- 1Charité – Universitätsmedizin Berlin, Department of Neurology and Experimental Neurology, Berlin, Germany
- 2German Center for Neurodegenerative Diseases (DZNE) Berlin, Berlin, Germany
- 3Charité – Universitätsmedizin Berlin, Department of Pediatric Neurology, Berlin, Germany
- 4Charité – Universitätsmedizin Berlin, Center for Chronically Sick Children, Berlin, Germany
- 5Charité – Universitätsmedizin Berlin, German Epilepsy Center for Children and Adolescents, Berlin, Germany
- 6Charité – Universitätsmedizin Berlin, Institute of Cell- and Neurobiology, Berlin, Germany
- 7Berlin Institute of Health (BIH), Berlin, Germany
- 8Charité - Universitätsmedizin Berlin, Institute of Integrative Neuroanatomy, Berlin, Germany
Maternal autoantibodies can be transmitted diaplacentally, with potentially deleterious effects on neurodevelopment. Synapsin 1 (SYN1) is a neuronal protein that is important for synaptic communication and neuronal plasticity. While monoallelic loss of function (LoF) variants in the SYN1 gene result in X-linked intellectual disability (ID), learning disabilities, epilepsy, behavioral problems, and macrocephaly, the effect of SYN1 autoantibodies on neurodevelopment remains unclear. We recruited a clinical cohort of 208 mothers and their children with neurologic abnormalities and analyzed the role of maternal SYN1 autoantibodies. We identified seropositivity in 9.6% of mothers, and seropositivity was associated with an increased risk for ID and behavioral problems. Furthermore, children more frequently had epilepsy, macrocephaly, and developmental delay, in line with the SYN1 LoF phenotype. Whether SYN1 autoantibodies have a direct pathogenic effect on neurodevelopment or serve as biomarkers requires functional experiments.
Introduction
Synapsin 1 (SYN1) is a neuronal phosphoprotein encoded by the SYN1 gene and plays an important role in neurotransmitter release and neuronal plasticity (1, 2). Patients with loss of function (LoF) variants in the SYN1 gene have been associated with X-linked phenotypes consisting of epilepsy, learning difficulties, intellectual disability (ID), macrocephaly, behavioral problems, and autism-spectrum disorders (ASD) (MIM#300491, MIM#300115) (3–5).
In recent years, antineuronal autoantibodies have been increasingly described to cause target-specific autoimmune neurologic disorders, in some cases matching the clinical phenotypes of patient carrying genetic variants of these targets. For example, both patients with variants in GABAA receptor subunit genes and those with GABAA receptor autoantibodies display epilepsy as a common phenotype (6–8). Autoantibodies are usually regarded as those generated within an individual; however, they can also be transferred diaplacentally from mother to child (9). As the blood brain barrier is not yet fully matured, these autoantibodies of maternal origin can target the developing brain and thereby potentially affect neurodevelopment (9). There is increasing evidence that maternal immune activation through autoantibodies can influence the occurrence of neurodevelopmental disorders, such as ASD (9). In addition, multiple murine models have demonstrated the direct deleterious effect of various antineuronal autoantibodies in offspring through gestational transfer (10–13).
In previous studies, autoantibodies against SYN1 have been identified in patients with neurologic and psychiatric disorders (14, 15). We have recently shown that SYN1 autoantibodies in pregnant women are associated with abnormalities of fetal development including intrauterine growth retardation (16). However, their role in mothers of children with defined neurologic disorders has not been studied so far. Thus, we assessed the prevalence of SYN1 autoantibodies in mothers of a cohort of 208 pediatric patients with neurological disorders.
Material and methods
Cell-based assay
Synapsin 1b (SYN1b) cell-based assay (CBA) was performed using human embryonic kidney (HEK293T) cells which were transfected with human SYN1b. Methanol-fixed cells were then incubated with sera diluted 1:300. IgG AF488-antibody (Dianova, #109-545-003), commercial rabbit SYN1/SYN2 antibody (Synaptic Systems, #106002) and anti-rabbit IgG AF594-antibody (Jackson IR, #111-585-003) were used to detect IgG binding. Two independent investigators scored the CBA using the following semi-quantitative score: 0, no binding; 1, unspecific signal (‘background’); 2, positive (intensive binding). Sera were tested on control cells overexpressing the NR1 subunit of the N-methyl-D-aspartate receptor (NMDAR), contactin-associated protein 2 (Caspr2), and SYN1 CBA-positive sera also on untransfected control HEK293T cells to exclude non-specific binding (14, 16).
Enzyme-linked immunosorbent assay
For the ELISA, 200 ng of recombinant rat Syn1-His-EGFP (17) was diluted in PBS and incubated overnight at 4°C in 96-well high-binding plates. Serum (1:200 diluted in PBS containing 1% BSA, 0.05% Tween) was incubated for one hour at room temperature, on which HRP-coupled anti-human IgG antibody (Dianova, #109-035-003) was added for an additional hour. Ultra TMB substrate solution was added, and reaction terminated after one minute with H2SO4. Absorbance at 450 nm was measured and corrected with controls (mean of wells without serum and reference absorbance at 630 nm).
Western blot
SDS-Page and Western blotting were performed as described using cortices from Syn1/2/3 triple knockout (TKO) mice and wild type (WT) mice (14). Sera of CBA with scores of 1-2 were diluted to 1:200 and used to incubate membranes. A rabbit polyclonal SYN1/2 antibody (Synaptic Systems, #106002) was used as positive control and a mouse monoclonal antibody against glyceraldehyde-3-phosphate dehydrogenase (GAPDH, Merck Millipore, #MAB374) served as loading control.
Clinical data and statistical analysis
We studied a cohort of 208 mothers of pediatric patients with neurological disorders treated at the Center for Chronically Sick Children, Charité – Universitätsmedizin Berlin, Germany. Healthy mothers of children with unclear developmental disabilities were approached regarding the study by a study team physician at their child’s regular treatment appointment. Due to the exploratory approach, no exclusion criteria were specified. Maternal sera were prospectively collected and analyzed. The finding of SYN1 autoantibodies did not influence the treatment of the patients. Patient data were collected retrospectively and blinded to the maternal antibody levels using a standardized data collection sheet. Statistical analysis was performed using R (RStudio version 4.2.1) and the packages crosstable, ggplot2, UpSetR, and psych. Descriptive statistics were performed to calculate percentages and frequencies. Differences in categorical variables are reported as odds ratio and 95% confidence interval. P values were calculated using the Chi-square test and Fisher’s Exact Probability Test, as applicable. Test results with p<0.05 were considered statistically significant. The study was approved by the local ethics committee (approval no. #EA2/220/20).
Results
We screened sera for SYN1 autoantibodies in 208 mothers to assess the relationship with neurodevelopment in children. Due to the explorative approach, no exclusion criteria were set. Mothers gave birth at an average age of 30.93 ± 5.81 years. Children (male n=134, 64.1%) were 6.6 ± 4.14 years old at testing. Developmental delay in the domains speech (n=158, 75.7%) and motor skills (n=153, 73.1%) were the most common findings. Furthermore, 135 patients had intellectual disability (64.4%), and 83 patients had epilepsy (39.9%) (Figure 1). Epilepsy (p=0.049), ASD (p=0.001) and behavioral problems (p=0.008) were more common in male, while microcephaly was more common in female patients (p=0.001).
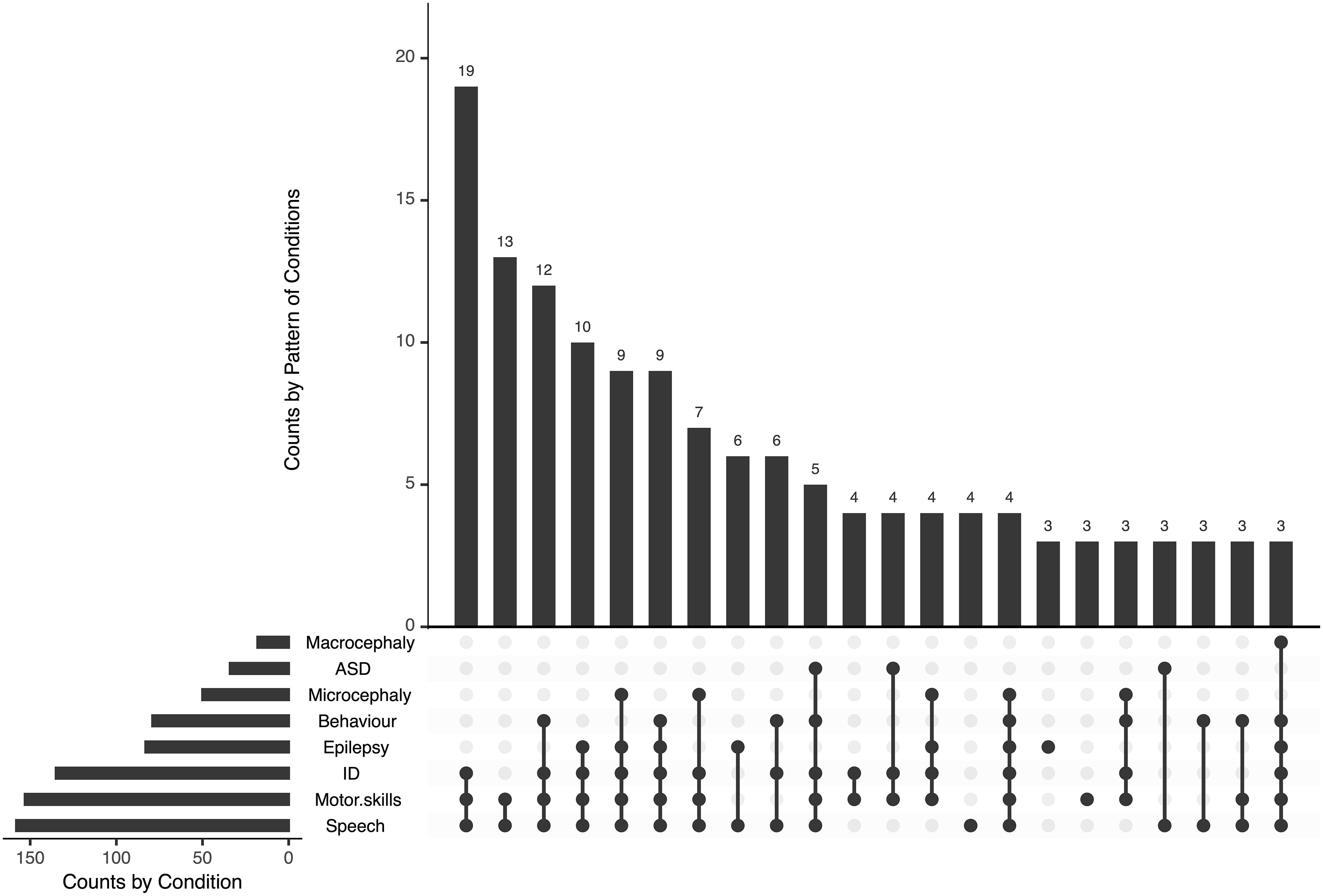
Figure 1 Phenotype of children of mothers tested for SYN1 autoantibodies. Developmental delay in the domains speech and motor skills were the most common findings, often associated with intellectual disability and epilepsy. ID, intellectual disability; ASD, autism-spectrum disorder.
In a first step, the patients’ mothers were screened using the previously described CBA (14). We used a visual scoring system for antibody binding in the CBA, and scores of 2 (intensive binding) were considered as positive (Figure 2A). Positive binding was identified in 20 mothers (9.6%). In 30 mothers, unspecific binding was detected while all others (n=158) showed no binding (Figure 2B). We further analyzed the sera of mothers with a SYN1 ELISA. Here, we found only a weak correlation between the CBA and ELISA results (r=0.29, Figure 2C). Possibly, these differing results can be explained by the binding of SYN1 autoantibodies to conformational epitopes that are not detected by ELISA. Therefore, we performed Western blots using the sera and cortex homogenates of wild-type and SynI/II/III triple knockout mice. We detected IgG binding to wild-type mouse brain homogenates in four of the 20 CBA-positive sera (Figure 2D). The other sera did not show immunoreactive bands at the expected molecular weight. These results point towards SYN1 IgG autoantibodies binding to linear SYN1 epitopes in only a small group, whereas autoantibodies against conformational synapsin epitopes predominate.
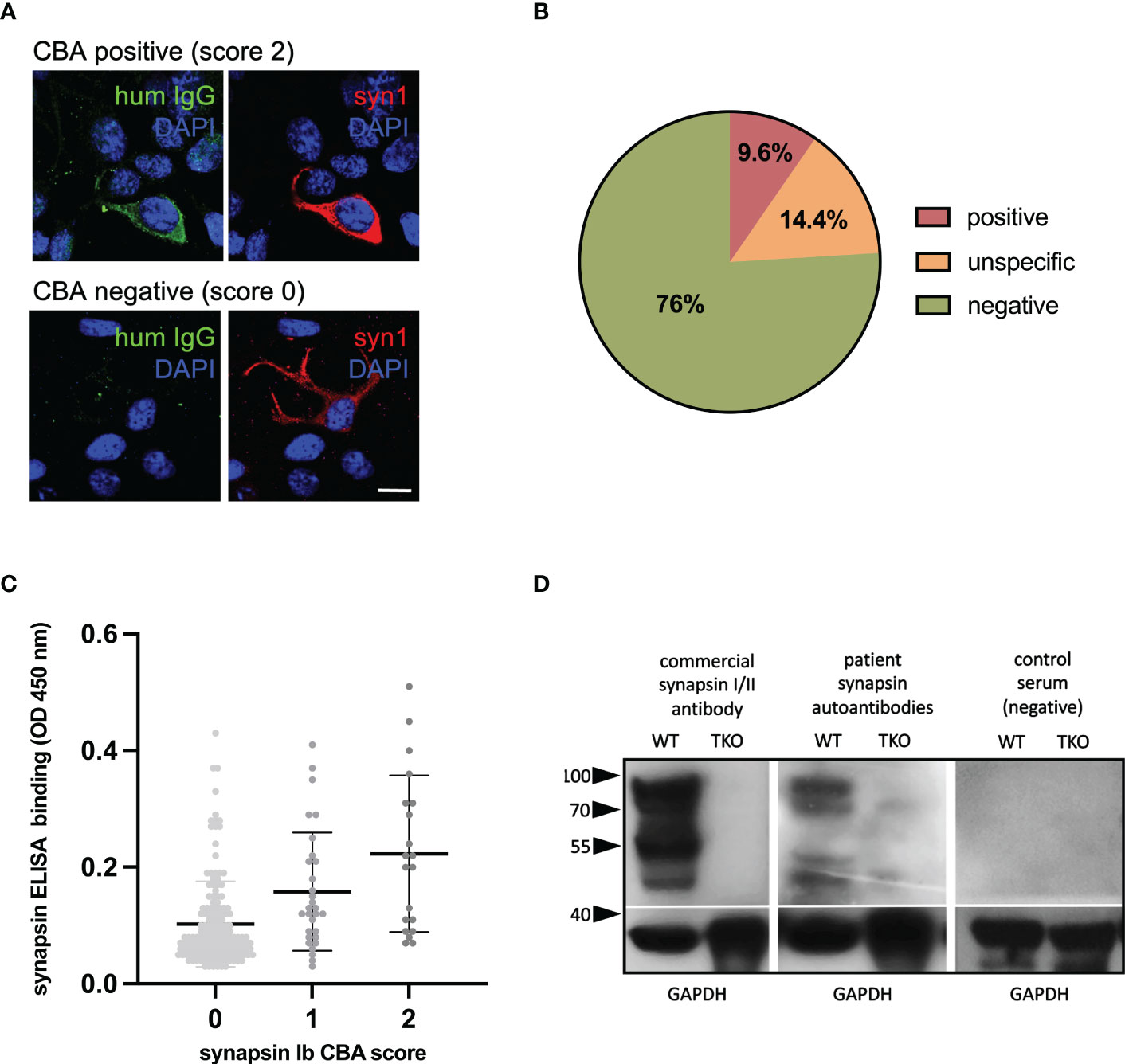
Figure 2 Detection of synapsin 1 (SYN1) autoantibodies. (A) Examples of immunofluorescence stainings, using human sera at a 1:300 dilution (green) with or without binding to HEK cells overexpressing human SYN1b are shown in the top row and bottom row. Using a commercial SYN1/2 antibody, protein expression is verified (red). DAPI is used to stain the nuclei (blue). Scale bar: 20 µm. (B) In 9.6% of mothers SYN1 autoantibodies were detected using a CBA. (C) CBA and in-house ELISA correlated weakly, with higher mean serum IgG levels in the CBA-positive group. (D) Representative immunoblots of wild type (WT) and Syn1/2/3 triple KO (TKO) mice cortex homogenates with a commercial synapsin 1/2 antibody as positive control, and with sera (1:200 dilution) from a CBA-positive mother and a CBA-negative control. Major bands at 80-90 kDa corresponding to the molecular weight of synapsin 1a/1b and additional bands at 50-55 kDa that could represent the synapsin 2b isoform or breakdown products of synapsin 1 were detected in wild type but not in TKO mouse tissue by both the commercial antibody and the serum of the CBA-positive mother. Detection of GAPDH served as loading control. HEK human embryonic kidney; CBA, cell-based assay; ELISA, enzyme-linked immunosorbent assay, WB, western blot.
We further analyzed children of mothers with a positive CBA (Table 1). Most commonly the children had ID (n=18, 90%), speech delay (n=17, 85%), motor delay (n=17, 85%), and epilepsy (n=12, 60%). Seven patients had a developmental and epileptic encephalopathy (DEE). Cranial MRI was performed in 15 patients, with abnormalities detected in eight patients (53.3%, Table 1). Genetic testing was carried out in 13 patients, with variants (n=7) and microdeletions (n=1) identified in eight patients (61.5%). Of these seven variants, one was classified as pathogenic (14.3%), three as probably pathogenic (42.9%), and three as variants of unclear significance (42.9%). Identified variants (gene, cDNA, ACMG classification and NM number) are listed in Table 1 for each patient. The four patients with IgG binding to wild-type mouse brain, showed no striking phenotypic differences to patients without binding (Table 1).
We next analyzed the clinical data based on the results of the CBA. While there was no association between CBA and age of mothers, age at birth or children’s age, we found an increased risk for specific phenotypes in patients of mothers with positive CBA. A positive CBA was a significant risk factor in their children for the presence of ID (OR: 5.46 [95% CI: 1.52 - 35.01], p = .0134) and behavioral problems (2.71 [1.07 - 7.23], p = .0328). Furthermore, patients showed a tendency for the presence of macrocephaly (3.11 [0.81 - 9.92], p = .0785), epilepsy (2.47 [0.98 - 6.59], p = .0536), ASD (1.32 [0.36 - 3.89], p = .7493), microcephaly (1.40 [0.47 - 3.72], p = .5882), and a developmental delay in the domains speech (1.89 [0.60 - 8.34], p = .4167) and motor skills (2.17 [0.69 - 9.55], p = .2223) (Figures 3A, B).
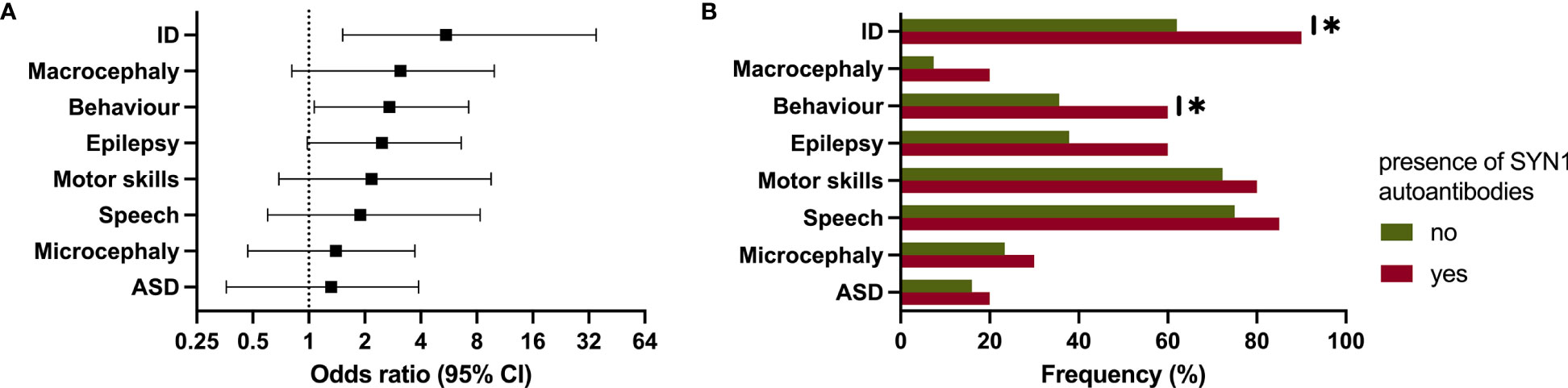
Figure 3 Clinical phenotype in patients of mothers with autoantibodies against synapsin 1 (SYN1). (A, B) Forest plot and bar chart showing the significantly increased risk for intellectual disability and behavioral problems. ID, intellectual disability; ASD, autism-spectrum disorder. * p ≤ 0.05.
Discussion
In this study, we recruited a clinical cohort of 208 mothers and their children and analyzed the prevalence of maternal SYN1 autoantibodies and their association with developmental phenotypes. SYN1 IgG autoantibodies were detected through CBA in 9.6% of mothers with neurologically sick children. Most of the SYN1 CBA positive cases were negative on Western blots under denaturing conditions, suggesting a conformational dependency of their target binding, as similarly observed in other human antineuronal autoantibodies (18). In a study by Höltje et al. that analyzed SYN1 autoantibodies in a cohort of patients with various psychiatric and neurological disorders, binding was detected in immunoblots in about half of all patients (14). It is unclear whether in less denaturing conditions more SYN1 CBA positive cases would be positive. As in this study, we recently reported IgG binding in immunoblots of 263 pregnant women screened for synapsin I autoantibodies in only 22.9% and found no correlation between ELISA and CBA antibody levels (16).
SYN1 is member of the family of synapsins, encoded by the three genes SYN1, SYN2, and SYN3 in humans. SYN1 regulates the trafficking of synaptic vessels between the readily releasable pool and reserve pool. Furthermore, Syn1 moderates neuronal development through neurite outgrowth and synaptogenesis (2). Syn1 mutant mice have severe epilepsy with generalized seizures (19).
In this present study of 208 children with various neurologic disorders, a positive maternal CBA showed a significant association with ID and behavioral problems, while there was and a trend towards other abnormalities such as macrocephaly and epilepsy. This is intriguing given that patients with LoF variants in SYN1 display a similar phenotype with epilepsy, ID, ASD, macrocephaly, and behavioral abnormalities (3–5). The lack of a clear association to these phenotypes may relate to the low statistical power of the study and emphasizes to investigate larger cohorts.
When further analyzing the 20 CBA-positive patients, seven had identified genetic variants/microdeletions of which one was considered pathogenic and three as likely pathogenic variants. This raises speculation as to whether genetic alterations in brain proteins may lead to neoantigen formation and increased autoimmunity. Autoantibodies arise from multilayered defects at immune checkpoints due to dysregulated negative selection of B cells which eventually develop into plasma cells or B memory cells (18). These autoantibodies can act through target-specific mechanisms. For example, in vitro experiments could show that SYN1 autoantibodies can be internalized through FcγII/III-mediated endocytosis and promote SYN1 aggregation resulting in impairment of synaptic transmission (20). Future experimental models will have to elucidate the functional role of maternal SYN1 autoantibodies and investigate whether they can reach the fetal brain and have a direct pathogenic effect on fetal development in vivo or rather represent a clinical biomarker of neuronal cell damage. Synaptic impairment by maternal SYN1 autoantibodies with possible detrimental effects on neurodevelopment may have a similar effect as other maternal antineuronal autoantibodies such as NMDAR, Caspr2, and aquaporin-4 antibodies, for which experimental studies have demonstrated pathogenic effects (10–13). Further, Caspr2 autoantibodies present during pregnancy were associated with ID and developmental disorders (21). However, functional studies on the effects of SYN1 autoantibodies on neurodevelopment are lacking and should be performed using patient-derived monoclonal antibodies (22).
In addition, it is unclear to what extent SYN1 autoantibodies cross the placenta and can be found in CSF. For this, functional experiments are necessary, in which human monoclonal autoantibodies are systemically applied into pregnant mice. It would be further interesting to assess if children of mothers with a positive CBA have SYN1 autoantibodies also in their CSF.
The study has multiple limitations including heterogeneity of the pediatric cohort. Other environmental factors, besides identified genetic variants, can also have an influence on the phenotype of these children. Furthermore, mothers were tested after pregnancy, Thus, it is unclear whether they were also positive during pregnancy which does not allow a direct statement about causality. To assess whether SYN1 autoantibodies have an effect during pregnancy we recently reported on a cohort of 263 pregnant women with seropositivity for SYN1 autoantibodies in 13.3%, matching the prevalence in this cohort (16). Seropositivity was associated with abnormalities of fetal development including intrauterine growth retardation, structural abnormalities and amniotic fluid disorders. The next step is to accurately phenotype these patients to assess the impact of SYN1 during pregnancy on the neurodevelopment of these children. Furthermore, the results of this study need to be validated by larger prospective multicentric studies with age-matched controls of healthy patients to increase power and counteract selection bias.
Despite these limitations, the study indicates a potential influence of maternal SYN1 autoantibodies on neurodevelopment. These results do not currently trigger any direct therapeutic or diagnostic consequences, but could influence standard treatment of pregnant women in the long term and possibly lead to new therapeutic approaches.
Data availability statement
The original contributions presented in the study are included in the article/supplementary materials. Further inquiries can be directed to the corresponding author.
Ethics statement
The studies involving human participants were reviewed and approved by Charité - Universitätsmedizin Berlin (approval no. #EA2/220/20). Written informed consent to participate in this study was provided by the participants’ legal guardian/next of kin. The animal study was reviewed and approved by Charité - Universitätsmedizin Berlin (approval no. #EA2/220/20).
Author contributions
Conceptualization: AK and HP. Patient recruitment: IB, KM, TU and ES. CBA and ELISA testing: IB, JK, CH, HR, MH, DM and JT. Western blotting: MH. Data collection, analysis, and visualization: IB and KM. Resources: AK and HP. Writing – original draft: IB, KM. Writing – review & editing: all authors. All authors contributed to the article and approved the submitted version.
Funding
This work was supported by grants from the German Research Foundation (DFG) (grants FOR3004, PR1274/4-1, PR1274/5-1, PR1274/9-1), by the Helmholtz Association (HIL-A03), the German Federal Ministry of Education and Research (Connect-Generate 01GM1908D), the Einstein Stiftung Fellowship through the Günter Endres Fond, and the Sonnenfeld-Stiftung.
Acknowledgments
We thank Stefanie Bandura, Matthias Sillmann, Doreen Brandl, Antje Dräger, and Monika Majer for excellent technical assistance and study nurse support. JK and MN are participants in the Berlin Institute of Health (BIH)-Charité Junior Clinician Scientist Program.
Conflict of interest
The authors declare that the research was conducted in the absence of any commercial or financial relationships that could be construed as a potential conflict of interest.
Publisher’s note
All claims expressed in this article are solely those of the authors and do not necessarily represent those of their affiliated organizations, or those of the publisher, the editors and the reviewers. Any product that may be evaluated in this article, or claim that may be made by its manufacturer, is not guaranteed or endorsed by the publisher.
References
1. Hilfiker S, Benfenati F, Doussau F, Nairn AC, Czernik AJ, Augustine GJ, et al. Structural domains involved in the regulation of transmitter release by synapsins. J Neurosci (2005) 25(10):2658–69. doi: 10.1523/jneurosci.4278-04.2005
2. Valtorta F, Benfenati F, Greengard P. Structure and function of the synapsins. J Biol Chem (1992) 267(11):7195–8. doi: 10.1016/S0021-9258(18)42501-X
3. Garcia CC, Blair HJ, Seager M, Coulthard A, Tennant S, Buddles M, et al. Identification of a mutation in synapsin I, a synaptic vesicle protein, in a family with epilepsy. J Med Genet (2004) 41(3):183–6. doi: 10.1136/jmg.2003.013680
4. Fassio A, Patry L, Congia S, Onofri F, Piton A, Gauthier J, et al. Syn1 loss-of-Function mutations in autism and partial epilepsy cause impaired synaptic function. Hum Mol Genet (2011) 20(12):2297–307. doi: 10.1093/hmg/ddr122
5. Giannandrea M, Guarnieri FC, Gehring NH, Monzani E, Benfenati F, Kulozik AE, et al. Nonsense-mediated mrna decay and loss-of-Function of the protein underlie the X-linked epilepsy associated with the W356× mutation in synapsin I. PloS One (2013) 8(6):e67724. doi: 10.1371/journal.pone.0067724
6. Kreye J, Wright SK, van Casteren A, Stöffler L, Machule ML, Reincke SM, et al. Encephalitis patient-derived monoclonal gabaa receptor antibodies cause epileptic seizures. J Exp Med (2021) 218(11). doi: 10.1084/jem.20210012
7. Noviello CM, Kreye J, Teng J, Prüss H, Hibbs RE. Structural mechanisms of gaba(a) receptor autoimmune encephalitis. Cell (2022) 185(14):2469–77.e13. doi: 10.1016/j.cell.2022.06.025
8. Maljevic S, Møller RS, Reid CA, Pérez-Palma E, Lal D, May P, et al. Spectrum of gabaa receptor variants in epilepsy. Curr Opin Neurol (2019) 32(2):183–90. doi: 10.1097/wco.0000000000000657
9. Han VX, Patel S, Jones HF, Dale RC. Maternal immune activation and neuroinflammation in human neurodevelopmental disorders. Nat Rev Neurol (2021) 17(9):564–79. doi: 10.1038/s41582-021-00530-8
10. García-Serra A, Radosevic M, Pupak A, Brito V, Ríos J, Aguilar E, et al. Placental transfer of nmdar antibodies causes reversible alterations in mice. Neurol Neuroimmunol Neuroinflamm (2021) 8(1). doi: 10.1212/nxi.0000000000000915
11. Mader S, Brimberg L, Vo A, Strohl JJ, Crawford JM, Bonnin A, et al. In utero exposure to maternal anti-Aquaporin-4 antibodies alters brain vasculature and neural dynamics in Male mouse offspring. Sci Transl Med (2022) 14(641):eabe9726. doi: 10.1126/scitranslmed.abe9726
12. Brimberg L, Mader S, Jeganathan V, Berlin R, Coleman TR, Gregersen PK, et al. Caspr2-reactive antibody cloned from a mother of an asd child mediates an asd-like phenotype in mice. Mol Psychiatry (2016) 21(12):1663–71. doi: 10.1038/mp.2016.165
13. Jurek B, Chayka M, Kreye J, Lang K, Kraus L, Fidzinski P, et al. Human gestational n-Methyl-D-Aspartate receptor autoantibodies impair neonatal murine brain function. Ann Neurol (2019) 86(5):656–70. doi: 10.1002/ana.25552
14. Höltje M, Mertens R, Schou MB, Saether SG, Kochova E, Jarius S, et al. Synapsin-antibodies in psychiatric and neurological disorders: Prevalence and clinical findings. Brain Behav Immun (2017) 66:125–34. doi: 10.1016/j.bbi.2017.07.011
15. Piepgras J, Höltje M, Otto C, Harms H, Satapathy A, Cesca F, et al. Intrathecal immunoglobulin a and G antibodies to synapsin in a patient with limbic encephalitis. Neurol Neuroimmunol Neuroinflamm (2015) 2(6):e169. doi: 10.1212/nxi.0000000000000169
16. Bünger I, Kreye J, Makridis K, Höltje M, Rasmussen HF, van Hoof S, et al. Synapsin autoantibodies during pregnancy are associated with fetal abnormalities. medRxiv (2022), 2022.09.23.22280284. doi: 10.1101/2022.09.23.22280284
17. Milovanovic D, Wu Y, Bian X, De Camilli P. A liquid phase of synapsin and lipid vesicles. Science (2018) 361(6402):604–7. doi: 10.1126/science.aat5671
18. Prüss H. Autoantibodies in neurological disease. Nat Rev Immunol (2021) 21(12):798–813. doi: 10.1038/s41577-021-00543-w
19. Rosahl TW, Spillane D, Missler M, Herz J, Selig DK, Wolff JR, et al. Essential functions of synapsins I and ii in synaptic vesicle regulation. Nature (1995) 375(6531):488–93. doi: 10.1038/375488a0
20. Rocchi A, Sacchetti S, De Fusco A, Giovedi S, Parisi B, Cesca F, et al. Autoantibodies to synapsin I sequestrate synapsin I and alter synaptic function. Cell Death Dis (2019) 10(11):1–16. doi: 10.1038/s41419-019-2106-z
21. Coutinho E, Jacobson L, Pedersen MG, Benros ME, Nørgaard-Pedersen B, Mortensen PB, et al. Caspr2 autoantibodies are raised during pregnancy in mothers of children with mental retardation and disorders of psychological development but not autism. J Neurol Neurosurg Psychiatry (2017) 88(9):718–21. doi: 10.1136/jnnp-2016-315251
Keywords: synapsin 1, antineuronal autoantibodies, transplacental transfer, maternofetal autoimmunity, developmental delay, epilepsy, behavioral problems
Citation: Bünger I, Makridis KL, Kreye J, Nikolaus M, Sedlin E, Ullrich T, Hoffmann C, Tromm JV, Rasmussen HF, Milovanovic D, Höltje M, Prüss H and Kaindl AM (2023) Maternal synapsin autoantibodies are associated with neurodevelopmental delay. Front. Immunol. 14:1101087. doi: 10.3389/fimmu.2023.1101087
Received: 17 November 2022; Accepted: 04 January 2023;
Published: 19 January 2023.
Edited by:
Honghao Wang, Guangzhou First People’s Hospital, ChinaReviewed by:
Jinming Han, Capital Medical University, ChinaZsolt Illes, University of Southern Denmark, Denmark
Copyright © 2023 Bünger, Makridis, Kreye, Nikolaus, Sedlin, Ullrich, Hoffmann, Tromm, Rasmussen, Milovanovic, Höltje, Prüss and Kaindl. This is an open-access article distributed under the terms of the Creative Commons Attribution License (CC BY). The use, distribution or reproduction in other forums is permitted, provided the original author(s) and the copyright owner(s) are credited and that the original publication in this journal is cited, in accordance with accepted academic practice. No use, distribution or reproduction is permitted which does not comply with these terms.
*Correspondence: Angela M. Kaindl, YW5nZWxhLmthaW5kbEBjaGFyaXRlLmRl
†These authors have contributed equally to this work and share first authorship
‡These authors have contributed equally to this work and share last authorship