation enhances endocytosis, phagosome-lysosome formation, and autophagy programs, resulting in reprogramming M2-like tumor associated macrophages to an antitumor M1-like phenotype. RP-182 suppresses tumor growth in a mouse pancreatic cancer model and synergizes with chemotherapy (105). Finally, a bispecific T-cell engager (BiTE) recognizing CD206/CD3 and a trispecific T-cell engager (TriTE) with bivalent anti-CD3 binding have also been developed. These BiTEs and TriTEs can activate T cells and induced cytotoxicity toward M2 macrophages in vitro (106). Etzerodt et al. demonstrated that depletion of CD163+ macrophages with doxorubicin-loaded, antibody-conjugated lipid nanoparticles inhibited tumor growth in an intraperitoneal metastasis model of melanoma (107). Moreover, OR2805, an anti-CD163 antibody, has been developed and is now in phase I/II clinical trials as a single agent or in combination with a PD-1 antibody against multiple tumor types (NCT05094804). Preliminary results demonstrate an anti-tumor activity in lung cancer xenograft models in humanized mice (108).
In summary, therapies targeting molecules that are regarded as markers of resident macrophages are being eagerly developed. In particular, an anti-CD163 antibody is being tested in a phase I/II clinical trial.
Future challenges about resident peritoneal macrophages in relation to ovarian cancer
During intraperitoneal metastasis of ovarian cancer, it is still not clear how much of the intra-tumoral macrophages are derived from the resident fraction, or how plastic they are (i.e., whether they switch between M1/M2 phenotypes). Although macrophages derived from circulating monocytes are generally considered to be highly plastic, tissue-resident macrophages appear to have a restricted plasticity (26). This may be because it is desirable to limit the plasticity of resident macrophages, which remain in tissues for a long time and whose main function is to maintain tissue homeostasis (26). Indeed, in lung cancer, resident macrophages and monocyte-derived macrophages coexist in early lung cancer lesions and retain their distinct phenotypic and molecular programs even in late-stage tumors (81). Therefore, reprograming resident macrophages within human tumors may pose a significant challenge. From this perspective, depletion might be better way than repolarization for targeting resident macrophage.
A lack of understanding resident peritoneal macrophages is another hurdle against the therapeutic development. All murine resident macrophages in the peritoneal cavity (fluid, membrane, omentum) act in a tumor-promoting manner during ovarian cancer metastasis, but the detailed mechanisms of how they contribute to tumor progression is still not fully elucidated. In addition, resident macrophages in the ovary and fallopian tubes have not been studied in detail, and the impact of these resident macrophages on primary tumor development is unknown. Finally, comprehensive characterization of resident macrophages in the human peritoneal cavity has only just begun to emerge. Specific transcriptional regulatory mechanisms modulated by the unique intraperitoneal environment (such as retinoic acid and GATA6 in murine LPMs) have not been identified in humans. Therefore, attempts to translate from preclinical mouse models to human therapies are significantly impinged. Although it remains difficult to collect steady-state samples, it is necessary to continuously attempt a comprehensive analysis of tissue macrophages, including in non-cancerous environments, when approaching the peritoneal cavity during benign or malignant surgeries.
Current therapies for late-stage ovarian cancer patients mainly rely on intravenous chemotherapy. However, the unique microenvironment of the peritoneal cavity (highly dynamic fluidics, crosstalk between multiple tissue compartments, and the peritoneal-plasma barrier) prompts the field to consider shifting to an intraperitoneal delivery (109). Although intraperitoneal chemotherapies show inconsistent results in ovarian cancer patients, they have shown a tremendous improvement in patients with intraperitoneal metastasis of other cancers (109). In order to efficiently and specifically target resident peritoneal macrophages, intraperitoneal injection is also a preferred option in comparison to intravenous injection. Future macrophage-based therapies need to comprehensively compare these two routes of drug delivery in preclinical models and clinical settings.
Concluding remarks
The peritoneal cavity is a dynamic microenvironment with a wide variety of functions that interact closely with all peritoneal tissues. Only recently have resident macrophages in the peritoneal cavity been identified and their pro-tumor roles in ovarian cancer metastasis has come under scrutiny. Because most ovarian cancer growth and metastasis occur in the peritoneal cavity, a better understanding of the unique microenvironment and cellular characteristics present in the peritoneal cavity is essential for combating ovarian cancer metastasis. Besides the well-established T cell-based immune therapies, we call for more extensive research on macrophage-targeting therapies, which may lead to a cure for ovarian cancer in combination with other established immune therapies and targeted therapies.
Author contributions
All authors listed have made a substantial, direct, and intellectual contribution to the work and approved it for publication.
Funding
BM is supported by T32CA009171 through the NCI Training Program in Basic Cancer Research at The Wistar Institute. NZ is supported by a Career Enhancement Program award through Hopkins-Penn-Wistar Ovarian Cancer SPORE (P50CA228991) and the Cancer Center Support Grant at The Wistar Institute (P30CA010815).
Acknowledgments
Figures were created with BioRender.
Conflict of interest
The authors declare that the research was conducted in the absence of any commercial or financial relationships that could be construed as a potential conflict of interest.
Publisher’s note
All claims expressed in this article are solely those of the authors and do not necessarily represent those of their affiliated organizations, or those of the publisher, the editors and the reviewers. Any product that may be evaluated in this article, or claim that may be made by its manufacturer, is not guaranteed or endorsed by the publisher.
References
1. Sung H, Ferlay J, Siegel RL, Laversanne M, Soerjomataram I, Jemal A, et al. Global cancer statistics 2020: GLOBOCAN estimates of incidence and mortality worldwide for 36 cancers in 185 countries. CA Cancer J Clin (2021) 71:209–49. doi: 10.3322/caac.21660
2. Henderson JT, Webber EM, Sawaya GF. Screening for ovarian cancer updated evidence report and systematic review for the US preventive services task force. JAMA - J Am Med Assoc (2018) 319:595–606. doi: 10.1001/jama.2017.21421
3. Armstrong DK, Alvarez RD, Bakkum-Gamez JN, Barroilhet L, Behbakht K, Berchuck A, et al. Ovarian cancer, version 2.2020. JNCCN J Natl Compr Cancer Network (2021) 19:191–226. doi: 10.6004/jnccn.2021.0007
4. Onda T, Satoh T, Ogawa G, Saito T, Kasamatsu T, Nakanishi T, et al. Comparison of survival between primary debulking surgery and neoadjuvant chemotherapy for stage III/IV ovarian, tubal and peritoneal cancers in phase III randomised trial. Eur J Cancer (2020) 130:114–25. doi: 10.1016/j.ejca.2020.02.020
5. Ray-Coquard I, Pautier P, Pignata S, Pérol D, González-Martín A, Berger R, et al. Olaparib plus bevacizumab as first-line maintenance in ovarian cancer. New Engl J Med (2019) 381:2416–28. doi: 10.1056/nejmoa1911361
6. González-Martín A, Pothuri B, Vergote I, DePont Christensen R, Graybill W, Mirza MR, et al. Niraparib in patients with newly diagnosed advanced ovarian cancer. New Engl J Med (2019) 381:2391–402. doi: 10.1056/nejmoa1910962
7. Hamanishi J, Takeshima N, Katsumata N, Kimio U, Kimura T, Takeuchi S, et al. Nivolumab versus gemcitabine or pegylated liposomal doxorubicin for patients with platinum-resistant ovarian cancer: open-label, randomized trial in Japan (NINJA). J Clin Oncol (2021) 39:3671–81. doi: 10.1200/JCO.21
8. Moore KN, Bookman M, Sehouli J, Miller A, Anderson C, Scambia G, et al. Atezolizumab, bevacizumab, and chemotherapy for newly diagnosed stage III or IV ovarian cancer: placebo-controlled randomized phase III trial (IMagyn050/GOG 3015/ENGOT-OV39). J Clin Oncol (2021) 39:1842–55. doi: 10.1200/JCO.21.00306
9. Monk BJ, Colombo N, Oza AM, Fujiwara K, Birrer MJ, Randall L, et al. Chemotherapy with or without avelumab followed by avelumab maintenance versus chemotherapy alone in patients with previously untreated epithelial ovarian cancer (JAVELIN ovarian 100): an open-label, randomised, phase 3 trial. Lancet Oncol (2021) 22:1275–89. doi: 10.1016/S1470-2045(21)00342-9
10. Pujade-Lauraine E, Fujiwara K, Ledermann JA, Oza AM, Kristeleit R, Ray-Coquard IL, et al. Avelumab alone or in combination with chemotherapy versus chemotherapy alone in platinum-resistant or platinum-refractory ovarian cancer (JAVELIN ovarian 200): an open-label, three-arm, randomised, phase 3 study. Lancet Oncol (2021) 22:1034–46. doi: 10.1016/S1470-2045(21)00216-3
11. Sehouli J, Senyuva F, Fotopoulou C, Neumann U, Denkert C, Werner L, et al. Intra-abdominal tumor dissemination pattern and surgical outcome in 214 patients with primary ovarian cancer. J Surg Oncol (2009) 99:424–7. doi: 10.1002/jso.21288
12. Amate P, Huchon C, Dessapt AL, Bensaid C, Medioni J, le Frère Belda MA, et al. Ovarian cancer: sites of recurrence. Int J Gynecological Cancer (2013) 23:1590–6. doi: 10.1097/IGC.0000000000000007
13. Tan DS, Agarwal R, Kaye SB. Mechanisms of transcoelomic metastasis in ovarian cancer. Lancet Oncol (2006) 7:925–34. doi: 10.1016/S1470-2045(06)70939-1
14. Motohara T, Masuda K, Morotti M, Zheng Y, El-Sahhar S, Chong KY, et al. An evolving story of the metastatic voyage of ovarian cancer cells: cellular and molecular orchestration of the adipose-rich metastatic microenvironment. Oncogene (2019) 38:2885–98. doi: 10.1038/s41388-018-0637-x
15. Lengyel E. Ovarian cancer development and metastasis. Am J Pathol (2010) 177:1053–64. doi: 10.2353/ajpath.2010.100105
16. Walker JL, Brady MF, Wenzel L, Gini, Fleming F, Huang HQ, et al. Randomized trial of intravenous versus intraperitoneal chemotherapy plus bevacizumab in advanced ovarian carcinoma: an NRG Oncology/Gynecologic oncology group study. J Clin Oncol (2019) 37:1380–90. doi: 10.1200/JCO.18.01568
17. Lim MC, Chang SJ, Park B, Yoo HJ, Yoo CW, Nam BH, et al. Survival after hyperthermic intraperitoneal chemotherapy and primary or interval cytoreductive surgery in ovarian cancer: a randomized clinical trial. JAMA Surg (2022) 157:374–83. doi: 10.1001/jamasurg.2022.0143
18. Liu M, Silva-Sanchez A, Randall TD, Meza-Perez S. Specialized immune responses in the peritoneal cavity and omentum. J Leukoc Biol (2021) 109:717–29. doi: 10.1002/JLB.5MIR0720-271RR
19. Rowley D. Bactericidal activity of macrophages in vitro against escherichia coli. Nature (1958) 181:1738–9. doi: 10.1038/1811738b0
20. Bou Ghosn EE, Cassado AA, Govoni GR, Fukuhara T, Yang Y, Monack DM, et al. Two physically, functionally, and developmentally distinct peritoneal macrophage subsets. Proc Natl Acad Sci U.S.A. (2010) 107:2568–73. doi: 10.1073/pnas.0915000107
21. Gautiar EL, Shay T, Miller J, Greter M, Jakubzick C, Ivanov S, et al. Gene-expression profiles and transcriptional regulatory pathways that underlie the identity and diversity of mouse tissue macrophages. Nat Immunol (2012) 13:1118–28. doi: 10.1038/ni.2419
22. Yona S, Kim KW, Wolf Y, Mildner A, Varol D, Breker M, et al. Fate mapping reveals origins and dynamics of monocytes and tissue macrophages under homeostasis. Immunity (2013) 38:79–91. doi: 10.1016/j.immuni.2012.12.001
23. Sheng J, Ruedl C, Karjalainen K. Most tissue-resident macrophages except microglia are derived from fetal hematopoietic stem cells. Immunity (2015) 43:382–93. doi: 10.1016/j.immuni.2015.07.016
24. Zhang N, Kim SH, Gainullina A, Erlich EC, Onufer EJ, Kim J, et al. Lyve1+ macrophages of murine peritoneal mesothelium promote omentum-independent ovarian tumor growth. J Exp Med (2021) 218:e20210924. doi: 10.1084/jem.20210924
25. Etzerodt A, Moulin M, Doktor TK, Delfini M, Mossadegh-Keller N, Bajenoff M, et al. Tissue-resident macrophages in omentum promote metastatic spread of ovarian cancer. J Exp Med (2020) 217:e20191869. doi: 10.1084/jem.20191869
26. Guilliams M, Svedberg FR. Does tissue imprinting restrict macrophage plasticity? Nat Immunol (2021) 22:118–27. doi: 10.1038/s41590-020-00849-2
27. DeNardo DG, Ruffell B. Macrophages as regulators of tumour immunity and immunotherapy. Nat Rev Immunol (2019) 19:369–82. doi: 10.1038/s41577-019-0127-6
28. Xia H, Li S, Li X, Wang W, Bian Y, Wei S, et al. Autophagic adaptation to oxidative stress alters peritoneal residential macrophage survival and ovarian cancer metastasis. JCI Insight (2020) 5:e141115. doi: 10.1172/JCI.INSIGHT.141115
29. Wang S, Yang Y, Ma P, Zha Y, Zhang J, Lei A, et al. CAR-macrophage: an extensive immune enhancer to fight cancer. EBioMedicine (2022) 76:103873. doi: 10.1016/j
30. Anderson NR, Minutolo NG, Gill S, Klichinsky M. Macrophage-based approaches for cancer immunotherapy. Cancer Res (2021) 81:1201–8. doi: 10.1158/0008-5472.CAN-20-2990
31. diZerega GS, Rodgers KE. The peritoneum. New York, NY: Springer New York (1992). doi: 10.1007/978-1-4613-9235-4
32. Koninckx PR, Kennedy SH, Barlow DH. Endometriotic disease: the role of peritoneal fluid. Hum Reprod Update (1998) 4:741–51. doi: 10.1093/humupd/4.5.741
33. Zou G, Wang J, Xu X, Xu P, Zhu L, Yu Q, et al. Cell subtypes and immune dysfunction in peritoneal fluid of endometriosis revealed by single-cell RNA-sequencing. Cell Biosci (2021) 11:98. doi: 10.1186/s13578-021-00613-5
34. Kubicka U, Olszewski WL, Tarnowski W, Bielecki K, Zio´ AZ, Kowska Z, et al. Normal human immune peritoneal cells: subpopulations and functional characteristics. Scand J Immunol (1996) 44:157–63. doi: 10.1046/j.1365-3083.1996.d01-297.x
35. Gao Q, Yang Z, Xu S, Li X, Yang X, Jin P, et al. Erratum: heterotypic CAF-tumor spheroids promote early peritoneal metastatis of ovarian cancer (Journal of experiments medicine DOI: 10.1084/jem.20181850). J Exp Med (2019) 216:2448. doi: 10.1084/jem.20180765
36. Yin M, Li X, Tan S, Zhou HJ, Ji W, Bellone S, et al. Tumor-associated macrophages drive spheroid formation during early transcoelomic metastasis of ovarian cancer. J Clin Invest (2016) 126:4157–73. doi: 10.1172/JCI87252
37. Mutsaers SE, Prêle CMA, Pengelly S, Herrick SE. Mesothelial cells and peritoneal homeostasis. Fertil Steril (2016) 106:1018–24. doi: 10.1016/j.fertnstert.2016.09.005
38. Kajiyama H, Shibata K, Terauchi M, Ino K, Nawa A, Kikkawa F. Involvement of SDF-1α/CXCR4 axis in the enhanced peritoneal metastasis of epithelial ovarian carcinoma. Int J Cancer (2008) 122:91–9. doi: 10.1002/ijc.23083
39. Kenny HA, Chiang CY, White EA, Schryver EM, Habis M, Romero IL, et al. Mesothelial cells promote early ovarian cancer metastasis through fibronectin secretion. J Clin Invest (2014) 124:4614–28. doi: 10.1172/JCI74778
40. Carroll MJ, Fogg KC, Patel HA, Krause HB, Mancha AS, Patankar MS, et al. Alternatively-activated macrophages upregulate mesothelial expression of p-selectin to enhance adhesion of ovarian cancer cells. Cancer Res (2018) 78:3560–73. doi: 10.1158/0008-5472.CAN-17-3341
41. Sandoval P, Jiménez-Heffernan JA, Rynne-Vidal Á, Pérez-Lozano ML, Gilsanz Á, Ruiz-Carpio V, et al. Carcinoma-associated fibroblasts derive from mesothelial cells via mesothelial-to-mesenchymal transition in peritoneal metastasis. J Pathol (2013) 231:517–31. doi: 10.1002/path.4281
42. Nakamura M, Ono YJ, Kanemura M, Tanaka T, Hayashi M, Terai Y, et al. Hepatocyte growth factor secreted by ovarian cancer cells stimulates peritoneal implantation via the mesothelial-mesenchymal transition of the peritoneum. Gynecol Oncol (2015) 139:345–54. doi: 10.1016/j.ygyno.2015.08.010
43. Peng Y, Kajiyama H, Yuan H, Nakamura K, Yoshihara M, Yokoi A, et al. PAI-1 secreted from metastatic ovarian cancer cells triggers the tumor-promoting role of the mesothelium in a feedback loop to accelerate peritoneal dissemination. Cancer Lett (2019) 442:181–92. doi: 10.1016/j.canlet.2018.10.027
44. Iwanicki MP, Davidowitz RA, Ng MR, Besser A, Muranen T, Merritt M, et al. Ovarian cancer spheroids use myosin-generated force to clear the mesothelium. Cancer Discovery (2011) 1:144–57. doi: 10.1158/2159-8274.CD-11-0010
45. Meza-Perez S, Randall TD. Immunological functions of the omentum. Trends Immunol (2017) 38:526–36. doi: 10.1016/j.it.2017.03.002
46. Krist LFG, Eestermans IL, Steenbergen JJE, Hoefsmit ECM, Cuesta MA, Meyer S, et al. Cellular composition of milky spots in the human greater omentum: an lmmunochemical and ultrastructural study. Anat Rec (1995) 241:163–74. doi: 10.1002/ar.1092410204
47. Liu J, Geng X, Li Y. Milky spots: omental functional units and hotbeds for peritoneal cancer metastasis. Tumor Biol (2016) 37:5715–26. doi: 10.1007/s13277-016-4887-3
48. Clark R, Krishnan V, Schoof M, Rodriguez I, Theriault B, Chekmareva M, et al. Milky spots promote ovarian cancer metastatic colonization of peritoneal adipose in experimental models. Am J Pathol (2013) 183:576–91. doi: 10.1016/j.ajpath.2013.04.023
49. Krishnan V, Tallapragada S, Schaar B, Kamat K, Chanana AM, Zhang Y, et al. Omental macrophages secrete chemokine ligands that promote ovarian cancer colonization of the omentum via CCR1. Commun Biol (2020) 3:524. doi: 10.1038/s42003-020-01246-z
50. Lee WJ, Ko SY, Mohamed MS, Kenny HA, Lengyel E, Naora H. Neutrophils facilitate ovarian cancer premetastatic niche formation in the omentum. J Exp Med (2019) 216:176–94. doi: 10.1084/jem.20181170
51. Pradeep S, Kim SW, Wu SY, Nishimura M, Chaluvally-Raghavan P, Miyake T, et al. Hematogenous metastasis of ovarian cancer: rethinking mode of spread. Cancer Cell (2014) 26:77–91. doi: 10.1016/j.ccr.2014.05.002
52. Sun C, Li X, Guo E, Li N, Zhou B, Lu H, et al. MCP-1/CCR-2 axis in adipocytes and cancer cell respectively facilitates ovarian cancer peritoneal metastasis. Oncogene (2020) 39:1681–95. doi: 10.1038/s41388-019-1090-1
53. Nieman KM, Kenny HA, Penicka CV., Ladanyi A, Buell-Gutbrod R, Zillhardt MR, et al. Adipocytes promote ovarian cancer metastasis and provide energy for rapid tumor growth. Nat Med (2011) 17:1498–503. doi: 10.1038/nm.2492
54. Miranda F, Mannion D, Liu S, Zheng Y, Mangala LS, Redondo C, et al. Salt-inducible kinase 2 couples ovarian cancer cell metabolism with survival at the adipocyte-rich metastatic niche. Cancer Cell (2016) 30:273–89. doi: 10.1016/j.ccell.2016.06.020
55. Rizi BS, Caneba C, Nowicka A, Nabiyar AW, Liu X, Chen K, et al. Nitric oxide mediates metabolic coupling of omentum-derived adipose stroma to ovarian and endometrial cancer cells. Cancer Res (2015) 75:456–71. doi: 10.1158/0008-5472.CAN-14-1337
56. Chu Y, Tang H, Guo Y, Guo J, Huang B, Fang F, et al. Adipose-derived mesenchymal stem cells promote cell proliferation and invasion of epithelial ovarian cancer. Exp Cell Res (2015) 337:16–27. doi: 10.1016/j.yexcr.2015.07.020
57. Mitra AK, Zillhardt M, Hua Y, Tiwari P, Murmann AE, Peter ME, et al. MicroRNAs reprogram normal fibroblasts into cancer-associated fibroblasts in ovarian cancer. Cancer Discovery (2012) 2:1100–8. doi: 10.1158/2159-8290.CD-12-0206
58. Cai J, Tang H, Xu L, Wang X, Yang C, Ruan S, et al. Fibroblasts in omentum activated by tumor cells promote ovarian cancer growth, adhesion and invasiveness. Carcinogenesis (2012) 33:20–9. doi: 10.1093/carcin/bgr230
59. Zhang N, Czepielewski RS, Jarjour NN, Erlich EC, Esaulova E, Saunders BT, et al. Expression of factor V by resident macrophages boosts host defense in the peritoneal cavity. J Exp Med (2019) 216:1291–300. doi: 10.1084/jem.20182024
60. Okabe Y, Medzhitov R. Tissue-specific signals control reversible program of localization and functional polarization of macrophages. Cell (2014) 157:832–44. doi: 10.1016/j.cell.2014.04.016
61. Buechler MB, Kim KW, Onufer EJ, Williams JW, Little CC, Dominguez CX, et al. A stromal niche defined by expression of the transcription factor WT1 mediates programming and homeostasis of cavity-resident macrophages. Immunity (2019) 51:119–130.e5. doi: 10.1016/j.immuni.2019.05.010
62. Kim KW, Williams JW, Wang YT, Ivanov S, Gilfillan S, Colonna M, et al. MHC II+ resident peritoneal and pleural macrophages rely on IRF4 for development from circulating monocytes. J Exp Med (2016) 213:1951–9. doi: 10.1084/jem.20160486
63. Bain CC, Louwe PA, Steers NJ, Bravo-Blas A, Hegarty LM, Pridans C, et al. CD11c identifies microbiota and EGR2-dependent MHCII+ serous cavity macrophages with sexually dimorphic fate in mice. Eur J Immunol (2022) 52:1243–57. doi: 10.1002/eji.202149756
64. Rosas M, Davies LC, Giles PJ, Liao C-T, Kharfan B, Stone TC, et al. The transcription factor Gata6 links tissue macrophage phenotype and proliferative renewal. Sci (1979) (2014) 344:645–8. doi: 10.1126/science.1251414
65. Casanova-Acebes M, Menéndez-Gutiérrez MP, Porcuna J, Álvarez-Errico D, Lavin Y, García A, et al. RXRs control serous macrophage neonatal expansion and identity and contribute to ovarian cancer progression. Nat Commun (2020) 11:1655. doi: 10.1038/s41467-020-15371-0
66. Bain CC, Hawley CA, Garner H, Scott CL, Schridde A, Steers NJ, et al. Long-lived self-renewing bone marrow-derived macrophages displace embryo-derived cells to inhabit adult serous cavities. Nat Commun (2016) 7:ncomms11852. doi: 10.1038/ncomms11852
67. Jayakumar P, Laganson A, Deng M. GATA6+ peritoneal resident macrophage: the immune custodian in the peritoneal cavity. Front Pharmacol (2022) 13:866993. doi: 10.3389/fphar.2022.866993
68. Liu Z, Gu Y, Chakarov S, Bleriot C, Kwok I, Chen X, et al. Fate mapping via Ms4a3-expression history traces monocyte-derived cells. Cell (2019) 178:1509–1525.e19. doi: 10.1016/j.cell.2019.08.009
69. Gundra UM, Girgis NM, Gonzalez MA, Tang MS, van der Zande HJP, da LJ, et al. Vitamin a mediates conversion of monocyte-derived macrophages into tissue-resident macrophages during alternative activation. Nat Immunol (2017) 18:642–53. doi: 10.1038/ni.3734
70. Louwe PA, Badiola Gomez L, Webster H, Perona-Wright G, Bain CC, Forbes SJ, et al. Recruited macrophages that colonize the post-inflammatory peritoneal niche convert into functionally divergent resident cells. Nat Commun (2021) 12:1770. doi: 10.1038/s41467-021-21778-0
71. Cain DW, O’Koren EG, Kan MJ, Womble M, Sempowski GD, Hopper K, et al. Identification of a tissue-specific, C/EBPβ-dependent pathway of differentiation for murine peritoneal macrophages. J Immunol (2013) 191:4665–75. doi: 10.4049/jimmunol.1300581
72. Vega-Pérez A, Villarrubia LH, Godio C, Gutiérrez-González A, Feo-Lucas L, Ferriz M, et al. Resident macrophage-dependent immune cell scaffolds drive anti-bacterial defense in the peritoneal cavity. Immunity (2021) 54:2578–2594.e5. doi: 10.1016/j.immuni.2021.10.007
73. Zindel J, Peiseler M, Hossain M, Deppermann C, Lee WY, Haenni B, et al. Primordial GATA6 macrophages function as extravascular platelets in sterile injury. Sci (1979) (2021) 371:eabe0595. doi: 10.1126/science.abe0595
74. Uderhardt S, Martins AJ, Tsang JS, Lämmermann T, Germain RN. Resident macrophages cloak tissue microlesions to prevent neutrophil-driven inflammatory damage. Cell (2019) 177:541–555.e17. doi: 10.1016/j.cell.2019.02.028
75. Menendez M, Drozd A, Borawska K, Chmielewska JJ, Wu ML, Griffin CT. IL-1β impacts vascular integrity and lymphatic function in the embryonic omentum. Circ Res (2022) 130:366–83. doi: 10.1161/CIRCRESAHA.121.319032
76. Robinson-Smith TM, Isaacsohn I, Mercer CA, Zhou M, van Rooijen N, Husseinzadeh N, et al. Macrophages mediate inflammation-enhanced metastasis of ovarian tumors in mice. Cancer Res (2007) 67:5708–16. doi: 10.1158/0008-5472.CAN-06-4375
77. Weiss JM, Davies LC, Karwan M, Ileva L, Ozaki MK, Cheng RYS, et al. Itaconic acid mediates crosstalk between macrophage metabolism and peritoneal tumors. J Clin Invest (2018) 128:3794–805. doi: 10.1172/JCI99169
78. Ruiz-Alcaraz AJ, Martínez-Banaclocha H, Marín-Sánchez P, Carmona-Martínez V, Iniesta-Albadalejo MA, Tristán-Manzano M, et al. Isolation of functional mature peritoneal macrophages from healthy humans. Immunol Cell Biol (2020) 98:114–26. doi: 10.1111/imcb.12305
79. Ruiz-Alcaraz AJ, Carmona-Martínez V, Tristán-Manzano M, Machado-Linde F, Sánchez-Ferrer ML, García-Peñarrubia P, et al. Characterization of human peritoneal monocyte/macrophage subsets in homeostasis: phenotype, GATA6, phagocytic/oxidative activities and cytokines expression. Sci Rep (2018) 8:12794. doi: 10.1038/s41598-018-30787-x
80. Chow A, Schad S, Green MD, Hellmann MD, Allaj V, Ceglia N, et al. Tim-4+ cavity-resident macrophages impair anti-tumor CD8+ T cell immunity. Cancer Cell (2021) 39:973–988.e9. doi: 10.1016/j.ccell.2021.05.006
81. Casanova-Acebes M, Dalla E, Leader AM, LeBerichel J, Nikolic J, Morales BM, et al. Tissue-resident macrophages provide a pro-tumorigenic niche to early NSCLC cells. Nature (2021) 595:578–84. doi: 10.1038/s41586-021-03651-8
82. Schukfeh N, Elyas A, Viemann D, Ure BM, Froemmel S, Park JK, et al. Phenotypic switch of human peritoneal macrophages during childhood. Eur J Pediatr Surg (2021) 31:86–94. doi: 10.1055/s-0040-1717088
83. Liao C, Andrews R, Wallace LE, Khan MWA, Kift-Morgan A, Topley N, et al. Peritoneal macrophage heterogeneity is associated with different peritoneal dialysis outcomes. Kidney Int (2017) 91:1088–103. doi: 10.1016/j.kint.2016.10.030
84. Stengel S, Quickert S, Lutz P, Ibidapo-Obe O, Steube A, Köse-Vogel N, et al. Peritoneal level of CD206 associates with mortality and an inflammatory macrophage phenotype in patients with decompensated cirrhosis and spontaneous bacterial peritonitis. Gastroenterology (2020) 158:1745–61. doi: 10.1053/j.gastro.2020.01.029
85. Irvine KM, Banh X, Gadd VL, Wojcik KK, Ariffin JK, Jose S, et al. CRIg-expressing peritoneal macrophages are associated with disease severity in patients with cirrhosis and ascites. JCI Insight (2016) 1:e86914. doi: 10.1172/jci.insight.86914
86. Izar B, Tirosh I, Stover EH, Wakiro I, Cuoco MS, Alter I, et al. A single-cell landscape of high-grade serous ovarian cancer. Nat Med (2020) 26:1271–9. doi: 10.1038/s41591-020-0926-0
87. Finkernagel F, Reinartz S, Lieber S, Adhikary T, Wortmann A, Hoffmann N, et al. The transcriptional signature of human ovarian carcinoma macrophages is associated with extracellular matrix reorganization . Available at: www.impactjournals.com/oncotarget.
88. Olalekan S, Xie B, Back R, Eckart H, Basu A. Characterizing the tumor microenvironment of metastatic ovarian cancer by single-cell transcriptomics. Cell Rep (2021) 35:109165. doi: 10.1016/j.celrep.2021.109165
89. Farooq M, Batool M, Kim MS, Choi S. Toll-like receptors as a therapeutic target in the era of immunotherapies. Front Cell Dev Biol (2021) 9:756315. doi: 10.3389/fcell.2021.756315
90. Klichinsky M, Ruella M, Shestova O, Lu XM, Best A, Zeeman M, et al. Human chimeric antigen receptor macrophages for cancer immunotherapy. Nat Biotechnol (2020) 38:947–53. doi: 10.1038/s41587-020-0462-y
91. Reiss K, Yuan Y, Barton D, Ronczka A, Cushing D, Klichinsky M, et al. 951 a phase 1 first in human study of adenovirally transduced anti-HER2 CAR macrophages in subjects with HER2 overexpressing solid tumors: preliminary safety, pharmacokinetics, and TME reprogramming data. J Immunother Cancer (2021) 9:A1000–0. doi: 10.1136/jitc-2021-sitc2021.951
92. Bowman RL, Klemm F, Akkari L, Pyonteck SM, Sevenich L, Quail DF, et al. Macrophage ontogeny underlies differences in tumor-specific education in brain malignancies. Cell Rep (2016) 17:2445–59. doi: 10.1016/j.celrep.2016.10.052
93. Zhu Y, Herndon JM, Sojka DK, Kim KW, Knolhoff BL, Zuo C, et al. Tissue-resident macrophages in pancreatic ductal adenocarcinoma originate from embryonic hematopoiesis and promote tumor progression. Immunity (2017) 47:323–338.e6. doi: 10.1016/j.immuni.2017.07.014
94. Loyher PL, Hamon P, Laviron M, Meghraoui-Kheddar A, Goncalves E, Deng Z, et al. Macrophages of distinct origins contribute to tumor development in the lung. J Exp Med (2018) 215:1–18. doi: 10.1084/jem.20180534
95. Liu W, Xu L, Liang X, Liu X, Zhao Y, Ma C, et al. Tim-4 in health and disease: friend or foe? Front Immunol (2020) 11:537. doi: 10.3389/fimmu.2020.00537
96. Zhou Y, Fei M, Zhang G, Liang WC, Lin WY, Wu Y, et al. Blockade of the phagocytic receptor MerTK on tumor-associated macrophages enhances P2X7R-dependent STING activation by tumor-derived cGAMP. Immunity (2020) 52:357–373.e9. doi: 10.1016/j.immuni.2020.01.014
97. Helmy KY, Katschke KJ, Gorgani NN, Kljavin NM, Elliott JM, Diehl L, et al. CRIg: a macrophage complement receptor required for phagocytosis of circulating pathogens. Cell (2006) 124:915–27. doi: 10.1016/j.cell.2005.12.039
98. Vogt L, Schmitz N, Kurrer MO, Bauer M, Hinton HI, Behnke S, et al. VSIG4, a B7 family-related protein, is a negative regulator of T cell activation. J Clin Invest (2006) 116:2817–26. doi: 10.1172/JCI25673
99. Sazinsky S, Nguyen P, Zafari M, Phennicie R, Wahle J, Komoroski V, et al. 886 targeting VSIG4, a novel macrophage checkpoint, repolarizes suppressive macrophages which induces an inflammatory response in primary cell in vitro assays and fresh human tumor cultures. J Immunother Cancer (2021) 9:A928–8. doi: 10.1136/jitc-2021-SITC2021.886
100. Roszer T. Understanding the mysterious M2 macrophage through activation markers and effector mechanisms. Mediators Inflammation (2015) 2015:816460. doi: 10.1155/2015/816460
101. Komohara Y, Jinushi M, Takeya M. Clinical significance of macrophage heterogeneity in human malignant tumors. Cancer Sci (2014) 105:1–8. doi: 10.1111/cas.12314
102. Miyamoto T, Murakami R, Hamanishi J, Tanigaki K, Hosoe Y, Mise N, et al. B7-H3 suppresses antitumor immunity via the CCL2-CCR2-M2 macrophage axis and contributes to ovarian cancer progression. Cancer Immunol Res (2022) 10:56–9. doi: 10.1158/2326-6066.CIR-21-0407
103. Lane D, Matte I, Rancourt C, Piché A. Prognostic significance of IL-6 and IL-8 ascites levels in ovarian cancer patients. BMC Cancer (2011) 11:210. doi: 10.1186/1471-2407-11-210
104. Zhou Y, Que KT, Tang HM, Zhang P, Fu QM, Liu ZJ. Anti-CD206 antibody-conjugated Fe3O4-based PLGA nanoparticles selectively promote tumor-associated macrophages to polarize to the pro-inflammatory subtype. Oncol Lett (2020) 20(6):298. doi: 10.3892/OL.2020.12161
105. Jaynes JM, Sable R, Ronzetti M, Bautista W, Knotts Z, Abisoye-Ogunniyan A, et al. Mannose receptor (CD206) activation in tumor-associated macrophages enhances adaptive and innate antitumor immune responses (2020). Available at: www.imperial.ac.uk/research/animallectins/.
106. Scott EM, Jacobus EJ, Lyons B, Frost S, Freedman JD, Dyer A, et al. Bi- and tri-valent T cell engagers deplete tumour-associated macrophages in cancer patient samples. J Immunother Cancer (2019) 7:320. doi: 10.1186/s40425-019-0807-6
107. Etzerodt A, Tsalkitzi K, Maniecki M, Damsky W, Delfini M, Baudoin E, et al. Specific targeting of CD163+ TAMs mobilizes inflammatory monocytes and promotes T cell-mediated tumor regression. J Exp Med (2019) 216:2394–411. doi: 10.1084/jem.20182124
108. Probst P, Simmons R, Wall V, Zuck M, Bouchlaka M, Lam S, et al. Abstract 1719: OR2805, an anti-CD163 antibody derived from an elite responder to checkpoint inhibitor therapy relieves immunosuppression caused by tumor associated macrophages. Cancer Res (2021) 81:1719. doi: 10.1158/1538-7445.AM2021-1719
Keywords: ovarian cancer, intraperitoneal metastasis, resident macrophages, peritoneal cavity, peritoneal fluid, peritoneum, omentum
Citation: Miyamoto T, Murphy B and Zhang N (2023) Intraperitoneal metastasis of ovarian cancer: new insights on resident macrophages in the peritoneal cavity. Front. Immunol. 14:1104694. doi: 10.3389/fimmu.2023.1104694
Received: 21 November 2022; Accepted: 13 April 2023;
Published: 25 April 2023.
Edited by:
Fengyin Li, University of Science and Technology of China, ChinaReviewed by:
Bryon Johnson, Medical College of Wisconsin, United StatesVenkatesh Krishnan, Genentech Inc., United States
Wenchao Zhou, University of Science and Technology of China, China
Copyright © 2023 Miyamoto, Murphy and Zhang. This is an open-access article distributed under the terms of the Creative Commons Attribution License (CC BY). The use, distribution or reproduction in other forums is permitted, provided the original author(s) and the copyright owner(s) are credited and that the original publication in this journal is cited, in accordance with accepted academic practice. No use, distribution or reproduction is permitted which does not comply with these terms.
*Correspondence: Nan Zhang, nzhang@wistar.org; Taito Miyamoto, tmiyamoto@wistar.org